- 1School of Rehabilitation Medicine, Shandong Second Medical University, Weifang, Shandong, China
- 2School of Rehabilitation Science and Engineering, University of Health and Rehabilitation Sciences, Qingdao, Shandong, China
- 3Department of Neurological Rehabilitation, Beijing Rehabilitation Hospital, Capital Medical University, Beijing, China
Introduction: MScanFit is a model-based algorithm for motor unit number estimation (MUNE) from compound muscle action potential (CMAP) scan data. It is a clinically applicable tool because of its quick and automatic implementation. Electrodes with different recording areas were employed to record CMAP scan data in existing studies. However, the effect of electrode recording area on MScanFit MUNE and other CMAP scan parameters has not been studied.
Methods: CMAP scan was performed on the abductor pollicis brevis muscle of both hands on 14 healthy subjects using three different electrodes with recording areas of 10 mm × 10 mm, 11 mm × 14 mm, and 22 mm × 26 mm, respectively. Motor unit number was estimated using MScanFit for each CMAP scan. Two motor unit number index parameters, i.e., D50 and step index (STEPIX), were also derived from the CMAP scan data.
Results: No significant difference in D50, STEPIX, and MScanFit MUNE was observed across three different electrode recording areas, although the amplitude of CMAP decreased significantly when a larger electrode was used. Intraclass correlation coefficients of 0.792 and 0.782 were obtained for MScanFit MUNE and STEPIX, respectively.
Discussion: Compared with CMAP amplitude, D50, STEPIX, and MScanFit MUNE are less sensitive to variation in electrode recording area. However, the repeatability of MScanFit MUNE could be compromised by the inconsistency in the electrode recording area.
1 Introduction
The reduction of motor units may lead to muscle weakness and muscle atrophy in neuromuscular diseases. Motor unit number estimation (MUNE) is a powerful tool for tracking loss of motor units and the compensatory phenomenon of collateral reinnervation (Gooch et al., 2014). A variety of MUNE methods have been proposed in past decades, but those traditional MUNE methods such as incremental stimulation MUNE and multiple point stimulation MUNE (de Carvalho et al., 2018; Wright et al., 2021) are likely biased to the sampling of motor units. By contrast, compound muscle action potential (CMAP) scan aims to gradually activate/deactivate all motor units by applying hundreds of transcutaneous stimuli to the motor nerve across a wide range of intensities (Blok et al., 2007). Compared with traditional MUNE methods, CMAP scan is less biased to the sampling of motor units, and various methods have been developed to process CMAP scan for examination of neuromuscular disorders (Sleutjes et al., 2014; Nandedkar et al., 2022; Chen et al., 2023a,b; Lu et al., 2023b). Of particular note, Bostock proposed a model-based MUNE algorithm named MScanFit, which estimates the number of motor units by fitting the detailed stimulus–response curve recorded from a CMAP scan (Bostock, 2016). MScanFit possesses the advantage of automated and quick (typically taking only a few minutes) implementation, making it so far the most often used CMAP scan processing method in basic and clinical electrophysiological studies (Kristensen et al., 2019; Zong et al., 2021; Schneider et al., 2023).
CMAP scan curve can be affected by experimental parameters including the number and the width of electrical stimuli. For example, our previous studies show that CMAP scan curve becomes denser when the number of stimuli is increased, which leads to an increase in derived step index (STEPIX; Lu et al., 2023a). Although MScanFit is not sensitive to the number of stimuli, it is significantly affected by the width of stimuli (Zong et al., 2020).
Electrode recording area (the area of the recording surface of an electrode) is another major factor affecting CMAP, especially on its shape and amplitude due to the different filtering effects (Wee and Ashley, 1990; Chang et al., 1993; Jonas et al., 1999; Barkhaus et al., 2006). The electrode recording area used for CMAP scan studies covered a wide range in literature from greater than 400 mm2 (Sørensen et al., 2022, 2023) to around 100 mm2 (Li et al., 2018; Song et al., 2023). Furthermore, inconsistent electrode recording areas were reported when examining the same muscle (Sleutjes et al., 2021). For example, when performing CMAP scan recordings on the abductor pollicis brevis (APB) muscle, square electrodes with the size of 30 mm × 22 mm (with the recording area of 474 mm2) and 30 mm × 24 mm (detailed recording area was not reported) were used in Araújo et al. (2015) and Sørensen et al. (2023), respectively, while disk electrodes with the diameter of 10 mm (i.e., 79 mm2) and 13 mm (i.e., 133 mm2) were applied in Stikvoort García et al. (2022) and Song et al. (2023), respectively. Note that 2-dimensional electrode arrays up to 128 channels have also been used for MUNE, where electrodes with diameters smaller than 2 mm are commonly used (Sleutjes et al., 2016).
The effect of electrode recording area on CMAP implies that the CMAP scan curves could also vary with different surface electrodes. However, it still remains unclear how the different surface electrode recording areas may affect CMAP scan processing parameters, such as MScanFit MUNE. The objective of this study was, therefore, to assess the effect of electrode recording area on MScanFit MUNE and other CMAP scan parameters. In addition, the repeatability of these parameters using different electrode recording areas was quantified.
