- 1Institute of Environmental Research at Greater Bay, Key Laboratory for Water Quality and Conservation of the Pearl River Delta, Ministry of Education, Guangzhou University, Guangzhou, China
- 2Key Laboratory of Environmental Remediation and Ecological Health, Ministry of Industry and Information Technology, Jiangsu Environmental Engineering Technology Co., Ltd, Jiangsu Environmental Protection Group Co., Ltd, Nanjing, China
- 3Key Laboratory of Integrated Regulation and Resources Development on Shallow Lakes, Ministry of Education, College of Environment, Hohai University, Nanjing, China
In the face of the escalating challenge of sludge production and disposal in wastewater treatment plants (WWTPs), in situ sludge reduction biotechnology (ISRB) has recently emerged as a highly promising strategy. It not only has the potential to curtail sludge generation at its origin but also ensures the sustained efficiency of the treatment process. Several key strategies have demonstrated exceptional potential in harnessing microbial processes for sludge degradation. They encompass enzymatic hydrolysis, microbial inoculation, protozoan/metazoan predation, bacteriophage lysis, and biofilm-based manipulation. Compared to traditional methods (e.g., incineration and landfilling), these biotechnologies offer significant advantages through lower costs, reduced energy consumption, and minimal environmental impacts. The efficacy of ISRB is substantially affected by various factors, where pH, microbial shift, and nutrient conditions play crucial roles. Despite the notable progress made in this field, significant challenges persist when it comes to scaling up these technologies for more extensive and widespread applications. This review comprehensively highlights the fundamental mechanisms, application strategies, and future prospects of ISRB, including one of the first studies to introduce bacteriophage-based approaches for in situ sludge reduction, offering a novel perspective on phage-mediated sludge control. By doing so, it aims to offer in-depth insights into the role of ISRB as a sustainable solution for sludge management, paving the way for further research and development in this crucial area of environmental biotechnology.
1 Introduction
Currently, it is estimated that global WWTPs generate more than 103 million tons of waste activated sludge (WAS) on a wet-matter basis (Li L. et al., 2023). WAS can be a reservoir of various contaminants, including heavy metals, organic pollutants, and pathogens (Hua et al., 2025; Du and Qiu, 2024). Improper management of WAS can lead to serious pollution of soil, water, and atmosphere, which in turn could pose significant risks to both human health and ecological safety (Newell et al., 2024; Khan et al., 2023). In response to these challenges, countries worldwide have been actively implementing strategies to curtail sludge production. For instance, China has set an ambitious target to promote the reduction and recycling of WAS by the end of 2025, aiming to achieve a disposal rate of over 90% (Zhu et al., 2025). At the EU level, the Council Directive 86/278/EEC promoted the safe utilization of sludge and enhances resource efficiency through nutrient recovery (Valchev et al., 2024). Sludge reduction strategies can be broadly classified into two categories, as shown in Figure 1, traditional sludge reduction (TSR), often regarded as end-of-pipe treatment, and in situ sludge reduction (ISR) (Zhou et al., 2023). In contemporary times, developed nations such as the United States have adopted TSR techniques and achieved comparatively high efficiency levels in sludge reduction. However, TSR methods such as incineration and landfilling are fraught with environmental drawbacks.
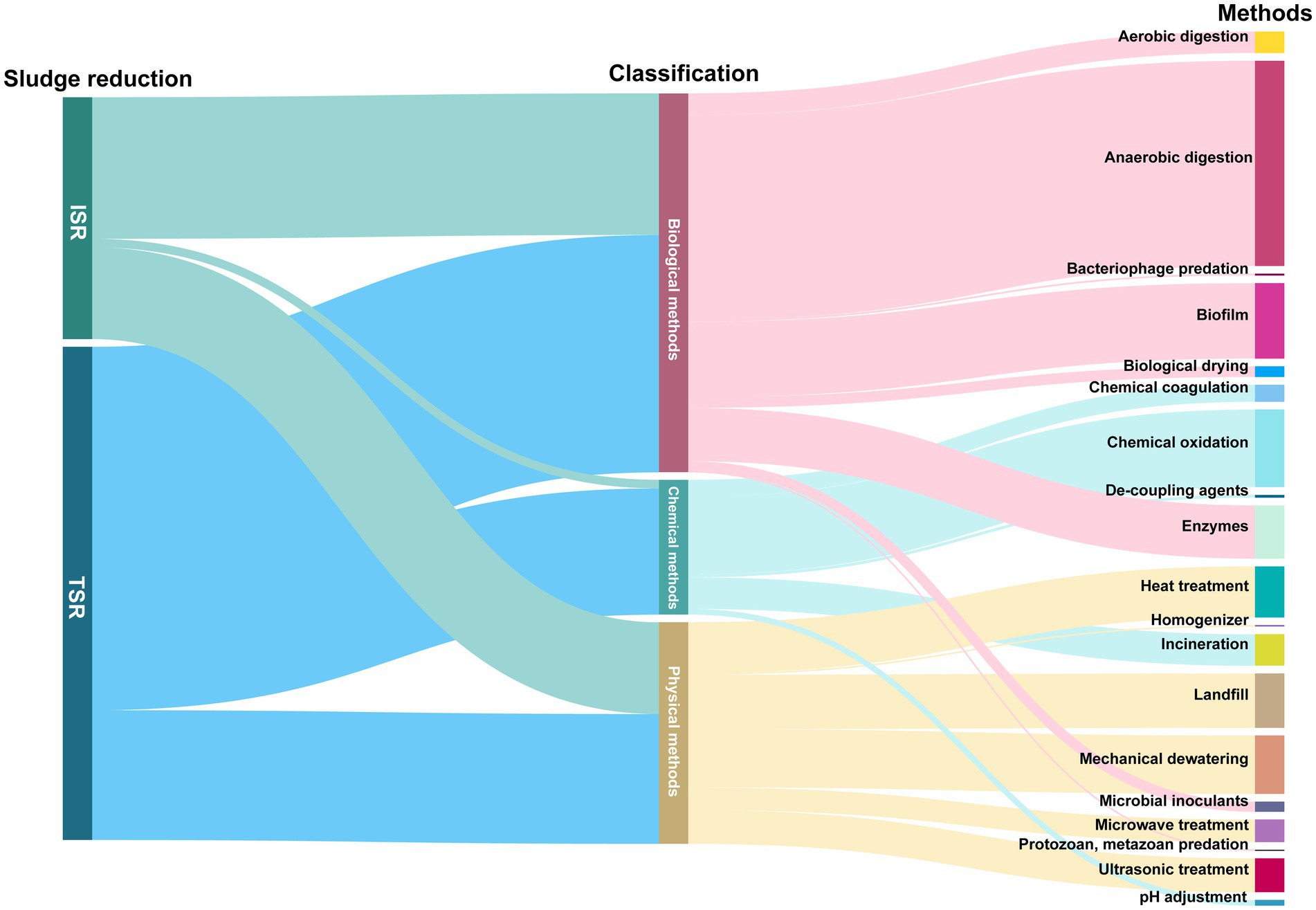
Figure 1. Theme distribution of published studies on sludge reduction methods in the Web of Science database.
These processes are known to trigger secondary pollution, manifested in multiple forms. For instance, they contribute to greenhouse gas emissions, which are a major driver of global climate change (Chu et al., 2024). In addition, the formation of dioxins, highly toxic and persistent organic pollutants, poses a significant threat to both human health and the ecosystem (Li X. et al., 2023). Heavy metal leakage from landfilled sludge can contaminate soil and groundwater, further exacerbating environmental degradation (Ma G. et al., 2023). Another critical concern associated with TSR is its substantial financial burden. Studies have reported that 50–60% of the operational costs of WWTPs are allocated to sludge reduction and disposal (Appels et al., 2008; Campos et al., 2009; Wang et al., 2011). This high cost not only strains the economic resources of WWTPs but also limits the long-term sustainability of these treatment facilities.
Therefore, the environmental and economic drawbacks of TSR call for the exploration and implementation of more sustainable alternatives, such as ISR methods, to reduce the WAS on site (Mu et al., 2021). ISR endeavors to curtail the generation of WAS while maintaining effluent water quality, which redirects the degradation pathway of organic matter from promoting microbial proliferation toward thermal dissipation (Guo et al., 2013). One hundred years ago, Ardern and Lockett (Sheik et al., 2014) invented activated sludge method to biologically treatment wastewater. Interestingly, now researchers are revisiting biotechnology as a new strategy to minimize sludge reduction (Wang K. et al., 2024; Sudharsan et al., 2023). When juxtaposed with physicochemical methods for ISR, biotechnological methods could harness indigenous microbes to break down organic substances in excess sludge, offering exclusive advantages such as cost-effectiveness, low energy consumption, minimal environmental risk, and ease of subsequent recycling (Wang Y. et al., 2023; Li W.S. et al., 2023; Thakur et al., 2024). Nevertheless, research on ISRB remains in its nascent stage and demands further exploration. Previous studies have primarily focused on one of the following aspects: (i) Enzymatic hydrolysis: A combination of cellulase, protease, and lipase enzymes were found effective for sludge reduction, which could diminish the organic component of biosolids by approximately 70% (Nalladiyil et al., 2023). (ii) Microbial inoculation: Hydrolytic bacteria (Candidatus_Competibacter) could break down large organic molecules to reduce the organic content in sludge, while predatory bacteria (Norank_f__Saprospiraceae) could facilitate cell lysis through predation, releasing intracellular materials (Peng et al., 2023). In addition, slow-growing bacteria (Azospira) could reduce sludge production through metabolic uncoupling; and fermentative bacteria (Anaerolineaceae) could further decompose organic matter via fermentation to achieve sludge reduction (Bian et al., 2020; Fan et al., 2023). (iii) Protozoan/metazoan predation: Protozoa (Ciliophora, Oligohymenophorea, Vermamoeba vermiformis) (Miwa et al., 2024) and metazoa (Oligochaete, Nematode, Rotifer) (Gad et al., 2024) could reduce biomass growth by preying on excess bacteria, thereby improving sludge settling performance. (iv) Bacteriophage lysis: Phage GTE7 can lyse bacteria of the genera Gordonia and Nocardia, thereby stabilizing foam. Phage HHY could target the filamentous bacterium Haliscomenobacter hydrossis responsible for sludge bulking, leading to an increased sludge settling rate and a reduced sludge volume index (Ragab et al., 2024; Shivaram et al., 2023). (v) Biofilm-based manipulation: Sludge reduction can be achieved through the synergistic effects of sludge fermentation, microbial enrichment in biofilms, and the reduction of suspended sludge discharge (Fan et al., 2023; Zhao et al., 2023; Riechelmann et al., 2024), as shown in Figure 2.
