- 1College of Animal Science and Technology, Inner Mongolia Minzu University, Tongliao, China
- 2College of Animal Science and Technology, China Agricultural University, Beijing, China
- 3Tongliao Agriculture and Animal Husbandry Development Centre, Tongliao, China
Methane is an important component of greenhouse gases, and ruminant production is a significant source of methane emissions. At present, flavonoid feed additives have certain applications in methane inhibition in ruminants. However, the effects of different doses of quercetin on rumen fermentation parameters, rumen bacteria and archaea are still unclear. Therefore, this study investigated the effects of quercetin on in vitro rumen fermentation parameters, methane production, and microflora in beef cattle. A completely randomized design was adopted. Quercetin was added to the fermentation substrates at 0% (group C), 0.5% (group Q1), 1% (group Q2) and 1.5% (group Q3). Anaerobic fermentation was carried out at 39°C for 48 h, gas production (GP) was recorded at different times, gas composition was determined, and methane (CH4) production was calculated. Fermentation parameters and dry matter digestibility (DMD) were determined after 48 h. Moreover, rumen fluid was collected for rumen bacterial and archaeal flora determination. The results were as follows: (1) After 32 h of fermentation, the GP decreased in response to the addition of quercetin. With increasing quercetin concentration, the theoretical maximum gas production decreased quadratically before 20 h (P quadratic = 0.032). There was a quadratic increase in gas production (P quadratic = 0.024). With increasing quercetin supplementation, the NH3-N content increased quadratically (P quadratic = 0.027). MCP increased linearly and quadratically with quercetin (P linear = 0.002, P quadratic = 0.005), whereas DMD decreased linearly and quadratically with quercetin (P linear = 0.013, P quadratic = 0.032). Both 0.5 and 1% quercetin significantly reduced the butyrate content (P quadratic = 0.002). With the addition of quercetin, the levels of butyrate, isobutyrate, isovalerate, and total volatile fatty acid (TVFA) first decreased but then increased (P quadratic < 0.05). (2) With increasing quercetin concentration, methane production (P quadratic = 0.009) and the methane proportion (P quadratic < 0.001) decreased quadratically. (3) The ACE index and Chao1 index increased quadratically with quercetin supplementation (P quadratic < 0.05). The relative abundance of Succiniclasticum in groups Q1 and Q3 increased, whereas the relative abundances of norank_f__norank_o__Rickettsiales and Curtobacterium decreased in all quercetin groups at the genus level (P < 0.05). (4) Quercetin supplementation did not affect the diversity of the archaeal community, but the relative abundance of Methanobrevibacter in group Q2 decreased. Overall, quercetin influenced in vitro rumen fermentation and the bacterial flora to decrease methane production and promote rumen nitrogen utilization and MCP synthesis.
1 Introduction
With the increasing impact of global climate change on ecosystems and human society, reducing greenhouse gas emissions, especially carbon dioxide and methane emissions, has become the focus of the international community. In animal husbandry, ruminants are considered one of the major sources of greenhouse gas emissions, with rumen methane production accounting for 80% of the total gastrointestinal methane production (Liebig et al., 2010). Methane emissions from ruminants are estimated to account for approximately 16% of global greenhouse gas emissions, with cattle and sheep accounting for approximately 70% and 20%, respectively (Lan and Yang, 2019). Methane in the rumen is produced mainly by the reduction of carbon dioxide by hydrogen, which is then excreted by ruminant hiccups (Soroye et al., 2020). In addition, the large amount of methane produced by rumen fermentation causes 2%−15% of feed energy to be converted to methane and lost (Ellis et al., 2007). Therefore, the methane produced by rumen fermentation in ruminants not only aggravates the greenhouse effect but also leads to the loss of feed energy. Therefore, inhibiting rumen methane production not only helps improve feed energy utilization efficiency but also effectively reduces the greenhouse effect.
Currently, various measures have been taken to reduce CH4 emissions from ruminants. These interventions include improving farm systems, optimizing forage, implementing dietary interventions, and adding methanobacteria inhibitors and feed additives (Belanche et al., 2020). The addition of certain plant extracts to diets has been proven to be simple and effective. Studies have shown that flavonoids have a direct effect on methanogens (Patra and Saxena, 2010; Bodas et al., 2012; Purba et al., 2019; Sinz et al., 2018). Kim et al. (2015) also reported that flavonoid-rich plant extracts can reduce methane emissions. In addition, Oskoueian et al. (2013) reported that flavonoids can inhibit the formation of methane. These findings have potential application value as rumen regulators for CH4 reduction in ruminants.
Quercetin is a flavonoid that is widely present in nature (Nam et al., 2016), is found in a variety of plants and forages, and has good anti-inflammatory (Carullo et al., 2017), antibacterial (Kim et al., 2022), antioxidant (Lespade, 2016), and anticancer effects (Geng et al., 2019). Flavonoids have been shown to improve the production performance of ruminants and maintain rumen health by reducing the bald area of the rumen wall and maintaining the pH, which could reduce the incidence of rumen acidosis (Bodas et al., 2012; Balcells et al., 2012). In addition, flavonoids, quercetin, and quercetin-containing plants reduce CH4 production in the rumen (Purba et al., 2019; Sinz et al., 2018). However, the effects of different doses of quercetin on rumen fermentation parameters, rumen bacteria and archaea are still unclear. Therefore, the purpose of this study was to evaluate the effects of quercetin on rumen fermentation, CH4 production and microflora in beef cattle via an in vitro method to provide a reference for the application of quercetin in ruminant rumen regulation and animal production.
2 Materials and methods
2.1 Materials
The quercetin used in this experiment was provided by Xi'an Musen Bioengineering Co., Ltd., Shanxi Province, China, with a purity of ≥98%, and the rumen fluid used in this experiment was collected from three Simmental crossbred bulls (bodyweights of 550 kg ± 30 kg) provided by the experimental base of Tongliao Mufeng Jiuyuan Husbandry Co., Ltd.
2.2 Composition and nutrient levels of the fermentation substrate
The substrate used for fermentation was the same as that used in the diet of the rumen fluid donor cattle, which was prepared according to the “Nutritional Requirements for Beef Cattle” (NASEM., 2016). The feed composition and nutrient levels of the substrate are shown in Table 1.
2.3 Experimental design
In this study, a completely randomized design was adopted. According to the dry matter calculation, quercetin was added to the fermentation substrates at 0% (group C), 0.5% (group Q1), 1% (group Q2), and 1.5% (group Q3), with 3 replicates in each group. The in vitro gas production (GP), methane proportion, dry matter digestibility (DMD), and fermentation parameters, including pH value, ammonia nitrogen (NH3-N), and volatile fatty acids (VFAs), were determined. Furthermore, the bacterial and archaeal flora of the rumen fluid were also analyzed.
2.4 Determination methods
2.4.1 Collection and treatment of rumen fluid
Two h before the morning feeding, the rumen fluid of 3 Simmental cattle was drawn through the esophagus via a vacuum sampler. To avoid the influence of saliva, the first 200 mL of rumen fluid from each cattle was discarded, and approximately 200 mL of rumen fluid was drawn and evenly mixed before being filtered through four layers of gauze and quickly transferred into a dispenser to form the artificial rumen culture fluid (rumen fluid: buffer = 1:2) according to the methods of Menke and Steingass (1988). The buffer in the dispenser was preheated (39°C) and purged to maintain anaerobic conditions with CO2.
2.4.2 Determination of in vitro gas production and methane production
The substrate of approximately 0.2000 g dry matter (DM) was weighed into a 100 mL graduated glass syringe (model HFT000025, Häberle Company, Germany), with 0% (control), 0.5%, 1%, or 1.5% quercetin added, and the glass syringe was preheated to 39°C. Approximately 30 mL of artificial rumen culture mixture was added to a 100 mL graduated glass syringe preheated at 39°C. The syringes were kept in a constant-temperature water bath shaker at 120 r/min at 39°C for 48 h. GP in each glass syringe was recorded at 0, 1, 2, 4, 6, 8, 12, 16, 20, 24, 32, 40, and 48 h, and the gas was collected in a 200 mL aluminum foil bag (Shanghai Huibin Instrument Co., Ltd., Shanghai, China).
The theoretical maximum gas production and the gas production rate were estimated according to the model of Ørskov and McDonald (1979):
where Y, a, B, c, and t represent the gas production (mL), the gas production from the immediately soluble fraction (mL), the theoretical maximum gas production (mL), the gas production rate (mL/h), and the incubation time (h), respectively.
The proportions of hydrogen (H2), CH4 and CO2 in the gas sampling bag (%, v/v) were determined via a gas chromatograph (model TP-2060T, Beijing Analytical Instrument Co., Ltd.). The instrument conditions were as follows: TCD detector, column model TDX-01, 1 m × 3 mm × 2 mm, column temperature of 120°C, detector temperature of 150°C, injection port temperature of 150°C, argon carrier gas flow rate of 50 mL/min, standard gas concentration of 24.80% CH4, 65.10% CO2, 2.01% H2, 3.00% O2, and 5.00% N2, and an injection volume of 0.1 mL. Methane production was calculated from total gas production and the proportion of methane produced at 48 h of fermentation.
