- 1Research Center for Plant Functional Components, Tobacco Research Institute of Chinese Academy of Agricultural Sciences, Qingdao, China
- 2Technological Center, China Tobacco Zhejiang Industrial Co., Ltd., Hangzhou, China
Endophytic fungi belonging to the Aspergillus genus have received substantial attention due to their notable secondary metabolic potential. In this study, chemical investigations using LC–MS/MS-based molecular networking on the endophytic fungus Aspergillus japonicus TE-739D led to the discovery of two new cyclohexadepsipeptides, namely japonamides C (1) and D (2), along with three known cyclodipeptides (3–5). Their structures, including the absolute configurations of the amino acid residues, were elucidated through spectroscopic data analysis and an optimized Marfey’s method. The newly discovered compounds, japonamides C (1) and D (2), were screened for broad-spectrum cell proliferation inhibitory activity against 20 different human cell lines. The results indicated that both compounds displayed broad-spectrum antiproliferative activity against MKN-45, HCT116, TE-1, 5,637, CAL-62, and A-637 cells, with inhibition rates ranging from 55.0 to 72.3%. Moreover, the antibacterial activity of compounds 1–5 against two Gram-positive bacteria and two Gram-negative bacteria was also evaluated.
1 Introduction
Filamentous fungi belonging to the Aspergillus genus are widely distributed across both terrestrial and marine environments (Sanchez et al., 2012). Numerous species of Aspergillus have demonstrated the ability to produce a diverse array of secondary metabolites, including polyketides, sterols, fatty acids, peptides, alkaloids, terpenoids, and other miscellaneous compounds. These metabolites posses a wide range of biological activities, such as antimicrobial, cytotoxic, anti-inflammatory, herbicidal, and antioxidant properties (El-Hawary et al., 2020; Orfali et al., 2021). For instance, A. ustus has been identified as a source of three rearranged ergostane-type aspersteroids A–C, which exhibit significant immunosuppressive and antimicrobial activities (Liu et al., 2021). Moreover, the compound 12β,25,28-trihydroxyergone isolated from A. terreus has been shown to possess promising cytotoxic effects against the human colon cancer SW620 cell line and five human leukemia cell lines, with IC50 values ranging from 5.7 to 8.9 μM (Zhang et al., 2024). These results highlight the genus Aspergillus as a crucial source of novel secondary metabolites with biomedical potential.
Cyclic peptides are polypeptide chains composed of both canonical and non-canonical amino acids that are linked at distant positions to form macrocyclic structures. Current reports indicate that more than 40 cyclic peptide drugs are utilized in clinical settings, the majority of which are derived from natural products (NPs) or their derivatives (Zorzi et al., 2017; Zhang et al., 2021). For instance, commercial vancomycin, a heptapeptide composed of seven amino acids, exhibits strong antimicrobial efficacy against Gram-positive bacteria and is employed in the treatment of severe infections caused by methicillin-resistant Staphylococcus aureus (Walsh, 1999). In addition, cyclosporin A, which is a cyclic undecapeptide discovered in the 1970s, is recognized as a potent immunosuppressive agent (Ruegger et al., 1976; Ono et al., 2021). The significant biological activities and potential therapeutic applications of cyclic peptides have garnered considerable interest from both researchers and pharmaceutical companies. Consequently, there is an urgent need to identify new cyclic peptides with promising medicinal properties.
Traditional approaches for discovering novel cyclic peptides often result in a high rate of rediscovering known cyclic peptides. Global Natural Products Social Molecular Networking (GNPS; http://gnps.ucsd.edu) is an open-access knowledge base for community-wide organization and sharing of raw, processed, or annotated fragmentation mass spectrometry (MS/MS) data. It is widely utilized in the search for novel natural products (Wang et al., 2016; Zou et al., 2023; Cui et al., 2022). In our ongoing search for new bioactive metabolites from endophytic fungi (Gu et al., 2023; Gu et al., 2024a; Wang et al., 2025), we employed LC–MS/MS-based molecular networking for the rapid discovery of novel natural products (NPs) from the Nicotiana tabacum-derived endophytic fungus A. japonicus TE-739D. As a result, two new cyclohexadepsipeptides, japonamides C (1) and D (2), along with three known cyclodipeptides (3–5), were isolated and characterized (Figure 1). In addition, some of these compounds were evaluated for their antiproliferative and antibacterial activities.
2 Materials and methods
2.1 General experimental procedures
UV spectra were obtained using a Techcomp UV2310II spectrophotometer (Techcomp, Ltd., Shanghai, China). Specific rotations were recorded on a Rudolph Autopol IV automatic polarimeter (Rudolph Research Analytical, NJ, USA). Circular dichroism (CD) spectra were recorded on a JASCO J-1500 CD spectrometer (JASCO Corp., Tokyo, Japan). High-resolution electrospray ionization mass spectrometry (HRESIMS) spectra were recorded on a Micromass Q-TOF spectrometer (Waters, Milford, MA). 1H, 13C, and 2D NMR (HSQC, HMBC, 1H-1H COSY, NOESY) spectra were measured on a JNM-ECZ600R/S1 600 MHz NMR spectrometer (JEOL Ltd., Tokyo, Japan) and an Agilent DD2 500 MHz NMR spectrometer (Agilent Technologies, Santa Clara, CA). Chemical shifts were expressed in δ (ppm) relative to the solvent residual peaks at δH 2.50 and δC 39.5 for DMSO-d6, with coupling constants (J) reported in hertz (Hz). Column chromatography (CC) was performed using silica gel (100–200 mesh; Qing Dao Hai Yang Chemical Group Co., Qingdao, China), Sephadex LH-20 (GE Healthcare, Pittsburgh, PA), and reversed-phase (RP)-18 gel (40–60 μm, Merck, Darmstadt, Germany). Semipreparative HPLC separation was carried out on an NS4201 instrument (Hanbon Sci.& Tech., Jiangsu, China), equipped with an NP7000 SERIALS pump (flow rate: 3 ml/min) and an NU3000 SERIALS UV detector, using a SunFire-C18 column (150 mm × 10 mm i.d., 5 μm, Waters, Milford, MA).
