- 1Fachbereich 1, Architektur, Bauingenieurwesen and Geomatik, Frankfurt University of Applied Sciences, Frankfurt am Main, Germany
- 2Curtin University Sustainability and Policy Institute (CUSP), Curtin University, Perth, WA, Australia
Companies globally have been trying to alter existing transportation systems to maximize public transport benefits, especially in the sector of high-speed (HS) ground transportation. The latest invention of such is Elon Musk's ultra-high-speed rail (UHSR), hyperloop. This technology enables a rail service to operate at a potential speed of 1,200 km/h. Hyperloop's ambitious speed goal has the potential to result in time-space shrinkage, which would then make distant cities more accessible with greatly reduced travel times. Since 2013, several companies have been vigorously promoting hyperloop development in various locations worldwide and competing to construct the world's first functional and commercially viable hyperloop. India, a country struggling with existing public transportation and urban mobility needs, agreed to construct a hyperloop through the company “Virgin Hyperloop One.” This paper first investigates the key technical, environmental, economic and human considerations in assessing the applicability of hyperloop to a particular location. It also considers in some detail the likely urban and regional planning and transport policy implications of the hyperloop technology based on the known effects of existing high-speed rail (HSR) systems. The paper concludes that many of the claims about hyperloop are subject to contrary information, meaning that great caution and prudence are currently needed regarding attempts at commercial deployment.
Introduction
Railways structured urban geographies for most of the 19th and 20th century. They brought prosperity and decline to towns depending on the location of the stations. By the end of the 20th century, the railway's impact on urban form waned considerably due to the proliferation of the automobile and air travel in many parts of the world (Sideris et al., 2012). Therefore, companies have been trying to alter existing public ground transportation systems to maximize public transportation benefits (Janić, 2018). At the beginning of the 21st century, the second railway revolution had emerged called high-speed rail (HSR), operational in numerous parts of the world, including Japan, western and northern Europe, Korea and China (Sideris et al., 2012). In 2013, the CEO of SpaceX, Elon Musk, proposed a concept of ultra-high-speed rail (UHSR) called hyperloop and open-sourced it (Rajendran and Harper, 2020). The technology significantly builds on a much older idea variously known as “gravity vacuum tube,” “gravity vacuum transit,” or “high-speed tube transportation,” which dates originally back to 1865 (Edwards, 1965; Özbek and Çodur, 2021).
Since 2013 several companies have been actively promoting hyperloop's development in various locations around the world (Ravenscroft, 2019). Hyperloop is the newest HS ground transport system currently in the early stage of testing. The concept works on electromagnetic attraction forces with travel pods operating in a very low-pressure environment with almost no air resistance or friction, thus enabling the theoretical speed of 1,200 km/h. The technology has an ambitious goal to result in a time-space shrinkage, which will increase the accessibility of cities through very low travel times over long distances (Musk, 2013; Rajendran and Harper, 2020). The main selling point used by Musk in his proposal for UHSR is cutting carbon emissions through very high energy efficiency and efficiently managing urban growth. UHSR claims to be superior to other HS transport in operational, economic, environmental and social performance (Janić, 2018). However, due to the hyperloop's very recent nature, its actual effects on cities and people are largely speculative. This paper therefore attempts to investigate the current state of knowledge about the technology and to identify some knowledge gaps in this new mode of transport.
The paper explores the urban and regional planning and transport policy implications of the hyperloop technology as well as its technical, economic, human and environmental dimensions through a wide-ranging literature review. It scrutinizes the validity of the promoters' claims concerning these factors. It discusses the possible development effects of a hyperloop line or network on cities at the regional, urban and station-area levels.
Due to HS ground transportation's relatively recent nature, many variables that influence urban change and spatial restructuring remain largely unmeasured (Sideris et al., 2012). Limitations regarding the availability of information restrict the study within a constrained framework of variables which attempt to cover the physical, technical, economic, environmental, human as well as possible urban planning and transport implications of hyperloop technology. The paper discusses the foundational technical aspects of the hyperloop, but not all the detail. Further, since the hyperloop is a new technology not operational anywhere globally, there are no bases to define its expected behavior; therefore, the paper assumes the hyperloop's expected impacts may be similar in character to that of conventional HSR.
