- 1CNC—Center for Neuroscience and Cell Biology, University of Coimbra, Coimbra, Portugal
- 2Faculty of Medicine, University of Coimbra, Coimbra, Portugal
Extracellular adenosine is produced with increased metabolic activity or stress, acting as a paracrine signal of cellular effort. Adenosine receptors are most abundant in the brain, where adenosine acts through inhibitory A1 receptors to decrease activity/noise and through facilitatory A2A receptors (A2AR) to promote plastic changes in physiological conditions. By bolstering glutamate excitotoxicity and neuroinflammation, A2AR also contribute to synaptic and neuronal damage, as heralded by the neuroprotection afforded by the genetic or pharmacological blockade of A2AR in animal models of ischemia, traumatic brain injury, convulsions/epilepsy, repeated stress or Alzheimer’s or Parkinson’s diseases. A2AR overfunction is not only necessary for the expression of brain damage but is actually sufficient to trigger brain dysfunction in the absence of brain insults or other disease triggers. Furthermore, A2AR overfunction seems to be an early event in the demise of brain diseases, which involves an increased formation of ATP-derived adenosine and an up-regulation of A2AR. This prompts the novel hypothesis that the evaluation of A2AR density in afflicted brain circuits may become an important biomarker of susceptibility and evolution of brain diseases once faithful PET ligands are optimized. Additional relevant biomarkers would be measuring the extracellular ATP and/or adenosine levels with selective dyes, to identify stressed regions in the brain. A2AR display several polymorphisms in humans and preliminary studies have associated different A2AR polymorphisms with altered morphofunctional brain endpoints associated with neuropsychiatric diseases. This further prompts the interest in exploiting A2AR polymorphic analysis as an ancillary biomarker of susceptibility/evolution of brain diseases.
Introduction
The increased use of intracellular ATP, either because of increased workload or need to cope with stressful conditions, is a main source of increased extracellular levels of adenosine, which generally acts as a paracrine allostatic regulator by locally decreasing metabolism through inhibitory A1 receptors (A1R) and increasing metabolic supply through A2AR (Agostinho et al., 2020). Adenosine receptors are most abundant in the brain, where adenosine fulfills a role as neuromodulator apart from its general paracrine allostatic role: post-synaptic as well as astrocytic integrative activity are major contributors of an adenosine tone acting through inhibitory A1 receptors to decrease activity/noise in excitatory synapses; ATP release, characteristic of increased firing rate conditions associated with synaptic plasticity, is the major source of a second pool of synaptic extracellular adenosine selectively activating facilitatory A2A receptors (A2AR) to promote synaptic plastic changes in physiological conditions (Cunha, 2016). However, ATP is also a general danger signal in the brain (Rodrigues et al., 2015), acting through a variety of ATP/ADP-activated P2 receptors to re-shape the function of astrocytes and microglia to cope with potential threats (Agostinho et al., 2020). Such threats also require adaptive plastic changes in neuronal circuits, which may explain the increased extracellular formation of ATP-derived adenosine by ecto-nucleotidases, with a burst of its rate-limiting step—ecto-5′-nucleotidase or CD73 (Cunha, 2001)—under noxious brain conditions to sustain an overfunction of A2AR that contributes to synaptotoxicity and neurotoxicity in different brain diseases (Cunha, 2016).
Adenosine A2A Receptors in Brain Diseases
Upon acute brain injury, probably best exemplified by an ischemic brain stroke, concurrent pharmacological and genetic evidence show that A2AR blockade affords a robust neuroprotection (reviewed in Chen and Pedata, 2008). In parallel, ischemia is accompanied by ATP release (Melani et al., 2005) and up-regulation of CD73 (Braun et al., 1997), thus increasing the formation of extracellular ATP-derived adenosine (Koos et al., 1997; Chu et al., 2014). Likewise, seizure-like activity characteristic of epileptic conditions triggers a neurodegeneration that is critically controlled by pharmacological or genetic A2AR blockade (Canas et al., 2018). Seizure activity also increases ATP release (Wieraszko and Seyfried, 1989) and up-regulates CD73 (e.g., Schoen et al., 1999; Rebola et al., 2003), increasing the contribution of extracellular ATP-derived adenosine formation to overactivate A2AR (reviewed in Tescarollo et al., 2020). A2AR blockade also attenuates brain damage following traumatic brain injury (TBI) (e.g., Li et al., 2009); TBI also bolsters the release of ATP (Faroqi et al., 2021) and CD73 levels (Zheng et al., 2020), although the contribution of extracellular ATP-derived adenosine has not yet been tested in TBI.
Overall, this evidence is compatible with an increase of extracellular adenosine, namely extracellular ATP-derived adenosine, leading to an overactivation of A2AR that contributes for brain dysfunction upon acute brain injury. A similar scenario seems to occur in chronic brain conditions. Thus, the pharmacological or genetic blockade of A2AR affords a consistent neuroprotection in animal models of Alzheimer’s disease (AD) (e.g., Canas et al., 2009; Laurent et al., 2016; Viana da Silva et al., 2016), Parkinson’s disease (PD) (reviewed in Schwarzschild et al., 2006)—where A2AR antagonists were approved by the US-FDA as novel anti-Parkinsonian drugs (Chen and Cunha, 2020), repeated stress/depression (Batalha et al., 2013; Kaster et al., 2015; Padilla et al., 2018), Machado-Joseph disease (Gonçalves et al., 2013), amyotrophic lateral sclerosis (ALS) (Ng et al., 2015; Rei et al., 2020; Seven et al., 2020), Angelman syndrome (Moreira-de-Sá et al., 2020, 2021), or glaucoma-like disorders (Madeira et al., 2015). Most of these chronic neuropsychiatric conditions are also associated with increased release of ATP, as occurs in animal models of AD (Gonçalves et al., 2019), PD (Carmo et al., 2019; Meng et al., 2019) or as concluded by the anti-depressant effects of P2 receptor antagonists (Ribeiro et al., 2019; but see Cao et al., 2013). Moreover, there is an increased contribution of extracellular ATP-derived adenosine for A2AR overactivation in chronic brain diseases, as best heralded by the observation that CD73 knockout mice phenocopy A2AR knockout mice (Augusto et al., 2013; Carmo et al., 2019; Gonçalves et al., 2019).
A2AR overactivation is not only necessary, but actually sufficient to trigger brain dysfunction, as concluded from the observation that the pharmacological overactivation of A2AR (Pagnussat et al., 2015), the optogenetic activation of A2AR transducing system (Li et al., 2015) or the over-expression of A2AR in the hippocampus (Coelho et al., 2014; Carvalho et al., 2019; Temido-Ferreira et al., 2020) are sufficient to trigger or aggravate brain dysfunction. Notably, A2AR overfunction seems to be an early event in different brain disorders (reviewed in Cunha, 2016), although A2AR antagonists seem to maintain their neuroprotective profile after the establishment of symptoms (e.g., Kaster et al., 2015; Faivre et al., 2018; Orr et al., 2018; Silva et al., 2018).