2.2 Fungal source
The fungal strain A. japonicus TE-739D was isolated in 2018 from the leaves of cultivated tobacco (N. tabacum L.) grown in Enshi, Hubei Province (People’s Republic of China). Based on the phylogenetic analyses of the 28S and internal transcribed spacer (ITS) rDNA regions, this fungus was identified as A. japonicus, which has been deposited in GenBank (NCBI) under number PP126510. The strain was also deposited in the China General Microbiological Culture Collection Center (CGMCC No. 40901).
2.3 Fermentation, extraction, and isolation
The endophytic fungus A. japonicus TE-739D was statically cultivated in 290 × 1 L Erlenmeyer flasks containing 400 ml of potato dextrose water medium at 28°C for 30 days. Then, the fermentation product was extracted with ethyl acetate (EtOAc), and the crude extract (68.8 g) was obtained by evaporation under vacuum at 40°C. The extract was eluted using column chromatography on silica gel with stepwise petroleum ether (PE)–EtOAc mixtures (100:0, 90:10, 80:20, 70:30, 50:50, 30:70, and 0:100, v/v) to yield fractions Fr.1–Fr.6. Fr.6 was subjected to ODS CC with a MeOH–H2O gradient, (30:70–100:0, v/v) yielding seven fractions (Fr.6.1–Fr.6.7). Fr.6.6 was separated using Sephadex LH-20 CC (CH2Cl2-MeOH) to yield three subfractions (Fr.6.6.1–Fr.6.6.3). Compounds 1 (6.2 mg, tR 32.042 min) and 2 (9.5 mg, tR 34.542 min) were obtained from Fr.6.6.1 using semipreparative HPLC with MeCN–H2O (40:60, v/v). Compounds 3 (16.3 mg, tR 11.045 min) and 4 (2.4 mg, tR 13.345 min) were obtained from Fr.6.3 using semipreparative HPLC with MeCN–H2O (17:83, v/v). Similarly, compound 5 (6.2 mg, tR 14.188 min) was obtained from Fr.6.3 using semipreparative HPLC with MeCN–H2O (22:78, v/v).
2.4 Spectral data of the isolated compounds
Japonamide C (1): Pale yellow powder; [α]24D–9.0 (c 0.30, MeOH); UV (MeOH) λmax 200, 226, 275 nm; 1H NMR (DMSO-d6, 500 MHz), 13C NMR (DMSO-d6, 125 MHz) (see Table 1); HRESIMS m/z 789.4180 [M + H]+ (calcd. For C42H57N6O9, 789.4182).
Japonamide D (2): Pale yellow powder; [α]24D + 106.7 (c 0.24, MeOH); UV (MeOH) λmax 200, 228, 280 nm; 1H NMR (DMSO-d6, 500 MHz), 13C NMR (DMSO-d6, 125 MHz) (see Table 1); HRESIMS m/z 819.4294 [M + H]+ (calcd. For C43H59N6O10, 819.4287).
2.5 Absolute configurations of amino acids using advanced Marfey’s analysis
Optimized Marfey’s analyses were carried out as previously reported (Wang et al., 2022). Compounds 1 and 2 (1.0 mg) were dissolved in 6 M HCl (1.0 ml) and heated at 100°C for 24 h. The solutions were then evaporated to dryness, transferred to a 4-ml reaction vial, and treated with a 10-mg/ml solution of 1-fluoro-2-4-dinitrophenyl-5-L-alanine amide (FDAA, 200 μl) in acetone, followed by 1.0 M NaHCO3 (40 μl). The reaction mixtures were heated at 45°C for 90 min, and the reactions were quenched by the addition of HCl (1 M, 40 μl). Similarly, standard L-and D-amino acids (Pro, N-Me-O-Me-Tyr, Phe, Thr, Ile, O-Me-Tyr) were derivatized separately. The derivatives of the acid hydrolysate and the standard amino acids were analyzed using HPLC (ACQUITY C18 column; 1.7 μm, 2.1 × 100 mm; 0.3 ml/min; UV detection at 340 nm), with a linear gradient of acetonitrile (30–45%) in water (TFA, 0.01%) for 20 min. Retention times for the authentic standards were as follows: L-Thr (1.85 min), D-Thr (2.35 min), L-Pro (2.88 min), D-Pro (3.25 min), FDAA (3.54 min), L-Ile (6.77 min), N-Me-O-Me-L-Tyr (6.91 min), O-Me-L-Tyr (7.15 min), L-Phe (7.24 min), O-Me-D-Tyr (9.70 min), D-Phe (10.03 min), and D-Ile (10.48 min). The absolute configurations of the chiral amino acids in compounds 1 and 2 were determined by comparing the retention times.
2.6 Cell proliferation inhibitory activity
The antiproliferative activity of the compounds was tested against 18 human cancer cell lines—the lung cancer cell line A549, stomach cancer cell line MKN-45, colon cancer cell line HCT 116, cervical cancer cell line HeLa, chronic myelogenous leukemia cell line lK-562, renal clear cell adenocarcinoma cell line 786-O, esophageal cancer cell line TE-1, bladder cancer cell line 5,637, gallbladder cancer cell line GBC-SD, breast cancer cell line MCF7, liver cancer cell line HepG2, brain tumor cell line SF126, prostate cancer cell line DU145, thyroid cancer cell line CAL-62, pancreatic cancer cell line PATU8988T, osteosarcoma cell line HOS, malignant melanocyte cell line A-375, and rhabdomyosarcoma cell line A-673. In addition, two non-cancerous cell lines—the human normal liver cell line L-02 and human embryonic kidney cell line 293 T—were also included. The Cell Counting Kit-8 (CCK-8) was used to evaluate the proliferation inhibitory activity of japonamides C (1) and D (2), as described previously (Xu et al., 2014; Wu et al., 2015). Cisplatin was used as a positive control.
