- 1Bacteriology Department, Noguchi Memorial Institute for Medical Research, University of Ghana, Accra, Ghana
- 2Medical Research Council Unit The Gambia at the London School of Hygiene and Tropical Medicine, Banjul, Gambia
- 3National Tuberculosis Reference Laboratory, Lome, Togo
- 4Biological Sciences Department, Faculty of Pharmacy at Cheikh Anta Diop University, Dakar, Senegal
- 5Centre Muraz, Institut National de Santé Publique, Bobo-Dioulasso, Burkina Faso
- 6Unité de Formation et de Recherche en Sciences de la Vie et de la Terre, Université Nazi Boni, Bobo-Dioulasso, Burkina Faso
- 7Microbiology Department, Center for Tuberculosis Research Laboratory, Nigerian Institute of Medical Research, Lagos, Nigeria
- 8University Clinical Research Center, University of Sciences, Techniques and Technologies of Bamako, Bamako, Mali
- 9Department of Medical Microbiology and Parasitology, College of Medicine, University of Ibadan, Ibadan, Nigeria
- 10Department of Infection Biology, Faculty of Infectious and Tropical Diseases, London School of Hygiene and Tropical Medicine, London, United Kingdom
- 11Centre for Epidemic Preparedness and Response, London School of Hygiene and Tropical Medicine, London, United Kingdom
Drug-resistant (DR) tuberculosis (TB) is a major public health concern globally, complicating TB control and management efforts. West Africa has historically faced difficulty in combating DR-TB due to limited diagnostic skills, insufficient access to excellent healthcare, and ineffective healthcare systems. This has aided in the emergence and dissemination of DR Mycobacterium tuberculosis complex (MTBC) strains in the region. In the past, DR-TB patients faced insufficient resources, fragmented efforts, and suboptimal treatment outcomes. However, current efforts to combat DR-TB in the region are promising. These efforts include strengthening diagnostic capacities, improving access to quality healthcare services, and implementing evidence-based treatment regimens for DR-TB. Additionally, many West African National TB control programs are collaborating with international partners to scale up laboratory infrastructure, enhance surveillance systems, and promote infection control measures. Moreso, novel TB drugs and regimens, such as bedaquiline and delamanid, are being introduced to improve treatment outcomes for DR-TB cases. Despite these obstacles, there is optimism for the future of DR-TB control in West Africa. Investments are being made to improve healthcare systems, expand laboratory capacity, and support TB research and innovation. West African institutions are now supporting knowledge sharing, capacity building, and resource mobilization through collaborative initiatives such as the West African Network for TB, AIDS, and Malaria (WANETAM), the West African Health Organization (WAHO), and other regional or global partners. These efforts hold promise for improved diagnostics, optimized treatment regimens, and provide better patient outcomes in the future where drug-resistant TB in WA can be effectively controlled, reducing the burden of the disease, and improving the health outcomes of affected individuals.
1 Introduction
Tuberculosis (TB) caused by drug resistant (DR) strains of the Mycobacterium tuberculosis complex (MTBC) is called DR-TB whose treatment requires the use of relatively more toxic drugs for extended period of time with comparatively low success rate (1–4). Over the years, TB has been the leading cause of mortality by a single infectious disease until the recent COVID-19 pandemic. In 2022, the World Health Organization (WHO) reported 10.6 million new cases of TB and 1.6 million deaths (5). There was an estimated 410,000 cases of people who developed TB caused by MTBC that is resistant to rifampicin; the backbone of the Directly Observed Treatment Short-Course (DOTS) regimen (5). Africa, home to about 15.19% of humans on earth carries ~24% of the global TB burden (5). Unlike other geographical areas which harbor single to few lineages of the MTBC, WA harbors all the six major lineages of the MTBC (6, 7).
The primary risk factors for DR-TB infection include close contact with a DR-TB patient, history of TB treatment, cavity pulmonary TB, poor drug quality, inadequate supply of anti-TB drugs, treatment failure, poor adherence to treatment, inappropriate use of TB drugs and having a weakened immunity, such as in the case of HIV/AIDS, uncontrolled diabetes mellitus (DM), or malnutrition (8–12). Close contact with a DR-TB patients exposes one to potential inhalation of air droplets containing DR-TB bacilli released by infected patient. Therefore, if such a person develops TB from this exposure, it is very likely that it will be DR-TB from the onset. History of TB treatment presupposes that a person had a prior exposure to anti-TB drugs. However, among TB patients with multiple episodes of TB, molecular characterization has shown that there is more relapse than reinfection (13, 14). Exposure to anti-TB drugs can serve as selective pressure for DR-MTBC (15, 16). Therefore, TB patients with history of treatment are more likely to be DR-TB patients. On the other hand, poor adherence to treatment, inadequate supply of drugs and poor drug quality can lead to suboptimal concentration of drugs in the blood stream of infected patients. Thus, promoting selection of DR-MTBC and development of DR-TB during treatment (17–19). Again, improper prescription practices, such as using suboptimal drug regimens or using the wrong combination of drugs, can contribute to the development of drug resistance. For example, misuse of TB drugs in the treatment of other infections can contribute to the emergence of DR-MTBC strains. Thence, the need to keep drugs at desired temperature, prescribe good quality medications, follow the prescribed treatment regimen for the full duration even if symptoms improve, and reserve TB drugs for treatment of TB disease only. Lastly, factors that can potentially compromise the immunity, including HIV/AIDs, uncontrolled DM and malnutrition, negatively impact recovery from TB infection by prolonging the time it takes to clear the bacilli thus, allowing the bacteria enough time to evolve to tolerate the drugs potentially leading to resistance (13–15, 17–20). Addressing these risk factors is crucial for preventing the emergence and spread of DR-TB and improving treatment outcomes.
Unlike other bacteria that can easily acquire DR-conferring genes through horizontal gene transfer, DR among the MTBC is mostly caused by point mutations within DR- associated genes or promotor region of such genes (21). Nevertheless, the emergence and fixation of mutations that cause resistance to specific drugs is influenced by the genetic background of the infecting MTBC (22–24). This shows that resistance to same drug can be caused by different mutations with respect to different lineages of the MTBC (25, 26). This could lead to misdiagnosis of DR-TB in West Africa (WA) which has unique lineages of the MTBC when using commercially available rapid diagnostic assays. There is, therefore, the need for research into studying the determinants of DR in WA to support global efforts to develop rapid TB diagnostics. This opinion review explores the various aspects of DR-TB, including its definitions, causes, prevalence, challenges in diagnosis and treatment, and the implications for public health in WA.
2 Types of drug-resistant tuberculosis
There are several categories of DR-TB based on the drugs to which the infecting bacteria have developed resistance (Table 1). Mono-resistance refers to resistance to any single TB drug. Multidrug-Resistant (MDR) TB (MDR-TB) is defined as resistance to at least isoniazid and rifampicin. These two drugs are the backbone of the first-line TB treatment regimen. They are critical for TB treatment because resistance to both drugs automatically leads to treatment failure with the first line regimen and significantly limits the available treatment options (27–29). Extensively DR-TB (XDR-TB) is a more severe form of DR-TB caused by an MDR-MTBC that has developed further resistance to any fluoroquinolone and at least one of bedaquiline and linezolid (30). This further narrows down the treatment options, making XDR-TB challenging to treat successfully (27). The precursor to XDR-TB is pre-XDR-TB which is any MDR-TB with additional resistance to any fluoroquinolone whereas Polyresistant (PR) TB is defined as resistance to at least two TB drugs excluding rifampicin (30).
3 Prevalence and distribution of drug-resistant tuberculosis in West Africa
Data on DR-TB in WA are scanty which reflect diagnostic limitation but not actual epidemiologic situation (31). Drug-resistant TB including but not limited to MDR and XDR is an emerging public health problem in WA with several countries reporting different prevalence over the years (7, 32–35). A collaborative DR-TB surveillance among nine WA countries by the West African Network for Tuberculosis, AIDS and Malaria (WANETAM) showed that the WHO estimates are almost always below the actual incidence (Figure 1) (33). While the WHO estimated the prevalence of MDR-TB in new and retreatment cases to be 2 and 17%, respectively, this study which analyzed 974 bacterial isolates found 6 and 35%, respectively. It was found that 39% of isolates (corresponding to 39% of TB patients) were resistant to at least one first-line TB drug whereas 22% were MDR/RR-TB. Of concern is that, pre-XDR-TB cases were found in all the eight participating countries (33). The most recent WHO TB report shows different burden of MDR/RR-TB across WA ranging from least 0.67% among new TB cases in Togo to the highest 54% among previously treated TB cases in Guinea-Conakry as summarized below (Figure 2). However, getting the actual current prevalence and distribution of DR-TB in WA is essential for effective control and future management strategies. This section reviews the current burden and distribution of DR-TB in WA.
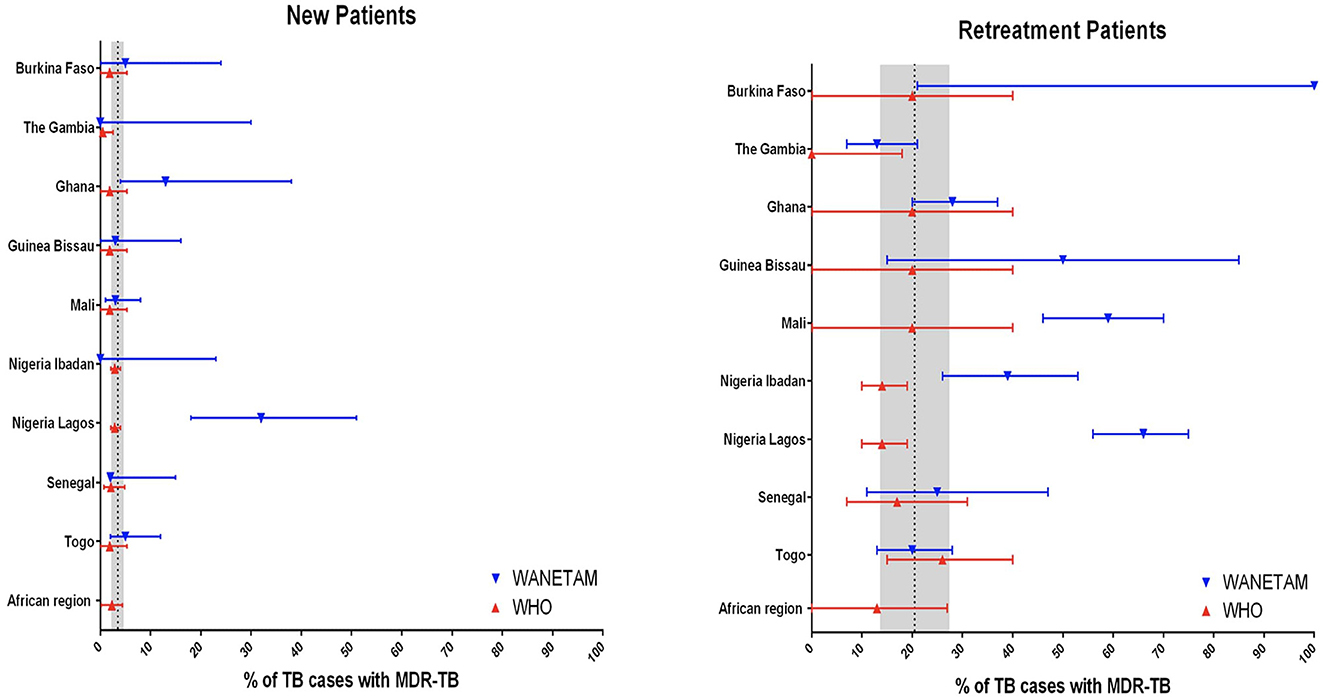
Figure 1. Comparing the 2016 WHO estimates of MDR-TB with actual incidence in nine West African countries [Adopted from Gehre et al. (33)].
