- Department of Radiology, The Second Affiliated Hospital of Chongqing Medical University, Chongqing, China
Background: Impulse control disorders (ICDs) are common neuropsychiatric symptoms (NPS), which are prevalent among patients with Parkinson’s disease (PD). Current research has not clarified the impact of ICDs on cognitive function nor provided sufficient objective evidence. This study aims to explore the effects of ICDs on cognitive functions in PD patients, and further investigate associated cerebral structural changes.
Methods: Two hundred PD patients with normal cognition (PDNC) and 69 healthy controls were included from the Parkinson’s Progression Markers Initiative (PPMI), among these PDNC, 81 patients with “pure” ICDs (p-ICDs), 69 ICDs combined with other NPS (c-ICDs), and 50 patients without NPS. The cognitive status of each PD patient was obtained every year in four-year follow-up. The difference in conversion rates was obtained by chi-square test. Survival analysis was used to explore the conversion time difference among these groups. Further analysis was conducted on the potential structural difference. Finally, the correlation between significant brain structural changes and neuropsychological assessments were evaluated.
Results: The survival analysis suggested that the conversion time of p-ICDs from normal cognition to MCI was significantly delayed compared to NPS-negative, with no significant difference relative to the c-ICDs. There is no significant difference in conversion rates among them. Morphological analysis revealed that compared to the NPS-negative group, the p-ICDs and c-ICDs groups exhibited thickness changes in certain regions (Bonferroni-corrected, p < 0.05).
Conclusion: Our findings suggest that ICDs might exert a protective effect against cognitive decline, potentially delay the occurrence of MCI in PDNC, which could be associated with alterations in cortical thickness.
1 Introduction
Cognitive deficits are commonly observed in Parkinson’s disease (PD) (1). Approximately 30–40% of individuals may exhibit mild cognitive impairment (MCI) early in the disease course (2, 3), which might emerge before or at the time of PD diagnosed. Previous studies have shown that MCI is a risk factor for Parkinson’s disease dementia (PDD) and an established stage of the condition (4, 5). Compared to PD patients with normal cognition (PDNC), PDMCI have a higher risk of developing to PDD (6, 7). Almost 60–80% of PDMCI eventually develop to PDD (8), which would significantly impact their quality of life and tend to deteriorate as the disease progress (9).
Besides cognitive impairment, the neuropsychiatric symptoms (NPS), such as depression, anxiety, apathy, psychosis and impulse control disorders (ICDs), are also common non-motor symptoms in PD patients (10). These clinical signs and symptoms may present either independently or simultaneously (11). Among these NPS, ICDs, which include compulsive, repetitive, and excessive behaviors such as gambling, sexual activity, shopping, and eating (12), have a relatively high incidence and are often accompanied by other NPS (13–15). For decades, the relationship between NPS and cognitive impairment in Parkinson’s disease has sparked considerable interest among researchers. Some studies found that NPS, such as depression and anxiety, apathy, are associated with cognitive impairment in Parkinson’s disease and serve as predictors for cognitive decline (16–20). While, other researchers indicated that PD with the single and coexistence of NPS exhibit relatively lower cognitive decline within a certain period (21–23). Due to the inconsistencies of these findings and the inherent limitations of neuropsychological assessments, this issue still remains controversial.
Structural magnetic resonance imaging provides an objective and non-invasive method to accurately measure changes in brain structure, providing biomarkers for monitoring cognitive decline, an advantage over conventional clinical assessment (24). To date, there have been few morphometric analyses on the impact of ICDs on cognitive function in PD. Biundo et al. found that cognitive function was relatively preserved in PD with ICD, but voxel-based morphometry analysis revealed no significant differences between PD with and without ICDs (25). Therefore, the impact of ICDs combined with other NPS on cognitive changes in PD and the relevant imaging evidence needs to be further explored.
In this study, we aim to investigate that (1) whether single ICDs and ICDs combined with other NPS have an impact on the progression of cognitive impairment; (2) The structural differences between single ICDs and ICDs combined with other NPS; (3) the correlation between cortical changes and cognitive assessments.
2 Materials and methods
2.1 Participants
The data were acquired from PPMI1 (26). The PPMI study protocols were approved by local ethics committees, which involved all 33 clinical research institutions. Written informed consent was obtained prior to their inclusion in the study. Methods in this study were in accordance with relevant guidelines and regulations. The inclusion criteria of PD patients were as follows: (I) the patients must have at least 2 of the following: resting tremor, bradykinesia, rigidity, and either asymmetric resting tremor or asymmetric bradykinesia; (II) At baseline, the patients remained untreated; during the follow-up period, they were required to strictly adhere to the prescribed dosage and medication protocols; (III) the patients have T1-weighted images without distortion or head motion artifacts. The exclusion criteria were as follows: (I) Cognitive decline caused by other diseases (such as brain tumors, cerebral hemorrhages, etc.); (II) the patient has taken PD medication recently; (III) MRI images are missing or of poor quality; (IV) The subjects were diagnosed with PDD.
The cognitive assessments were evaluated at baseline and with 12 months interval in four-year follow-up. Based on the cognitive assessments, the cognitive status of all participants were obtained in four-year follow-up. Patients were classified as PDMCI if they met one of the following criteria: (I) cognitive impairment evidenced by the Montreal Cognitive Assessment (MoCA) (27, 28), MOCA<26 as a cut-off, or (II) scores below 1.5 standard deviations from the age/education normative mean on at least two neuropsychological tests, including the Hopkins Verbal Learning Test (HVLT), Judgment of Line Orientation (JLO), Letter Number Sequencing (LNS), Semantic Fluency Test (SFT), and Symbol Digit Modality Test (SDMT) (29).
Neuropsychiatric symptoms were assessed at baseline, Depression was evaluated with the 15-item Geriatric Depression Scale (GDS-15), and a scores ≥5 indicated clinically significant depression (30). Anxiety was evaluated with the State–Trait Anxiety Inventory (STAI), and a score ≥40 on any subscale was considered as clinically significant anxiety (31). ICDs and related behaviors were assessed with the short form of the Questionnaire for ICDs in PD (32). Psychosis and apathy were separately assessed through the MDS-Psychiatric Rating Scale 17, part I, focusing on the hallucination/psychosis and apathy items (33). The presence of any level 1 or higher symptoms of psychosis or apathy was considered indicative of these conditions.