The tight association between increased release of ATP and its extracellular catabolism to overactivate A2AR as part of the expression of neuronal dysfunction at the onset and throughout the evolution of several brain diseases prompts exploiting this danger signaling pathway as new biomarkers to identify dysfunctional brain circuits in brain diseases. Although the tools are yet to developed, it may be promising to devise soluble sensors to detect altered levels of extracellular ATP to allow an in vivo estimate of brain circuits undergoing a particular purinergic pressure and, consequently, are at risk of undergoing dysfunction. An alternative could be the development of PET ligands (not yet available) to assess the density of CD73, which is paramount to link ATP upsurge with the selective overactivation of A2AR; CD73 seems to be consistently up-regulated upon brain stressful conditions and may be a selective biomarker of glia and synapses undergoing adaptive processes (Schoen and Kreutzberg, 1997).
Up-Regulation of Adenosine A2A Receptors in Brain Diseases
The A2AR overactivation associated with brain dysfunction and disease is not only sustained by an increased bioavailability of the trigger of A2AR—ATP-derived extracellular adenosine—but also involves an up-regulation of A2AR in the afflicted brain areas (reviewed in Cunha, 2016). Indeed, an increased density of cortical A2AR has been reported in animal models of epilepsy (Rebola et al., 2005; Cognato et al., 2010; Canas et al., 2018; Crespo et al., 2018), Rasmussen’s encephalopathy (He et al., 2020), TBI (Zhao et al., 2017), AD (Espinosa et al., 2013; Viana da Silva et al., 2016; Silva et al., 2018), Lyme neuroborreliosis (Smith et al., 2014), ALS (Seven et al., 2020), or chronic stress/depression (Kaster et al., 2015; Machado et al., 2017), as well as in the diseased human brain (Albasanz et al., 2008; Temido-Ferreira et al., 2020). Likewise, A2AR levels are also increased in the cerebellum of Machado-Joseph’s ataxic mice (Gonçalves et al., 2013) and in the amygdala or fear-conditioned mice (Simões et al., 2016). A2AR up-regulation is in fact an upsurge since it occurs shortly (within hours) after abnormal neuronal function (i.e., convulsions; Canas et al., 2018), but it gradually increases with aggravation of brain dysfunction (Temido-Ferreira et al., 2020). A2AR up-regulation mostly occurs in synapses, in accordance with the involvement of synaptic alterations at the onset of most brain diseases (e.g., Rebola et al., 2005; Kaster et al., 2015; Viana da Silva et al., 2016; Canas et al., 2018), but is also observed in glia cells in the progression of chronic brain diseases (Matos et al., 2012; Orr et al., 2015; Barros-Barbosa et al., 2016; Patodia et al., 2020). It is still unclear if this A2AR up-regulation only involves an increased readout of A2AR mRNAs (Canas et al., 2018) or also involves an overexpression of A2AR mRNA, which has been reported in the dysfunctional or diseased brain (e.g., Costenla et al., 2011; Espinosa et al., 2013; Hu et al., 2016; Dias et al., 2021). In fact, the triggers and mechanisms of this A2AR up-regulation in the diseased brain are essentially unknown. The A2AR gene in both rodents and humans has a complex promoter region and can give raise to multiple transcripts (Peterfreund et al., 1996; Lee et al., 2003a; Yu et al., 2004; Kreth et al., 2008; Huin et al., 2019). Although multiple controllers of the A2AR gene have been proposed, such as methylation patterns of the promoter (Falconi et al., 2019; Micioni Di Bonaventura et al., 2019), transcription factors ZBP-89 and Yin Yang-1 (Buira et al., 2010), microRNAs (e.g., Heyn et al., 2012; Villar-Menéndez et al., 2014; Zhao et al., 2015; Tian et al., 2016), NFk-B (Morello et al., 2006), cAMP-response element-binding protein (Chiang et al., 2005), hypoxia inducible factor-2α (Ahmad et al., 2009; Brown et al., 2011), AP1 transcription factor (Kobayashi and Millhorn, 1999; Lee et al., 2014), or nuclear factor 1 (Lee et al., 2003b), the regulation of the relative expression of these transcripts is largely unknown (Yu et al., 2004; Huin et al., 2019) and little is also known about the relative stability of the different mRNA transcripts. This is certainly an area of research that might open new avenues to design neuroprotective strategies linked to A2AR.
The association of A2AR up-regulation with brain diseases offers another promising opportunity to develop informative biomarkers of the susceptibility and/or evolution of different brain diseases once PET ligands are optimized to detect extra-striatal A2AR. In fact, A2AR throughout the brain are most abundant in the striatum (reviewed in Svenningsson et al., 1999) and the available PET ligands have been optimized to detect striatal A2AR (e.g., Mishina et al., 2011; Ishibashi et al., 2018); however, this population of A2AR has a different pharmacology (Orrú et al., 2011; Cunha, 2016), a different adaptive profile (Cunha et al., 1995) and a different role in most brain conditions (Shen et al., 2008, 2013; Yu et al., 2008; Wei et al., 2014). Thus, it is likely that the currently available PET ligands might not be useful to assess modifications of extra-striatal A2AR. New cortical A2AR-directed PET ligands need to be designed based on the particular properties and interacting partners of cortical A2AR (reviewed in Franco et al., 2020) to allow an in vivo detection of A2AR upsurge as potential general biomarkers of brain dysfunction (Sun et al., 2020).
A2AR are not only located in the brain, but are also present in several peripheral tissues, namely in different blood cells such as leukocytes and platelets (reviewed in Gessi et al., 2000). Based on the association of brain diseases with A2AR up-regulation in afflicted brain regions, several studies explored if A2AR in blood cells could be biomarkers of brain diseases, such as AD (Arosio et al., 2010, 2016; Merighi et al., 2021), PD (Falconi et al., 2019), or ALS (Vincenzi et al., 2013). However, only the understanding of the mechanisms underlying A2AR up-regulation in brain diseases will allow providing a rationale (or lack of thereof) to consider alterations of the density of peripheral A2AR as valid readouts of altered A2AR density that occurs selectively in afflicted brain circuits in the diseased brain.
Polymorphisms of Adenosine A2A Receptors and Brain Diseases
The gene encoding human A2AR (ADORA2A gene) harbors several single nucleotide polymorphisms (SPNs), which have been associated to an altered susceptibility to several neuropsychiatric and neurodegenerative disorders (Huin et al., 2019). In fact, as listed in Tables 1A,B, naturally occurring variabilities in the ADORA2A gene collectively influence predisposition risk and even age of onset for several CNS disorders as well as individual susceptibility to the anxiogenic and sleep-related consequences of caffeine. Although, it is still unknown if the different A2AR polymorphisms are associated with a different expression, subcellular location, trafficking, heteromerization or pharmacological properties of A2AR, the relation between A2AR polymorphisms and the susceptibility and age of onset of brain dysfunction prompts the interest in exploiting A2AR polymorphic analysis as an ancillary biomarker of susceptibility/evolution of brain diseases.