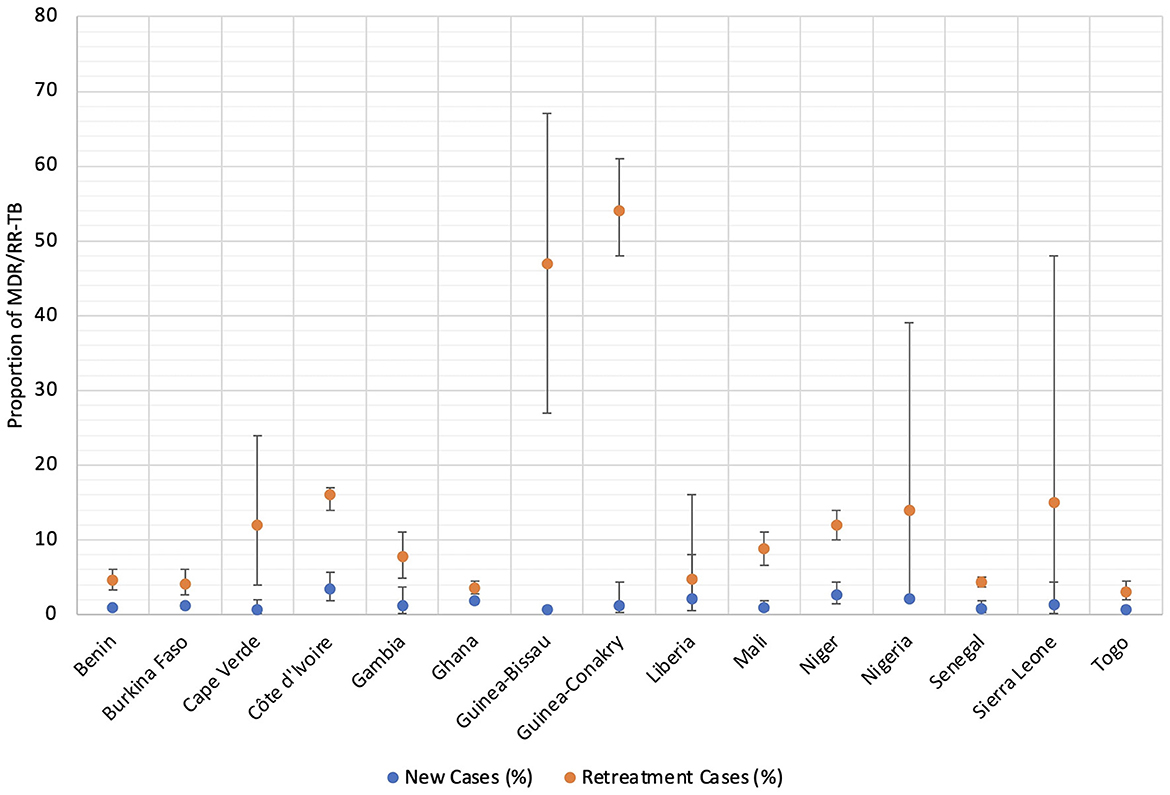
Figure 2. Comparing the 2022 WHO estimates of MDR/RR-TB between new and retreatment TB cases in West Africa (According to Global tuberculosis report, (5)).
3.1 Benin
The WHO estimated 0.93 and 4.6% MDR/RR-TB, respectively, among new and retreatment cases (5). However, there are varying prevalence rates of DR-TB reported in the country including 1.6 and 11% among new and previously treated TB patients, respectively (36), similar to another report of 12% MDR-TB among retreatment cases (34).
3.2 Burkina Faso
The WHO estimates of MDR/RR-TB in the country are 1.2 and 4.1% among new and retreatment cases, respectively (5), a slight reduction from 1.6 to 14% estimated in 2016. However, national survey between 2006 and 2007 found 2 and 14.5% MDR-TB, respectively, among new and retreatment cases (37).
3.3 Cape Verde
The island country has an estimated 0.69 and 12% MDR/RR-TB among new and previously treated TB cases, respectively, whereas the actual number of cases confirmed stood at two patients who were all put on an appropriate treatment regimen (5).
3.4 Côte d'Ivoire
The estimated figures are 3.5 and 16% MDR/RR-TB, respectively, among new and retreatment cases in the country (5). This correlates with a 10-year national survey which found 4.5 and 22% RR-TB among new and previously treated TB cases in the country (35). A cohort of 81 MDR-TB patients monitored in a pneumology unit, faced debilitating side effects leading to dropouts (39.2%), deaths (15.2%), diverse attacks (30.4%), and only 5% cured (38).
3.5 Gambia
The WHO estimated 1.2 and 7.8% MDR/RR-TB among new and retreatment cases, respectively (5). However, an earlier study reported no MDR-TB among new cases but 12.6% among retreatment cases in The Country (33).
3.6 Ghana
The WHO estimated 1.9 and 3.6% MDR/RR-TB among new and retreatment cases in Ghana (5). The first national DR-TB survey reported MDR/RR-TB rate of 3.1% (39) whereas a recent survey also reported a high rate of 5.6% (40) with 3.1% among new cases and as high as 33.3% among retreatment cases. Other studies in different parts of the country reported MDR/RR rates of 2.9% in the Greater Accra region (41), 3.31% in the Southern and Northern sectors (23), 3.5% in the Southern and Northern sectors (42), 3.1% in the Northern sector (43), and 8.1% in the Volta region (44). Additionally, a recent analysis of MTBC isolates from difficult-to-treat TB cases from Ghana over 2 years reported MDR/RR rate of 42% and pre-XDR-TB rate of 6.4% (45) agreeing with an earlier reported higher proportion of pre-XDR-TB (35%) among MDR-TB patients in the first pre-XDR-TB (33, 46) and XDR-TB case in the country (47).
3.7 Guinea-Bissau
The WHO estimated 0.73 and 47% MDR/RR-TB, respectively, among new and retreatment TB cases in the country (5). A study in the country also estimated 10 and 40% MDR/RR-TB, respectively, among new and retreatment cases but a mere 25% of these cases are diagnosed (48). To exacerbate the situation, about 45% of the diagnosed never complete treatment and for the remaining 55% who completed the treatment, success rate was only 34% (48).
3.8 Guinea-Conakry
The WHO estimated 1.2 and 54% MDR/RR-TB among new and retreatment cases, respectively (5). However, there is limited information on the actual burden of TB as well as DR-TB in the country. Nevertheless, there is a report of a high treatment cure rate (80.6%) MDR-TB in the country (49).
3.9 Liberia
As one of the three high TB burden countries in WA, the WHO estimated 2.1 and 4.8% MDR/RR-TB among new and retreatment cases, respectively (5). However, report by Borgenproject.org in collaboration with the national TB control program stipulates 2.5% prevalence of MDR/RR-TB in the country (https://borgenproject.org/tuberculosis-in-liberia/). Contrary to these estimates, two independent studies have reported very high MDR-TB prevalence of including 38.3% (50) and 23.5% (51) in the country.
3.10 Mali
The WHO estimate 1 and 8.8% MDR/RR-TB (5) contrary to the 3.3 and 58.6% MDR-TB (33), respectively, among new and retreatment TB cases in Mali. A similar report (3.4 and 66.3% among new and retreatment TB patients, respectively) has also been published (52). Furthermore, the investigators identified 18.8% resistance to second-line TB drugs among a random 38 (50%) of MDR isolates. The first 2 cases of XDR-TB were reported in 2017 with genomic evidence of nosocomial transmission from the first patient to the second. Of great concern, it took 10 months after diagnosis for the patients to receive appropriate treatment (53).
3.11 Niger
The WHO estimated 2.7 and 12% MDR/RR-TB among, respectively, new and previously treated TB cases in the country. The country seems to have a good program with 132 out of the 135 confirmed cases put on an appropriate treatment regimen (5).
3.12 Nigeria
Nigeria is one of the WHO recognized 30 high MDR/RR-TB burden countries with estimated 2.1% among new cases and 14% among previously treated TB cases (5). A recent study reported 3.5 and 7% MDR-TB among new cases and retreatment cases, respectively, in the country (54). However, relatively high prevalence of MDR/RR-TB 6 and 32%, respectively, among new and retreatment cases was also reported from a systematic review of studies from different parts of the country (55). A study did not distinguish new and retreatment cases reported MDR/RR-TB rate of 6.3 and 0.8% pre-XDR-TB (56).
3.13 Senegal
The WHO estimated 0.82 and 4.4% MDR/RR-TB, respectively, among new and retreatment cases (5). The National TB Control Program (NTP) of Senegal reported an increase in number of MDR-TB cases from 36 in 2010 to 77 in 2015 and MDR-TB rates of 0.5 and 17.9% among new and previously treated TB cases in 2014. However, an independent study in Senegal found the rate of MDR-TB to be 5.5 and 25% among new cases and retreatment cases, respectively (33).