The specific questions the paper seeks to answer are: What are the key technical, environmental, economic and human considerations in assessing the potential application of hyperloop technology in a location? What are its likely urban and regional planning and transport policy implications?
To answer these questions a review of the literature on hyperloop was undertaken to arrive at some consensus about what might be expected from this technology and where caution is due. In the absence of a commercially operational hyperloop, literature on the known impacts of existing HSR on urban and regional planning and transport policy is used to explore the likely effects of hyperloop on those factors.
Literature Review
The word hyperloop was used for the first time in Elon Musk's paper “Hyperloop Alpha” in 2013 (Musk, 2013). However, ultra-HS ground transportation was conceptualized over 100 years ago to achieve fast ground transportation and efficient inter-city travel, gradually evolving over time (Janzen, 2017). There have been various attempts to make this transportation system successful, with hyperloop being the newest invention. Various authors have criticized Musk's work. This review highlights the expected implications of a hyperloop by, and of necessity, drawing on HSR examples worldwide as proxies for hyperloop's possible future urban and regional development and transport policy impacts. It is thus useful at the outset to briefly compare some of hyperloop's claimed characteristics with that of existing and established forms of high speed rail travel, including magnetic levitation rail (maglev). The literature review is then divided into the technical, environmental, economic and human considerations and implications of hyperloop followed by its urban and regional planning and transport policy implications.
Hyperloop Compared to HSR and Maglev—A Brief Overview
To date, HSR, maglev and hyperloop are the only three forms of high speed ground transportation (van Goeverden et al., 2018). HSR and maglevs are commercialized and operate in different parts of the world, the earliest being Japan's Shinkansen line in 1964 between Tokyo and Osaka. Evidence shows that HSR and maglevs have improved the competitiveness of rail against other modes of transport through their dedicated guideways and very fast operating speeds. Additionally, they do not suffer the capacity constraints faced by road-based personal vehicles, they require less energy and produce a lower amount of carbon emission than both cars and planes (Janić, 2018).
By the end of 2011, 17,166 km of HSR was already operational globally (Garmendia et al., 2012). HSR generally connects population centers that are 100 km or more apart (Rodrigue, 2013). Maglevs are also commercially operational, but not at the same level as HSR (Janić, 2018). Currently, just six maglevs are operational in China, Japan, and South Korea and are the highest speed systems available (600 km/h). The three forms of HSR differ from one another partly based on their level of development and maturity (Ch2m, 2018). Table 1 provides a brief comparison between hyperloop, HSR and maglev technologies to show some of their current similarities and differences, which are then further developed and expanded.
The following sections describe in more detail the characteristics of hyperloop and where relevant, its comparison to existing forms of HSR.
Technical, Environmental, Economic and Human Considerations of Hyperloop
Criteria for the Selection of Hyperloop Corridor
For hyperloop to be successful, it requires certain pre-conditions to achieve its proposed benefits. Musk specified in his proposal that the hyperloop is an ideal solution only for specific cases (Musk, 2013). In their papers, Taylor et al. (2016) and WSDOT (2018) shortlisted six criteria expected to lead to a successful corridor selection for a hyperloop in any country—distance, size, transit connections, economic productivity, congestion and megaregion. These criteria are briefly explained below.
Megaregion, Distance and Size
The hyperloop should connect two important growing cities of the same megaregion. This will ensure selecting cities which are not too far apart. According to Taylor et al. (2016), with a potential speed of 1,200 km/h, the hyperloop will benefit cities that are at least 500–800 km apart. For trips shorter than 500 km, the net saving time over the automobile will be less due to the check-in procedure, security scanning, and time taken to access hyperloop terminals. Whereas, for station-to-station trips >800 km the cost-effectiveness advantage over aviation will gradually decline.
