- 1Department of Pediatrics, School of Medicine, Aichi Medical University, Nagakute, Japan
- 2Department of Pediatrics and Neonatology, Graduate School of Medical Sciences, Nagoya City University, Nagoya, Japan
- 3Department of Pediatrics, Fukuchiyama City Hospital, Fukuchiyama, Japan
- 4Adachi Pediatric Clinic, Fukuchiyama, Japan
- 5Department of Pediatrics, Hamamatsu University School of Medicine, Hamamatsu, Japan
- 6Department of Pediatrics, Shinshu University School of Medicine, Nagano, Japan
- 7Center for Medical Genetics, Shinshu University Hospital, Matsumoto, Japan
- 8Department of Pediatrics, Fujita Health University School of Medicine, Toyoake, Japan
Background: Monocarboxylate transporter 8 (MCT8) is a thyroid hormone transmembrane transporter protein. MCT8 deficiency induces severe X-linked psychomotor retardation. Previous reports have documented delayed myelination in the central white matter (WM) in these patients; however, the regional pattern of myelination has not been fully elucidated. Here, we describe the regional evaluation of myelination in four patients with MCT8 deficiency. We also reviewed the myelination status of previously reported Japanese patients with MCT8 deficiency based on magnetic resonance imaging (MRI).
Case Reports: Four patients were genetically diagnosed with MCT8 deficiency at the age of 4–9 months. In infancy, MRI signal of myelination was observed mainly in the cerebellar WM, posterior limb of internal capsule, and the optic radiation. There was progression of myelination with increase in age.
Discussion: We identified 36 patients with MCT8 deficiency from 25 families reported from Japan. The available MRI images were obtained at the age of <2 years in 13 patients, between 2 and 4 years in six patients, between 4 and 6 years in three patients, and at ≥6 years in eight patients. Cerebellar WM, posterior limb of internal capsule, and optic radiation showed MRI signal of myelination by the age of 2 years, followed by centrum semiovale and corpus callosum by the age of 4 years. Most regions except for deep anterior WM showed MRI signal of myelination at the age of 6 years.
Conclusion: The sequential pattern of myelination in patients with MCT8 deficiency was largely similar to that in normal children; however, delayed myelination of the deep anterior WM was a remarkable finding. Further studies are required to characterize the imaging features of patients with MCT8 deficiency.
Introduction
Monocarboxylate transporter 8 (MCT8) is a thyroid hormone (TH) transmembrane transporter protein encoded by the MCT8 (SLC16A2) gene located on human chromosome Xq13.2 (1, 2). Males affected by MCT8 deficiency exhibit severe cognitive deficit, spastic or dystonic quadriplegia, and axial hypotonia, known as the Allan–Herndon–Dudley syndrome (AHDS) (3). An estimated prevalence of MCT8 deficiency is 1 in 70,000 men (4). Disease features in large cohorts have been reported, including brain magnetic resonance imaging (MRI) (4, 5). Brain MRI of patients with MCT8 deficiency shows delayed myelination in the central white matter (WM) (4, 6, 7).
MCT8 is crucial for the transport of triiodothyronine (T3) and thyroxine (T4) in several tissues, including the brain (4). MCT8 is highly expressed in neuronal populations of the cerebral and cerebellar cortex, hippocampus, striatum, and hypothalamus (8). MCT8 is also expressed in the choroid plexus and large capillaries, indicating its involvement in TH transport across the blood–brain barrier (BBB) (9) and/or blood–cerebrospinal fluid (CSF) barrier (8). MCT8 protein is present in neurons and astrocytes of the paraventricular and infundibular nuclei at the human blood–hypothalamus border. Therefore, MCT8 is believed to provide T3 to the central nervous system (CNS) (8).
Oligodendrocytes are the myelinating glia in the CNS (6, 10, 11). Oligodendrocyte precursor cells (OPCs) are established during pre- and perinatal development (6). TH receptors are expressed in the oligodendrocytes in the CNS during embryonic and adult lives (10). The differentiation of OPCs is thought to be regulated by T3 (12). T3 transferred via MCT8 is reported to induce OPC differentiation into mature oligodendrocytes and facilitate the formation of myelin extensions (13). Lack of MCT8 at the OPC level induced reduction of intracellular T3 action, blocking the OPC differentiation to mature and myelinating oligodendrocytes (13). Therefore, abnormal myelination in MCT8 deficiency may be caused by the disruption of OPC differentiation.
Myelination starts early, but the big acceleration phase occurs postnatally up to 2 years of age and is dependent on the brain region. During normal brain development, myelination begins from the 2nd trimester of the fetal life (14–16). Myelination of WM in the brain can be evaluated by conventional MRI. By the age of 3 months, normal infants exhibit high signal intensity in the anterior limb of the internal capsule on brain T1-weighted images (T1WI) (15). On T2-weighted images (T2WI), the splenium of the corpus callosum shows low signal intensity by 6 months of age, the genu of the corpus callosum by 8 months of age, and the anterior limb of the internal capsule by 11 months of age (15). With the exception of the subcortical WM, adult appearance of cerebral WM is seen by the age of 18 months (15).