2 Methods
2.1 Experimental protocol
Three electrodes with different recording areas were used for CMAP scan recording in this study. Their recording areas are 10 mm × 10 mm (denoted as E1), 11 mm × 14 mm (denoted as E2), and 22 mm × 26 mm (denoted as E3), respectively.
Fourteen right-handed healthy subjects (7 males and 7 females, aged 30.6 ± 9.5 years) participated in this study. Each subject’s bilateral APB muscles were recorded. The order of the left and right hand was randomized. In the experiment, each subject was seated comfortably in a chair with his/her testing hand rested on a table and restrained in the pronation position. CMAP scan was performed three times on each hand using one of the three active electrodes in a random order, while all the other experimental parameters remained the same. Before each recording, the subject was given sufficient rest to avoid mental and muscle fatigue.
2.2 CMAP scan recording
Before each recording, the range of stimulating current intensity was determined by performing an automatic search. The range of current intensity was then manually tuned in order to cover the entire motor unit recruitment range. The pulse duration was set to 0.1 ms, the number of stimuli was set to 500, and the frequency of stimuli was set to 2 Hz. All the data were collected using Nicolet EDX system (Natus Neurology Incorporated, Middleton, WI, United States).
As shown in Figure 1, the active electrode was placed on the abdominal eminence of the APB muscle, and the reference electrode was placed on the metacarpophalangeal joint of the thumb. The ground electrode was placed on the bony protuberance on the back of the hand between the active electrode and the reference electrode. The stimulating electrode (Ag/AgCl electrode) with two contact surfaces spaced 20 mm apart and each having a diameter of 9 mm, was placed 1–2 cm proximal to the wrist to activate the median nerve. The electrode was coated with conductive paste and the cathode was oriented distally. Both recording and stimulating electrodes were carefully tuned in order to optimize electrode positions where the largest CMAP amplitude can be evoked with a relatively low stimulating current intensity. Once the stimulating site was determined, the electrode was fixed with surgical tape or self-adherent wrap. Alcohol pads were used to clean the thumb, thenar, wrist, and back of the hand before the electrodes were attached.
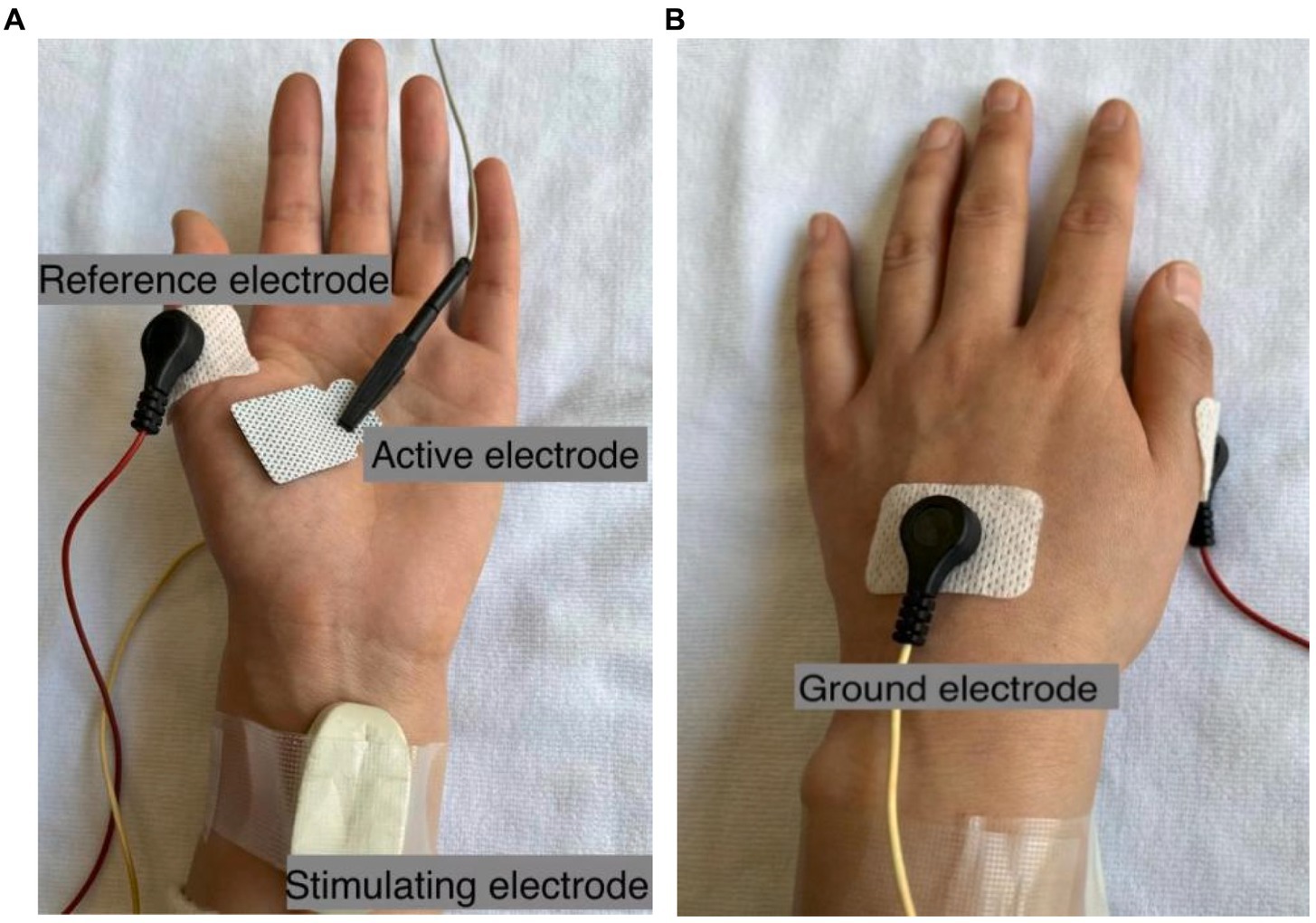
Figure 1. Electrode configurations for CMAP scan recording of the APB muscle. The active electrode E3 with the size of 22 mm × 26 mm is shown as an example in (A), and the ground electrode is shown in (B).