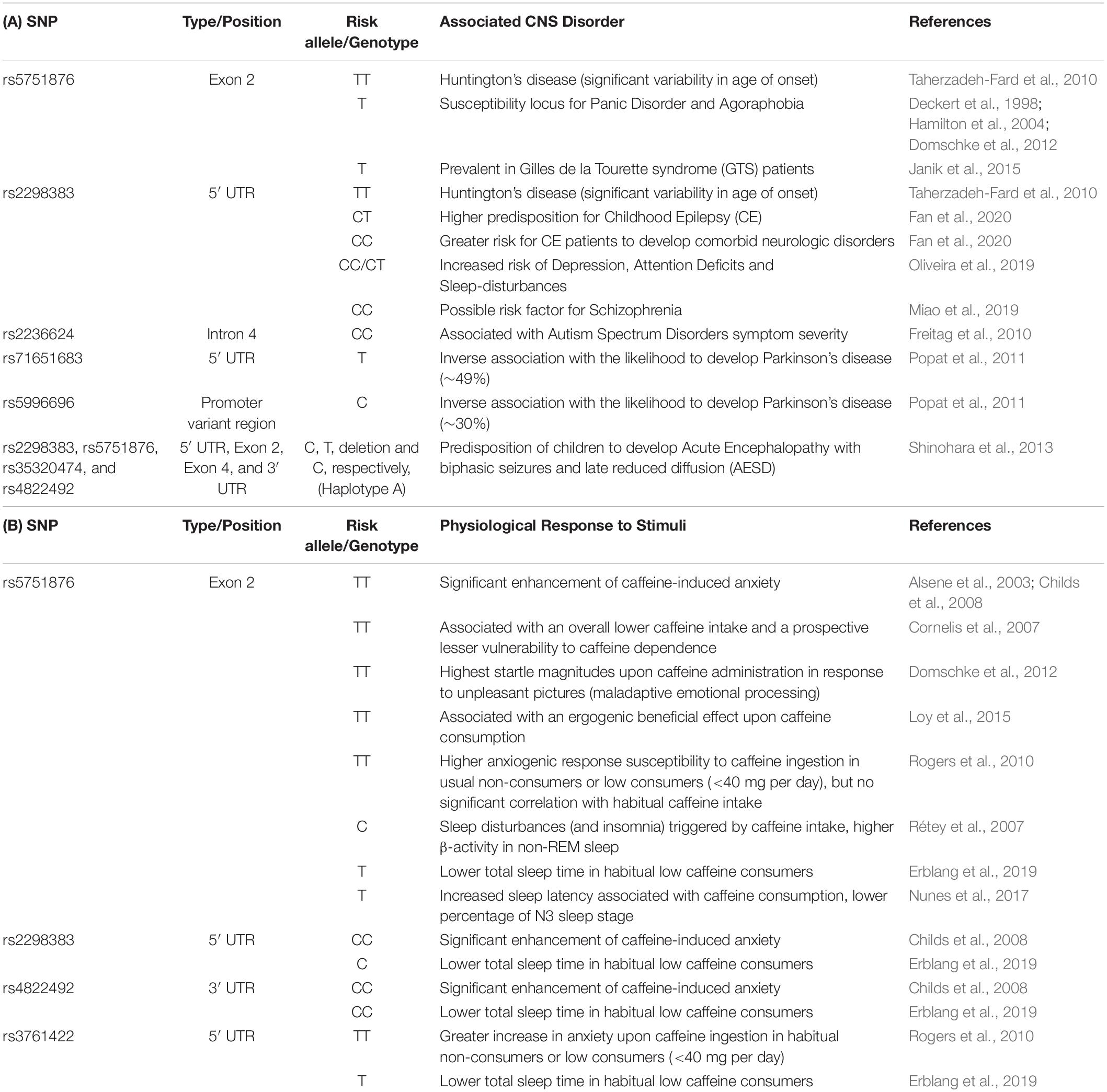
Table 1. Summary of the reported associations between known polymorphic variants of the human adenosine A2A receptor gene (ADORA2A) and different response susceptibility to either pathological threats (A) or distinct physiological responses to external stimulus (B).
Discussion
A2AR overfunction is necessary and actually sufficient for the expression of neuronal dysfunction upon brain diseases. In particular, A2AR overfunction associated with aberrant synaptic plasticity and synaptotoxicity seems to be associated with the onset of symptoms of brain diseases. However, some of these symptoms are comorbidities of other brain diseases, associated with their aggravation, which often involves a spreading of neuroinflammation, also known to be controlled by A2AR. Thus, it is also likely that A2AR overfunction might be also associated with the evolution of brain diseases. These neuropathological roles of A2AR prompts considering the exploitation of this system as candidate biomarkers of the susceptibility and evolution of brain diseases. The development of PET ligand with adequate signal-to-noise ratio and selectivity to detect the relevant extra-striatal A2AR may allow a minimally invasive assessment of A2AR in different brain regions. This may be complemented by the definition of A2AR polymorphisms as an ancillary biomarker for the susceptibility and evolution of brain diseases, which still requires a firm establishment of structural-functional relationships between A2AR polymorphisms and brain dysfunction. Finally, the future development of PET-based sensors of extracellular ATP and/or adenosine may well be of additional interest as a biomarker of the status of brain diseases to be used in complement of other available methods.
Author Contributions
All authors contribute to the organization and writing of the review.
Funding
This study was supported by La Caixa Foundation (LCF/PR/HP17/52190001), Centro 2020 (CENTRO-01-0145-FEDER-000008:BrainHealth 2020 and CENTRO-01-0246-FEDER-000010), and FCT (POCI-01-0145-FEDER-03127 and UIDB/04539/2020).
Conflict of Interest
RC is a scientific consultant for the Institute for Scientific Information on Coffee.
The remaining authors declare that the research was conducted in the absence of any commercial or financial relationships that could be construed as a potential conflict of interest.
References
Agostinho, P., Madeira, D., Dias, L., Simões, A. P., Cunha, R. A., and Canas, P. M. (2020). Purinergic signaling orchestrating neuron-glia communication. Pharmacol. Res. 162:105253. doi: 10.1016/j.phrs.2020.105253
Ahmad, A., Ahmad, S., Glover, L., Miller, S. M., Shannon, J. M., Guo, X., et al. (2009). Adenosine A2A receptor is a unique angiogenic target of HIF-2alpha in pulmonary endothelial cells. Proc. Natl. Acad. Sci. USA 106, 10684–10689. doi: 10.1073/pnas.0901326106
Albasanz, J. L., Perez, S., Barrachina, M., Ferrer, I., and Martín, M. (2008). Up-regulation of adenosine receptors in the frontal cortex in Alzheimer’s disease. Brain Pathol. 18, 211–219. doi: 10.1111/j.1750-3639.2007.00112.x
Alsene, K., Deckert, J., Sand, P., and de Wit, H. (2003). Association between A2A receptor gene polymorphisms and caffeine-induced anxiety. Neuropsychopharmacology 28, 1694–1702. doi: 10.1038/sj.npp.1300232
Arosio, B., Casati, M., Gussago, C., Ferri, E., Abbate, C., Scortichini, V., et al. (2016). Adenosine type A2A receptor in peripheral cell from patients with Alzheimer’s disease, vascular dementia, and idiopathic normal pressure hydrocephalus: a new/old potential target. J. Alzheimers. Dis. 54, 417–425. doi: 10.3233/JAD-160324
Arosio, B., Viazzoli, C., Mastronardi, L., Bilotta, C., Vergani, C., and Bergamaschini, L. (2010). Adenosine A2A receptor expression in peripheral blood mononuclear cells of patients with mild cognitive impairment. J. Alzheimers. Dis. 20, 991–996. doi: 10.3233/JAD-2010-090814
Augusto, E., Matos, M., Sevigny, J., El-Tayeb, A., Bynoe, M. S., Müller, C. E., et al. (2013). Ecto-5′-nucleotidase (CD73)-mediated formation of adenosine is critical for the striatal adenosine A2A receptor functions. J. Neurosci. 33, 11390–11399. doi: 10.1523/JNEUROSCI.5817-12.2013
Barros-Barbosa, A. R., Ferreirinha, F., Oliveira, A., Mendes, M., Lobo, M. G., Santos, A., et al. (2016). Adenosine A2A receptor and ecto-5′-nucleotidase/CD73 are upregulated in hippocampal astrocytes of human patients with mesial temporal lobe epilepsy (MTLE). Purinergic Signal. 12, 719–734. doi: 10.1007/s11302-016-9535-2
Batalha, V. L., Pego, J. M., Fontinha, B. M., Costenla, A. R., Valadas, J. S., Baqi, Y., et al. (2013). Adenosine A2A receptor blockade reverts hippocampal stress-induced deficits and restores corticosterone circadian oscillation. Mol. Psychiatry 18, 320–331. doi: 10.1038/mp.2012.8
Braun, N., Lenz, C., Gillardon, F., Zimmermann, M., and Zimmermann, H. (1997). Focal cerebral ischemia enhances glial expression of ecto-5′-nucleotidase. Brain Res. 766, 213–226. doi: 10.1016/s0006-8993(97)00559-3
Brown, S. T., Reyes, E. P., and Nurse, C. A. (2011). Chronic hypoxia upregulates adenosine 2A receptor expression in chromaffin cells via hypoxia inducible factor-2alpha: role in modulating secretion. Biochem. Biophys. Res. Commun. 412, 466–472. doi: 10.1016/j.bbrc.2011.07.122
Buira, S. P., Dentesano, G., Albasanz, J. L., Moreno, J., Martín, M., Ferrer, I., et al. (2010). DNA methylation and Yin Yang-1 repress adenosine A2A receptor levels in human brain. J. Neurochem. 115, 283–295. doi: 10.1111/j.1471-4159.2010.06928.x