Following a series of screenings, 200 PD patients with normal cognition (PDNC) were diagnosed with ICDs. Taking into account the mediating effects of other neuropsychiatric symptoms, among these PDNC, 81 participants which had ICDs only were diagnosed as “pure” ICDs (p-ICDs), while 69 PD-ICDs with other NPS such as depression, anxiety, psychosis, and apathy were diagnosed as PD-ICDs combined other NPS (c-ICDs). Fifty PD patients without NPS were classified as NPS-negative. The study also included 69 healthy controls (HC).
2.2 Image acquisition
The magnetic resonance imaging parameters were standardized across all scanners with a slice thickness of 1 mm and a matrix size of 256 × 256. For GE MEDICAL SYSTEMS scanners, the Signa HDxt model used a field of view (FOV) of 250 mm, echo time (TE) of 3.6 ms, inversion time (TI) of 450.0 ms, repetition time (TR) of 9.1 ms, and a flip angle of 13.0°; the SIGNA EXCITE model featured a FOV of 230 mm, TE of 4.0 ms, TI of 0.0 ms (no inversion recovery), TR of 8.3 ms, and a flip angle of 15.0°; while the DISCOVERY MR750 employed a FOV of 260 mm, TE of 4.2 ms, TI of 450.0 ms, TR of 8.2 ms, and a flip angle of 13.0°. Siemens scanners included the Espree (FOV = 250 mm, TE = 3.2 ms, TI = 1,100.0 ms, TR = 1,970.0 ms, flip angle = 15.0°), Verio (FOV = 240 mm, TE = 3.0 ms, TI = 900.0 ms, TR = 2,300.0 ms, flip angle = 9.0°), and TrioTim (FOV = 250 mm, TE = 3.0 ms, TI = 900.0 ms, TR = 2,300.0 ms, flip angle = 9.0°). Philips Medical Systems scanners comprised the Achieva (FOV = 220 mm, TE = 3.2 ms, TI = 0.0 ms, TR = 7.0 ms, flip angle = 8.0°) and Intera (FOV = 250 mm, TE = 4.0 ms, TI = 0.0 ms, TR = 8.5 ms, flip angle = 8.0°), with TI = 0.0 ms indicating no inversion recovery (Table 1). And the number of participants scanned across eight different manufacturers are provided as follows (Table 2).
2.3 Image processing
Cortical thickness was assessed by FreeSurfer,2 which computed the average distance between the gray/white matter boundary and the pial surface at each vertex on the cortical surface. Employing well-established FreeSurfer processing pipeline (34), a robust, unbiased subject-specific template was generated through inverse consistent registration across all available MRI scans for each participant (35, 36). Several processing steps, such as skull stripping, Talairach transformation, atlas registration, and the creation of spherical surface maps and parcellations, were executed based on the subject-specific templates. The cerebral cortex was parcellated into 68 distinct anatomical regions, within which the average thickness was determined. Prior to further analysis, each individual brain map underwent visual inspection to ensure proper registration.
2.4 Statistical analysis
Statistical analysis was performed using SPSS software 27.0. For quantitative data that followed a normal distribution, inter-group comparisons were conducted using one-way ANOVA. Non-parametric tests were conducted using the Kruskal-Wallis test. For multiple comparisons, the Bonferroni correction was applied to adjust the probability of Type I errors. Categorical data were analyzed using the Chi-square test, with a significance level of α = 0.05. Subsequently, with progression to MCI as the status variable and follow-up time as the time variable, differences in conversion rates among the p-ICDs, c-ICDs, NPS-negative and HC were investigated using the Kaplan–Meier survival analysis. The power analysis was performed through univariate ANOVA methodology to systematically validate the reliability of cognitive function assessments. Differences in cortical thickness among groups were further compared using one-way ANOVA then. Based on this, Pearson analysis was used to explore the correlation between cortical thickness and neuropsychological tests, with a significance level of α = 0.05.
3 Results
3.1 Demographics and cognitive analysis
At baseline, no significant differences were observed in age, sex, years of education, and H&Y stages among the p-ICDs group, c-ICDs group, NPS-negative group, and the control group (p > 0.05).
The HVLT, JLO, LNS, SFT and SDMT showed no significant differences at baseline across the p-ICDs group, c-ICDs group, NPS-negative group, and the control group (p > 0.05) (Table 3).
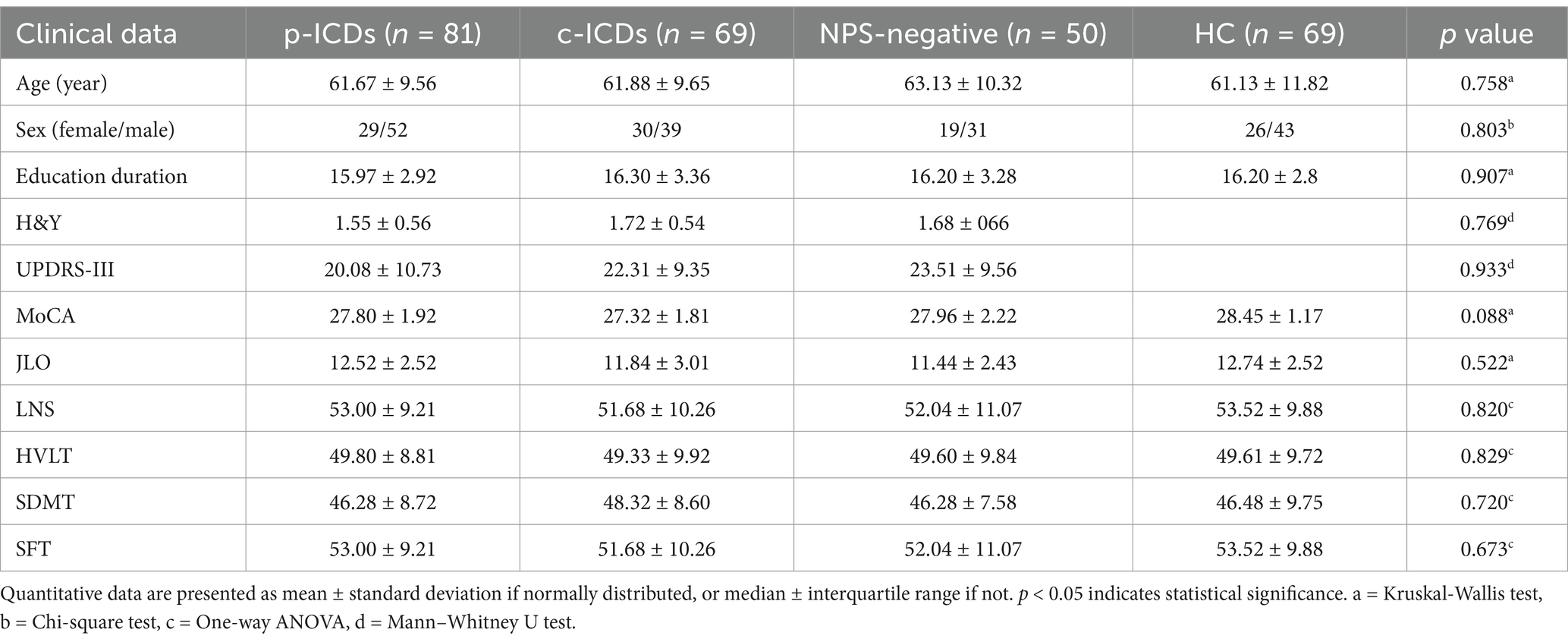
Table 3. Cognitive data and basic characteristics of Parkinson’s disease patients with p-ICDs, c-ICDs, NPS-negative and healthy control participants.