As far as we know, there have been very limited studies summarizing the advancements of abovementioned five aspects, and even fewer have delved into the impact of bacteriophages on in situ sludge reduction. This study endeavors to showcase the latest progress of ISRB in wastewater treatment systems. It comprehensively presents the unique merits, underlying mechanisms, practical application strategies, and future prospects of ISRB, offering novel perspectives for the eco-friendly treatment of surplus sludge.
2 Exclusive advantages of ISRB
2.1 TSR and ISR
Figure 3 illustrated the common process flow for sludge reduction treatment. TSR methods primarily encompass incineration, landfilling, and anaerobic digestion, all of which entail substantial fixed-asset expenditures. Incineration is a method that can efficiently diminish sludge volume through the complete oxidation of organic matter. However, to minimize air pollution, it necessitates investment in state-of-the-art emission control technologies (Liu et al., 2023). Landfilling has faced limitations in its application due to its long-term adverse environmental impacts on the soil. The leachate generated from landfilled sludge can contaminate groundwater, while the decomposition of organic matter in landfills leads to significant greenhouse gas emissions (Zhao et al., 2019). Anaerobic digestion, on the other hand, demands a considerable amount of thermal energy to maintain the ideal temperature for the anaerobic microorganisms to function effectively. Even after the digestion process, the residual sludge still has a high moisture content, which requires additional treatment steps (Wang et al., 2022). Overall, each of these TSR methods has its own drawbacks in terms of cost, environmental impact, and post-treatment requirements, highlighting the need for more sustainable and efficient sludge management strategies.
The advent of ISR methods presents a promising solution to the issues mentioned above, which has consequently captured global attention (Cheng et al., 2021; Wang et al., 2019; Zhou et al., 2023). ISR methods are designed to curtail sludge production at its source during the generation process, thereby effectively alleviating the burden and associated costs of subsequent sludge handling. In the treatment process, the volume of WAS has been minimized, and the removal efficiency was significantly enhanced by meticulously adjusting biological, chemical, or physical conditions (Li Y.L. et al., 2022; Li C. et al., 2022; Li W. et al., 2022). Specifically, traditional ISR methods encompass multiple strategies. One approach involves precisely controlling the microbial oxygen consumption. By regulating the oxygen supply, the metabolic activities of microorganisms can be optimized, which in turn affects the growth and decomposition of sludge. Another important measure is to reduce the usage of chemical coagulants. Excessive use of chemical coagulants can lead to increased sludge production and potential environmental risks. Minimizing their use not only cuts down on costs but also promotes a more sustainable treatment process. Furthermore, advanced oxidation techniques, such as ozone or ultrasonic treatment, have been employed. These techniques are capable of breaking down complex organic substances, enhancing the biodegradability of the sludge (Apollo et al., 2023). The improvement in biodegradability facilitates the subsequent treatment steps and ultimately contributes to a more efficient sludge reduction and removal process.
The implementation of ISR method demonstrated profound environmental and economic implications. By leveraging advanced biological and physical–chemical processes, ISR can effectively curtail sludge production, mitigate the potential for secondary pollution and offer notable economic advantages. The adoption of ISR can lead to a remarkable improvement in the operational efficiency of WWTPs and align seamlessly with the overarching goals of sustainable development (Wang L. et al., 2024).
2.2 ISRB and physicochemical counterparts
In comparison to ISRB, physicochemical methods exhibit pronounced drawbacks. Physical treatment techniques such as ultrasonic and microwave treatment primarily rely on mechanical shear, cavitation effects, or localized high temperatures and pressures to disrupt sludge flocs and lyse microbial cells (Parvathy Eswari et al., 2022). These processes can effectively reduce sludge volume or enhance subsequent anaerobic digestion efficiency. However, the substantial energy input required for these treatments can significantly increase the operational costs of WWTPs and considerably amplify their carbon footprint (Shen et al., 2022). Chemical methods, for instance, the addition of coagulants, flocculants, or oxidizing agents, achieve rapid sludge thickening and volume reduction by altering the surface charge, structural stability, and water-binding characteristics of sludge particles. While effective in the short term, these methods often result in the accumulation of chemical residues (Guo et al., 2024). These residues may enter aquatic ecosystems through treated water discharge, posing potential threats to the ecological balance, biodiversity, and overall health of these ecosystems (Kong et al., 2023). In addition, when evaluating the economic viability of physicochemical methods, one must take into account both the initial investment and subsequent maintenance costs. The high-cost equipment, continuous energy consumption, and the need for a steady supply of chemicals could offset the overall economic benefits of these methods, making them less attractive from a long-term financial perspective (Liu et al., 2022).
Conversely, ISRB could offer a more sustainable alternative. Strategies such as the addition of enzymes, microbial agents and bacteriophage cocktails can effectively reduce WAS production on-site. These approaches harness specific biological processes to achieve sludge reduction (Yin et al., 2020). By artificially enhancing the biodegradation capacity, ISRB could minimize the reliance on chemical inputs and energy-intensive operations, leading to a reduction in greenhouse gas emissions and aligning with the principles of green and sustainable wastewater treatment (Keb-Fonseca et al., 2021). Table 1 summarized ISR methods, highlighting the mechanisms, advantages, disadvantages, and sludge reduction rates of various methods.
2.3 Sludge reduction and carbon emissions
WWTPs are significant contributors to carbon emissions, accounting for approximately 2% of the total societal carbon footprint. If all WASs were treated via incineration, this could lead to the generation of over 7,023 tons of carbon dioxide equivalent (tCO2-eq) (Zhang et al., 2022). ISRB could offer an effective approach to indirectly mitigate these carbon emissions by curbing the volume of sludge produced. When calculated based on an 80% sludge reduction rate achieved through ISRB, subsequent sludge disposal methods such as anaerobic digestion result in a substantially lower carbon emission of merely 576 tCO2-eq, as shown in Table 2.
Compared with physicochemical methods, ISRB can significantly reduce carbon emissions through the following “3R” strategies (Guo et al., 2013):
i. Energy consumption reduction: It is well-established that sludge transportation, incineration, and landfilling are energy-intensive processes. By minimizing the amount of sludge, ISRB reduces the energy demand associated with these processes (Zhao et al., 2019).
ii. Reconfiguration of external chemical agents: Avoiding the use of sludge dewatering agents can cut down on the carbon emissions associated with their upstream production processes. ISRB presents an alternative that circumvents the need for such agents (Yu et al., 2024).
iii. Reinforcement of removal efficiency: Through the regulation of the microbial community, ISRB can enhance the biodegradability of sludge and optimize metabolic pathways, thereby leading to a decrease in greenhouse gas emissions during the treatment process (Guo et al., 2013).
3 ISRB mechanisms
3.1 Enzymatic hydrolysis
Enzymes could break down complex organic molecules, thereby facilitating the reduction of WAS and enhancing its biodegradability (Sharma and Leung, 2021). The biotechnological mechanism underlying enzyme-mediated ISR primarily encompasses the following key aspects, as shown in Figure 4:
i. Hydrolysis of extracellular polymeric substances (EPS): EPS serve as a protective shield for diverse microorganisms, acting as the initial line of defense during the process of excess sludge lysis (Flemming, 2016). Recent investigations have revealed that alpha-amylase derived from Streptomyces griseus could effectively disrupt the EPS of multidrug-resistant bacteria, with a particular efficacy against Pseudomonas aeruginosa. In the case of P. aeruginosa, the total carbohydrate content within the EPS matrix was notably reduced to 74.09% (Lakshmi et al., 2022). This hydrolysis of EPS has far-reaching implications for the rheological properties of the sludge and, consequently, the stability of biofilms (Kanwar et al., 2019).
ii. Cell lysis and cryptic growth: Enzyme-based pretreatment methods have been compellingly demonstrated to substantially enhance sludge solubilization and curtail suspended solids, with reductions of up to 22 and 17.14% achieved, respectively. Enzyme-induced cell lysis could trigger the liberation of intracellular substances, such as phosphorus and nitrogen. These released nutrients can be assimilated by symbiotic microorganisms through a process referred to as cryptic growth. This mechanism could facilitate nutrient cycling and play a pivotal role in biomass reduction, thereby significantly augmenting the overall efficiency of ISR (Preethi Banu et al., 2022).
iii. Alteration of sludge dewaterability: A synergistic mixture of protease, β-glucanase, cellulase, lipase, and α-amylase has been shown to remarkably improve the dewaterability of sludge (Nesterov et al., 2024; Wang C. et al., 2024). When introduced into the anaerobic digester, this enzyme blend resulted in a notable decline in the CST, from 827 s to 755 s. This treatment could enhance the sludge dewaterability and hold great promise for optimizing the overall efficiency of anaerobic digestion processes. Consequently, it presents a highly promising strategy for effective sludge management (Tas et al., 2018).
3.2 Microbial inoculation
Microbial inoculants could modulate and optimize the microbial community structure within sludge treatment systems, which serves multiple crucial functions, including the reduction of biomass production, enhancement of system stability, and improvement of removal efficiency, ultimately leading to the achievement of sludge reduction goals. However, to ensure the long-term effectiveness and sustainability of this process, precise microbial selection and well-thought-out system management strategies are indispensable.
i. Introduction of slow-growing microorganisms as dominant species: The enrichment of ammonia-oxidizing bacteria and anaerobic ammonia oxidizing bacteria has been effectively implemented in the SNADF system, where they were characterized by a low growth rate and high efficiency in converting ammonia nitrogen, consequently leading to a reduction in the total amount of sludge (Ma L. et al., 2023). Remarkably, the denitrification efficiency of the system can reach up to 94.6%, with the observed yield coefficient as low as 0.05 kgMLSS/kgCOD (Zhang Y. et al., 2023). Additionally, optimizing operational parameters, such as employing aerobic granular sludge process, anaerobic feeding, periodic feast-famine cycling, and shortened settling durations, can enhance sludge granulation. Meanwhile, the selection of slow-growing microorganisms with unique metabolic traits further advances this granulation process (Nancharaiah and Sarvajith, 2019).
ii. Inhibition of high growth rate species: Introducing bacteria from the Bacillus genus, which are capable of producing antibiotics and exoenzymes, can effectively suppress the growth of other rapidly growing microorganisms, such as V. vulnificus. This suppression helps in inhibiting sludge accumulation. For instance, You et al. (Yaylacı, 2021) reported that when B. pumilus PJ_11 culture was inoculated at a level of 1.0 × 108 CFU/mL, the growth of V. vulnificus was inhibited by 58.1% after 120 h.
iii. Optimization of microbial composition: The utilization of specific microbial species, such as Acetobacter and Syntrophomonas, into anaerobic membrane bioreactors has been demonstrated to enhance the hydrolysis and acetogenesis of primary sludge (Amha et al., 2019). Compared to non-bioaugmented operation, bioaugmentation has shown significant improvements, which could increase the overall hydrolysis by 38%, elevate the acetic acid levels in the acid-phase by 105%, and reduce solids by 55% (Martin-Ryals et al., 2020). On one hand bioaugmentation strengthens the system’s adaptability to environmental changes, on the other hand it enhances the synergistic interactions among different microbes, which in turn optimizes the degradation of organic matter and nutrient removal (Gao et al., 2024).
