- 1School of Biological Sciences and Technology, Liupanshui Normal University, Liupanshui, China
- 2College of Agronomy and Life Sciences, Zhaotong University, Zhaotong, China
When forage crops are ravaged by leaf spot disease, producers face an unavoidable dilemma: a careful consideration of adopting specific measures to make use of the damaged forage. Furthermore, silage is often exposed to air during production, feeding, storage, and transportation. The aim of this study is to investigate the effects of three ensiling periods (15, 30, and 60 days), two crops (Italian ryegrass, and oats), and three additives [control group (CK), inoculated with Lactobacillus plantarum YM3, and L. rhamnosus HT1] on the health and fermentation quality of silage infected with leaf spot disease during aerobic exposure. Silage ensiled for 15 days had the highest lactic acid concentration and the lowest butyric acid concentration (p < 0.05). The acetic acid concentration of Italian ryegrass silage (5.77 g kg−1 DM) was higher than that of oat silage (2.89 g kg−1 DM), and the butyric acid concentration was lower (2.70 g kg−1 DM versus 5.94 g kg−1 DM) (p < 0.05). The lactic acid concentration of silage inoculated with L. rhamnosus HT1 (92.0 g kg−1 DM) was significantly higher than that inoculated with L. plantarum YM3 (57.3 g kg−1 DM) and the CK (69.5 g kg−1 DM) (p < 0.05). For most undesirable bacterial species, such as Stenotrophomonas, Providencia, Paenalcaligenes, Myroides, and Alcaligenes, the relative abundances in the silage ensiled for 60 d were generally higher than in those ensiled for 15 and 30 days. The relative abundance of harmful bacteria in oat silage was higher than that in Italian ryegrass silage. The relative abundances of Stenotrophomonas and Providencia in the CK treatment were higher than those in the silage inoculated with L. plantarum YM3 and L. rhamnosus HT1. The addition of lactic acid bacteria (LAB) helped to inhibit the increase in the relative abundance of harmful bacteria. Therefore, when silage has to be exposed to air, this study recommends that Italian ryegrass be inoculated with Lactobacillus rhamnosus HT1 and used within 15 days of ensiling.
Introduction
Silage, a crucial feed source in animal husbandry, directly influences the growth rate and health of livestock (Little et al., 2017). However, forage crops often encounter various disease challenges during their growth, with leaf spot disease being the most prevalent (Victoria Arellano et al., 2022). To date, over 40 countries have reported cases of leaf spot disease, primarily affecting alfalfa (Lan et al., 2024). This disease not only causes yield losses ranging from 31% to 82% in greenhouse environments and 56% in field conditions but also severely compromises the nutritional composition of forage, potentially impacting livestock reproductive capability, particularly posing a significant risk to ovulation rates (Lan et al., 2024). However, when forage crops are ravaged by leaf spot disease, producers face an unavoidable dilemma. They must carefully consider adopting specific measures to make use of this damaged forage, as seeking alternative feed sources often entails a greater economic burden. Therefore, effectively enhancing the quality of silage made from forage infected with leaf spot disease has emerged as a core challenge that needs to be addressed in the animal husbandry sector. Lactic acid bacteria (LAB) are probiotics that play a pivotal role in silage fermentation (Okoye et al., 2023). Through their unique fermentation mechanism, they significantly reduce the pH of forage, effectively inhibiting the growth of harmful microorganisms, thereby extending the shelf life of silage and improving its fermentation quality (Jiang et al., 2020). However, the current understanding of how LAB additives regulate fermentation quality and microbial community health of silage made from forage infected with leaf spot disease remains unclear.
Silage duration is a key factor that affects silage quality. If the silage period is too short, the feed may not ferment sufficiently; conversely, a period that is too long may lead to significant nutrient loss. Although prolonged silage duration can inhibit the growth of harmful microorganisms, it often results in greater nutrient loss. For example, when the silage duration increases from 0 to 45 days, the ammonia nitrogen content in whole-plant maize (Zea mays) silage increases from 15.5–20.0 (g kg−1 total N) to 31.9–41.7 (g kg−1 total N) (Huang et al., 2021), and this situation may be exacerbated in alfalfa (Medicago sativa) (Gao et al., 2021). However, after the fermentation period exceeds 7 days, LAB in silage are primarily beneficial microorganisms such as Weissella and Lactobacillus (Li et al., 2020). Therefore, selecting an appropriate duration is crucial for improving the fermentation quality of silage. Additionally, silage is often exposed to air during production, feeding, storage, and transportation (Bolsen, 2018; Yin et al., 2021). Aerobic exposure increases the oxygen content of silage, promoting the growth and reproduction of aerobic microorganisms, thereby affecting its fermentation quality and microbial community structure (Zhang et al., 2023). Therefore, exploring how aerobic exposure and silage duration affect the fermentation quality and microbial health status of silage is crucial for improving storage and transportation conditions, enhancing nutritional value, and extending shelf life. However, current research in this field, particularly in-depth studies on silage fermentation quality and microbial health, remains relatively scarce, and the details and underlying mechanisms require comprehensive elucidation.
This study focused on exploring the variation patterns in microbial community health and silage fermentation quality of forage infected with leaf spot disease after treatment with LAB and subjected to different ensiling periods under aerobic conditions. Based on this, the study proposes the following two hypotheses: (1) the extension of silage duration may promote the improvement of microbial health in silage exposed to air, and (2) the addition of LAB may lead to an improvement in the health status of silage exposed to air.
Materials and methods
Materials and silage additives
Italian ryegrass and oats were cultivated in the experimental field (27°33′N, 103°77′E) at Zhaotong University (Zhaotong, China). Forage with > 30% leaf spot was selected for ensiling when the Italian ryegrass and oats were at the flowering and milking stages, respectively. These materials were chopped into 20–30 mm for silage preparation. The silage additives used were L. plantarum YM3 (YM3) and L. rhamnosus HT1 (HT1), and the strains were obtained from the forage processing laboratory of South China Agricultural University.
Experimental design and silage preparation
The experimental design included three ensiling periods (15 days, AS15; 30 days, AS30; 60 days, AS60), two crop types (Italian ryegrass, IR_A; oats, Oat_A), and three additives (without, ASCK, YM3, ASYM3; HT1, and ASHT1). The chopped materials were mixed thoroughly with the three additives separately, packed into polyethylene plastic bags (200 × 300 mm; Zhongshan, China), and sealed with a vacuum sealer (P-290; Shenzhen, China). Strains YM3 and HT1 were inoculated at a rate of 1.0 × 105 colony-forming units (cfu)/g fresh matter (FM), and appropriate amounts were added to the sterilized small spray and diluted with 10 mL of sterile water. An equal volume of sterile water was added to the chopped material in the control group (CK). Strains YM3 and HT1 require activation in de Man, Rogosa, and Sharpe (MRS) broth medium before inoculation and detection of viable bacteria by the plate count method (Chen et al., 2022). The plastic bag silos were stored in a laboratory cabinet (15°C–25°C, dark environment) for 15, 30, and 60 days, respectively. The plastic bag silos were opened at the designated ensiling time and aerobically exposed for 7 days at room temperature (15°C–25°C) to analyze the microbial, fermentation and chemical characteristics.
Microbial population and fermentation quality analyses
The number of microorganisms was determined using a culture medium cultivation method (Chen X. et al., 2021; Tang et al., 2023). LAB, aerobic bacteria, yeasts, and molds were counted on MRS, nutrient, and potato dextrose agar, respectively. LAB were cultured under anaerobic conditions at 37°C for 2 days. Aerobic bacteria, yeasts and molds were cultured under aerobic conditions at 37°C for 3 days.
The pH of the filtrate (10 g fresh material added to 90 mL distilled water) was determined using a pH meter (LE438; Mettler Toledo, Shanghai, China). After measuring the pH, the filtrate was used to determine the organic acid concentration (using a small amount of cation-exchange resin). The organic acid concentration (lactic, acetic, propionic, and butyric acids) was measured using high-performance liquid chromatography (column: Sodex RS Pak KC-811, Showa Denko K.K., Kawasaki, Japan; detector: diode array detector, 210 nm, serial presence detect-20A, Shimadzu Co., Ltd., Kyoto, Japan; eluent: 3 mmol L−1 HClO4, 1.0 mL/min; temperature: 60°C) (Li et al., 2016).
Bacterial community analyses
According to the method described by Wang et al. (2020), a 10 g frozen sample was placed in 40-mL sterile water, and after homogenization, filtered with two layers of sterile medical gauze, subsequently, the gauze was rinsed with 40 mL sterile water three times to recover the residual microbes, which were recycled using a centrifuge at 12,000 g for 15 min at 4°C after the filtrate was combined. Genomic DNA was extracted from the silage samples using a bacterial DNA isolation kit (DE05311; Foregene, Chengdu, China). DNA concentration and purity were detected using NanoDrop2000C, with the optical density set at 260/280 nm (Chen et al., 2022). Qualified DNA samples were used for subsequent analyses. The DNA samples were paired-end sequenced using the Illumina NovaSeq6000 platform. The sequencing data were analyzed using the Majorbio Bio-Pharm cloud platform. The community structure was analyzed at the phylum and genus levels using the Silva database with a confidence threshold of 90% (Wang et al., 2020). Alpha diversity at the operational taxonomic unit (OTU) level was applied to analyze the complexity of species diversity for a sample through Chao and Shannon indices, and principal coordinate analysis was performed to obtain the bacterial community structural variance. Spearman’s correlation heatmap analysis was conducted to explore the relationship between the microbial community and fermentation products.