2.4.3 Determination of in vitro dry matter digestibility
The dry matter digestibility (DMD) was measured according to the two-stage method of Tilley and Terry (1963): approximately 0.3000 g of substrate was weighed and put into a special bag (Beijing-Beef and Cattle Information Technology Research Center, size 35 mm × 75 mm, pore size 38–40 μm), with 3 replicates in each group. Three bags were placed in a 100 mL culture tube, in which 35 mL of artificial rumen fluid (rumen fluid: buffer = 1:1) was added, and the tubes were incubated anaerobically at 39°C for 48 h. After that, the bags were removed and rinsed with distilled water to remove the rumen fluid, and 35 mL of pepsin solution was added to the culture tube to perform another 48 h of anaerobic culture at 39°C. The bag was subsequently removed, rinsed with distilled water to remove pepsin, and dried at 105°C to a constant weight to calculate the DMD according to the following equation:
where DMD is the in vitro dry matter digestibility (%); m is the DM weight of the sample (g); m1 is the DM weight of the empty bag (g); m2 is the DM weight of the bag and the sample residue (g); and c is the correction factor of the blank bag (the DM weight after incubation/the DM weight before incubation).
2.4.4 Determination of rumen fermentation parameters
The syringes were immediately placed in ice water to terminate fermentation after 48 h. Part of the fermentation residue was transferred into a 5 mL centrifuge tube to determine the microbial protein (MCP) content according to the method of Sedmak and Grossberg (1977). The other fermentation residue was transferred into a 50 mL centrifuge tube to determine the pH value immediately with a portable pH meter (Testo 205, Germany), followed by centrifugation (8,000 r/min, 4°C for 15 min). The supernatant was collected for the determination of NH3-N and VFAs according to the colorimetric methods of Verdouw et al. (1978) and Chen et al. (2022). The gas chromatographic parameters (model GC-6800, Beijing Beifen Tianpu Instrument Technology Co., Ltd.) were as follows: Φ6 mm × 2 m quartz glass-packed column, column temperature of 150°C, injection port temperature of 220°C, injection volume of 1 μL, and FID detector temperature of 280°C. The carrier gas was high-purity N2, the flow rate was 30 mL/min, and the pressure was 200 kPa. The gas used was H2, and the flow rate was 30 mL/min. The auxiliary gas used was air, and the flow rate was 300 mL/min.
2.4.5 Determination of bacteria in the rumen
Five milliliters of the fermentation residue in the glass syringe was transferred to a cryopreservation tube and stored at −80°C for further determination. The tube was placed on a sterile platform and thawed in a mixture of ice water. Total DNA extraction was performed according to the E.Z.N.A.® soil kit (Omega Biotek, Norcross, GA, U.S.). A NanoDrop 2000 was used to determine the DNA concentration and purity, and 1% agarose gel electrophoresis was used to determine the quality of the extracted DNA. PCR amplification was performed via bacterial V3-V4 region primers 338F (5′-ACTCCTACGGGAGGCAGCAG-3′) and 806R (5′-GGACTACHVGGGTWTCTAAT-3′), and the resulting PCR products were purified, washed, and detected after recovery. The Illumina MiSeq PE300 platform was used for sequencing (Shanghai MegiBiomedical Technology Co., Ltd.).
2.4.6 Determination of archaea in the rumen
The V4-V5 variable region of the 16S rRNA gene was amplified via PCR via 524F10ext (5′-TGYCAGCCGCCGCGGTAA-3′) and Arch958RmodR (5′-YCCGGCGTTGAVTCCAATT-3′) after DNA extraction. There were 3 PCR repeats per sample, and the products were detected after mixing 3 repeats of PCR products, recovered products, purified and detected, and quantified with a Quantus™ fluorometer (Promega, USA). The purified PCR products were constructed via the NEXTFLEX Rapid DNA-Seq Kit and sequenced via Illumina's MiSeq PE300/NovaSeq PE250 platform (Shanghai Magi Biomedical Technology) Limited Company.
2.5 Data statistics and analysis
The gas production at each time point and the rumen fermentation parameters at 48 h were collated with Microsoft Excel 2010 (Microsoft Corp., USA). Duncan's method in SPSS 22 (SPSS Inc., USA) was used for analysis of variance and multiple comparisons. Moreover, orthogonal polynomial contrasts were used to analyse the linear and quadratic effects of different doses of quercetin. The rumen microflora data were analyzed for differences via the Kruskal–Wallis test. The results were considered significant at P < 0.05, and the results are expressed as the means and means of standard errors (SEMs). All microbial data were analyzed via the biological cloud platform (https://cloud.majorbio.com). UPARSE software (http://drive5.com/uparse/, version 7.1) was used to cluster the sequences according to 97% similarity. Taxonomic annotation of OTU species was performed by comparing the 16S rRNA gene database (Silva v138) with the RDP classifier, and the community composition of each sample was calculated at different species classification levels. In addition, partial least square discriminant analysis (PLS-DA) was used. Mothur software was used to calculate and draw dilution curves and Venn diagrams according to the OTU information. LEfSe analysis (LDA > 3.5) was used to identify the bacterial groups with significant differences in abundance from phylum to genus. The Spearman correlations between the rumen microorganisms and the rumen fermentation parameters were subsequently analyzed.
3 Results
3.1 Effect of quercetin on in vitro gas production
As shown in Figure 1, quercetin had no effect on GP at the initial stage of in vitro fermentation, but after 32 h of fermentation, quercetin reduced GP. As shown in Table 2, with increasing quercetin supplementation level, the theoretical maximum gas production decreased quadratically before 20 h (P quadratic = 0.032). Gas production showed a secondary increase (P quadratic = 0.024), but the increase was small between the treatments. With increasing quercetin concentration, methane production (P quadratic = 0.009) and the methane proportion (P quadratic < 0.001) decreased quadratically. Compared with those in the control group, when 1.5% quercetin was added, the methane production and methane proportion were reduced by 15.82% and 8.78%, respectively.
3.2 Effect of quercetin on rumen fermentation parameters and dry matter digestibility
As shown in Table 3, there was no statistically significant difference in pH among all the groups (P > 0.05), and the values were within the normal range (6.68–6.72). However, NH3-N increased quadratically (P quadratic = 0.027) with increasing quercetin concentration. MCP increased linearly and quadratically (P linear = 0.002, P quadratic = 0.005), whereas DMD decreased linearly and quadratically with increasing quercetin concentration (Plinear = 0.013, P quadratic = 0.032). After 48 h of in vitro culture, the addition of 0.5% quercetin significantly reduced butyrate and isovalerate (P quadratic = 0.002 and 0.019, respectively), and 1% quercetin significantly reduced butyrate, isobutyrate, isovalerate, and total volatile fatty acid (TVFA) (P quadratic = 0.002, 0.043, 0.019 and 0.027, respectively), whereas 1.5% quercetin significantly increased propionate (P linear = 0.052) but had no significant effect on the other VFAs.
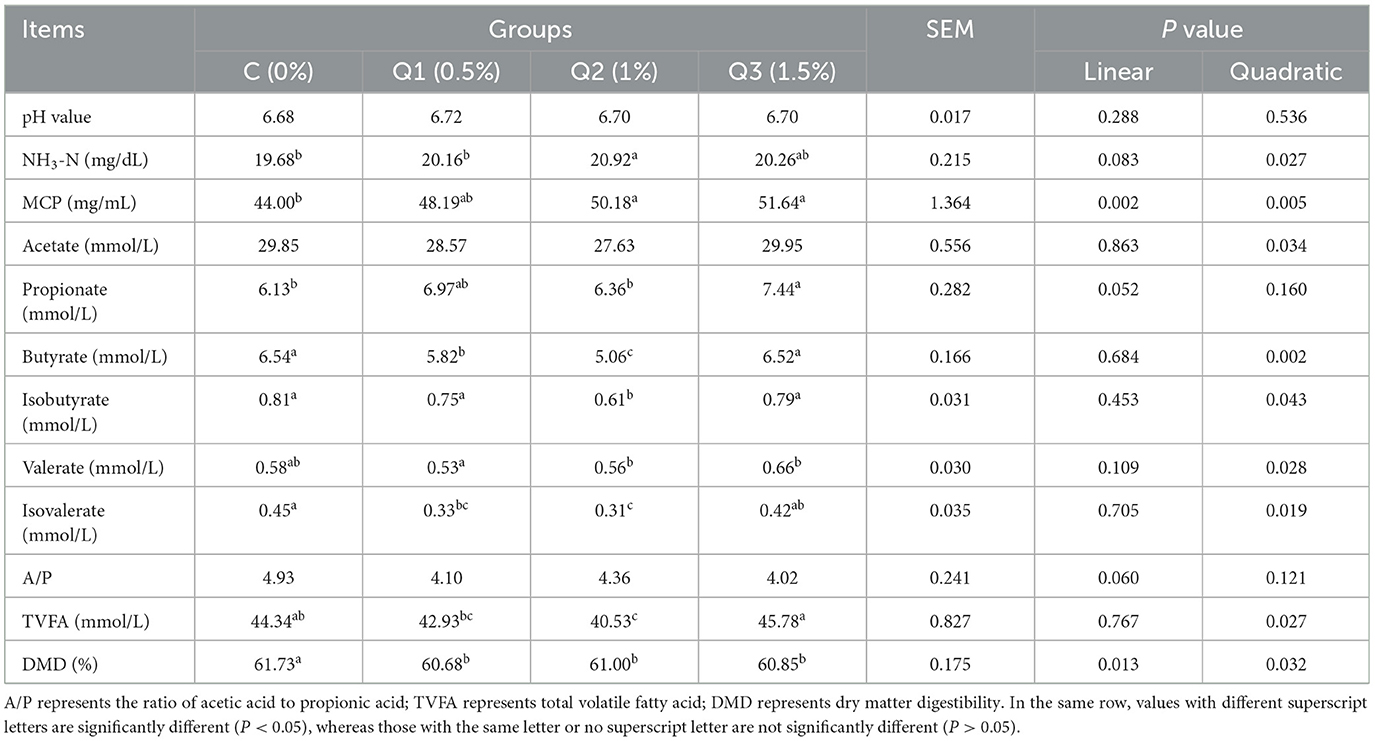
Table 3. Fermentation parameters and dry matter digestibility after 48 h of in vitro rumen fermentation.