2.3 CMAP scan data analysis
The MScanFit program (free version 2016; Bostock, 2016) was applied to estimate motor unit number. Each recording was analyzed multiple times until three valid estimations were obtained (i.e., percentage error < 7%), and the one with the smallest error was accepted. The pre-scan and post-scan limits were manually selected each time, while all the other settings remained at their default values.
D50, step index, S0, S100, and the difference between S0 and S100 (i.e., S100 − S0) were also derived from each scan using a customized Matlab script. D50 is the number of largest consecutive differences from each scan that are required to build up 50% of the maximum CMAP amplitude (Sleutjes et al., 2014). STEPIX, which is a recently proposed index based on the logarithmic relation between the step amplitude and step number in a CMAP scan, reflects the number of motor units (Nandedkar et al., 2022). S0 is the maximum electrical intensity that cannot activate any motor unit. S100 is the minimum electrical intensity that can activate all the motor units.
2.4 Statistical analysis
One-way repeated measures analysis of variance was performed to examine the differences in the maximum CMAP amplitude, S0, S100, S100 − S0, D50, STEPIX, and MScanFit MUNE parameters for different electrode recording areas. Statistical significance was set as p < 0.05. Repeatability was quantified using consistency intraclass correlation coefficient (ICC) if significant difference was observed; otherwise, absolute agreement ICC was used. Results are presented as mean ± standard error.
3 Results
Mild or tolerable pain was reported by the subjects in our experiment. The CMAP amplitude of one subject’s left hand was lower than 50% of the amplitude of his right hand, and thus CMAP scan data from his left hand was excluded. As a result, a total of 81 CMAP scan curves (3 trials per hand × 27 hands) recorded from 14 subjects were analyzed.
The maximum CMAP amplitude of the APB muscle was 10.18 ± 0.58 mV for electrode E1, 9.65 ± 0.56 mV for electrode E2, and 8.54 ± 0.50 mV for electrode E3 (as shown in Table 1). Significant difference was observed across the three electrodes (p < 0.0001), and the maximum CMAP amplitude decreased at a larger electrode recording area. No significant difference was observed across the three electrode recording areas in S0 (p = 0.592), S100 (p = 0.482), S100 − S0 (p = 0.536), D50 (p = 0.463), STEPIX (p = 0.654), or MScanFit MUNE (p = 0.155). The ICC of MScanFit MUNE (0.792) was greater than that of the other two indexes (0.782 for STEPIX and 0.686 for D50). The median value of difference in D50, STEPIX, and MScanFit MUNE between individual subject’s CMAP scan curves recorded using electrodes with different recording areas was distributed close to 0 (i.e., no significant difference across three electrodes). However, it was observed that the difference in these parameters between two electrodes could be distributed in a large range, as shown in Figure 2.
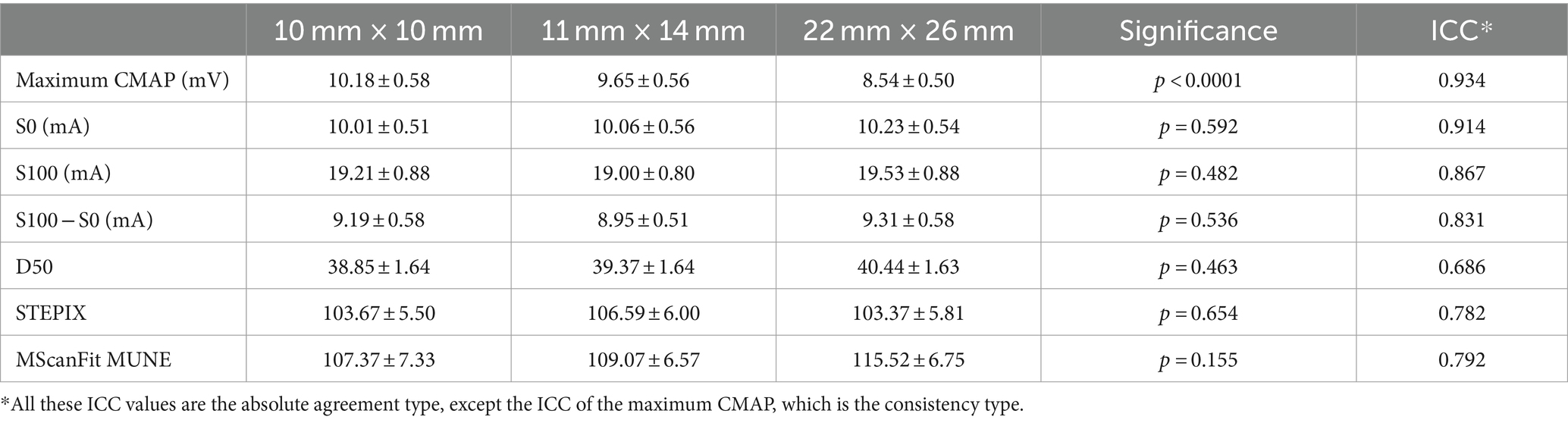
Table 1. Parameters derived from CMAP scan of the APB muscle on healthy subjects using electrodes with different recording areas.
