- 1Department of Pharmacology and Toxicology, Medical College of Georgia at Augusta University, Augusta, GA, United States
- 2Department of Neuroscience and Regenerative Medicine, Medical College of Georgia at Augusta University, Augusta, GA, United States
1 Introduction
The prevalence of neurodegenerative diseases and mental disorders have been increasing over the past few decades. While genetic and lifestyle factors are important to the etiology of these illnesses, the pathogenic role of environmental factors, especially toxicants such as pesticides encountered over the life span is receiving increased attention. In addition, some toxicants have been utilized as chemical warfare agents and they may pose a greater risk to human health than biological weapons because of easier access. Chemical warfare agents have been used in World War I and II, the Iran-Iraq War, and other more recent conflicts in the Middle East. Toxic chemicals have also increasingly attracted the attention of terrorists, since these weapons have potentially devastating effects on the general population. As an example, the Japanese terrorist cult Aum Shinrikyo released the nerve agent sarin in the Tokyo subway in 1995 killing 13 people and causing more than 5,800 people to seek medical attention. In addition, there have been a number of accidental toxicant exposures during storage and shipments of hazardous chemicals over the years including a recent example, the Ohio train derailment in 2023. It is thus important to establish more effective medical countermeasures to improve recovery from toxicant exposures to counter an ever present environmental health concern (National Toxicology Program, 2019; Reddy, 2024).
Among the toxicants found in many pesticides and chemical warfare agents, the chemicals known as organophosphates are most frequently associated with adverse long-term neurological consequences (Figueiredo et al., 2018; Naughton and Terry, 2018). Organophosphates are defined as any organic compound whose molecule contains phosphate ester groups. In addition to pesticides and chemical warfare agent organophosphorus compounds have been utilized as fuel additives, plasticizers, flame retardants, lubricants, and pharmaceuticals. Their primary mechanism of acute neurotoxicity is based on the irreversible inhibition of acetylcholinesterase which is the major enzyme responsible for hydrolysis of acetylcholine in the cholinergic synapse. In the body, acetylcholinesterase prevents overstimulation of postsynaptic neurons by reducing levels of acetylcholine available to the cholinergic receptor. However, as a result of organophosphorus exposure, the breakdown of acetylcholine is prevented, resulting in a state of sustained excitation of cholinergic receptors. Organophosphate chemical agents cause severe convulsions and seizures, leading to severe damage of the central nervous system (CNS). Antidotal therapy is available to combat the symptoms of acute organophosphate toxicity, however, there are no established interventions for the long-term neurological consequences of organophosphate exposure. It is thus highly desirable to better understand the mechanisms by which these chemical agents affect the nervous system chronically and lead to dysfunctional neuronal activity.
In the nervous system, lipids are the most abundant organic compounds, and a variety of lipids control the biophysical nature of lipid membranes. Glycosphingolipids are a class of lipids that are unique amphipathic molecules with a hydrophilic carbohydrate portion and hydrophobic lipid component. Gangliosides are sialic acid-containing glycosphingolipids known to play essential roles in cell-cell recognition, adhesion, signal transduction, and cellular migration, and that are crucial in all phases of neurogenesis (Itokazu and Yu, 2023; Itokazu et al., 2023). Mice lacking major glycolipids show lethal phenotypes, indicating the significance of glycolipids to mammalian physiology. Moreover, patients with ganglioside deficiencies exhibit severe clinical phenotypes and altered glycosphingolipid expression has been associated with neurodegenerative diseases and mental health disorders. It is also important to note that alterations in glycolipid composition after organophosphate exposure in mammals and birds have been reported (Rozengart and Taranova, 1969; Taranova, 1978; Islam et al., 1983; Khan and Hasan, 1988; Bush et al., 1995). Here we focus on the potential of glycolipids to protect and promote regeneration of the CNS in chemical-induced neurodegenerative diseases and mental health disorders as a therapeutic strategy.
2 Potential functions of gangliosides in chemical-induced neurodegenerative diseases and mental health disorders
2.1 Toxic chemicals are associated with neurological and psychiatric disorders
Multiple reports have made associations between environmental organophosphate exposure and neurodevelopmental deficits including impaired cognitive and neurobehavioral development, decreased intelligence quotient (IQ), and increased abnormal reflexes (reviewed, Jokanovic et al., 2023). Both prenatal and postnatal exposure to organophosphates have been associated with cognitive and attentional deficits as well as, attention deficit hyperactivity disorder (ADHD). Occupational exposure to organophosphates has also been associated with elevated levels of anxiety and depression in adults, although children were more sensitive to neurotoxicity caused by organophosphates during brain development. Organophosphorus ester-induced chronic neurotoxicity (OPICN) also called chronic organophosphate-induced neuropsychiatric disorder (COPIND) appears as a set of long-term, persistent, chronic neuropsychiatric symptoms including apathy, decreased visual memory, impaired vigilance, reduced abstract reasoning, anxiety, depression, increased social isolation, reduced fine motor coordination, confusion, dizziness, insomnia, reduced vibrotactile sensitivity, decreased academic skills, emotional lability, irritability, short-term memory deficits, decreased verbal attention, fatigue, problems with concentration, and slowing of reaction time. After single dose exposure to organophosphates in Japan in the Aum Shinrikyo terrorist attack, assault victims had eye-related symptoms, physical fatigue, numb muscles, headache, depressive mood, forgetfulness, and chronic lack of concentration for 5–10 years afterwards (Kawana et al., 2001; Yanagisawa et al., 2006). Despite considerable research to investigate the association between these toxic chemicals and persistent neurological and psychiatric disorders, neuropathologic mechanisms of the long-term impairments by organophosphate exposure remain unclear.