Statistical analyses
The plate count results for microorganisms were log-transformed before statistical analysis. One-and multi-way analysis of variance were performed using SPSS (version 25.0) for ensiling time, crop type, and additives. Duncan’s test was used to evaluate differences among treatments. Differences were considered statistically significant at p < 0.05. The model used was: Y = μ + Tj + εij, where Y was the response variable, μ was the overall mean, Tj was the treatment effect, and εij was the residual error.
Results
Effects of ensiling time, crop type and additives on organic acids and microbial number of silage after aerobic exposure
Regarding ensiling time, the number of aerobic bacteria in the AS15 treatment reached its peak compared to that in the AS30 and AS60 treatments (p < 0.05). The number of LAB gradually increased with prolonged silage duration and reached its highest value in the AS60 treatment (p < 0.05). The numbers of molds and yeasts in the AS30 and AS60 treatments were significantly higher than those in the AS15 treatment (p < 0.05). However, the effects of crop type and additives on the number of microorganisms were not significant (Table 1).
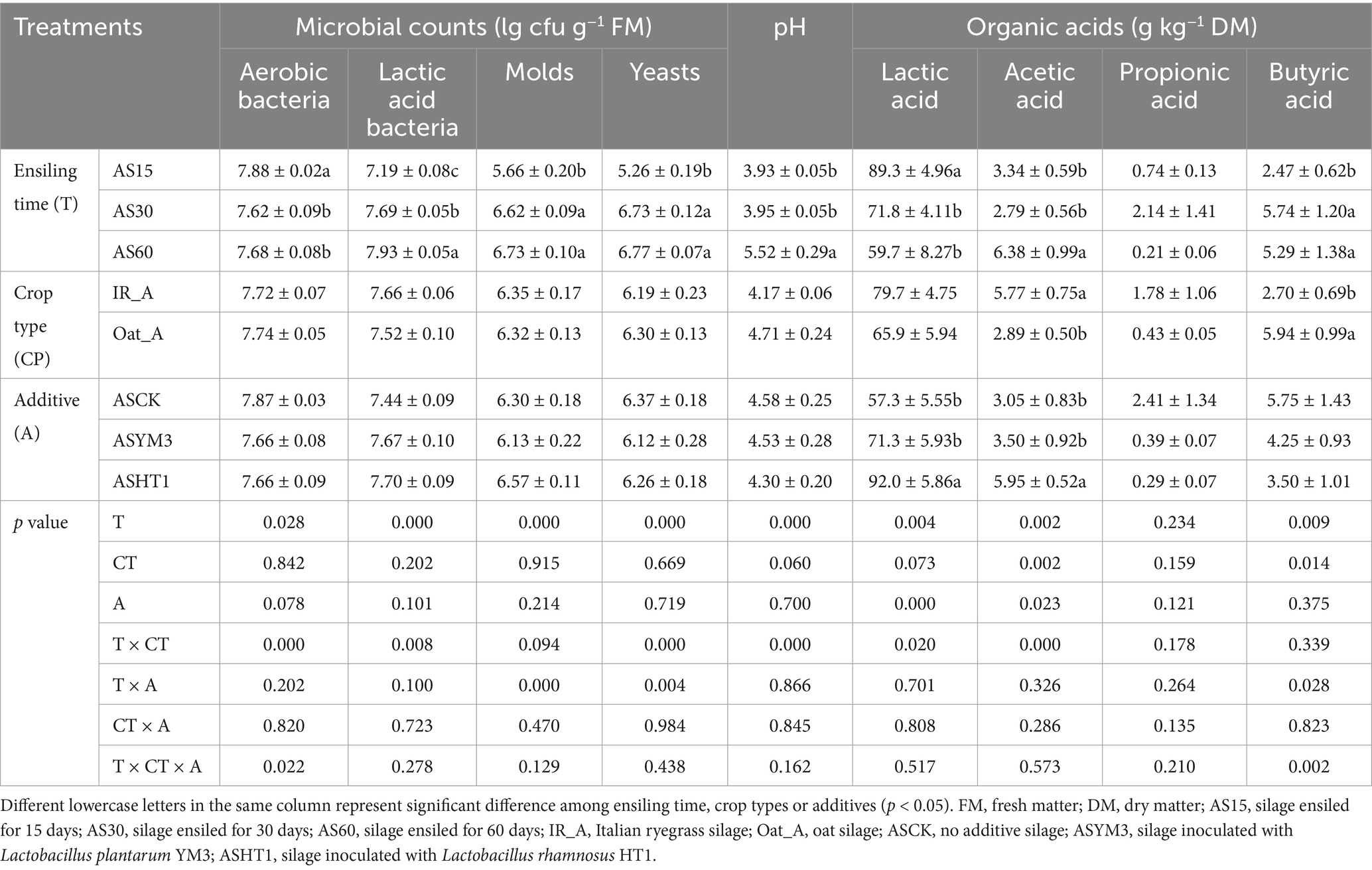
Table 1. Microbial counts, pH, and organic acids changes of silage after exposed to the air from different additives, crop types, and ensiling time (n = 54).
Regarding silage fermentation quality, the pH of the AS60 treatment was significantly higher than those of the AS15 and AS30 treatments (p < 0.05). However, crop type and additives did not significantly affect the pH. The AS15 treatment resulted in the highest lactic acid and lowest butyric acid concentrations (p < 0.05) (Table 1). The acetic acid concentration in the IR_A treatment (5.77 g kg−1 DM) was significantly higher than that in the Oat_A treatment (2.89 g kg−1 DM), and a lower butyric acid concentration was observed in the IR_A treatment (2.70 g kg−1 DM versus 5.94 g kg−1 DM) (p < 0.05). The ASHT1 treatment (92.0 g kg−1 DM) had a higher lactic acid concentration than those of the ASYM3 (57.3 g kg−1 DM) and ASCK (69.5 g kg−1 DM) treatments (p < 0.05).
Effects of ensiling time, crop type and additives on silage bacterial diversity after aerobic exposure
During ensiling, AS15, AS30, and AS60 had 4, 12, and 39 OTUs, respectively, yet they shared 56 OTUs in common (accounting for 42.1% of the total) (Figure 1A). Regarding crop type, the IR_A and Oat_A treatments shared 66 OTUs, however, it is noteworthy that the Oat_A treatment had an additional 17 unique OTUs compared to the IR_A treatment (Figure 1B). In total, 84, 76, and 73 OTUs were identified in the silage samples obtained from the ASCK, ASYM3, and ASHT1 treatments, respectively. This study found that the number of OTUs in the ASCK treatment was 11 (nine more than those in the ASHT1 and ASYM3 treatments), although these three treatments also shared 66 OTUs (Figure 1C). The highest relative abundance of Bacillus was observed in the AS60 treatment. However, the highest relative abundance of Lactobacillus was observed in the AS15 and AS30 treatments (Figure 2A). The community relative abundance of Lactobacillus was high and was not affected by crop type or additives (Figures 2B,C).
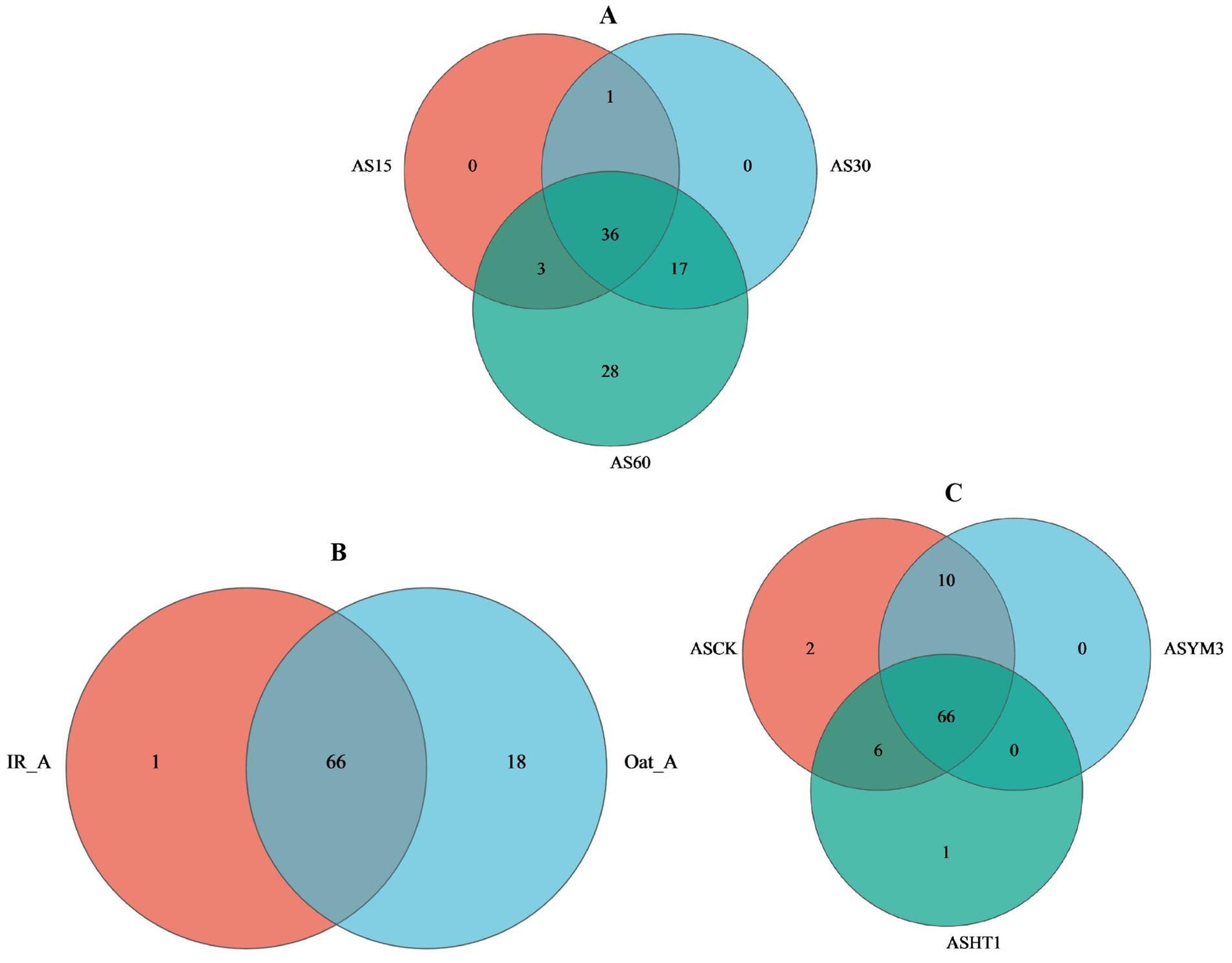
Figure 1. Venn diagram of bacterial communities in silage different ensiling period (A), crop types (B), and additives (C).