3.14 Sierra Leone
There has been no national survey but the WHO estimated 1.3 and 15% MDR/RR-TB among new and retreatment cases, respectively (5). A recent study reported 14.7% MDR/RR-TB incidence (57) and a systematic review of reports from the country showed that among 628 identified MDR/RR-TB cases, 440 (70.1%) were retreatment cases with most being males (374/440) (58).
3.15 Togo
The WHO estimated 0.67 and 3% MDR/RR-TB, respectively, among new and retreatment cases (5). Nevertheless, a study monitoring a cohort of previously treated TB patients using GeneXpert and BACTEC MGIT found 24 and 25% of MDR-TB cases, respectively (59).
4 Challenges in diagnosis and treatment of drug-resistant tuberculosis
4.1 Diagnostic methods for detecting DR-TB and challenges
Accurate and timely diagnosis of DR-TB is essential for appropriate treatment plan. Several diagnostic methods including both phenotypic (culture-based) and DNA-based tests are available for detecting DR-TB. Due to the drawbacks of culture-based drug susceptibility testing (DST) methods such as requiring specialized laboratories, high expertise and often take weeks to provide results (60, 61), DNA-based using of polymerase chain reaction (PCR) are preferably used. For example, many of the countries are usingXpert MTB/RIF assay (Cepheid) detects rifampicin resistance (RR) and the line probe assays (Hain LifeSciences) (62). Nevertheless, these may not be accessible in some remote settings (63, 64). Furthermore, difficulty in maintenance of these equipment leads to referral of diagnostic services to distant reference labs leading to delayed diagnosis and initiation of appropriate treatment (64–66). Additionally, the paucibacillary nature of TB among HIV co-infected cases and children poses a diagnostic challenge, resulting in false-negative results and eventual underestimation of DR-TB (67).
4.2 Drug resistant TB treatment challenges
Treating DR-TB requires the use of multiple drugs for an extended duration, typically from 6 to 9 months (https://www.cdc.gov/tb/topic/drtb/bpal/default.htm#print). This long and complex treatment regimen poses significant challenges. First, the combination of drugs used can have significant side effects, including gastrointestinal disturbances, hepatotoxicity, ototoxicity, and psychiatric effects (68). These side effects can potentially lead to treatment discontinuation, non-adherence, and hence decreased treatment effectiveness. Secondly, availability of these second-line drugs may be limited in resource-constrained settings such as WA, affecting the ability to provide appropriate treatment to all diagnosed DR-TB patients (69). For example, out of the total 1,131 MDR-TB cases diagnosed in Ghana from 2018 to 2022, those that were put on treatment were 956 (84.5%) (Ghana, National Tuberculosis Control Program, Annual Report 2022, Unpublished). Albeit this proportion can be considered one of the highest in WA, it is still below the 90% enrolment recommended by the WHO (70). Consequently, limited access to these drugs can potentially lead to suboptimal treatment regimens that compromise treatment outcomes and contribute to the development of additional drug resistance.
5 Public health challenges for control of DR-TB in West Africa
Preventing the emergence and spread of DR-TB in WA is a multifaceted problem that demands a wholistic solution. Firstly, limited access to rapid diagnostics for accurate and timely detection of DR-TB leads to delayed diagnosis and inadequate treatment regimens (69, 71, 72). Delayed diagnosis compromises patient outcomes and allows for prolonged infectious period of DR-TB patients which potentiates transmission to others (73). Timely diagnosis and initiation of appropriate treatment are therefore crucial for better treatment outcomes that interrupt the transmission chain. Secondly, TB is highly stigmatized as a disease associated with either death or curse among many WA ethnic groups (64, 74, 75), thus the affected do not accept and seek help early, leading to high community transmissions (76).
Furthermore, due to the limited counseling at diagnosis and community engagement, some TB patients after diagnosis spend prolonged periods seeking spiritual assistance. Some also alternates between taking certified TB drugs from the clinics and seeking spiritual help leading to emergence and spread of DR-TB and poor treatment outcomes (77–79). Thus, incompetent treatment and poor adherence to treatment regimen undermines positive treatment outcomes (80, 81). Additionally, difficulty in tracing contacts of DR-TB patients for screening also contribute to delay in identifying potentially infected DR-TB patients for proper management (82). Contact tracing can further be complicated by the inability of sputum-based diagnostics to pick-up latent infections which may progress to active disease later (82–84).
Congregate settings, such as correctional facilities, homeless shelters, and refugee camps, create environments conducive for DR-TB spread (70). WA has over the past decade faced political instability in some countries leading to creation of several overcrowded refugee or internally displaced people camps with poor ventilation, and limited access to proper healthcare services conducive for spread of TB including DR-TB (85). Additionally, malnutrition and poor immunity negatively affect effectiveness of TB treatment and can lead to emergence of DR-TB (70). The interaction between TB and HIV can lead to more severe disease and treatment complications. The co-management of TB and HIV is a complex process involving multifaceted drug therapy, needing careful monitoring of drug-drug interactions (86). Therefore, the high prevalence of HIV/AIDS in WA further exacerbates the challenge of managing DR-TB. Notwithstanding these factors, the contribution of the TB bacteria itself to emergence, evolution and spread of DR cannot be overlooked. WA is a hot-spot of MTBC diversity with seven of the nine phylogenetic lineages including two WA restricted lineages with some genotypes associated with DR as well as specific DR-conferring and/or associated mutations (7, 23, 87–91).
Drug resistant MTBC strains require more expensive and prolonged treatment regimens, straining healthcare systems and diverting resources from other public health priorities (70, 71, 92, 93). This is even more apparent in resource limited settings in WA where the demand for these resources overwhelms supply. The high cost and intermittent supply of diagnostics and medications for DR-TB limits their accessibility and subsequently hinder effective control and management of DR-TB (71). DR-TB undermines TB control efforts by reducing treatment success rate and increasing cost of management (94). The average treatment success rate for uncomplicated TB is 85% whereas that for MDR/RR-TB albeit more expensive is reduced to 60% and that for pre-XDR/XDR-TB is even worse and requires using relatively more expensive drugs compared to MDR-TB (70, 95). With the continuous increase in the number of drugs becoming ineffective against the MTBC, the affected TB patients are gradually challenged with limited treatment options.
The Economic Community of West African States (ECOWAS) allows free movement of goods and services among member states. While there is daily heavy movement of people including nomads, herdsmen and refugees as well as animals along approved and unapproved routes (96–98), the sub-region has no border control measures. The non-existent TB testing strategies at these ports of entry concomitant with TB endemicity of the region, encourages people and/or livestock with either active or latent TB infection (LTBI) including DR-TB to cross borders unhindered with it accompanying public health implications (36, 99). There is therefore the need for regional collaborations and coordination in addressing DR-TB through regional active surveillance and creation of awareness.
6 Potential consequences of uncontrolled DR-TB
The consequences of uncontrolled DR-TB transmission are far-reaching. Firstly, it will result in hyper transmission of DR-TB and consequently a higher DR-TB burden and increased mortality (100, 101). Again, uncontrolled DR-TB can result in economic burdens at both individual and societal levels, because the costs of extended treatment regimens, hospitalization, and the loss of productivity have profound implications for individuals and healthcare systems (102–104). The economic impact can be exacerbated because DR-TB disproportionately affects poor populations, thus furthering poverty and inequality (105–107). Moreover, uncontrolled transmission of DR-TB in WA will compromise global health security because the interconnectedness of countries and ease of travel within the sub-region and overseas will facilitate the spread of DR-TB across borders, posing a threat to populations worldwide (70, 86).
7 Strategies for addressing drug-resistant tuberculosis in West Africa
7.1 Strengthening healthcare infrastructure and capacity
A critical step in addressing DR-TB in WA is political will of respective governments to reduce overreliance on foreign aid (71, 108). They must be committed to strengthen their health care systems to be more responsive/sensitive and resilient. Notwithstanding the significant help these aid agencies offer, their activities are affected by shocks such as outbreaks of other diseases as was seen with COVID-19 (64, 66, 86, 109, 110). Therefore, ensuring uninterrupted DR-TB control activities in WA demands governments in WA to set aside significant portions of their annual budgets toward improving laboratory facilities for accurate and timely diagnosis of DR-TB, ensuring access to DST, and establishing robust treatment programs that do not run out of required drugs (71, 111). In addition to physical structure and supplies, there is also the need to offer specialized training for healthcare providers in the diagnosis, management, and treatment of DR-TB, as well as improving infection control (112, 113).
7.2 Enhanced surveillance for early detection of DR-TB
There is the need for comprehensive surveillance system that tracks DR-TB patterns and monitors the spread of resistant strains in endemic communities (82, 114). Such surveillance system requires sensitive, rapid diagnostics for point-of-care testing and real-time data collection analysis tools to promote early detection of outbreaks as well as monitor cross-border importation/exportation of DR-TB (115). The deployment of GeneXpert MTB/RIF in WA, allowing dual diagnosis of TB and rifampicin resistance within hours is commendable (116, 117). The new GeneXpert MTB/XDR deployed in few reference labs in the sub-region work in concert with the GeneXpert MTB/RIF to give better resolution on MTBC susceptibility to RIF, INH, RQs and (118, 119). Nevertheless, the GeneXpert is inapplicable as a point of care tool in rural areas. On the other hand, the newly introduced Seegene-Anyplex-II-MTB/MDR/XDR (120), a real-time PCR-based diagnostics may account for some limitations of the GeneXpert platform, but requires evaluation in WA the hot-spot of MTBC diversity to access its sensitivity and specificity (7, 121, 122). Furthermore, efficient contact tracing and follow-up of individuals exposed to DR-TB patients with regular testing and screening can promote early detection of potential emerging cases and help prevent secondary transmissions whilst improving treatment outcomes with appropriate treatment regimens (123, 124).