Patients with MCT8 deficiency are known to exhibit delayed myelination (4). Children with MCT8 deficiency aged <5 years usually show MRI signal of severely delayed myelination, which improve gradually with increase in age (17). MCT8 deficiency was found in 6 of 53 families affected by hypomyelinating leukodystrophies of unknown etiology (18). Nevertheless, analysis of the myelination status in patients with MCT8 deficiency has led to conflicting interpretations (6). The patient heterogeneity in WM phenotype was described (5). Different groups have made different interpretations of the same MRI (7, 19). Hypomyelination rather than delayed myelination has been reported in another study (5). In addition, few studies have characterized the specific myelination pattern in different brain regions in these patients (5). Therefore, regional evaluation using a standardized methodology is necessary to determine the status of myelination in patients with MCT8 deficiency.
In the present study, we describe the regional pattern of myelination in four patients with MCT8 deficiency, based on conventional MRI. In addition, we reviewed the previous case reports of Japanese patients with MCT8 deficiency and assessed the myelination status based on MRI findings.
Subjects and Methods
Patients' Background
Case 1 was a nine-month-old child with developmental delay and abnormal thyroid function test (TFT). The child was referred to the Aichi Medical University from regional core hospital (Fukuchiyama City Hospital) for genetic analysis due to suspected MCT8 deficiency. Case 2 was diagnosed as MCT8 deficiency at the age of 9 months due to poor head control and abnormal TFT at the University hospital (Nagoya City University). He was referred to the Aichi Medical University for continuing medical care at the age of 9 months. Cases 3 and 4 were referred from University hospitals (Hamamatsu University Hospital and Shinshu University Hospital) to the Aichi Medical University at the age of 2 years and 3 years, respectively, for the evaluation of TFTs in the neonatal period and the myelination status on MRI.
The clinical course and the TFTs of the four patients with MCT8 deficiency are summarized in Table 1. A cousin of Case 3's mother and the half-brother of Case 4's mother had intellectual disability. Missense mutation of the MCT8 gene was identified in Cases 1 and 4, while a non-sense mutation was identified in Case 2, and a frameshift mutation was identified in Case 3. All patients showed normal thyroid stimulating hormone (TSH), increased free triiodothyronine (FT3), and decreased free thyroxine (FT4) levels.
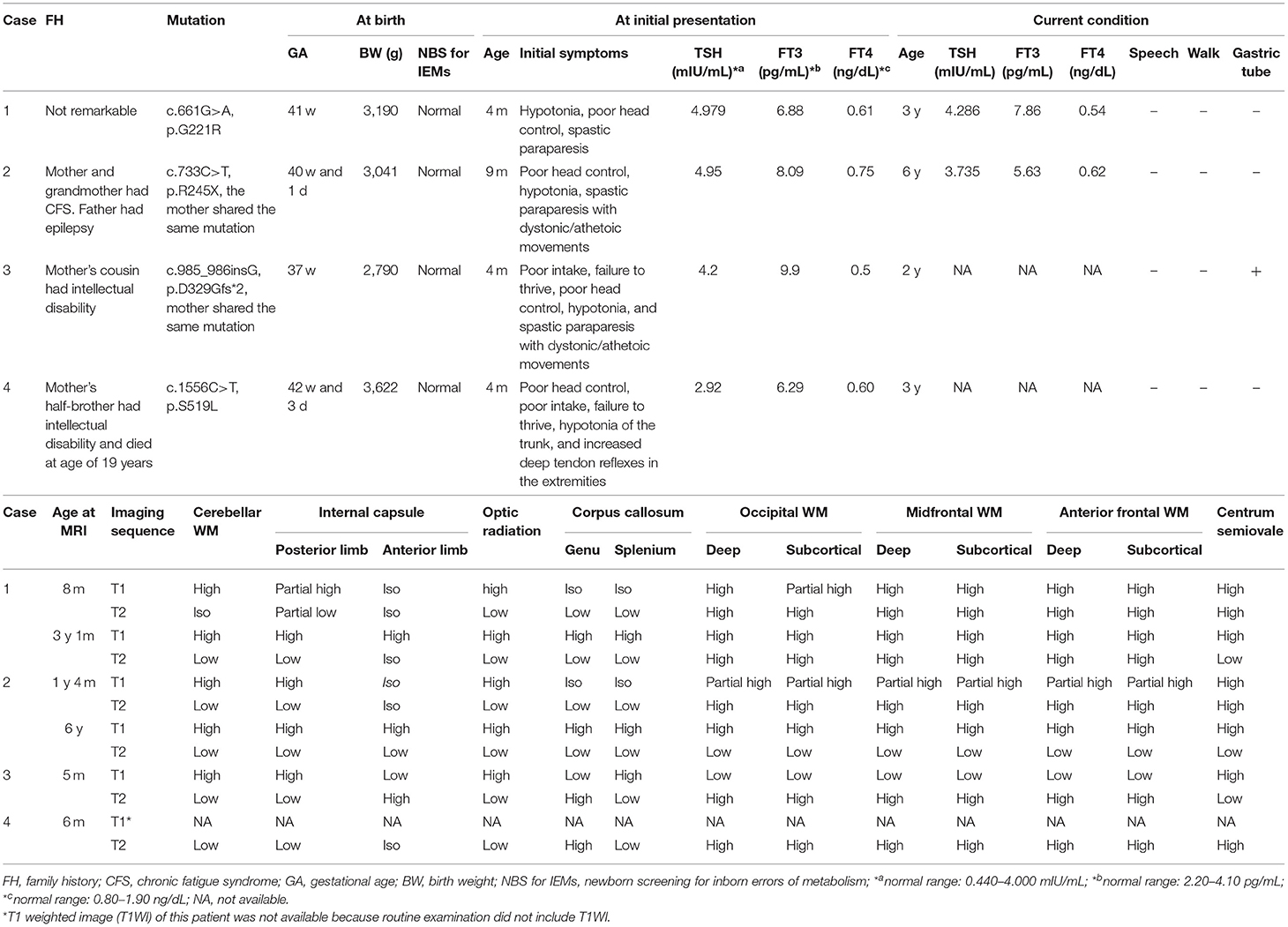
Table 1. Summary of the clinical course, thyroid function tests, and myelination status in brain T1- and T2-weighted MRI images of the four patients with MCT8 deficiency.