Canas, P. M., Porciúncula, L. O., Cunha, G. M., Silva, C. G., Machado, N. J., Oliveira, J. M., et al. (2009). Adenosine A2A receptor blockade prevents synaptotoxicity and memory dysfunction caused by beta-amyloid peptides via p38 mitogen-activated protein kinase pathway. J. Neurosci. 29, 14741–14751. doi: 10.1523/jneurosci.3728-09.2009
Canas, P. M., Porciúncula, L. O., Simões, A. P., Augusto, E., Silva, H. B., Machado, N. J., et al. (2018). Neuronal adenosine A2A receptors are critical mediators of neurodegeneration triggered by convulsions. eNeuro 5:ENEURO.0385-18.2018. doi: 10.1523/ENEURO.0385-18.2018
Cao, X., Li, L. P., Wang, Q., Wu, Q., Hu, H. H., Zhang, M., et al. (2013). Astrocyte-derived ATP modulates depressive-like behaviors. Nat. Med. 19, 773–777. doi: 10.1038/nm.3162
Carmo, M., Gonçalves, F. Q., Canas, P. M., Oses, J. P., Fernandes, F. D., Duarte, F. V., et al. (2019). Enhanced ATP release and CD73-mediated adenosine formation sustain adenosine A2A receptor over-activation in a rat model of Parkinson’s disease. Br. J. Pharmacol. 176, 3666–3680. doi: 10.1111/bph.14771
Carvalho, K., Faivre, E., Pietrowski, M. J., Marques, X., Gomez-Murcia, V., Deleau, A., et al. (2019). Exacerbation of C1q dysregulation, synaptic loss and memory deficits in tau pathology linked to neuronal adenosine A2A receptor. Brain 142, 3636–3654. doi: 10.1093/brain/awz288
Chen, J. F., and Pedata, F. (2008). Modulation of ischemic brain injury and neuroinflammation by adenosine A2A receptors. Curr. Pharm. Des. 14, 1490–1499. doi: 10.2174/138161208784480126
Chen, J., and Cunha, R. A. (2020). The belated US FDA approval of the adenosine A2A receptor antagonist istradefylline for treatment of Parkinson’s disease. Purinergic Signal. 16, 167–174. doi: 10.1007/s11302-020-09694-2
Chiang, M. C., Lee, Y. C., Huang, C. L., and Chern, Y. (2005). cAMP-response element-binding protein contributes to suppression of the A2A adenosine receptor promoter by mutant Huntingtin with expanded polyglutamine residues. J. Biol. Chem. 280, 14331–14340. doi: 10.1074/jbc.M413279200
Childs, E., Hohoff, C., Deckert, J., Xu, K., Badner, J., and de Wit, H. (2008). Association between ADORA2A and DRD2 polymorphisms and caffeine-induced anxiety. Neuropsychopharmacology 33, 2791–2800. doi: 10.1038/npp.2008.17
Chu, S., Xiong, W., and Parkinson, F. E. (2014). Effect of ecto-5′-nucleotidase (eN) in astrocytes on adenosine and inosine formation. Purinergic Signal. 10, 603–609. doi: 10.1007/s11302-014-9421-8
Coelho, J. E., Alves, P., Canas, P. M., Valadas, J. S., Shmidt, T., Batalha, V. L., et al. (2014). Overexpression of adenosine A2A receptors in rats: effects on depression, locomotion, and anxiety. Front. Psychiatry 5:67. doi: 10.3389/fpsyt.2014.00067
Cognato, G. P., Agostinho, P. M., Hockemeyer, J., Müller, C. E., Souza, D. O., and Cunha, R. A. (2010). Caffeine and an adenosine A2A receptor antagonist prevent memory impairment and synaptotoxicity in adult rats triggered by a convulsive episode in early life. J. Neurochem. 112, 453–462. doi: 10.1111/j.1471-4159.2009.06465.x
Cornelis, M. C., El-Sohemy, A., and Campos, H. (2007). Genetic polymorphism of the adenosine A2A receptor is associated with habitual caffeine consumption. Am. J. Clin. Nutr. 86, 240–244. doi: 10.1093/ajcn/86.1.240
Costenla, A. R., Diógenes, M. J., Canas, P. M., Rodrigues, R. J., Nogueira, C., Maroco, J., et al. (2011). Enhanced role of adenosine A2A receptors in the modulation of LTP in the rat hippocampus upon ageing. Eur. J. Neurosci. 34, 12–21. doi: 10.1111/j.1460-9568.2011.07719.x
Crespo, M., León-Navarro, D. A., and Martín, M. (2018). Early-life hyperthermic seizures upregulate adenosine A2A receptors in the cortex and promote depressive-like behavior in adult rats. Epilepsy Behav. 86, 173–178. doi: 10.1016/j.yebeh.2018.06.048
Cunha, R. A. (2001). Regulation of the ecto-nucleotidase pathway in rat hippocampal nerve terminals. Neurochem. Res. 26, 979–991. doi: 10.1023/a:1012392719601
Cunha, R. A. (2016). How does adenosine control neuronal dysfunction and neurodegeneration? J. Neurochem. 139, 1019–1055. doi: 10.1111/jnc.13724
Cunha, R. A., Constantino, M. C., Sebastião, A. M., and Ribeiro, J. A. (1995). Modification of A1 and A2A adenosine receptor binding in aged striatum, hippocampus and cortex of the rat. Neuroreport 6, 1583–1588. doi: 10.1097/00001756-199507310-00029
Deckert, J., Nöthen, M. M., Franke, P., Delmo, C., Fritze, J., Knapp, M., et al. (1998). Systematic mutation screening and association study of the A1 and A2A adenosine receptor genes in panic disorder suggest a contribution of the A2A gene to the development of disease. Mol. Psychiatry 3, 81–85. doi: 10.1038/sj.mp.4000345
Dias, L., Lopes, C. R., Gonçalves, F. Q., Nunes, A., Pochmann, D., Machado, N. J., et al. (2021). Crosstalk between ATP-P2X7 and adenosine A2A receptors controlling neuroinflammation in rats subject to repeated restraint stress. Front. Cell. Neurosci. 15:639322. doi: 10.3389/fncel.2021.639322
Domschke, K., Gajewska, A., Winter, B., Herrmann, M. J., Warrings, B., Muhlberger, A., et al. (2012). ADORA2A Gene variation, caffeine, and emotional processing: a multi-level interaction on startle reflex. Neuropsychopharmacology 37, 759–769. doi: 10.1038/npp.2011.253
Erblang, M., Drogou, C., Gomez-Merino, D., Metlaine, A., Boland, A., Deleuze, J. F., et al. (2019). The impact of genetic variations in ADORA2A in the association between caffeine consumption and sleep. Genes 10:1012. doi: 10.3390/genes10121021
Espinosa, J., Rocha, A., Nunes, F., Costa, M. S., Schein, V., Kazlauckas, V., et al. (2013). Caffeine consumption prevents memory impairment, neuronal damage, and adenosine A2A receptors upregulation in the hippocampus of a rat model of sporadic dementia. J. Alzheimers. Dis. 34, 509–518. doi: 10.3233/JAD-111982