3.3 Effect of quercetin on rumen bacteria
3.3.1 Changes in bacterial diversity in the rumen
UPARSE software was used to cluster each sample at the 97% similarity level, and a total of 1,827 OTUs were obtained (Figure 2A). As shown by the coverage curve (Figure 2B), the rumen microbial coverage of both the quercetin group and the control group exceeded 99%, indicating a high sequencing depth, which could indicate the rationality of the sequencing quantity and depth of the sequencing samples in this study. As shown in Table 4, the Ace index and Chao index increased quadratically with quercetin supplementation (P < 0.05), whereas the other indices were not affected by quercetin.
3.3.2 Effect of quercetin on bacterial composition in the rumen
At the taxonomic level, a total of 21 phyla, 41 classes, 96 orders, 162 families, 314 genera, 575 species, and 1,827 OTUs were obtained. At the phylum level, Firmicutes, Bacteroidetes, Proteobacteria, Actinobacteria, and Patescibacteria were the dominant phyla. The relative abundance was >1%, and the relative abundances of Bacteroideaceae and Firmicutes were the highest among all the groups, accounting for more than 88% of the total abundance (Figure 3A). At the phylum level, there was no significant difference among the groups (P > 0.05). At the genus level, there were 17 genera with relative abundances >1.0%, of which Rikenellaceae_RC9_gut_group, NK4A214, and unranked norank_f_Muribaculaceae were the dominant bacterial genera in each group (Figure 3B). Compared with those in group C, the relative abundances of Succiniclasticum in groups Q1 and Q3 were significantly greater (P < 0.05), whereas those of norank_f__norank_o__Rickettsiales and Curtobacterium were significantly lower in the quercetin groups (P < 0.05). The LEfSe results (Figure 3C) revealed significant differences in 2, 4, and 3 biomarkers in groups C, Q1 and Q3, respectively (LDA score > 3.5).
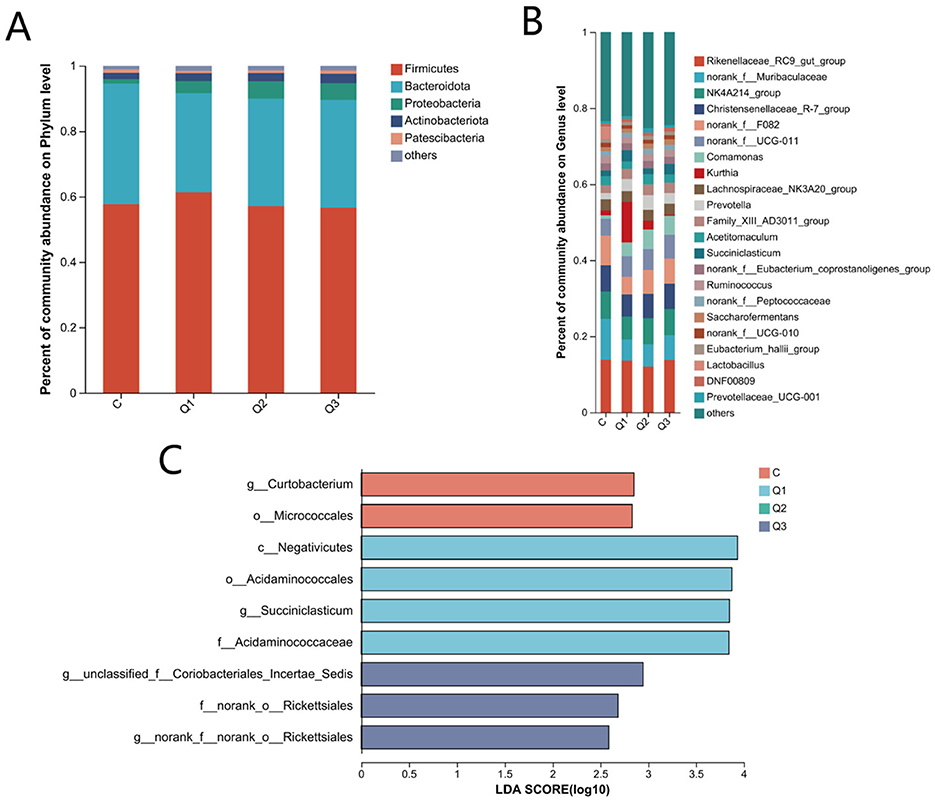
Figure 3. (A) Relative abundance of rumen bacteria at the phylum level; (B) relative abundance of rumen bacteria at the genus level; (C) significantly different bacterial taxa identified by linear discriminant analysis effect size (LEfSe).
3.3.3 Correlation analysis of the rumen bacterial composition, the rumen fermentation parameters and methane production
The correlation analysis results are shown in Figure 4. WPS-2 (P < 0.05) and Desulfobacterota (P < 0.01) were significantly and positively correlated with A/P. Proteobacteria and Armatimonadota were significantly negatively correlated with A/P (P < 0.05). WPS-2 was positively correlated with CH4 (P < 0.01). There was a significant positive correlation between propionate and Proteobacteria (P < 0.05), Armatimonadota (P < 0.01), and Synergistota (P < 0.05). Desulfobacterota was significantly negatively correlated with propionate (P < 0.05) and WPS-2 (P < 0.05). Unclassified_k_norank_d_Bacteria and Armatimonadota were significantly positively correlated (P < 0.05), whereas Fibrobacterota and valerate were significantly negatively correlated. Proteobacteria (P < 0.01) and Synergistota (P < 0.01) were significantly positively correlated with NH3-N, whereas WPS-2 was significantly negatively correlated with NH3-N. Spirochaetota (P < 0.05), Chloroflexi (P < 0.05), and Fibrobacterota (P < 0.05) were significantly positively correlated with MCP, whereas WPS-2 was significantly negatively correlated with MCP (P < 0.05).
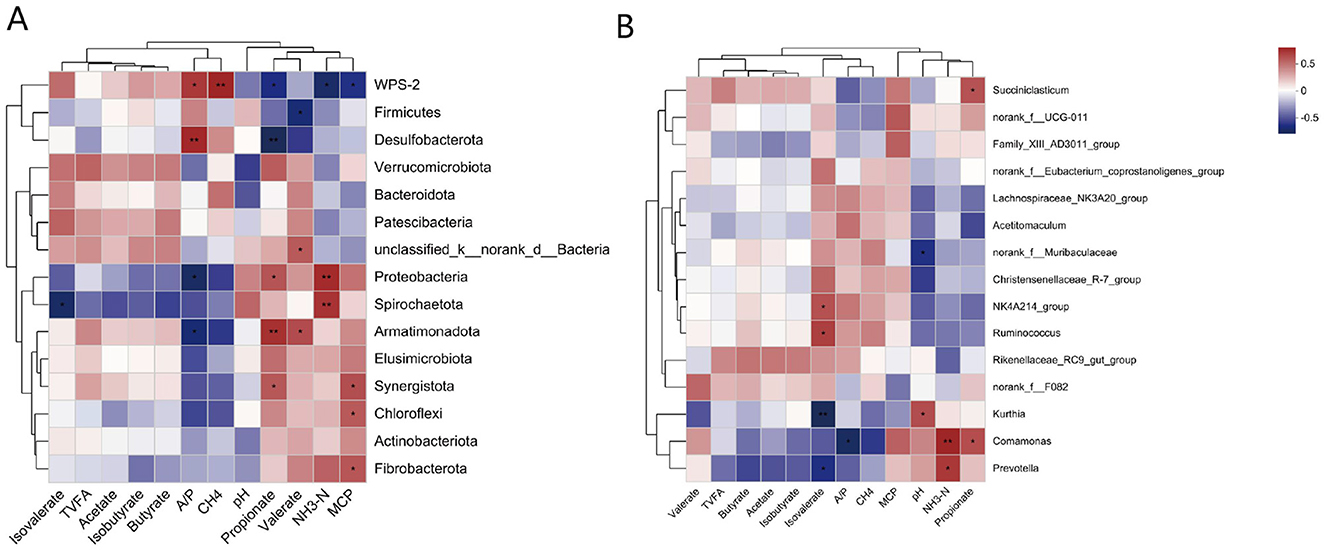
Figure 4. (A) Pearson correlation heatmap analysis of the bacterial phyla with rumen fermentation parameters and methane production. (B) Pearson correlation heatmap analysis of the bacterial genuses with rumen fermentation parameters and methane production. *, P < 0.05; **, P < 0.01; ***, P < 0.001. The legend in the upper right corner is the color interval of the different correlation R values.