7.3 Research into the impact of MTBC diversity on TB control
In the past two decade, some West African scientists are gradually making progress in basic scientific research toward understanding the biology of the MTBC (122, 125–130) and the potential impact of MTBC diversity on TB control (47, 131–133). This is because WA harbors seven out of the nine phylogenetic lineages (L1-L4, L7 & L8 making up M. tuberculosis sensu stricto [Mtbss] and L5, L6 & L9 making up M. africanum [Maf]) of the MTBC (6, 90) with a couple of WA-restricted several lineages (Maf lineages) and sub-lineages (Ghana and Cameroun sub-lineages of L4) (7). Some highlights of research findings from WA are described below. Maf transmits equally as Mtbss but progresses relatively slowly to active disease and is associated with relatively smaller transmission clusters (134, 135). Whereas, INH-resistance among Mtbss is driven by katG mutations conferring high-level resistance, INH-resistance among Maf is driven by inhApro mutations conferring low-level resistance (23). Maf lineages L5 and L6 are two distinct pathogens restricted to WA by unknown mechanisms (122). Maf L5 is also more likely to be associated with DR-TB compared to L6 similar to the association of Mtbss L4 Ghana genotype with DR-TB in WA (23, 43). Finally slower in vitro growth of Maf (136) in concomitance with its slower sputum-smear conversion rate (137) may warrant review of DOTS in West Africa as the 6 months may not be adequate for TB caused by Maf (138). Nevertheless, there is still room for further TB research in WA to contribute knowledge toward development of more efficient control tools.
7.4 Development of new drugs from traditional medicinal plants
The continuous evolution of DR limits treatment options for DR-TB and threatens to eventually make TB untreatable. Therefore, new and more effective drugs are crucial for combating DR-TB. Current treatment regimens for DR-TB are lengthy, complex, and associated with significant side effects (70). Investing in research and development of potent but less toxic drugs is essential whereas those that can give shorter treatment duration would be an added advantage. Most current efforts toward development of new TB treatment regimens involve exploring new drug targets and repurposing existing drugs (94, 139, 140). However, WA is home to numerous plant-based medicines for treating myriad of diseases including TB for centuries. Some of these plant preparations have recently been scientifically assessed for their anti-mycobacteria activity with promising outcomes (141). There is the need to invest in taking these promising preparations into pre-clinical trials for further development and advance research into identify and characterizing the active compound (s) as leads for new anti-TB drugs. Expedite development of novel anti-TB agents originating from the sub-region as well as integration of traditional, complementary, and alternative medicine into the health care system, particularly in rural areas where they are most prevalent, can enhance access to TB treatment, provided safety and efficacy standards are met. The provision of independent expert advice and support to countries in this regard by the WHO and African Centres for Disease Control are thence commendable (142).
7.5 Efficient public education and awareness campaigns for promoting knowledge, dispelling myths, and encouraging behavioral change
There are many innovative communication platforms that can be used for public education on DR-TB including mass media, social media, community meetings, workshops, and educational materials tailored to different populations using local languages to enhance the reach and impact of the message (71, 143–147). Such campaigns must engage communities, healthcare providers, policymakers, and other influential persons including but not limited to celebrities and local leaders in efforts to prevent the spread of DR-TB (143). The campaign should rely on experts to provide accurate and accessible information about TB, its causes, transmission, and preventive measures to help dispel misconceptions surrounding TB that negatively impact timely diagnosis and treatment (70, 147–150). Dispelling such myths will dispel stigma and discriminations against TB patients and fostering understanding, empathy, and acceptance as well as support for those affected. Secondly, such campaigns must raise awareness on antibiotic stewardship that promotes responsible use of antibiotics emphasizing the importance of completing prescribed courses of treatment and avoiding the use of antibiotics without prescription to help protect the potency of available TB drugs (151, 152). In addition, there should be a system for monitoring the campaign activities and evaluating the impact of the campaign on knowledge acquisition and attitudinal changes in relation to TB and DR-TB in the catchment communities as well as identify areas for improvement. Finally, such public education efforts must be sustained for longer periods to ensure that the intended knowledge becomes part and parcel of the target.
7.6 Fostering collaborations and partnerships among stakeholders
Addressing the complex challenge of DR-TB requires collaboration and partnerships among stakeholders including national TB control programs, government agencies, non-governmental organizations, healthcare providers, donors, religious bodies, traditional leaders, industry, and academia across the region. Such collaborative efforts can help mobilize resources, share expertise, coordinate activities, and advocate for enactment of new policies as well as modification of existing policies to fight DR-TB in the sub-region (153, 154). The initiative by the West African Network for Tuberculosis, AIDs, and Malaria (WANETAM) including 14 institutions in eight West African countries needs commendation. WANETAM is funded by the European and Development Countries Clinical Trials Partnership (EDCTP) to build capacity in standardized laboratory techniques essential for clinical trials and public health relevant diagnostics and research since 2008 (https://wanetam.org). Over the years the network has trained several clinicians, laboratory technicians, research administrators and research scientists as well as built both physical and electronic infrastructure in WA contributing to the fight against diseases of public health importance including DR-TB. There is, however, the need for several of such within sub-region collaborations funded by local governments for sustainability.
8 Conclusion
The growing burden of DR-TB in WA demands collective action and a unified approach to combat this daunting public health challenge. The strategies and interventions discussed in this article, including strengthening healthcare infrastructure, enhancing surveillance and infection control measures, developing innovative rapid, accurate and affordable diagnostics, developing new potent but less toxic drugs, ensuring access to care, and fostering partnerships and collaborations with academic institutions, pharmaceutical companies, and NGOs provide a roadmap for addressing DR-TB in the sub-region. However, the actual fight against DR-TB requires sustained commitment and innovations to overcome the existing barriers and improve patient outcomes. To effectively combat DR-TB in WA, it is imperative for West African governments, regional bodies, international organizations, healthcare providers, researchers, and affected communities and other interested stakeholders to work together to mobilize resources, advocate for policy changes, and ensure the implementation of comprehensive interventions in the sub-region. By implementing these comprehensive strategies against DR-TB, we can achieve a sub-region that is free from the threat of TB including DR-TB and ensuring the wellbeing of people living in WA and beyond.
Author contributions
IO: Conceptualization, Formal analysis, Validation, Visualization, Writing—original draft, Writing—review & editing. AA-P: Writing—review & editing. KA: Writing—review & editing. AD: Writing—review & editing. AS: Writing—review & editing. PA: Visualization, Writing—review & editing. SO-W: Writing—review & editing. NO: Writing—review & editing. BD: Writing—review & editing. YD: Writing—review & editing. AK: Writing—review & editing. MA: Conceptualization, Data curation, Funding acquisition, Resources, Supervision, Validation, Writing—review & editing. DY-M: Conceptualization, Data curation, Funding acquisition, Resources, Supervision, Validation, Writing—review & editing.
Funding
The author(s) declare financial support was received for the research, authorship, and/or publication of this article. The authors are members of the TB Work Package of the WANETAM-3 consortium funded by the European & Developing Countries Clinical Trials Partnership (EDCTP) Grant No. CSA-2020-NOE-3103.
Acknowledgments
We thank the coordinator of WANETAM Prof. Souleymane Mboup and the program manager Mr. Jean Pierre Nguessan both at the Institut de Recherche en Santé, de Surveillance Epidémiologique et de Formation (IRESSEF) for their unflinching support for the consortium. We also thank We thank Prof. Assan Jaye, Mr. Dembo Kante both at the Medical Research Council, The Gambia Unit at the London School of Hygiene and Tropical Medicine for their unrelenting support.
Conflict of interest
The authors declare that the research was conducted in the absence of any commercial or financial relationships that could be construed as a potential conflict of interest.
Publisher's note
All claims expressed in this article are solely those of the authors and do not necessarily represent those of their affiliated organizations, or those of the publisher, the editors and the reviewers. Any product that may be evaluated in this article, or claim that may be made by its manufacturer, is not guaranteed or endorsed by the publisher.
References
1. El Hamdouni M, Bourkadi JE, Benamor J, Hassar M, Cherrah Y, Ahid S. Treatment outcomes of drug resistant tuberculosis patients in Morocco: multi-centric prospective study. BMC Infect Dis. (2019) 19:1–7. doi: 10.1186/s12879-019-3931-5
2. Abubakar M, Ahmad N, Ghafoor A, Latif A, Ahmad I, Atif M, et al. Treatment outcomes of extensively drug-resistant tuberculosis in pakistan: a countrywide retrospective record review. Front Pharmacol. (2021) 12:1–9. doi: 10.3389/fphar.2021.640555
3. Baluku JB, Nakazibwe B, Naloka J, Nabwana M, Mwanja S, Mulwana R, et al. Treatment outcomes of drug resistant tuberculosis patients with multiple poor prognostic indicators in Uganda: a countrywide 5-year retrospective study. J Clin Tuberc Other Mycobact Dis. (2021) 23:100221. doi: 10.1016/j.jctube.2021.100221
4. Zhang MW, Zhou L, Zhang Y, Chen B, Peng Y, Wang F, et al. Treatment outcomes of patients with multidrug and extensively drug-resistant tuberculosis in Zhejiang, China. Eur J Med Res. (2021) 26:1–10. doi: 10.1186/s40001-021-00502-0
6. de Jong BC, Antonio M, Gagneux S. Mycobacterium africanum-review of an important cause of human tuberculosis in West Africa. PLoS Negl Trop Dis. (2010) 4:e744. doi: 10.1371/journal.pntd.0000744
7. Yeboah-Manu D, Asare P, Asante-Poku A, Otchere ID, Osei-Wusu S, Danso E, et al. Spatio-temporal distribution of Mycobacterium tuberculosis complex strains in Ghana. PLoS ONE. (2016) 11:e0161892. doi: 10.1371/journal.pone.0161892
8. Baghaei P, Tabarsi P, Chitsaz E, Novin A, Alipanah N, Kazempour M, et al. Risk factors associated with multidrug-resistant tuberculosis. Tanaffos. (2009) 8:17–21. doi: 10.3329/imcj.v3i1.2917
9. Abdel O, Akl M, Ali A, Mahalli E. Drug resistant tuberculosis: risk factors and resources- utilization at a chest disease clinic, Alexandria, Egypt. Rep Opin. (2012) 4:16–22.
10. Mulu W, Mekonnen D, Yimer M, Admassu A, Abera B. Risk factors for multidrug resistant tuberculosis patients in amhara national regional state. Afr Health Sci. (2015) 15:368–77. doi: 10.4314/ahs.v15i2.9
11. De Santa, Sujibala N, Saji J, John L, Memane K. A study to assess the knowledge regarding tuberculosis among adults from selected urban and rural areas of Pune district. Pharma Innov J. (2019) 8:231–2.
