- Stroke and Ageing Research (STAR), Department of Medicine, School of Clinical Sciences at Monash Health, Monash University, Clayton, VIC, Australia
Background and purpose: Computed tomography perfusion (CTP) has successfully extended the time window for reperfusion therapies in ischemic stroke. However, the published perfusion parameters and thresholds vary between studies. Using Preferred Reporting Items for Systematic Reviews and Meta-Analyses of Diagnostic Test Accuracy Studies (PRISMA-DTA) guidelines, we conducted a systematic review to investigate the accuracy of parameters and thresholds for identifying core and penumbra in adult stroke patients.
Methods: We searched Medline, Embase, the Cochrane Library, and reference lists of manuscripts up to April 2022 using the following terms “computed tomography perfusion,” “stroke,” “infarct,” and “penumbra.” Studies were included if they reported perfusion thresholds and undertook co-registration of CTP to reference standards. The quality of studies was assessed using the Quality Assessment of Diagnostic Accuracy Studies-2 (QUADAS-2) tool and Standards for Reporting of Diagnostic Accuracy (STARD) guidelines.
Results: A total of 24 studies were included. A meta-analysis could not be performed due to insufficient data and significant heterogeneity in the study design. When reported, the mean age was 70.2 years (SD+/−3.69), and the median NIHSS on admission was 15 (IQR 13–17). The perfusion parameter identified for the core was relative cerebral blood flow (rCBF), with a median threshold of <30% (IQR 30, 40%). However, later studies reported lower thresholds in the early time window with rapid reperfusion (median 25%, IQR 20, 30%). A total of 15 studies defined a single threshold for all brain regions irrespective of collaterals and the gray and white matter.
Conclusion: A single threshold and parameter may not always accurately differentiate penumbra from core and oligemia. Further refinement of parameters is needed in the current era of reperfusion therapy.
Introduction
Perfusion imaging has revolutionized hyperacute stroke care, enabling a move beyond the traditional 4.5-h threshold and toward a tissue-based approach to treatment and precision medicine in stroke care (1). Perfusion imaging aims to aid decision-making by identifying the tissue at risk of infarction that is still viable, ‘ischemic penumbra,’ (2) and differentiate it from the tissue that has already infarcted, ‘core.’ Therefore, permitting treatment of those who would benefit from reperfusion therapies and avoiding unnecessary harm. At present, the optimal perfusion parameter to identify ‘core’ is thought to be relative cerebral blood flow (rCBF) < 30%, and a mismatch between this and a time to maximum (Tmax) > 6 s is operationally defined as ischemic penumbra (3). Although these perfusion parameters and thresholds have been used to successfully treat patients in randomized controlled trials in the extended time window (1, 4–6), recent reports have questioned the diagnostic accuracy of rCBF <30% in identifying the volume of infarct (7).
Previous systematic reviews investigating parameters and thresholds have included studies using magnetic resonance perfusion-weighted imaging (MR-PWI) (8, 9) or positron emission tomography (PET) (9) as well as CT perfusion (CTP). A recent meta-analysis (10) examining volumetric and spatial accuracy of CTP for identifying core did not account for the multiple thresholds reported for each parameter (11) and included several studies that failed to perform co-registration of CTP to the reference standard. Co-registration is essential to ensure the accuracy of volumetric analysis as images are aligned in the same coordinate space (12). The purpose of this systematic review is to critically evaluate the diagnostic accuracy of CTP parameters and thresholds to differentiate penumbra from core and oligemia in adult stroke patients.
Methods
Search strategy
This systematic review of the literature was performed according to the Preferred Reporting Items for Systematic Reviews and Meta-Analyses of Diagnostic Test Accuracy Studies (PRISMA-DTA) guidelines (13) and the Cochrane Database of Systematic Reviews (14). We conducted a standardized search in Medline, Embase, and the Cochrane Library using the terms “computed tomography perfusion,” “stroke,” “infarct core,” and “penumbra” for relevant studies published in peer-reviewed journals up to April 2022. We screened all titles and abstracts and removed duplication. For each eligible study, full-text papers were obtained. Reference lists from previous systematic reviews and meta-analyses were searched for additional studies. Relevant studies were assessed independently by two authors (T.T., S.A.), and discrepancies were resolved by a third reviewer (T.P.).
Selection criteria
Original articles were included of studies investigating CTP within 24 h of symptom onset, using different perfusion parameters to define infarcted ‘core’ from the salvageable tissue or ‘penumbra’ and tissue which is hypoperfused but not at risk of infarction, ‘benign oligemia.’ We only included studies that reported results of (1) adult acute ischemic stroke patients; (2) published in English; (3) with sample sizes above five; (4) investigated multiple thresholds; (5) used CTP as the index test; (6) used follow-up MR diffusion-weighted imaging (DWI), MRI-flair, and/or non-contrast CT (NCT) as the reference standard; and (7) co-registered index and reference images. The exclusion criteria included (1) studies using animal models or children and (2) studies using other modalities to assess perfusion such as MR-PWI, single photon emission computed tomography (SPECT), or PET.
Data extraction and analysis
Using a structured template, the following data were extracted: first author’s name, publication year, study setting and design, number of subjects, baseline demographic data of study population, time between symptom onset and CTP imaging, presence of large vessel occlusion, reference imaging standard, time to reference imaging, use of reperfusion therapies between index and reference test, and time to reperfusion. Information relating to imaging protocol including CTP acquisition, post-processing software, perfusion parameters and thresholds investigated, methods for imaging, and statistical analysis was also collected. Data were extracted independently by two reviewers (T.T. and S.A.), with disagreements resolved by a third reviewer (T.P.). We contacted the corresponding author to obtain further information if data were not easily extractable. Parameters and thresholds with the highest AUC were identified for penumbra and core in each study. If not provided, the Youden index was calculated as the sum of sensitivity and specificity minus one (values range from 0 to 1). In this way, the Youden index summarizes the performance of a test at the optimal cutoff threshold by taking into account the sensitivity and specificity. A Youden index value of less than 0.5 does not meet the optimal performance standard for a diagnostic test (15, 16). For studies that used both acute and delayed MRI to define infarct core, we have only reported results for acute DWI imaging.