Succiniclasticum was significantly positively correlated with propionate, whereas norank_f_Muribaculaceae was significantly negatively correlated with pH. NK4A214_group and Ruminococcus were significantly positively correlated with isobutyrate, whereas Kurthia was significantly negatively correlated with isobutyrate. Comamonas was positively correlated with propionate, negatively correlated with A/P and positively correlated with NH3-N. Prevotella was significantly negatively correlated with isobutyrate and positively correlated with NH3-N.
3.4 Effect of quercetin on rumen archaea
3.4.1 Changes in archaeal diversity in the rumen
Each sample was clustered and labeled at a 97% similarity level via UPARSE software (Figures 5A, B). As shown in Figures 5C, D, the contribution rate of the first principal component was 16.34%, and the contribution rate of the second principal component was 15.58% at the OTU level. At the genus level, the contribution rate of the first principal component was 23.24%, and the contribution rate of the second principal component was 19.02%, which could be clearly distinguished among groups Q1, Q2, and C, indicating that the compositions of the control group and the treatment groups in the rumen fluid samples were significantly different. Table 5 shows that quercetin had no significant effect on the richness or diversity of the rumen archaeal communities (P > 0.05).
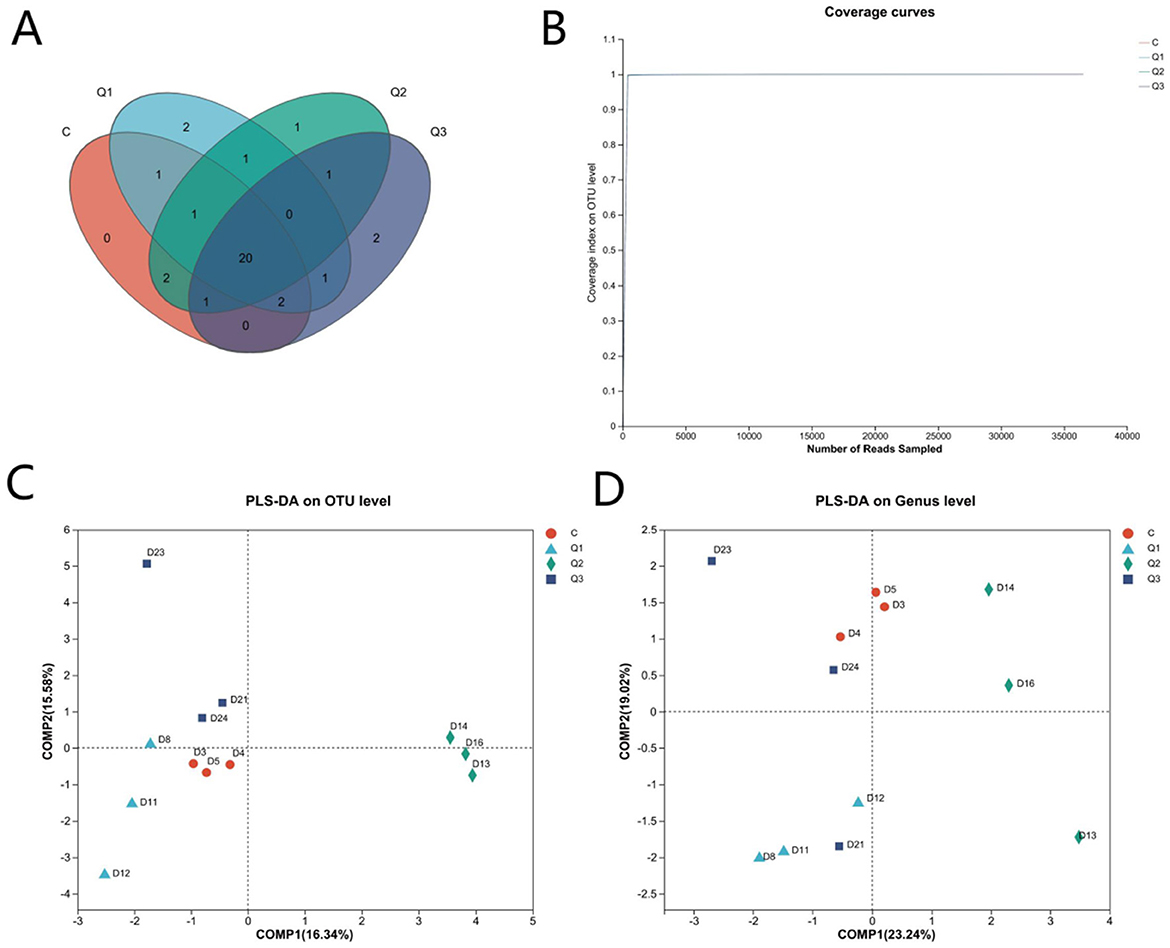
Figure 5. (A) Operational taxonomic unit-Venn (OTU-Venn) analysis; (B) dilution curve analysis; (C) PLS-DA analysis of quercetin on rumen archaea at the OUT level; (D) PLS-DA analysis of quercetin on rumen archaea at the genus level.
3.4.2 Effect of quercetin on the archaeal community in the rumen
The relative abundance distribution of the archaeal community at the phylum level is shown in Figure 6A. There was no significant difference between the groups (P < 0.05), and more than 99% of the archaea in each group were Euryarchaeota.
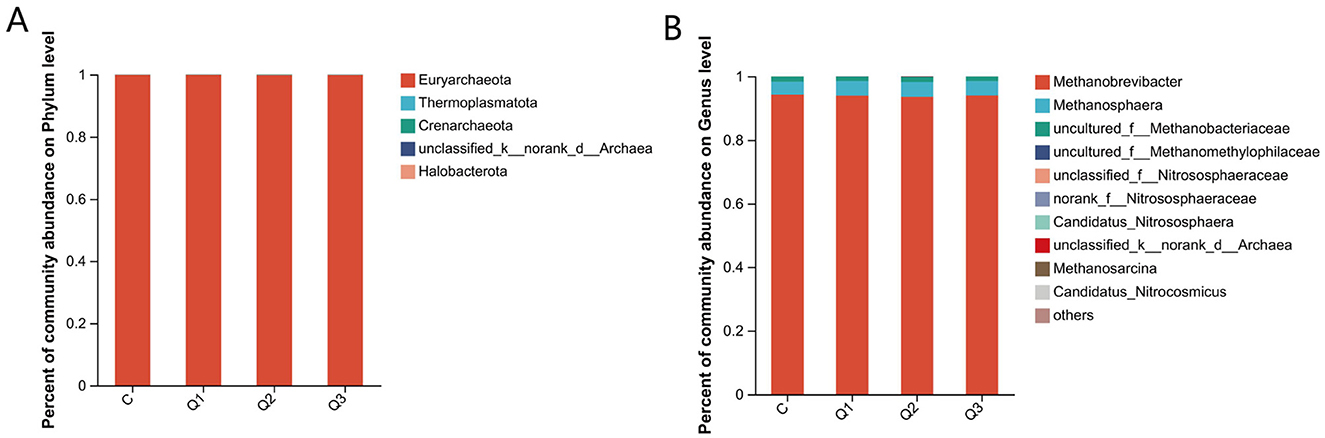
Figure 6. (A) Relative abundance of rumen archaea at the phylum level; (B) relative abundance of rumen archaea at the genus level.
The relative abundance at the genus level is shown in Figure 6B. The archaea with relative abundances higher than 1.0% included Methanobrevibacter, Methanosphaeria, and unclassified Methanobacteriaceae. All groups were dominated by Brevibacterium methanescens, but the content of each group differed. The content of Brevibacterium methanescens in group Q2 (93.56%) was significantly lower than that in group C (94.19%) and group Q1 (93.87%) (P < 0.05), and the content in group Q3 was in the middle (93.96%).