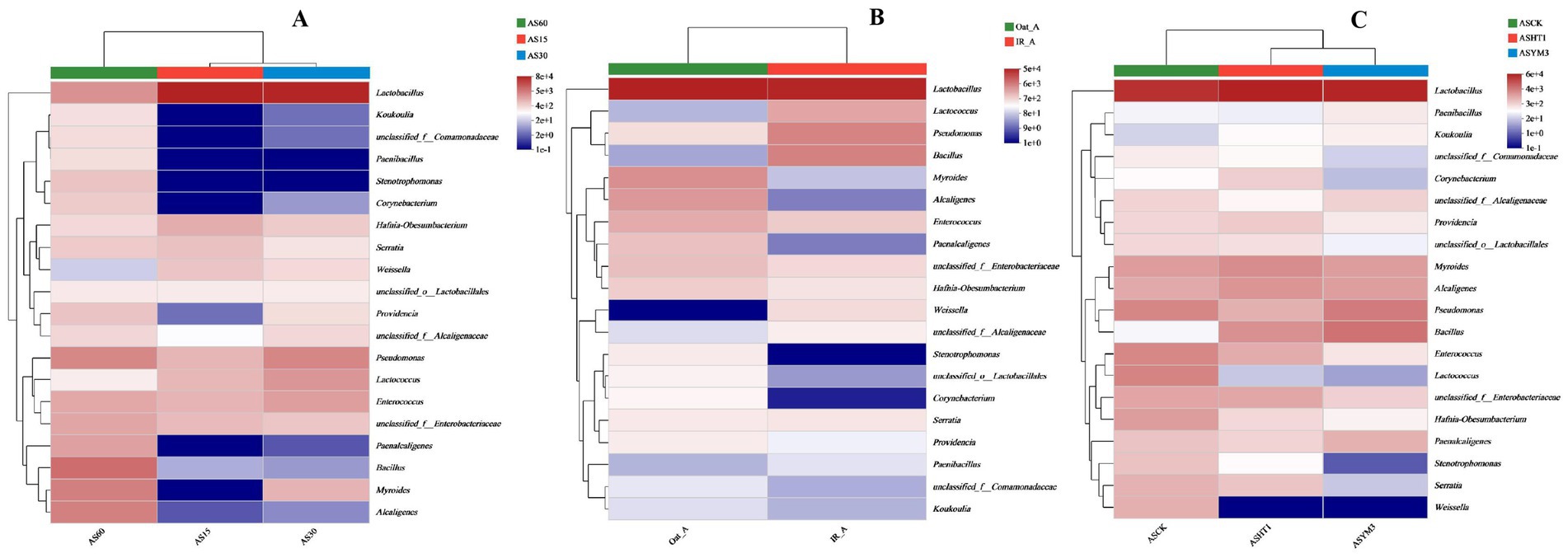
Figure 2. Community heatmap of silage bacterial genus under different ensiling period (A), crop types (B), and additives (C).
At different ensiling durations, the relative abundance of Lactobacillus in the AS15 and AS30 treatments was significantly higher than that in the AS60 treatment (p < 0.05). In contrast, the relative abundances of various bacteria, including Bacillus, Myroides, Alcaligenes, Paenalcaligenes, Stenotrophomonas, Providencia, and Corynebacterium in the AS60 treatment were all higher than in the AS15 and AS30 treatments (p < 0.05) (Figure 3A). Among the relative abundances of various bacteria, the relative abundances of Bacillus, Pseudomonas, and Weissella in the IR_A treatment were significantly higher than those in the Oat_A treatment (p < 0.05). In contrast, the relative abundances of Myroides, Enterococcus, Paenalcaligenes, Hafnia-Obesumbacterium, Providencia, Corynebacterium, Brevundimonas, Leucobacter, and Sphingobacterium were significantly lower in the IR_A treatment than in the Oat_A treatment (p < 0.05) (Figure 3B). The additive significantly affected the relative abundances of bacteria, such as Enterococcus, Lactococcus, Hafnia-Obesumbacterium, and Weissella (p < 0.05). Compared to the ASHT1 and ASYM3 treatments, the relative abundance of these bacteria in the ASCK treatment was the highest (Figure 3C).
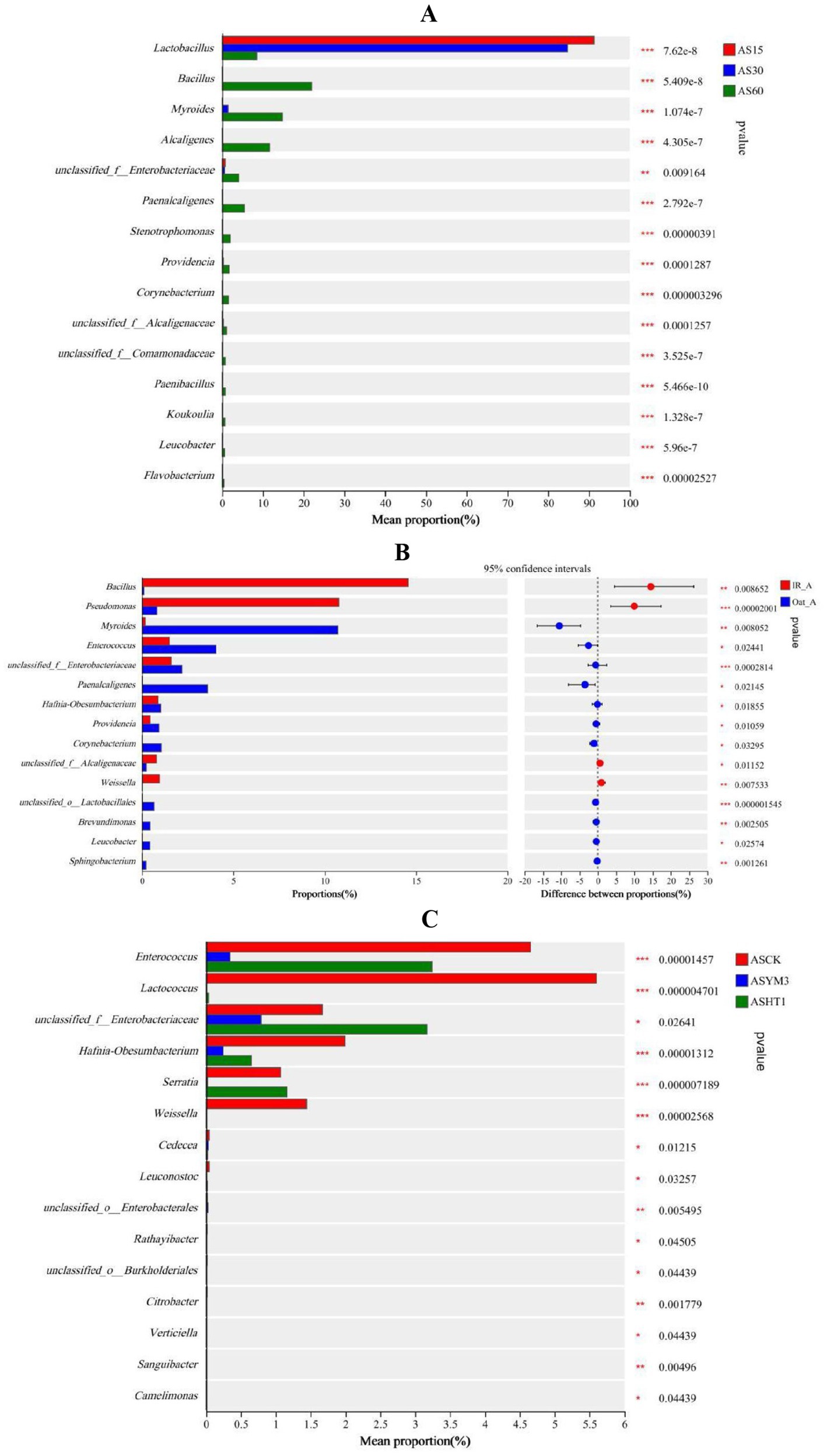
Figure 3. Differences of silage bacterial genus under different ensiling period (A), crop types (B), and additives (C).