2.7 Antibacterial assays
The antibacterial activity of compounds 1–5 was evaluated against two Gram-positive bacteria—Bacillus cereus and B. subtilis—and two Gram-negative bacteria—Ralstonia solanacearum and Xanthomonas oryzae. Streptomycin sulfate was used as a positive control. The minimum inhibitory concentrations (MICs) of each compound were determined using the broth micro-dilution method with certain modifications (Song et al., 2021; Gu et al., 2024b).
2.8 UHPLC-Q-TOF and molecular networking analysis
The extract of the fungus A. japonicus TE-739D was dissolved in MeOH (10 mg/ml) and then analyzed using LC–MS/MS. The mobile phase consisted of 0.1% formic acid in water (A) and 0.1% formic acid in MeCN (B). The elution started with a 5% solvent B isocratic phase for 5 min, followed by a linear gradient from 5 to 95% solvent B over 5 to 35 min and then maintained at 95% solvent B for 5 min. The flow rate was 0.3 ml/min, and the injection volume was 5 μl. The MS survey scan covered a range of 200–2,000 m/z, and the MS/MS scan ranged from 100 to 1,000 m/z. The raw MS/MS data were converted to mzML files using the MSConvert 3.0 software, and the data were then submitted to the GNPS platform (https://gnps.ucsd.edu, accessed on 1 January 2016). The parameters for molecular network generation were set as follows: Precursor ion mass tolerance set to 0.02 Da, fragment ion mass tolerance at 0.02 Da, min pairs cos at 0.6, a minimum of 5 matched fragment ions, maximum shift of 600, a minimum cluster size of 2, and a network TopK value of 10. The generated molecular network was visualized using Cytoscape 3.8.2.
3 Results
3.1 Molecular networking analysis for the discovery of new cyclopeptides from A. japonicas TE-739D
Following the above-mentioned extraction and concentration procedures, a total of 68.8 g of ethyl acetate (EtOAc) extract was obtained from 116 L of the PDB fermentation broth derived from A. japonicus TE-739D. This extract was analyzed using high-resolution tandem mass spectrometry (HR-MS/MS). Subsequently, a molecular network (MN) was constructed utilizing the GNPS platform (Figure 2A). The analysis revealed the presence of more than 13 prominent clusters. One particular cluster within this molecular network comprised precursor ions in the m/z range of 700–900 (Figure 2B), which were highly similar to the cluster reported in the literature (Wang et al., 2022). The MS/MS spectrum of two nodes with protonated ions at m/z 791.400 and 805.415 [M + H]+ matched the cyclohexadepsipeptides japonamide A (Figure 2C) and japonamide B (Figure 2D). The presence of surrounding nodes with similar masses within this cluster suggested that A. japonicus TE-739D may possess unique potential to produce novel cyclohexadepsipeptide analogs.
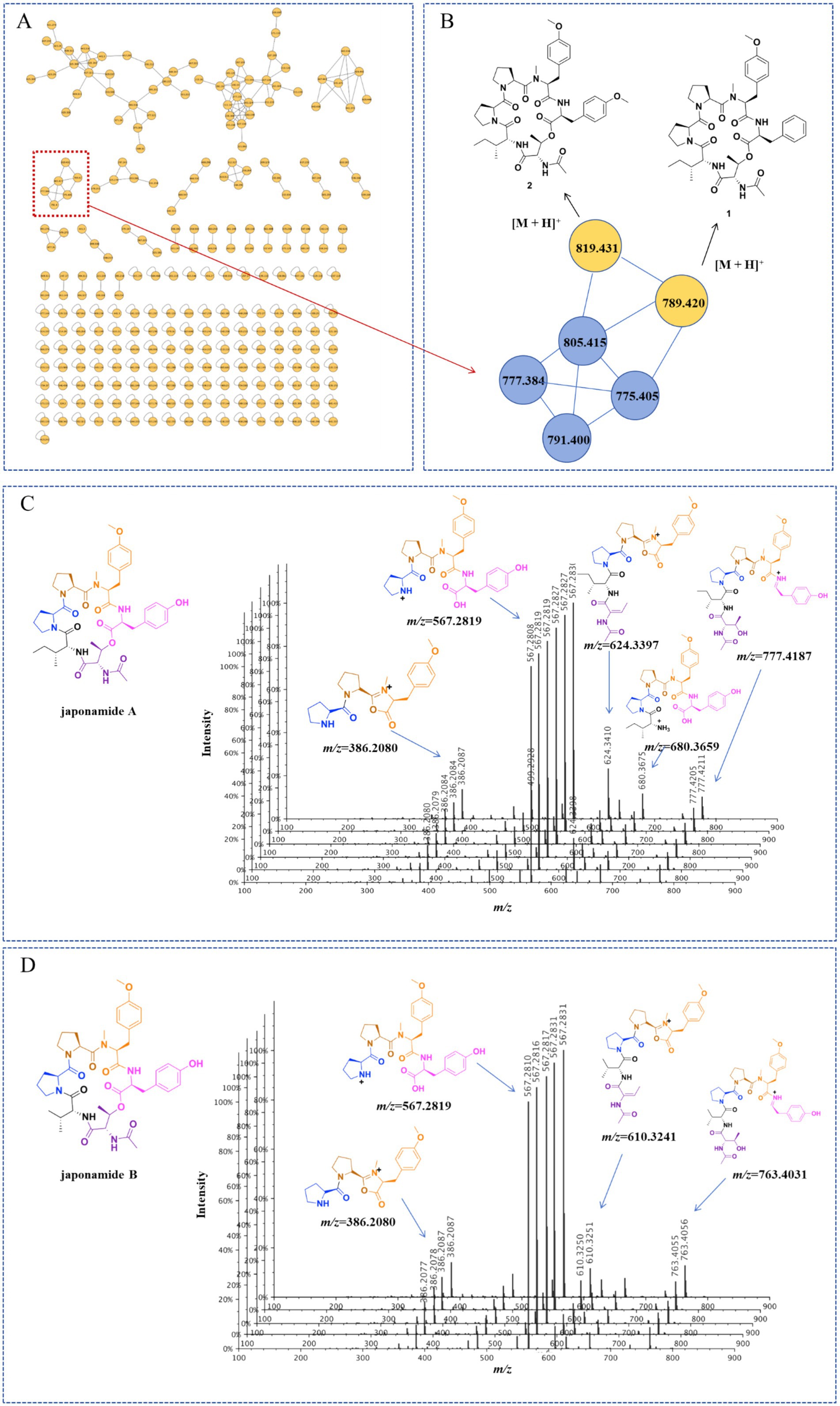
Figure 2. Molecular networking-based prioritization of the EtOAc extract of A. japonicus TE-739D for peptide natural products: (A) complete network; (B) cluster of peptides including compounds 1 and 2; tandem mass spectrometry of protonated ions at m/z 791.400 (C) and 805.415 (D), showing typical amino acid imine ion fragments of japonamides A and B.