Ethical Compliance
This study was approved by the Institutional Review Board Committee at the Aichi Medical University (H2015-H359). Written informed consent was obtained from the parents. All study evaluations and procedures were performed in accordance with the Declaration of Helsinki.
Description of Mutations in MCT8
MCT8 encodes two potential proteins comprising of 539 and 613 amino acids depending on the use of two alternative translational start sites in exon 1 (1). Since MCT8 mutations have traditionally been numbered based on the long MCT8 protein, we adhered to this system (1, 16).
Review of the Literature
We also reviewed previously published cases of MCT8 deficiency reported from Japan. Case reports of Japanese patients with MCT8 deficiency were identified in PubMed and Ichushi-Web (Japanese database of medical literature updated by the Japan Medical Abstracts Society) using the following search terms: Allan–Herndon–Dudley syndrome; MCT8; monocarboxylate transporter 8; SLC16A2. Articles written in English or Japanese published between 2003 and 2020, which described patients with a genetically confirmed MCT8 mutation, were retrieved and the phenotypic and genotypic descriptions were analyzed. If a mutation was described based on a short protein, it was converted to a long protein, as described in the previous section (Description of mutations in MCT8).
Regional Evaluation on MRI
The initial and current MRI of the four cases and all MRI images available from the published literature were re-evaluated in terms of myelination by two pediatric neurologists. It should be noted that all MRI images of our study's cases were available, whereas for the cases reported in the literature, only those published in the paper were available. Sequential MRIs were available for ten patients. First, two pediatricians, AO and HI, who are authors of this study independently interpreted the MRI images of our four cases and those available in the literature. Subsequently, a consensus on the interpretation was reached after discussion. Cerebellar WM, posterior limb of the internal capsule, anterior limb of the internal capsule, optic radiation, genu corpus callosum, splenium corpus callosum, deep and subcortical occipital WM, deep and subcortical midfrontal WM, deep and subcortical anterior frontal matter, and centrum semiovale were selected for regional evaluation. Signal intensity of WM on T1WI was classified into four grades: high, partial high, iso, and low. Signal intensity of WM on T2WI was classified into four grades: high, iso, partial low, and low. The myelinated appearance was defined as WM showing high intensity on T1WI and low intensity on T2WI.
Results
Myelination Status in the Brain MRI Studies of Four Cases
In Case 1, optic radiation was myelinated at the age of 8 months (Figures 1A,B). However, other regions were not myelinated (Table 1). At the age of 3 years, MRI signal of myelination was seen in all regions except for the anterior limb of the internal capsule, and the deep and subcortical WM (Figures 1C,D; Table 1).
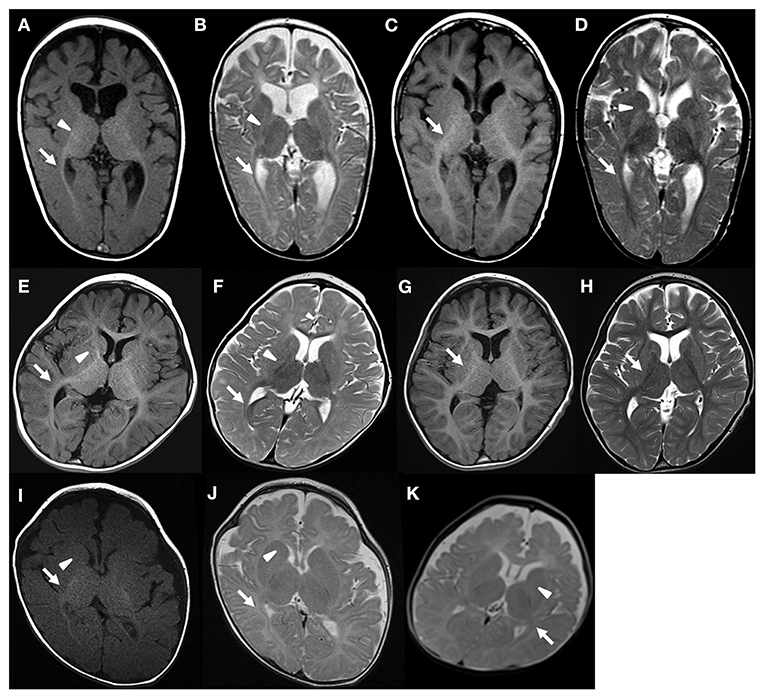
Figure 1. Brain MRI of patient 1 (A,B) 8 months, (C,D) 3 years and 1 months, patient 2 (E,F) 1 year and 4 months, (G,H) 6 years, patient 3 (I,J) 5 months, and patient 4 (K) 6 months. Arrow and arrow head indicates myelination and lack of myelination, respectively. The regional myelination status can be found in Table 1.
In Case 2, the cerebellar WM, the posterior limb of the internal capsule, and the optic radiation showed MRI signal of myelination at the age of 16 months (Figures 1E,F). Other regions were not myelinated (Table 1). Brain MRI at the age of 6 years showed myelination of all regions (Figures 1G,H).