The interrelationship between organic acids and bacterial communities
After aerobic exposure of the silage, the acetic acid concentration exhibited a significantly positive correlation with the abundances of Bacillus and Paenibacillus (p < 0.05), whereas it was negatively correlated with the abundances of Lactobacillus and Enterococcus (p < 0.05), as illustrated in Figure 4. Additionally, pH was positively correlated with the abundances of Stenotrophomonas, Corynebacterium, Koukoulia, Paenalcaligenes, Myroides, and Alcaligenes (p < 0.05). Furthermore, lactic acid concentration was positively correlated with Lactobacillus abundance (p < 0.05) but negatively correlated with Bacillus abundance (p < 0.05). Finally, the butyric acid concentration was positively correlated with the abundances of Stenotrophomonas, Myroides, and Alcaligenes (p < 0.05). In the principal component analysis, the first and second principal components exhibited variance contribution rates of 46.01 and 17.02%, respectively (Figure 5). Specifically, the 95% confidence intervals of the AS15 and AS30 treatments showed a high degree of overlap but did not intersect with the confidence interval of the AS60 treatment (p = 0.001) (Figure 5a). In contrast, in the two crops, the 95% confidence intervals of the IR_A and Oat_A silages showed only minimal overlap (p = 0.012) (Figure 5b). However, in the additive treatments, the 95% confidence intervals of the ASCK, ASHT1, and ASYM3 treatments exhibited an extensive overlap (p = 0.066) (Figure 5c).
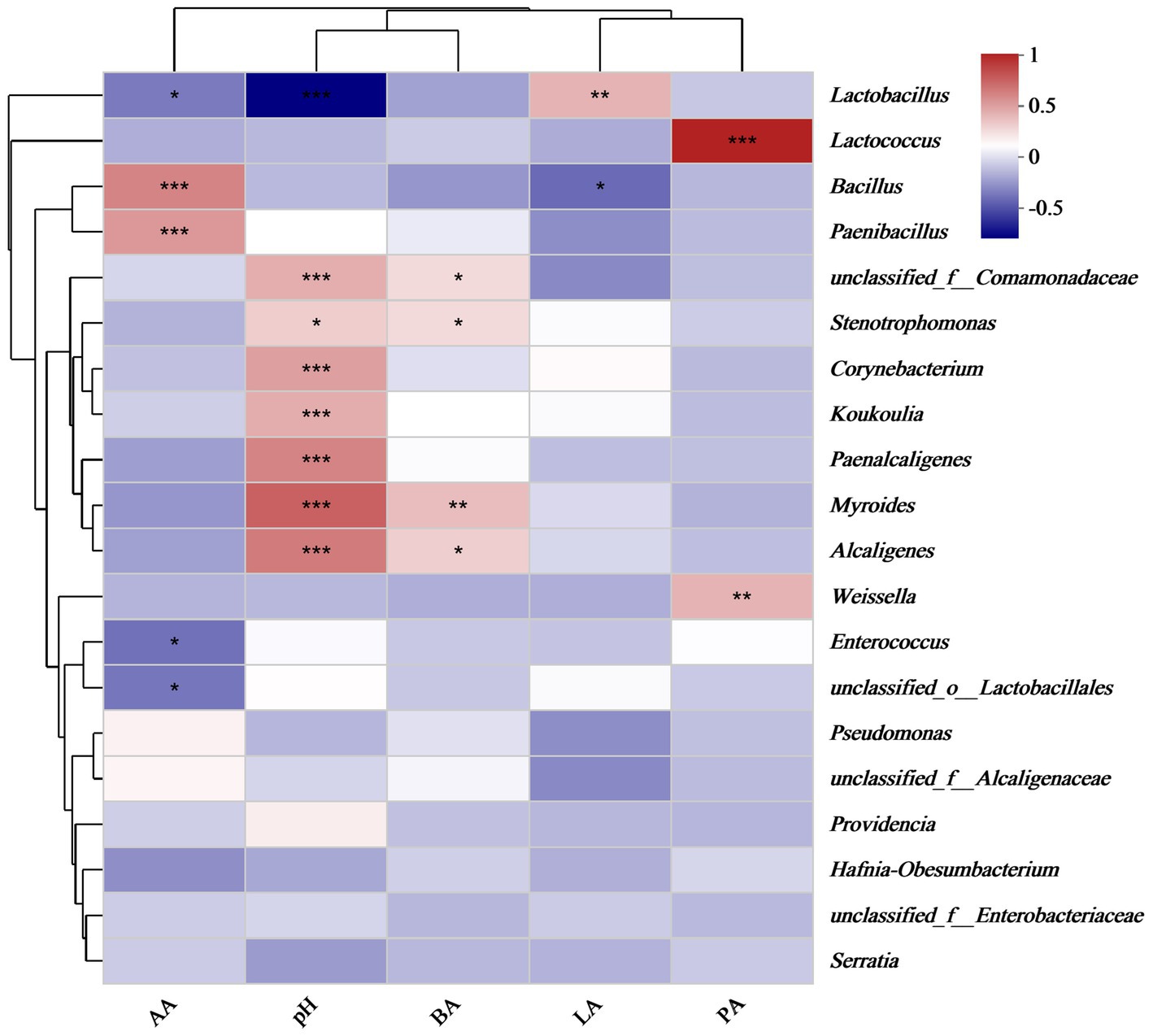
Figure 4. Correlation analysis between organic acids, pH, and bacterial relative abundance. *indicate significant differences at *p < 0.05, **p < 0.01 and ***p < 0.001 respectively.
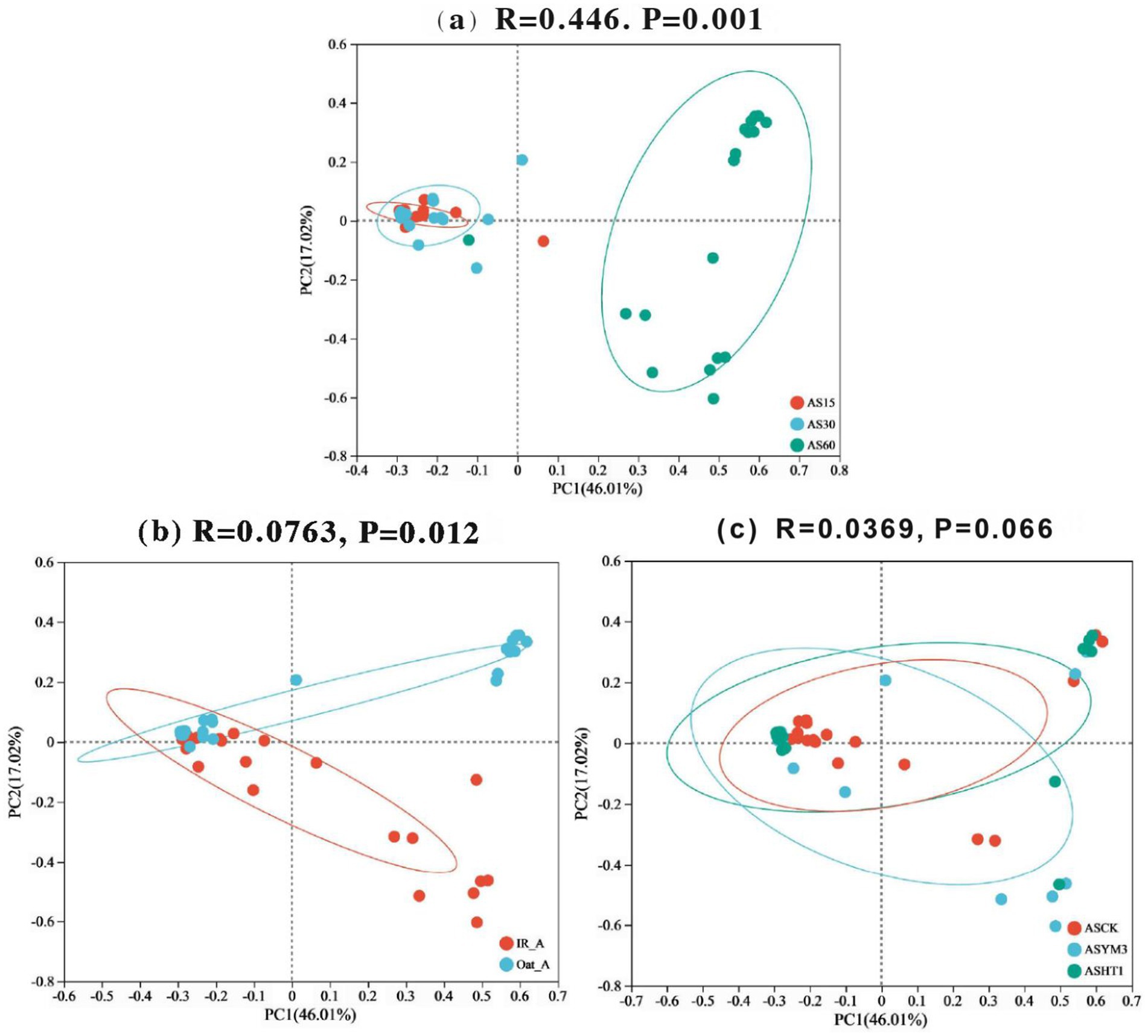
Figure 5. Principal component analysis diagram of bacteria in silage of different ensiling period (a), crop types (b), and additives (c).