Collectively, these strategies could significantly elevate the wastewater treatment system’s efficiency and minimize sludge production (Zhou et al., 2024), as shown in Figure 5.
3.3 Protozoan/metazoan predation
Protozoa and metazoa exert a sophisticated and effective influence on the sludge reduction via biodegradation and predation processes. These organisms are pivotal in regulating the microbial structure and the associated metabolic functions (Huang et al., 2023). The following are the key biotechnological mechanisms at play:
i. Microbial predation: Protozoa, including ciliates, flagellates, and amoebae, along with certain small metazoa like rotifers, contribute to the regulation of microbial communities by preying on excessive bacteria (Hailei et al., 2017). A study reported that nematodes could have a significant impact on the microbial community in biological reactors, where grazing by nematodes was observed to reduce biomass growth by 45% in a biotrickling filter (Klein et al., 2016).
ii. Correlation between predation and aerobic granule formation: Previous studies, utilizing non-metric multidimensional scaling analysis, have revealed a positive correlation between the presence of protozoa and the size of sludge particles during aerobic granulation (Chan et al., 2021). In the absence of protozoan predators, flocculent sludge could demonstrate diminished compactness and suboptimal settling characteristics, which can impede the formation of granular sludge (Wilén et al., 2018).
iii. Maximization of bacterial diversity by moderate predation pressure: Optimizing the predation intensity could increase prey diversity with enhanced ecosystem productivity. In high-yielding activated sludge communities, the biomass of protozoa can account for up to 20% of the total biomass (Burian et al., 2022). However, as a medium of top-down control, reducing predation pressure leads to a significant decrease in the richness, evenness, and phylogenetic distinctiveness of the bacterial community. The relationship between predation pressure and prey diversity is not linear but rather resembles a hump-shaped pattern, as shown in Figure 6a.
3.4 Bacteriophage lysis
Bacteriophages, as viruses capable of infecting and eradicating specific bacteria, exert a substantial influence on the dynamics of microbial communities and the composition of sludge through intricate infection processes (Carroll-Portillo et al., 2023). Phages can be primarily classified into two distinct types, as shown in Figure 6b. Lytic phages, the first type, are responsible for the lysis of bacteria. They hold significant potential in regulating bacteria with high-growth rates. If competing bacterial cells are lysed by these phages, the intracellular nutrients will be released. This nutrient release serves as a supplementary resource for the growth of neighboring WAS-degrading microbes (Chan et al., 2021). In a particular study, a lytic phage targeting H. hydrossis was isolated from the mixed liquor at a wastewater treatment facility. After the addition of the phage at an optimal virus-to-host ratio of 1:1,000, the SVI remarkably decreased from 155 to 105. Interestingly, H. hydrossis-infecting phages showed no cross infectivity with other bacteria in activated sludge systems (Kotay et al., 2011).
The second type is the lysogenic phage, which has a symbiotic relationship with slow-growing bacteria and proliferate with the host genome replication accordingly. Under certain conditions, slow-growing bacteria can selectively retain prophage-derived genomic elements, especially under the circumstance that these genes could confer some survival advantages (Gebhart et al., 2012). For example, R-type bacteriocins derived from phage tail-like particles, could eliminate neighboring competitor bacteria to provide slow-growing Bacillus subtilis with a competitive edge in the microbial community (Howard-Varona et al., 2017).
In summary, bacteriophages, through their diverse interaction mechanisms with bacteria, play a crucial and multi-faceted role in shaping the ecological balance and functional characteristics of microbial communities in sludge systems, offering new perspectives for the optimization of sludge reduction processes.
3.5 Biofilm-based manipulation
Biofilms represent highly organized microbial communities where microorganisms adhere to one another and to surfaces, ensconced within a self-synthesized EPS matrix (Wang X.B. et al., 2021). These biofilms exert a multifaceted influence on the sludge reduction process in several ways, where Figure 7 illustrated the cycling process during biofilm formation.
i. Enhanced microbial metabolism: In the biofilm environment, microbial cells are in close proximity, which greatly promotes the exchange of genetic materials and metabolic by-products (Xia et al., 2025). Wei et al. (2023) reported that biofilm formation could lead to a remarkable increase in microbial richness. Specifically, the Chao index reached 1,700 on the second day of cultivation. This heightened microbial activity results in more comprehensive degradation of organic matter, which in turn significantly contributes to sludge reduction (Li Y.L. et al., 2022; Li C. et al., 2022; Li W. et al., 2022).
ii. Selective enrichment of specializers: Biofilms possess the ability to selectively enrich specific microbial species, and the stable microenvironment they establish favors the proliferation of slow-growing microbes and endogenous decay organisms. This promotes microbial self-digestion and renewal, contributing to highly efficient pollutant degradation (Jin et al., 2024). In a sequencing batch biofilm reactor, by adjusting the aeration mode, the removal efficiency of total nitrogen was observed to increase from 58.92 to 94.88%. After biofilm formation, genes related to nitrogen removal, such as amoABC, hao, and napAB, were upregulated. This genetic upregulation redirected the energy flow from WAS accumulation toward nitrogen gas production (Lu et al., 2023).
iii. Reduction of biomass yield: In a FCS-SBBR bioreactor, Bacteroidetes and Mizugakiibacter, associated with sludge reduction, were identified as the dominant phylum and genus, respectively, leading to a 49.65% reduction in sludge production (Wang et al., 2018). Compared to suspended growth systems, biofilm-based systems enhance key metabolic processes in the lower sludge layer, such as denitrification, phosphorus release, and anaerobic digestion, thereby accelerating organic matter breakdown and cell lysis while yielding lower biomass production (Freyschmidt and Beier, 2023). This reduced biomass yield is attributed to the slower growth rates and elevated respiratory activity of microorganisms within biofilms, where a greater proportion of metabolic energy is directed toward maintenance and catabolism rather than biomass synthesis (Philipp et al., 2024).
4 Application strategies for ISRB
4.1 Effects of EPS characteristics
EPS, primarily composed of proteins, carbohydrates, humic substances, nucleic acids, uronic acids, and lipids secreted by microorganisms or released through cell lysis, form a complex polymeric network (Pan M. et al., 2023; To et al., 2020). Approximately 80% of the mass of WAS can be attributed to EPS, which have a profound influence on the viscosity and water-retention capacity of the sludge, thereby exerting a substantial impact on its dewatering performance (Li et al., 2024; Frølund et al., 1996).
Water molecules interact with the EPS structure predominantly via electrostatic forces associated with hydroxyl groups and hydrogen bonds, which could contribute significantly to the overall binding energy of EPS. Consequently, the hydrolysis of EPS can enhance the dewaterability of sludge by reducing the polymer concentration (Neyens et al., 2004). A high content of EPS has been shown to undermine the flocculation and sedimentation performance of sludge, posing challenges for conventional mechanical dewatering processes to attain optimal efficiency (Li Y.L. et al., 2022; Li C. et al., 2022; Li W. et al., 2022). To et al. (2020) discovered that the WAS dewatering performance in the majority of WWTPs is suboptimal (averaging 20 wt%), with TB-EPS playing a decisive role in dewatering efficiency (Li Y.L. et al., 2022; Li C. et al., 2022; Li W. et al., 2022).
Additionally, microbial EPS are well-known for providing a multitude of protective functions, such as cryoprotection, resistance to desiccation, buffering of salinity and pH, which could facilitate the formation of aggregates and biofilms (Nagar et al., 2021). The protective layer formed by EPS can hinder the degradation of organic matter in sludge by microorganisms, thus affecting sludge reduction. Wang S. et al. (2023a) summarized that three major families of biofilm dispersal enzymes, namely glycosidases, proteases, and deoxyribonucleases, which target exopolysaccharides, extracellular proteins, and extracellular DNA respectively, can significantly reduce sludge viscosity, enhance dewatering efficiency, and disrupt the protective functions of EPS by regulating the synthesis and degradation processes. It has also been reported that EPS influence the degradation pathways of pollutants in WWTPs. Shapley additive explanations indicated that soluble EPS, S-EPSPS and S-EPSPN, were the most critical factors affecting methane productivity and membrane contamination, respectively. Consequently, these factors impact the “gasification” reaction process of organic carbon (Niu et al., 2024).
4.2 Effects of operational parameters
4.2.1 pH
pH stands as a crucial determinant dictating the efficiency of the ISRB process. It exerts its influence by fine-tuning the biochemical and physiological pathways of enzymes, microbial agents, protozoa, metazoa, and bacteriophages (Yan et al., 2023; Liu et al., 2012; Kotay et al., 2011).
Alkaline proteases, secreted by Bacillus licheniformis, show a marked increase in production as the pH of the culture medium ascends from 6.0 to 8.0. At a pH of 9.5, these enzymes attain an optimal conformational state, which maximizes their activity. This optimal conformation facilitates enhanced substrate binding and catalytic efficiency, thereby expediting the relevant biochemical reactions (Zhang J.H. et al., 2023). Conversely, cellulases sourced from Trichoderma reesei display peak activity at pH 6.0, where the enzyme’s tertiary structure is stabilized, conducive to the efficient hydrolysis of cellulose (Juhász et al., 2004).
Microbial agents such as Bacillus amyloliquefacien thrive in a neutral pH environment. In such conditions, their metabolic activities, including the breakdown of complex organic compounds, are optimized. This is primarily due to the pH-sensitive regulatory networks that govern their metabolic functions (Zhang X. et al., 2023).
Amoebas and rotifers exhibit vigorous proliferation within distinct pH ranges. Amoebas proliferate optimally at pH values between 5.9 and 7.1, while rotifers at pH values from 8.2 to 9.4. These pH ranges are essential for maintaining membrane integrity and osmoregulation, which are critical for their effective predation of harmful bacteria (Ramirez et al., 2014; Ergönül et al., 2016).
Bacteriophages, known for their ability to infect sludge foaming and bulking filamentous bacteria, demonstrate remarkable stability and infectivity within a pH range of 5–8. In this range, the adsorption of the phage onto the host bacteria and the subsequent injection of its DNA are highly efficient (Kotay et al., 2011).
However, the application of pH control in sludge reduction processes faces significant challenges. The biological entities involved in the ISRB process have widely varying optimal pH values. This disparity makes it extremely difficult to maintain a single, uniform pH that can optimize all relevant biological and biochemical processes. Additionally, the inherent heterogeneity of sludge can lead to local pH variations. These variations result in sub-optimal conditions for different microbial and enzymatic activities, thereby impeding the overall efficiency of the sludge reduction process (Guimet et al., 2007; van den Berg et al., 2020).