3.4.3 Correlation analysis of the rumen archaeal composition with fermentation parameters and methane production
The results of the correlation analysis between the rumen archaeal composition and fermentation parameters and methane production at the phylum level are shown in Figure 7A. Crenarchaeota was significantly negatively correlated with propionate and negatively correlated with TVFAs. Thermoplasmatota was negatively correlated with A/P.
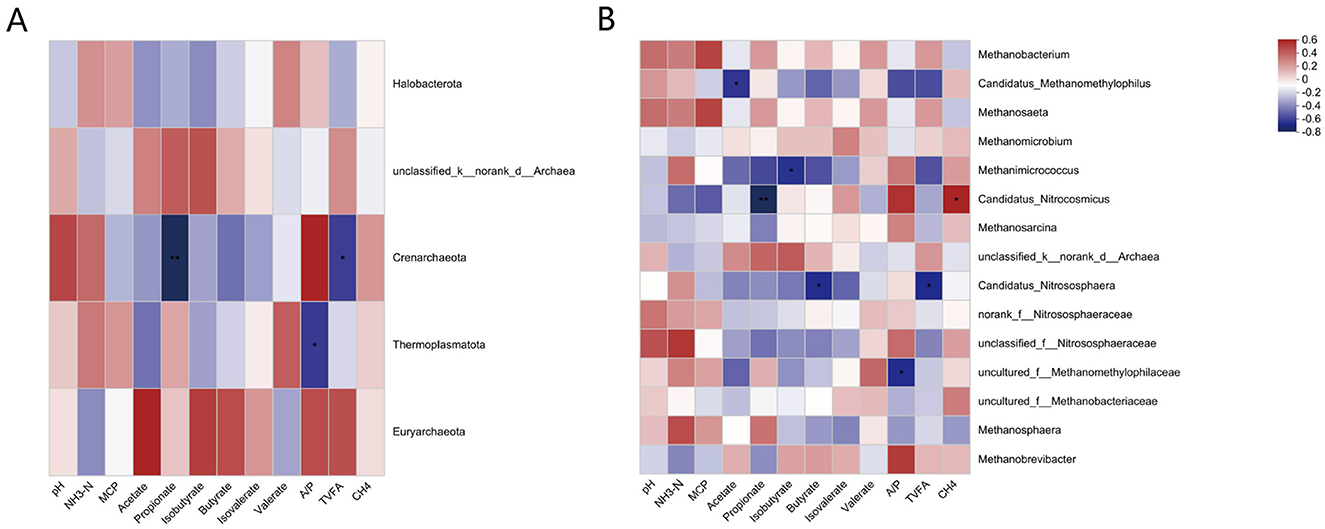
Figure 7. (A) Pearson correlation heatmap analysis of the archaeal phyla with rumen fermentation parameters and methane production. (B) Pearson correlation heatmap analysis of the archaeal genus with rumen fermentation parameters and methane production. *, P < 0.05, **, P < 0.01, ***, P < 0.001. The legend in the upper right corner is the color interval of the different correlation R values.
The results of the correlation analysis between the rumen archaeal composition and VFA and methane production are shown in Figure 7B. The Candidatus Methanomethylophilus was significantly negatively correlated with acetate. The abundances of Methanicrococcus and isobutyrate were significantly negatively correlated. Candidatus Nitroscosmicus was significantly negatively correlated with propionate and significantly positively correlated with CH4 production. Candidatus_Nitrososphaera was significantly negatively correlated with butyrate and TVFAs. uncultured_f_Methanomethylophilaceae was also negatively correlated with A/P.
4 Discussion
4.1 Effect of quercetin on in vitro rumen gas production
Gas production reflects the degradation of dry matter, especially carbohydrates, in feed (Liu et al., 2002). Therefore, in vitro gas production can be used as a reference index to measure feed degradability. Moreover, it can also preliminarily reflect the number of fermentable substances in the rumen, the activity of microorganisms and the availability of substrates (Patra and Saxena, 2010). Generally, an increase in the fermentability of nutrients in the rumen or an increase in microbial activity will increase the rumen GP. Flavonoids have different effects on rumen GP (Wang et al., 2022). Some studies have shown that flavonoids reduce total rumen GP (Amin et al., 2021). However, other studies have shown that quercetin and some flavonoid extracts increase rumen GP (Leiber et al., 2012). On the one hand, the effect of flavonoids on the rumen GP is related to the antibacterial effect of the flavonoids themselves (Cushnie and Lamb, 2005). In addition, the composition of the fermentation substrate and the rumen environment also influence the rumen GP. In this study, the decrease in the rumen GP after 32 h of fermentation and the theoretical maximum gas production may be related to the decreased DMD of the substrate, which is also reflected in the VFA results. Moreover, according to the microflora results, rumen bacteria such as Proteobacteria, Synergistota, Armatimonadota, and Proteobacteria were identified. WPS-2 and Desulfobacterota, Candidatus_Nitrocosmicus, Candidatus_Nitrososphaera, Methanimicrococcus, and Candidatus Methanomethylophilus may also influence the results. On the other hand, the gas production rate increased at the early phase (before 20 h) of fermentation, which indicated that the fermentation of carbohydrates by some microorganisms was promoted, and the composition of these microorganisms remains to be further studied. Since this experiment was conducted under simulated conditions in vitro, which could not fully replicate the dynamic rumen environment, further in vivo experiments are still needed to verify the results.
Rumen microorganisms produce large amounts of CO2, CH4, H2, and nitrogenous gas during the degradation of dietary carbohydrates and other nutrients, and it is thought that the production of CH4 is related to the reduction of CO2 by methanogens via H2 in acetate and butyrate fermentation (Bata and Rahayua, 2017). In this study, quercetin reduced the CH4 content in rumen gas (Table 2) but also decreased the acetate and butyrate concentrations in group Q2 and decreased the abundance of B. methane, which further confirmed the above inference. Several in vitro experiments reported that quercetin reduced CH4 production by 43% after 48 h (Nørskov et al., 2023), and even plant leaves containing quercetin had significant CH4-inhibiting effects (Purba et al., 2019). This may be related to the widespread antimicrobial properties of flavonoids (Cushnie and Lamb, 2005). Since methanogens live on rumen ciliates (protozoa) and can use the hydrogen produced by the former to synthesize CH4 (Vogels et al., 1980), the inhibitory effect of quercetin on protozoa indirectly reduces CH4 production (Kim et al., 2015). This is similar to the effect of tea saponin on reducing rumen CH4 production (Guo et al., 2008; Hess et al., 2004), but it is different from the effect of tannins because tannins bind directly with proteins on the surface of methanogens to reduce CH4 (Nørskov et al., 2023). Ruminant animals can use their ingested energy more effectively by reducing methane emissions, thereby improving growth performance and production efficiency (Sun et al., 2023). Moreover, by reducing methane emissions, the sustainability of agriculture can be improved, and the burden on the environment can be reduced (Nørskov et al., 2023). Therefore, reducing methane emissions has important nutritional and ecological importance.
4.2 Effect of quercetin on in vitro rumen fermentation parameters and dry matter digestibility
pH is an important index for evaluating the acid production of rumen microorganisms and is affected by factors such as saliva secretion, rumen organic acid accumulation, and the ratio of grain to roughage. Studies have shown that the pH value of rumen fluid fluctuates between 5.5 and 7.5 and that excessively acidic and alkaline environments are not conducive to the survival of rumen microorganisms or rumen fermentation (Feng et al., 2020). Under in vitro experimental conditions, the rumen pH value excludes the influence of saliva entry and the secretory function of the rumen wall and is completely dependent on the acid produced by substrate fermentation. The results of this study revealed that quercetin reduces the concentration of TVFAs in the rumen and promotes the production of alkaline substances (NH3-N), which is conducive to maintaining the normal pH value of the rumen; these findings are consistent with those of Purba et al. (2019), who recommended 0.1–15 mg of Piper betle L. that contains 1.84% quercetin on a DM basis.
The NH3-N concentration can be affected by decomposed protein in feed by microorganisms (Zheng et al., 2021) and can also reflect the utilization of protein by rumen microorganisms. Therefore, the NH3-N concentration is an important indicator of the utility of protein in the rumen. Thao et al. (2014) reported that NH3-N concentrations ranging from 5–30 mg·dL−1 were most suitable for rumen microbial growth and that the higher the NH3-N concentration was, the more MCP was synthesized (Lu et al., 2019). MCP can meet 60%−70% of the protein requirements of ruminants and is the main nitrogen source of ruminants and an important indicator reflecting the nitrogen use efficiency of microorganisms (Zheng et al., 2020). In this study, with increasing quercetin supplementation, the NH3-N and MCP concentrations increased quadratically, indicating that quercetin favors the utilization of nitrogen in the rumen. On the other hand, the increase in microorganisms can also promote the degradation of nitrogenous substances in the rumen, thereby increasing the NH3-N concentration in the rumen (Ramos-Morales et al., 2018).