Faivre, E., Coelho, J. E., Zornbach, K., Malik, E., Baqi, Y., Schneider, M., et al. (2018). Beneficial effect of a selective adenosine A2A receptor antagonist in the APPswe/PS1dE9 mouse model of Alzheimer’s disease. Front. Mol. Neurosci. 11:235. doi: 10.3389/fnmol.2018.00235
Falconi, A., Bonito-Oliva, A., Di Bartolomeo, M., Massimini, M., Fattapposta, F., Locuratolo, N., et al. (2019). On the role of adenosine A2A receptor gene transcriptional regulation in Parkinson’s disease. Front. Neurosci. 13:683. doi: 10.3389/fnins.2019.00683
Fan, X., Chen, Y., Li, W., Xia, H., Liu, B., Guo, H., et al. (2020). Genetic polymorphism of ADORA2A is associated with the risk of epilepsy and predisposition to neurologic comorbidity in Chinese southern children. Front. Neurosci. 14:590605. doi: 10.3389/fnins.2020.590605
Faroqi, A. H., Lim, M. J., Kee, E. C., Lee, J. H., Burgess, J. D., Chen, R., et al. (2021). In vivo detection of extracellular adenosine triphosphate in a mouse model of traumatic brain injury. J. Neurotrauma 38, 655–664. doi: 10.1089/neu.2020.7226
Franco, R., Rivas-Santisteban, R., Reyes-Resina, I., and Navarro, G. (2020). The old and new visions of biased agonism through the prism of adenosine receptor signaling and receptor/receptor and receptor/protein interactions. Front. Pharmacol. 11:628601. doi: 10.3389/fphar.2020.628601
Freitag, C. M., Agelopoulos, K., Huy, E., Rothermundt, M., Krakowitzky, P., Meyer, J., et al. (2010). Adenosine A2A receptor gene ADORA2A variants may increase autistic symptoms and anxiety in autism spectrum disorder. Eur. Child Adolesc. Psychiatry 19, 67–74. doi: 10.1007/s00787-009-0043-6
Gessi, S., Varani, K., Merighi, S., Ongini, E., and Borea, P. A. (2000). A2A adenosine receptors in human peripheral blood cells. Br. J. Pharmacol. 129, 2–11. doi: 10.1038/sj.bjp.0703045
Gonçalves, F. Q., Lopes, J. P., Silva, H. B., Lemos, C., Silva, A. C., Gonçalves, N., et al. (2019). Synaptic and memory dysfunction in a beta-amyloid model of early Alzheimer’s disease depends on increased formation of ATP-derived extracellular adenosine. Neurobiol. Dis. 132:104570. doi: 10.1016/j.nbd.2019.104570
Gonçalves, N., Simões, A. T., Cunha, R. A., and de Almeida, L. P. (2013). Caffeine and adenosine A2A receptor inactivation decrease striatal neuropathology in a lentiviral-based model of Machado-Joseph disease. Ann. Neurol. 73, 655–666. doi: 10.1002/ana.23866
Hamilton, S. P., Slager, S. L., De Leon, A. B., Heiman, G. A., Klein, D. F., Hodge, S. E., et al. (2004). Evidence for genetic linkage between a polymorphism in the adenosine A2A receptor and panic disorder. Neuropsychopharmacology 29, 558–565. doi: 10.1038/sj.npp.1300311
He, X., Chen, F., Zhang, Y., Gao, Q., Guan, Y., Wang, J., et al. (2020). Upregulation of adenosine A2A receptor and downregulation of GLT1 is associated with neuronal cell death in Rasmussen’s encephalitis. Brain Pathol. 30, 246–260. doi: 10.1111/bpa.12770
Heyn, J., Ledderose, C., Hinske, L. C., Limbeck, E., Mohnle, P., Lindner, H. A., et al. (2012). Adenosine A2A receptor upregulation in human PMNs is controlled by miRNA-214, miRNA-15, and miRNA-16. Shock 37, 156–163. doi: 10.1097/SHK.0b013e31823f16bc
Hu, Q., Ren, X., Liu, Y., Li, Z., Zhang, L., Chen, X., et al. (2016). Aberrant adenosine A2A receptor signaling contributes to neurodegeneration and cognitive impairments in a mouse model of synucleinopathy. Exp. Neurol. 283, 213–223. doi: 10.1016/j.expneurol.2016.05.040
Huin, V., Dhaenens, C.-M., Homa, M., Carvalho, K., Buée, L., and Sablonnière, B. (2019). Neurogenetics of the human adenosine receptor genes: genetic structures and involvement in brain diseases. J. Caffeine Adenosine Res. 9, 73–88. doi: 10.1089/caff.2019.0011
Ishibashi, K., Miura, Y., Wagatsuma, K., Toyohara, J., Ishiwata, K., and Ishii, K. (2018). Occupancy of adenosine A2A receptors by istradefylline in patients with Parkinson’s disease using 11C-preladenant PET. Neuropharmacology 143, 106–112. doi: 10.1016/j.neuropharm.2018.09.036
Janik, P., Berdynski, M., Safranow, K., and Zekanowski, C. (2015). Association of ADORA1 rs2228079 and ADORA2A rs5751876 polymorphisms with Gilles de la Tourette syndrome in the Polish population. PLoS One 10:e0136754. doi: 10.1371/journal.pone.0136754
Kaster, M. P., Machado, N. J., Silva, H. B., Nunes, A., Ardais, A. P., Santana, M., et al. (2015). Caffeine acts through neuronal adenosine A2A receptors to prevent mood and memory dysfunction triggered by chronic stress. Proc. Natl. Acad. Sci. USA 112, 7833–7838. doi: 10.1073/pnas.1423088112
Kobayashi, S., and Millhorn, D. E. (1999). Stimulation of expression for the adenosine A2A receptor gene by hypoxia in PC12 cells. A potential role in cell protection. J. Biol. Chem. 274, 20358–20365. doi: 10.1074/jbc.274.29.20358
Koos, B. J., Kruger, L., and Murray, T. F. (1997). Source of extracellular brain adenosine during hypoxia in fetal sheep. Brain Res. 778, 439–442. doi: 10.1016/s0006-8993(97)01207-9
Kreth, S., Ledderose, C., Kaufmann, I., Groeger, G., and Thiel, M. (2008). Differential expression of 5′-UTR splice variants of the adenosine A2A receptor gene in human granulocytes: identification, characterization, and functional impact on activation. FASEB J. 22, 3276–3286. doi: 10.1096/fj.07-101097
Laurent, C., Burnouf, S., Ferry, B., Batalha, V. L., Coelho, J. E., Baqi, Y., et al. (2016). A2A adenosine receptor deletion is protective in a mouse model of Tauopathy. Mol. Psychiatry 21, 97–107. doi: 10.1038/mp.2015.115
Lee, C. F., Lai, H. L., Lee, Y. C., Chien, C. L., and Chern, Y. (2014). The A2A adenosine receptor is a dual coding gene: a novel mechanism of gene usage and signal transduction. J. Biol. Chem. 289, 1257–1270. doi: 10.1074/jbc.M113.509059