Economic Productivity, Transit Connection and Congestion
Cities lying at the end of the corridor should have high economic productivity. Also, their intercity connection should be one of the most important in the country and should consist of minimal interconnections with inefficient transportation modes. This will ensure a dense corridor that comprises a high passenger flow, ensuring the maximum potential utilization of the hyperloop benefits after becoming operational (Taylor et al., 2016; WSDOT, 2018).
Additionally, according to Walker (2018), for the hyperloop to operate at its full potential speed (1,200 km/h), the corridor must have flat landscapes. The hyperloop is also expected to be more successful in countries offering political and economic support as there are still uncertainties in its outcome and costs that probably need to be under-written by governments.
Corridor and Terminals
Hyperloop is a guided transportation mode with a specific infrastructure along the route it covers (Pérez, 2019). Therefore, locating the route close to any existing transport infrastructure can minimize the total amount of land required for the new hyperloop infrastructure. Land used to facilitate transport systems above the ground creates opportunity costs as the area cannot be used for other purposes. The hyperloop corridor would ideally be elevated on pillars 30 m apart to limit the land occupation on the ground. However, the narrow space of 30 m between each pillar may remain unutilized. The effective land occupation on the ground (net area) for a hyperloop system's line is estimated to be 0.5 ha/km (van Goeverden et al., 2018). Nevertheless, due to the speed at which hyperloop operates, it has lower flexibility, such that sharp turns are challenging to incorporate, unlike with metro systems or other rail. Therefore, to compensate, the system may use a much larger radius, increasing the required length of guideway, land area and overall cost (van Goeverden et al., 2018).
As indicated previously, hyperloops are currently not commercially operational anywhere, so conventional HSR must be used to speculate on its plausible land-use impacts. Available evidence shows a rapid change in the land use pattern due to the HSR. Zhang et al. (2019) determine the impact of HSR on urban land use in two aspects.
1. HSR stations and routes will cause a direct effect on land use by often consuming arable land. Zhang et al. (2019) analysis of land use transformation in China showed a negative effect on the absolute size of agricultural land in HSR cities due to urban sprawl caused by the HSR. Contrariwise, there was an increase in the amount of new agricultural land in non-HSR cities, offsetting the losses in HSR cities.
2. There will be an extended effect caused by the new transport system's impact on urban development because hyperloop may accelerate urbanization by acting as a catalyst. Various examples of this can be seen globally, such as in Madrid (Atocha Station) (Zhang et al., 2019), Amsterdam (Amsterdam Zuidas), Brussels (Midi) in Belgium and London (King's Cross St Pancreas) in England, where HSR has had a positive impact on land cover rates (Yin et al., 2015).
By extension, hyperloop may accelerate urban development, which might promote urban sprawl and changes in development strategies. It may also lead to the reconstruction of the urban hierarchy which could affect land use policy implementation (Zhang et al., 2019).
Hyperloop is like rail travel in its general appearance and how passengers use it for boarding, traveling and disembarking (Pérez, 2019). It would consist of terminals like at airports with dedicated stations for hyperloop. A HSR station's function is divided into two perspectives according to the node-place model (Zhang et al., 2019), which explains and analyses the station area using a conceptual framework (Yin et al., 2015). As with the node-place model of HSR, as a node, the hyperloop station is expected to connect the catchment area to the hyperloop network. On the contrary, as a place, the hyperloop station may be regarded as a new gateway to the city, increasing the catchment area's status and accelerating land-use changes. This may enable the city to relocate the gravity of the city centre, creating more centres or a polycentric city (Kenworthy, 2021). The hyperloop terminals may lie within the city centre or its outskirts (Zhang et al., 2019) and have different urban impacts depending on their location. Depending on the HSR station's location in a city, Hall (2009) explains three types of urban impacts.
Type 1: It may strengthen a place for commercial investment when the station is located at the city's commercial and business centre. Examples are Chamartín in Madrid, Kings Cross-St Pancras in London and Rotterdam central in the Netherlands.
Type 2: It develops complementary sub-centres for stations situated on the edge of a city. Examples are Kassel Wilhelmshöhe, Germany and Stratford in London.