3.2 Survival analysis and conversion rate analysis
Among 81 p-ICDs, 69 c-ICDs, and 50 NPS-negative, the conversion rates to MCI were 20, 28, and 38%, respectively. And there was no significant difference in the conversion rates among these groups (p = 0.073) (Supplementary Table S1).
The Kaplan–Meier analysis revealed that the time in the p-ICDs group to progress from normal cognition to MCI was significantly longer than that of the NPS-negative group. (p = 0.011) (Figure 1A). There were no significant difference in conversion rates between the c-ICDs group and NPS-negative group (p = 0.152) (Figure 1B), p-ICDs group and c-ICDs group (p = 0.250) (Figure 1C).
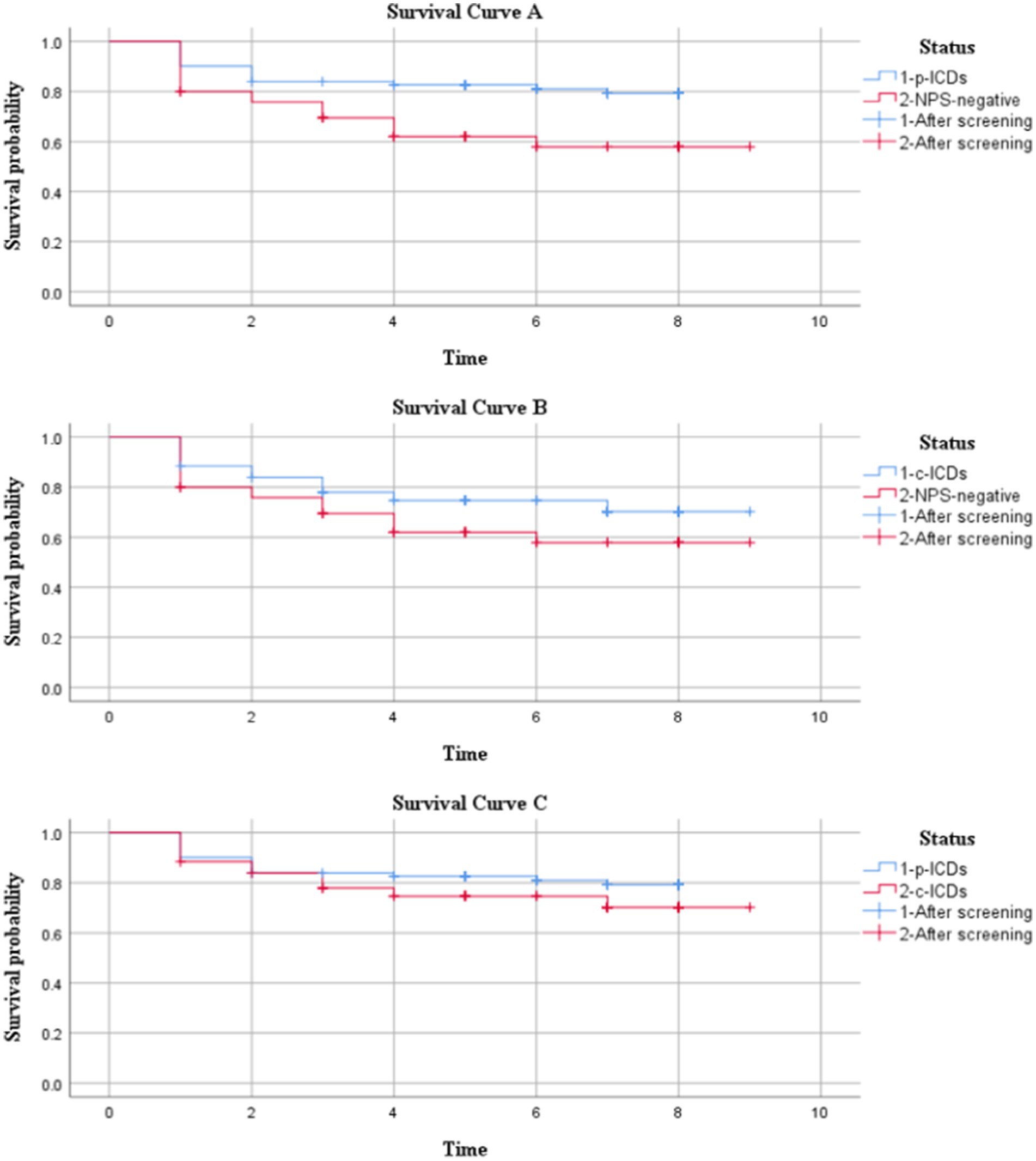
Figure 1. Survival analysis of Parkinson’s disease patients with p-ICDs and NPS-negative, c-ICDs and NPS-negative, p-ICDs and c-ICDs. (A) p-ICDs VS NPS-negative, (B) c-ICDs VS NPS-negative, (C) p-ICDs VS c-ICDs.