Lee, Y. C., Chien, C. L., Sun, C. N., Huang, C. L., Huang, N. K., Chiang, M. C., et al. (2003a). Characterization of the rat A2A adenosine receptor gene: a 4.8-kb promoter-proximal DNA fragment confers selective expression in the central nervous system. Eur. J. Neurosci. 18, 1786–1796. doi: 10.1046/j.1460-9568.2003.02907.x
Lee, Y. C., Lai, H. L., Sun, C. N., Chien, C. L., and Chern, Y. (2003b). Identification of nuclear factor 1 (NF1) as a transcriptional modulator of rat A2A adenosine receptor. Brain Res. Mol. Brain Res. 111, 61–73. doi: 10.1016/s0169-328x(02)00670-8
Li, P., Rial, D., Canas, P. M., Yoo, J. H., Li, W., Zhou, X., et al. (2015). Optogenetic activation of intracellular adenosine A2A receptor signaling in the hippocampus is sufficient to trigger CREB phosphorylation and impair memory. Mol. Psychiatry 20, 1339–1349. doi: 10.1038/mp.2015.43
Li, W., Dai, S., An, J., Xiong, R., Li, P., Chen, X., et al. (2009). Genetic inactivation of adenosine A2A receptors attenuates acute traumatic brain injury in the mouse cortical impact model. Exp. Neurol. 215, 69–76. doi: 10.1016/j.expneurol.2008.09.012
Loy, B. D., O’Connor, P. J., Lindheimer, J. B., and Covert, S. F. (2015). Caffeine is ergogenic for adenosine A2A receptor gene (ADORA2A) T allele homozygotes: a pilot study. J. Caffeine Res. 5, 73–81. doi: 10.1089/jcr.2014.0035
Machado, N. J., Simões, A. P., Silva, H. B., Ardais, A. P., Kaster, M. P., Garção, P., et al. (2017). Caffeine reverts memory but not mood impairment in a depression-prone mouse strain with up-regulated adenosine A2A receptor in hippocampal glutamate synapses. Mol. Neurobiol. 54, 1552–1563. doi: 10.1007/s12035-016-9774-9
Madeira, M. H., Elvas, F., Boia, R., Gonçalves, F. Q., Cunha, R. A., Ambrósio, A. F., et al. (2015). Adenosine A2AR blockade prevents neuroinflammation-induced death of retinal ganglion cells caused by elevated pressure. J. Neuroinflammation 12:115. doi: 10.1186/s12974-015-0333-5
Matos, M., Augusto, E., Machado, N. J., dos Santos-Rodrigues, A., Cunha, R. A., and Agostinho, P. (2012). Astrocytic adenosine A2A receptors control the amyloid-beta peptide-induced decrease of glutamate uptake. J. Alzheimers. Dis. 31, 555–567. doi: 10.3233/JAD-2012-120469
Melani, A., Turchi, D., Vannucchi, M. G., Cipriani, S., Gianfriddo, M., and Pedata, F. (2005). ATP extracellular concentrations are increased in the rat striatum during in vivo ischemia. Neurochem. Int. 47, 442–448. doi: 10.1016/j.neuint.2005.05.014
Meng, F., Guo, Z., Hu, Y., Mai, W., Zhang, Z., Zhang, B., et al. (2019). CD73-derived adenosine controls inflammation and neurodegeneration by modulating dopamine signalling. Brain 142, 700–718. doi: 10.1093/brain/awy351
Merighi, S., Battistello, E., Casetta, I., Gragnaniello, D., Poloni, T. E., Medici, V., et al. (2021). Upregulation of cortical A2A adenosine receptors is reflected in platelets of patients with Alzheimer’s disease. J. Alzheimers. Dis. 80, 1105–1117. doi: 10.3233/jad-201437
Miao, J., Liu, L., Yan, C., Zhu, X., Fan, M., Yu, P., et al. (2019). Association between ADORA2A gene polymorphisms and schizophrenia in the North Chinese Han population. Neuropsychiatr. Dis. Treat. 15, 2451–2458. doi: 10.2147/NDT.S205014
Micioni Di Bonaventura, M. V., Pucci, M., Giusepponi, M. E., Romano, A., Lambertucci, C., Volpini, R., et al. (2019). Regulation of adenosine A2A receptor gene expression in a model of binge eating in the amygdaloid complex of female rats. J. Psychopharmacol. 33, 1550–1561. doi: 10.1177/0269881119845798
Mishina, M., Ishiwata, K., Naganawa, M., Kimura, Y., Kitamura, S., Suzuki, M., et al. (2011). Adenosine A2A receptors measured with [11C]TMSX PET in the striata of Parkinson’s disease patients. PLoS One 6:e17338. doi: 10.1371/journal.pone.0017338
Moreira-de-Sá, A., Gonçalves, F. Q., Lopes, J. P., Silva, H. B., Tomé, A. R., Cunha, R. A., et al. (2020). Adenosine A2A receptors format long-term depression and memory strategies in a mouse model of Angelman syndrome. Neurobiol. Dis. 146:105137. doi: 10.1016/j.nbd.2020.105137
Moreira-de-Sá, A., Gonçalves, F. Q., Lopes, J. P., Silva, H. B., Tomé, A. R., Cunha, R. A., et al. (2021). Motor deficits coupled to cerebellar and striatal alterations in Ube3am–/p+ mice modelling Angelman syndrome are attenuated by adenosine A2A receptor blockade. Mol. Neurobiol. 58, 2543–2557. doi: 10.1007/s12035-020-02275-9
Morello, S., Ito, K., Yamamura, S., Lee, K. Y., Jazrawi, E., Desouza, P., et al. (2006). IL-1 beta and TNF-alpha regulation of the adenosine receptor A2A expression: differential requirement for NF-kappa B binding to the proximal promoter. J. Immunol. 177, 7173–7183. doi: 10.4049/jimmunol.177.10.7173
Ng, S. K., Higashimori, H., Tolman, M., and Yang, Y. (2015). Suppression of adenosine 2A receptor (A2AR)-mediated adenosine signaling improves disease phenotypes in a mouse model of amyotrophic lateral sclerosis. Exp. Neurol. 267, 115–122. doi: 10.1016/j.expneurol.2015.03.004
Nunes, R. A., Mazzotti, D. R., Hirotsu, C., Andersen, M. L., Tufik, S., and Bittencourt, L. (2017). The association between caffeine consumption and objective sleep variables is dependent on ADORA2A c.1083T>C genotypes. Sleep Med. 30, 210–215. doi: 10.1016/j.sleep.2016.06.038
Oliveira, S., Ardais, A. P., Bastos, C. R., Gazal, M., Jansen, K., de Mattos Souza, L., et al. (2019). Impact of genetic variations in ADORA2A gene on depression and symptoms: a cross-sectional population-based study. Purinergic Signal. 15, 37–44. doi: 10.1007/s11302-018-9635-2
Orr, A. G., Hsiao, E. C., Wang, M. M., Ho, K., Kim, D. H., Wang, X., et al. (2015). Astrocytic adenosine receptor A2A and Gs-coupled signaling regulate memory. Nat. Neurosci. 18, 423–434. doi: 10.1038/nn.3930