12. Ndiaye M, Yanogo PK, Sawadogo B, Diallo F, Antara S, Meda N. Factorsmulti-drug-resistantassociated with 2010-2016 tuberculosis in Dakar, Senegal. J Public Health Africa. (2019) 10:80–3. doi: 10.4081/jphia.2019.1099
13. Horsburgh CR, O'Donnell M, Chamblee S, Moreland JL, Johnson J, Marsh BJ, et al. Revisiting rates of reactivation tuberculosis: population-based approach. Am J Respir Crit Care Med. (2010) 182:420–5. doi: 10.1164/rccm.200909-1355OC
14. Zong Z, Huo F, Shi J, Jing W, Ma Y, Liang Q, et al. Relapse versus reinfection of recurrent tuberculosis patients in a national tuberculosis specialized hospital in Beijing, China. Front Microbiol. (2018) 9:1–8. doi: 10.3389/fmicb.2018.01858
15. Cohen T, van Helden PD, Wilson D, Colijn C, McLaughlin MM, Abubakar I, et al. Mixed-strain Mycobacterium tuberculosis infections and the implications for tuberculosis treatment and control. Clin Microbiol Rev. (2012) 25:708–19. doi: 10.1128/CMR.00021-12
16. Jenkins HE, Tolman AW, Yuen CM, Parr JB, Keshavjee S, Pérez-Vélez CM, et al. Incidence of multidrug-resistant tuberculosis disease in children: systematic review and global estimates. Lancet. (2014) 383:1572–9. doi: 10.1016/S0140-6736(14)60195-1
17. Bagchi S, Ambe G, Sathiakumar N. Determinants of poor adherence to anti-tuberculosis treatment in Mumbai, India. Int J Prev Med. (2010) 1:223–32.
18. Gashu KD, Gelaye KA, Tilahun B. Adherence to TB treatment remains low during continuation phase among adult patients in Northwest Ethiopia. BMC Infect Dis. (2021) 21:1–10. doi: 10.1186/s12879-021-06428-6
19. Monde N, Munyeme M, Chongwe G, Wensman JJ, Zulu M, Siziya S, et al. First and second-line anti-tuberculosis drug-resistance patterns in pulmonary tuberculosis patients in Zambia. Antibiotics. (2023) 12:166. doi: 10.3390/antibiotics12010166
20. Jenkins HE, Crudu V, Soltan V, Ciobanu A, Domente L, Cohen T. High risk and rapid appearance of multidrug resistance during tuberculosis treatment in Moldova. Eur Respir J. (2014) 43:1132–41. doi: 10.1183/09031936.00203613
21. Smith T, Wolff KA, Nguyen L. Molecular biology of drug resistance in Mycobacterium tuberculosis. Curr Top Microbiol Immunol. (2013) 374:53–80. doi: 10.1007/82_2012_279
22. Park YK, Shin S, Ryu S, Cho SN, Koh WJ, Kwon OJ, et al. Comparison of drug resistance genotypes between Beijing and non-Beijing family strains of Mycobacterium tuberculosis in Korea. J Microbiol Methods. (2005) 63:165–72. doi: 10.1016/j.mimet.2005.03.002
23. Otchere I, Asante-Poku A, Osei-Wusu S, Baddoo A, Sarpong E, Ganiyu AHH, et al. Detection and characterization of drug-resistant conferring genes in Mycobacterium tuberculosis complex strains: A prospective study in two distant regions of. Tuberculosis. (2016) 99:147–54. doi: 10.1016/j.tube.2016.05.014
24. Luo D, Zhao J, Lin M, Liu F, Huang S, Zhang Y, et al. Drug resistance in newly presenting and previously treated tuberculosis patients in Guangxi Province, People's Republic of China. Asia Pacific J Public Heal. (2017) 29:296–303. doi: 10.1177/1010539517700474
25. Siu GKH, Zhang Y, Lau TCK, Lau RWT, Ho PL, Yew WW, et al. Mutations outside the rifampicin resistance-determining region associated with rifampicin resistance in Mycobacterium tuberculosis. J Antimicrob Chemother. (2011) 66:730–3. doi: 10.1093/jac/dkq519
26. Castro RAD, Ross A, Kamwela L, Reinhard M, Loiseau C, Feldmann J, et al. The genetic background modulates the evolution of fluoroquinolone-resistance in Mycobacterium tuberculosis. Mol Biol Evol. (2020) 37:195–207. doi: 10.1093/molbev/msz214
27. Kliiman K, Altraja A. Predictors of poor treatment outcome in multi- and extensively drug-resistant pulmonary TB. Eur Respir J. (2009) 33:1085–94. doi: 10.1183/09031936.00155708
28. Panford V, Kumah E, Kokuro C, Adoma PO, Baidoo MA, Fusheini A, et al. Treatment outcomes and associated factors among patients with multidrug-resistant tuberculosis in Ashanti Region, Ghana: a retrospective, cross-sectional study. BMJ Open. (2022) 12:1–9. doi: 10.1136/bmjopen-2022-062857
29. Soeroto AY, Nurhayati RD, Purwiga A, Lestari BW, Pratiwi C, Santoso P, et al. Factors associated with treatment outcome of MDR/RR-TB patients treated with shorter injectable based regimen in West Java Indonesia. PLoS ONE. (2022) 17:e0263304. doi: 10.1371/journal.pone.0263304
30. WHO. Meeting Report of the WHO Expert Consultation on the Definition of Extensively Drug-Resistant Tuberculosis. Available online at: https://www.who.int/publications/i/item/meeting-report-of-the-who-expert-consultation-on-the-definition-of-extensively-drug-resistant-tuberculosis (accessed August 4, 2022).
31. Saleri N, Badoum G, Ouedraogo M, Dembélé SM, Nacanabo R, Bonkoungou V, et alExtensively drug-resistant tuberculosis, Burkina Faso. Emerg Infect Dis. (2010) 16:840–2. doi: 10.3201/eid1605.091262
32. Lukoye D, Ssengooba W, Musisi K, Kasule GW, Cobelens FGJ, Joloba M, et al. Variation and risk factors of drug resistant tuberculosis in sub-Saharan Africa: a systematic review and meta-analysis. BMC Public Health. (2015) 15. doi: 10.1186/s12889-015-1614-8
33. Gehre F, Otu J, Kendall L, Forson A, Kwara A, Kudzawu S, et al. The emerging threat of pre-extensively drug-resistant tuberculosis in West Africa: preparing for large-scale tuberculosis research and drug resistance surveillance. BMC Med. (2016). 14:160. doi: 10.1186/s12916-016-0704-5
34. Affolabi D, Sanoussi N, Codo S, Sogbo F, Wachinou P, Massou F, et al. First insight into a nationwide genotypic diversity of Mycobacterium tuberculosis among previously treated pulmonary tuberculosis cases in Benin, West Africa. Can J Infect Dis Med Microbiol. (2017) 2017:6–11. doi: 10.1155/2017/3276240
35. N'Guessan K, Ouassa T, Dean AS, Alagna R, Adagra GD, Ibode V, et al. Multidrug-resistant tuberculosis in Côte d'Ivoire from 1995 to 2016: results of national surveys. Eur J Microbiol Immunol. (2018) 8:91–4. doi: 10.1556/1886.2018.00001
36. Affolabi D, Adjagba OABG, Tanimomo-Kledjo B, Gninafon M, Anagonou SY, Portaels F. Anti-tuberculosis drug resistance among new and previously treated pulmonary tuberculosis patients in Cotonou, Benin. Int J Tuberc Lung Dis. (2007) 11:1221–4.