Type 3: Hall (2009) states the last urban impact as that of developing a new base for the commercial purpose called the “edge city” when the station is located on the urban periphery. Examples are Amsterdam South, Netherlands and Shin Yokohama in Japan.
Type 1 may offer more significant potential for urban revitalization compared to the other two. However, the benefits suggested by existing HSR impacts depend on the current physical and economic circumstances. There are many challenges like competitive dynamics between the old and new station areas and integrating new development into the existing urban structure. Type 2 and 3 effects can spur redevelopment of underutilized areas, giving rise to more urban centres. In return, there will be a connection between more urban centres and nodes within the urban pattern and infrastructure network (Hall, 2009). Similar types of impacts are posited here to occur with hyperloop since there are no commercial systems in place by which to test this conclusively.
Travel Time, Capacity, and Efficiency
The hyperloop's primary value proposition is its energy-efficiency and station-to-station travel time, which would theoretically be faster than other competing modes, flights and transnational rail. However, in its current testing phase, the hyperloop has “only” achieved 400 km/h. Assuming its predicted speed, 1,200 km/h, journey time on hyperloop would be 10–15 times lower than that of conventional rail. This projected time saving has been gaining political and public support (Walker, 2018). Nevertheless, other factors that affect the journey time require consideration.
Transit Time in Terminals
The transit time would take the same as air travel, which would undermine the overall speed advantage of hyperloop, by increasing its overall journey time (Walker, 2018). This is because the hyperloop could be vulnerable to terrorist activity as it is a high-profile asset. To manage the safety risks, there is a requirement for terminal and security screening. The baggage handling process would require special handling staff who would store luggage in separate portions of the hyperloop, like flights (Taylor et al., 2016).
Additional Travel Time to Terminals
The additional time for travel to the terminal adds to the total journey time. Terminals within the city centre will be much easier to access due to their central location and existing access options such as other rail-based modes nearby. The terminals situated in the outskirts might be more of a challenge to access, especially if the terminals are not integrated with other public transport, especially metros or other forms of urban or regional rail (Walker, 2018).
Musk (2013) states that the checking-in process will impact the overall travel less than flights due to the higher frequency between pods' departures. The hyperloop will, ideally, run for 19 h and use 5 h for maintenance (Pérez, 2019). A single pod can hold up to 28 passengers and meet the demand of 840 passengers (off-peak) and 3,360 passengers during peak hours. Walker (2018) criticizes this and argues that this means the pods would have a headway of 30 s during peak hours (or 120 departures per hour), which is questionable due to safety reasons at such high speed. He argues that there should be a minimum gap of 80 s between the pods, allowing 45 departures per hour or 1,260 passengers per hour (only 37.5% of the theoretical capacity).
Other technical problems arise when detailed calculations are performed based on testing the claimed technical characteristics of hyperloop. For example, a 600-km tube would expand and contract by 300 m, taking the California desert with a 40C temperature change between day and night as the test case. This is highly problematic for a system that relies on close to a vacuum for operation (personal communication, Eric Bruun, 12.11.21). Additionally, Bruun calculates and concludes the following:
“If there are only two “tracks” where the vehicles cross over every second run, then minimum headway must be 2 min and capacity is 840–1,200 spaces per hour.” (personal communication, Eric Bruun, 12.11.21). He further concludes that a headway of 40 s is not possible even with a lower safety regime and that a minimum of 60 s headway with no additional margin of safety for braking and acceleration degradation, could result in a collision at +160 km/h speed. And finally, he concludes that even this would require a large terminal with 6 parallel loading tracks. With a realistic two-track terminal, the hyperloop capacity would replace about four wide-body planes per hour, but with no intermediate stops as a bonus, he asks, can billions of dollars in investment costs be justified?