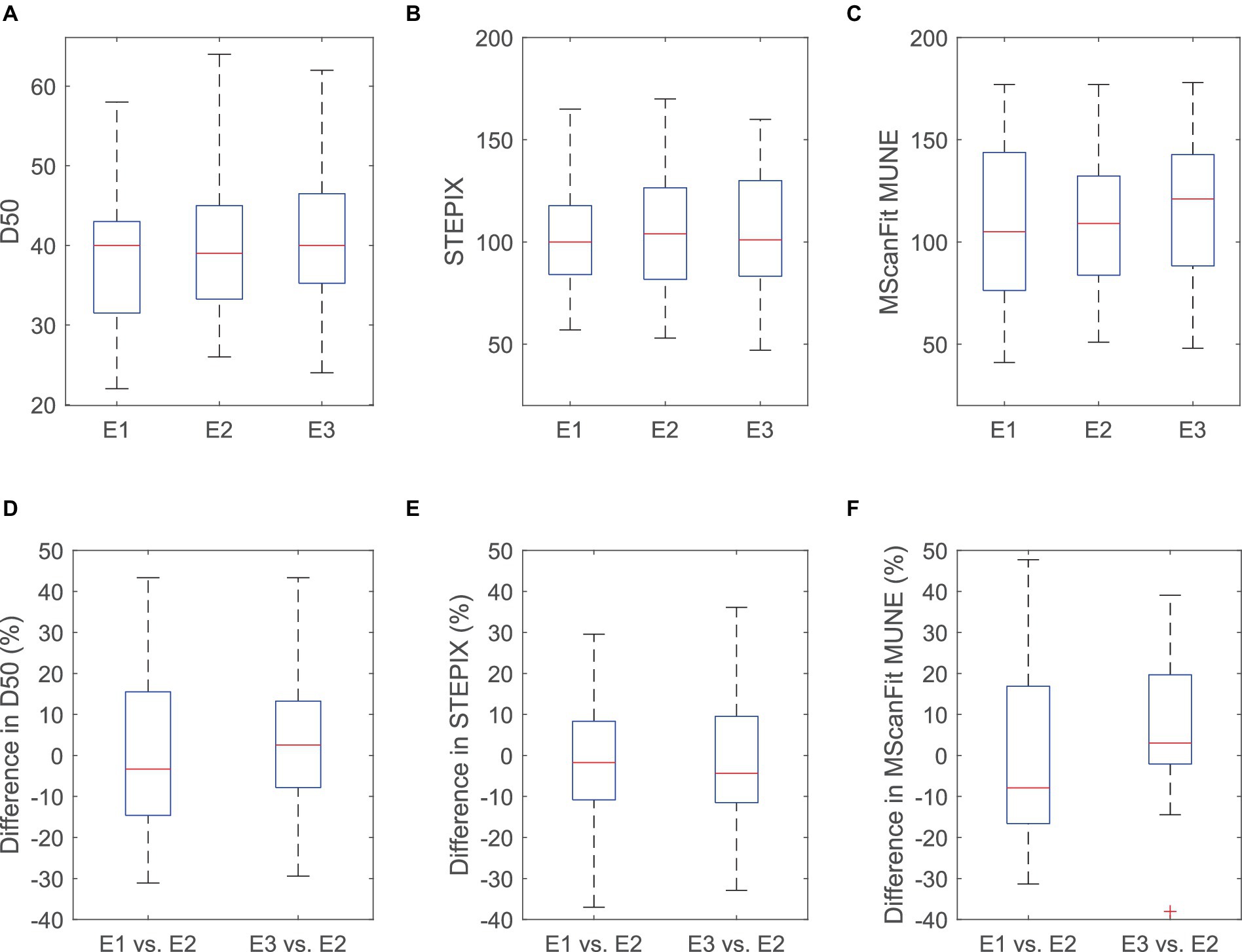
Figure 2. D50 (A), STEPIX (B), MScanFit MUNE (C), and the difference in D50 (D), STEPIX (E), and MScanFit MUNE (F) between CMAP scan curves of the APB muscle of healthy subjects recorded using electrodes with different recording areas. The difference was calculated as (Parameter derived from E1 or E3−Parameter derived from E2)/Parameter derived from E2 for each subject. One outlier (above 50% in both STEPIX and MScanFit) is not shown in this figure.