3.3 Analysis of differential brain regions
Compared to the HC, both p-ICDs group and c-ICDs group exhibited thinning in the bilateral caudal cingulate cortex (cACC), bilateral frontal pole and bilateral isthmus cingulate cortex (ICC). Similarly, both c-ICDs group and NPS-negative group exhibited thinning in the right rostral middle frontal gyrus (rMFG) in contrast to the HC. Additionally, the p-ICDs group showed thinning in the right parahippocampal gyrus (PHG) and right rostral anterior cingulate cortex (rACC) in comparison to HC (Bonferroni-corrected, p < 0.05, Table 4).
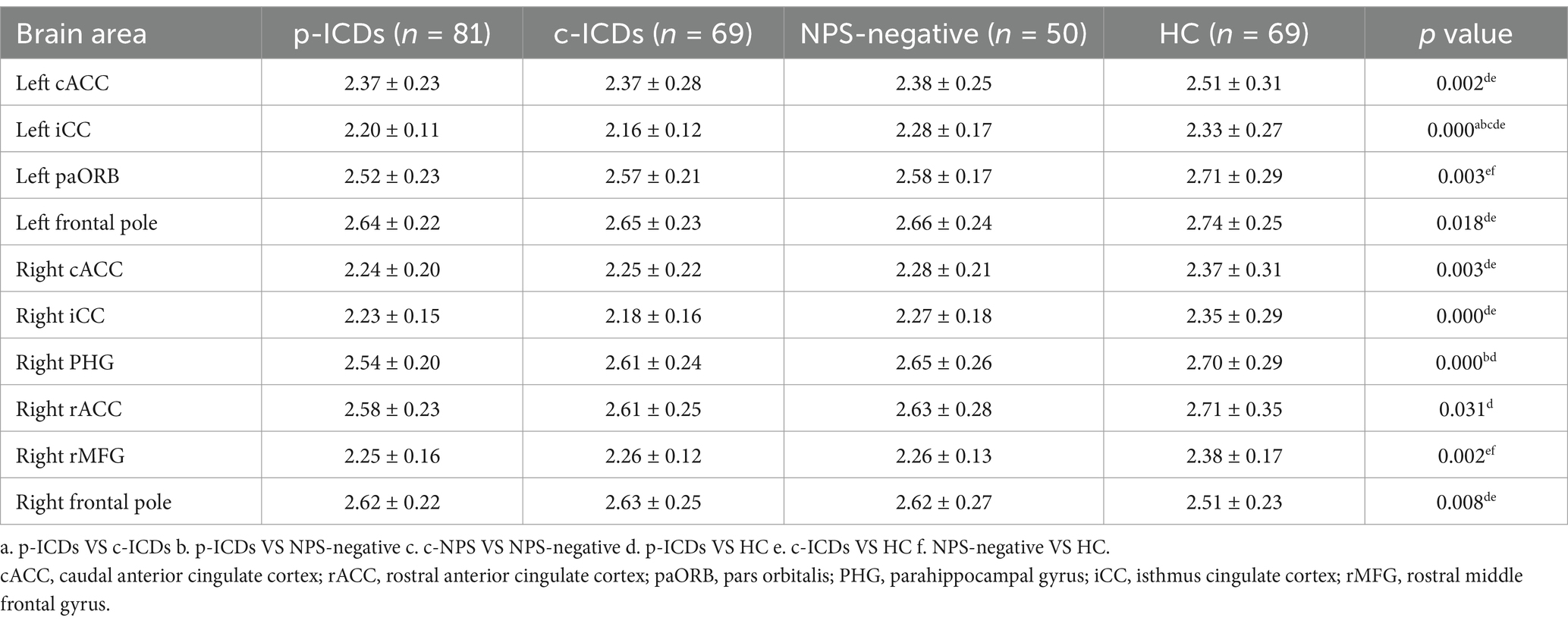
Table 4. Differences in cortical thickness among patients with p-ICDs, c-ICDs, NPS-negative and healthy control participants.
Compared to the NPS-negative group, both the p-ICDs group and the c-ICDs group showed significant thinning in the left ICC, and the p-ICDs group exhibited thinning in the right PHG (Bonferroni-corrected, p < 0.05, Figure 2). The p-ICDs group exhibited thickening in the left ICC compared to the c-ICDs group (Bonferroni-corrected, p < 0.05, Table 4; Figure 2).
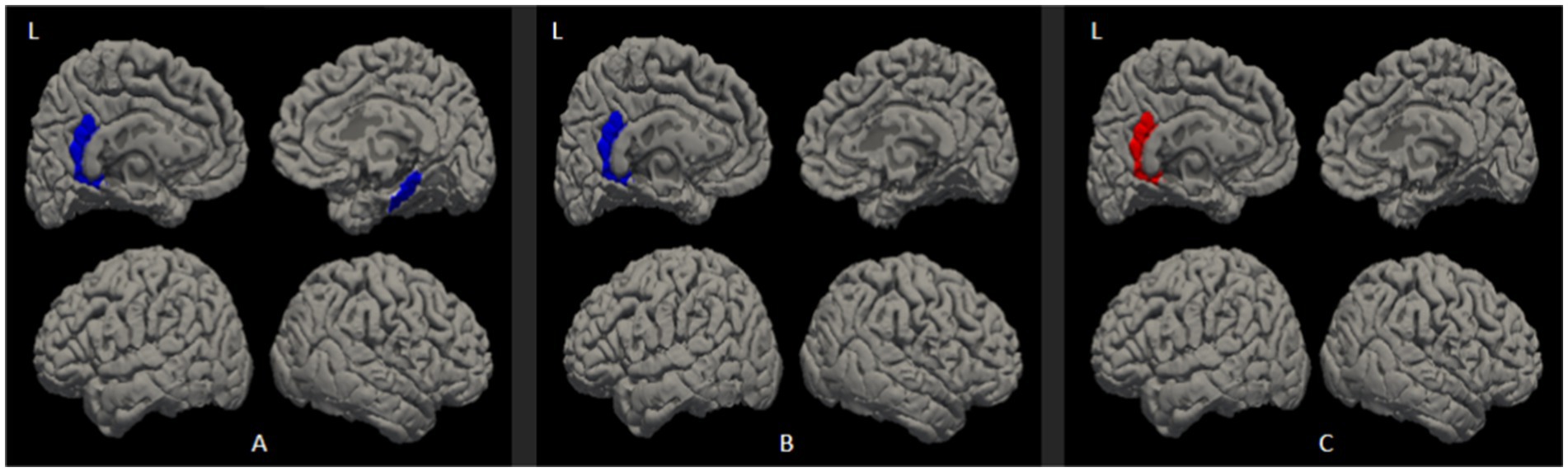
Figure 2. Differential cortical thickness map between group comparison. (A) p-ICDs VS NPS-negative, (B) c-ICDs VS NPS-negative, (C) p-ICDs VS c-ICDs. Blue represents areas of cortical thinning, while red represents areas of cortical thickening.