Mantel test analysis revealed that the effects of the ensiling period, crop type, and additives on organic acids and pH values exhibited diverse characteristics (Figure 6). However, a significantly negative correlation between propionic and acetic acid concentrations could not be ignored, and a negative correlation was observed between lactic and butyric acid concentrations (p < 0.05). Linear regression analysis of bacterial beta diversity showed that the lactic and acetic acid concentrations, as well as pH, had significant effects on bacterial beta diversity (p < 0.05) (Table 2). The ensiling time, crop type, and additive treatments had significant effects on the normalized shuffle test (NST) index (Figure 7).
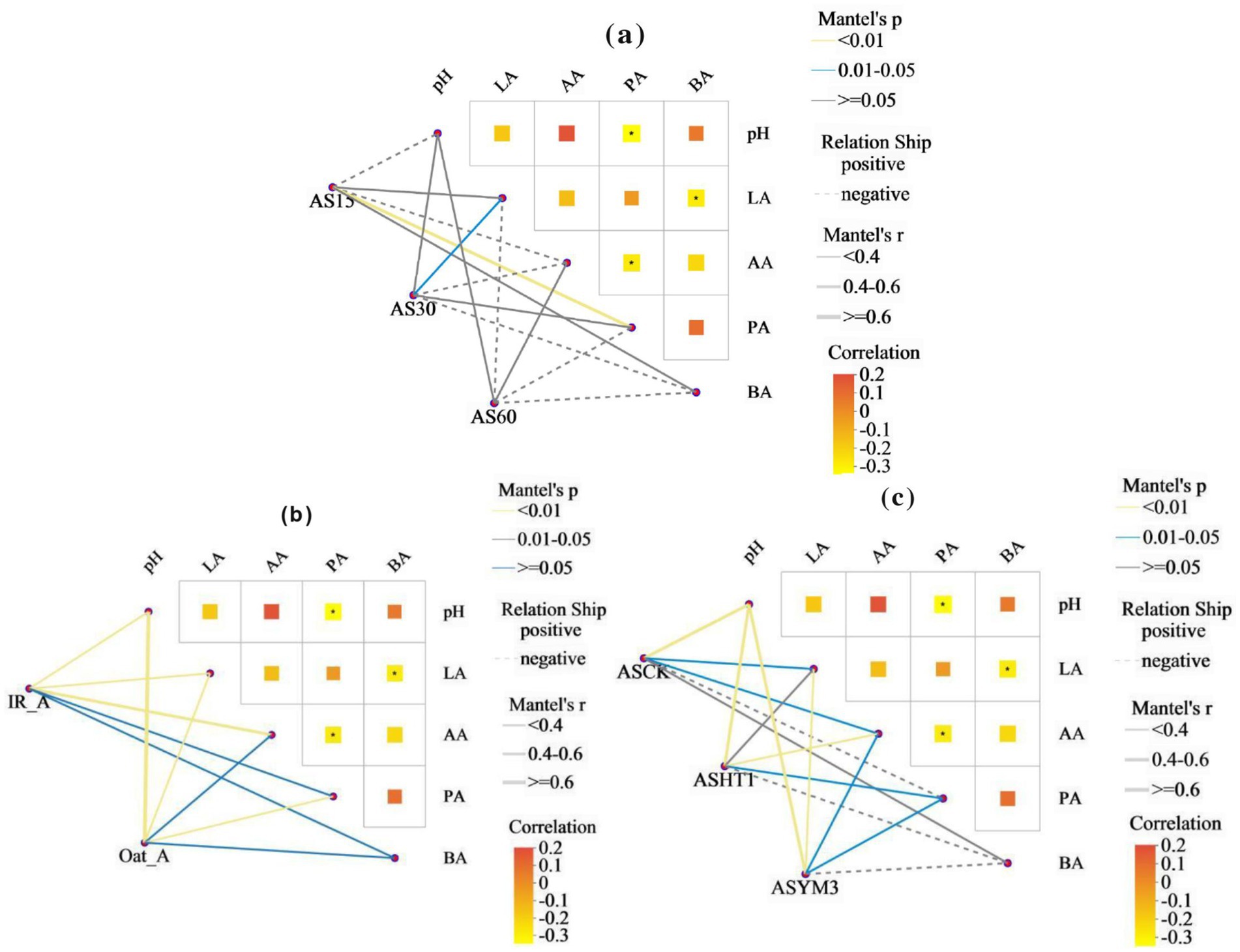
Figure 6. Mantel test heatmap of bacteria in silage of different ensiling period (a), crop types (b), and additives (c).
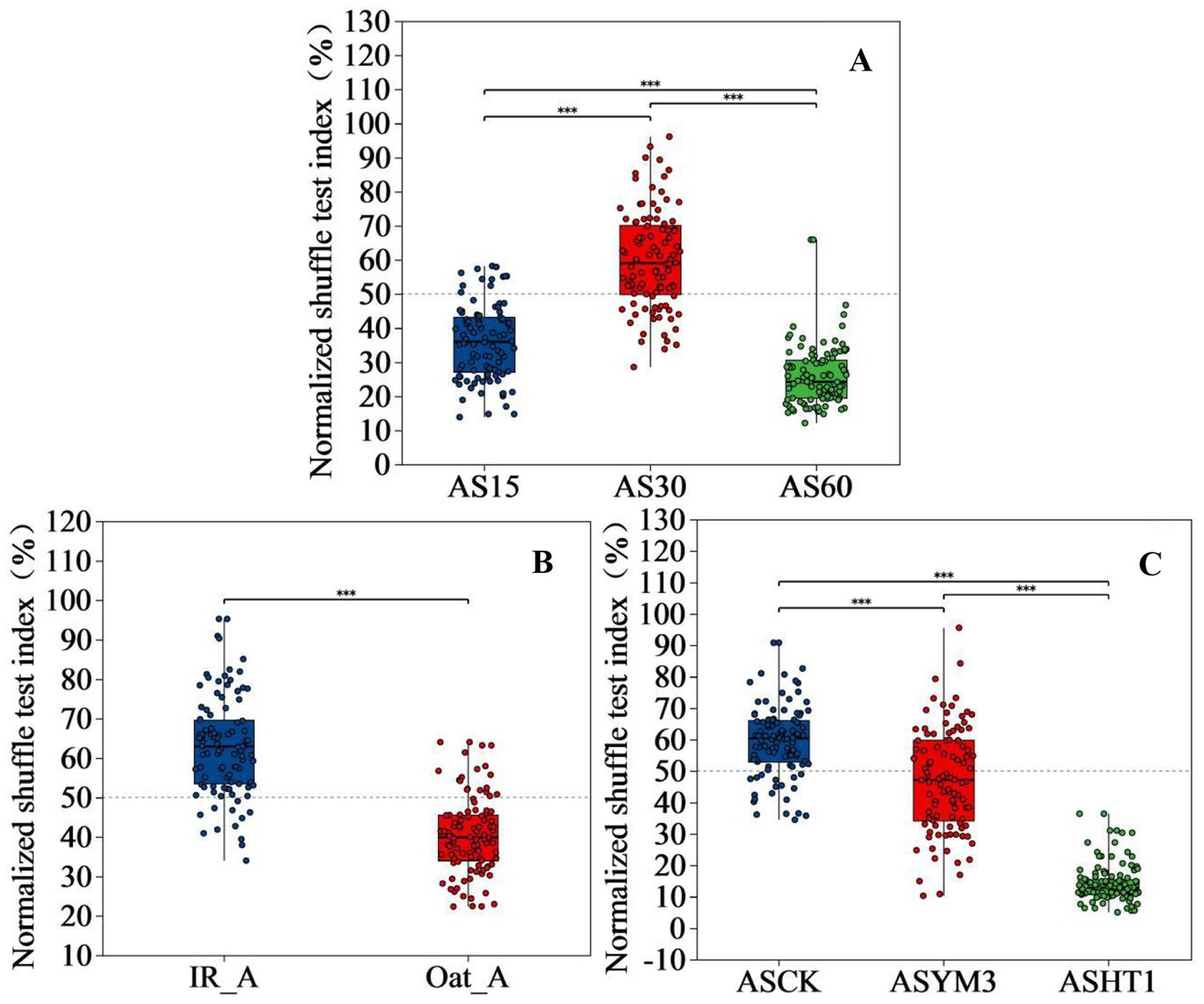
Figure 7. The effect of pesticide concentration on normalized shuffle test index of bacterial communities in silage of different ensiling period (A), crop types (B), and additives (C). *indicate significant differences at *p < 0.05, **p < 0.01 and ***p < 0.001 respectively.