To address these challenges, the development of a prioritization model could be a viable strategy. Such a model would enable a comprehensive evaluation of the impact of these factors on the overall performance of the system (Zhai et al., 2024). Consequently, it would assist in formulating optimized pH control strategies for more effective sludge reduction and biodegradation.
4.2.2 Microbial interactions
In the activated sludge (AS) system, the microbial community exhibits remarkable dynamism and complexity, encompassing a rich array of microorganisms, including bacteria, protozoa, fungi, and other taxa. This intricate microbial community plays an indispensable role in modulating metabolic pathways and interactions during the degradation of complex organic matter, where functional diversity and stability are pivotal determinants of the efficiency of these degradation processes.
Notably, the microbial communities belonging to the phyla Bacteroidetes and Proteobacteria collectively constitute over 50% of the AS system, with genera such as Fluviicola, Thiobacillus, Sphingopyxis, and Thermomonas being predominant (Tian and Wang, 2020). Bacteroidota are regarded as prime candidates for degrading high-molecular-weight organic matter, such as proteins and carbohydrates. Proteobacteria, on the other hand, are crucial for anaerobic hydrolysis and acidification, responsible for the degradation of organic matter and nitrogen/phosphorus removal. The rapid metabolism and reproduction of both phyla make substantial contributions to sludge reduction. A microbial community with such a structure can enhance sludge reduction efficiency by up to 50%, in stark contrast to monoculture approaches, which achieve significantly lower reduction rates due to the absence of complementary metabolic activities (Bian et al., 2020).
Furthermore, AS systems harbor a substantial number of prokaryotic viruses, with concentrations ranging from 10 to 1,000 times higher than those in natural environments (Chen et al., 2021). These phages are closely associated with functional microorganisms involved in processes like nitrogen and phosphorus removal. Phages are typically characterized by their broad host ranges and can be applied through a phage cocktail strategy. This approach involves the targeted combination of multiple phages to enhance bacterial suppression and meet the specific requirements of different bacterial strains present in wastewater treatment plants.
Introducing exogenous microorganisms is a promising approach for improving the performance of AS systems. However, the most significant limitation lies in the difficulty of maintaining an optimal microbial community that can function consistently under diverse environmental conditions. Additionally, predicting and managing the interactions between introduced microbial populations and the indigenous sludge microbial communities is a complex task. Competition or antagonistic effects between these populations may impede the overall efficacy of the microbial agents, thereby posing challenges to the efficient operation of the AS system.
4.2.3 Aeration mode
Oxygen conditions exert a direct and profound influence on the overall efficiency of hydrolytic enzymes, such as cellulases and proteases (Kumari et al., 2024). Aerobic microorganisms, including Pseudomonas, Bacillus, and Actinobacteria, display distinct growth and metabolic patterns under different dissolved oxygen (DO) levels. In high-oxygen environments, these microbes are highly active, effectively facilitating the decomposition of organic matter in sludge. On the other hand, anaerobic conditions favor hydrolysis and fermentation, leading to the establishment of a lower redox potential (approximately −350 mV), which in turn enriches anaerobic hydrolytic bacteria and enhances downstream sludge reduction (Zhou et al., 2023). Although aerobic conditions accelerate the breakdown of organic matter and stimulate microbial activity, they are energy-intensive and may not ensure long-term sludge reduction. In contrast, anaerobic environments may result in incomplete degradation of organic matter, producing hard-to-degrade intermediate products such as sulfides (Jørgensen et al., 2019).
In the context of ISRB, the aeration mode and its duration, which directly impact DO concentrations, are of paramount importance. Direct aeration is more efficient in introducing oxygen, supporting the growth of aerobic microbial populations. This, in turn, enhances enzymatic activity and leads to a higher degradation rate of organic matter in the sludge. For instance, Guo et al. reported that when DO levels were increased from 2 to 6 mg/L, sludge reduction reached 25% (Guo et al., 2020). Intermittent aeration, on the other hand, often creates localized oxygen-deficient zones. These zones promote anaerobic microbial processes, which, although slower, contribute to sludge reduction through pathways such as fermentation and methanogenesis. However, prolonged aeration can cause overoxidation and reduce sludge reduction efficiency by decreased microbial diversity. For example, the proportion of Proteobacteria increased significantly from 0.08 to 19.9 and 22.6% when the DO was decreased from 4 mg/L to 2 mg/L and 0.8 mg/L, respectively. This clearly demonstrates that precise optimization of aeration parameters is crucial for maximizing sludge reduction (Izadi et al., 2021).
4.3 Effects of nutrient supply
4.3.1 Key nutrients
In the ISRB process, key nutrients including carbon, nitrogen, and phosphorus play a pivotal role. They serve as the foundation for microbial growth and metabolism, exerting a direct influence on the overall stability of the system (Guo et al., 2020).
Denitrification systems employing diverse carbon sources exhibit significant disparities in macroscopic carbon utilization efficiency and microscopic microbial metabolism. Long term bioreactor operation and kinetic studies indicated that the reactor utilizing acetate achieved the highest denitrification rates, while the one utilizing methanol produced the least sludge after the acclimation period (Pan Y. et al., 2023). In addition, glyoxylate acts as a crucial intermediate in the glyoxylate cycle. This cycle enables bacteria, fungi, and plants to harness two-carbon compounds such as acetate for energy production and biosynthesis under carbon-limited conditions. Disrupting this cycle in bacteria could lead to the loss of respiratory activity, biofilm formation capacity, and essential energy supply. This is primarily attributed to imbalanced material and energy metabolism, the collapse of the antioxidant system and reduced extracellular polymer synthesis. Nevertheless, the excessive addition of carbon sources can trigger over-active microbial activity, thereby increasing the total sludge volume (Qi et al., 2023).
Nitrogen sources, such as ammonia and nitrate, are indispensable for the synthesis of amino acids and proteins, which have a profound impact on the community structure, and metabolic pathways of AS microorganisms. With a high C/N ratio, an excess of carbon but a deficiency of nitrogen forces microorganisms to rely on heterotrophic metabolism for energy. However, the lack of nitrogen constrains cell growth and division. Conversely, at a low C/N ratio, although nitrogen is abundant, carbon deficiency restricts microbial growth, resulting in reduced metabolic rates and inferior sludge reduction efficiency (Wang S. et al., 2023b).
Phosphorus, a vital component of adenosine triphosphate (ATP) and cell membranes, is crucial for regulating cellular activities and metabolic processes. Different forms of phosphorus, such as phosphate, superphosphate, and phytate, could modify metabolic pathways, including glutathione metabolism, cysteine metabolism, and galactose metabolism. Nevertheless, an increase in phosphorus levels can stimulate algae growth, especially when phosphorus concentrations in aquatic environments rise, leading to eutrophication and a deterioration of water quality. Additionally, excessive phosphorus can disrupt the decoupling process, thereby affecting system stability and efficiency (Kang et al., 2023).
4.3.2 Trace nutrients
Trace nutrients, including iron, zinc, nickel, and cobalt, primarily influence sludge reduction by modulating enzyme activity and microbial metabolic functions. Iron acts as a cofactor for redox enzymes, such as iron–sulfur proteins and ferredoxin (Rai et al., 2021). Its presence is crucial as it participates in electron transfer processes, which are fundamental for many microbial redox reactions within the AS system. Zinc, characterized by its strong proclivity to form tetrahedral complexes, is a key component of proteins associated with DNA and RNA synthesis. Transcription factors, reverse transcriptase, and RNA polymerases rely on zinc for their proper functioning (Rai et al., 2021). These proteins are essential for genetic information transfer and regulation in microorganisms. Cobalt is intricately involved in the synthesis of vitamin B12, an essential coenzyme in numerous anaerobic microbial metabolic pathways (Stabler, 2020).
The application of trace nutrients in sludge reduction demands well-thought-out strategies. In anaerobic digestion systems, maintaining adequate levels of nickel and cobalt is imperative to sustain stable microbial metabolism. However, an increase in metal solubility can potentially intensify microbial toxicity (Zadeh et al., 2022). This is a critical concern as excessive metal concentrations can disrupt microbial cell membranes, inhibit enzyme activities, and ultimately impede the sludge reduction process.
Furthermore, the application of trace nutrients in sludge reduction still faces several challenges. These elements are often costly and susceptible to loss during wastewater treatment, leading to increased operational expenses. Their bioavailability is limited, as they tend to precipitate or exist in forms that are difficult for microorganisms to utilize (Pereira et al., 2015). Interactions between different elements, including antagonistic and synergistic effects, may further influence their effectiveness. To address these challenges, optimizing treatment processes by incorporating chelating agents to enhance bioavailability, along with precise control of micronutrient concentrations, can improve sludge reduction efficiency while minimizing costs (Bardi et al., 2023).
5 Discussion and conclusion
This comprehensive review systematically synthesizes the latest advancements in biotechnological strategies for in situ sludge minimization within wastewater treatment systems. Particular emphasis is placed on the underlying mechanisms and application tactics of various ISRB methods, including enzymatic hydrolysis, microbial inoculation, protozoan/metazoan predation, bacteriophage lysis, and biofilm-based strategies.
The findings presented herein indicate that ISRBs confer several remarkable advantages over traditional sludge reduction techniques. These include lower energy consumption, reduced chemical usage, and minimized environmental risks. ISRB has demonstrated promising performance and engineering feasibility under both laboratory and pilot-scale conditions, with representative technologies such as anaerobic membrane bioreactors (AnMBRs) and anaerobic ammonium oxidation (Anammox) (Su et al., 2023; Rong et al., 2023). However, its effectiveness is strongly influenced by key operational parameters, including pH, microbial community structure, aeration mode, and nutrient availability. Despite the remarkable progress achieved in ISRB relevant studies, several limitations outlined still pose obstacles to its widespread application in large-scale wastewater treatment systems. Enzymatic treatment has demonstrated substantial potential in decomposing complex macromolecules and enhancing sludge biodegradability. However, challenges related to enzyme stability and high production costs remain unresolved. Similarly, microbial inoculants can effectively optimize the composition of the sludge microbial community and enhance process stability. Yet, their long-term effectiveness is frequently hindered by intricate interspecies interactions. Strategies based on predation by protozoa, metazoa, and bacteriophages represent innovative biocontrol methods for regulating microbial populations. However, further in-depth studies will be required to clarify the predator–prey dynamics and determine the optimal application conditions.
Looking ahead, future research endeavors should focus on integrating multiple ISRB strategies to generate synergistic effects that improve sludge reduction efficiency and stability. The development of customized microbial consortia, genetic engineering of microbial strains to enhance degradation capabilities, and the application of emerging technologies such as bioelectrochemical systems and advanced biofilm reactors offer promising avenues for research and development. Moreover, conducting comprehensive whole-life-cycle assessments to address the environmental and economic impacts of these technologies is crucial for evaluating their contribution to carbon neutrality.