The post hoc power analysis showed that the Cohen d (Eta squared) between the three groups (p-ICDs, c-ICDs, NPS negative) was 0.72, reaching a moderate effect (Supplementary Figure S2).
3.4 Correlation analysis
The correlation analysis was conducted between the significantly altered cortical regions and clinical neuropsychiatric tests (Supplementary Figure S1). The left cACC was negatively correlated with the Judgment of Line Orientation in the p-ICDs group (r = −0.220, p = 0.049). The right PHG showed a positive correlation with the Hopkins Verbal Learning Test in p-ICDs group (r = 0.376, p = 0.001) and HC group (r = 0.239, p = 0.048), but showed a negative correlation with the Hopkins Verbal Learning Test in NPS-negative group (r = −0.304, p = 0.034). The right PHG in HC group (r = 0.250, p = 0.038), and the left ICC (r = 0.262, p = 0.030), the left paORB (r = 0.398, p = 0.001) and left frontal pole (r = 0.291, p = 0.015) in c-ICDs group showed a positive correlation with Letter Number Sequencing. The right frontal pole was negatively correlated with the MOCA (r = −0.250, p = 0.039) and Semantic Fluency Test (r = −0.283, p = 0.019) in c-ICDs group. There was no significant correlation between other brain regions and neuropsychological assessments (p > 0.05). There was no significant correlation between other brain regions and neuropsychological assessments (p > 0.05).
4 Discussion
In this study, we investigate the impact of ICDs on PD patients from normal cognition to MCI. Notably, in the four-year follow up, we observed that patients with PD who exhibited ICDs had notably longer survival times compared to those who were NPS-negative, while the conversion rates not significantly differed. And the survival time and conversion rates were also not significantly differed between the p-ICDs group and c-ICDs group. In the brain structural analysis, the p-ICDs group showed increased cortical thickness in the left ICC relative to the c-ICDs group, and both the p-ICDs group, and the c-ICDs group showed decreased cortical thickness of left ICC than NPS-negative group. And the p-ICDs group exhibited thinning in the right PHG than the NPS-negative group. The correlation analysis revealed that the right PHG showed a positive correlation with the Hopkins Verbal Learning Test in p-ICDs and HC group, but showed a negative correlation with the Hopkins Verbal Learning Test in NPS-negative group.
The longer survival time of PD with ICDs from MCI was observed, which indicating a potential protection effect of ICDs. Similar phenomenon were reported previously, Thomas et al. found that ICDs were associated with an increased psychiatric burden at baseline, but they were also linked to a better cognitive prognosis, it is believed that PD patients with ICDs at baseline, the intake of a certain dose of dopamine agonists (DA) leads to drug-induced overstimulation, impairing the top-down inhibitory control of ICDs, which somewhat delays the decline in cognitive function (37). Similarly, Chiara et al. found that PD patients with ICDs exhibited relatively lower cognitive decline over time (22, 38), which was then attributed to the drug-induced overstimulation exerted relatively preservation on prefrontal cognitive functions. Such possibility should also take in to consideration currently. In this work, patients were only receiving therapy of dopamine to control PD symptom. Our findings might result from the different reaction of the brain of PD patients with ICDs on the dopamine therapy, and further investigation were warranted. And with the not significantly differed conversion rates between patients with or without ICDs in 4 years, we speculate that the incidence of MCI in PD patient was certainly fixed, and dopamine induced effects in PD patients with ICDs might delayed the occurrence of MCI.
In our study, patients with ICDs were divided into p-ICDs group, PD with only ICDs, and c-ICDs group, PD with ICDs and other NPS. Relative to healthy controls, all three groups (p-ICDs, c-ICDs, and NPS) exhibited shared cortical alterations in the cingulate cortex, parahippocampal gyrus (PHG), and frontal regions, which correlate with deficits in emotional regulation, cognitive control, memory, and executive function (39–41). These structural changes are consistent with previous reports on PD (42, 43). Besides changes associated with PD, cortical thinning at left ICC was observed in p-ICDs group and c-ICDs group in relation to NPS negative group. The ICC is closely related to emotional process and regulation, and important in cognitive control and executive function (41, 44). The cortical atrophy of ICC was also reported in neurodegenerative diseases and psychiatric disorders, such as PD, AD and schizophrenia (45–48). Prior investigations have demonstrated that PD patients with ICDs exhibit greater cortical thickness in the left ICC following pharmacological induction compared to non-ICD counterparts, which was then assumed to be associated with drug effects and reward mechanisms (49). In our study, PD subjects did not receive dopamine treatment at baseline, and the drug effects and reward mechanisms maybe not activate. Notably, in the decreased cortical thickness of ICC in relation to NPS-negative group, the c-ICDs group showed even thinner ICC than p-ICD group. Given on our analysis was conducted at the baseline, and the dopamine therapy after image acquisition. Such phenomenon might result from two possibilities, the confounding effects of other NPS and/or the differed reaction to dopamine therapy, and further research is needed to validate these hypotheses. Additionally, PD participants from the PPMI cohort received standardized drug therapy during the follow-up period. Previous study reported the magnitude of polytherapy and anticholinergic drugs burden does not appear to modulate PD-MCI risk (50). in other words, the cognitive impact of impulse control disorders (ICDs) in Parkinson’s disease appears independent of medication classes or dosage levels.
Besides, the p-ICDs group exhibited thinning in the right PHG at baseline. Although previous study have shown that the PHG of PD patients with ICDs is slightly thicker than that of patients without ICDs after dopamine treatment, no significant statistical difference was observed, They believe that the structural changes are secondary to neuroplastic adaptations related to non-physiological dopaminergic stimulation (49). Our subjects had not received dopamine therapy at baseline, the thinning of the PHG at baseline may be due to the dopamine-induced reward mechanism not being activated. In addition, we found that the PHG in the c-ICDs group was slightly thinner than in PD patients without NPS, although this difference was not statistically significant, possibly due to the confounding effects of other NPS.