Effects of ensiling time, crop type and additives on unfavorable silage bacteria after aerobic exposure
Among the top 50 bacterial genera, this study identified 10 undesirable species that were closely related to protein degradation in silage, and detrimental to animal and human health (Table 3). For most undesirable bacterial species, such as Stenotrophomonas, Providencia, Paenalcaligenes, Myroides, and Alcaligenes, the relative abundances in the AS60 treatment were generally higher than those in the AS30 and AS15 treatments. Among these harmful bacteria, their relative abundances in the Oat_A treatment were higher than those in the IR_A treatment. However, it is noteworthy that in the IR_A treatment, the relative abundances of Bacillus and Pseudomonas were significantly higher than those in the Oat_A treatment (p < 0.05). For Stenotrophomonas and Providencia, the relative abundances in the ASCK treatment were higher than those in the ASHT1 and ASYM3 treatments. Pseudomonas and Rathayibacter exhibited relatively consistent trends in the additive treatments, with the relative abundances in the ASYM3 treatment being higher than those in the ASCK and ASHT1 treatments. In summary, extending treatment time typically led to an increase in the relative abundance of the most harmful bacteria. In the crop-type treatments, the Oat_A treatment tended to be more conducive to the growth and reproduction of harmful bacteria. The addition of LAB helped to inhibit the increase in the relative abundance of harmful bacteria.
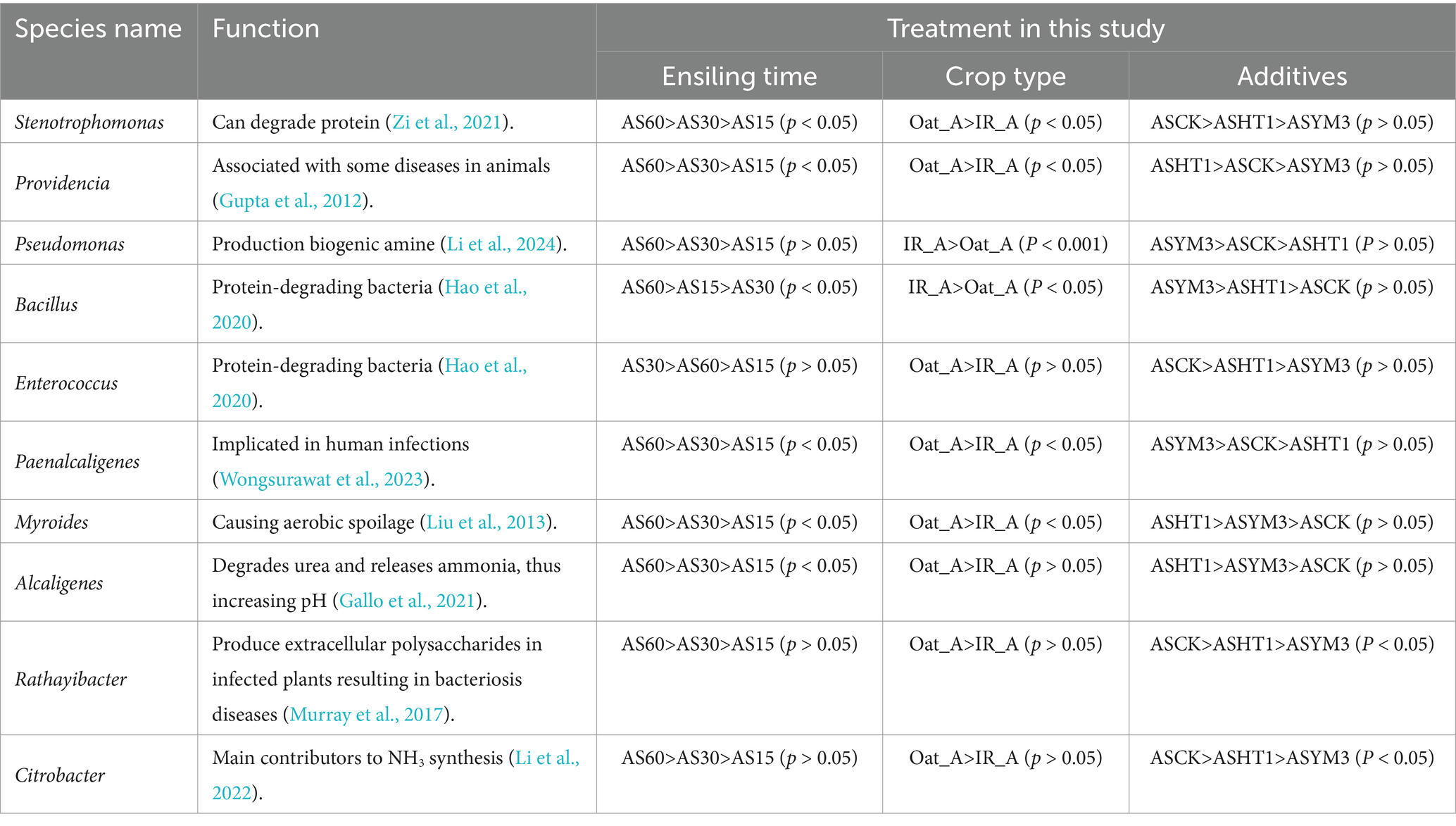
Table 3. The presence of unfavorable bacteria in silage and their specific manifestations in this study.
Discussion
Storing forage infected with leaf spot disease through ensiling not only helps prevent further spread of the disease but also converts forage that may otherwise be discarded due to the disease into valuable feed resources, thereby improving production efficiency and reducing feed costs (Juskiw et al., 2021). Although the silage process aims to create an anaerobic environment to promote LAB fermentation during the actual production, feeding, storage, and transportation processes, silage often encounters the problem of aerobic exposure (Avila and Carvalho, 2020). The primary causes of aerobic exposure included, but were not limited to, improper operations during production, poor feeding and storage management, and vibrations and bumps during transportation. Aerobic exposure increased the oxygen content in silage, thereby promoting the growth and reproduction of aerobic microorganisms such as yeasts and molds (Weiß et al., 2022). These aerobic microorganisms can decompose nutrients, such as lactic acid and the remaining soluble sugar substrates, leading to a continuous rise in pH and temperature (Zhao et al., 2024), ultimately resulting in silage spoilage and deterioration. In this study, regardless of the ensiling period, the difference in crop species, or the variation in additive treatments, even after 7 days of aerobic exposure, the detected LAB counts and their lactic acid production remained higher than the values reported in some published studies (Zhang et al., 2019; Zhang et al., 2023). Simultaneously, the yeast and mold counts, as well as the butyric acid content, remained elevated. These findings suggest that the fermentation quality of silage with leaf spot disease may be affected by complex microbial activity under prolonged aerobic exposure.
After 7 days of aerobic exposure, the LAB count in all silage remained > 7 lg cfu g−1 FM, and further extension of the ensiling period triggered an upward trend of yeast and mold numbers under aerobic exposure. The pH of the silage also increased, which can be attributed to the organic acid metabolic pathway of yeast initiated by silage oxygenation. This pathway effectively reduces the lactic acid concentration and promotes an increase in pH (Chen et al., 2022). The significant decrease in lactic acid content as a direct consequence of extended ensiling duration and aerobic exposure further supports this observation. This phenomenon was verified in previous studies (Kung et al., 2018). During silage fermentation, the microbial community structure undergoes significant changes, with certain microorganisms gradually gaining dominance while others are suppressed (Jatkauskas et al., 2024). This evolution of the community structure had a profound impact on the microbial activity characteristics of silage after aerobic exposure. This study found that in silage fermented for 60 days, Lactobacillus became the dominant species (data not shown) (Xin et al., 2022), however, their dominance rapidly weakened after aerobic exposure. Furthermore, the relative abundances of harmful bacteria, represented by Myroides and Alealigenes, increased sharply, significantly increasing the safety risk of the silage. In contrast, in silage from the AS15 and AS30 treatments, even when exposed to oxygen, the relative abundance of Lactobacillus remained high and dominant. Particularly in the AS15 treatment, the relative abundances of harmful bacteria were much lower than those in the AS60 treatment, indicating a healthier bacterial community structure. This finding suggests that in silage infected with leaf spot disease, shortening the ensiling period helps optimize the silage bacterial community structure after aerobic exposure, reducing the growth potential of harmful bacteria, thereby enhancing the safety and quality of feed. Therefore, reasonable regulation of the ensiling period is the key to improving the bacterial community structure and ensuring silage safety.
This study observed that compared to the IR_A treatment, the Oat_A treatment exhibited higher acetic acid and lower butyric acid concentrations (p < 0.05). Notably, the relative abundances of Bacillus and Pseudomonas in the IR_A treatment were higher than those in the Oat_A treatment, and both bacterial groups showed a significantly positive correlation with the acetic acid concentration. Conversely, the relative abundances of Myroides, Alcaligenes, and Stenotrophomonas in the Oat_A treatment were higher than those in the IR_A treatment, and all demonstrated a significantly positive correlation with the butyric acid concentration. This suggests that different bacteria have distinct mechanisms for the production and regulation of acetic and butyric acids (Kung et al., 2018). Specifically, Bacillus and Pseudomonas may have promoted the production of acetic acid or inhibited the generation of butyric acid (Mumtaz et al., 2019; Chen Y. et al., 2021), leading to higher acetic acid content in the IR_A treatment, whereas Myroides, Alcaligenes, and Stenotrophomonas may possess the ability to enhance butyric acid production or inhibit acetic acid formation, resulting in higher butyric acid content in the Oat_A treatment. In the current study, the relative abundances of Lactobacillus, Lactococcus, Pseudomonas, and Bacillus in the IR_A treatment were dominant, far exceeding those of other species. Compared to the Oat_A treatment, the number of OTUs exhibited a noticeably decreasing trend. However, the NST index of the IR_A treatment was higher than that of the Oat_A treatment (p < 0.001). This phenomenon indicates that despite the relatively concentrated microbial species in the IR_A treatment, microbial system activity was exceptionally vigorous, potentially owing to the enhanced microbial metabolic activity prompted by the high abundances of specific species. Additionally, the higher NST indices revealed that the environmental stability of the IR_A samples was relatively poor, which may have been triggered by microbial interactions or changes in environmental factors. Consequently, we speculated that the characteristics of this microbial community structure may have profoundly influenced certain physiological or biochemical properties of the IR_A treatment, further affecting its overall performance and behavior. Unfortunately, this study did not conduct a more in-depth analysis of silage metabolites, thus failing to verify this speculation.