The DMD reflects the overall digestion of nutrients in the rumen and is an important indicator of the nutritional value of feed, and a high DMD is usually due to the strong activity of rumen microbes and fuller fermentation (Kamra et al., 2006). Sinz et al. (2018) showed that there was no significant difference in the effect of 4.5% quercetin on DMD compared with the control group. In this study, the addition of quercetin decreased DMD in linear and quadratic forms (P linear = 0.013, P quadratic = 0.032). According to the study of Cushnie and Lamb (2005), this result may be due to the antibacterial properties of flavonoids. It may also be that the activity of cellulolytic bacteria and amylolytic bacteria reduces DMD.
VFAs can provide approximately 70%−80% of the energy available for ruminant metabolism. Acetate is one of the main products of rumen microbial activity, the main decomposition product of cellulose and hemicellulose, and as the main precursor of ruminant fat synthesis, it is essential for the synthesis of milk fat and body fat (Jian et al., 2020). Propionate is mainly used for the synthesis of body fat and lactose and can be converted into glucose through hepatic gluconeogenesis to supply energy; the greater the proportion of propionate is, the more energy it can provide to the body (Ballard, 1972). Fermentation of propionate (usually an acetate to propionate ratio < 3) is also conducive to weight gain in beef cattle. Moreover, the production of propionate competitively consumes a large amount of H2, which in turn inhibits the production of CH4 (Janssen, 2010). In this study, a significant increase in the propionate concentration and a decrease in the CH4 content in the rumen gas were also observed in group Q3. This is also confirmed by the results of Kamra et al. (2006) and Patra and Saxena (2010). That is, the addition of flavonoids can reduce the production of rumen CH4 and reduce A/P. TVFA was generally positively correlated with DMD, and the decrease in DMD in this study led to a significant decrease in the TVFA concentration in group Q2, which was related to the significant decrease in acetate and butyrate in this group. However, there was no significant difference in TVFA concentration between groups Q1 and Q3.
4.3 Effect of quercetin on in vitro rumen bacteria
In this study, the Ace index and Chao index increased quadratically with quercetin supplementation (P < 0.05), indicating that the addition of quercetin may increase the richness of microorganisms but does not affect the diversity of the rumen microbiota, as demonstrated by Oskoueian et al. (2013). The results of this study are consistent with those of previous reports and are likely related to the antibacterial effects of flavonoids on certain bacterial phyla and genera.
The dominant bacterial phyla in the rumen fluid include Firmicutes, Proteobacteria, Bacteroidetes, Actinomycetes, and Patellar Bacteria, and their abundance changes with rumen digestion (Thao et al., 2014). In this study, Firmicutes and Bacteroidetes, which work together to hydrolyse and synthesize carbohydrates and proteins (Bäckhed et al., 2005), were the dominant phyla in the rumen, followed by Proteobacteria, Actinomycetes, and Patellar Bacteria. On the other hand, the sum of Firmicutes and Bacteroidetes in group C (94.55%) was greater than that in the other quercetin groups, and the lowest was in group Q3 (89.59%). This suggests the inhibitory effect of quercetin on the degradation of nutrients in the rumen, which is consistent with the reduction in the rumen GP in group Q3. This is caused mainly by the antibacterial action of quercetin, which has been reported in many studies (Li et al., 2024; Wang et al., 2018; Aljadaan et al., 2020).
At the genus level, the abundance of Succiniclasticum increased with the addition of quercetin. It also accounts for the increase in propionate content in groups Q1 and Q3 because of its ability to degrade starch, fiber and cellobiose as fermentation substrates and convert succinic acid to propionate (Li et al., 2018). Succinic acid can be converted to propionic acid through a variety of pathways, one of which is that Acetyle-CoA enters the citrate cycle (TCA cycle) and finally synthesizes propionic acid through the succinic acid pathway or lactic acid pathway (Mu et al., 2021). In terms of rumen gas production, methanogens are thought to be able to use metabolites (including acetate, propionate, CH4, CO2, and H2) produced by other microorganisms to synthesize CH4 with H2 and CO2, which ensures the relative stability of the partial pressure of hydrogen in the rumen (Leiber et al., 2012). However, when the abundance of Succiniclasticum increased in the rumen, it competed with hydrotrophic methanogens, which also explained why quercetin reduces CH4 production via a molecular mechanism. Although norank_f__norank_o__ Rickettsiales and Curtobacterium are also significantly reduced, they account for a very low proportion of the microflora and may therefore have a relatively low impact on CH4 production.
4.4 Effect of quercetin on in vitro rumen archaea
Archaea are thought to be most closely associated with methane emissions. However, there is no such correlation between methane emissions and the total abundance of archaea (Tapio et al., 2017). This happens not only to cattle but also to sheep (Kittelmann et al., 2013). In this study, there was no significant difference in the Chao1 index, ACE index, Shannon index or Simpson index between the quercetin groups and the control group, indicating that the addition of quercetin affected methane production without affecting the diversity or richness of the archaea.
Methanogens are the main archaea in the rumen (Janssen and Kirs, 2008). Approximately 89.3% of the total number of archaea in the rumen fluid are Methanobacteridae (Janssen and Kirs, 2008). In this study, 99% of the archaea at the phylum level were euryarchaeota, and the relative abundance of Methanobacteridae was similar in every group. These findings further confirmed that rumen methane production is not necessarily related to archaea. Rumen methane production is a complex process in which methanogens cooperate with bacteria, protozoa and fungi to form a symbiotic system in which CO2, formic acid, acetate, ethanol, and methyl compounds (methanol, monomethylamine, dimethylamine, or dimethyl sulfur) are used to carry out carbon cycling. Although most archaea, such as B. methane and B. vannamei, can use H2 and CO2 to produce CH4 through the hydrotrophic pathway (Sharp et al., 1998; Danielsson et al., 2017), other ways in which methane can be produced (Qiao et al., 2014). Flavonoids (including quercetin) inhibit methane production (Patra and Saxena, 2010; Tavendale et al., 2005). The same results were also observed in this study, but only Methanobrevibacter in group Q2 was significantly reduced, indicating that quercetin had a greater effect on gas-producing bacteria than on rumen archaea. However, whether quercetin has other influences or a specific effect at a certain dose remains to be confirmed.
5 Conclusions
In summary, quercetin decreased in vitro rumen gas production and methane production, increased the enriched NH3-N and MCP concentrations, and decreased the abundance of Brevibacterium methanescens in the rumen. Supplementation with 1.5% quercetin significantly increased the rumen propionate concentration, increased the abundance of rumen succinate bacteria, and decreased the relative abundances of norank_f__norank_o__Rickettsiales and Curtobacterium. Overall, quercetin influenced in vitro rumen fermentation and the rumen bacterial flora to decrease methane production and promote rumen nitrogen utilization and MCP synthesis.
Data availability statement
The original contributions presented in the study are publicly available. This data can be found here: https://www.ncbi.nlm.nih.gov/, accession numbers: PRJNA1141169, PRJNA1141186.
Ethics statement
The animal study was approved by the Laboratory Animal Ethics Committee of Inner Mongolia Minzu University (Approval Code: 2024031016001). The study was conducted in accordance with the local legislation and institutional requirements.
Author contributions
MX: Conceptualization, Data curation, Investigation, Methodology, Software, Visualization, Writing – original draft, Writing – review & editing. LD: Conceptualization, Data curation, Investigation, Methodology, Software, Writing – original draft, Writing – review & editing. MW: Methodology, Project administration, Resources, Supervision, Writing – original draft, Writing – review & editing, Conceptualization, Formal analysis, Funding acquisition. YaW: Conceptualization, Formal analysis, Funding acquisition, Project administration, Resources, Supervision, Writing – original draft, Writing – review & editing. CD: Formal analysis, Investigation, Software, Supervision, Writing – original draft, Writing – review & editing. JJ: Data curation, Formal analysis, Investigation, Software, Supervision, Writing – original draft, Writing – review & editing. RZ: Formal analysis, Investigation, Methodology, Software, Writing – original draft, Writing – review & editing. WP: Investigation, Software, Supervision, Visualization, Writing – original draft, Writing – review & editing. YuW: Investigation, Software, Supervision, Visualization, Writing – original draft, Writing – review & editing. YZ: Formal analysis, Investigation, Software, Supervision, Writing – original draft, Writing – review & editing. WM: Formal analysis, Investigation, Software, Supervision, Writing – original draft, Writing – review & editing.
Funding
The author(s) declare that financial support was received for the research and/or publication of this article. This research was funded by the Inner Mongolia Science and Technology Support Project (No. 2022YFXZ0015), development and demonstration of comprehensive supporting technology for efficient and safe utilization of forage and healthy and high yield for dairy cows and the Inner Mongolia Natural Science Foundation (No. 2022MS03072).
Acknowledgments
We thank the students in the laboratory for their help in sample collection and index determination. We also thank Tongliao Mufeng Jiuyuan Animal Husbandry Co., Ltd., for providing the experimental animals.