Energy Consumption and Emissions of Greenhouse Gases
Musk's (2013) proposal is a low energy design. This is mainly due to its very low-pressure inner tube design, enabling it to have negligible air resistance and near zero friction due to magnetic levitation or “maglev” (van Goeverden et al., 2018). According to preliminary estimates, the hyperloop is expected to be 2 to 3 times more energy-efficient than its counterpart, HSR. Musk (2013) underlines that the technology will be self-sustaining and cost-effective due to a low energy demand system powered by solar energy. Solar panels mounted on top of the tube will provide the electrical energy which will propel the hyperloop. Based on the research claims, hyperloop may be able to generate more energy than required to operate. Its energy may be stored and utilized to operate the system in situations such as cloudy weather, at night or in tunnels (van Goeverden et al., 2018). However, other research findings suggest that the way to power the system might differ depending on system requirements and terrains (Walker, 2018).
Based on the above facts, more energy would be generated than required for operating the system in places where there is an abundance of sunlight, like Los Angeles and San Francisco or other high insolation locations such as the Middle East (Musk, 2013). Nevertheless, it is not possible to generate solar energy in every country due to climate or altitude limitations. This subverts the potential of the hyperloop's energy performance, so that it may be more efficient and cheaper to generate renewable energy such as solar, wind, water etc., on a large scale depending on region, altitude and climate, which could further be used to power hyperloop via the electrical grid (Walker, 2018; Pérez, 2019). Although the emissions of GHGs may be less during hyperloop operations, the indirect emissions may be high due to embodied energy, including constructing the hyperloop corridor, rolling stock and other equipment (van Goeverden et al., 2018).
Construction and Operational Costs
Flyvbjerg (2005, p. 18) states that it is not necessarily the best mega project that gets constructed “but those projects for which proponents best succeed in conjuring a fantasy world of underestimated costs, overestimated revenues, undervalued environmental impacts and overvalued regional development effects.” One of Musk (2013) proposal's key selling points is its low cost of construction and operation, which would be sourced from public funding (van Goeverden et al., 2018). The construction cost will, however, vary significantly depending on the geographical location, local conditions and engineering challenges (Walker, 2018). Construction in an urbanized area, through mountains or requirement of tunneling would be more expensive than in an empty area of flat sandy soil (van Goeverden et al., 2018). Musk's (2013) proposal shows the construction cost between Los Angeles and San Francisco at $10 million (USD) per km. However, other research companies and media show higher cost estimates of hyperloop (Mclean, 2016; Walker, 2018).
Virgin Hyperloop One (VHO) estimates the minimum cost of construction along the same route to be $75.6 million (USD) per km, or 7.5 times more (Walker, 2018). VHO estimated the route from Abu Dhabi to Dubai and Helsinki to Stockholm to be $32.5 million (USD) (Arabian Business, 2016) and $40 million (USD) per km, respectively (Upbin, 2016). However, Walker (2018) further argues that a more realistic value of construction cost would be an increase of 32.3% from the VHO calculations. According to that, the cost of constructing a hyperloop from Los Angeles to San Francisco would be a minimum of $100 million (USD) per km. Ninety-eight per cent of megaprojects constructed around the world suffer overrunning costs. Most rail projects have shown a cost overrun of 50% or higher according to Flyvbjerg (2005).
Table 2 shows an increase in the value of costs after adding Walker's (2018) assumed realistic value of 32.3% to the costs calculated by VHO. Further, the value increased more after adding the minimum per cent of overrunning costs (50%) for railways to Walker's (2018) calculations.
Table 2 shows a significant difference in costs from Musk's (2013) proposal compared to the cost with the addition of Flyvbjerg's (2005) minimum amount of overrunning capital costs. This suggests that Musk's and VHO's calculations are optimistic. Many project proponents believe low costs and high benefit forecasts fit politicians, stakeholders and the media, which allow the project to be realized by sheer momentum (Flyvbjerg, 2005; Zidane et al., 2013). One can ultimately only speculate on the actual real-life cost of this megaproject, which, are likely underestimated and therefore will probably cost significantly more than the minimum anticipated cost.