Author contributions
YC: Conceptualization, Funding acquisition, Writing – original draft. XJ: Formal analysis, Investigation, Visualization, Writing – original draft. MY: Visualization, Writing – review & editing. ZW: Supervision, Writing – review & editing.
Funding
The author(s) declare that financial support was received for the research and/or publication of this article. The authors acknowledge the generous support from the National Natural Science Foundation of China (42407190), the National Key R&D Program of China (2023YFE0100900), Funded by Basic Research Program of Jiangsu (BK20230763) and the Young Elite Scientists Sponsorship Program by JSAST (JSTJ-2023-XH022).
Conflict of interest
YC was employed by Jiangsu Environmental Engineering Technology Co., Ltd and Jiangsu Environmental Protection Group Co., Ltd.
The remaining authors declare that the research was conducted in the absence of any commercial or financial relationships that could be construed as a potential conflict of interest.
Generative AI statement
The author(s) declare that no Gen AI was used in the creation of this manuscript.
Publisher’s note
All claims expressed in this article are solely those of the authors and do not necessarily represent those of their affiliated organizations, or those of the publisher, the editors and the reviewers. Any product that may be evaluated in this article, or claim that may be made by its manufacturer, is not guaranteed or endorsed by the publisher.
References
Amha, Y. M., Corbett, M., and Smith, A. L. (2019). Two-phase improves performance of anaerobic membrane bioreactor treatment of food waste at high organic loading rates. Environ. Sci. Technol. 53, 9572–9583. doi: 10.1021/acs.est.9b02639
Apollo, S., Seretlo, M., and Kabuba, J. (2023). In-situ sludge degradation and kinetics of a full scale modified activated sludge system achieving near zero sludge production. J. Water Process Eng. 53:103864. doi: 10.1016/j.jwpe.2023.103864
Appels, L., Baeyens, J., Degrève, J., and Dewil, R. (2008). Principles and potential of the anaerobic digestion of waste-activated sludge. Prog. Energy Combust. Sci. 34, 755–781. doi: 10.1016/j.pecs.2008.06.002
Bardi, M. J., Vinardell, S., Astals, S., and Koch, K. (2023). Opportunities and challenges of micronutrients supplementation and its bioavailability in anaerobic digestion: a critical review. Renew. Sustain. Energy Rev. 186:113689. doi: 10.1016/j.rser.2023.113689
Bian, B., Hu, X., Zhang, L., Yan, X., and Shen, N. (2020). Pilot-scale textile dyeing wasted sludge reduction via microbial agent and aeration: parameter optimization and mechanisms exploration. Environ. Technol. Innov. 20:101053. doi: 10.1016/j.eti.2020.101053
Burian, A., Pinn, D., Peralta-Maraver, I., Sweet, M., Mauvisseau, Q., Eyice, O., et al. (2022). Predation increases multiple components of microbial diversity in activated sludge communities. ISME J. 16, 1086–1094. doi: 10.1038/s41396-021-01145-z
Campos, J. L., Otero, L., Franco, A., Mosquera-Corral, A., and Roca, E. (2009). Ozonation strategies to reduce sludge production of a seafood industry WWTP. Bioresour. Technol. 100, 1069–1073. doi: 10.1016/j.biortech.2008.07.056
Carroll-Portillo, A., Rumsey, K. N., Braun, C. A., Lin, D. M., Coffman, C. N., Alcock, J. A., et al. (2023). Mucin and agitation shape predation of Escherichia coli by lytic coliphage. Microorganisms 11:508. doi: 10.3390/microorganisms11020508
Chan, S. H., Ismail, M. H., Tan, C. H., Rice, S. A., and McDougald, D. (2021). Microbial predation accelerates granulation and modulates microbial community composition. BMC Microbiol. 21:91. doi: 10.1186/s12866-021-02156-8
Chen, Y., Wang, Y., Paez-Espino, D., Polz, M. F., and Zhang, T. (2021). Prokaryotic viruses impact functional microorganisms in nutrient removal and carbon cycle in wastewater treatment plants. Nat. Commun. 12:5398. doi: 10.1038/s41467-021-25678-1
Cheng, Y., Chon, K., Ren, X., Lee, Y., Kou, Y., Wu, Y., et al. (2021). Contributions of enhanced endogenous microbial metabolism via inoculation with a novel microbial consortium into an anoxic side-stream reactor to in-situ sludge reduction for landfill leachate treatment. J. Environ. Manag. 295:113088. doi: 10.1016/j.jenvman.2021.113088
Chiavola, A., Salvati, C., Bongirolami, S., Di Marcantonio, C., and Boni, M. R. (2021). Techno-economic evaluation of ozone-oxidation for sludge reduction at the full-scale. Comparison between the application to the return activated sludge (RAS) and the sludge digestion unit. J. Water Process Eng. 42:102114. doi: 10.1016/j.jwpe.2021.102114
Chu, X., Fei, Z., Chu, Z., and Huang, W.-C. (2024). Decarbonizing the sludge treatment industry: assessing the feasibility of achieving carbon reduction from carbon peaking to carbon neutrality. J. Clean. Prod. 434:140023. doi: 10.1016/j.jclepro.2023.140023
Ding, H. S., and Jiang, H. (2013). Self-heating co-pyrolysis of excessive activated sludge with waste biomass: energy balance and sludge reduction. Bioresour. Technol. 133, 16–22. doi: 10.1016/j.biortech.2013.01.090
Du, M., and Qiu, B. (2024). Food production from sludge. Nat. Water 2, 1053–1054. doi: 10.1038/s44221-024-00331-5
Ergönül, M. B., Erdogan, S., Altindag, A., and Atasagun, S. (2016). Rotifera and Cladocera fauna of several lakes from the Central Anatolia, Marmara, and Western Black Sea regions of Turkey. Turkish J. Zool. 40, 141–146. doi: 10.3906/zoo-1503-22
Fan, X., Zhang, L., Lan, S., Wang, B., Qi, W., Wu, Y., et al. (2023). A pilot study of situ sludge fermentation-driven multiple biological nitrogen removal pathways (SFBNR): revealing microbial synergy mechanism based on co-occurrence network analysis. Water Res. 247:120796. doi: 10.1016/j.watres.2023.120796
Fang, F., Hu, H. L., Qin, M. M., Xue, Z. X., Cao, J. S., and Hu, Z. R. (2015). Effects of metabolic uncouplers on excess sludge reduction and microbial products of activated sludge. Bioresour. Technol. 185, 1–6. doi: 10.1016/j.biortech.2015.02.054
Freyschmidt, A., and Beier, M. (2023). Activity of ammonium-oxidizing Bacteria—An essential parameter for model-based N2O mitigation control strategies for biofilms. Water 15:2389. doi: 10.3390/w15132389
Frølund, B., Palmgren, R., Keiding, K., and Nielsen, P. H. (1996). Extraction of extracellular polymers from activated sludge using a cation exchange resin. Water Res. 30, 1749–1758. doi: 10.1016/0043-1354(95)00323-1
Gad, M., Yosri, M., Fawzy, M. E., Moghazy, R. M., Elfeky, E. M. S., Marouf, M. A., et al. (2024). Microeukaryotic communities diversity with a special emphasis on protozoa taxa in an integrated wastewater treatment system. Environ. Sci. Eur. 36:81. doi: 10.1186/s12302-024-00907-8
Gao, B., Zhang, X. N., Zhu, X. R., Ji, L. M., Zhao, R., and Wu, P. (2024). A critical review of impact and synergistic mechanisms of iron and co-elements in anammox. Chem. Eng. J. 499:155949. doi: 10.1016/j.cej.2024.155949
Gebhart, D., Williams Steven, R., Bishop-Lilly Kimberly, A., Govoni Gregory, R., Willner Kristin, M., Butani, A., et al. (2012). Novel high-molecular-weight, R-type bacteriocins of Clostridium difficile. J. Bacteriol. 194, 6240–6247. doi: 10.1128/jb.01272-12
Guimet, V., Honore, T., Audic, J.-M., and Do-Quang, Z. (2007). Rheology and oxygen transfer in CFD models for wastewater. Prog. Comput. Fluid Dyn. 7, 219–229. doi: 10.1504/pcfd.2007.013014
Guo, J. S., Fang, F., Yan, P., and Chen, Y. P. (2020). Sludge reduction based on microbial metabolism for sustainable wastewater treatment. Bioresour. Technol. 297:122506. doi: 10.1016/j.biortech.2019.122506
Guo, J., Hu, Y., Qian, Y., Shi, Y., and An, D. (2024). Review analysis and challenges of sludge-conditioner interactions: promoting the harmonious integration of experiments and simulations. Chem. Eng. J. 496:153983. doi: 10.1016/j.cej.2024.153983
Guo, W. Q., Yang, S. S., Xiang, W. S., Wang, X. J., and Ren, N. Q. (2013). Minimization of excess sludge production by in-situ activated sludge treatment processes - a comprehensive review. Biotechnol. Adv. 31, 1386–1396. doi: 10.1016/j.biotechadv.2013.06.003
Hailei, W., Ping, L., Ying, W., Lei, L., and Jianming, Y. (2017). Metagenomic insight into the bioaugmentation mechanism of Phanerochaete chrysosporium in an activated sludge system treating coking wastewater. J. Hazard. Mater. 321, 820–829. doi: 10.1016/j.jhazmat.2016.09.072
Howard-Varona, C., Hargreaves, K. R., Abedon, S. T., and Sullivan, M. B. (2017). Lysogeny in nature: mechanisms, impact and ecology of temperate phages. ISME J. 11, 1511–1520. doi: 10.1038/ismej.2017.16
Hua, Y., Chen, S., Tong, T., Li, X., Ji, R., Xu, Q., et al. (2025). Elucidating the molecular mechanisms and comprehensive effects of sludge-derived plant biostimulants on crop growth: insights from metabolomic analysis. Adv. Sci. 12:e2404210. doi: 10.1002/advs.202404210
Huang, Y. D., Liu, Y. L., Fan, K. M., and Xia, S. J. (2023). Influence of powdered activated carbon on gravity-driven ultrafiltration for decentralized drinking water treatment: insights from microbial community and biofilm structure. Sep. Purif. Technol. 313:123451. doi: 10.1016/j.seppur.2023.123451
Izadi, P., Izadi, P., and Eldyasti, A. (2021). Understanding microbial shift of enhanced biological phosphorus removal process (EBPR) under different dissolved oxygen (DO) concentrations and hydraulic retention time (HRTs). Biochem. Eng. J. 166:107833. doi: 10.1016/j.bej.2020.107833