Quality assessment
The Quality Assessment of Diagnostic Accuracy Studies-2 (QUADAS-2) tool (17) was used to assess the methodological quality of included studies. Two different authors assessed the methodological quality of the studies (A.L. and C.D.).
Results
Our MEDLINE, EMBASE, and Cochrane searches yielded 1,509 results. Manual searching yielded an additional 23 studies. We screened 1,426 records using the title and abstract. In total, 186 articles were screened by full text for eligibility. A total of 70 articles were reviewed, and 24 fulfilled the inclusion criteria (see Figure 1). Despite contacting authors, we were unable to determine the exact details of overlapping patients between studies published by the same research groups (18–23). Studies that may have reported results of the same patients were counted once unless they examined different parameters and thresholds for the gray and white matter (24, 25), post-processing software (16, 20, 26–28), brain coverage (19, 23), and the impact of reperfusion therapies (18, 20). A list of excluded studies and reasons for exclusion are available in Supplementary material. Nineteen studies, including three studies that used RAPID® software (29–31), were excluded because the co-registration of index and reference images was not conducted (32–38). Two additional studies using RAPID® software were excluded because they used a combination of MR-PWI and CTP as the index test (39, 40). A meta-analysis was not performed due to insufficient data and significant heterogeneity in methodological characteristics between studies. Results will be discussed under the headings of study design, perfusion parameters, and thresholds.
Study characteristics
The 24 included studies are shown in Table 1. All were observational cohort studies published between 2006 and 2020. Nine were prospective (21, 22, 24, 25, 41–45). When reported, the mean age was 70.2 years (+/−3.69), and the median NIHSS score on admission was 15, interquartile range (IQR) 13–17. Twenty-three studies investigated core (15, 16, 18–27, 41–51), and 13 studies investigated penumbra (15, 16, 19, 20, 22–25, 28, 41, 43, 49, 50). Most studies included less than 50 patients per study (see Tables 1, 2 in Supplementary material). Of these, it is possible that some studies have included patients from the same period (18–23). When reported, median onset to CTP was 182 (IQR 162–196) min for studies investigating core and 164 (IQR 148–184) min for penumbra (see Tables 1, 2 in Supplementary material). Only 10 studies (51) included patients between 6 and 12 h from stroke onset, and no study reported onset to perfusion imaging beyond 12 h.
Study quality
The results of the QUADAS-2 tool (17) are shown in Table 2. Only six studies were classified by both reviewers as having an overall judgment of low risk of bias and low concern regarding applicability (19, 20, 43–46). Only seven studies reported blinding of assessors to analysis of perfusion imaging and the reference test (15, 23, 25, 26, 43, 44, 46). Two studies reported adherence to STARD (52) (Standards for Reporting of Diagnostic Accuracy) guidelines1 (43, 48). One study included a flow diagram outlining patient selection process (23). Others included details of excluded patients in the Results section (15, 16, 18–22, 24–26, 42, 45, 46, 49) or in online data supplement (47).
Study design
Brain coverage, scan acquisition time, and post-processing algorithms
Reported brain coverage, scan acquisition time, and post-processing methods varied between studies (see Table 1). In general, three main deconvolution methods were used. Nine studies used delay and dispersion correction deconvolution models producing delay time (DT) maps with the post-processing software, MIStar (15, 16, 18–22, 48, 49). Six studies used standard or ‘delay sensitive’ (16, 23, 27, 28, 43, 44) deconvolution which does not correct for arrival delay of contrast and six studies used ‘delay-insensitive’ also known as ‘delay corrected’ which does correct for arrival delay of contrast (23, 28, 42, 45–47). Studies investigating the impact of post-processing methods showed thresholds to define core and penumbra varied depending on the deconvolution method used (16, 20, 27, 28).
Time to reference standard
Imaging reference was a combination of MRI and NCT (6 studies) (15, 28, 42, 47, 49, 50), MRI alone (16 studies) (16, 18–23, 26, 27, 41, 44–46, 48, 51), or NCT alone (2 studies) (24, 25). Two studies investigating thresholds for penumbra also used contemporaneous MR-PWI (43) or CTP or MRP at 24 h (15). Time interval to reference varied between study protocols. For studies investigating core, most did not record median or mean time to reference imaging. When reported, median interval to acute MRI was 27.5 (IQR 22–31.5) min (see Table 1; Supplementary material) (16, 21, 22, 26, 27, 41, 44, 46). Other studies used DWI 24 h after CTP in patients with major reperfusion (18–23), delayed MRI, at a median interval of 38 h in patients with complete recanalization (48) or at 5–7 days (45) to determine infarct core. For studies investigating penumbra, several studies did not report median or mean time to reference scan (see Table 2 in Supplementary material).
Vessel occlusion and reperfusion therapies
Seventeen studies included patients with evidence of large vessel occlusion on imaging using CTA, MRA, or DSA (16, 18, 23–25, 27, 28, 41–45, 47–51). When reported, recanalization was determined using three scales: the Mori (50), thrombolysis in myocardial infarction (TIMI) (23, 49), and modified thrombolysis in cerebral infarction (mTICI) scales (18, 42, 48). Reperfusion therapies included intravenous thrombolysis (15, 16, 18–26, 41, 43–46, 50), local intra-arterial fibrinolysis (24, 25, 50), and clot retrieval in combination with thrombolysis (16, 18, 26, 50). Since 2015, three studies examined perfusion parameters following treatment with endovascular clot retrieval alone (42, 47, 48).