37. Diandé S, Badoum G, Combary A, Zombra I, Saouadogo T, Sawadogo LT, et al. Multidrug-resistant tuberculosis in Burkina Faso from 2006 to 2017: results of national surveys. Eur J Microbiol Immunol. (2019) 9:23–8. doi: 10.1556/1886.2018.00029
38. Horo K, Aka-Danguy É, Kouassi Boko A, N'gom AS, Gode CV, Ahui Brou JM, et al. Tuberculose multirésistante: à propos de 81 patients suivis dans un service de pneumologie en Côte d'Ivoire. Rev Pneumol Clin. (2011) 67:82–8. doi: 10.1016/j.pneumo.2010.06.002
39. Addo KK, Ampofo WK, Owusu R, Bonsu C, Nartey N, Mensah GI, et al. First nationwide survey of the prevalence of TB/HIV co-infection in Ghana. J Tuberc Res. (2018) 06:135–47. doi: 10.4236/jtr.2018.62013
40. Sylverken AA, Kwarteng A, Twumasi-Ankrah S, Owusu M, Arthur RA, Dumevi RM, et al. The burden of drug resistance tuberculosis in Ghana; results of the First National Survey. PLoS ONE. (2021) 16:e0252819. doi: 10.1371/journal.pone.0252819
41. Addo SO, Mensah GI, Mosi L, Abrahams AOD, Addo KK. Genetic diversity and drug resistance profiles of Mycobacterium tuberculosis complex isolates from patients with extrapulmonary tuberculosis in Ghana and their associated host immune responses. IJID Reg. (2022) 4:75–84. doi: 10.1016/j.ijregi.2022.06.005
42. Asare P, Asante-Poku A, Prah DA, Borrell S, Osei-Wusu S, Otchere ID, et al. Reduced transmission of Mycobacterium africanum compared to M. tuberculosis in urban West Africa. bioRxiv. (2017). doi: 10.1101/206136
43. Acquah SKE, Asare P, Osei-Wusu S, Morgan P, Afum T, Asandem DA, et al. Molecular epidemiology and drug susceptibility profiles of Mycobacterium tuberculosis complex isolates from Northern Ghana. Int J Infect Dis. (2021) 109:294–303. doi: 10.1016/j.ijid.2021.07.020
44. Ameke S, Asare P, Aboagye SY, Otchere ID, Osei-Wusu S, Yeboah-Manu D, et al. Molecular epidemiology of Mycobacterium tuberculosis complex in the Volta Region of Ghana. PLoS ONE. (2021) 16:e0238898. doi: 10.1371/journal.pone.0238898
45. Otchere ID, Morgan PA, Asare P, Osei-Wusu S, Aboagye SY, Yirenkyi SO, et al. Analysis of drug resistance among difficult-to-treat tuberculosis patients in Ghana identifies several pre-XDR TB cases. Front Microbiol. (2023) 13:1–9. doi: 10.3389/fmicb.2022.1069292
46. Forson A, Kwara A, Kudzawu S, Omari M, Otu J, Gehre F, et al. A cross-sectional study of tuberculosis drug resistance among previously treated patients in a tertiary hospital in Accra, Ghana: public health implications of standardized regimens. BMC Infect Dis. (2018) 18:1–6. doi: 10.1186/s12879-018-3053-5
47. Osei-Wusu S, Omari MA, Asante-Poku A, Otchere ID, Asare P, Forson A, et al. Second-line anti-tuberculosis drug resistance testing in Ghana identifies the first extensively drug-resistant tuberculosis case. Infect Drug Resist Volume. (2018) 11:239–46. doi: 10.2147/IDR.S152720
48. Ismail N, Ismail F, Omar SV, Blows L, Gardee Y, Koornhof H, et al. Drug resistant tuberculosis in Africa: current status, gaps and opportunities. Afr J Lab Med. (2018) 7:1–11. doi: 10.4102/ajlm.v7i2.781
49. Diallo A, Diallo BD, Camara LM, Kounoudji LAN, Bah B, N'Zabintawali F, et al. Different profiles of body mass index variation among patients with multidrug-resistant tuberculosis: a retrospective cohort study. BMC Infect Dis. (2020) 20:1–10. doi: 10.1186/s12879-020-05028-0
50. Carter BB, Zhang Y, Zou H, Zhang C, Zhang X, Sheng R, et al. Survival analysis of patients with tuberculosis and risk factors for multidrug-resistant tuberculosis in Monrovia, Liberia. PLoS ONE. (2021) 16:1–12. doi: 10.1371/journal.pone.0249474
51. López MG, Dogba JB, Torres-Puente M, Goig GA, Moreno-Molina M, Villamayor LM, et al. Tuberculosis in liberia: high multidrug-resistance burden, transmission and diversity modelled by multiple importation events. Microb Genom. (2020) 6:325. doi: 10.1099/mgen.0.000325
52. Diarra B, Goita D, Tounkara S, Sanogo M, Baya B, Togo ACG, et al. Tuberculosis drug resistance in < city>Bamako < /city>, Mali, from 2006 to 2014. BMC Infect Dis. (2016) 16:1–8. doi: 10.1186/s12879-016-2060-7
53. Diarra B, Toloba Y, Konate B, Sanogo M, Togo ACG, Camara F, et al. Extensively drug resistant tuberculosis in Mali: a case report. BMC Res Notes. (2017) 10:1–5. doi: 10.1186/s13104-017-2890-4
54. Oyedeji GJ, Adeyemo C, Dissou A, Abiodun T, Alli OAT, Onaolapo OJ, et al. Prevalence of multi-drug resistant tuberculosis among tuberculosis patients attending chest clinics in Osun-State, Nigeria. Curr Pharm Biotechnol. (2020) 21:939–47. doi: 10.2174/1389201021666200226100242
55. Onyedum CC, Alobu I, Ukwaja KN. Prevalence of drug-resistant tuberculosis in Nigeria: a systematic review and metaanalysis. PLoS ONE. (2017) 12:e0180996. doi: 10.1371/journal.pone.0180996
56. Muhammad Dayyab F, Iliyasu G, Garba Ahmad B, Aliyu Umar I, Musa Shuaib N, Bajehson M, et al. Emerging threat of drug-resistant tuberculosis and trends in the era of COVID-19: A descriptive study from northwestern Nigeria. J Clin Tuberc Other Mycobact Dis. (2022) 28:10–4. doi: 10.1016/j.jctube.2022.100319
57. Hamilton DO, Vas Nunes J, Grobusch MP. Improving the diagnostics of tuberculosis and drug resistance with Xpert MTB/RIF in a district general hospital in Sierra Leone: a quality improvement project. BMJ Open Qual. (2019) 8:478. doi: 10.1136/bmjoq-2018-000478
58. Koroma JA, Elduma AH. Factors associated with unfavorable treatment outcomes among multidrug-resistant tuberculosis patients, Sierra Leone : a cross-sectional secondary data analysis. Res Sq. (2023) 1:1–14. doi: 10.21203/rs.3.rs-3006102/v1
59. Dagnra AY, Mlaga KD, Adjoh K, Kadanga E, Disse K, Adekambi T. Prevalence of multidrug-resistant tuberculosis cases among HIV-positive and HIV-negative patients eligible for retreatment regimen in Togo using GeneXpert MTB/RIF. New Microbes New Infect. (2015) 8:24–7. doi: 10.1016/j.nmni.2015.09.001
60. Amini S, Hoffner S, Allahyar Torkaman MR, Hamzehloo G, Nasiri MJ, Salehi M, et al. Direct drug susceptibility testing of Mycobacterium tuberculosis using the proportional method: a multicenter study. J Glob Antimicrob Resist. (2019) 17:242–4. doi: 10.1016/j.jgar.2018.12.022
61. Yusoof KA, García JI, Schami A, Garcia-Vilanova A, Kelley HV, Wang SH, et al. Tuberculosis phenotypic and genotypic drug susceptibility testing and immunodiagnostics: a review. Front Immunol. (2022) 13:870768. doi: 10.3389/fimmu.2022.870768
62. Boehme CC, Nabeta P, Hillemann D, Nicol MP, Shenai S, Krapp F, et al. Rapid molecular detection of tuberculosis and rifampin resistance. N Engl J Med. (2010) 363:1005. doi: 10.1056/NEJMoa0907847
63. Miotto P, Cabibbe AM, Mantegani P, Borroni E, Fattorini L, Tortoli E, et al. GenoType MTBDRs/ performance on clinical samples with diverse genetic background. Eur Respir J. (2012) 40:690–8. doi: 10.1183/09031936.00164111
64. Owolabi OA, Jallow AO, Jallow M, Sowe G, Jallow R, Genekah MD, et al. Delay in the diagnosis of pulmonary tuberculosis in The Gambia, West Africa: a cross-sectional study. Int J Infect Dis. (2020) 101:102–6. doi: 10.1016/j.ijid.2020.09.029
65. UNITAID. Tuberculosis Diagnostics Technology Landscape. WHO Press (2017). p. 1–90. Available online at: https://unitaid.eu/assets/2017-Unitaid-TB-Diagnostics-Technology-Landscape.pdf (accessed January 06, 2024).
66. Diallo A, Combary A, Veronese V, Dahourou DL, Ouédraogo S, Traoré IT, et al. Delays in TB Diagnosis and treatment initiation in Burkina Faso during the COVID-19 pandemic. Trop Med Infect Dis. (2022) 7:237. doi: 10.3390/tropicalmed7090237
67. Marais BJ, Gie RP, Hesseling AC, Schaaf HS, Lombard C, Enarson DA, et al. A refined symptom-based approach to diagnose pulmonary tuberculosis in children. Pediatrics. (2006) 118:e1350–9. doi: 10.1542/peds.2006-0519
68. Yang TW, Park HO, Jang HN, Yang JH, Kim SH, Moon SH, et al. Side effects associated with the treatment of multidrug-resistant tuberculosis at a tuberculosis referral hospital in South Korea. Medicine. (2017) 96:e7482. doi: 10.1097/MD.0000000000007482
69. African Union. Africa Continental 2019 Africa Continental Tb Scorecard. Brazzaville: WHO Press (2019).
70. WHO. Global Tuberculosis Report 2020. (2020). Available online at: http://apps.who.int/bookorders (accessed September 3, 2021).
71. Nathanson E, Nunn P, Uplekar M, Floyd K, Jaramillo E, Lönnroth K, et al. MDR tuberculosis-critical steps for prevention and control. N Engl J Med. (2010) 363:1050–8. doi: 10.1056/NEJMra0908076
73. Atre SR, Jagtap JD, Faqih MI, Dumbare YK, Sawant TU, Ambike SL, et al. Tuberculosis pathways to care and transmission of multidrug resistance in India. Am J Respir Crit Care Med. (2022) 205:233–41. doi: 10.1164/rccm.202012-4333OC
74. Oladimeji O, Tsoka-Gwegweni JM, Adeyinka DA, Makola L, Mitonga KH, Udoh EE, et al. Knowledge, attitude and perception of tuberculosis management among tuberculosis-infected patients in resource constraint setting: field experience from Oyo state, South-West, Nigeria. Int J Community Med Public Heal. (2018) 5:1694–706. doi: 10.18203/2394-6040.ijcmph20181379
75. Kaaffah S, Kusuma IY, Renaldi FS, Pratiwi ADE, Bahar MA, Lestari YE. Knowledge, attitudes, and perceptions of tuberculosis in Indonesia: a multi-center cross-sectional study. Infect Drug Resist. (2023) 16:1787–800. doi: 10.2147/IDR.S404171
76. Asare P, Otchere ID, Bedeley E, Brites D, Loiseau C, Baddoo NA, et al. Whole genome sequencing and spatial analysis identifies recent tuberculosis transmission hotspots in Ghana. Front Med. (2020) 7:161. doi: 10.3389/fmed.2020.00161
77. Tadesse T, Demissie M, Berhane Y, Kebede Y, Abebe M. Long distance travelling and financial burdens discourage tuberculosis DOTs treatment initiation and compliance in Ethiopia: a qualitative study. BMC Public Health. (2013) 13:424. doi: 10.1186/1471-2458-13-424
78. Datiko DG, Jerene D, Suarez P. Patient and health system delay among TB patients in Ethiopia: nationwide mixed method cross-sectional study. BMC Public Health. (2020) 20:1–10. doi: 10.1186/s12889-020-08967-0
79. Makgopa S, Cele LP, Mokgatle MM. Pre-diagnosis health seeking behaviors and experiences post-diagnosis, among men diagnosed with tuberculosis in a district of Gauteng Metropolitan City, South Africa: in-depth interviews. Int J Environ Res Public Health. (2022) 19:1–13. doi: 10.3390/ijerph192013635
80. Ifebunandu NA, Ukwaja KN. Tuberculosis treatment default in a large tertiary care hospital in urban Nigeria: prevalence, trend, timing and predictors. J Infect Publ Heal. (2012) 5:340–5. doi: 10.1016/j.jiph.2012.06.002
81. Tesfahuneygn G, Medhin G, Legesse M. Adherence to Anti-tuberculosis treatment and treatment outcomes among tuberculosis patients in Alamata District, northeast Ethiopia. BMC Res Notes. (2015) 8:1–11. doi: 10.1186/s13104-015-1452-x
82. Ekwueme OEC, Omotowo BI, Agwuna KK. Strengthening contact tracing capacity of pulmonary tuberculosis patients in Enugu, southeast Nigeria: a targeted and focused health education intervention study. BMC Public Health. (2014) 14:1175. doi: 10.1186/1471-2458-14-1175
83. Gideon HP, Flynn JL. Latent tuberculosis: what the host “sees”? Immunol. Res. (2011) 50:202–12. doi: 10.1007/s12026-011-8229-7
84. Gong W, Wu X. Differential diagnosis of latent tuberculosis infection and active tuberculosis: a key to a successful tuberculosis control strategy. Front Microbiol. (2021) 12:745592. doi: 10.3389/fmicb.2021.745592
85. Teye JK. Migration in West Africa: an introduction. IMISCOE Res Ser. (2022) 3–17. doi: 10.1007/978-3-030-97322-3_1
87. Borrell S, Teo Y, Giardina F, Streicher EM, Klopper M, Feldmann J, et al. Epistasis between antibiotic resistance mutations drives the evolution of extensively drug-resistant tuberculosis. Evol Med public Heal. (2013) 2013:65–74. doi: 10.1093/emph/eot003
88. Wang L. Correlations between drug resistance of Beijing/W lineage clinical isolates of Mycobacterium tuberculosis and sublineages: a 2009–2013 prospective study in Xinjiang Province, China. Med Sci Monit. (2015) 21:1313–8. doi: 10.12659/MSM.892951
89. Yuan L, Huang Y, Mi LG, Li YX, Liu PZ, Zhang J, et al. There is no correlation between sublineages and drug resistance of Mycobacterium tuberculosis Beijing/W lineage clinical isolates in Xinjiang, China. Epidemiol Infect. (2015) 143:141–9. doi: 10.1017/S0950268814000582
90. Coscolla M, Gagneux S, Menardo F, Loiseau C, Ruiz-Rodriguez P, Borrell S, et al. Phylogenomics of Mycobacterium africanum reveals a new lineage and a complex evolutionary history. Microb Genom. (2021) 000477. doi: 10.1099/mgen.0.000477
91. Loiseau C, Windels EM, Gygli SM, Jugheli L, Maghradze N, Brites D, et al. The relative transmission fitness of multidrug-resistant Mycobacterium tuberculosis in a drug resistance hotspot. Nat Commun. (2023) 14:1988. doi: 10.1038/s41467-023-37719-y
92. Couvin D, Rastogi N. Tuberculosis - a global emergency: Tools and methods to monitor, understand, and control the epidemic with specific example of the Beijing lineage. Tuberculosis. (2015) 95:S177–89. doi: 10.1016/j.tube.2015.02.023
94. WHO. Global Tubercolosis Report 2018. (2018). Available online at: https://apps.who.int/iris/handle/10665/274453 (accessed January 10, 2024).