3.2 Structure elucidation of the new compounds
Compound 1 was obtained as a pale yellow powder. The HRESIMS data (Supplementary Figure S8) revealed a protonated molecule at m/z 789.4185 (calculated value: 789.4182), which was consistent with the molecular formula C42H56N6O9, implying 18 degrees of unsaturation. The 1H NMR data (Table 1) and HSQC spectrum of compound 1 showed signals for three amide protons [δH 8.70 (1H, d, J = 8.5 Hz), 7.82 (1H, d, J = 9.1 Hz), 7.38 (1H, d, J = 8.9 Hz)], a monosubstituted phenyl group (δH 7.23, 7.23, 7.21, 7.18, and 7.18), a 1,4-disubstituted phenyl group (δH 7.10, 7.10, 6.84, and 6.84), seven oxygenated or nitrogenated methine protons (δH 4.97, 4.80, 4.73, 4.63, 4.42, 4.35, and 4.32), two oxygenated or nitrogenated methylene protons (δH 3.85/3.62, 3.65/3.59), one O-methyl group (δH 3.70), one N-methyl group (δH 2.14), four methyl groups (δH 1.98, 1.22, 0.85, and 0.82), and several aliphatic protons. The 13C NMR data (Table 1), combined with the HSQC spectra, revealed 38 carbon resonance signals, including 7 carbonyl compounds (δC 172.2, 170.5, 170.0, 169.8, 169.7, 169.3, and 167.7), 12 olefinic carbons including four superimposable ones (δC 113.9 and 130.4), 9 oxygenated or nitrogenated carbons (δC 70.0, 61.7, 57.3, 55.4, 54.5, 54.5, 54.4, 47.1, and 46.8), 6 methyl carbons including one methoxy (δC 55.1), and 8 aliphatic carbons (δC 37.0, 36.3, 32.2, 28.1, 27.4, 25.1, 25.0, and 24.0).
Further analysis of the 2D NMR spectra (Supplementary Figures S3–S5) led to the identification of six amino acid components. The first aliphatic amino acid (Pro-1) was confirmed by the 1H–1H COSY correlations of H-2/H2-3/H2-4/H2-5 and the HMBC correlations from H-2 to C-4 (Figure 3). Another proline (Pro-2) residue was constructed with similar resonance signals (Figure 3). An N,O-dimethyl-tyrosine unit (N-Me-O-Me-Tyr) was confirmed by the HMBC correlations from H3-20 to C-11, from H-12 to C-6/C-11/C-20, from H2-13 to C-12/C-15, from H-15 to C-13/C-17, from H-16 to C-14, and from H3-21 to C-17, as well as by the 1H–1H COSY correlations between H-2/H2-3, H-15/H-16, and H-18/H-19 (Figure 3). In addition, a spin system consisting of five adjacent aromatic protons (δH 7.23, 7.23, 7.21, 7.21, and 7.18), recognized in the 1H–1H COSY correlations (Figure 3), was identified as a monosubstituted phenyl group. The HMBC correlations from H-23 to C-22/C-24/C-25 and from H2-24 to C-22/C-23/C-25/C-26 indicated the presence of a phenylalanine unit (Phe). The N-acetyl-threonine residue (N-Ac-Thr) was confirmed by the 1H–1H COSY correlations of H-32/H-33/H3-34 and the HMBC correlations from H-32 to C-31/C-33/C-35, from H-33 to C-31/C-34, from H3-34 to C-32/C-33, and from H3-36 to C-35. The last unit was identified as isoleucine (Ile) by the 1H–1H COSY correlations of H-38/H-39/H2-40/H3-41, H2-40/H3-42, as well as the HMBC correlations from H3-41 to C-38/C-39/C-40 and from H3-42 to C-39/C-40.
The connectivity among the six amino acid residues was determined as Pro-Pro-(N-Me-O-Me-Tyr)-Phe-(N-Ac-Thr)-Ile by the NOESY correlations of H-2/Ha-10 (δH 3.65) and the HMBC correlations from H3-20 to C-6, from H-23 to C-11, from H-33 to C-22, and from Ile-NH to C-31 (Figure 3). The peptide chain was closed by the NOESY correlations between H2-5 and H-38. Two proline residues were confirmed by the small chemical shift difference between C-3/C-4 (δC-3–C-4 = 3.4 ppm) in Pro-1 and C-8/C-9 (δC-8–C-9 = 3.1 ppm) in Pro-2, observed in the 13C NMR spectrum (Supplementary Figure S2), which are consistent with the presence of a trans proline (Siemion et al., 1975; Li et al., 2024).
Tandem mass spectrometry (MS/MS) enables the precise determination of amino acid sequences by leveraging diagnostic fragmentation patterns under controlled collision energies. Under low-energy collision-induced dissociation (CID), protonated peptides predominantly undergo amide bond cleavage, generating characteristic N-terminal (bn), C-terminal (yn), and a-type (bn–CO) ions, which are critical for sequence elucidation (Wang et al., 2022; Polce et al., 2000). As detailed in Scheme 1 and Supplementary Figure S17, compound 1 initially underwent lactone ring opening to yield a linear intermediate (Step I), followed by sequential elimination of amino acid residues via two distinct fragmentation pathways (Step II). The observed cleavage patterns closely resembled the patterns of the structurally characterized reference compound japonamide A (Wang et al., 2022), thereby corroborating both the fragmentation mechanism and the derived amino acid sequences.