Conflict of interest
The authors declare that the research was conducted in the absence of any commercial or financial relationships that could be construed as potential conflicts of interest.
Generative AI statement
The author(s) declare that no Gen AI was used in the creation of this manuscript.
Publisher's note
All claims expressed in this article are solely those of the authors and do not necessarily represent those of their affiliated organizations, or those of the publisher, the editors and the reviewers. Any product that may be evaluated in this article, or claim that may be made by its manufacturer, is not guaranteed or endorsed by the publisher.
References
Aljadaan, S. A. N., Elias, R. S., and Al-Anssari, R. A. (2020). Investigation of the antioxidant and antibacterial activity of novel quercetin derivatives. Biointerface Res. Appl. Chem. 10, 7329–7336. doi: 10.33263/BRIAC106.73297336
Amin, N., Bailoni, L., Wendler, K. R., Caldwell, J., and Pourazad, P. (2021). “Effects of citrus flavonoid, vanillin, and their combination on in vitro rumen fermentation parameters and methane production,” in Boku-Symposium Tierernährung (Universität für Bodenkultur Wien), 1–5.
Bäckhed, F., Ley, R. E., Sonnenburg, J. L., Peterson, D. A., and Gordon, J. I. (2005). Host-bacterial mutualism in the human intestine. Science 307, 1915–1920. doi: 10.1126/science.1104816
Balcells, J., Aris, A., Serrano, A., Seradj, A. R., Crespo, J., and Devant, M. (2012). Effects of an extract of plant flavonoids (Bioflavex) on rumen fermentation and performance in heifers fed high-concentrate diets. J. Anim. Sci. 90, 4975–4984. doi: 10.2527/jas.2011-4955
Ballard, F. J. (1972). Supply and utilization of acetate in mammals. Am. J. Clin. Nutr. 25, 773–779. doi: 10.1093/ajcn/25.8.773
Bata, M., and Rahayua, S. (2017). Evaluation of bioactive substances in Hibiscus tiliaceus and its potential as a ruminant feed additive. Curr. Bioact. Compd. 13, 157–164. doi: 10.2174/1573407213666170109151904
Belanche, A., Newbold, C. J., Morgavi, D. P., Bach, A., Zweifel, B., and Yáñez-Ruiz, D. R. (2020). A meta-analysis describing the effects of the essential oils blend agolin ruminant on performance, rumen fermentation and methane emissions in dairy cows. Animals 10, 620–635. doi: 10.3390/ani10040620
Bodas, R., Prieto, N., García-González, R., Andrés, S., Giráldez, F. J., and López, S. (2012). Manipulation of rumen fermentation and methane production with plant secondary metabolites. Anim. Feed Sci. Tech. 176, 78–93. doi: 10.1016/j.anifeedsci.2012.07.010
Carullo, G., Cappello, A. R., Frattaruolo, L., Badolato, M., Armentano, B., and Aiello, F. (2017). Quercetin and derivatives: useful tools in inflammation and pain management. Future Med. Chem. 9, 79–93. doi: 10.4155/fmc-2016-0186
Chen, J., Lei, X. J., Wang, L., Zhang, Y. L., Wang, D. D., and Zhao, L. C. (2022). Effects of rumen-protected leucine on production performance and starch digestion in the small intestine of lactating goats. Anim. Feed. Sci. 287:115270. doi: 10.1016/j.anifeedsci.2022.115270
Cushnie, T. P., and Lamb, A. J. (2005). Antimicrobial activity of flavonoids. Int. J. Antimicrob. Agents. 26, 343–356. doi: 10.1016/j.ijantimicag.2005.09.002
Danielsson, R., Dicksved, J., Sun, L., Gonda, H., Müller, B., and Schnürer, A. (2017). Methane production in dairy cows correlates with rumen methanogenic and bacterial community structure. Front. Microbiol. 8:226. doi: 10.3389/fmicb.2017.00226
Ellis, J. L., Kebreab, E., Odongo, N. E., McBride, B. W., Okine, E. K., and France, J. (2007). Prediction of methane production from dairy and beef cattle. J. Dairy Sci. 90, 3456–3466. doi: 10.3168/jds.2006-675
Feng, F., Li, H., Yang, J., Gao, Q., Yang, Y., and Zhang, J. (2020). Effects of mixed silage containing cornstraw and rape on the in vitro fermentation characteristics of rumen fluid from Tan sheep. Pratac. Sci. 37, 2343–2350. doi: 10.11829/j.issn.1001-0629.2020-0242
Geng, L., Liu, Z., Zhang, W., Li, W., Wu, Z., and Wang, W. (2019). Chemical screen identifies a geroprotective role of quercetin in premature aging. Protein Cell 10, 417–435. doi: 10.1007/s13238-018-0567-y
Guo, Y. Q., Liu, J. X., Lu, Y., Zhu, W. Y., Denman, S. E., and McSweeney, C. S. (2008). Effect of tea saponin on methanogenesis, microbial community structure and expression of mcrA gene, in cultures of rumen micro-organisms. Lett. Appl. Microbiol. 47, 421–426. doi: 10.1111/j.1472-765X.2008.02459.x
Hess, H. D., Beuret, R. A., Lötscher, M., Hindrichsen, I. K., Machmüller, A., and Carulla, J. E. (2004). Ruminal fermentation, methanogenesis and nitrogen utilization of sheep receiving tropical grass hay-concentrate diets offered with Sapindus saponaria fruits and Cratylia argentea foliage. Animal Sci. 79, 177–189. doi: 10.1017/S1357729800054643
Janssen, P. H. (2010). Influence of hydrogen on rumen methane formation and fermentation balances through microbial growth kinetics and fermentation thermodynamics. Anim. Feed Sci. Tech. 160, 1–22. doi: 10.1016/j.anifeedsci.2010.07.002
Janssen, P. H., and Kirs, M. (2008). Structure of the archaeal community of the rumen. Appl. Environ. Microbiol. 74, 3619–3625. doi: 10.1128/AEM.02812-07
Jian, S., Wang, F., Guo, Z., Ma, Z., Chen, X., and Wu, W. (2020). Evaluation of red yeast rice distillers' grains used as feed for goats by rumen fermentation in vitro. Chin. J. Animal Nutr. 32, 3780–3788
Kamra, D. N., Agarwal, N., and Chaudhary, L. C. (2006). Inhibition of ruminal methanogenesis by tropical plants containing secondary compounds. Int. Congr. Ser. 1293, 156–163. doi: 10.1016/j.ics.2006.02.002
Kim, E. T., Guan, le, L., Lee, S. J., Lee, S. M., Lee, S. S., and Lee, I. D. (2015). Effects of flavonoid-rich plant extracts on in vitro ruminal methanogenesis, microbial populations and fermentation characteristics. Asian-Austral. J. Animal Sci. 28, 530–537. doi: 10.5713/ajas.14.0692
Kim, Y. K., Roy, P. K., Ashrafudoulla, M., Nahar, S., Toushik, S. H., and Hossain, M. I. (2022). Antibiofilm effects of quercetin against Salmonella enterica biofilm formation and virulence, stress response, and quorum-sensing gene expression. Food Control. 137:108964. doi: 10.1016/j.foodcont.2022.108964
Kittelmann, S., Seedorf, H., Walters, W. A., Clemente, J. C., Knight, R., and Gordon, J. I. (2013). Simultaneous amplicon sequencing to explore co-occurrence patterns of bacterial, archaeal and eukaryotic microorganisms in rumen microbial communities. PLoS ONE 8:e47879. doi: 10.1371/journal.pone.0047879
Lan, W., and Yang, C. (2019). Ruminal methane production: associated microorganisms and the potential of applying hydrogen-utilizing bacteria for mitigation. Sci. Total Environ. 654, 1270–1283. doi: 10.1016/j.scitotenv.2018.11.180
Leiber, F., Kunz, C., and Kreuzer, M. (2012). Influence of different morphological parts of buckwheat (Fagopyrum esculentum) and its major secondary metabolite rutin on rumen fermentation in vitro. Czech J. Anim. Sci. 57, 10–18. doi: 10.17221/5479-CJAS
Lespade, L. (2016). Ab initio molecular dynamics of the reaction of quercetin with superoxide radical. Chem. Phys. 475, 32–38. doi: 10.1016/j.chemphys.2016.06.006
Li, B., Yan, Y., Zhang, T., Xu, H., Wu, X., and Yao, G. (2024). Quercetin reshapes gut microbiota homeostasis and modulates brain metabolic profile to regulate depression-like behaviors induced by CUMS in rats. Front. Pharmacol. 15:1362464. doi: 10.3389/fphar.2024.1362464
Li, L., Qu, M., Liu, C., Xu, L., Pan, K., and Song, X. (2018). Expression of a recombinant lentinula edodes xylanase by pichia pastoris and its effects on ruminal fermentation and microbial community in in vitro incubation of agricultural straws. Front. Microbiol. 9:2944. doi: 10.3389/fmicb.2018.02944
Liebig, M. A., Gross, J. R., Kronberg, S. L., Phillips, R. L., and Hanson, J. D. (2010). Grazing management contributions to net global warming potential: a long-term evaluation in the Northern Great Plains. J. Environ. Qual. 39, 799–809. doi: 10.2134/jeq2009.0272
Liu, J. X., Susenbeth, A., and Südekum, K. H. (2002). In vitro gas production measurements to evaluate interactions between untreated and chemically treated rice straws, grass hay, and mulberry leaves. J. Anim. Sci. 80, 517–524. doi: 10.2527/2002.802517x
Lu, Z., Shen, H., and Shen, Z. (2019). Effects of dietary-SCFA on microbial protein synthesis and urinal Urea-N excretion are related to microbiota diversity in rumen. Front. Physiol. 10:1079. doi: 10.3389/fphys.2019.01079
Menke, H. H., and Steingass, H. (1988). Estimation of the energetic feed value obtained from chemical analysis and in vitro gas production using rumen fluid. Anim Res Dev. 28, 7–55.