Jin, D., Zhang, X., Zhang, X., Zhou, L., Zhu, Z., Deogratias, U. K., et al. (2024). A critical review of comammox and synergistic nitrogen removal coupling anammox: mechanisms and regulatory strategies. Sci. Total Environ. 948:174855. doi: 10.1016/j.scitotenv.2024.174855
Jørgensen, B. B., Findlay, A. J., and Pellerin, A. (2019). The biogeochemical sulfur cycle of marine sediments. Front. Microbiol. 10:849. doi: 10.3389/fmicb.2019.00849
Juhász, T., Szengyel, Z., Szijártó, N., and Réczey, K. (2004). Effect of pH on cellulase production of Trichoderma ressei RUT C30. Appl. Biochem. Biotechnol. 113, 201–212. doi: 10.1385/ABAB:113:1-3:201
Kacprzak, M., Neczaj, E., Fijalkowski, K., Grobelak, A., Grosser, A., Worwag, M., et al. (2017). Sewage sludge disposal strategies for sustainable development. Environ. Res. 156, 39–46. doi: 10.1016/j.envres.2017.03.010
Kang, J., Qiu, W., Zhang, W., Liu, J., Yang, Z., Wu, Z., et al. (2023). Understanding how various forms of phosphorus stress affect microbiome functions and boost plant disease resistance: insights from metagenomic analysis. Sci. Total Environ. 904:166899. doi: 10.1016/j.scitotenv.2023.166899
Kanwar, K., Pandey, R., Gezici, S., and Azmi, W. (2019). Original article enzymes as competent tool for efficient management of pathogen’s biofilms. Ann. Phytomed. Int. J. 8, 70–81. doi: 10.21276/ap.2019.8.1.8
Keb-Fonseca, G. A., Guerrero-Barajas, C., and Ordaz, A. (2021). Characterization of the biological sulfide oxidation process: in-situ pulse respirometry and ex-situ pulse microrespirometry approach. Revista Mexicana de Ingeniería Química 21, 1–16. doi: 10.24275/rmiq/IA2575
Khan, R., Shukla, S., Kumar, M., Zuorro, A., and Pandey, A. (2023). Sewage sludge derived biochar and its potential for sustainable environment in circular economy: advantages and challenges. Chem. Eng. J. 471:144495. doi: 10.1016/j.cej.2023.144495
Klein, T., Zihlmann, D., Derlon, N., Isaacson, C., Szivak, I., Weissbrodt, D. G., et al. (2016). Biological control of biofilms on membranes by metazoans. Water Res. 88, 20–29. doi: 10.1016/j.watres.2015.09.050
Kong, W. K., Li, Y. J., Zhang, Y. Y., and Liu, H. B. (2023). Enhanced degradation of refractory organics by bioelectrochemical systems: a review. J. Clean. Prod. 423:138675. doi: 10.1016/j.jclepro.2023.138675
Kotay, S. M., Datta, T., Choi, J. D., and Goel, R. (2011). Biocontrol of biomass bulking caused by using a newly isolated lytic bacteriophage. Water Res. 45, 694–704. doi: 10.1016/j.watres.2010.08.038
Kumari, N., Hussain, A., and Ghosh Sachan, S. (2024). Microbes as a tool for the bioremediation of fish waste from the environment and the production of value-added compounds: a review. Lett. Appl. Microbiol. 77:ovae 028. doi: 10.1093/lambio/ovae028
Lakshmi, S. A., Alexpandi, R., Shafreen, R. M. B., Tamilmuhilan, K., Srivathsan, A., Kasthuri, T., et al. (2022). Evaluation of antibiofilm potential of four-domain α-amylase from Streptomyces griseus against exopolysaccharides (EPS) of bacterial pathogens using Danio rerio. Arch. Microbiol. 204:243. doi: 10.1007/s00203-022-02847-4
Li, L., Hua, Y., Zhao, S., Yang, D., Chen, S., Song, Q., et al. (2023). Worldwide research progress and trend in sludge treatment and disposal: a bibliometric analysis. ACS ES T Eng. 3, 1083–1097. doi: 10.1021/acsestengg.3c00149
Li, Y. L., Liu, L., Li, X. R., Xie, J. P., Guan, M. Y., Wang, E. C., et al. (2022). Influence of alternating electric field on deep dewatering of municipal sludge and changes of extracellular polymeric substance during dewatering. Sci. Total Environ. 842:156839. doi: 10.1016/j.scitotenv.2022.156839
Li, Y., Liu, L.-X., Xiong, M., Li, K., Xie, J., Wang, Z., et al. (2024). Deep dewatering of municipal sludge by combining alternating electric field electro-osmosis and nano-CaO2 oxidation. Chem. Eng. J. 490:151769. doi: 10.1016/j.cej.2024.151769
Li, X., Liu, W., Zhang, W., Zhou, A., Xu, Q., He, Z., et al. (2023). Short-chain fatty acid production from waste activated sludge and in situ use in wastewater treatment plants with life cycle assessment. Resour. Conserv. Recycl. 198:107186. doi: 10.1016/j.resconrec.2023.107186
Li, C., Maqbool, T., Kang, H., and Zhang, Z. (2022). In-situ sludge reduction in membrane-controlled anoxic-oxic-anoxic bioreactor: performance and mechanism. Membranes 12:659. doi: 10.3390/membranes12070659
Li, W. S., Yan, D. H., Li, L., Wen, Z. Y., Liu, M. J., Lu, S. X., et al. (2023). Review of thermal treatments for the degradation of dioxins in municipal solid waste incineration fly ash: proposing a suitable method for large-scale processing. Sci. Total Environ. 875:162565. doi: 10.1016/j.scitotenv.2023.162565
Li, L. P., Zhang, J., Tian, Y., Sun, L., Zuo, W., Li, H., et al. (2019). A novel approach for fouling mitigation in anaerobic-anoxic-oxic membrane bioreactor (A O-MBR) by integrating worm predation. Environ. Int. 127, 615–624. doi: 10.1016/j.envint.2019.02.041
Li, W., Zhen, Y., Li, N., Wang, H., Lin, M., Sui, X., et al. (2022). Sulfur transformation and bacterial community dynamics in both desulfurization-denitrification biofilm and suspended activated sludge. Bioresour. Technol. 343:126108. doi: 10.1016/j.biortech.2021.126108
Liu, Z. X., Ling, Q., Cai, Y. W., Xu, L. F., Su, J. H., Yu, K., et al. (2022). Synthesis of carbon-based nanomaterials and their application in pollution management. Nanoscale Adv. 4, 1246–1262. doi: 10.1039/d1na00843a
Liu, J., Lu, Z. B., Yang, J., Xing, M. Y., Yu, F., and Guo, M. T. (2012). Effect of earthworms on the performance and microbial communities of excess sludge treatment process in vermifilter. Bioresour. Technol. 117, 214–221. doi: 10.1016/j.biortech.2012.04.096
Liu, H., Qiao, H., Liu, S., Wei, G., Zhao, H., Li, K., et al. (2023). Energy, environment and economy assessment of sewage sludge incineration technologies in China. Energy 264:126294. doi: 10.1016/j.energy.2022.126294
Lu, Z. Y., Li, Z. F., Cheng, X. J., Xie, J., Li, X. Y., Jiang, X. T., et al. (2023). Treatment of nitrogen-rich wastewater by mixed aeration combined with bioaugmentation in a sequencing batch biofilm reactor: biofilm formation and nitrogen-removal capacity analysis. J. Environ. Chem. Eng. 11:109316. doi: 10.1016/j.jece.2023.109316
Ma, G., Yu, D., Zhang, J., Miao, Y., Zhao, X., Li, J., et al. (2023). A novel simultaneous partial nitrification, anammox, denitrification and fermentation process: enhancing nitrogen removal and sludge reduction in a single reactor. Bioresour. Technol. 369:128484. doi: 10.1016/j.biortech.2022.128484
Ma, L., Zhao, Y., Zhang, C., Su, X. Q., Qiao, Y., Fang, Q. Y., et al. (2023). Reducing CO/NO and absorbing heavy metals in self-sustained smouldering of high-moisture sludge by regulating inert media with low-cost natural zeolite. Environ. Pollut. 337:122556. doi: 10.1016/j.envpol.2023.122556
Martin-Ryals, A. D., Schideman, L. C., and Ong, M. (2020). Utilizing bioaugmentation to improve performance of a two-phase AnMBR treating sewage sludge. Environ. Technol. 41, 1322–1336. doi: 10.1080/09593330.2018.1533041
Merrylin, J., Kaliappan, S., Kumar, S. A., Yeom, I. T., and Rajesh, B. J. (2013). Effect of extracellular polymeric substances on sludge reduction potential of. Int. J. Environ. Sci. Technol. 10, 85–92. doi: 10.1007/s13762-012-0141-8
Miwa, T., Nagatsuma, S., Hirakata, Y., Nagai, M., Ikarashi, T., Takimoto, Y., et al. (2024). Combination of a membrane bioreactor with a rotating biological contactor holding several diverse metazoans can reduce excess sludge with fouling mitigation. Water Res. 266:122352. doi: 10.1016/j.watres.2024.122352
Mu, B. A., Zhu, W., Zhong, J., Chen, L., Lin, N. X., Wang, C. Y., et al. (2021). Mechanism of separation and removal of water from oily sludge using liquid dimethyl ether to dissolve hydrocarbons. Chemosphere 279:130452. doi: 10.1016/j.chemosphere.2021.130452
Nagar, S., Antony, R., and Thamban, M. (2021). Extracellular polymeric substances in Antarctic environments: a review of their ecological roles and impact on glacier biogeochemical cycles. Pol. Sci. 30:100686. doi: 10.1016/j.polar.2021.100686
Nalladiyil, A., Prakash, P., and Sivakumar Babu, G. L. (2023). Garbage enzyme-mediated treatment of landfill leachate: a sustainable approach. Bioresour. Technol. 385:129361. doi: 10.1016/j.biortech.2023.129361
Nancharaiah, Y. V., and Sarvajith, M. (2019). Aerobic granular sludge process: a fast growing biological treatment for sustainable wastewater treatment. Curr. Opin. Environ. Sci. Health 12, 57–65. doi: 10.1016/j.coesh.2019.09.011
Navaratna, D., Shu, L., and Jegatheesan, V. (2014). Performance of a laboratory-scale membrane bioreactor consisting mixed liquor with aquatic worms under toxic conditions. Bioresour. Technol. 155, 41–49. doi: 10.1016/j.biortech.2013.12.061
Nesterov, D., Barrera-Martínez, I., Martínez-Sánchez, C., Sandoval-González, A., and Bustos, E. (2024). Approaching the circular economy: biological, physicochemical, and electrochemical methods to valorize agro-industrial residues, wastewater, and industrial wastes. J. Environ. Chem. Eng. 12:113335. doi: 10.1016/j.jece.2024.113335