Imaging analysis
Imaging analysis performed by studies included either a region of interest analysis (24, 25, 42–44, 47, 48, 50), or as defined by the authors a pixel (18–23, 41, 46, 49) or voxel-based analysis (15, 16, 26–28, 45, 51). The term ‘voxel’ is used to encompass both pixels and voxels. Eleven studies used semi-automatic or automatic delineation of infarct on MRI using signal intensity thresholds (16, 18–23, 27, 28, 46, 48). Others manually outlined the maximal visual extent of the infarct on follow-up imaging (MRI or NCT) (15, 24–26, 41–44, 47, 49–51). Voxels within both the hypoperfusion region on CTP and infarct on DWI or NCT were regarded as “true-positive,” and those within the region of hypoperfusion on the index test but not in the reference test was “false-positive.” Most studies analyzed only the ischemic hemisphere (15, 16, 18–23, 43, 44). One study delineated non-infarcted voxels in the whole brain parenchyma (41). Two studies only examined the section with the largest tissue which evolved to infarction (27, 28).
Two different approaches were employed to determine the ability of perfusion parameters to accurately identify the final infarct on the follow-up scan. The first, a volume analysis approach defined the optimal parameter as the one with the least volume difference between index and reference standard. The second used receiver operator characteristic (ROC) curve analysis to identify parameters with the highest sensitivity and specificity to predict the final infarction on the reference image. Most diagnostic accuracy studies used ROC curve analysis alone (27, 28, 41, 42, 45, 47, 50, 51) or in conjunction with a volume analysis approach (15, 16, 18–22, 24–26, 44, 46, 49). Only one study used a volume analysis approach alone (48). Sensitivity, specificity, area under the curve (AUC), and the Youden index for the optimal parameters for core and penumbra from each study are included in Tables 3 4, respectively. The parameter with the highest Youden index of 0.94 was a product of CBF and CBV which at a threshold of 31.3 differentiated core from penumbra in an early study (24). The Youden index for rCBF <31% to represent ischemic core was less than 0.5 in three out of five studies (median 0.60, IQR 0.48, 0.63) (15, 16, 44).
Perfusion parameters and thresholds
Parameters for core
The most reported parameter was rCBF, described in a total of 16 studies. The median rCBF threshold was <30% (IQR 30–40%). Figure 2 shows that rCBF thresholds varied between studies even from the same research groups. A regression analysis evaluating the relationship between published rCBF thresholds over a 10-year period showed no statistically significant association (regression coefficient: 0.1; p = 0.21) between rCBF threshold values and the publication year (see Figure 2). Five studies constrained rCBF within the perfusion lesion (using TTP > 4 s or DT > 2 s or DT > 3 s) to reduce false positives from regions of leukoaraiosis (15, 20, 22, 23, 43). In the early time window, one study showed thresholds were dependent on the reperfusion method used. They reported a lower threshold of rCBF <20% in patients treated with clot retrieval due to faster reperfusion [see Figure 2; Bivard (18)].
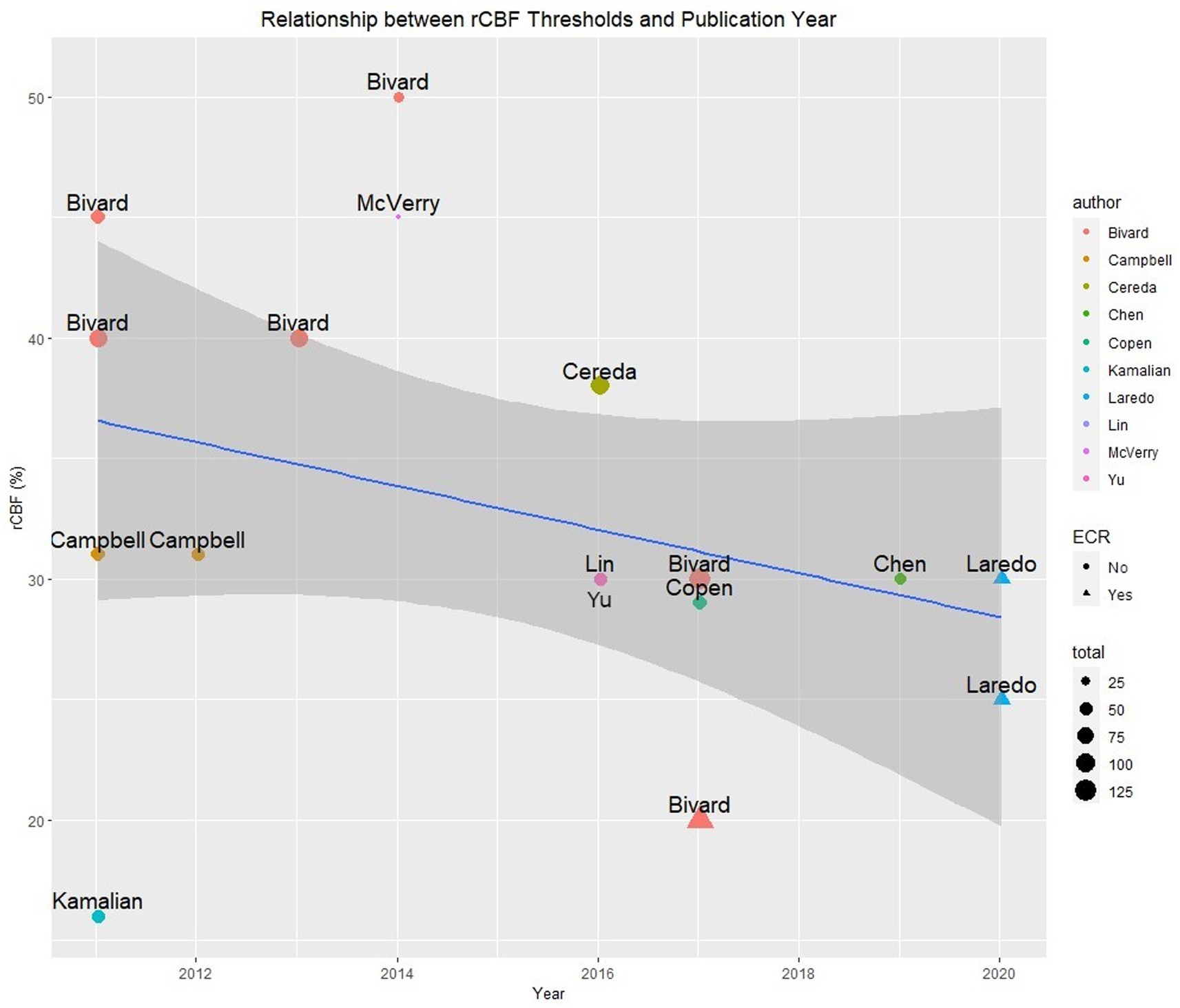
Figure 2. Reported optimal rCBF (%) thresholds to identify core by publication year, sample sizes, author, and reperfusion therapy (triangle—ECR only).