In adverse environments such as high pH, temperature, and osmotic pressure, Enterococcus typically demonstrated stronger survival, stress resistance, and proliferation capabilities compared to other LAB (Acciarri et al., 2023). Furthermore, during the initial stages of silage fermentation, Enterococcus faecalis, a primary lactic acid bacterium, rapidly produces lactic acid, albeit with relatively weak acid tolerance (Wang et al., 2020). Subsequently, as fermentation progressed, Enterococcus was gradually replaced by the more acid-tolerant Lactobacillus (Parvin et al., 2010). However, when exposed to aerobic conditions, despite its lack of acid tolerance, Enterococcus faecalis’s strong oxygen tolerance allowed it to survive. The results of this study confirm this characteristic of Enterococcus, with higher pH values and lower lactic acid concentrations in the ASCK treatment. Even under aerobic conditions, the effect of LAB additives remained significant. For example, compared to the ASCK treatment, the ASHT1 and ASYM3 treatments effectively inhibited the number of OTUs by increasing the content of lactic and acetic acids, while optimizing microbial competition and environmental stability. Additionally, this study found that the types of bacteria closely related to human health and nutritional loss were generally higher in the ASCK treatment than in the ASHT1 and ASYM3 treatments, indicating that LAB additives effectively improved the health and nutritional value of silage exposed to air. This was independent of the health status of the plant.
Notably, based on the analysis of the NST index, only the additive treatment affected the stability of the silage environment by adjusting the number of OTUs, whereas silage duration and crop type were more likely to influence the stability of the silage environment by regulating the types and quantities of metabolites. For example, the content of l-phenylalanyl-l-proline increases with the number of Enterococcus, whereas the content of glutamylvaline decreases as the number of Enterococci increases (Fu et al., 2022). Bacillus species can grow anaerobically either by using nitrate or nitrite as terminal electron acceptors or through fermentation (Ramos et al., 2000) and are metabolized to produce acetic, formic, and succinic acids (Ouhib-Jacobs et al., 2009). The influence of pH and lactic acid concentration on the relative abundance of bacteria was limited, further demonstrating that changes in acid-tolerant microorganisms are crucial for the silage environment. Microorganisms that are capable of producing acetic, propionic, and butyric acids are of secondary importance.
Conclusion
Forage affected by leaf spot disease can still be used for silage, even when exposed to air for up to 7 days. However, as the ensiling period progressed, the fermentation quality and health of the silage exposed to air gradually decreased. In comparison, the silage fermentation quality and health benefits of Italian ryegrass under aerobic conditions were superior to those of oats. The use of LAB additives can enhance the fermentation quality and health of aerobically exposed silage. Therefore, when silage has to be exposed to air, this study recommends that Italian ryegrass be inoculated with Lactobacillus rhamnosus HT1 and used within 15 days of ensiling.
Data availability statement
This data is available through the National Microbiology Data Center (NMDCR1693659043309: Index of /NMDCSra/public/202309/NMDC40041972/NMDCE1693659043305/NMDCR169365904 3309/).
Author contributions
JY: Data curation, Investigation, Methodology, Writing – review & editing. YM: Investigation, Methodology, Resources, Writing – review & editing. XZ: Data curation, Investigation, Writing – review & editing. MW: Data curation, Investigation, Writing – review & editing. TS: Data curation, Investigation, Writing – review & editing. DW: Conceptualization, Formal analysis, Investigation, Resources, Software, Visualization, Writing – review & editing. LX: Conceptualization, Data curation, Formal analysis, Funding acquisition, Project administration, Resources, Validation, Visualization, Writing – review & editing. GT: Writing – original draft, Writing – review & editing.
Funding
The author(s) declare that financial support was received for the research and/or publication of this article. This research was funded by Yunnan Fundamental Research Projects (202301AU070035), Natural Science Research Project of Education Department of Guizhou Province (QJJ[2024]153), Xingzhao Talent Support Program, Special Basic Cooperative Research Programs of Yunnan Provincial Undergraduate Universities’ Association (202301BA070001-120), and Liupanshui Municipal Key Laboratory for Development and Utilization of Feed Resources (52020-2024-PT-02).
Conflict of interest
The authors declare that the research was conducted in the absence of any commercial or financial relationships that could be construed as a potential conflict of interest.
Generative AI statement
The authors declare that no Gen AI was used in the creation of this manuscript.
Publisher’s note
All claims expressed in this article are solely those of the authors and do not necessarily represent those of their affiliated organizations, or those of the publisher, the editors and the reviewers. Any product that may be evaluated in this article, or claim that may be made by its manufacturer, is not guaranteed or endorsed by the publisher.
References
Acciarri, G., Gizzi, F. O., Torres Manno, M. A., Stulke, J., Espariz, M., Blancato, V. S., et al. (2023). Redundant potassium transporter systems guarantee the survival of Enterococcus faecalis under stress conditions. Front. Microbiol. 14:1117684. doi: 10.3389/fmicb.2023.1117684
Avila, C. L. S., and Carvalho, B. F. (2020). Silage fermentation-updates focusing on the performance of micro-organisms. J. Appl. Microbiol. 128, 966–984. doi: 10.1111/jam.14450
Bolsen, K. K. (2018). Silage review: safety considerations during silage making and feeding. J. Dairy Sci. 101, 4122–4131. doi: 10.3168/jds.2017-13738
Chen, X., Dong, Z., and Zhang, J. (2021). Spraying sugars, growth temperatures and N application levels change epiphytic lactic acid bacteria composition on Italian ryegrass. Grassl. Sci. 68, 145–154. doi: 10.1111/grs.12350
Chen, R., Li, M., Yang, J., Chen, L., Zi, X., Zhou, H., et al. (2022). Exploring the effect of wilting on fermentation profiles and microbial community structure during ensiling and air exposure of king grass silage. Front Microbiol 13:971426. doi: 10.3389/fmicb.2022.971426
Chen, Y., Yin, Y., and Wang, J. (2021). Influence of butyrate on fermentative hydrogen production and microbial community analysis. Int. J. Hydrog. Energy 46, 26825–26833. doi: 10.1016/j.ijhydene.2021.05.185
Fu, Z., Sun, L., Hou, M., Hao, J., Lu, Q., Liu, T., et al. (2022). Effects of different harvest frequencies on microbial community and metabolomic properties of annual ryegrass silage. Front Microbiol 13:971449. doi: 10.3389/fmicb.2022.971449
Gallo, A., Ghilardelli, F., Atzori, A. S., Zara, S., Novak, B., Faas, J., et al. (2021). Co-occurrence of regulated and emerging mycotoxins in corn silage: relationships with fermentation quality and bacterial communities. Toxins 13:232. doi: 10.3390/toxins13030232
Gao, R., Wang, B., Jia, T., Luo, Y., and Yu, Z. (2021). Effects of different carbohydrate sources on alfalfa silage quality at different ensiling days. Agriculture 11:58. doi: 10.3390/agriculture11010058
Gupta, A. K., Nayduch, D., Verma, P., Shah, B., Ghate, H. V., Patole, M. S., et al. (2012). Phylogenetic characterization of bacteria in the gut of house flies (Musca domestica L.). FEMS Microbiol. Ecol. 79, 581–593. doi: 10.1111/j.1574-6941.2011.01248.x
Hao, W., Tian, P., Zheng, M., Wang, H., and Xu, C. (2020). Characteristics of proteolytic microorganisms and their effects on proteolysis in total mixed ration silages of soybean curd residue. Asian Australas. J. Anim. Sci. 33, 100–110. doi: 10.5713/ajas.18.0933
Huang, Y., Liang, L., Dai, S., Wu, C., Chen, C., and Hao, J. (2021). Effect of different regions and ensiling periods on fermentation quality and the bacterial community of whole-plant maize silage. Front. Microbiol. 12:743695. doi: 10.3389/fmicb.2021.743695