In Case 3, MRI signal of myelination was observed in the cerebellar WM, posterior limb of the internal capsule, the optic radiation, splenium corpus, and centrum semiovale at the age of 5 months (Figures 1I,J; Table 1). In Case 4, the cerebellar WM, posterior limb of the internal capsule, and optic radiation showed myelination at the age of 6 months (Figure 1K; Table 1). Follow-up MRI was not available for Cases 3 and 4.
Review of MRI Images
We identified a total of 36 Japanese patients with MCT8 deficiency from 25 families (Table 2). The median age of patients at the time of reporting was 6.5 years. The age at which MRI and other tests, including TFT, were performed varied in each case. TFT revealed slightly elevated TSH, elevated FT3, and decreased FT4 levels. We reviewed myelination status on T1WI and T2WI. MRI images were available for 30 patients. MRI was performed at the age of <2 years in 13 patients, 2–4 years in 6 patients, 4–6 years in 3 patients, and >6 years in 8 patients (Table 3).
On T1WI performed at the age of <2 years, cerebellar WM and centrum semiovale showed high intensity in all patients and posterior limb of internal capsule and optic radiation showed high intensity in most patients. The other regions infrequently exhibited high intensity. In contrast, all regions showed high intensity on T1WI at the age of ≥2 years.
On T2WI performed at the age of <2 years, low intensity was mostly restricted to the cerebellar WM, corpus callosum, posterior limb of the internal capsule, and optic radiation. At the age of 2–4 years, additional low intensity was observed in centrum semiovale. At the age of 4–6 years, low intensity appeared in anterior limb of the internal capsule and deep occipital WM in 2 of 3 patients, and in subcortical occipital, and anterior frontal WM in 1 of 3 patients. At the age of ≥6 years, low intensity was mostly seen in all regions other than the deep anterior WM.
After integration of T1WI and T2WI sequences, cerebellar WM, posterior limb of the internal capsule, and optic radiation were myelinated at the age of <2 years. Then, centrum semiovale and corpus callosum were myelinated at the age of 2–4 years. At 4–6 years of age, anterior limb of the internal capsule and the occipital deep WM had become myelinated. At age >6 years, most regions were found to be myelinated. Considering only T2WI, 50% of patients aged >6 years showed low intensity in the deep anterior WM (Table 3), indicating that myelination was particularly delayed in that area.
Discussion
We examined regional differences in myelination in four patients with MCT8 deficiency. At the age of <2 years, MRI signal of myelination was observed mainly in the cerebellar WM, posterior limb of the internal capsule, and the optic radiation. There was gradual increase in myelination with further increase in age. On review of MRI images of previously reported Japanese patients, the cerebellar WM, posterior limb of the internal capsule, and optic radiation were myelinated by the age of 2 years, followed by centrum semiovale and corpus callosum by the age of 4 years. Thereafter, most regions with the exception of deep anterior WM showed MRI signal of myelination at the age of 6 years.
Myelination in Patients With MCT8 Deficiency
Postnatal myelination begins in the cerebellar WM (14–16). In the next few months, posterior limb of the internal capsule, optic radiation, genu corpus callosum, and splenium corpus callosum are myelinated. The anterior limb of the internal capsule is myelinated a little later, by 7–11 months. Myelination progresses from deep and postnatal myelination begins in the cerebellar occipital lobe to the frontal lobe, consecutively. Finally, myelination of the subcortical anterior frontal WM is completed within 2–2.5 years of age.
One of the difficulties in the cases of MCT8 deficiency is that myelination is extremely slow and is therefore often classified as true hypomyelination. In fact, it might take years, but in some cases, myelin restores eventually, against expectations. Autopsy of MCT8-deficient fetus showed a delay in cortical and cerebellar myelination (32). The expression of myelin basic protein (MBP), which is important in the process of myelination, was found to be very low or absent in the MCT8-deficient fetal cerebellum, compared to the control fetus.
Vancamp et al. reviewed data on all literature cases available at that time (6). Abnormal or delayed myelination was reported in 26 out of 31 (84%) patients with MCT8 deficiency aged ≤ 2 years (6). Among patients in the age group of 2–6 years, 63% displayed some form of myelination delay. In our study, delayed myelination was observed in all patients aged <6 years. After the age of 6 years, 67% of patients caught up with the delay and showed full myelination, whereas 33% of patients did not show full myelination but showed partial myelination (6). Remerand et al. reported that out of ten patients with MCT8 deficiency aged >6 years, three had hypomyelination (5). In our study, low intensity on T2WI was not observed in some patients aged <6 years. These data indicated is the presence of patient heterogeneity in myelination after the age of 6 years.
Other papers report subregion differences in myelination (5, 33). Remerand et al. performed regional examination such as enlarged ventricular spaces and hypoplasia of the corpus callosum or cerebellum (5). They also reported a general view of T2 hypersignal on MRI. Matheus et al. reported the myelination status based on the anatomy of WM (33). A comprehensive analysis of myelination status based on WM anatomy will help understand myelination in MCT8 deficiency.
Autopsy study of an 11-year-old boy with MCT8 deficiency showed deficient myelination (32). MRI performed at the age of 6.5 years did not show delayed myelination, although hypomyelination was observed on histopathology at the age of 11 years. Paler MBP staining in the cerebellum was observed in this patient as compared to the control subject. Several other reports have indicated persistence of hypomyelinated areas in some regions in later life (6).