The definition of ‘infarct core’ continued to vary between studies (8, 9), with some defining core using the acute DWI lesion (16, 21, 22, 26, 27, 41, 43, 44, 46, 51), and others using delayed imaging in patients with complete recanalization [TIMI 3 (23, 49), TICI 2b (42, 47), or TICI 3 (42, 47, 48)] and clinical improvement. Five studies defined core in patients with evidence of ‘major’ reperfusion following treatment with thrombolysis or clot retrieval. This was defined as more than 80% reduction in the perfusion lesion volume between acute CTP and 24-h MR imaging, using MTT >145% of the normal tissue (16, 19, 20, 22) or Tmax >6 s (15). Others did not specify the threshold for MTT (21). One study used TICI 3 recanalization and ‘complete’ reperfusion defined as more than 90% reduction from baseline CTP using DT > 3 s (18). One early study defined core as the infarcted tissue on delayed NCT or T2-weighted MRI with reduced CBV and CBF on initial CTP (50). Another early study defined penumbra as the tissue with CBF <25 mL/100 g/min, which did not infarct on delayed NCT and then applied logistic regression to differentiate between penumbra and core in the gray (24) and white (25) matter in recanalized patients.
Parameters for penumbra
Three studies reported Tmax >6 s as the most accurate parameter for penumbra (AUC 0.78–0.87) (16, 23, 43). Using the MIStar software, three studies found DT > 2 s was the most accurate parameter and threshold for penumbra (20, 22, 49). One study (n = 22) reported a higher threshold (DT > 3 s) in a Chinese population (15). Two older studies suggested that relative MTT (28, 41) with thresholds varying from 145 to 249% was optimal for penumbra.
Three different methods were used to define penumbra. One pivotal study (43) used MR-PWI within an hour of CTP and matched thresholds for penumbra to MR-PWI threshold of Tmax >6 s. Five studies defined penumbra using CTP parameters and thresholds to identify ‘no or minimal reperfusion’ (<20% reperfusion) at 24 h (15, 16, 19, 20, 22). Studies defined minimal or no reperfusion using MTT >145% of the normal tissue (16, 19, 20, 22) or Tmax>6 s (15). Three studies used infarct growth between the area of hypoperfusion on CTP and the infarcted region found on 24-h MRI (23, 41) alone or combined with delayed NCT (49) in patients with persistent symptomatic occlusion on follow-up CTA or MRA (23, 41, 49). One study assumed persistent occlusion by including patients with vessel occlusion who in the absence of thrombolysis had no late clinical or radiological confirmation of reperfusion (28). Only two studies investigated thresholds for differentiating penumbra or ‘tissue at risk’ from ‘oligemia.’ (28, 50) Oligemia or ‘penumbra that recovers’ was defined as hypoperfusion on CTP (28) or reduced CBF and normal CBV (50), which did not infarct on delayed imaging.
Perfusion parameters and time
The relationship between thresholds and time to reperfusion was investigated in three studies (42, 47, 48). Two of these studies demonstrated a statistically significant relationship between Tmax and aCBF and time to reperfusion from CTP (42, 47). If reperfusion occurred within 90 min, the optimal Tmax thresholds for the core was higher, and aCBF threshold lower than if reperfusion occurred between 90 and 180 min (42, 47). Optimal threshold values reported for rCBF were also lower if reperfusion occurred within 90 min (42) or when recanalization occurred within 4.5 h of stroke onset (see Figure 2; Laredo 2020) (48).
Thresholds for gray and white matter
Eight studies investigated thresholds for the gray matter (GM) and the white matter (WM) (16, 24, 25, 28, 42, 45, 47, 50). Two early studies reported higher threshold values for the product of CBF and CBV in GM compared to WM (24, 25). For identifying penumbra, studies reported higher thresholds in WM than GM for aMTT (n = 23) (28) and Tmax (n = 31) (16) and lower thresholds in WM than GM for parameters, aCBF, and aCBV (n = 14) (50). However, thresholds for DT were constant (n = 31) (16). For identifying core, no significant difference in Tmax thresholds was reported between GM and WM (42, 47). Two studies reported consistently lower thresholds in WM than GM for CBF (16, 47), but one study (n = 132) did not (42). Reported thresholds for CBV were also generally lower in WM than GM (42, 47). One study reported higher thresholds for rCBF in highly vulnerable brain regions including the caudate body, putamen, and insular ribbon (51).
Discussion
An important finding of this systematic review is that a single parameter and threshold may not always accurately differentiate penumbra from core and oligemia. Although each diagnostic accuracy study has reported an optimal parameter and threshold for core and penumbra as the gold standard, these varied significantly depending on the cohorts investigated. This is especially evident from the studies published by the same research groups which reported different parameters for penumbra (15, 19, 22, 28, 43, 50) and varying rCBF thresholds for core (16, 18–23, 27, 46, 50) (see Figure 2).
In the assessment of a diagnostic test, the results obtained are usually evaluated in an independent cohort of patients to ensure replicability and reliability. Only two studies validated reported thresholds in an independent dataset to confirm their findings (45, 49). Most studies that used small sample sizes did not perform cross-validation or follow the STARD (52) guidelines and continued to be limited by non-blinding to the reference standard. Consequently, the results varied even when published by the same group. The use of time-dependent thresholds for ischemic core, such as rCBF and Tmax, which are impacted by time to reperfusion, and highly effective reperfusion therapies such as thrombectomy may also impact variability (18, 47, 48) (8, 10). Additionally, 15 studies defined a single threshold for all brain regions irrespective of collaterals and the gray and white matter. Differing thresholds seem to exist between the gray and white matter for some parameters (16, 28, 42, 47, 50) and brain regions (51), but further exploration of these findings is needed. Developing maps that overcome the issue of time-dependent thresholds and applying different thresholds for the gray and white matter or brain regions may increase accuracy in determining core infarct volume.