The absolute configurations of the amino acid residues in compound 1 were unambiguously determined using an optimized Marfey’s method (Wang et al., 2022), involving acid hydrolysis, chiral derivatization with FDAA, and reversed-phase HPLC comparison with authentic L/D standards (Figure 4). The targeted acid hydrolysis (6 M HCl, 100°C) of compound 1, followed by FDAA derivatization, revealed characteristic adducts for Pro, N-methyl-O-methyl-Tyr, Phe, Thr (from N-Ac-Thr hydrolysis), and Ile. The comparative HPLC analysis with authentic standards confirmed the configurations as L-Pro (×2), N-Me-O-Me-L-Tyr, L-Phe, N-Ac-L-Thr, and D-Ile, establishing the complete sequence as cyclo-(L-Pro)-(L-Pro)-(N-Me-O-Me-L-Tyr)-(L-Phe)-(N-Ac-L-Thr)-(D-Ile).
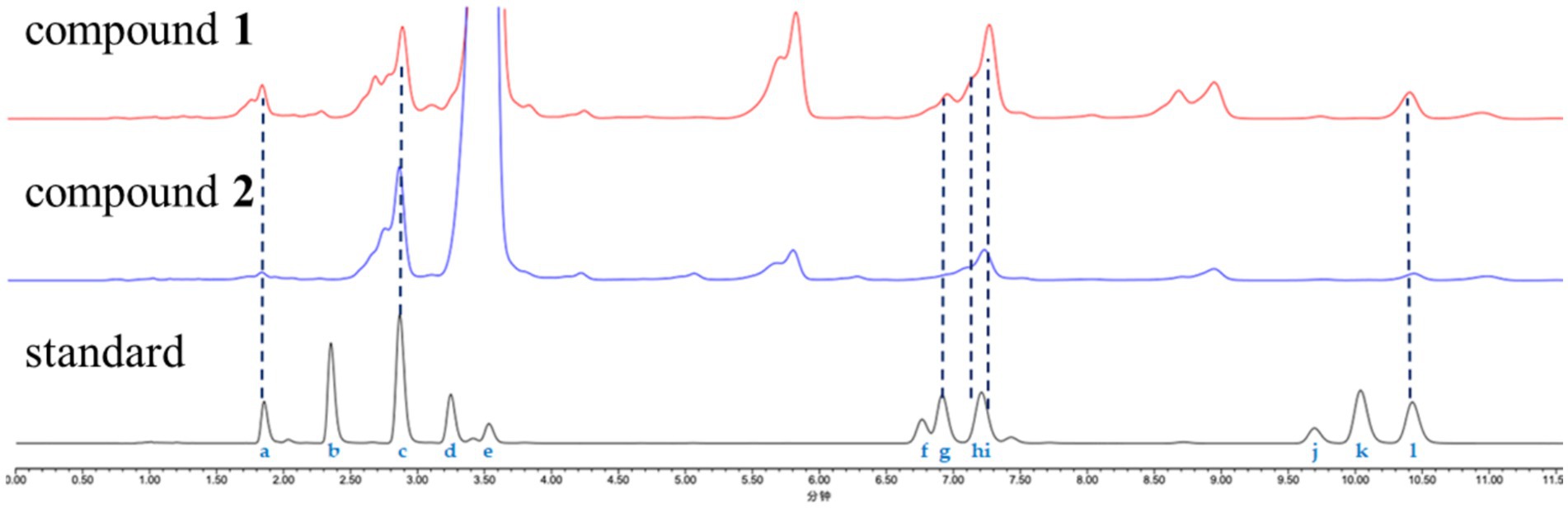
Figure 4. Advanced Marfey’s analysis of compounds 1 and 2. The FDAA derivatives of the standard compounds a–l were as follows: L-Thr (1.85 min), D-Thr (2.35 min), L-Pro (2.88 min), D-Pro (3.25 min), FDAA (3.54 min), L-Ile (6.77 min), N-Me-O-Me-L-Tyr (6.91 min), O-Me-L-Tyr (7.15 min), L-Phe (7.24 min), O-Me-D-Tyr (9.70 min), D-Phe (10.03 min), and D-Ile (10.48 min). The derivatives of the acid hydrolysate and standard amino acids were analyzed using HPLC (ACQUITY C18 column; 1.7 μm, 2.1 × 100 mm; 0.3 ml/min; UV detection at 340 nm) with a linear gradient of acetonitrile (30–45%) in water (TFA, 0.01%) for 20 min.
Compound 2 was obtained as a pale yellow powder. The molecular formula C43H58N6O10 was deduced from a positive HRESIMS ion at m/z [M + H]+ 819.4287 (calculated value: 819.4287), with 18 degrees of unsaturation. The 1H and 13C NMR data (Table 1) of compound 2 closely resembled those of compound 1, indicating that they were structurally similar. The main difference was the absence of an aromatic proton in compound 2, which was instead substituted by a methoxy group to form a 1,4-disubstituted phenyl group. The 1H–1H COSY correlations of H-26/H-27 and H-29/H-30, as well as the HMBC correlations from 28-OCH3 to C-28 and from H-26 to C-28, unequivocally supported this assignment (Figure 3). The remaining similar NMR spectra could establish the planar structure of compound 2, as shown in Figure 3. Subsequent MS/MS analysis and Marfey’s analysis (Scheme 1; Supplementary Figure S17; Figure 4) established the structure of compound 2 as cyclo-(L-Pro)-(L-Pro)-(N-Me-O-Me-L-Tyr)-(O-Me-L-Tyr)-(N-Ac-L-Thr)-(D-Ile).
The known compounds were identified based on the literature data as cis-cyclo(Leu-Leu) (3) (He et al., 2013), trans-cyclo(Pro-Phe) (4) (Wang et al., 2010), and cis-cyclo(Phe-Val) (5) (Harizani et al., 2020).