Three CMAP scan curves recorded using electrode E1, E2, and E3 from the APB muscle of a representative subject are demonstrated in Figure 3. It is worth noting that the curves recorded using electrode E2 and E3 demonstrate a close pattern, and there is only a difference of 2 (or 1.7%) in MScanFit MUNE although the difference in maximum CMAP amplitude is as high as 1.13 mV (or 9.5%).
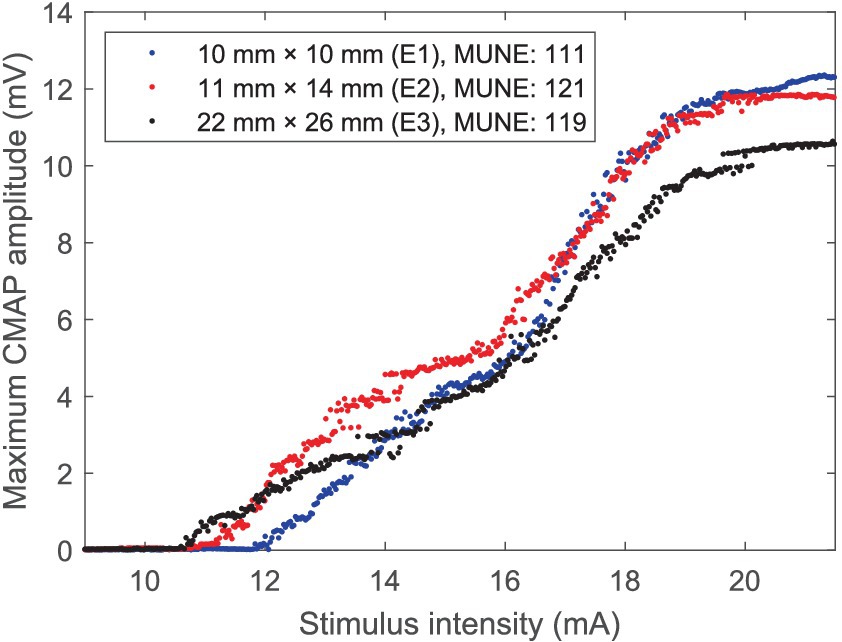
Figure 3. CMAP scan curves of the APB muscle of a representative healthy subject recorded using three electrodes with different recording areas.
4 Discussion
This study presents a novel analysis of CMAP scan parameters with respect to the different electrode recording areas. By testing three different electrodes on CMAP scan recordings of the APB muscle we observed that changes in the electrode recording area had no significant impact on the examined CMAP processing parameters including D50, STEPIX and MScanFit MUNE. In recent years, different electrodes or recording areas have been used in CMAP scan experiments on various muscles. For example, a disk electrode with 11 mm in diameter was used to examine the abductor hallucis muscle (Li et al., 2018); a smaller disk electrode with 10 mm in diameter was used to collect CMAP scan data from the first dorsal interosseous (FDI) muscle (Zong et al., 2020). Additionally, some researchers used 13 mm diameter disk electrodes to examine the FDI and abductor digiti minimi (ADM) muscles (Song et al., 2023). For the APB muscle examined in this study, literature shows that the surface electrode used for CMAP scan recording ranged from 10 mm to 15 mm in diameter (i.e., 79 mm2 to 177 mm2; Farschtschi et al., 2017; Stikvoort García et al., 2022; Zong et al., 2022c; Song et al., 2023). Square electrodes with different recording areas were also reported for APB muscle CMAP scan recordings (Araújo et al., 2015; Sørensen et al., 2023). Considering various recording areas reported in previous CMAP scan studies, we chose to use three electrodes with recording areas of 100 mm2, 154 mm2, and 572 mm2, respectively. The three electrode recording areas used in this study can cover the commonly used surface electrodes in research and clinical practice.
In addition to motor unit number parameters (e.g., MScanFit MUNE), it was also observed that the three different electrode recording areas had no significant impact on CMAP scan’s stimulus intensity parameters including S0, S100, and S100 − S0. This is not surprising since the stimulus intensities that elicit motor unit responses are independent of the electrode size. Our results indicate that both large and small electrode recording areas have a similar sensitivity in capturing the recruited motor unit activity of the examined muscle.
The only observed significant difference across three different electrode recording areas was CMAP amplitude. Our results indicated that increased electrode recording area significantly reduced CMAP amplitude of the APB muscle. This is consistent to previous experimental (Wee and Ashley, 1990; Barkhaus et al., 2006) and theoretical findings (Fuglevand et al., 1992). The same tendency was also reported by Chang et al. (1993) although the significance level was not reached. Large surface electrodes can capture relatively more muscle volume but impose an increased low pass filtering effect on the recorded signal compared with small ones. These two factors have opposite effects on the CMAP amplitude. For the examined APB muscle in this study, it seems the difference in low pass filtering effect caused by different surface electrodes was more dominant than the difference in captured muscle volume, thus the CMAP amplitude was reduced with increased recording surface area. Nonetheless, the alterations in CMAP amplitude did not have a significant influence on its processing parameters, such as MScanFit MUNE. This is likely because MScanFit MUNE applies a number of operations to refine the CMAP scan model to meet the predefined error score, including adjusting individual motor unit parameters, splitting or merging motor units, etc. This is different from conventional MUNE methods, calculated as the ratio of the CMAP measurement to the mean motor unit action potential measurement estimated from a small sample of motor units.