As previously reported, significant heterogeneity in technical imaging parameters including acquisition times, varied pre- and post-processing techniques (15, 28, 53), scan duration, modality and timing of reference standard, time from stroke onset to CTP scan, and multiple definitions for penumbra and core may also contribute to the variability in reported thresholds and parameters. Additionally, patient factors (10) including the presence of white matter disease, collaterals, location of stroke (anterior or posterior), cardiac output, vessel occlusion or stenosis, and hematocrit ratio may also be important. Comparable to studies investigating MR perfusion, most CT perfusion studies were unable to distinguish the penumbral tissue from the benign oligemia and may have overestimated the region of hypoperfusion representative of the penumbra (28, 54).
We have documented sensitivity, specificity, and AUC as reported by the published journal articles or their supplementary pages (see Tables 3, 4). In several cases, the reported values for AUC seem to be dissociated with values for sensitivity and specificity (15, 18, 20, 27, 42, 44, 46, 47, 51). This situation can arise as the AUC is calculated for the range of thresholds for a test, and the sensitivity and specificity of a test are described for a particular threshold. The Youden index was also used to define the performance at the optimal cutoff point. Using the rCBF threshold of 31% to describe the infarct core, the median value of the Youden index was 0.6. In three of the five studies, the calculated Youden index was less than optimal (15).
Study limitations
There are several strengths of this systematic review. First, we followed the guidance outlined by the Cochrane Database of Systematic Reviews and PRISMA-DTA guidelines. Second, we performed an extensive literature search using different electronic databases with two review authors conducting data extraction. Third, the assessment of quality was carried out by two independent review authors using the QUADAS-2 tool (17) for quality assessments. While the QUADAS-2 tool (17) had suggested that 14 studies were classified as high quality, these studies did not fully meet the STARD (52) guidelines (described above). Finally, we only included studies that used co-registration of index images to the reference standard to ensure the accuracy of tissue location and volume analysis.
The following limitations are worth noting. We only included studies published in the English language, and most included studies were retrospective cohort studies, with sample sizes of less than 50 patients which can run the risk of producing an overestimation of sensitivity and specificity. We acknowledge that a limitation of the Youden index is its inability to distinguish a test with a high sensitivity and low specificity from one with low sensitivity and high specificity. Studies that were published from the same study sites and groups may have included the same patients more than once. Despite approaching authors (18–23), we were unable to obtain this information. Only seven studies were blinded which increases the risk of bias in interpretation of the index test and reference results. Due to our selection criteria, we have reported results pertaining to anterior circulation stroke from mostly two post-processing software manufacturers, excluding studies comparing other commonly used post-processing software such as RAPID® (29–31), Vitrea (55), and Sphere (56). Finally, there was significant methodological heterogeneity between studies which unfortunately precluded further analysis.
Conclusion
Although CT perfusion has found its place in clinical practice, clinicians involved in the hyperacute care of stroke patients need to recognize its current limitations. An accurate determination of infarct volume is essential to ensure the appropriate selection of patients for reperfusion therapies and identify patients for transfer to regional stroke centers. This may not always be possible with a single threshold and parameter. Prospectively designed, multicenter diagnostic accuracy studies following STARD (52) guidelines using standardized imaging acquisition and analysis protocols are still needed to improve the accuracy of CT perfusion. Future research may involve thresholds that consider variations in the gray and white matter and identifying parameters that can precisely differentiate the penumbra from the oligemic tissue and core from the penumbra irrespective of time from stroke onset. The creation of voxel maps using the product of rCBF, rCBV, and hypoattenuation in Hounsfield units on NCT may enable a less time-dependent and more accurate determination of the core.
Data availability statement
The original contributions presented in the study are included in the article/Supplementary material, further inquiries can be directed to the corresponding author.
Author contributions
TT: Writing – original draft, Writing – review & editing, Formal analysis. SA: Formal analysis, Writing – review & editing. AL: Investigation, Writing – review & editing, Formal analysis. CD: Investigation, Writing – review & editing, Formal analysis. HM: Conceptualization, Writing – review & editing. TP: Methodology, Writing – review & editing, Conceptualization.
Funding
The author(s) declare that no financial support was received for the research, authorship, and/or publication of this article.
Conflict of interest
The authors declare that the research was conducted in the absence of any commercial or financial relationships that could be construed as a potential conflict of interest.
Publisher’s note
All claims expressed in this article are solely those of the authors and do not necessarily represent those of their affiliated organizations, or those of the publisher, the editors and the reviewers. Any product that may be evaluated in this article, or claim that may be made by its manufacturer, is not guaranteed or endorsed by the publisher.
Supplementary material
The Supplementary material for this article can be found online at: https://www.frontiersin.org/articles/10.3389/fneur.2023.1255526/full#supplementary-material
Abbreviations
CBF, Cerebral blood flow; CBV, Cerebral blood volume; CTP, Computed tomography perfusion; DT, Delay time; MRI, Magnetic resonance imaging; MRI-PWI, Magnetic resonance perfusion-weighted imaging; MTT, Mean transit time; mTICI, Modified thrombolysis in cerebral infarction; NCT, Non-contrast computed tomography; PET, Positron emission tomography; Tmax, Time to maximum; TIMI, Thrombolysis in myocardial infarction.