Orr, A. G., Lo, I., Schumacher, H., Ho, K., Gill, M., Guo, W., et al. (2018). Istradefylline reduces memory deficits in aging mice with amyloid pathology. Neurobiol. Dis. 110, 29–36. doi: 10.1016/j.nbd.2017.10.014
Orrú, M., Quiroz, C., Guitart, X., and Ferré, S. (2011). Pharmacological evidence for different populations of postsynaptic adenosine A2A receptors in the rat striatum. Neuropharmacology 61, 967–974. doi: 10.1016/j.neuropharm.2011.06.025
Padilla, K. M., Quintanar-Setephano, A., López-Vallejo, F., Berumen, L. C., Miledi, R., and Garcia-Alcocer, G. (2018). Behavioral changes induced through adenosine A2A receptor ligands in a rat depression model induced by olfactory bulbectomy. Brain Behav. 8:e00952. doi: 10.1002/brb3.952
Pagnussat, N., Almeida, A. S., Marques, D. M., Nunes, F., Chenet, G. C., Botton, P. H., et al. (2015). Adenosine A2A receptors are necessary and sufficient to trigger memory impairment in adult mice. Br. J. Pharmacol. 172, 3831–3845. doi: 10.1111/bph.13180
Patodia, S., Paradiso, B., Garcia, M., Ellis, M., Diehl, B., Thom, M., et al. (2020). Adenosine kinase and adenosine receptors A1R and A2AR in temporal lobe epilepsy and hippocampal sclerosis and association with risk factors for SUDEP. Epilepsia 61, 787–797. doi: 10.1111/epi.16487
Peterfreund, R. A., MacCollin, M., Gusella, J., and Fink, J. S. (1996). Characterization and expression of the human A2A adenosine receptor gene. J. Neurochem. 66, 362–368. doi: 10.1046/j.1471-4159.1996.66010362.x
Popat, R. A., Van Den Eeden, S. K., Tanner, C. M., Kamel, F., Umbach, D. M., Marder, K., et al. (2011). Coffee, ADORA2A, and CYP1A2: the caffeine connection in Parkinson’s disease. Eur. J. Neurol. 18, 756–765. doi: 10.1111/j.1468-1331.2011.03353.x
Rebola, N., Coelho, J. E., Costenla, A. R., Lopes, L. V., Parada, A., Oliveira, C. R., et al. (2003). Decrease of adenosine A1 receptor density and of adenosine neuromodulation in the hippocampus of kindled rats. Eur. J. Neurosci. 18, 820–828. doi: 10.1046/j.1460-9568.2003.02815.x
Rebola, N., Porciúncula, L. O., Lopes, L. V., Oliveira, C. R., Soares-da-Silva, P., and Cunha, R. A. (2005). Long-term effect of convulsive behavior on the density of adenosine A1 and A2A receptors in the rat cerebral cortex. Epilepsia 46(Suppl. 5), 159–165. doi: 10.1111/j.1528-1167.2005.01026.x
Rei, N., Rombo, D. M., Ferreira, M. F., Baqi, Y., Müller, C. E., Ribeiro, J. A., et al. (2020). Hippocampal synaptic dysfunction in the SOD1(G93A) mouse model of Amyotrophic Lateral Sclerosis: Reversal by adenosine A2AR blockade. Neuropharmacology 171:108106. doi: 10.1016/j.neuropharm.2020.108106
Rétey, J. V., Adam, M., Khatami, R., Luhmann, U. F., Jung, H. H., Berger, W., et al. (2007). A genetic variation in the adenosine A2A receptor gene (ADORA2A) contributes to individual sensitivity to caffeine effects on sleep. Clin. Pharmacol. Ther. 81, 692–698. doi: 10.1038/sj.clpt.6100102
Ribeiro, D. E., Roncalho, A. L., Glaser, T., Ulrich, H., Wegener, G., and Joca, S. (2019). P2X7 receptor signaling in stress and depression. Int. J. Mol. Sci. 20:2778. doi: 10.3390/ijms20112778
Rodrigues, R. J., Tomé, A. R., and Cunha, R. A. (2015). ATP as a multi-target danger signal in the brain. Front. Neurosci. 9:148. doi: 10.3389/fnins.2015.00148
Rogers, P. J., Hohoff, C., Heatherley, S. V., Mullings, E. L., Maxfield, P. J., Evershed, R. P., et al. (2010). Association of the anxiogenic and alerting effects of caffeine with ADORA2A and ADORA1 polymorphisms and habitual level of caffeine consumption. Neuropsychopharmacology 35, 1973–1983. doi: 10.1038/npp.2010.71
Schoen, S. W., and Kreutzberg, G. W. (1997). 5′-nucleotidase enzyme cytochemistry as a tool for revealing activated glial cells and malleable synapses in CNS development and regeneration. Brain Res. Brain Res. Protoc. 1, 33–43. doi: 10.1016/s1385-299x(96)00006-2
Schoen, S. W., Ebert, U., and Löscher, W. (1999). 5′-Nucleotidase activity of mossy fibers in the dentate gyrus of normal and epileptic rats. Neuroscience 93, 519–526. doi: 10.1016/s0306-4522(99)00135-9
Schwarzschild, M. A., Agnati, L., Fuxe, K., Chen, J. F., and Morelli, M. (2006). Targeting adenosine A2A receptors in Parkinson’s disease. Trends Neurosci. 29, 647–654. doi: 10.1016/j.tins.2006.09.004
Seven, Y. B., Simon, A. K., Sajjadi, E., Zwick, A., Satriotomo, I., and Mitchell, G. S. (2020). Adenosine A2A receptor inhibition protects phrenic motor neurons from cell death induced by protein synthesis inhibition. Exp. Neurol. 323:113067. doi: 10.1016/j.expneurol.2019.113067
Shen, H. Y., Canas, P. M., Garcia-Sanz, P., Lan, J. Q., Boison, D., Moratalla, R., et al. (2013). Adenosine A2A receptors in striatal glutamatergic terminals and GABAergic neurons oppositely modulate psychostimulant action and DARPP-32 phosphorylation. PLoS One 8:e80902. doi: 10.1371/journal.pone.0080902
Shen, H. Y., Coelho, J. E., Ohtsuka, N., Canas, P. M., Day, Y. J., Huang, Q. Y., et al. (2008). A critical role of the adenosine A2A receptor in extrastriatal neurons in modulating psychomotor activity as revealed by opposite phenotypes of striatum and forebrain A2A receptor knock-outs. J. Neurosci. 28, 2970–2975. doi: 10.1523/JNEUROSCI.5255-07.2008
Shinohara, M., Saitoh, M., Nishizawa, D., Ikeda, K., Hirose, S., Takanashi, J., et al. (2013). ADORA2A polymorphism predisposes children to encephalopathy with febrile status epilepticus. Neurology 80, 1571–1576. doi: 10.1212/WNL.0b013e31828f18d8
Silva, A. C., Lemos, C., Gonçalves, F. Q., Pliássova, A. V., Machado, N. J., Silva, H. B., et al. (2018). Blockade of adenosine A2A receptors recovers early deficits of memory and plasticity in the triple transgenic mouse model of Alzheimer’s disease. Neurobiol. Dis. 117, 72–81. doi: 10.1016/j.nbd.2018.05.024