Newell, M. E., Babbrah, A., Aravindan, A., Rathnam, R., Kiernan, R., Driver, E. M., et al. (2024). Prevalence rates of neurodegenerative diseases versus human exposures to heavy metals across the United States. Sci. Total Environ. 928:172260. doi: 10.1016/j.scitotenv.2024.172260
Neyens, E., Baeyens, J., Dewil, R., and De heyder, B. (2004). Advanced sludge treatment affects extracellular polymeric substances to improve activated sludge dewatering. J. Hazard. Mater. 106, 83–92. doi: 10.1016/j.jhazmat.2003.11.014
Niu, C., Zhang, Z., Cai, T., Pan, Y., Lu, X., and Zhen, G. (2024). Sludge bound-EPS solubilization enhance CH4 bioconversion and membrane fouling mitigation in electrochemical anaerobic membrane bioreactor: insights from continuous operation and interpretable machine learning algorithms. Water Res. 264:122243. doi: 10.1016/j.watres.2024.122243
Pan, M., Li, H., Han, X., Jiang, S., Diao, Y., Ma, W., et al. (2023). Impact of hydrodynamic conditions on the production and distribution of extracellular polymeric substance in river biofilms. Water 15:3821. doi: 10.3390/w15213821
Pan, Y., Sun, R. Z., Wang, Y., Chen, G. L., Fu, Y. Y., and Yu, H. Q. (2023). Carbon source shaped microbial ecology, metabolism and performance in denitrification systems. Water Res. 243:120330. doi: 10.1016/j.watres.2023.120330
Panepinto, D., Fiore, S., Genon, G., and Acri, M. (2016). Thermal valorization of sewer sludge: perspectives for large wastewater treatment plants. J. Clean. Prod. 137, 1323–1329. doi: 10.1016/j.jclepro.2016.08.014
Parmar, N., Singh, A., and Ward, O. P. (2001). Enzyme treatment to reduce solids and improve settling of sewage sludge. J. Ind. Microbiol. Biotechnol. 26, 383–386. doi: 10.1038/sj.jim.7000150
Parvathy Eswari, A., Kavitha, S., Yukesh Kannah, R., Kumar, G., Bhatia, S. K., Hoon Park, J., et al. (2022). Dispersion assisted pretreatment for enhanced anaerobic biodegradability and biogas recovery-strategies and applications. Bioresour. Technol. 361:127634. doi: 10.1016/j.biortech.2022.127634
Peng, S. M., Luo, H. C., Wang, Z. H., Yang, S. S., Guo, W. Q., and Ren, N.-Q. (2023). Enhanced in-situ sludge reduction of the side-stream process via employing micro-aerobic approach in both mainstream and side-stream. Bioresour. Technol. 377:128914. doi: 10.1016/j.biortech.2023.128914
Pereira, L., Mehboob, F., Stams, A. J. M., Mota, M. M., Rijnaarts, H. H. M., and Alves, M. M. (2015). Metallic nanoparticles: microbial synthesis and unique properties for biotechnological applications, bioavailability and biotransformation. Crit. Rev. Biotechnol. 35, 114–128. doi: 10.3109/07388551.2013.819484
Philipp, L.-A., Bühler, K., Ulber, R., and Gescher, J. (2024). Beneficial applications of biofilms. Nat. Rev. Microbiol. 22, 276–290. doi: 10.1038/s41579-023-00985-0
Preethi Banu, J. R., Kavitha, S., Kannah, R. Y., Varjani, S., and Gunasekaran, M. (2022). Mild hydrogen peroxide interceded bacterial disintegration of waste activated sludge for efficient biomethane production. Sci. Total Environ. 817:152873. doi: 10.1016/j.scitotenv.2021.152873
Qi, Z., Sun, N., and Liu, C. (2023). Glyoxylate cycle maintains the metabolic homeostasis of Pseudomonas aeruginosa in viable but nonculturable state induced by chlorine stress. Microbiol. Res. 270:127341. doi: 10.1016/j.micres.2023.127341
Ragab, S., Mustafa, M. K., Hassan, Y. Y., Nasr, A., Hady, B. H. A. E., and El-Shibiny, A. (2024). Potential use of bacteriophages as biocontrol agents against multidrug-resistant pathogens in wastewater treatment: a review. Environ. Sustain. 7, 287–302. doi: 10.1007/s42398-024-00322-y
Rai, S., Singh, P. K., Mankotia, S., Swain, J., and Satbhai, S. B. (2021). Iron homeostasis in plants and its crosstalk with copper, zinc, and manganese. Plant Stress 1:100008. doi: 10.1016/j.stress.2021.100008
Ramirez, E., Robles, E., Martinez, B., Ayala, R., Sainz, G., Martinez, M. E., et al. (2014). Distribution of free-living amoebae in a treatment system of textile industrial wastewater. Exp. Parasitol. 145, S34–S38. doi: 10.1016/j.exppara.2014.07.006
Riechelmann, C., Habashy, M. M., Rene, E. R., Moussa, M. S., and Hosney, H. (2024). Assessment of hybrid fixed and moving bed biofilm applications for wastewater treatment capacity increase–in situ tests in El-Gouna WWTP, Egypt. Chemosphere 355:139783. doi: 10.1016/j.chemosphere.2023.139783
Rong, C., Wang, T., Luo, Z., and Li, Y.-Y. (2023). Achieving low-carbon municipal wastewater treatment by anaerobic membrane bioreactor at seasonal temperatures: a pilot scale investigation on reducing sludge yield and greenhouse gas emissions. Chem. Eng. J. 463:142415. doi: 10.1016/j.cej.2023.142415
Sharma, N., and Leung, I. K. H. (2021). Novel thermophilic bacterial laccase for the degradation of aromatic organic pollutants. Front. Chem. 9:711345. doi: 10.3389/fchem.2021.711345
Sheik, A. R., Muller, E. E., and Wilmes, P. (2014). A hundred years of activated sludge: time for a rethink. Front. Microbiol. 5:47. doi: 10.3389/fmicb.2014.00047
Shen, J., Wu, Y. H., Yang, Y. H., Zhou, Q. Z., Liu, X., Wei, T. X., et al. (2022). Dyeing sludge carbons activated by alkali-etching for outperformed 4-chlorophenol degradation in electro-Fenton process. J. Water Process Eng. 49:103103. doi: 10.1016/j.jwpe.2022.103103
Shivaram, K. B., Bhatt, P., Applegate, B., and Simsek, H. (2023). Bacteriophage-based biocontrol technology to enhance the efficiency of wastewater treatment and reduce targeted bacterial biofilms. Sci. Total Environ. 862:160723. doi: 10.1016/j.scitotenv.2022.160723
Stabler, S. P. (2020). “Chapter 15- Vitamin B12” in Present knowledge in nutrition. eds. B. P. Marriott, D. F. Birt, V. A. Stallings, and A. A. Yates. 11th ed (Cambridge: Academic Press), 257–271.
Su, Y., Du, R., Wang, J., Li, X., Zhang, Q., Xue, X., et al. (2023). Pilot-scale demonstration of self-enrichment of anammox bacteria in a two-stage nitrification-denitrification suspended sludge system treating municipal wastewater under extremely low nitrogen loading rate. Bioresour. Technol. 387:129693. doi: 10.1016/j.biortech.2023.129693
Sudharsan, G., Sarvajith, M., and Nancharaiah, Y. V. (2023). Selenite reduction and biogenesis of selenium-nanoparticles by different size groups of aerobic granular sludge under aerobic conditions. J. Environ. Manag. 334:117482. doi: 10.1016/j.jenvman.2023.117482
Sun, D. D., Zhang, K., Wang, Y. N., Ma, H. C., and Ma, C. (2020). Reduction of excess sludge production by microwave/activated carbon fibre pretreatment process based on lysis-cryptic growth. Desalin. Water Treat. 185, 99–104. doi: 10.5004/dwt.2020.25425
Tas, D. O., Yangin-Gomec, C., Olmez-Hanci, T., Arikan, O. A., Cifci, D. I., Gencsoy, E. B., et al. (2018). Comparative assessment of sludge pre-treatment techniques to enhance sludge dewaterability and biogas production. Clean-Soil Air Water 46:1700569. doi: 10.1002/clen.201700569
Thakur, H., Kumar, S., Kumar, G., Dhar, A., and Powar, S. (2024). In situ treatment of domestic food waste and bioflocculated sewage sludge in decentralized wastewater treatment plant. J. Environ. Eng. 150:04024028. doi: 10.1061/joeedu.Eeeng-7623
Tian, L., and Wang, L. (2020). A meta-analysis of microbial community structures and associated metabolic potential of municipal wastewater treatment plants in global scope. Environ. Pollut. 263:114598. doi: 10.1016/j.envpol.2020.114598
To, V. H. P., Nguyen, T. V., Bustamante, H., and Vigneswaran, S. (2020). Effects of extracellular polymeric substance fractions on polyacrylamide demand and dewatering performance of digested sludges. Sep. Purif. Technol. 239:116557. doi: 10.1016/j.seppur.2020.116557
Valchev, D., Ribarova, I., Borisov, B., Radovanov, V., Lyubomirova, V., Kostova, I., et al. (2024). Valuable elements in sludge from eight municipal wastewater treatment plants in relation to their recovery potential. Environ. Sci. Eur. 36:11. doi: 10.1186/s12302-023-00837-x
van den Berg, L., Kirkland, C. M., Seymour, J. D., Codd, S. L., van Loosdrecht, M. C. M., and de Kreuk, M. K. (2020). Heterogeneous diffusion in aerobic granular sludge. Biotechnol. Bioeng. 117, 3809–3819. doi: 10.1002/bit.27522
Wang, K., Chen, Y., Cao, M. K., Zheng, G. D., and Cai, L. (2024). Influence of microbial community succession on biodegradation of municipal sludge during biodrying coupled with photocatalysis. Chemosphere 349:140901. doi: 10.1016/j.chemosphere.2023.140901
Wang, Y., Chen, Z., Feng, M., Ma, Q., and Liu, C. (2023). Enhanced nitrogen removal performance of microorganisms to low C/N ratio black-odorous water by coupling with iron-carbon micro-electrolysis. J. Environ. Chem. Eng. 11:110893. doi: 10.1016/j.jece.2023.110893
Wang, X. B., Chen, T. N., Qi, X. F., Zhang, Y. D., Gao, C. Y., Xie, Y. L., et al. (2021). Organic matter release from primary sludge by mechanical cutting. J. Water Process Eng. 40:101896. doi: 10.1016/j.jwpe.2020.101896
Wang, Y., Du, Z., Liu, B., Liu, Y., Wang, H., Liu, W., et al. (2019). Comparison of in-situ sludge reduction in a sequencing batch biofilm reactor (SBBR) under different carriers: operation parameter optimizations. Desalin. Water Treat. 163, 36–47. doi: 10.5004/dwt.2019.24417
Wang, L., Hao, X., Jiang, T., Li, X. D., Yang, J. Y., and Wang, B. (2024). Feasibility of sludge fermentation coupled with partial denitrification: key roles of initial organic matters and alkaline pH. Bioresour. Technol. 401:130730. doi: 10.1016/j.biortech.2024.130730