Footnotes
References
1. Ma, H, Campbell, BCV, Parsons, MW, Churilov, L, Levi, CR, Hsu, C, et al. Thrombolysis guided by perfusion imaging up to 9 hours after onset of stroke. N Engl J Med. (2019) 380:1795–803. doi: 10.1056/NEJMoa1813046
2. Astrup, J, Siesjö, BK, and Symon, L. Thresholds in cerebral ischemia – the ischemic penumbra. Stroke. (1981) 12:723–5. doi: 10.1161/01.STR.12.6.723
3. Vagal, A, Wintermark, M, Nael, K, Bivard, A, Parsons, M, Grossman, AW, et al. Automated ct perfusion imaging for acute ischemic stroke: pearls and pitfalls for real-world use. Neurology. (2019) 93:888–98. doi: 10.1212/WNL.0000000000008481
4. Campbell, BCV, Majoie, C, Albers, GW, Menon, BK, Yassi, N, Sharma, G, et al. Penumbral imaging and functional outcome in patients with anterior circulation ischaemic stroke treated with endovascular thrombectomy versus medical therapy: a meta-analysis of individual patient-level data. Lancet Neurol. (2019) 18:46–55. doi: 10.1016/S1474-4422(18)30314-4
5. Nogueira, RG, Jadhav, AP, Haussen, DC, Bonafe, A, Budzik, RF, Bhuva, P, et al. Thrombectomy 6 to 24 hours after stroke with a mismatch between deficit and infarct. N Engl J Med. (2018) 378:11–21. doi: 10.1056/NEJMoa1706442
6. Albers, GW, Marks, MP, Kemp, S, Christensen, S, Tsai, JP, Ortega-Gutierrez, S, et al. Thrombectomy for stroke at 6 to 16 hours with selection by perfusion imaging. N Engl J Med. (2018) 378:708–18. doi: 10.1056/NEJMoa1713973
7. Martins, N, Aires, A, Mendez, B, Boned, S, Rubiera, M, Tomasello, A, et al. Ghost infarct core and admission computed tomography perfusion: redefining the role of neuroimaging in acute ischemic stroke. Intervent Neurol. (2018) 7:513–21. doi: 10.1159/000490117
8. Dani, KA, Thomas, RG, Chappell, FM, Shuler, K, MacLeod, MJ, Muir, KW, et al. Computed tomography and magnetic resonance perfusion imaging in ischemic stroke: definitions and thresholds. Ann Neurol. (2011) 70:384–401. doi: 10.1002/ana.22500
9. Bandera, E, Botteri, M, Minelli, C, Sutton, A, Abrams, KR, and Latronico, N. Cerebral blood flow threshold of ischemic penumbra and infarct core in acute ischemic stroke: a systematic review. Stroke. (2006) 37:1334–9. doi: 10.1161/01.STR.0000217418.29609.22
10. Lim, NE, Chia, B, Bulsara, MK, Parsons, M, Hankey, GJ, and Bivard, A. Automated ct perfusion detection of the acute infarct core in ischemic stroke: a systematic review and meta-analysis. Cerebrovasc Dis. (2023) 52:97–109. doi: 10.1159/000524916
11. Steinhauser, S, Schumacher, M, and Rucker, G. Modelling multiple thresholds in meta-analysis of diagnostic test accuracy studies. BMC Med Res Methodol. (2016) 16:97. doi: 10.1186/s12874-016-0196-1
12. Thirugnanachandran, T, Ma, H, Singhal, S, Slater, LA, Davis, SM, Donnan, GA, et al. Refining the ischemic penumbra with topography. Int J Stroke. (2018) 13:277–84. doi: 10.1177/1747493017743056
13. McInnes, MDF, Moher, D, Thombs, BD, McGrath, TA, Bossuyt, PM, the P-DTAG, et al. Preferred reporting items for a systematic review and meta-analysis of diagnostic test accuracy studies: the prisma-dta statement. JAMA. (2018) 319:388–96. doi: 10.1001/jama.2017.19163
14. Leeflang, MM, Deeks, JJ, Takwoingi, Y, and Macaskill, P. Cochrane diagnostic test accuracy reviews. Syst Rev. (2013) 2:82.
15. Yu, Y, Han, Q, Ding, X, Chen, Q, Ye, K, Zhang, S, et al. Defining core and penumbra in ischemic stroke: a voxel- and volume-based analysis of whole brain ct perfusion. Sci Rep. (2016) 6:20932. doi: 10.1038/srep20932
16. Chen, C, Bivard, A, Lin, L, Levi, CR, Spratt, NJ, and Parsons, MW. Thresholds for infarction vary between gray matter and white matter in acute ischemic stroke: a ct perfusion study. J Cereb Blood Flow Metab. (2019) 39:536–46. doi: 10.1177/0271678X17744453
17. Whiting, PF, Rutjes, AW, Westwood, ME, Mallett, S, Deeks, JJ, Reitsma, JB, et al. Quadas-2: a revised tool for the quality assessment of diagnostic accuracy studies. Ann Intern Med. (2011) 155:529–36. doi: 10.7326/0003-4819-155-8-201110180-00009
18. Bivard, A, Kleinig, T, Miteff, F, Butcher, K, Lin, L, Levi, C, et al. Ischemic core thresholds change with time to reperfusion: a case control study. Ann Neurol. (2017) 82:995–1003. doi: 10.1002/ana.25109
19. Bivard, A, Levi, C, Krishnamurthy, V, Hislop-Jambrich, J, Salazar, P, Jackson, B, et al. Defining acute ischemic stroke tissue pathophysiology with whole brain ct perfusion. J Neuroradiol. (2014) 41:307–15. doi: 10.1016/j.neurad.2013.11.006
20. Bivard, A, Levi, C, Spratt, N, and Parsons, M. Perfusion ct in acute stroke: a comprehensive analysis of infarct and penumbra. Radiology. (2013) 267:543–50. doi: 10.1148/radiol.12120971
21. Bivard, A, McElduff, P, Spratt, N, Levi, C, and Parsons, M. Defining the extent of irreversible brain ischemia using perfusion computed tomography. Cerebrovasc Dis. (2011) 31:238–45. doi: 10.1159/000321897
22. Bivard, A, Spratt, N, Levi, C, and Parsons, M. Perfusion computer tomography: imaging and clinical validation in acute ischaemic stroke. Brain. (2011) 134:3408–16. doi: 10.1093/brain/awr257