95. Marks SM, Flood J, Seaworth B, Hirsch-Moverman Y, Armstrong L, Mase S, et al. Treatment practices, outcomes, and costs of multidrug-resistant and extensively drug-resistant tuberculosis, United States, 2005-2007. Emerg Infect Dis. (2014) 20:812–21. doi: 10.3201/eid2005.131037
96. Adepoju A. Fostering free movement of persons in West Africa: achievements, constraints, and prospects for intraregional migration. Int Migr. (2002) 40:3–28. doi: 10.1111/1468-2435.00188
97. Turner MD, Schlecht E. Livestock mobility in sub-Saharan Africa: a critical review. Pastoralism. (2019) 9. doi: 10.1186/s13570-019-0150-z
98. Sanders R, Zachariah KC, Conde J. Migration in West Africa. New York, NY: Springer Publishing (2022).
99. Marks GB, Bai J, Stewart GJ, Simpson SE, Sullivan EA. Effectiveness of postmigration screening in controlling tuberculosis among refugees: a historical cohort study, 1984-1998. Am J Public Health. (2001) 91:1797–9. doi: 10.2105/AJPH.91.11.1797
100. Wandwalo E. Why Drug-Resistant Tuberculosis Poses a Major Risk to Global Health Security. Global Fund (2020). p. 26–8. Available online at: https://www.theglobalfund.org/en/blog/2020-11-19-why-drug-resistant-tuberculosis-poses-a-major-risk-to-global-health-security/ (accessed January 10, 2024).
101. Simbwa BN, Katamba A, Katana EB, Laker EAO, Nabatanzi S, Sendaula E, et al. The burden of drug resistant tuberculosis in a predominantly nomadic population in Uganda: a mixed methods study. BMC Infect Dis. (2021) 21:1–11. doi: 10.1186/s12879-021-06675-7
102. Udwadia ZF, Pinto LM, Uplekar MW. Tuberculosis management by private practitioners in Mumbai, India: has anything changed in two decades? PLoS ONE. (2010) 5:e0012023. doi: 10.1371/journal.pone.0012023
103. Farmer P. Call for New Approach to Combating TB Epidemic. (2012). Available online at: https://ghsm.hms.harvard.edu/news/keshavjee-farmer-call-new-approach-combating-tb-epidemic (accessed January 18, 2024).
104. The Global Fund. Best Practices and Lessons Learned in Implementing TB Innovative Approaches in West and Central African Countries. Geneva: Meet. Rep. (2021). p. 1–45.
105. TB-Alert. TB and Poverty. Global Tuberculosis Challenges (n.d.). Available online at: https://www.tbalert.org/about-tb/global-tb-challenges/tb-poverty/ (accessed October 7, 2023).
106. Barter DM, Agboola SO, Murray MB, Bärnighausen T. Tuberculosis and poverty: the contribution of patient costs in sub-Saharan Africa – a systematic review. BMC Public Health. (2012) 12:980. doi: 10.1186/1471-2458-12-980
107. Tanimura T, Jaramillo E, Weil D, Raviglione M, Lönnroth K. Financial burden for tuberculosis patients in low- and middle-income countries: a systematic review. Eur Respir J. (2014) 43:1763–75. doi: 10.1183/09031936.00193413
108. Global Fund. Approval of the Global Fund Strategy Narrative 46th Board Meeting. (2021). Available online at: https://www.theglobalfund.org/board-decisions/eb01-2021-dp3/ (accessed January 18, 2024).
109. Alene KA, Wangdi K, Clements ACA. Impact of the COVID-19 pandemic on tuberculosis control: an overview. Trop Med Infect Dis. (2020) 5:123. doi: 10.3390/tropicalmed5030123
110. Pai M, Kasaeva T, Swaminathan S. Covid-19's devastating effect on tuberculosis care — A path to recovery. N Engl J Med. (2022) 386:1490–3. doi: 10.1056/NEJMp2118145
111. Africa Development Bank Group. Strategy for Quality Health Infrastructure in Africa 2022–2030. Abidjan.
112. Ho J, Byrne AL, Linh NN, Jaramillo E, Fox GJ. Decentralized care for multidrug-resistant tuberculosis: a systematic review and meta-analysis. Bull World Health Organ. (2017) 95:584–93. doi: 10.2471/BLT.17.193375
113. Lyakurwa D, Lyimo J, Mulder C, Pelzer PT, Koppelaar I, Heus M. Assessment of training and mentoring for DR-TB care decentralization in Tanzania. Hum Resour Health. (2021) 19:56. doi: 10.1186/s12960-021-00600-4
114. Stop TB Partnership. Digital TB Surveillance System Assessment Report. (2022). Available online at: https://tbassessment.stoptb.org/ (accessed January 10, 2024).