Jatkauskas, J., Vrotniakiene, V., Amaral, R. C. D., Witt, K. L., and Cappellozza, B. L. (2024). Influence of ensiling timing and inoculation on whole plant maize silage fermentation and aerobic stability (preliminary research). Plan. Theory 13:2894. doi: 10.3390/plants13202894
Jiang, F. G., Cheng, H. J., Liu, D., Wei, C., An, W. J., Wang, Y. F., et al. (2020). Treatment of whole-plant corn silage with lactic acid bacteria and organic acid enhances quality by elevating acid content, reducing pH, and inhibiting undesirable microorganisms. Front. Microbiol. 11:593088. doi: 10.3389/fmicb.2020.593088
Juskiw, P. E., Kabeta, Y., Nyachiro, J. M., Duggan, T., Oatway, L., Oro, M., et al. (2021). Registration of ‘AB wrangler’, a two-rowed feed and forage barley with enhanced resistance to Fusarium head blight. J Plant Registr 15, 223–235. doi: 10.1002/plr2.20091
Kung, L., Shaver, R. D., Grant, R. J., and Schmidt, R. J. (2018). Silage review: interpretation of chemical, microbial, and organoleptic components of silages. J. Dairy Sci. 101, 4020–4033. doi: 10.3168/jds.2017-13909
Lan, Y., Zhou, W., Duan, T., Li, Y., Matthew, C., and Nan, Z. (2024). Alfalfa spring black stem and leaf spot disease caused by Phoma medicaginis: epidemic occurrence and impacts. Microorganisms 12:1279. doi: 10.3390/microorganisms12071279
Li, R., Jiang, D., Zheng, M., Tian, P., Zheng, M., and Xu, C. (2020). Microbial community dynamics during alfalfa silage with or without clostridial fermentation. Sci. Rep. 10:17782. doi: 10.1038/s41598-020-74958-1
Li, C. J., Xu, Z. H., Dong, Z. X., Shi, S. L., and Zhang, J. G. (2016). Effects of nitrogen application rate on the yields, nutritive value and silage fermentation quality of whole-crop wheat. Asian Australas. J. Anim. Sci. 29, 1129–1135. doi: 10.5713/ajas.15.0737
Li, R., Zheng, M., Zheng, M., Cai, R., Cui, X., Wang, Y., et al. (2022). Metagenomic analysis reveals the linkages between bacteria and the functional enzymes responsible for potential ammonia and biogenic amine production in alfalfa silage. J. Appl. Microbiol. 132, 2594–2604. doi: 10.1111/jam.15411
Li, M., Zi, X., Sun, R., Ou, W., Chen, S., Hou, G., et al. (2024). Co-ensiling whole-plant cassava with corn stalk for excellent silage production: fermentation characteristics, bacterial community, function profile, and microbial ecological network features. Agronomy 14:501. doi: 10.3390/agronomy14030501
Little, M. W., O’Connell, N. E., Welsh, M. D., Mulligan, F. J., and Ferris, C. P. (2017). Concentrate supplementation of a diet based on medium-quality grass silage for 4 weeks prepartum: effects on cow performance, health, metabolic status, and immune function. J. Dairy Sci. 100, 4457–4474. doi: 10.3168/jds.2016-11806
Liu, Q. H., Shao, T., and Zhang, J. G. (2013). Determination of aerobic deterioration of corn stalk silage caused by aerobic bacteria. Anim. Feed Sci. Technol. 183, 124–131. doi: 10.1016/j.anifeedsci.2013.05.012
Mumtaz, M. Z., Barry, K. M., Baker, A. L., Nichols, D. S., Ahmad, M., Zahir, Z. A., et al. (2019). Production of lactic and acetic acids by Bacillus sp. ZM20 and Bacillus cereus following exposure to zinc oxide: a possible mechanism for Zn solubilization. Rhizosphere 12:100170. doi: 10.1016/j.rhisph.2019.100170
Murray, T. D., Schroeder, B. K., Schneider, W. L., Luster, D. G., Sechler, A., Rogers, E. E., et al. (2017). Rathayibacter toxicus, other Rathayibacter species inducing bacterial head blight of grasses, and the potential for livestock poisonings. Phytopathology 107, 804–815. doi: 10.1094/PHYTO-02-17-0047-RVW
Okoye, C. O., Wu, Y., Wang, Y., Gao, L., Li, X., and Jiang, J. (2023). Fermentation profile, aerobic stability, and microbial community dynamics of corn straw ensiled with Lactobacillus buchneri PC-C1 and Lactobacillus plantarum PC1-1. Microbiol. Res. 270:127329. doi: 10.1016/j.micres.2023.127329
Ouhib-Jacobs, O., Lindley, N. D., Schmitt, P., and Clavel, T. (2009). Fructose and glucose mediates enterotoxin production and anaerobic metabolism of Bacillus cereus ATCC14579T. J. Appl. Microbiol. 107, 821–829. doi: 10.1111/j.1365-2672.2009.04254.x
Parvin, S., Wang, C., Li, Y., and Nishino, N. (2010). Effects of inoculation with lactic acid bacteria on the bacterial communities of Italian ryegrass, whole crop maize, guinea grass and Rhodes grass silages. Anim. Feed Sci. Technol. 160, 160–166. doi: 10.1016/j.anifeedsci.2010.07.010
Ramos, H. C., Hoffmann, T., Marino, M., Nedjari, H., Presecan-Siedel, E., Dreesen, O., et al. (2000). Fermentative metabolism of Bacillus subtilis: physiology and regulation of gene expression. J. Bacteriol. 182, 3072–3080. doi: 10.1128/jb.182.11.3072-3080.2000
Tang, G., Fan, Y., Li, X., Tian, R., Tang, R., Xu, L., et al. (2023). Effects of leaf properties on the counts of microbes on the leaf surfaces of wheat, rye and triticale. FEMS Microbiol. Ecol. 99, 1–10. doi: 10.1093/femsec/fiad024
Victoria Arellano, A. D., Furtado, E. B., Holz, T. M., Pazdiora, P. C., da Silva, G. M., Del Ponte, E. M., et al. (2022). Silicon, biological seed treatment and cutting reduce the intensity of leaf spot diseases affecting Lolium multiflorum. Plant Pathol. 71, 1056–1065. doi: 10.1111/ppa.13553
Wang, B., Gao, R., Wu, Z., and Yu, Z. (2020). Functional analysis of sugars in modulating bacterial communities and metabolomics profiles of medicago sativa silage. Front. Microbiol. 11:641. doi: 10.3389/fmicb.2020.00641
Weiß, K., Kroschewski, B., and Auerbach, H. U. (2022). The influence of delayed sealing and repeated air ingress during the storage of maize slage on fermentation patterns, yeast development and aerobic stability. Fermentation 8:48. doi: 10.3390/fermentation8020048
Wongsurawat, T., Sutheeworapong, S., Jenjaroenpun, P., Charoensiddhi, S., Khoiri, A. N., Topanurak, S., et al. (2023). Microbiome analysis of thai traditional fermented soybeans reveals short-chain fatty acid-associated bacterial taxa. Sci. Rep. 13:7573. doi: 10.1038/s41598-023-34818-0
Xin, Y., Chen, C., Zhong, Y., Bu, X., Huang, S., Tahir, M., et al. (2022). Effect of storage time on the silage quality and microbial community of mixed maize and faba bean in the Qinghai-Tibet plateau. Front Microbiol 13:1090401. doi: 10.3389/fmicb.2022.1090401
Yin, X., Tian, J., and Zhang, J. (2021). Effects of re-ensiling on the fermentation quality and microbial community of napier grass (Pennisetum purpureum) silage. J. Sci. Food Agric. 101, 5028–5037. doi: 10.1002/jsfa.11147
Zhang, J., Liu, Y., Wang, Z., Bao, J., Zhao, M., Si, Q., et al. (2023). Effects of different types of LAB on dynamic fermentation quality and microbial community of native grass silage during anaerobic fermentation and aerobic exposure. Microorganisms 11:513. doi: 10.3390/microorganisms11020513
Zhang, L., Zhou, X., Gu, Q., Liang, M., Mu, S., Zhou, B., et al. (2019). Analysis of the correlation between bacteria and fungi in sugarcane tops silage prior to and after aerobic exposure. Bioresour. Technol. 291:121835. doi: 10.1016/j.biortech.2019.121835
Zhao, X., Sun, Y., Chang, Z., Yao, B., Han, Z., Wang, T., et al. (2024). Innovative lactic acid production techniques driving advances in silage fermentation. Fermentation 10:533. doi: 10.3390/fermentation10100533
Keywords: aerobic exposure, leaf spot disease, silage fermentation quality, aerobic stability, ensiling period, additives
Citation: Tang G, Yang J, Meng Y, Zhang X, Wen M, Sun T, Wu D and Xu L (2025) Effects of ensiling period and lactic acid bacteria inoculation on aerobic stability of silage in forage infected with leaf spot disease. Front. Microbiol. 16:1548678. doi: 10.3389/fmicb.2025.1548678
Edited by:
Aldo Corsetti, University of Teramo, ItalyReviewed by:
Ping Li, Guizhou University, ChinaCláudia Braga Pereira Bento, Universidade Federal dos Vales do Jequitinhonha e Mucuri, Brazil
Copyright © 2025 Tang, Yang, Meng, Zhang, Wen, Sun, Wu and Xu. This is an open-access article distributed under the terms of the Creative Commons Attribution License (CC BY). The use, distribution or reproduction in other forums is permitted, provided the original author(s) and the copyright owner(s) are credited and that the original publication in this journal is cited, in accordance with accepted academic practice. No use, distribution or reproduction is permitted which does not comply with these terms.
*Correspondence: Dan Wu, ODM0NDg3MjkxQHFxLmNvbQ==; Liuxing Xu, MjAyMTAwMDZAenR1LmVkdS5jbg==