Simões, A. P., Machado, N. J., Gonçalves, N., Kaster, M. P., Simões, A. T., Nunes, A., et al. (2016). Adenosine A2A receptors in the amygdala control synaptic plasticity and contextual fear memory. Neuropsychopharmacology 41, 2862–2871. doi: 10.1038/npp.2016.98
Smith, M. D., Bhatt, D. P., Geiger, J. D., and Rosenberger, T. A. (2014). Acetate supplementation modulates brain adenosine metabolizing enzymes and adenosine A2A receptor levels in rats subjected to neuroinflammation. J. Neuroinflammation 11:99. doi: 10.1186/1742-2094-11-99
Sun, M. J., Liu, F., Zhao, Y. F., and Wu, X. A. (2020). In vivo positron emission tomography imaging of adenosine A2A receptors. Front. Pharmacol. 11:599857. doi: 10.3389/fphar.2020.599857
Svenningsson, P., Le Moine, C., Fisone, G., and Fredholm, B. B. (1999). Distribution, biochemistry and function of striatal adenosine A2A receptors. Prog. Neurobiol. 59, 355–396. doi: 10.1016/s0301-0082(99)00011-8
Taherzadeh-Fard, E., Saft, C., Wieczorek, S., Epplen, J. T., and Arning, L. (2010). Age at onset in Huntington’s disease: replication study on the associations of ADORA2A, HAP1 and OGG1. Neurogenetics 11, 435–439. doi: 10.1007/s10048-010-0248-3
Temido-Ferreira, M., Ferreira, D. G., Batalha, V. L., Marques-Morgado, I., Coelho, J. E., Pereira, P., et al. (2020). Age-related shift in LTD is dependent on neuronal adenosine A2A receptors interplay with mGluR5 and NMDA receptors. Mol. Psychiatry 25, 1876–1900. doi: 10.1038/s41380-018-0110-9
Tescarollo, F. C., Rombo, D. M., DeLiberto, L. K., Fedele, D. E., Alharfoush, E., Tomé, A. R., et al. (2020). Role of adenosine in epilepsy and seizures. J. Caffeine Adenosine Res. 10, 45–60. doi: 10.1089/caff.2019.0022
Tian, T., Zhou, Y., Feng, X., Ye, S., Wang, H., Wu, W., et al. (2016). MicroRNA-16 is putatively involved in the NF-kappaB pathway regulation in ulcerative colitis through adenosine A2A receptor (A2AR) mRNA targeting. Sci. Rep. 6:30824. doi: 10.1038/srep30824
Viana da Silva, S., Haberl, M. G., Zhang, P., Bethge, P., Lemos, C., Gonçalves, N., et al. (2016). Early synaptic deficits in the APP/PS1 mouse model of Alzheimer’s disease involve neuronal adenosine A2A receptors. Nat. Commun. 7:11915. doi: 10.1038/ncomms11915
Villar-Menéndez, I., Porta, S., Buira, S. P., Pereira-Veiga, T., Díaz-Sánchez, S., Albasanz, J. L., et al. (2014). Increased striatal adenosine A2A receptor levels is an early event in Parkinson’s disease-related pathology and it is potentially regulated by miR-34b. Neurobiol. Dis. 69, 206–214.
Vincenzi, F., Corciulo, C., Targa, M., Casetta, I., Gentile, M., Granieri, E., et al. (2013). A2A adenosine receptors are up-regulated in lymphocytes from amyotrophic lateral sclerosis patients. Amyotroph. Lateral Scler. Frontotemporal Degener. 14, 406–413. doi: 10.3109/21678421.2013.793358
Wei, C. J., Augusto, E., Gomes, C. A., Singer, P., Wang, Y., Boison, D., et al. (2014). Regulation of fear responses by striatal and extrastriatal adenosine A2A receptors in forebrain. Biol. Psychiatry 75, 855–863. doi: 10.1016/j.biopsych.2013.05.003
Wieraszko, A., and Seyfried, T. N. (1989). Increased amount of extracellular ATP in stimulated hippocampal slices of seizure prone mice. Neurosci. Lett. 106, 287–293. doi: 10.1016/0304-3940(89)90178-x
Yu, L., Frith, M. C., Suzuki, Y., Peterfreund, R. A., Gearan, T., Sugano, S., et al. (2004). Characterization of genomic organization of the adenosine A2A receptor gene by molecular and bioinformatics analyses. Brain Res. 1000, 156–173. doi: 10.1016/j.brainres.2003.11.072
Yu, L., Shen, H. Y., Coelho, J. E., Araújo, I. M., Huang, Q. Y., Day, Y. J., et al. (2008). Adenosine A2A receptor antagonists exert motor and neuroprotective effects by distinct cellular mechanisms. Ann. Neurol. 63, 338–346. doi: 10.1002/ana.21313
Zhao, L., Liu, Y. W., Yang, T., Gan, L., Yang, N., Dai, S. S., et al. (2015). The mutual regulation between miR-214 and A2AR signaling plays an important role in inflammatory response. Cell Signal. 27, 2026–2034. doi: 10.1016/j.cellsig.2015.07.007
Zhao, Z. A., Li, P., Ye, S. Y., Ning, Y. L., Wang, H., Peng, Y., et al. (2017). Perivascular AQP4 dysregulation in the hippocampal CA1 area after traumatic brain injury is alleviated by adenosine A2A receptor inactivation. Sci. Rep. 7:2254. doi: 10.1038/s41598-017-02505-6
Keywords: adenosine A2A receptors, central nervous system, antagonism, caffeine, biomarkers, polymorphisms
Citation: Moreira-de-Sá A, Lourenço VS, Canas PM and Cunha RA (2021) Adenosine A2A Receptors as Biomarkers of Brain Diseases. Front. Neurosci. 15:702581. doi: 10.3389/fnins.2021.702581
Received: 29 April 2021; Accepted: 22 June 2021;
Published: 16 July 2021.
Edited by:
Rui Daniel Prediger, Federal University of Santa Catarina, BrazilReviewed by:
Thomas Heinbockel, Howard University, United StatesErnest Jennings, James Cook University, Australia
Copyright © 2021 Moreira-de-Sá, Lourenço, Canas and Cunha. This is an open-access article distributed under the terms of the Creative Commons Attribution License (CC BY). The use, distribution or reproduction in other forums is permitted, provided the original author(s) and the copyright owner(s) are credited and that the original publication in this journal is cited, in accordance with accepted academic practice. No use, distribution or reproduction is permitted which does not comply with these terms.
*Correspondence: Rodrigo A. Cunha, cmN1bmhhQGZtZWQudWMucHQ=; Y3VuaGFyb2RAZ21haWwuY29t
†These authors have contributed equally to this work and share first authorship