2.2 The cholinergic system and neurological and psychiatric diseases
Acetylcholine is the neurotransmitter of the cholinergic system and it has an important role in memory, attention, motivation, novelty seeking and arousal. Dysfunction of the cholinergic system is clinically significant in several diseases and thought to play a major role, in Alzheimer's disease (Terry and Buccafusco, 2003). Acetylcholine acts on two main types of receptors, nicotinic and muscarinic acetylcholine receptors. Neuronal nicotinic acetylcholine receptors (nAChRs) are expressed both pre- and post-synaptically in most regions of the brain (Terry et al., 2023) and it has been reported that dysfunctional muscarinic and nicotinic receptors contribute to the symptoms of multiple neurologic and psychiatric illnesses in addition to AD and include, Parkinson's disease (PD), epilepsy, schizophrenia, and depression. In the brains of patients with AD, nAChR mRNAs are decreased in brain regions that are important for memory and cognition and significant correlations between the levels of the loss of nAChRs in the brain and the degree of cognitive decline in AD have been reported. In the brains of patients with schizophrenia, decreases in the expression of nAChRs have also been observed. Imaging studies have also revealed that nAChR availability in depressed patients across all brain regions that were evaluated was lower than that in healthy controls (Terry et al., 2023).
Gangliosides have been reported to interact with neurotrophin receptors and support neuroprotective phenomena as well as other many essential functions (Fantini and Barrantes, 2009; Ledeen and Wu, 2015; Furukawa et al., 2019) that are important to cholinergic neurons. For example, cholinergic innervations of the rat cortex were decorticated and intracerebroventricular infusions of GM1 showed successful attenuation of reduced choline acetyltransferase levels. This GM1 effect was similar to that of NGF infusion, while a combination infusion of NGF and GM1 had a synergistic effect to significantly increase cholinergic presynaptic terminal size (Garofalo et al., 1993). In non-human primates, administration of NGF alone or in combination with GM1 induced a long-term protective effect on nucleus basalis cholinergic neurons after neocortical infarction. Although a protection of the cholinergic cell bodies was found with both treatments, a significant recovery of the neuritic processes was observed only in the double-treated monkeys (Liberini et al., 1993). A potential mechanism for the positive effects of GM1 described above may be related to its binding to the high affinity NGF receptor, TrkA (Mutoh et al., 1995, 1998). The studies described here indicate that modulation of important components of the molecular environments of cholinergic neurons (via gangliosides) may have broad therapeutic potential.
2.3 Altered ganglioside composition in chemical agent exposure and in CNS diseases
Patients with ganglioside-synthase mutations have symptomatic epilepsy syndrome with severe neuronal dysfunction or hereditary spastic paraplegias with additional neurological symptoms (reviewed, Itokazu et al., 2023). A majority of patients with neurodegenerative diseases have alterations in their glycosphingolipid metabolism and, those changes may contribute to their accompanying pathogenesis. Concentrations of complex gangliosides such as GM1 are significantly lower in the brains of patients with AD and PD (Wu et al., 2012; Hadaczek et al., 2015; Ariga, 2017; Seyfried et al., 2018). Some glycosphingolipid-synthase-knockouts (KO) are embryonic lethal. GM2 synthase (GM2S) is one of the key enzymes needed for synthesis of the major brain-type gangliosides, including GM1. GM2S-KO mice exhibit impaired movement and have virtually all the neuropathological symptoms of PD and cognitive impairment (Wu et al., 2011, 2012, 2020). GD3 synthase (GD3S)-KO mice led to depression-like behaviors and memory defect (Wang et al., 2014; Tang et al., 2021). With regard to the cholinergic system, Chol-1α gangliosides (GT1aα and GQ1bα, see Figure 1A) are minor species in the brain and serve as unique markers of cholinergic neurons (Ando et al., 1992; Hirabayashi et al., 1992; Itokazu and Yu, 2023). Chol-1α gangliosides may support cognitive functions such as memory and learning, and the administration of Chol-1α gangliosides appeared to alleviate the decreased synaptic functions in aged brains (Ando, 2014). GT1aα and GQ1ba, were found to be elevated in the brains of the patients with AD and AD model mice (Ariga et al., 2013; Ariga, 2017; Fukami et al., 2017). Neural stem cells (NSCs), non-differentiated precursor cells defined by their capacity for self-renewal and mulitpotency, express Chol-1α gangliosides (Ngamukote et al., 2007). Chol-1α antigens may play an important role in cholinergic synaptic transmission and participate in cognitive function, although the detailed mechanisms need to be determined. Overall, ganglioside deficiency leads to development of neurodegenerative diseases and mental health disorders, and a proper ganglioside composition may rescue the disease phenotypes.