3.3 Cell proliferation inhibitory activity and antibacterial activity
The antiproliferative activity of the two isolated cyclohexadepsipeptides, compounds 1 and 2, was evaluated against 20 different cell lines, including 18 human tumor cell lines and 2 human normal cell lines. Both compounds showed broad-spectrum antiproliferative activity against MKN-45, HCT116, TE-1, 5,637, CAL-62, and A-637 cells at 20 μM, with inhibition rates ranging from 55.0 to 72.3% (Figure 5). No detectable cytotoxicity was observed for the two tested compounds against A549 and A-375 cells, indicating the selective cytotoxicity of the test compounds. In general, compound 1 exhibited superior cytotoxic activity compared to compound 2. The available data from structure–activity relationship (SAR) analysis suggest that the C-28 substituent may serve as a critical pharmacophore for cytotoxic effects.
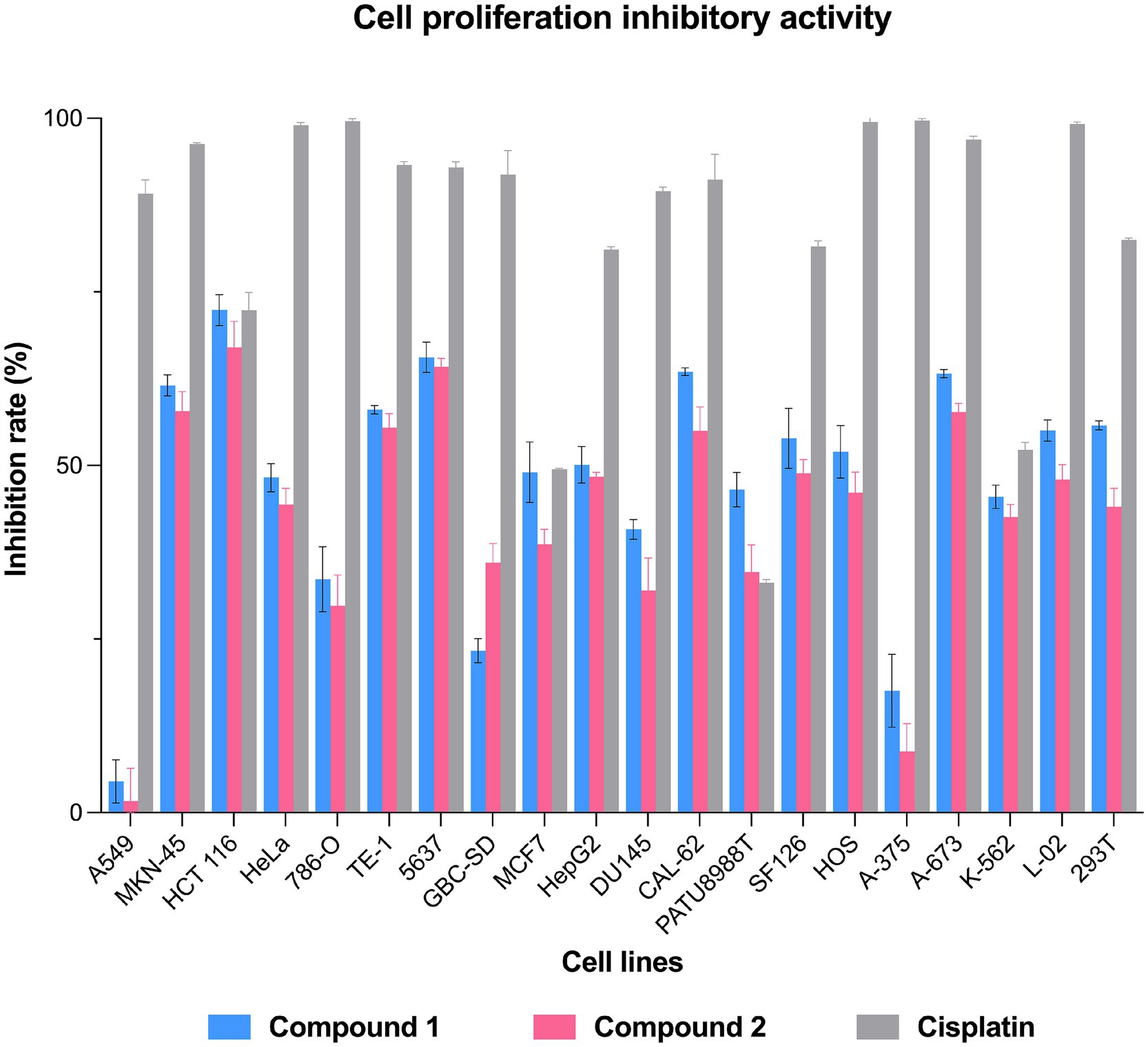
Figure 5. Cell inhibition rates of compounds 1 and 2 against the lung cancer cell line A549, stomach cancer cell line MKN-45, colon cancer cell line HCT 116, cervical cancer cell line HeLa, renal clear cell adenocarcinoma cell line 786-O, esophageal cancer cell line TE-1, bladder cancer cell line 5,637, gallbladder cancer cell line GBC-SD, breast cancer cell line MCF7, liver cancer cell line HepG2, prostate cancer cell line DU145, thyroid cancer cell line CAL-62, pancreatic cancer cell line PATU8988T, brain tumor cell line SF126, osteosarcoma cell line HOS, malignant melanocyte cell line A-375, rhabdomyosarcoma cell line A-673, chronic myeloid leukemia cell line K-562, human normal liver cell line L-02, and embryonic kidney cell line 293 T. Cisplatin was used as a positive control.
The antibacterial activity of compounds 1–5 was evaluated against four bacterial strains: Ralstonia solanacearum (G−), Xanthomonas oryzae (G−), Bacillus cereus (G+), and Bacillus subtilis (G+). As presented in Table 2, compounds 4 and 5 displayed only weak antibacterial activity against the two Gram-positive bacteria B. cereus and B. subtilis.