Mu, Y. Y., Qi, W. P., Zhang, T., Zhang, J. Y., and Mao, S. Y. (2021). Gene function adjustment for carbohydrate metabolism and enrichment of rumen microbiota with antibiotic resistance genes during subacute rumen acidosis induced by a high-grain diet in lactating dairy cows. J. Dairy Sci. 104, 2087–2105. doi: 10.3168/jds.2020-19118
Nam, J. S., Sharma, A. R., Nguyen, L. T., Chakraborty, C., Sharma, G., and Lee, S. S. (2016). Application of bioactive quercetin in oncotherapy:from nutrition to nanomedicine. Molecules 21:108. doi: 10.3390/molecules21010108
NASEM. (2016). Nutrient Requirements of Beef Cattle: Eighth Revised Edition. Washington, DC: The National Academies Press.
Nørskov, N. P., Battelli, M., Curtasu, M. V., Olijhoek, D. W., Chass,é, É., and Nielsen, M. O. (2023). Methane reduction by quercetin, tannic and salicylic acids: influence of molecular structures on methane formation and fermentation in vitro. Sci. Rep. 13:16023. doi: 10.1038/s41598-023-43041-w
Ørskov, E. R., and McDonald, I. (1979). The estimation of protein degradability in the rumen from incubation measurements weighted according to rate of passage. J. Agric. Sci. 92, 499–503. doi: 10.1017/S0021859600063048
Oskoueian, E., Abdullah, N., and Oskoueian, A. (2013). Effects of flavonoids on rumen fermentation activity, methane production, and microbial population. Biomed Res. Int. 2013:349129. doi: 10.1155/2013/349129
Patra, A. K., and Saxena, J. (2010). A new perspective on the use of plant secondary metabolites to inhibit methanogenesis in the rumen. Phytochemistry 71, 1198–1222. doi: 10.1016/j.phytochem.2010.05.010
Purba, R. A. P., Paengkoum, P., and Yuangklang, C. (2019). In vitro ruminal fermentation and methane production of PUFA-containing rations as treated by flavonoid and essential oil from Piper betle L. Preprints. 2019:2019040156. doi: 10.20944/preprints201904.0156.v1
Qiao, J., Tan, Z., and Wang, M. (2014). Potential and existing mechanisms of enteric methane production in ruminants. Sci. Agric. 71, 430–440. doi: 10.1590/0103-9016-2013-0423
Ramos-Morales, E., Rossi, G., Cattin, M., Jones, E., Braganca, R., and Newbold, C. J. (2018). The effect of an isoflavonid-rich liquorice extract on fermentation, methanogenesis and the microbiome in the rumen simulation technique. FEMS Microbiol Ecol. 94:fiy009. doi: 10.1093/femsec/fiy009
Sedmak, J. J., and Grossberg, S. E. (1977). A rapid, sensitive, and versatile assay for protein using Coomassie brilliant blue G250. Anal. Biochem. 79, 544–552. doi: 10.1016/0003-2697(77)90428-6
Sharp, R., Ziemer, C. J., Stern, M. D., and Stahl, D. A. (1998). Taxon-specific associations between protozoal and methanogen populations in the rumen and a model rumen system. FEMS Microbiol. Ecol. 26, 71–78. doi: 10.1111/j.1574-6941.1998.tb01563.x
Sinz, S., Kunz, C., Liesegang, A., Braun, U., Marquardt, S., and Soliva, C. R. (2018). In vitro bioactivity of various pure flavonoids in ruminal fermentation, with special reference to methane formation. Czech J. Anim. Sci. 63, 293–304. doi: 10.17221/118/2017-CJAS
Soroye, P., Newbold, T., and Kerr, J. (2020). Climate change contributes to widespread declines among bumble bees across continents. Science 367, 685–688. doi: 10.1126/science.aax8591
Sun, J., Zhao, G., and Li, M. M. (2023). Using nutritional strategies to mitigate ruminal methane emissions from ruminants. Front. Agr. Sci. Eng. 10, 390–402. doi: 10.15302/J-FASE-2023504
Tapio, I., Snelling, T. J., Strozzi, F., and Wallace, R. J. (2017). The ruminal microbiome associated with methane emissions from ruminant livestock. J. Anim. Sci. Biotechnol. 8:7. doi: 10.1186/s40104-017-0141-0
Tavendale, M. H., Meagher, L. P., Pacheco, D., Walker, N., Attwood, G. T., and Sivakumaran, S. (2005). Methane production from in vitro rumen incubations with Lotus pedunculatus and Medicago sativa, and effects of extractable condensed tannin fractions on methanogenesis. Anim. Feed Sci. Tech. 123, 403–419. doi: 10.1016/j.anifeedsci.2005.04.037
Thao, N. T., Wanapat, M., Cherdthong, A., and Kang, S. (2014). Effects of eucalyptus crude oils supplementation on rumen fermentation, microorganism and nutrient digestibility in swamp buffaloes. Asian-australas. J. Anim. Sci. 27, 46–54. doi: 10.5713/ajas.2013.13301
Tilley, J. M. A., and Terry, D. R. (1963). A two-stage technique for the in vitro digestion of forage crops. Grass Forage Sci. 18, 104–111. doi: 10.1111/j.1365-2494.1963.tb00335.x
Verdouw, H., Van Echteld, C. J. A., and Dekkers, E. M. J. (1978). Ammonia determination based on indophenol formation with sodium salicylate. Water Res. 12, 399–402. doi: 10.1016/0043-1354(78)90107-0
Vogels, G. D., Hoppe, W. F., and Stumm, C. K. (1980). Association of methanogenic bacteria with rumen ciliates. Appl. Environ. Microbiol. 40, 608–612. doi: 10.1128/aem.40.3.608-612.1980
Wang, S., Yao, J., Zhou, B., Yang, J., Chaudry, M. T., and Wang, M. (2018). Bacteriostatic effect of quercetin as an antibiotic alternative in vivo and its antibacterial mechanism in vitro. J. Food Prot. 81, 68–78. doi: 10.4315/0362-028X.JFP-17-214
Wang, Z., Li, X., Zhang, L., Wu, J., Zhao, S., and Jiao, T. (2022). Effect of oregano oil and cobalt lactate on sheep in vitro digestibility, fermentation characteristics and rumen microbial community. Animals 12:118. doi: 10.3390/ani12010118
Zheng, Y., Xue, S., Zhao, Y., and Li, S. (2020). Effect of cassava residue substituting for crushed maize on in vitro ruminal fermentation characteristics of dairy cows at mid-lactation. Animals 10:893. doi: 10.3390/ani10050893
Keywords: quercetin, beef cattle, in vitro, rumen fermentation, gas production, microflora
Citation: Xiao M, Du L, Wei M, Wang Y, Dong C, Ju J, Zhang R, Peng W, Wang Y, Zheng Y and Meng W (2025) Effects of quercetin on in vitro rumen fermentation parameters, gas production and microflora of beef cattle. Front. Microbiol. 16:1527405. doi: 10.3389/fmicb.2025.1527405
Received: 13 November 2024; Accepted: 11 April 2025;
Published: 30 April 2025.
Edited by:
Grzegorz Bełżecki, Polish Academy of Sciences, PolandReviewed by:
Tarique Hussain, Nuclear Institute for Agriculture and Biology, PakistanZhipeng He, Nanchang University, China
Copyright © 2025 Xiao, Du, Wei, Wang, Dong, Ju, Zhang, Peng, Wang, Zheng and Meng. This is an open-access article distributed under the terms of the Creative Commons Attribution License (CC BY). The use, distribution or reproduction in other forums is permitted, provided the original author(s) and the copyright owner(s) are credited and that the original publication in this journal is cited, in accordance with accepted academic practice. No use, distribution or reproduction is permitted which does not comply with these terms.
*Correspondence: Manlin Wei, d2VpbWFubGluQDE2My5jb20=
†These authors have contributed equally to this work