Additionally, we observed a positive correlation between the cortical thickness of right PHG and HVLT in p-ICDs and HC group, suggesting a potential link between ICDs and memory function in PD patients. Recent study also reported that PD with ICDs and normal elderly exhibited poor performance in language learning and memory functions (51, 52), notably, we found that the PHG thinning is accompanied by memory improvement in NPS-negative group, which might be that the brain maintains certain cognitive functions through adaptation and compensation mechanisms (53).
This study has several limitations. First, the distribution of other neuropsychiatric symptoms in this study is uneven, for example, the number of NPS-negative group is too small, although our results are interpretable, a larger and more evenly distributed sample is needed to further investigate the mediating effects of each psychiatric symptom on cognitive function. Second, our imaging structural study is limited to the baseline, and the imaging modalities are relatively single. Further longitudinal design and multimodal imaging analysis are needed to confirm our results.
In conclusion, by survival analysis and cortical structural analysis, this study provides valuable imaging markers and supplementary information on the impact of ICDs on cognitive decline in PD patients. It suggests that ICDs may play a protective role in cognitive ability, potentially delaying the onset of MCI in PDNC patients and being accompanied by corresponding changes in cortical thickness.
Data availability statement
The datasets presented in this study can be found in online repositories. The names of the repository/repositories and accession number(s) can be found at: https://www.ppmi-info.org/.
Ethics statement
The studies involving humans were approved by the Parkinson’s Progression Markers Initiative (PPMI) database was used in this study. For up-to-date information on the study, visit [PPMI website] (https://www.ppmi-info.org/). The study protocol and informed consent forms are available upon request from the PPMI website. The studies were conducted in accordance with the local legislation and institutional requirements. All participants provided written informed consent in accordance with the ethical standards of the institutional and/or national research committee and with the 1964 Helsinki declaration and its later amendments or comparable ethical standards.
Author contributions
RX: Writing – original draft, Writing – review & editing. BY: Formal analysis, Funding acquisition, Visualization, Writing – review & editing. JL: Validation, Writing – original draft. XW: Software, Writing – review & editing. LZ: Conceptualization, Investigation, Writing – review & editing. JT: Data curation, Writing – original draft. WZ: Project administration, Supervision, Writing – original draft, Writing – review & editing.
Funding
The author(s) declare that financial support was received for the research and/or publication of this article. This research work was supported by Chongqing medical scientific research project (joint project of Chongqing Health Commission and Science and Technology Bureau) (Grant number 2025MSXM175).
Conflict of interest
The authors declare that the research was conducted in the absence of any commercial or financial relationships that could be construed as a potential conflict of interest.
Generative AI statement
The authors declare that no Gen AI was used in the creation of this manuscript.
Publisher’s note
All claims expressed in this article are solely those of the authors and do not necessarily represent those of their affiliated organizations, or those of the publisher, the editors and the reviewers. Any product that may be evaluated in this article, or claim that may be made by its manufacturer, is not guaranteed or endorsed by the publisher.
Supplementary material
The Supplementary material for this article can be found online at: https://www.frontiersin.org/articles/10.3389/fneur.2025.1565046/full#supplementary-material
Footnotes
2. ^V6.0.0, http://surfer.nmr.mgh.harvard.edu/.
References
2. Fengler, S, Liepelt-Scarfone, I, Brockmann, K, Schäffer, E, Berg, D, and Kalbe, E. Cognitive changes in prodromal Parkinson's disease: a review. Mov Disord. (2017) 32:1655–66. doi: 10.1002/mds.27135
3. Weil, RS, Costantini, AA, and Schrag, AE. Mild cognitive impairment in Parkinson's disease-what is it? Curr Neurol Neurosci Rep. (2018) 18:17. doi: 10.1007/s11910-018-0823-9
4. Wallace, ER, Segerstrom, SC, van Horne, CG, Schmitt, FA, and Koehl, LM. Meta-analysis of cognition in Parkinson's disease mild cognitive impairment and dementia progression. Neuropsychol Rev. (2022) 32:149–60. doi: 10.1007/s11065-021-09502-7
5. Yu, RL, and Wu, RM. Mild cognitive impairment in patients with Parkinson's disease: an updated mini-review and future outlook. Front Aging Neurosci. (2022) 14:943438. doi: 10.3389/fnagi.2022.943438
6. Janvin, CC, Larsen, JP, Aarsland, D, and Hugdahl, K. Subtypes of mild cognitive impairment in Parkinson's disease: progression to dementia. Mov Disord. (2006) 21:1343–9. doi: 10.1002/mds.20974
7. Pedersen, KF, Larsen, JP, Tysnes, OB, and Alves, G. Natural course of mild cognitive impairment in Parkinson disease: a 5-year population-based study. Neurology. (2017) 88:767–74. doi: 10.1212/WNL.0000000000003634
8. Delgado-Alvarado, M, Gago, B, Navalpotro-Gomez, I, Jiménez-Urbieta, H, and Rodriguez-Oroz, MC. Biomarkers for dementia and mild cognitive impairment in Parkinson's disease. Mov Disord. (2016) 31:861–81. doi: 10.1002/mds.26662