4 Conclusion
In summary, this study reports the LC–MS/MS-guided isolation and structural characterization of two novel cyclohexadepsipeptides, japonamides C (1) and D (2), along with three known cyclodipeptides (3–5), from the Nicotiana tabacum-derived endophytic fungus A. japonicus TE-739D. The planar structures were unequivocally established through comprehensive 1D/2D NMR analysis and HRESIMS data, while the absolute configurations were resolved using an optimized Marfey’s protocol. A plausible formation mechanism for the MS/MS ion fragments of compounds 1 and 2 was proposed. In the broad-spectrum screening for antiproliferative activity, compounds 1 and 2 displayed moderate cytotoxicity against selected cancer cell lines. The observed SAR trends suggest a possible functional significance of the C-28 substituent in cytotoxicity, although further validation is needed. The results not only expand the chemical diversity of fungal-derived cyclopeptides with bioactivity but also exemplify the utilization of LC–MS/MS-based GNPS in accelerating the discovery of natural products from underexplored microbial sources.
Data availability statement
The datasets presented in this study can be found in online repositories. The names of the repository/repositories and accession number(s) can be found in the article/Supplementary material.
Author contributions
HW: Conceptualization, Data curation, Formal analysis, Writing – original draft. XH: Formal analysis, Investigation, Methodology, Writing – original draft. CD: Methodology, Resources, Writing – original draft. XY: Methodology, Resources, Writing – original draft. PZ: Funding acquisition, Writing – review & editing. GG: Conceptualization, Supervision, Writing – review & editing.
Funding
The author(s) declare that financial support was received for the research and/or publication of this article. This research was funded by the National Natural Science Foundation of China (32270422 and 32200328), the Central Public-interest Scientific Institution Basal Research Fund (Y2022QC32), the Taishan Scholars Program (No. tsqn202211272), and the Agricultural Science and Technology Innovation Program (ASTIP-TRIC05).
Conflict of interest
XH was employed by China Tobacco Zhejiang Industrial Co., Ltd.
The remaining authors declare that the research was conducted in the absence of any commercial or financial relationships that could be construed as a potential conflict of interest.
Generative AI statement
The author(s) declare that no Gen AI was used in the creation of this manuscript.
Publisher’s note
All claims expressed in this article are solely those of the authors and do not necessarily represent those of their affiliated organizations, or those of the publisher, the editors and the reviewers. Any product that may be evaluated in this article, or claim that may be made by its manufacturer, is not guaranteed or endorsed by the publisher.
Supplementary material
The Supplementary material for this article can be found online at: https://www.frontiersin.org/articles/10.3389/fmicb.2025.1595569/full#supplementary-material
References
Cui, Z. R., Wang, Y. Y., Li, J. X., Chi, J., Zhang, P. P., Kong, L. Y., et al. (2022). Natural and Pseudonatural lindenane heterodimers from Sarcandra glabra by molecular networking. Org. Lett. 24, 9107–9111. doi: 10.1021/acs.orglett.2c03769
El-Hawary, S. S., Moawad, A. S., Bahr, H. S., Abdelmohsen, U. R., and Mohammed, R. (2020). Natural product diversity from the endophytic fungi of the genus Aspergillus. RSC Adv. 10, 22058–22079. doi: 10.1039/D0RA04290K
Gu, G., Gong, X., Xu, D., Yang, Y. L., Yin, R. Y., Dai, J. G., et al. (2023). Diphenyl ether derivative rhexocerins and rhexocercosporins from the endophytic fungus Rhexocercosporidium sp. Dzf14 active against gram-positive bacteria with multidrug-resistance. J. Nat. Prod. 86, 1931–1938. doi: 10.1021/acs.jnatprod.3c00295
Gu, G., Hou, X. W., Xue, M. Y., Jia, X. W., Pan, X. Q., Xu, D., et al. (2024a). Rosellichalasins A-H, cytotoxic cytochalasans from the endophytic fungus Rosellinia sp. Glinf021. Phytochemistry 222:114103. doi: 10.1016/j.phytochem.2024.114103
Gu, G., Hou, X. W., Xue, M. Y., Pan, X. Q., Dong, J., Yang, Y. L., et al. (2024b). Diphenyl ethers from endophytic fungus Rhexocercosporidium sp. Dzf14 and their antibacterial activity by affecting homeostasis of cell membranes. Pest Manag. Sci. 80, 2658–2667. doi: 10.1002/ps.7972
Harizani, M., Katsini, E., Georgantea, P., Roussis, V., and Ioannou, E. (2020). New chlorinated 2,5-diketopiperazines from marine-derived Bacteria isolated from sediments of the eastern Mediterranean Sea. Molecules 25:1509. doi: 10.3390/molecules25071509
He, R., Wang, B. C., Wakimoto, T., Wang, M. Y., Zhu, L. C., and Abe, I. (2013). Cyclodipeptides from metagenomic library of a Japanese marine sponge. J. Braz. Chem. Soc. 24, 1926–1932. doi: 10.5935/0103-5053.20130240
Li, H., Chen, Y. L., Tang, B. Q., Liu, Z. J., Peng, B., Li, J. J., et al. (2024). Cyclopeptide Avellanins D-O with antimalarial activity from the Mariana trench Anemone-derived Hamigera ingelheimensis MSC5. J. Nat. Prod. 87, 2695–2708. doi: 10.1021/acs.jnatprod.4c00740
Liu, L., Duan, F. F., Gao, Y., Peng, X. G., Chang, J. L., Chen, J., et al. (2021). Aspersteroids A-C, three rearranged Ergostane-type steroids from Aspergillus ustus NRRL 275. Org. Lett. 23, 9620–9624. doi: 10.1021/acs.orglett.1c03863
Ono, S., Naylor, M. R., Townsend, C. E., Okumura, C., Okada, O., Lee, H. W., et al. (2021). Cyclosporin a: conformational complexity and chameleonicity. J. Chem. Inf. Model. 61, 5601–5613. doi: 10.1021/acs.jcim.1c00771
Orfali, R., Aboseada, M. A., Abdel-Wahab, N. M., Hassan, H. M., Perveen, S., Ameen, F., et al. (2021). Recent updates on the bioactive compounds of the marine-derived genus Aspergillus. RSC Adv. 11, 17116–17150. doi: 10.1039/D1RA01359A
Polce, M. J., Ren, D., and Wesdemiotis, C. (2000). Dissociation of the peptide bond in protonated peptides. J. Mass Spectrom. 35, 1391–1398.