High-resolution MRI detected abnormalities in WM throughout adolescence, suggesting permanent hypomyelination (6). These facts indicate that MRI signal of myelination do not necessarily imply histopathologically complete myelination. Diffusion tensor imaging is reportedly useful in the evaluation of demyelination disease (34) or leukodystrophy (35). More advanced methods for assessment of myelination such as diffusion tensor imaging may help clarify minute abnormalities in myelination in patients with MCT8 deficiency.
Difference of Myelination According to the Region of the Brain
The myelination status varies in different regions of the brain. In normal brain, MRI signal of myelination is observed in the cerebellar WM, posterior limb of the internal capsule, and the optic radiation by 2 years of age. Myelination of corpus callosum and anterior limb of the internal capsule is observed by the age of 1 year. Subsequently, myelination of WM becomes obvious initially in the deep WM followed by subcortical WM. This study showed the sequential pattern of myelination in patients with MCT8 deficiency was largely similar to that in the normal brain. However, it is remarkable that the MRI signal of myelination was not seen in the deep anterior WM even at the age of 6 years, although subcortical WM showed myelination. T2WI in a 6-year-old patient with MCT8 deficiency showed iso-intensity in the deep anterior WM (33). Conversely, T2WI in a patient with the same age showed low intensity in the deep anterior WM (5).
The primary function of the deep anterior WM is cognition (36). The examples that we have include patients with frontal lobotomy who lose their cognitive capability and become “calm” (37). Furthermore, these symptoms are similar to the patients with MCT8 deficiency. Therefore, we believe that this may explain the good nature of MCT8 deficient children.
The absence of myelination in deep anterior WM has also been proposed in Alexander's disease, which presents as a progressive leukodystrophy (38). The demyelination in Alexander's disease differs from that of MCT8 deficiency in terms of progression and having a remarkably high signal on T1WI. Although the reason for the delayed myelination in the deep anterior WM in patients with MCT8 deficiency is not clear, this finding may be unique to MCT8 deficiency and can be a clue to diagnosis. Further studies are required to validate whether delayed myelination in deep anterior WM may be an imaging feature of MCT8 deficiency.
Mechanism of Hypomyelination and Recovery of Myelination in MCT8 Deficiency
Oligodendrocytes are the myelinating glia in the CNS (6, 10, 11). OPCs are established during pre- and perinatal development (6). TH receptors are expressed in the oligodendrocytes in the CNS during embryonic and adult lives (10). The differentiation of OPCs is thought to be regulated by T3 (12). T3 transferred via MCT8 was reported to induce differentiation of OPCs into mature oligodendrocytes, and facilitate the formation of myelin extensions (6, 11). Lack of MCT8 at the OPC level induced reduction of intracellular T3 action, blocking the differentiation of OPCs to mature and myelinating oligodendrocytes (6, 11). Therefore, abnormal myelination in MCT8 deficiency may be caused by the disruption of OPC differentiation.
T3 was reported to drive cascade that regulated the timing of OPC differentiation and remyelination of toxic demyelination (39). 3,5-diiodothyropropionic acid (DITPA), which is the analog of TH, can bypass such a deficiency to salvage OPCs and still promote their maturation toward myelinating oligodendrocyte (11). Besides the CNS, hypomyelination occurs in the peripheral nerve system (PNS) due to the lack of T3 in the cell (10). Schwann and satellite glial cells, which are the glial cells of the PNS, transiently express TH receptor only for limited periods of development and regeneration (10). A previous study reports that T3 administration had a positive effect on remyelination in PNS rodent models of inflammatory-demyelinating diseases (40). These data indicate that hypomyelination in MCT8 deficiency might be reversible in the CNS and PNS.
It is difficult to further discuss the mechanisms of spontaneous recovery of myelination, as demonstrated by MRI, and specifically delayed myelination of deep anterior WM as shown in this study. Contrary to the human MCT8 deficiency, a decrease in myelination in a murine MCT8 deficiency model has been reported as permanent (41). In addition, no in vitro or animal studies have demonstrated specifically delayed myelination of deep anterior WM. Therefore, the mechanism of spontaneous recovery of myelination and specifically delayed myelination of deep anterior WM remains to be clarified.
Possible Treatments for MCT8 Deficiency
Physiological or high doses of TH administered postnatally, for most patients even a few years after birth, could not correct hypothyroidism in the brain and psychomotor retardation in MCT8 deficiency (8). Research on several TH analogs, such as DITPA or 3, 3', 5-tri-iodothyroacetic acid (Triac), are still ongoing. DITPA is relatively MCT8 independent for entry into the brain of the MCT8-deficient mouse model (8). Progression in psychomotor development was observed in 2 of 4 patients treated with 22 months of DITPA (42), although their advancement remained at about the same level when expressed as percentage of the chronological age. In two cases with improvement, MRI at 47 months of age showed delayed myelination, which normalized at 62 months of age. It has been reported that motor function in children younger than 4 years treated with Triac was improved (43); however, due to the lack of a control group and the open-label study design, whether the improvement was due to Triac remains unclear. Pre- and post-treatment MRI findings were not reported in the study. Prenatal treatment of intra-amniotic instillation of levothyroxine induced neurodevelopmental improvement and near-normal myelination in the MRI (44). The timing and route of administration, as well as the type of drug, may affect the prognosis. These types of information provide a deeper understanding of myelination in MCT8 deficiency.