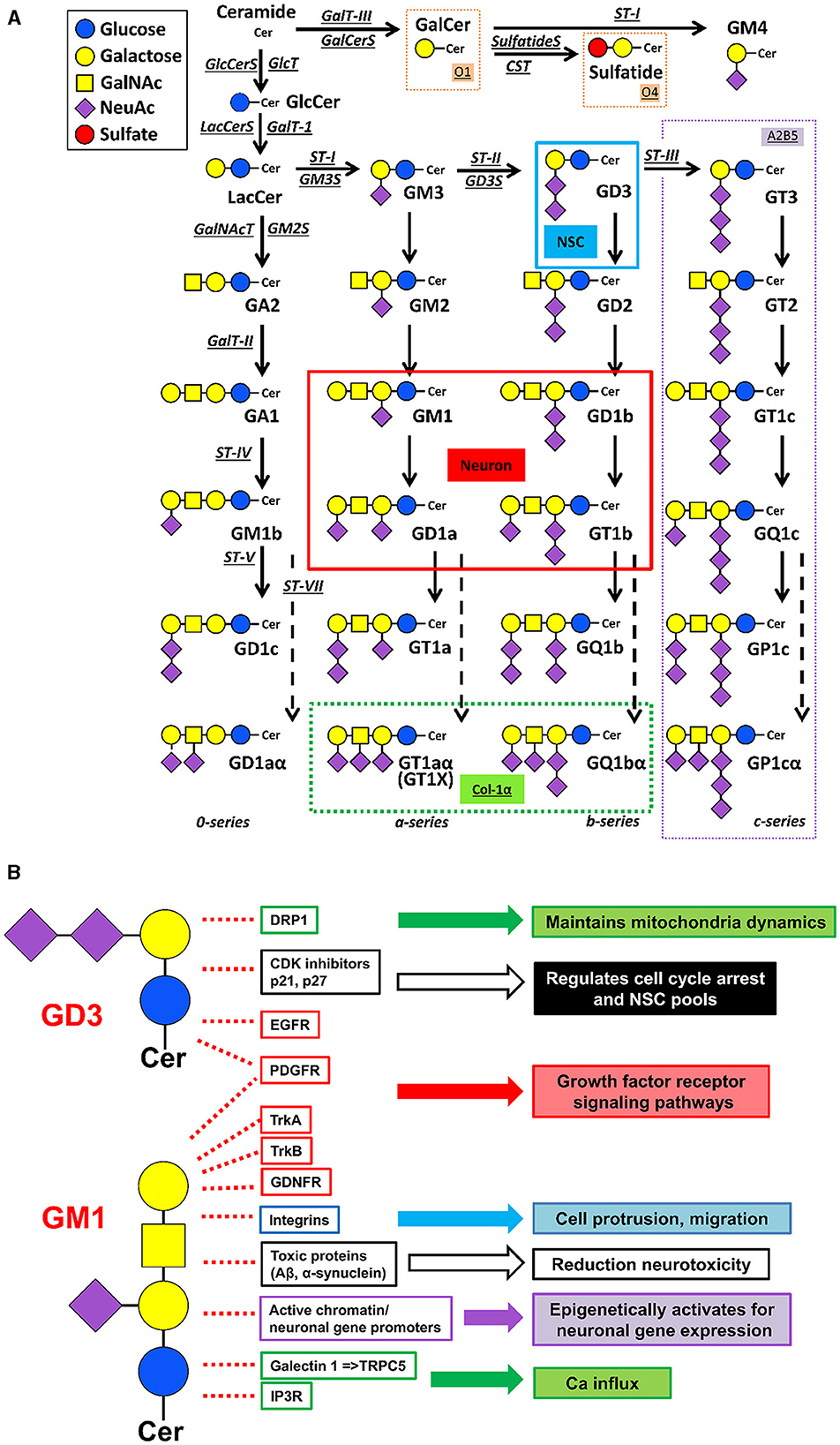
Figure 1. Metabolic pathways and functional roles of glycosphingolipids, including gangliosides. (A) Metabolic pathways and structure. Cer ceramide, CST cerebroside sulfotransferase (Gal3st1, sulfatide synthase), GalNAc-T N-acetylgalactosaminyltransferase I (B4galnt1, GA2/GM2/GD2/GT2 synthase), GalT-I galactosyltransferase I (B4galT5 & B4galt6, lactosylceramide synthase), GalT-II galactosyltransferase II (B3galt4, GA1/GM1/GD1b/GT1c synthase), GalT-III galactosyltransferase III (Ugt8a, galactosylceramide synthase), GlcT glucosyltransferase (Ugcg, glucosylceramide synthase), ST-I sialyltransferase I (St3gal5, GM3 synthase), ST-II sialyltransferase II (St8Sia1, GD3 synthase), ST-III sialyltransferase III (St8Sia3, GT3 synthase), ST-IV sialyltransferase IV (St3gal2, GM1b/GD1a/GT1b/GQ1c synthase), ST-V sialyltransferase V (St8sia5, GD1c/GT1a/GQ1b/GP1c synthase), ST-VII sialyltransferase VII (St6galnac6, GD1aα/GT1aα/GQ1bα/GP1cα-synthase). Official symbols of genes are represented in italics in this figure legend. GD3 is the most abundant ganglioside in neural stem cells (NSCs). c-Series gangliosides are A2B5 antigens. GM1, GD1a, GD1b, and GT1b are the most abundant ganglioside species in adult mammalian brain and neurons. Oligodendrocyte markers O1 and O4 are GalCer and sulfatide, respectively. GT1aα and GQ1bα are cholinergic-specific antigens (Chol-1α). (B) Examples of ganglioside functions. Without GD3, Drp1 levels are increased, and aberrant mitochondrial fragmentation is augmented. GD3 suppresses p21 expression and maintains p27 expression in NSCs. Gangliosides bind to the neurotrophic factor receptors to regulate their signalings. GM1 promotes integrin signaling for cell protrusion and migration. Gangliosides prevent and even reduce the accumulation of toxic proteins [e.g., amyloid-beta peptides (Aβs) and alpha-synuclein (α-syn)] in neurodegenerative diseases. Nuclear GM1 epigenetically promotes neuronal gene expression to sustain healthy neuronal functions. Elevation of GM1 levels induces Ca influx and neurite outgrowth. The listed in the figure is a limited examples, but, ganglioside-based multifunctional therapy is promising.
Effects of organophosphates on glycolipid compositions have been reported. Subcutaneously injected organophosphate, diisopropylphosphorofluoridate (DFP) into chickens, resulted in an increase in GQ1b and GT1b levels but the proportion of GD3 decreased, while the concentrations of protein, total lipid, total cholesterol, and phospholipid were not affected (Bush et al., 1995). These progressive changes in ganglioside composition correlated with increasing ataxia. Intraperitoneal administration of the organophosphate, metasystox depleted levels of gangliosides in cerebral hemisphere, cerebellum, brain stem and spinal cord of rats (Islam et al., 1983). Other studies also reported that organophosphate effects on glycolipid expression in the CNS of mammals and birds (Rozengart and Taranova, 1969; Taranova, 1978; Khan and Hasan, 1988). As the organophosphate-induced alteration of ganglioside composition may impact brain dysfunction, the mechanisms of ganglioside changes after the administration of organophosphate need to be determined.
2.4 Curative gangliosides are expected to protect from CNS damages against chemical threat