Wang, S., Hu, C., Cheng, F., and Lu, X. (2023a). Performance of a combined low-consumption biotreatment system with cost-effective ecological treatment technology for rural domestic sewage treatment. J. Water Process Eng. 51:103380. doi: 10.1016/j.jwpe.2022.103380
Wang, Y., Liu, Y., Wang, S., Li, J., Liu, Y., Yu, B., et al. (2021). Enhanced biological nitrogen removal and sludge reduction by a~2O biofilm process. Ind. Water Treat. 41, 72–76. doi: 10.11894/iwt.2020-0398
Wang, Y. L., Liu, B. Z., Zhang, K. F., Liu, Y. J., Xu, X. X., and Jia, J. Q. (2018). Investigate of in situ sludge reduction in sequencing batch biofilm reactor: performances, mechanisms and comparison of different carriers. Front. Environ. Sci. Eng. 12:5. doi: 10.1007/s11783-018-1077-0
Wang, G. H., Sui, J., Shen, H. S., Liang, S. K., He, X. M., Zhang, M. J., et al. (2011). Reduction of excess sludge production in sequencing batch reactor through incorporation of chlorine dioxide oxidation. J. Hazard. Mater. 192, 93–98. doi: 10.1016/j.jhazmat.2011.04.099
Wang, J. F., Sun, Y. P., Zhang, D. A., Broderick, T., Strawn, M., Santha, H., et al. (2022). Unblocking the rate-limiting step of the municipal sludge anaerobic digestion. Water Environ. Res. 94:e10793. doi: 10.1002/wer.10793
Wang, C., Wei, W., Wu, L., Wang, Y., Dai, X. H., and Ni, B. J. (2024). A novel sustainable and self-sufficient biotechnological strategy for directly transforming sewage sludge into high-value liquid biochemicals. Environ. Sci. Technol. 58, 12520–12531. doi: 10.1021/acs.est.4c03165
Wang, S., Zhao, Y., Breslawec, A. P., Liang, T., Deng, Z., Kuperman, L. L., et al. (2023b). Strategy to combat biofilms: a focus on biofilm dispersal enzymes. npj Biofilms Microb. 9:63. doi: 10.1038/s41522-023-00427-y
Wei, J., Huang, X., Wang, H. J., Wang, F. P., Liu, X. Y., Yan, Y., et al. (2023). Insight into biofilm formation of wastewater treatment processes: nitrogen removal performance and biological mechanisms. Sci. Total Environ. 903:166550. doi: 10.1016/j.scitotenv.2023.166550
Wei, L., Zhu, F., Li, Q., Xue, C., Xia, X., Yu, H., et al. (2020). Development, current state and future trends of sludge management in China: based on exploratory data and CO2-equivaient emissions analysis. Environ. Int. 144:106093. doi: 10.1016/j.envint.2020.106093
Wilén, B.-M., Liébana, R., Persson, F., Modin, O., and Hermansson, M. (2018). The mechanisms of granulation of activated sludge in wastewater treatment, its optimization, and impact on effluent quality. Appl. Microbiol. Biotechnol. 102, 5005–5020. doi: 10.1007/s00253-018-8990-9
Xia, L., Wang, J., Chen, M., Li, G., Wang, W., and An, T. (2025). Biofilm formation mechanisms of mixed antibiotic-resistant bacteria in water: bacterial interactions and horizontal transfer of antibiotic-resistant plasmids. J. Hazard. Mater. 481:136554. doi: 10.1016/j.jhazmat.2024.136554
Yan, H., Ali, A., Su, J. F., Shi, J., Xu, L., Huang, T. L., et al. (2023). Sodium alginate/sinter gel spheres immobilized lysozyme producing strain SJ25 enhanced sludge reduction: optimization and mechanism. Bioresour. Technol. 371:128643. doi: 10.1016/j.biortech.2023.128643
Yang, G., Zhang, G. M., and Wang, H. C. (2015). Current state of sludge production, management, treatment and disposal in China. Water Res. 78, 60–73. doi: 10.1016/j.watres.2015.04.002
Yaylacı, E. U. (2021). Isolation and characterization of Bacillus spp. from aquaculture cage water and its inhibitory effect against selected Vibrio spp. Arch. Microbiol. 204:26. doi: 10.1007/s00203-021-02657-0
Yin, X. F., Li, J., Li, X. F., Hua, Z. Z., Wang, X. H., and Ren, Y. P. (2020). Self-generated electric field to suppress sludge production and fouling development in a membrane bioreactor for wastewater treatment. Chemosphere 261:128046. doi: 10.1016/j.chemosphere.2020.128046
Yu, X. L., Ding, J., Yang, S. S., Pang, J. W., Lu, M. Y., Zhao, X., et al. (2024). Strategic carbon emission assessment in sludge treatment: a dynamic tool for low-carbon transformation. Environ. Int. 193:109124. doi: 10.1016/j.envint.2024.109124
Zadeh, P. H., Serrano, A., Collins, G., and Fermoso, F. G. (2022). Interrelating EPS, soluble microbial products and metal solubility in a methanogenic consortium stressed by nickel and cobalt. Ecotoxicol. Environ. Saf. 238:113579. doi: 10.1016/j.ecoenv.2022.113579
Zhai, S., Chen, K., Yang, L., Li, Z., Yu, T., Chen, L., et al. (2024). Applying machine learning to anaerobic fermentation of waste sludge using two targeted modeling strategies. Sci. Total Environ. 916:170232. doi: 10.1016/j.scitotenv.2024.170232
Zhang, Y., Hu, J., Zhang, Q., Cai, D., Chen, S., and Wang, Y. (2023). Enhancement of alkaline protease production in recombinant Bacillus licheniformis by response surface methodology. Bioresour. Bioprocess. 10:27. doi: 10.1186/s40643-023-00641-8
Zhang, J. H., Ma, G. C., Bi, X. J., Zhao, X. C., Li, J. W., Zhang, Y., et al. (2023). Achieving advanced nitrogen removal and excess sludge treatment via single nitritation/anammox-fermentation combined system. Bioresour. Technol. 387:129550. doi: 10.1016/j.biortech.2023.129550
Zhang, J., Xu, D. Y., Zhang, G. M., Ren, Z. J., and Zhu, Y. C. (2021). Critical review on ultrasound lysis-cryptic growth for sludge reduction. J. Environ. Chem. Eng. 9:106263. doi: 10.1016/j.jece.2021.106263
Zhang, C., Yang, X., Tan, X. J., Wan, C. L., and Liu, X. (2022). Sewage sludge treatment technology under the requirement of carbon neutrality: recent progress and perspectives. Bioresour. Technol. 362:127853. doi: 10.1016/j.biortech.2022.127853
Zhang, X., Zhang, Y., Wang, L., Li, W., and Hou, L. (2023). Identification and control of gas-producing bacteria isolated from the swollen bagged soy sauce. Int. J. Food Microbiol. 407:110396. doi: 10.1016/j.ijfoodmicro.2023.110396
Zhao, Q., Li, J. W., Deng, L. Y., Jia, T. P., Zhao, Y., Li, X. Y., et al. (2023). From hybrid process to pure biofilm anammox process: suspended sludge biomass management contributing to high-level anammox enrichment in biofilms. Water Res. 236:119959. doi: 10.1016/j.watres.2023.119959
Zhao, X. D., Yang, J. Z., Tu, C. Q., Zhou, Z., Wu, W., Chen, G., et al. (2019). A full-scale survey of sludge landfill: sludge properties, leachate characteristics and microbial community structure. Water Sci. Technol. 80, 1185–1195. doi: 10.2166/wst.2019.362
Zhou, P., Li, D. J., Zhang, C., Ping, Q., Wang, L., and Li, Y. M. (2024). Comparison of different sewage sludge pretreatment technologies for improving sludge solubilization and anaerobic digestion efficiency: a comprehensive review. Sci. Total Environ. 921:171175. doi: 10.1016/j.scitotenv.2024.171175
Zhou, Z., Sun, Y., Fu, L., Zuo, Y., Shao, Y., Wang, L., et al. (2023). Unraveling roles of the intermediate settler in a microaerobic hydrolysis sludge in situ reduction process. Bioresour. Technol. 384:129228. doi: 10.1016/j.biortech.2023.129228
Zhu, M. Y., Smith, S. J., Chen, M. P., Evans, M., Chai, Q. M., Teng, F., et al. (2025). A comparative review of methane policies of the United States and China in the context of US-China climate cooperation. Clim. Pol. 25, 171–189. doi: 10.1080/14693062.2024.2366902
Glossary
WWTPs - wastewater treatment plants
ISRB - in situ sludge reduction biotechnology
WAS - waste activated sludge
TSR - traditional sludge reduction
ISR - in situ sludge reduction
EPS - extracellular polymeric substances
CST - capillary suction time
SNADF - simultaneous partial nitrification anammox denitrification and fermentation
MLSS - mixed liquor suspended solids
COD - chemical oxygen demand
CFU - colony-forming unit
SVI - sludge volume index
FCS-SBBR - sequencing batch biofilm reactor with composite floating spherical carries
TB-EPS - tightly bound extracellular polymeric substances
S-EPSPS - soluble extracellular polymeric substances polysaccharide
S-EPSPN - soluble extracellular polymeric substances protein
pH - potential of hydrogen
DNA - deoxyribonucleic acid
AS - activated sludge
DO - dissolved oxygen
ATP - adenosine triphosphate
RNA - ribonucleic acid
AnMBRs - anaerobic membrane bioreactors
Anammox - anaerobic ammonium oxidation
Keywords: in situ sludge reduction, environmental biotechnology, bacteriophage lysis, carbon emission, carbon neutrality
Citation: Chen Y, Jiang X, Yang M and Wang Z (2025) Biotechnology revival: in situ sludge minimization in wastewater. Front. Microbiol. 16:1603215. doi: 10.3389/fmicb.2025.1603215
Edited by:
Bo Li, Guangdong University of Technology, ChinaReviewed by:
Shuyu Jia, Nanjing Agricultural University, ChinaYuchun Yang, Sun Yat-sen University, China
Junfeng Wan, Zhengzhou University, China
Copyright © 2025 Chen, Jiang, Yang and Wang. This is an open-access article distributed under the terms of the Creative Commons Attribution License (CC BY). The use, distribution or reproduction in other forums is permitted, provided the original author(s) and the copyright owner(s) are credited and that the original publication in this journal is cited, in accordance with accepted academic practice. No use, distribution or reproduction is permitted which does not comply with these terms.
*Correspondence: Zhu Wang, d2FuZ3podUBnemh1LmVkdS5jbg==