The repeatability of CMAP scan parameters was also examined in this study across three different surface electrodes. The repeatability of MScanFit MUNE across the three different electrodes was observed to be slightly higher than that of the two index parameters (i.e., D50 and STEPIX). The repeatability of MScanFit MUNE can be exemplified by Figure 3, as it indicates a relative large variation in CMAP scan amplitude (induced from different electrodes) does not necessarily impose a similar extent of variation in MScanFit MUNE. Existing studies reported that the test–retest repeatability of MScanFit MUNE was excellent using the same electrode for CMAP scan recordings. For example, the ICC of test–retest repeatability achieved 0.93, 0.90, and 0.96 in our previous studies on the abductor hallucis (Li et al., 2018), the anconeus (Zong et al., 2022b), and the second lumbrical (Zong et al., 2022a) muscles, respectively. ICCs greater than 0.8 were also reported for MScanFit MUNE in three repeated tests on the APB, FDI, and ADM muscles (Higashihara et al., 2020). In this study, as expected, although MScanFit MUNE values were not significantly different across three different surface electrode recording areas, the repeatability of MScanFit MUNE was not as high as previously reported numbers, due to inconsistency in surface electrode recording area.
As an important neuromuscular electrophysiological method, MUNE is often used to compare the difference between two groups (for example, between healthy control subjects and subjects with neuromuscular diseases) in a cross-sectional study or track the same muscle in a longitudinal study. Although group analysis revealed no significant difference in MScanFit MUNE of the APB muscle across three different surface electrodes, variation up to ±50% in individual subject was observed between two different electrode recording areas. Such a variation may reduce the reliability of tracking motor unit loss. Therefore, we advocate the same experimental settings (including the same recording electrode) should be used in MUNE studies for both research and clinical settings. This can help to avoid confounding factors for comparing MUNE and other CMAP scan parameters in different situations.
The current study is limited by only examining the APB muscle of neurologically intact subjects. It remains to be determined whether the findings can be generalized to other muscles, particularly to those large muscles. In addition, it is important in the future work to investigate how different electrode recording areas may affect the sensitivity of MScanFit and other CMAP scan processing parameters in quantifying motor unit number and size changes in clinical application.
5 Conclusion
The effect of electrode recording area on MScanFit MUNE and other parameters derived from a CMAP scan was assessed by testing three different electrode recording areas. The experimental results from APB muscles indicate that although CMAP amplitude was sensitive to surface electrode recording area, CMAP scan processing parameters including D50, STEPIX, and MScanFit MUNE were not significantly affected by the changes in electrode recording area. However, inconsistency in electrode recording area may compromise the repeatability of CMAP scan processing. The findings of the study can help to understand the effect of experimental factors on different CMAP scan parameters, thus facilitating their analysis and interpretation.
Data availability statement
The raw data supporting the conclusions of this article will be made available by the authors, without undue reservation.
Ethics statement
The studies involving humans were approved by the Ethics Committee of the University of Health and Rehabilitation Sciences. The studies were conducted in accordance with the local legislation and institutional requirements. Written informed consent for participation in this study was provided by the participants.
Author contributions
DZ: Data curation, Formal analysis, Investigation, Software, Visualization, Writing – original draft. ZL: Conceptualization, Funding acquisition, Investigation, Methodology, Project administration, Resources, Software, Validation, Writing – original draft. WG: Methodology, Project administration, Validation, Resources, Supervision, Writing – review & editing. PZ: Conceptualization, Funding acquisition, Methodology, Resources, Supervision, Validation, Writing – review & editing.
Funding
The author(s) declare that financial support was received for the research, authorship, and/or publication of this article. This research was funded by the National Natural Science Foundation of China (grant no. 82102179), the Shandong Provincial Natural Science Foundation (grant no. ZR2021QH267), and the Taishan Scholar of Shandong Province (tsqn202211226 and tstp20221144).
Conflict of interest
The authors declare that the research was conducted in the absence of any commercial or financial relationships that could be construed as a potential conflict of interest.
The author(s) declared that they were an editorial board member of Frontiers, at the time of submission. This had no impact on the peer review process and the final decision.
Publisher’s note
All claims expressed in this article are solely those of the authors and do not necessarily represent those of their affiliated organizations, or those of the publisher, the editors and the reviewers. Any product that may be evaluated in this article, or claim that may be made by its manufacturer, is not guaranteed or endorsed by the publisher.