An often overlooked but key aspect for successful translational studies is that functional activities of proteins and genes are highly dependent upon their molecular environments. Cells and their subcellular organelles are surrounded by biological lipid membranes that define their individual cellular shape and maintain cellular organization as well as provide functional platforms for cellular signaling (reviewed, Itokazu and Yu, 2023). Specific gangliosides control molecular functions on biological membranes, including the plasma, mitochondrial, and nuclear membranes. For example, GD3 is involved in the maintenance of NSC fate determination by interacting with epidermal growth factor receptors (EGFRs), by modulating expression of cyclin-dependent kinase (CDK) inhibitors p27 and p21, and by regulating mitochondrial dynamics via associating a mitochondrial fission protein, the dynamin-related protein-1 (Drp1) (Wang and Yu, 2013; Tang et al., 2021; Fuchigami et al., 2023, 2024). The postnatal NSC pools are declined in GD3S-KO mice, resulting in depressive symptoms, olfactory dysfunction, and impaired memory, with the deficiency of postnatal neurogenesis (Wang and Yu, 2013; Itokazu et al., 2018; Tang et al., 2021; Fuchigami et al., 2024). On the other hand, intranasal or intracerebroventricular infusion of GD3 restored postnatal NSC pools of GD3S-KO and AD mice (Itokazu et al., 2019; Fuchigami et al., 2023). A major ganglioside, GM1 promotes neuronal differentiation by an epigenetic regulatory mechanism (Tsai and Yu, 2014; Itokazu et al., 2016; Tsai et al., 2016). Nuclear GM1 binds with acetylated histones on the promoters of GM2S, a critical enzyme for GM1 synthesis, as well as on the NeuroD1 genes in differentiating neurons. Further, intranasal GM1 infusion increased nuclear expression of nuclear receptor-related 1 (Nurr1), an essential transcription factor for differentiation, maturation, and maintenance of midbrain dopaminergic neurons in A53T and GM2S-KO mice (Itokazu et al., 2021). GM1 induces epigenetic activation of the tyrosine hydroxylase (TH) gene, including augmentation of acetylated histones and recruitment of Nurrr1 to the TH promoter region. To attenuate the effects of neurotoxic proteins in PD model mice, in our exciting data, nasal administration of gangliosides GM1 and/or GD3 reduced the neurotoxic α-synuclein levels and restored mitochondrial functions (Itokazu et al., 2021, 2023). Furthermore, exogenous GM1 seems beneficial to attenuate chronic microglia activation and neuroinflammation in neurodegenerative diseases (Galleguillos et al., 2022). GM1 also facilitates internalization of toxic proteins, such as α-synuclein into microglia through GM1-mediated endocytosis (Park et al., 2009). Pretreatment with intracerebroventricular infusion of GM1 for 4 days reduced organophosphate-, soman-induced seizure-related brain damage, while intraperitoneal injection of GM1 failed to provide protection (Ballough et al., 1998). So far, intracerebroventricular administration is the most reliable method to deliver gangliosides into the brain, we developed a more convenient non-invasive delivery procedure by intranasal infusion of gangliosides with success (Itokazu et al., 2021; Fuchigami et al., 2023). Intranasal administration can be a suitable route for efficient and non-invasive means for delivery of gangliosides to the brain, reducing systemic exposure and potential undesirable side effects.