Limitations of This Study
This study had several limitations. First, some of the articles reviewed reported T2WI only. If MRI images were limited to T2WI, fewer MRI images were analyzed. Table 3 shows high intensity at T1WI, low intensity at T2WI, and myelination on MRI. High intensity on T1WI and low intensity on T2WI were considered myelination. However, since imaging changes of myelination occur later in T2 than in T1, even the case of low intensity at T2WI alone was considered as myelination when considering deep anterior WM. Therefore, results in this study may be slightly different from the actual percentage of myelination. Second, due to limited research resources, we evaluated four cases and representative MRI images reported in the literature. The myelination of regions not reported in the literature was evaluated only in our cases. Therefore, results in these regions may not be generalizable. For future study, we plan to centrally evaluate images from Japanese patients with MCT8 deficiency.
Conclusion
The present study demonstrated regional differences in myelination in patients with MCT8 deficiency based on MRI. The sequential pattern of myelination in different regions of brain in MCT8 deficiency was largely similar to that in normal brain; however, delayed myelination in deep anterior WM was a remarkable feature of MCT8 deficiency. Further studies are required to characterize the imaging features of patients with MCT8 deficiency.
Data Availability Statement
The original contributions presented in the study are included in the article/supplementary material, further inquiries can be directed to the corresponding author/s.
Ethics Statement
The studies involving human participants were reviewed and approved by Institutional Review Board Committee at Aichi Medical University. Written informed consent to participate in this study was provided by the participants' legal guardian/next of kin.
Author Contributions
HI contributed to the study design, interpretation of data, and was a major contributor in writing the manuscript. TT, KA, MM, SA, YF, HMa, and KT contributed to the acquisition and analysis of data from the patients. HMi and AO revised the manuscript critically for important intellectual content. All authors read and approved the final manuscript.
Funding
The research of HI was supported by JSPS KAKENHI (Grant Nos. 16K19676 and 18K15691), The Nitto Foundation, Aichi Medical University Aikei-Kai Foundation, The Japanese Society for Pediatric Endocrinology Future Development Grant, Forum on Growth Hormone Research Grant, and the Yoshiko & Seizo Foundation.
Conflict of Interest
The authors declare that the research was conducted in the absence of any commercial or financial relationships that could be construed as a potential conflict of interest.
Acknowledgments
We thank Masatsune Itoh from Kanazawa Medical University for the genetic analysis of MCT8.
References
1. Dumitrescu AM, Liao XH, Best TB, Brockmann K, Refetoff S. A novel syndrome combining thyroid and neurological abnormalities is associated with mutations in a monocarboxylate transporter gene. Am J Hum Genet. (2004) 74:168–75. doi: 10.1086/380999
2. Friesema EC, Grueters A, Biebermann H, Krude H, von Moers A, Reeser M, et al. Association between mutations in a thyroid hormone transporter and severe X-linked psychomotor retardation. Lancet. (2004) 364:1435–7. doi: 10.1016/S0140-6736(04)17226-7
3. Schwartz CE, May MM, Carpenter NJ, Rogers RC, Martin J, Bialer MG, et al. Allan-herndon-dudley syndrome and the monocarboxylate transporter 8 (MCT8) gene. Am J Hum Genet. (2005) 77:41–53. doi: 10.1086/431313
4. Groeneweg S, van Geest FS, Abaci A, Alcantud A, Ambegaonkar GP, Armour CM, et al. Disease characteristics of MCT8 deficiency: an international, retrospective, multicentre cohort study. Lancet Diabetes Endocrinol. (2020) 8:594–605. doi: 10.1016/S2213-8587(20)30153-4
5. Remerand G, Boespflug-Tanguy O, Tonduti D, Touraine R, Rodriguez D, Curie A, et al. Expanding the phenotypic spectrum of allan-herndon-dudley syndrome in patients with SLC16A2 mutations. Dev Med Child Neurol. (2019) 61:1439–47. doi: 10.1111/dmcn.14332
6. Vancamp P, Demeneix BA, Remaud S. Monocarboxylate transporter 8 deficiency: delayed or permanent hypomyelination? Front Endocrinol. (2020) 11:283. doi: 10.3389/fendo.2020.00283
7. Azzolini S, Nosadini M, Balzarin M, Sartori S, Suppiej A, Mardari R, et al. Delayed myelination is not a constant feature of allan-herndon-dudley syndrome: report of a new case and review of the literature. Brain Dev. (2014) 36:716–20. doi: 10.1016/j.braindev.2013.10.009
8. Friesema EC, Visser WE, Visser TJ. Genetics and phenomics of thyroid hormone transport by MCT8. Mol Cell Endocrinol. (2010) 322:107–13. doi: 10.1016/j.mce.2010.01.016
9. Roberts LM, Woodford K, Zhou M, Black DS, Haggerty JE, Tate EH, et al. Expression of the thyroid hormone transporters monocarboxylate transporter-8 (SLC16A2) and organic ion transporter-14 (SLCO1C1) at the blood-brain barrier. Endocrinology. (2008) 149:6251–61. doi: 10.1210/en.2008-0378
10. Barakat-Walter I, Kraftsik R. Stimulating effect of thyroid hormones in peripheral nerve regeneration: research history and future direction toward clinical therapy. Neural Regen Res. (2018) 13:599–608. doi: 10.4103/1673-5374.230274
11. Lee JY, Kim MJ, Deliyanti D, Azari MF, Rossello F, Costin A, et al. Overcoming monocarboxylate transporter 8 (MCT8)-deficiency to promote human oligodendrocyte differentiation and myelination. EBioMedicine. (2017) 25:122–35. doi: 10.1016/j.ebiom.2017.10.016
12. Domingues HS, Portugal CC, Socodato R, Relvas JB. Oligodendrocyte, astrocyte, and microglia crosstalk in myelin development, damage, and repair. Front Cell Dev Biol. (2016) 4:71. doi: 10.3389/fcell.2016.00071
13. Barres BA, Lazar MA, Raff MC. A novel role for thyroid hormone, glucocorticoids and retinoic acid in timing oligodendrocyte development. Development. (1994) 120:1097–108. doi: 10.1242/dev.120.5.1097
14. Cowan FM. Magnetic resonance imaging of the normal infant brain: term to 2 years. In: Rutherford M, editor. MRI of the Neonatal Brain. London: WB Saunders (2002).