References
Araújo, T., Candeias, R., Nunes, N., and Gamboa, H. (2015). Evaluation of motor neuron excitability by CMAP scanning with electric modulated current. Neurosci J 2015, 1–5. doi: 10.1155/2015/360648
Barkhaus, P. E., Periquet, M. I., and Nandedkar, S. D. (2006). Influence of the surface EMG electrode on the compound muscle action potential. Electromyogr. Clin. Neurophysiol. 46, 235–239.
Blok, J. H., Ruitenberg, A., Maathuis, E. M., and Visser, G. H. (2007). The electrophysiological muscle scan. Muscle Nerve 36, 436–446. doi: 10.1002/mus.20838
Bostock, H. (2016). Estimating motor unit numbers from a CMAP scan: CMAP scan MUNE. Muscle Nerve 53, 889–896. doi: 10.1002/mus.24945
Chang, H., Chan, R. C., and Hsu, T. C. (1993). The effect of surface area of active recording electrode to CMAP parameters. Zhonghua Yi Xue Za Zhi 51, 419–424.
Chen, M., Lu, Z., Li, X., Zong, Y., Xie, Q., Li, S., et al. (2023a). Compound muscle action potential (CMAP) scan examination of paretic and contralateral muscles reveals motor unit alterations after stroke. Sci. China Life Sci. 66, 2604–2613. doi: 10.1007/s11427-022-2308-8
Chen, M., Lu, Z., Zong, Y., Li, X., and Zhou, P. (2023b). A novel analysis of compound muscle action potential scan: staircase function fitting and StairFit motor unit number estimation. IEEE J. Biomed. Health Inform. 27, 1579–1587. doi: 10.1109/JBHI.2022.3229211
de Carvalho, M., Barkhaus, P. E., Nandedkar, S. D., and Swash, M. (2018). Motor unit number estimation (MUNE): where are we now? Clin. Neurophysiol. 129, 1507–1516. doi: 10.1016/J.CLINPH.2018.04.748
Farschtschi, S., Gelderblom, M., Buschbaum, S., Bostock, H., Grafe, P., and Mautner, V. F. (2017). Muscle action potential scans and ultrasound imaging in neurofibromatosis type 2: CMAP scans and nerve imaging in NF2. Muscle Nerve 55, 350–358. doi: 10.1002/mus.25256
Fuglevand, A. J., Winter, D. A., Patla, A. E., and Stashuk, D. (1992). Detection of motor unit action potentials with surface electrodes: influence of electrode size and spacing. Biol. Cybern. 67, 143–153. doi: 10.1007/BF00201021
Gooch, C. L., Doherty, T. J., Chan, K. M., Bromberg, M. B., Lewis, R. A., Stashuk, D. W., et al. (2014). Motor unit number estimation: a technology and literature review. Muscle Nerve 50, 884–893. doi: 10.1002/mus.24442
Higashihara, M., Menon, P., Bos, M., Pavey, N., and Vucic, S. (2020). Reproducibility of motor unit number index and MScanFit motor unit number estimation across intrinsic hand muscles. Muscle Nerve 62, 192–200. doi: 10.1002/mus.26839
Jonas, D., Bischoff, C., and Conrad, B. (1999). Infuence of different types of surface electrodes on amplitude, area and duration of the compound muscle action potential. Clin. Neurophysiol. 110, 2171–2175. doi: 10.1016/S1388-2457(99)00116-9
Kristensen, R. S., Bostock, H., Tan, S. V., Witt, A., Fuglsang-Frederiksen, A., Qerama, E., et al. (2019). MScanFit motor unit number estimation (MScan) and muscle velocity recovery cycle recordings in amyotrophic lateral sclerosis patients. Clin. Neurophysiol. 130, 1280–1288. doi: 10.1016/j.clinph.2019.04.713
Li, X., Zong, Y., Klein, C. S., and Zhou, P. (2018). Motor unit number estimation of human abductor Hallucis from a CMAP scan. Muscle Nerve 58, 735–737. doi: 10.1002/mus.26295
Lu, Z., Chen, M., Zong, Y., Huang, C., Li, X., and Zhou, P. (2023a). Sensitivity analysis of CMAP scan step index to different stimulation parameters and examination of muscles affected by spinal cord injury. IEEE Trans. Biomed. Eng. 70, 2834–2840. doi: 10.1109/TBME.2023.3266327
Lu, Z., Chen, M., Zong, Y., Li, X., and Zhou, P. (2023b). A novel analysis of CMAP scans from perspective of information theory: CMAP distribution index (CDIX). I.E.E.E. Trans. Biomed. Eng. 70, 1182–1188. doi: 10.1109/TBME.2022.3212312
Nandedkar, S. D., Barkhaus, P. E., and Stålberg, E. V. (2022). Analysis of the compound muscle action potential scan: step index (STEPIX) and amplitude index (AMPIX). Clin. Neurophysiol. 139, 119–127. doi: 10.1016/j.clinph.2022.04.011
Schneider, C., Svačina, M. K. R., Kohle, F., Sprenger-Svačina, A., Fink, G. R., and Lehmann, H. C. (2023). Motor unit number estimation by MScanFit in myotonic dystrophies. J. Neurol. Sci. 451:120728. doi: 10.1016/j.jns.2023.120728