3 Conclusion
The rapid rise in elderly populations worldwide is resulting in a dramatic increase in the incidence of age-related neurodegenerative illnesses. Currently, we lack both an understanding of the causes of these illnesses as well as how to effectively treat them. There is increasing interest in how environmental exposures over the lifespan might contribute to these diseases as well as the development of effective therapeutic interventions that are truly disease modifying and not just designed to provide temporary symptomatic relief. The purpose of this review was thus (1) to introduce one major environmental factor, toxicant (more specifically organophosphate) exposure and how it may lead to neurodegenerative illnesses, and (2) to introduce the role of gangliosides in the etiology of neurodegenerative diseases, how they may interact with organophosphates, and how exogenously administered gangliosides (especially by the intranasal route) might have diseases modifying capabilities for people who have neurodegenerative illnesses resulting from organophosphate exposures as well as other toxic insults. Gangliosides undergo dynamic qualitative and quantitative developmental, age-related, and pathological changes that correlate with neuronal function. Ganglioside microdomains provide a functional platform for cellular signaling of interacting molecules (Figure 1B). Progressive imbalance of cell membrane lipid composition is a physicochemical property that changes during normal aging, but further disruptions are observed in neurodegenerative diseases. A key aspect for successful translational studies is that the functional activities of proteins and genes are highly dependent upon their molecular environments, such as glycolipid micodomains. Multifunctional gangliosides can modulate protein and gene activities on plasma, mitochondrial, and nuclear membranes, in theory, may restore functions of specific molecules in the brains of patients with chemical-induced neurodegenerative diseases and mental health disorders.
Author contributions
YI: Conceptualization, Funding acquisition, Investigation, Resources, Writing—original draft, Writing—review & editing. AT: Conceptualization, Investigation, Project administration, Resources, Supervision, Writing—review & editing.
Funding
The author(s) declare that financial support was received for the research, authorship, and/or publication of this article. This work was partly supported by a National Institute of Neurological Disorders and Stroke grant (YI: R01NS100839), a Sheffield Memorial Grant of the CSRA Parkinson's Disease Support Group (YI), and the excellent infrastructural support of the Department of Pharmacology and Toxicology, Medical College of Georgia at Augusta University.
Conflict of interest
The authors declare that the research was conducted in the absence of any commercial or financial relationships that could be construed as a potential conflict of interest.
Publisher's note
All claims expressed in this article are solely those of the authors and do not necessarily represent those of their affiliated organizations, or those of the publisher, the editors and the reviewers. Any product that may be evaluated in this article, or claim that may be made by its manufacturer, is not guaranteed or endorsed by the publisher.