23. Lin, L, Bivard, A, Krishnamurthy, V, Levi, CR, and Parsons, MW. Whole-brain ct perfusion to quantify acute ischemic penumbra and core. Radiology. (2016) 279:876–87. doi: 10.1148/radiol.2015150319
24. Murphy, BD, Fox, AJ, Lee, DH, Sahlas, DJ, Black, SE, Hogan, MJ, et al. Identification of penumbra and infarct in acute ischemic stroke using computed tomography perfusion-derived blood flow and blood volume measurements. Stroke. (2006) 37:1771–7. doi: 10.1161/01.STR.0000227243.96808.53
25. Murphy, BD, Fox, AJ, Lee, DH, Sahlas, DJ, Black, SE, Hogan, MJ, et al. White matter thresholds for ischemic penumbra and infarct core in patients with acute stroke: Ct perfusion study. Radiology. (2008) 247:818–25. doi: 10.1148/radiol.2473070551
26. Cereda, CW, Christensen, S, Campbell, BCV, Mishra, NK, Mlynash, M, Levi, C, et al. A benchmarking tool to evaluate computer tomography perfusion infarct core predictions against a dwi standard. J Cereb Blood Flow Metab. (2016) 36:1780–9. doi: 10.1177/0271678X15610586
27. Kamalian, S, Kamalian, S, Maas, MB, Goldmacher, GV, Payabvash, S, Akbar, A, et al. Ct cerebral blood flow maps optimally correlate with admission diffusion-weighted imaging in acute stroke but thresholds vary by postprocessing platform. Stroke. (2011) 42:1923–8. doi: 10.1161/STROKEAHA.110.610618
28. Kamalian, S, Kamalian, S, Konstas, AA, Maas, MB, Payabvash, S, Pomerantz, SR, et al. Ct perfusion mean transit time maps optimally distinguish benign oligemia from true "at-risk" ischemic penumbra, but thresholds vary by postprocessing technique. AJNR Am J Neuroradiol. (2012) 33:545–9. doi: 10.3174/ajnr.A2809
29. Mokin, M, Levy, EI, Saver, JL, Siddiqui, AH, Goyal, M, Bonafe, A, et al. Predictive value of rapid assessed perfusion thresholds on final infarct volume in swift prime (solitaire with the intention for thrombectomy as primary endovascular treatment). Stroke. (2017) 48:932–8. doi: 10.1161/STROKEAHA.116.015472
30. Muehlen, I, Sprugel, M, Hoelter, P, Hock, S, Knott, M, Huttner, HB, et al. Comparison of two automated computed tomography perfusion applications to predict the final infarct volume after thrombolysis in cerebral infarction 3 recanalization. Stroke. (2022) 53:1657–64. doi: 10.1161/STROKEAHA.121.035626
31. Koopman, MS, Berkhemer, OA, Geuskens, R, Emmer, BJ, van Walderveen, MAA, Jenniskens, SFM, et al. Comparison of three commonly used ct perfusion software packages in patients with acute ischemic stroke. J Neurointerv Surg. (2019) 11:1249–56. doi: 10.1136/neurintsurg-2019-014822
32. Copen, WA, Morais, LT, Wu, O, Schwamm, LH, Schaefer, PW, González, RG, et al. In acute stroke, can ct perfusion-derived cerebral blood volume maps substitute for diffusion-weighted imaging in identifying the ischemic core? PLoS One. (2015) 10:e0133566. doi: 10.1371/journal.pone.0133566
33. Koenig, M, Kraus, M, Theek, C, Klotz, E, Gehlen, W, and Heuser, L. Quantitative assessment of the ischemic brain by means of perfusion-related parameters derived from perfusion ct. Stroke. (2001) 32:431–7. doi: 10.1161/01.STR.32.2.431
34. Klotz, E, and König, M. Perfusion measurements of the brain: using dynamic ct for the quantitative assessment of cerebral ischemia in acute stroke. Eur J Radiol. (1999) 30:170–84. doi: 10.1016/S0720-048X(99)00009-1
35. Kameda, K, Uno, J, Otsuji, R, Ren, N, Nagaoka, S, Maeda, K, et al. Optimal thresholds for ischemic penumbra predicted by computed tomography perfusion in patients with acute ischemic stroke treated with mechanical thrombectomy. J Neurointerv Surg. (2018) 10:279–84. doi: 10.1136/neurintsurg-2017-013083
36. Qiao, Y, Zhu, G, Patrie, J, Xin, W, Michel, P, Eskandari, A, et al. Optimal perfusion computed tomographic thresholds for ischemic core and penumbra are not time dependent in the clinically relevant time window. Stroke. (2014) 45:1355–62. doi: 10.1161/STROKEAHA.113.003362
37. Pan, J, Zhang, J, Huang, W, Cheng, X, Ling, Y, Dong, Q, et al. Value of perfusion computed tomography in acute ischemic stroke: diagnosis of infarct core and penumbra. J Comput Assist Tomogr. (2013) 37:645–9. doi: 10.1097/RCT.0b013e31829866fc
38. Fainardi, E, Busto, G, Rosi, A, Scola, E, Casetta, I, Bernardoni, A, et al. Tmax volumes predict final infarct size and functional outcome in ischemic stroke patients receiving endovascular treatment. Ann Neurol. (2022). doi: 10.1002/ana.26354
39. Rao, V, Christensen, S, Yennu, A, Mlynash, M, Zaharchuk, G, Heit, J, et al. Ischemic core and hypoperfusion volumes correlate with infarct size 24 hours after randomization in defuse 3. Stroke. (2019) 50:626–31. doi: 10.1161/STROKEAHA.118.023177
40. Albers, GW, Goyal, M, Jahan, R, Bonafe, A, Diener, HC, Levy, EI, et al. Ischemic core and hypoperfusion volumes predict infarct size in swift prime. Ann Neurol. (2016) 79:76–89. doi: 10.1002/ana.24543
41. Wintermark, M, Flanders, AE, Velthuis, B, Meuli, R, van Leeuwen, M, Goldsher, D, et al. Perfusion-ct assessment of infarct core and penumbra: receiver operating characteristic curve analysis in 130 patients suspected of acute hemispheric stroke. Stroke. (2006) 37:979–85. doi: 10.1161/01.STR.0000209238.61459.39