Sleutjes, B. T. H. M., Jacobsen, A. B., Tankisi, H., Sirin, N. G., Oge, A. E., Henderson, R. D., et al. (2021). Advancing disease monitoring of amyotrophic lateral sclerosis with the compound muscle action potential scan. Clin. Neurophysiol. 132, 3152–3159. doi: 10.1016/j.clinph.2021.09.014
Sleutjes, B. T. H. M., Maathuis, E. M., van Doorn, P. A., Blok, J. H., and Visser, G. H. (2016). Electrically evoked multiplet discharges are associated with more marked clinical deterioration in motor neuron disease. Muscle Nerve 53, 222–226. doi: 10.1002/mus.24700
Sleutjes, B. T. H. M., Montfoort, I., Maathuis, E. M., Drenthen, J., Van Doorn, P. A., Visser, G. H., et al. (2014). CMAP scan discontinuities: automated detection and relation to motor unit loss. Clin. Neurophysiol. 125, 388–395. doi: 10.1016/j.clinph.2013.07.016
Song, X., Cui, L., Zong, Y., Chen, M., Lu, Z., Xie, Q., et al. (2023). A single center report of MScanFit motor unit number estimation in five muscles of healthy subjects. Front. Hum. Neurosci. 16:1078848. doi: 10.3389/fnhum.2022.1078848
Sørensen, D. M., Bostock, H., Abrahao, A., Alaamel, A., Alaydin, H. C., Ballegaard, M., et al. (2023). Estimating motor unit numbers from a CMAP scan: repeatability study on three muscles at 15 centres. Clin. Neurophysiol. 151, 92–99. doi: 10.1016/j.clinph.2023.04.008
Sørensen, D. M., Bostock, H., Ballegaard, M., Fuglsang-Frederiksen, A., Graffe, C. C., Grötting, A., et al. (2022). Assessing inter-rater reproducibility in MScanFit MUNE in a 6-subject, 12-rater “round Robin” setup. Neurophysiol. Clin. 52, 157–169. doi: 10.1016/j.neucli.2021.11.002
Stikvoort García, D. J. L., Kovalchuk, M. O., Goedee, H. S., van Schelven, L. J., van den Berg, L. H., Franssen, H., et al. (2022). Motor unit integrity in multifocal motor neuropathy: a systematic evaluation with CMAP scans. Muscle Nerve 65, 317–325. doi: 10.1002/mus.27469
Wee, A. S., and Ashley, R. A. (1990). Relationship between the size of the recording electrodes and morphology of the compound muscle action potentials. Electromyogr. Clin. Neurophysiol. 30, 165–168.
Wright, R. D., Sivak, A., Abrahão, A., and Jones, K. E. (2021). Fifty years of motor unit number estimation. Can. J. Neurol. Sci. 50, 109–111. doi: 10.1017/cjn.2021.500
Zong, Y., Lu, Z., Chen, M., Deng, L., Xie, Q., and Zhou, P. (2022a). Motor unit number estimation of the second Lumbrical muscle in human hand. Front. Physiol. 13:854385. doi: 10.3389/fphys.2022.854385
Zong, Y., Lu, Z., Chen, M., Li, X., Stampas, A., Deng, L., et al. (2021). CMAP scan examination of the first dorsal interosseous muscle after spinal cord injury. IEEE Trans. Neural Syst. Rehabil. Eng. 29, 1199–1205. doi: 10.1109/TNSRE.2021.3088061
Zong, Y., Lu, Z., Chen, M., Xie, Q., and Zhou, P. (2022b). MScanFit motor unit number estimation of human anconeus muscle. Muscle Nerve 65, 460–463. doi: 10.1002/mus.27487
Zong, Y., Lu, Z., Xu, P., Chen, M., Deng, L., Li, S., et al. (2022c). MScanFit motor unit number estimation of abductor pollicis brevis: findings from different experimental parameters. Front. Aging Neurosci. 14:953173. doi: 10.3389/fnagi.2022.953173
Keywords: MScanFit, motor unit number estimation, compound muscle action potential scan, electromyography, electrode recording area
Citation: Zhang D, Lu Z, Gong W and Zhou P (2024) Effect of surface electrode recording area on compound muscle action potential scan processing for motor unit number estimation. Front. Neurosci. 18:1382871. doi: 10.3389/fnins.2024.1382871
Edited by:
Mingming Zhang, Southern University of Science and Technology, ChinaReviewed by:
Tugrul Artug, Istanbul University-Cerrahpasa, TürkiyeLin Wang, Chinese Academy of Sciences (CAS), China
Copyright © 2024 Zhang, Lu, Gong and Zhou. This is an open-access article distributed under the terms of the Creative Commons Attribution License (CC BY). The use, distribution or reproduction in other forums is permitted, provided the original author(s) and the copyright owner(s) are credited and that the original publication in this journal is cited, in accordance with accepted academic practice. No use, distribution or reproduction is permitted which does not comply with these terms.
*Correspondence: Zhiyuan Lu, emhpeXVhbi5sdUB1b3IuZWR1LmNu; Weijun Gong, Z3dqMTk3MTA0QGNjbXUuZWR1LmNu