9. Aarsland, D, Batzu, L, Halliday, GM, Geurtsen, GJ, Ballard, C, Ray Chaudhuri, K, et al. Parkinson disease-associated cognitive impairment. Nat Rev Dis Primers. (2021) 7:47. doi: 10.1038/s41572-021-00280-3
10. Meng, D, Jin, Z, Wang, Y, and Fang, B. Longitudinal cognitive changes in patients with early Parkinson's disease and neuropsychiatric symptoms. CNS Neurosci Ther. (2023) 29:2259–66. doi: 10.1111/cns.14173
11. Hu, M, Shu, X, Wu, X, Chen, F, Hu, H, Zhang, J, et al. Neuropsychiatric symptoms as prognostic makers for the elderly with mild cognitive impairment: a meta-analysis. J Affect Disord. (2020) 271:185–92. doi: 10.1016/j.jad.2020.03.061
12. Gatto, EM, and Aldinio, V. Impulse control disorders in Parkinson's disease. A brief and comprehensive review. Front Neurol. (2019) 10:351. doi: 10.3389/fneur.2019.00351
13. Marín-Lahoz, J, Sampedro, F, Martinez-Horta, S, Pagonabarraga, J, and Kulisevsky, J. Depression as a risk factor for impulse control disorders in Parkinson disease. Ann Neurol. (2019) 86:762–9. doi: 10.1002/ana.25581
14. Santos-García, D, de Deus Fonticoba, T, Cores Bartolomé, C, Suárez Castro, E, Jesús, S, Mir, P, et al. Depression is associated with impulse-compulsive behaviors in Parkinson's disease. J Affect Disord. (2021) 280:77–89. doi: 10.1016/j.jad.2020.11.075
15. Waskowiak, P, Koppelmans, V, and Ruitenberg, MFL. Trait anxiety as a risk factor for impulse control disorders in de novo Parkinson's disease. J Parkinsons Dis. (2022) 12:689–97. doi: 10.3233/JPD-212959
16. Ehgoetz Martens, KA, Silveira, CRA, Intzandt, BN, and Almeida, QJ. State anxiety predicts cognitive performance in patients with Parkinson's disease. Neuropsychology. (2018) 32:950–7. doi: 10.1037/neu0000478
17. Jones, JD, Kurniadi, NE, Kuhn, TP, Szymkowicz, SM, Bunch, J, and Rahmani, E. Depressive symptoms precede cognitive impairment in de novo Parkinson's disease patients: analysis of the PPMI cohort. Neuropsychology. (2019) 33:1111–20. doi: 10.1037/neu0000583
18. Martin, GP, McDonald, KR, Allsop, D, Diggle, PJ, and Leroi, I. Apathy as a behavioural marker of cognitive impairment in Parkinson's disease: a longitudinal analysis. J Neurol. (2020) 267:214–27. doi: 10.1007/s00415-019-09538-z
19. Petkus, AJ, Filoteo, JV, Schiehser, DM, Gomez, ME, and Petzinger, G. Worse cognitive performance predicts increased anxiety and depressive symptoms in patients with Parkinson's disease: a bidirectional analysis. Neuropsychology. (2019) 33:35–46. doi: 10.1037/neu0000498
20. Pirogovsky-Turk, E, Moore, RC, Filoteo, JV, Litvan, I, Song, DD, Lessig, SL, et al. Neuropsychiatric predictors of cognitive decline in Parkinson disease: a longitudinal study. Am J Geriatr Psychiatry. (2017) 25:279–89. doi: 10.1016/j.jagp.2016.10.004
21. Martini, A, Weis, L, Fiorenzato, E, Schifano, R, Cianci, V, Antonini, A, et al. Impact of cognitive profile on impulse control disorders presence and severity in Parkinson's disease. Front Neurol. (2019) 10:266. doi: 10.3389/fneur.2019.00266
22. Siri, C, Cilia, R, Reali, E, Pozzi, B, Cereda, E, Colombo, A, et al. Long-term cognitive follow-up of Parkinson's disease patients with impulse control disorders. Mov Disord. (2015) 30:696–704. doi: 10.1002/mds.26160
23. Szymkowicz, SM, Dotson, VM, Jones, JD, Okun, MS, and Bowers, D. Symptom dimensions of depression and apathy and their relationship with cognition in Parkinson's disease. J Int Neuropsychol Soc. (2018) 24:269–82. doi: 10.1017/S1355617717001011
24. Meijer, FJ, and Goraj, B. Brain MRI in Parkinson's disease. Front Biosci (Elite Ed). (2014) 6:360–9. doi: 10.2741/E711
25. Biundo, R, Formento-Dojot, P, Facchini, S, Vallelunga, A, Ghezzo, L, Foscolo, L, et al. Brain volume changes in Parkinson's disease and their relationship with cognitive and behavioural abnormalities. J Neurol Sci. (2011) 310:64–9. doi: 10.1016/j.jns.2011.08.001
26. Ge, S, Liu, J, Jia, Y, Li, Z, Wang, J, and Wang, M. Topological alteration of the brain structural network in Parkinson's disease with apathy. Brain Res Bull. (2024) 208:110899:110899. doi: 10.1016/j.brainresbull.2024.110899
27. Yu, RL, Lee, WJ, Li, JY, Chang, YY, Chen, CC, Lin, JJ, et al. Evaluating mild cognitive dysfunction in patients with Parkinson's disease in clinical practice in Taiwan. Sci Rep. (2020) 10:1014. doi: 10.1038/s41598-020-58042-2
28. Yu, YW, Tan, CH, Su, HC, Chien, CY, Sung, PS, Lin, TY, et al. A new instrument combines cognitive and social functioning items for detecting mild cognitive impairment and dementia in Parkinson's disease. Front Aging Neurosci. (2022) 14:913958. doi: 10.3389/fnagi.2022.913958
29. Litvan, I, Goldman, JG, Tröster, AI, Schmand, BA, Weintraub, D, Petersen, RC, et al. Diagnostic criteria for mild cognitive impairment in Parkinson's disease: Movement Disorder Society task force guidelines. Mov Disord. (2012) 27:349–56. doi: 10.1002/mds.24893
30. Weintraub, D, Oehlberg, KA, Katz, IR, and Stern, MB. Test characteristics of the 15-item geriatric depression scale and Hamilton depression rating scale in Parkinson disease. Am J Geriatr Psychiatry. (2006) 14:169–75. doi: 10.1097/01.JGP.0000192488.66049.4b
31. Knight, RG, Waal-Manning, HJ, and Spears, GF. Some norms and reliability data for the state--trait anxiety inventory and the Zung self-rating depression scale. Br J Clin Psychol. (1983) 22:110899, 110899–249. doi: 10.1111/j.2044-8260.1983.tb00610.x
32. Weintraub, D, Hoops, S, Shea, JA, Lyons, KE, Pahwa, R, Driver-Dunckley, ED, et al. Validation of the questionnaire for impulsive-compulsive disorders in Parkinson's disease. Mov Disord. (2009) 24:1461–7. doi: 10.1002/mds.22571