42. d'Esterre, CD, Boesen, ME, Ahn, SH, Pordeli, P, Najm, M, Minhas, P, et al. Time-dependent computed tomographic perfusion thresholds for patients with acute ischemic stroke. Stroke. (2015) 46:3390–7. doi: 10.1161/STROKEAHA.115.009250
43. Campbell, BC, Christensen, S, Levi, CR, Desmond, PM, Donnan, GA, Davis, SM, et al. Comparison of computed tomography perfusion and magnetic resonance imaging perfusion-diffusion mismatch in ischemic stroke. Stroke. (2012) 43:2648–53. doi: 10.1161/STROKEAHA.112.660548
44. Campbell, BC, Christensen, S, Levi, CR, Desmond, PM, Donnan, GA, Davis, SM, et al. Cerebral blood flow is the optimal ct perfusion parameter for assessing infarct core. Stroke. (2011) 42:3435–40. doi: 10.1161/STROKEAHA.111.618355
45. Eilaghi, A, d'Esterre, CD, Lee, TY, Jakubovic, R, Brooks, J, Liu, RT, et al. Toward patient-tailored perfusion thresholds for prediction of stroke outcome. AJNR Am J Neuroradiol. (2014) 35:472–7. doi: 10.3174/ajnr.A3740
46. Copen, WA, Yoo, AJ, Rost, NS, Morais, LT, Schaefer, PW, González, RG, et al. In patients with suspected acute stroke, ct perfusion-based cerebral blood flow maps cannot substitute for dwi in measuring the ischemic core. PLoS One. (2017) 12:e0188891. doi: 10.1371/journal.pone.0188891
47. Qiu, W, Kuang, H, Lee, TY, Boers, AM, Brown, S, Muir, K, et al. Confirmatory study of time-dependent computed tomographic perfusion thresholds for use in acute ischemic stroke. Stroke. (2019) 50:3269–73. doi: 10.1161/STROKEAHA.119.026281
48. Laredo, C, Renú, A, Tudela, R, Lopez-Rueda, A, Urra, X, Llull, L, et al. The accuracy of ischemic core perfusion thresholds varies according to time to recanalization in stroke patients treated with mechanical thrombectomy: a comprehensive whole-brain computed tomography perfusion study. J Cereb Blood Flow Metab. (2020) 40:966–77. doi: 10.1177/0271678X19855885
49. McVerry, F, Dani, KA, MaCdougall, NJJ, MaCleod, MJ, Wardlaw, J, and Muir, KW. Derivation and evaluation of thresholds for core and tissue at risk of infarction using ct perfusion. J Neuroimaging. (2014) 24:562–8. doi: 10.1111/jon.12134
50. Schaefer, PW, Roccatagliata, L, Ledezma, C, Hoh, B, Schwamm, LH, Koroshetz, W, et al. First-pass quantitative ct perfusion identifies thresholds for salvageable penumbra in acute stroke patients treated with intra-arterial therapy. AJNR Am J Neuroradiol. (2006) 27:20–5.
51. Payabvash, S, Souza, LC, Wang, Y, Schaefer, PW, Furie, KL, Halpern, EF, et al. Regional ischemic vulnerability of the brain to hypoperfusion: the need for location specific computed tomography perfusion thresholds in acute stroke patients. Stroke. (2011) 42:1255–60. doi: 10.1161/STROKEAHA.110.600940
52. Cohen, JF, Korevaar, DA, Altman, DG, Bruns, DE, Gatsonis, CA, Hooft, L, et al. Stard 2015 guidelines for reporting diagnostic accuracy studies: explanation and elaboration. BMJ Open. (2016) 6:e012799. doi: 10.1136/bmjopen-2016-012799
53. Man, F, Patrie, JT, Xin, W, Zhu, G, Hou, Q, Michel, P, et al. Delay-sensitive and delay-insensitive deconvolution perfusion-ct: similar ischemic core and penumbra volumes if appropriate threshold selected for each. Neuroradiology. (2015) 57:573–81. doi: 10.1007/s00234-015-1507-7
54. Christensen, S, Mouridsen, K, Wu, O, Hjort, N, Karstoft, H, Thomalla, G, et al. Comparison of 10 perfusion mri parameters in 97 sub-6-hour stroke patients using voxel-based receiver operating characteristics analysis. Stroke. (2009) 40:2055–61. doi: 10.1161/STROKEAHA.108.546069
55. Rava, RA, Snyder, KV, Mokin, M, Waqas, M, Allman, AB, Senko, JL, et al. Effect of computed tomography perfusion post-processing algorithms on optimal threshold selection for final infarct volume prediction. Neuroradiol J. (2020) 33:273–85. doi: 10.1177/1971400920934122
56. Rava, RA, Snyder, KV, Mokin, M, Waqas, M, Zhang, X, Podgorsak, AR, et al. Assessment of computed tomography perfusion software in predicting spatial location and volume of infarct in acute ischemic stroke patients: a comparison of sphere, vitrea, and rapid. J Neurointerv Surg. (2021) 13:130–5. doi: 10.1136/neurintsurg-2020-015966
Keywords: computed tomography perfusion, penumbra, infarct, thresholds, parameters
Citation: Thirugnanachandran T, Aitchison SG, Lim A, Ding C, Ma H and Phan T (2023) Assessing the diagnostic accuracy of CT perfusion: a systematic review. Front. Neurol. 14:1255526. doi: 10.3389/fneur.2023.1255526
Edited by:
Hugo De Jong, University Medical Center Utrecht, NetherlandsReviewed by:
Thomas Lindner, University of Hamburg, GermanyFreda Werdiger, The University of Melbourne, Australia
Copyright © 2023 Thirugnanachandran, Aitchison, Lim, Ding, Ma and Phan. This is an open-access article distributed under the terms of the Creative Commons Attribution License (CC BY). The use, distribution or reproduction in other forums is permitted, provided the original author(s) and the copyright owner(s) are credited and that the original publication in this journal is cited, in accordance with accepted academic practice. No use, distribution or reproduction is permitted which does not comply with these terms.
*Correspondence: Thanh Phan, thanh.phan@monash.edu