References
Ando, S. (2014). Glycoconjugate changes in aging and age-related diseases. Adv. Neurobiol. 9, 415–447. doi: 10.1007/978-1-4939-1154-7_19
Ando, S., Hirabayashi, Y., Kon, K., Inagaki, F., Tate, S., and Whittaker, V. P. (1992). A trisialoganglioside containing a sialyl alpha 2-6 N-acetylgalactosamine residue is a cholinergic-specific antigen, Chol-1 alpha. J. Biochem. 111, 287–290. doi: 10.1093/oxfordjournals.jbchem.a123751
Ariga, T. (2017). The pathogenic role of ganglioside metabolism in Alzheimer's disease-cholinergic neuron-specific gangliosides and neurogenesis. Mol. Neurobiol. 54, 623–638. doi: 10.1007/s12035-015-9641-0
Ariga, T., Itokazu, Y., Mcdonald, M. P., Hirabayashi, Y., Ando, S., and Yu, R. K. (2013). Brain gangliosides of a transgenic mouse model of Alzheimer's disease with deficiency in GD3-synthase: expression of elevated levels of a cholinergic-specific ganglioside, GT1aalpha. ASN Neuro 5, 141–148. doi: 10.1042/AN20130006
Ballough, G. P., Cann, F. J., Smith, C. D., Forster, J. S., Kling, C. E., and Filbert, M. G. (1998). GM1 monosialoganglioside pretreatment protects against soman-induced seizure-related brain damage. Mol. Chem. Neuropathol. 34, 1–23. doi: 10.1007/BF02815133
Bush, D. M., Lehning, E. J., and Bursian, S. J. (1995). The effects of diisopropylphosphorofluoridate (DFP) on the ganglioside profile in the chicken (Gallus domesticus) hindbrain. Neurotoxicology 16, 55–61.
Fantini, J., and Barrantes, F. J. (2009). Sphingolipid/cholesterol regulation of neurotransmitter receptor conformation and function. Biochim. Biophys. Acta 1788, 2345–2361. doi: 10.1016/j.bbamem.2009.08.016
Figueiredo, T. H., Apland, J. P., Braga, M. F. M., and Marini, A. M. (2018). Acute and long-term consequences of exposure to organophosphate nerve agents in humans. Epilepsia 59(Suppl. 2), 92–99. doi: 10.1111/epi.14500
Fuchigami, T., Itokazu, Y., Morgan, J. C., and Yu, R. K. (2023). Restoration of adult neurogenesis by intranasal administration of gangliosides GD3 and GM1 in the olfactory bulb of A53T alpha-synuclein-expressing Parkinson's-disease model mice. Mol. Neurobiol. 60, 3329–3344. doi: 10.1007/s12035-023-03282-2
Fuchigami, T., Itokazu, Y., and Yu, R. K. (2024). Ganglioside GD3 regulates neural stem cell quiescence and controls postnatal neurogenesis. Glia 72, 167–183. doi: 10.1002/glia.24468
Fukami, Y., Ariga, T., Yamada, M., and Yuki, N. (2017). Brain gangliosides in Alzheimer's disease: increased expression of cholinergic neuron-specific gangliosides. Curr. Alzheimer Res. 14, 586–591. doi: 10.2174/1567205014666170117094038
Furukawa, K., Ohmi, Y., Ohkawa, Y., Bhuiyan, R. H., Zhang, P., Tajima, O., et al. (2019). New era of research on cancer-associated glycosphingolipids. Cancer Sci. 110, 1544–1551. doi: 10.1111/cas.14005
Galleguillos, D., Wang, Q., Steinberg, N., Zaidi, A., Shrivastava, G., Dhami, K., et al. (2022). Anti-inflammatory role of GM1 and other gangliosides on microglia. J. Neuroinflamm. 19:9. doi: 10.1186/s12974-021-02374-x
Garofalo, L., Ribeiro-Da-Silva, A., and Cuello, A. C. (1993). Potentiation of nerve growth factor-induced alterations in cholinergic fibre length and presynaptic terminal size in cortex of lesioned rats by the monosialoganglioside GM1. Neuroscience 57, 21–40. doi: 10.1016/0306-4522(93)90109-S
Hadaczek, P., Wu, G., Sharma, N., Ciesielska, A., Bankiewicz, K., Davidow, A. L., et al. (2015). GDNF signaling implemented by GM1 ganglioside; failure in Parkinson's disease and GM1-deficient murine model. Exp. Neurol. 263, 177–189. doi: 10.1016/j.expneurol.2014.10.010
Hirabayashi, Y., Nakao, T., Irie, F., Whittaker, V. P., Kon, K., and Ando, S. (1992). Structural characterization of a novel cholinergic neuron-specific ganglioside in bovine brain. J. Biol. Chem. 267, 12973–12978. doi: 10.1016/S0021-9258(18)42369-1
Islam, F., Tayyaba, K., and Hasan, M. (1983). Organophosphate metasystox-induced increment of lipase activity and lipid peroxidation in cerebral hemisphere: diminution of lipids in discrete areas of the rat brain. Acta Pharmacol. Toxicol. 53, 121–124. doi: 10.1111/j.1600-0773.1983.tb01878.x