33. Goetz, CG, Tilley, BC, Shaftman, SR, Stebbins, GT, Fahn, S, Martinez-Martin, P, et al. Movement Disorder Society-sponsored revision of the unified Parkinson's disease rating scale (MDS-UPDRS): scale presentation and clinimetric testing results. Mov Disord. (2008) 23:2129–70. doi: 10.1002/mds.22340
34. Bernal-Rusiel, JL, Greve, DN, Reuter, M, Fischl, B, and Sabuncu, MR. Statistical analysis of longitudinal neuroimage data with linear mixed effects models. NeuroImage. (2013) 66:249–60. doi: 10.1016/j.neuroimage.2012.10.065
35. Reuter, M, and Fischl, B. Avoiding asymmetry-induced bias in longitudinal image processing. NeuroImage. (2011) 57:19–21. doi: 10.1016/j.neuroimage.2011.02.076
36. Reuter, M, Rosas, HD, and Fischl, B. Highly accurate inverse consistent registration: a robust approach. NeuroImage. (2010) 53:1181–96. doi: 10.1016/j.neuroimage.2010.07.020
37. Wirth, T, Goetsch, T, Corvol, JC, Roze, E, Mariani, LL, Vidailhet, M, et al. Prognosis of impulse control disorders in Parkinson's disease: a prospective controlled study. J Neurol. (2024) 271:2412–22. doi: 10.1007/s00415-023-12170-7
38. Siri, C, Cilia, R, De Gaspari, D, Canesi, M, Meucci, N, Zecchinelli, AL, et al. Cognitive status of patients with Parkinson's disease and pathological gambling. J Neurol. (2010) 257:247–52. doi: 10.1007/s00415-009-5301-5
39. Burgess, N, Maguire, EA, and O'Keefe, J. The human hippocampus and spatial and episodic memory. Neuron. (2002) 35:625–41. doi: 10.1016/s0896-6273(02)00830-9
40. Koechlin, E, Ody, C, and Kouneiher, F. The architecture of cognitive control in the human prefrontal cortex. Science. (2003) 302:1181–5. doi: 10.1126/science.1088545
41. Rolls, ET. The cingulate cortex and limbic systems for emotion, action, and memory. Brain Struct Funct. (2019) 224:3001–18. doi: 10.1007/s00429-019-01945-2
42. Gao, Y, Nie, K, Mei, M, Guo, M, Huang, Z, Wang, L, et al. Changes in cortical thickness in patients with early Parkinson's disease at different Hoehn and Yahr stages. Front Hum Neurosci. (2018) 12:469. doi: 10.3389/fnhum.2018.00469
43. Uribe, C, Segura, B, Baggio, HC, Abos, A, Marti, MJ, Valldeoriola, F, et al. Patterns of cortical thinning in nondemented Parkinson's disease patients. Mov Disord. (2016) 31:699–708. doi: 10.1002/mds.26590
44. Carter, CS, Botvinick, MM, and Cohen, JD. The contribution of the anterior cingulate cortex to executive processes in cognition. Rev Neurosci. (1999) 10:49–57.
45. Caspell-Garcia, C, Simuni, T, Tosun-Turgut, D, Wu, IW, Zhang, Y, Nalls, M, et al. Multiple modality biomarker prediction of cognitive impairment in prospectively followed de novo Parkinson disease. PLoS One. (2017) 12:e0175674:e0175674. doi: 10.1371/journal.pone.0175674
46. Gu, L, Shu, H, Wang, Y, and Xu, H. Exploring brain changes of impulse control disorders in Parkinson's disease: an ALE study. Front Aging Neurosci. (2022) 14:966525. doi: 10.3389/fnagi.2022.966525
47. Li, C, Wang, J, Gui, L, Zheng, J, Liu, C, and Du, H. Alterations of whole-brain cortical area and thickness in mild cognitive impairment and Alzheimer's disease. J Alzheimers Dis. (2011) 27:281–90. doi: 10.3233/JAD-2011-110497
48. Takayanagi, M, Wentz, J, Takayanagi, Y, Schretlen, DJ, Ceyhan, E, Wang, L, et al. Reduced anterior cingulate gray matter volume and thickness in subjects with deficit schizophrenia. Schizophr Res. (2013) 150:484–90. doi: 10.1016/j.schres.2013.07.036
49. Pellicano, C, Niccolini, F, Wu, K, O'Sullivan, SS, Lawrence, AD, Lees, AJ, et al. Morphometric changes in the reward system of Parkinson's disease patients with impulse control disorders. J Neurol. (2015) 262:2653–61. doi: 10.1007/s00415-015-7892-3
50. Cicero, CE, Monastero, R, Terravecchia, C, Donzuso, G, Luca, A, Baschi, R, et al. Influence of drugs on mild cognitive impairment in Parkinson's disease: evidence from the PACOS study. Curr Neuropharmacol. (2022) 20:998–1003. doi: 10.2174/1570159X20666211223122800
51. Tupler, LA, Krishnan, KR, Greenberg, DL, Marcovina, SM, Payne, ME, Mac Fall, JR, et al. Predicting memory decline in normal elderly: genetics, MRI, and cognitive reserve. Neurobiol Aging. (2007) 28:1644–56. doi: 10.1016/j.neurobiolaging.2006.07.001
52. Vitale, C, Santangelo, G, Trojano, L, Verde, F, Rocco, M, Grossi, D, et al. Comparative neuropsychological profile of pathological gambling, hypersexuality, and compulsive eating in Parkinson's disease. Mov Disord. (2011) 26:830–6. doi: 10.1002/mds.23567
Keywords: Parkinson’s disease, impulse control disorders, mild cognitive impairment, cortical thickness, survival analysis
Citation: Xu R, Yang B, Li J, Wei X, Zhang L, Tian J and Zhang W (2025) The impact of impulse control disorders on cognitive decline in de novo Parkinson’s disease: a study based on structural MRI. Front. Neurol. 16:1565046. doi: 10.3389/fneur.2025.1565046
Edited by:
Andrea Perrottelli, University of Campania “Luigi Vanvitelli”, ItalyReviewed by:
Rwei-Ling Yu, National Cheng Kung University, TaiwanShangpei Wang, Second Hospital of Anhui Medical University, China
Copyright © 2025 Xu, Yang, Li, Wei, Zhang, Tian and Zhang. This is an open-access article distributed under the terms of the Creative Commons Attribution License (CC BY). The use, distribution or reproduction in other forums is permitted, provided the original author(s) and the copyright owner(s) are credited and that the original publication in this journal is cited, in accordance with accepted academic practice. No use, distribution or reproduction is permitted which does not comply with these terms.
*Correspondence: Wei Zhang, emhhbmd3ZWlAaG9zcGl0YWwuY3FtdS5lZHUuY24=
†These authors have contributed equally to this work and share first authorship