15. Barkovich MJ, Barkovich AJ. Normal development of the fetal, neonatal, and infant brain, skull, and spine. In: Barkovich AJ, Raybaud C, editors. Pediatric Neuroimaging, 6th ed. Philadelphia, PA: Wolters Kluwer (2019). p. 18–80.
16. Namba N, Etani Y, Kitaoka T, Nakamoto Y, Nakacho M, Bessho K, et al. Clinical phenotype and endocrinological investigations in a patient with a mutation in the MCT8 thyroid hormone transporter. Eur J Pediatr. (2008) 167:785–91. doi: 10.1007/s00431-007-0589-6
17. Sarret C, Oliver Petit I, Tonduti D. Allan-herndon-dudley syndrome. In: GeneReviews. Available online at: https://www.ncbi.nlm.nih.gov/books/NBK26373/
18. Vaurs-Barrière C, Deville M, Sarret C, Giraud G, Des Portes V, Prats-Viñas JM, et al. Pelizaeus-merzbacher-like disease presentation of MCT8 mutated male subjects. Ann Neurol. (2009) 65:114–8. doi: 10.1002/ana.21579
19. Yamamoto T, Takanashi J, Kurosawa K, Deguchi K, Osaka H, Inoue K. delayed myelination is not a constant feature of allan-herndon-dudley syndrome: report of a new case and review of the literature. Brain Dev. (2015) 37:988–9. doi: 10.1016/j.braindev.2015.03.007
20. Kakinuma H, Itoh M, Takahashi H. A novel mutation in the monocarboxylate transporter 8 gene in a boy with putamen lesions and low free T4 levels in cerebrospinal fluid. J Pediatr. (2005) 147:552–4. doi: 10.1016/j.jpeds.2005.05.012
21. Tsurusaki Y, Osaka H, Hamanoue H, Shimbo H, Tsuji M, Doi H, et al. Rapid detection of a mutation causing X-linked leucoencephalopathy by exome sequencing. J Med Genet. (2011) 48:606–9. doi: 10.1136/jmg.2010.083535
22. Goto M, Ito K, Okamoto N, Sato N, Sasaki M. Cerebral blood flow on (99m) Tc ethyl cysteinate dimer SPECT in 2 siblings with monocarboxylate transporter 8 deficiency. Clin Nucl Med. (2013) 38:e276–8. doi: 10.1097/RLU.0b013e31827082d8
23. Yamamoto S, Okuhara K, Tonoki H, Iizuka S, Nihei N, Tajima T. A novel deletion mutation of SLC16A2 encoding monocarboxylate transporter (MCT) 8 in a 26-year-old japanese patient with allan-herndon-dudley syndrome. Clin Pediatr Endocrinol. (2013) 22:83–6. doi: 10.1297/cpe.22.83
24. Yamamoto T, Shimojima K, Umemura A, Uematsu M, Nakayama T, Inoue K. SLC16A2 mutations in two Japanese patients with allan-herndon-dudley syndrome. Hum Genome Var. (2014) 1:14010. doi: 10.1038/hgv.2014.10
25. Kobayashi S, Onuma A, Inui T, Wakusawa K, Tanaka S, Shimojima K, et al. Clinical course and images of four familial cases of allan-herndon-dudley syndrome with a novel monocarboxylate transporter 8 gene mutation. Pediatr Neurol. (2014) 51:414–6. doi: 10.1016/j.pediatrneurol.2014.05.004
26. Morimoto K, Ito M, Yoshida T, Seto M. A case of MCT8 deficiency in an infant with a novel mutation (in Japanese). J Jpn Pediat Soc. (2014) 118:371.
27. Ono E, Ariga M, Oshima S, Hayakawa M, Imai M, Ochiai Y, et al. Three novel mutations of the MCT8 (SLC16A2) gene: individual and temporal variations of endocrinological and radiological features. Clin Pediatr Endocrinol. (2016) 25:23–35. doi: 10.1297/cpe.25.23
28. Shimojima K, Maruyama K, Kikuchi M, Imai A, Inoue K, Yamamoto T. Novel SLC16A2 mutations in patients with allan-herndon-dudley syndrome. Intractable Rare Dis Res. (2016) 5:214–7. doi: 10.5582/irdr.2016.01051
29. Yamamoto T, Lu Y, Nakamura R, Shimojima K, Kira R. Novel A178P mutation in SLC16A2 in a patient with allan-herndon-dudley syndrome. Congenit Anom. (2018) 58:143–4. doi: 10.1111/cga.12251
30. Honda Y, Haneda K. A case of severe developmental delay due to poor feeding immediately after birth, diagnosed as MCT8 deficiency by genetic analysis (in Japanese). J Jpn Soc Perinat Neonat Med. (2017) 53:749.