Itokazu, Y., Fuchigami, T., Morgan, J. C., and Yu, R. K. (2021). Intranasal infusion of GD3 and GM1 gangliosides downregulates alpha-synuclein and controls tyrosine hydroxylase gene in a PD model mouse. Mol. Ther. 29, 3059–3071. doi: 10.1016/j.ymthe.2021.06.005
Itokazu, Y., Fuchigami, T., and Yu, R. K. (2023). Functional impairment of the nervous system with glycolipid deficiencies. Adv. Neurobiol. 29, 419–448. doi: 10.1007/978-3-031-12390-0_14
Itokazu, Y., Li, D., and Yu, R. K. (2019). Intracerebroventricular infusion of gangliosides augments the adult neural stem cell pool in mouse brain. ASN Neuro 11:1759091419884859. doi: 10.1177/1759091419884859
Itokazu, Y., Tsai, Y. T., and Yu, R. K. (2016). Epigenetic regulation of ganglioside expression in neural stem cells and neuronal cells. Glycoconj. J. 34, 749–756. doi: 10.1007/s10719-016-9719-6
Itokazu, Y., Wang, J., and Yu, R. K. (2018). Gangliosides in nerve cell specification. Prog. Mol. Biol. Transl. Sci. 156, 241–263. doi: 10.1016/bs.pmbts.2017.12.008
Itokazu, Y., and Yu, R. K. (2023). Ganglioside microdomains on cellular and intracellular membranes regulate neuronal cell fate determination. Adv. Neurobiol 29, 281–304. doi: 10.1007/978-3-031-12390-0_10
Jokanovic, M., Oleksak, P., and Kuca, K. (2023). Multiple neurological effects associated with exposure to organophosphorus pesticides in man. Toxicology 484:153407. doi: 10.1016/j.tox.2022.153407
Kawana, N., Ishimatsu, S., and Kanda, K. (2001). Psycho-physiological effects of the terrorist sarin attack on the Tokyo subway system. Mil. Med. 166, 23–26. doi: 10.1093/milmed/166.suppl_2.23
Khan, N. A., and Hasan, M. (1988). Dose-related neurochemical changes in the levels of gangliosides and glycogen in various regions of the rat brain and spinal cord following methyl parathion administration. Exp. Pathol. 35, 61–65. doi: 10.1016/S0232-1513(88)80125-7
Ledeen, R. W., and Wu, G. (2015). The multi-tasked life of GM1 ganglioside, a true factotum of nature. Trends Biochem. Sci. 40, 407–418. doi: 10.1016/j.tibs.2015.04.005
Liberini, P., Pioro, E. P., Maysinger, D., Ervin, F. R., and Cuello, A. C. (1993). Long-term protective effects of human recombinant nerve growth factor and monosialoganglioside GM1 treatment on primate nucleus basalis cholinergic neurons after neocortical infarction. Neuroscience 53, 625–637. doi: 10.1016/0306-4522(93)90611-I
Mutoh, T., Tokuda, A., Inokuchi, J., and Kuriyama, M. (1998). Glucosylceramide synthase inhibitor inhibits the action of nerve growth factor in PC12 cells. J. Biol. Chem. 273, 26001–26007. doi: 10.1074/jbc.273.40.26001
Mutoh, T., Tokuda, A., Miyadai, T., Hamaguchi, M., and Fujiki, N. (1995). Ganglioside GM1 binds to the Trk protein and regulates receptor function. Proc. Natl. Acad. Sci. U. S. A. 92, 5087–5091. doi: 10.1073/pnas.92.11.5087
National Toxicology Program (2019). NTP monograph on the systematic review of long-term neurological effects following acute exposure to sarin. NTP Monogr. NTP-MGRAPH-6. doi: 10.22427/NTP-MGRAPH-6
Naughton, S. X., and Terry, A. V. Jr. (2018). Neurotoxicity in acute and repeated organophosphate exposure. Toxicology 408, 101–112. doi: 10.1016/j.tox.2018.08.011
Ngamukote, S., Yanagisawa, M., Ariga, T., Ando, S., and Yu, R. K. (2007). Developmental changes of glycosphingolipids and expression of glycogenes in mouse brains. J. Neurochem. 103, 2327–2341. doi: 10.1111/j.1471-4159.2007.04910.x
Park, J. Y., Kim, K. S., Lee, S. B., Ryu, J. S., Chung, K. C., Choo, Y. K., et al. (2009). On the mechanism of internalization of alpha-synuclein into microglia: roles of ganglioside GM1 and lipid raft. J. Neurochem. 110, 400–411. doi: 10.1111/j.1471-4159.2009.06150.x
Reddy, D. S. (2024). Special section on medical countermeasures, from bench to battlefield: translating experimental therapies for effective combat against chemical threats-editorial. J. Pharmacol. Exp. Ther. 388, 257–259. doi: 10.1124/jpet.123.002025
Rozengart, V. I., and Taranova, N. P. (1969). Effect of organic phosphate cholinesterase inhibitors on the content of gangliosides and other lipids in rabbit brain. Vopr. Med. Khim. 15, 404–409.