116. Steingart KR, Schiller I, Horne DJ, Pai M, Boehme CC, Dendukuri N. Xpert® MTB/RIF assay for pulmonary tuberculosis and rifampicin resistance in adults. Cochrane Database Syst Rev. (2014) 2014:CD009593. doi: 10.1002/14651858.CD009593.pub3
117. Ochang EA, Udoh UA, Emanghe UE, Tiku GO, Offor JB, Odo M, et al. Evaluation of rifampicin resistance and 81-bp rifampicin resistant determinant region of rpoB gene mutations of Mycobacterium tuberculosis detected with XpertMTB/Rif in Cross River State, Nigeria. Int J Mycobacteriol. (2016) 5:S145–6. doi: 10.1016/j.ijmyco.2016.09.007
118. Nguyen TNA, Berre VA, Le, Bañuls AL, Nguyen TVA. Molecular diagnosis of drug-resistant tuberculosis; a literature review. Front Microbiol. (2019) 10:794. doi: 10.3389/fmicb.2019.00794
119. Naidoo K, Dookie N. Can the GeneXpert MTB/XDR deliver on the promise of expanded, near-patient tuberculosis drug-susceptibility testing? Lancet Infect Dis. (2022) 22:e121–7. doi: 10.1016/S1473-3099(21)00613-7
120. Igarashi Y, Chikamatsu K, Aono A, Yi L, Yamada H, Takaki A, et al. Laboratory evaluation of the AnyplexTM II MTB/MDR and MTB/XDR tests based on multiplex real-time PCR and melting-temperature analysis to identify Mycobacterium tuberculosis and drug resistance. Diagn Microbiol Infect Dis. (2017) 89:276–81. doi: 10.1016/j.diagmicrobio.2017.08.016
121. Ofori-Anyinam B, Kanuteh F, Agbla SC, Adetifa I, Okoi C, Dolganov G, et al. Impact of the Mycobaterium africanum West Africa 2 lineage on TB diagnostics in West Africa: decreased sensitivity of rapid identification tests in The Gambia. PLoS Negl Trop Dis. (2016) 10:e0004801. doi: 10.1371/journal.pntd.0004801
122. Otchere ID, Coscollá M, Sánchez-Busó L, Asante-Poku A, Brites D, Loiseau C, et al. Comparative genomics of Mycobacterium africanum Lineage 5 and Lineage 6 from Ghana suggests distinct ecological niches. Sci Rep. (2018) 8:11269. doi: 10.1038/s41598-018-29620-2
123. Velen K, Shingde RV, Ho J, Fox GJ. The effectiveness of contact investigation among contacts of tuberculosis patients: a systematic review and meta-analysis. Eur Respir J. (2021) 58:2100266. doi: 10.1183/13993003.00266-2021
124. Koura KG, Mbitikon OB, Fiogbé AA, Ouédraogo AR, Kuate Kuate A, Magassouba AS, et al. Programmatic implementation of contact investigation in eight African countries. Trop Med Infect Dis. (2023) 8:29. doi: 10.3390/tropicalmed8010029
125. Gehre F, Otu J, Deriemer K, Sessions PF, De Hibberd ML, Mulders W, et al. Deciphering the growth behaviour of Mycobacterium africanum. PLoS Negl Trop Dis. (2013) 7:e2220. doi: 10.1371/journal.pntd.0002220
126. Tientcheu LD, Sutherland JS, de Jong BC, Kampmann B, Jafali J, Adetifa IM, et al. Differences in T-cell responses between Mycobacterium tuberculosis and Mycobacterium africanum-infected patients. Eur J Immunol. (2014) 44:1387–98. doi: 10.1002/eji.201343956
127. Tientcheu LD, Haks MC, Agbla SC, Sutherland JS, Adetifa IM, Donkor S, et al. host immune responses differ between M. africanum- and M. tuberculosis-infected patients following standard anti-tuberculosis treatment. PLoS Negl Trop Dis. (2016) 10:e0004701. doi: 10.1371/journal.pntd.0004701
128. Asante-Poku A, Yeboah-Manu D, Otchere ID, Aboagye SY, Stucki D, Hattendorf J, et al. Mycobacterium africanum is associated with patient ethnicity in Ghana. PLoS Negl Trop Dis. (2015) 9:e3370. doi: 10.1371/journal.pntd.0003370
129. Winglee K, Manson McGuire A, Maiga M, Abeel T, Shea T, Desjardins CA, et al. whole genome sequencing of Mycobacterium africanum strains from mali provides insights into the mechanisms of geographic restriction. PLoS Negl Trop Dis. (2016) 10:e0004332. doi: 10.1371/journal.pntd.0004332
130. Osei-wusu S, Tetteh JKA, Musah AB, Ntiamoah DO, Arthur N, Adjei A, et al. Macrophage susceptibility to infection by Ghanaian Mycobacterium tuberculosis complex lineages 4 and 5 varies with self-reported ethnicity. Front Cell Infect Microbiol. (2023) 5:1163993. doi: 10.3389/fcimb.2023.1163993
131. Asante-poku A, Otchere ID, Danso E, Mensah DD. Europe PMC funders group evaluation of genotype MTBDR plus for rapid detection of drug resistant tuberculosis in Ghana. Int J Tuberc Lung Dis. (2015) 19:954–9. doi: 10.5588/ijtld.14.0864
132. Sanoussi CN, de Jong BC, Odoun M, Arekpa K, Ali Ligali M, Bodi O, et al. Low sensitivity of the MPT64 identification test to detect lineage 5 of the Mycobacterium tuberculosis complex. J Med Microbiol. (2018) 67:1718–27. doi: 10.1099/jmm.0.000846
133. Otchere ID, Afum T, Morgan P, Musah AB, Yaboagye S, Asare P, et al. Evaluation of a loop-mediated amplification test for rapid diagnosis of tuberculosis in Ghana. Heal Sci Investig J. (2022) 3:335–40.
134. de Jong BC, Hill PC, Aiken A, Awine T, Antonio M, Adetifa IM, et al. Progression to active tuberculosis, but not transmission, varies by Mycobacterium tuberculosis lineage in The Gambia. J Infect Dis. (2008) 198:1037–43. doi: 10.1086/591504
135. Asare P, Asante-Poku A, Prah DA, Borrell S, Osei-Wusu S, Otchere ID, et al. Reduced transmission of Mycobacterium africanum compared to Mycobacterium tuberculosis in urban West Africa. Int J Infect Dis. (2018) 73:30–42. doi: 10.1016/j.ijid.2018.05.014
136. Osei-Wusu S, Otchere ID, Morgan P, Musah AB, Siam IM, Asandem D, et al. Genotypic and phenotypic diversity of Mycobacterium tuberculosis complex genotypes prevalent in West Africa. PLoS ONE. (2021) 16:e0255433. doi: 10.1371/journal.pone.0255433
137. Diarra B, Kone M, Togo ACG, Sarro Y, dit S, Cisse AB, et al. Mycobacterium africanum (lineage 6) shows slower sputum smear conversion on tuberculosis treatment than Mycobacterium tuberculosis (lineage 4) in Bamako, Mali. PLoS ONE. (2018) 13:e0208603. doi: 10.1371/journal.pone.0208603
138. Asare P, Asante-Poku A, Osei-Wusu S, Otchere ID, Yeboah-manu D, Yeboah-manu D. The relevance of genomic epidemiology for control of tuberculosis in West Africa. Front Public Heal. (2021) 9:706651. doi: 10.3389/fpubh.2021.706651
139. Zumla AI, Gillespie SH, Hoelscher M, Philips PPJ, Cole ST, Abubakar I, et al. New antituberculosis drugs, regimens, and adjunct therapies: needs, advances, and future prospects. Lancet Infect Dis. (2014) 14:327–40. doi: 10.1016/S1473-3099(13)70328-1
140. Jang JG, Chung JH. Diagnosis and treatment of multidrug-resistant tuberculosis. Yeugnam Univ J Med. (2020) 37:277–85. doi: 10.12701/yujm.2020.00626
141. Nguta JM, Appiah-Opong R, Nyarko AK, Yeboah-Manu D, Addo PG, Otchere ID, et al. In vitro antimycobacterial activity and toxicity of eight medicinal plants against pathogenic and nonpathogenic mycobacterial strains. Int J Mycobacteriol. (2016) 5:6–7. doi: 10.1016/j.ijmyco.2016.05.004
142. Mutombo PN, Kasilo OMJ, James PB, Wardle J, Kunle O, Katerere D, et al. Experiences and challenges of African traditional medicine: lessons from COVID-19 pandemic. BMJ Glob Heal. (2023) 8:1–6. doi: 10.1136/bmjgh-2022-010813
143. Hoffman SJ, Mansoor Y, Natt N, Sritharan L, Belluz J, Caulfield T, et al. Celebrities' impact on health-related knowledge, attitudes, behaviors, and status outcomes: protocol for a systematic review, meta-analysis, and meta-regression analysis. Syst Rev. (2017) 6:1–13. doi: 10.1186/s13643-016-0395-1
144. Samal J, Dehury RK. Impact of a structured tuberculosis awareness strategy on the knowledge and behaviour of the families in a slum area in Chhattisgarh, India. J Clin Diagnostic Res. (2017) 11:LC11–5. doi: 10.7860/JCDR/2017/24107.9489
145. Huang GKL, Pawape G, Taune M, Hiasihri S, Ustero P, O'Brien DP, et al. Telemedicine in resource-limited settings to optimize care for multidrug-resistant tuberculosis. Front Public Heal. (2019) 7:222. doi: 10.3389/fpubh.2019.00222
146. Nwagu EN, Abugu LI, Yohanna W, Eze DN, Ononuju AH, Obayi AN. Behaviour change communication for control of tuberculosis by healthcare workers in dots facilities in Nigeria. Pan Afr Med J. (2020) 36:1–10. doi: 10.11604/pamj.2020.36.306.21640
148. Fana TE, Ijeoma E, Sotana L. Knowledge, attitudes, and prevention practices of drug resistant tuberculosis in the Eastern Cape Province, South Africa. Tuberc Res Treat. (2019) 2019:8978021. doi: 10.1155/2019/8978021
149. Idris NA, Zakaria R, Muhamad R, Husain NRN, Ishak A, Wan Mohammad WMZ. The effectiveness of tuberculosis education programme in kelantan, malaysia on knowledge, attitude, practice and stigma towards tuberculosis among adolescents. Malaysian J Med Sci. (2020) 27:102–14. doi: 10.21315/mjms2020.27.6.10
150. Huq KATME, Moriyama M, Krause D, Shirin H, Awoonor-Willaims JK, Rahman M, et al. Perceptions, attitudes, experiences and opinions of tuberculosis associated stigma: a qualitative study of the perspectives among the Bolgatanga municipality people of Ghana. Int J Environ Res Public Health. (2022) 19:1–19. doi: 10.3390/ijerph192214998
151. Ha DR, Haste NM, Gluckstein DP. The role of antibiotic stewardship in promoting appropriate antibiotic use. Am J Lifestyle Med. (2019) 13:376–83. doi: 10.1177/1559827617700824
152. Nahar P, Unicomb L, Lucas PJ, Uddin MR, Islam MA, Nizame FA, et al. What contributes to inappropriate antibiotic dispensing among qualified and unqualified healthcare providers in Bangladesh? A qualitative study. BMC Health Serv Res. (2020) 20:1–11. doi: 10.1186/s12913-020-05512-y
153. Stop TB Partnership. Advocacy, Cummunication, & Social Mobilisation (ACSM) for Tuberculosis Control. (2007). Available online at: http://medcontent.metapress.com/index/A65RM03P4874243N.pdf (accessed January 06, 2024).
Keywords: drug resistance, tuberculosis, West Africa, challenges, control measures, collaboration, WANETAM
Citation: Otchere ID, Asante-Poku A, Akpadja KF, Diallo AB, Sanou A, Asare P, Osei-Wusu S, Onyejepu N, Diarra B, Dagnra YA, Kehinde A, Antonio M and Yeboah-Manu D (2024) Opinion review of drug resistant tuberculosis in West Africa: tackling the challenges for effective control. Front. Public Health 12:1374703. doi: 10.3389/fpubh.2024.1374703
Received: 22 January 2024; Accepted: 29 April 2024;
Published: 16 May 2024.
Edited by:
Sydney Kabuswe Malama, University of Zambia, ZambiaReviewed by:
Eustachio Cuscianna, University of Bari Aldo Moro, ItalySanjay Gautam, International Vaccine Institute, Republic of Korea
Copyright © 2024 Otchere, Asante-Poku, Akpadja, Diallo, Sanou, Asare, Osei-Wusu, Onyejepu, Diarra, Dagnra, Kehinde, Antonio and Yeboah-Manu. This is an open-access article distributed under the terms of the Creative Commons Attribution License (CC BY). The use, distribution or reproduction in other forums is permitted, provided the original author(s) and the copyright owner(s) are credited and that the original publication in this journal is cited, in accordance with accepted academic practice. No use, distribution or reproduction is permitted which does not comply with these terms.
*Correspondence: Isaac Darko Otchere, iotchere@noguchi.ug.edu.gh; idotchere@mrc.gm
†These authors have contributed equally to this work and share senior as well as last authorships