31. Islam MS, Namba N, Ohata Y, Fujiwara M, Nakano C, Takeyari S, et al. Functional analysis of monocarboxylate transporter 8 mutations in Japanese allan-herndon-dudley syndrome patients. Endocr J. (2019) 66:19–29. doi: 10.1507/endocrj.EJ18-0251
32. López-Espíndola D, Morales-Bastos C, Grijota-Martínez C, Liao XH, Lev D, Sugo E, et al. Mutations of the thyroid hormone transporter MCT8 cause prenatal brain damage and persistent hypomyelination. J Clin Endocrinol Metab. (2014) 99:E2799–804. doi: 10.1210/jc.2014-2162
33. Matheus MG, Lehman RK, Bonilha L, Holden KR. Redefining the pediatric phenotype of X-linked monocarboxylate transporter 8 (MCT8) deficiency: implications for diagnosis and therapies. J Child Neurol. (2015) 30:1664–8. doi: 10.1177/0883073815578524
34. Waldman AT, Sollee JR, Datta R, Lavery AM, Liu G, Aleman TS, et al. Structural correlates of atypical visual and motor cortical oscillations in pediatric-onset multiple sclerosis. Hum Brain Mapp. (2020) 41:4299–313. doi: 10.1002/hbm.25126
35. Ashrafi MR, Amanat M, Garshasbi M, Kameli R, Nilipour Y, Heidari M, et al. An update on clinical, pathological, diagnostic, and therapeutic perspectives of childhood leukodystrophies. Expert Rev Neurother. (2020) 20:65–84. doi: 10.1080/14737175.2020.1699060
36. Schmahmann JD, Smith EE, Eichler FS, Filley CM. Cerebral white matter: neuroanatomy, clinical neurology, and neurobehavioral correlates. Ann N Y Acad Sci. (2008) 1142:266–309. doi: 10.1196/annals.1444.017
37. Mashour GA, Walker EE, Martuza RL. Psychosurgery: past, present, and future. Brain Res Brain Res Rev. (2005) 48:409–19. doi: 10.1016/j.brainresrev.2004.09.002
38. van der Voorn JP, Pouwels PJ, Salomons GS, Barkhof F, van der Knaap MS. Unraveling pathology in juvenile alexander disease: serial quantitative MR imaging and spectroscopy of white matter. Neuroradiology. (2009) 51:669–75. doi: 10.1007/s00234-009-0540-9
39. Dugas JC, Ibrahim A, Barres BA. The T3-induced gene KLF9 regulates oligodendrocyte differentiation and myelin regeneration. Mol Cell Neurosci. (2012) 50:45–57. doi: 10.1016/j.mcn.2012.03.007
40. D'Intino G, Lorenzini L, Fernandez M, Taglioni A, Perretta G, Del Vecchio G, et al. Triiodothyronine administration ameliorates the demyelination/remyelination ratio in a non-human primate model of multiple sclerosis by correcting tissue hypothyroidism. J Neuroendocrinol. (2011) 23:778–90. doi: 10.1111/j.1365-2826.2011.02181.x
41. Mayerl S, Müller J, Bauer R, Richert S, Kassmann CM, Darras VM, et al. Transporters MCT8 and OATP1C1 maintain murine brain thyroid hormone homeostasis. J Clin Invest. (2014) 124:1987–99. doi: 10.1172/JCI70324
42. Verge CF, Konrad D, Cohen M, Di Cosmo C, Dumitrescu AM, Marcinkowski T, et al. Diiodothyropropionic acid (DITPA) in the treatment of MCT8 deficiency. J Clin Endocrinol Metab. (2012) 97:4515–23. doi: 10.1210/jc.2012-2556
43. Groeneweg S, Peeters RP, Moran C, Stoupa A, Auriol F, Tonduti D, et al. Effectiveness and safety of the tri-iodothyronine analogue triac in children and adults with MCT8 deficiency: an international, single-arm, open-label, phase 2 trial. Lancet Diabetes Endocrinol. (2019) 7:695–706. doi: 10.1016/S2213-8587(19)30155-X
Keywords: magnetic resonance imaging, thyroid hormone transporter, white matter, Allan-Herdon-Dudlley syndrome, reginal analysis, delayed myelination, monocarboxylate transporter 8 deficiency
Citation: Iwayama H, Tanaka T, Aoyama K, Moroto M, Adachi S, Fujisawa Y, Matsuura H, Takano K, Mizuno H and Okumura A (2021) Regional Difference in Myelination in Monocarboxylate Transporter 8 Deficiency: Case Reports and Literature Review of Cases in Japan. Front. Neurol. 12:657820. doi: 10.3389/fneur.2021.657820
Received: 27 February 2021; Accepted: 03 June 2021;
Published: 15 July 2021.
Edited by:
Alessandro Simonati, University of Verona, ItalyReviewed by:
Pieter Vancamp, Center National de la Recherche Scientifique (CNRS), FranceW. Edward Visser, Erasmus University Rotterdam, Netherlands
Copyright © 2021 Iwayama, Tanaka, Aoyama, Moroto, Adachi, Fujisawa, Matsuura, Takano, Mizuno and Okumura. This is an open-access article distributed under the terms of the Creative Commons Attribution License (CC BY). The use, distribution or reproduction in other forums is permitted, provided the original author(s) and the copyright owner(s) are credited and that the original publication in this journal is cited, in accordance with accepted academic practice. No use, distribution or reproduction is permitted which does not comply with these terms.
*Correspondence: Hideyuki Iwayama, aXdhaGlkZTE5NzZAZ21haWwuY29t