Seyfried, T. N., Choi, H., Chevalier, A., Hogan, D., Akgoc, Z., and Schneider, J. S. (2018). Sex-related abnormalities in substantia nigra lipids in parkinson's disease. ASN Neuro 10:1759091418781889. doi: 10.1177/1759091418781889
Tang, F. L., Wang, J., Itokazu, Y., and Yu, R. K. (2021). Ganglioside GD3 regulates dendritic growth in newborn neurons in adult mouse hippocampus via modulation of mitochondrial dynamics. J. Neurochem. 156, 819–833. doi: 10.1111/jnc.15137
Taranova, N. P. (1978). Guinea pig brain and spinal cord glycolipids in tricresyl phosphate poisoning. Vopr. Med. Khim. 24, 188–193.
Terry, A. V. Jr., and Buccafusco, J. J. (2003). The cholinergic hypothesis of age and Alzheimer's disease-related cognitive deficits: recent challenges and their implications for novel drug development. J. Pharmacol. Exp. Ther. 306, 821–827. doi: 10.1124/jpet.102.041616
Terry, A. V. Jr., Jones, K., and Bertrand, D. (2023). Nicotinic acetylcholine receptors in neurological and psychiatric diseases. Pharmacol. Res. 191:106764. doi: 10.1016/j.phrs.2023.106764
Tsai, Y. T., Itokazu, Y., and Yu, R. K. (2016). GM1 ganglioside is involved in epigenetic activation loci of neuronal cells. Neurochem. Res. 41, 107–115. doi: 10.1007/s11064-015-1742-7
Tsai, Y. T., and Yu, R. K. (2014). Epigenetic activation of mouse ganglioside synthase genes: implications for neurogenesis. J. Neurochem. 128, 101–110. doi: 10.1111/jnc.12456
Wang, J., Cheng, A., Wakade, C., and Yu, R. K. (2014). Ganglioside GD3 is required for neurogenesis and long-term maintenance of neural stem cells in the postnatal mouse brain. J. Neurosci. 34, 13790–13800. doi: 10.1523/JNEUROSCI.2275-14.2014
Wang, J., and Yu, R. K. (2013). Interaction of ganglioside GD3 with an EGF receptor sustains the self-renewal ability of mouse neural stem cells in vitro. Proc. Natl. Acad. Sci. U. S. A. 110, 19137–19142. doi: 10.1073/pnas.1307224110
Wu, G., Lu, Z. H., Kulkarni, N., Amin, R., and Ledeen, R. W. (2011). Mice lacking major brain gangliosides develop parkinsonism. Neurochem. Res. 36, 1706–1714. doi: 10.1007/s11064-011-0437-y
Wu, G., Lu, Z. H., Kulkarni, N., and Ledeen, R. W. (2012). Deficiency of ganglioside GM1 correlates with Parkinson's disease in mice and humans. J. Neurosci. Res. 90, 1997–2008. doi: 10.1002/jnr.23090
Wu, G., Lu, Z. H., Seo, J. H., Alselehdar, S. K., Defrees, S., and Ledeen, R. W. (2020). Mice deficient in GM1 manifest both motor and non-motor symptoms of Parkinson's disease; successful treatment with synthetic GM1 ganglioside. Exp. Neurol. 329:113284. doi: 10.1016/j.expneurol.2020.113284
Keywords: chemical warfare, cholinergic system, ganglioside, mental health disorder, neurodegenerative disease, neurotoxicity, organophosphate, pesticide
Citation: Itokazu Y and Terry AV Jr (2024) Potential roles of gangliosides in chemical-induced neurodegenerative diseases and mental health disorders. Front. Neurosci. 18:1391413. doi: 10.3389/fnins.2024.1391413
Received: 25 February 2024; Accepted: 12 April 2024;
Published: 22 April 2024.
Edited by:
Suman Chowdhury, The State University of New Jersey, United StatesReviewed by:
Sara Grassi, University of Milan, ItalyCopyright © 2024 Itokazu and Terry. This is an open-access article distributed under the terms of the Creative Commons Attribution License (CC BY). The use, distribution or reproduction in other forums is permitted, provided the original author(s) and the copyright owner(s) are credited and that the original publication in this journal is cited, in accordance with accepted academic practice. No use, distribution or reproduction is permitted which does not comply with these terms.
*Correspondence: Yutaka Itokazu, eWl0b2thenVAYXVndXN0YS5lZHU=; Alvin V. Terry Jr., YXRlcnJ5QGF1Z3VzdGEuZWR1
†ORCID: Yutaka Itokazu orcid.org/0000-0001-7800-8262
Alvin V. Terry Jr. orcid.org/0000-0003-2071-4767