Ruegger, A., Kuhn, M., Lichti, H., Loosli, H. R., Huguenin, R., Quiquerez, C., et al. (1976). Cyclosporin a, a peptide metabolite from Trichoderma polysporum (link ex Pers.) Rifai, with a remarkable immunosuppressive activity. Helv. Chim. Acta 59, 1075–1092. doi: 10.1002/hlca.19760590412
Sanchez, J. F., Somoza, A. D., Keller, N. P., and Wang, C. C. C. (2012). Advances in Aspergillus secondary metabolite research in the post-genomic era. Nat. Prod. Rep. 29, 351–371. doi: 10.1039/c2np00084a
Siemion, I. Z., Wieland, T., and Pook, K. H. (1975). Influence of the distance of the proline carbonyl from the beta and gamma carbon on the C-13 chemical-shifts. Angew. Chem.-Int. Edit. 14, 702–703. doi: 10.1002/anie.197507021
Song, M. R., Liu, Y., Li, T. T., Liu, X. J., Hao, Z. H., Ding, S. Y., et al. (2021). Plant natural flavonoids against multidrug resistant pathogens. Adv. Sci. 8:e2100749. doi: 10.1002/advs.202100749
Walsh, C. (1999). Microbiology - deconstructing vancomycin. Science 284, 442–443. doi: 10.1126/science.284.5413.442
Wang, M. X., Carver, J. J., Phelan, V. V., Sanchez, L. M., Garg, N., Peng, Y., et al. (2016). Sharing and community curation of mass spectrometry data with global natural products social molecular networking. Nat. Biotechnol. 34, 828–837. doi: 10.1038/nbt.3597
Wang, G. H., Dai, S. K., Chen, M. J., Wu, H. B., Xie, L. W., Luo, X. M., et al. (2010). Two Diketopiperazine Cyclo(pro-Phe) isomers from marine Bacteria Bacillus subtilis sp. 13-2. Chem. Nat. Compd. 46, 583–585. doi: 10.1007/s10600-010-9680-8
Wang, H. S., Yuan, X. L., Huang, X. R., Zhang, P., and Gu, G. (2025). Herbicidal and antibacterial secondary metabolites isolated from the Nicotiana tabacum-derived Endophytic fungus Aspergillus japonicus TE-739D. Plants-Basel 14:173. doi: 10.3390/plants14020173
Wang, H. F., Zhang, R., Ma, B., Wang, W. Z., Yu, C., Han, J. J., et al. (2022). Japonamides a and B, two new Cyclohexadepsipeptides from the marine-sponge-derived fungus Aspergillus japonicus and their synergistic antifungal activities. J. Fungi 8:1058. doi: 10.3390/jof8101058
Wu, J. T., Lv, S. M., Lu, C. H., Gong, J., and An, J. B. (2015). Effect of 3,3′-Biisofraxidin on apoptosis of human gastric Cancer BGC-823 cells. Trop. J. Pharm. Res. 14, 1803–1811. doi: 10.4314/tjpr.v14i10.10
Xu, M. Y., Lee, S. Y., Kang, S. S., and Kim, Y. S. (2014). Antitumor activity of jujuboside B and the underlying mechanism via induction of apoptosis and autophagy. J. Nat. Prod. 77, 370–376. doi: 10.1021/np401022g
Zhang, Z., Li, Y. L., Wang, H. N., Xu, W., Wang, C. Y., Ma, H. B., et al. (2024). Ergone derivatives from the deep-sea-derived fungus Aspergillus terreus YPGA10 and 25,28-dihydroxyergone-induced apoptosis in human colon cancer SW620 cells. J. Nat. Prod. 87, 1563–1573. doi: 10.1021/acs.jnatprod.4c00154
Zhang, J. J., Yuan, J. M., Li, Z. J., Fu, C. J., Xu, M. L., Yang, J., et al. (2021). Exploring and exploiting plant cyclic peptides for drug discovery and development. Med. Res. Rev. 41, 3096–3117. doi: 10.1002/med.21792
Zorzi, A., Deyle, K., and Heinis, C. (2017). Cyclic peptide therapeutics: past, present and future. Curr. Opin. Chem. Biol. 38, 24–29. doi: 10.1016/j.cbpa.2017.02.006
Keywords: endophytic fungus, Aspergillus japonicas , cyclohexadepsipeptides, cell proliferation inhibitory activity, japonamides
Citation: Wang H, Hao X, Dong C, Yuan X, Zhang P and Gu G (2025) LC–MS/MS-guided discovery of japonamides C and D, two new cyclohexadepsipeptides, from the Nicotiana tabacum-derived endophytic fungus Aspergillus japonicus TE-739D. Front. Microbiol. 16:1595569. doi: 10.3389/fmicb.2025.1595569
Edited by:
Dewu Zhang, Chinese Academy of Medical Sciences, ChinaReviewed by:
Haibo Zhang, South China Sea Institute of Oceanology (CAS), ChinaSonia Ilaria Maffioli, Naicons Srl, Italy
Copyright © 2025 Wang, Hao, Dong, Yuan, Zhang and Gu. This is an open-access article distributed under the terms of the Creative Commons Attribution License (CC BY). The use, distribution or reproduction in other forums is permitted, provided the original author(s) and the copyright owner(s) are credited and that the original publication in this journal is cited, in accordance with accepted academic practice. No use, distribution or reproduction is permitted which does not comply with these terms.
*Correspondence: Gan Gu, Z3VnYW5AY2Fhcy5jbg==
†These authors have contributed equally to this work