Contrary to Musk's (2013) findings, hyperloop may also not be self-sufficient in an operational cost sense, as not every corridor will produce more energy than utilized; therefore, the highest part of the operational costs (assuming it is driverless), may not be covered. Musk's proposal overlooked some main and mostly operating and maintenance costs that need to also be considered in a full commercial analysis: management planning, infrastructure inspection, insurance, license, infrastructure maintenance (ongoing investment cost), staff, station operating costs (e.g., station labor costs, utilities and water for restrooms, customer amenities, baggage handling, security, connection to other ground transportation, system control, and daily management) (Taylor et al., 2016; Walker, 2018). Since hyperloop has no commercial operation experience, these costs are more difficult to estimate (Pérez, 2019). Therefore, it is clear from the above that the hyperloop's operational costs are expected to be much higher than estimated. Indeed, at present, the only people who seem to benefit from any hyperloop profit include engineers, contractors, bankers, landowners, construction workers, lawyers and developers, as the benefits and affordability of such a technology are questionable for the general population, which is detailed in the following section.
Affordability, Equity and Impact on Lifestyle
According to Musk (2013), a round trip from Los Angeles to San Francisco on hyperloop would cost $40 (USD), which would be affordable to a large portion of the population. As per his findings, the operating and construction costs would be recovered in 20 years through patronage at this fare. However, based on the previous section's findings, such as a likely overestimated number of passengers during peak hour, underestimated construction costs, labor and maintenance, the fare of $40 (USD) appears unrealistic and understated. The costs are more likely to be similar to flights or higher (Walker, 2018). Therefore, the hyperloop might end up being more expensive than anticipated by its investors, resulting in high break-even fares (Nikitas et al., 2017; van Goeverden et al., 2018). That being the case, the hyperloop will be “more applicable for the premium passenger transport market” (Nikitas et al., 2017, p. 11). Besides this, politicians with a “monument” complex gain satisfaction, administrators get larger budgets and cities get investments that could otherwise be used to develop other cheaper public mobility infrastructures (Flyvbjerg, 2005). This can lead to the loss of “public goodwill” and political capital (Sideris et al., 2012). The resulting high break-even fares would be affordable only by the rich, which leads to more inequity in society. This would cause penalization of the poor, reproduce social policies that are austere and not address climate change (Monbiot, 2015). Therefore, a particular region's economic conditions and political context must be considered in whether such an investment is appropriate and necessary. More precisely, a less technically and economically demanding and appropriate alternative mode or modes of transport should be carefully considered before committing to hyperloop (Garmendia et al., 2012).
Social equity is an essential objective of any form of transportation and is a significant pillar of sustainable development (Caulfield et al., 2014) and therefore important to evaluate and assess before construction. Hyperloops will attract retail activity and raise the value of nearby property and rent (Zhang et al., 2019). Without necessary countervailing policies, this can produce the negative by-product of gentrification, resulting in displacement of poorer populations in favor of wealthier residents who move in to avail themselves of hyperloop's enhanced accessibility. The nature of the station areas may be transformed into higher income enclaves. Taking HSR station examples, this can be seen in the neighborhoods around Brussels Midi, while several disadvantaged ethnic minorities have been displaced in London's King's Cross neighborhood as they could not bid for space in the new development (Sideris et al., 2012). In developing nations, big megaprojects have underperformed in their social and environmental benefits (Flyvbjerg, 2005). Roy (1999, 2013) describes these impacts in her books The Cost of Living and The Algebra of Infinite Justice by demonstrating the violence and human suffering frequently involved in such megaprojects.
Safety and Comfort
The design proposed by Musk (2013) indicates hyperloop will be safer compared to other rival transport modes, such as airplanes and trains. However, most researchers have criticized this (Mclean, 2016; van Goeverden et al., 2018; Zhang et al., 2019). Hyperloop is a fully autonomous system consisting of windowless pods that run inside a thick steel tube with a diameter of 6.6 m (Mclean, 2016) built on pillars or pylons (Walker, 2018). The tube acts as a protective cover for the pods, which blocks any interaction with its immediate environment (van Goeverden et al., 2018). This will protect the pod from adverse weather conditions.
As discussed earlier, the small headway between the pods can cause safety concerns if a single pod fails. The kinetic energy generated at the speed of the hyperloop would be massive. It could generate similar impact to 75–200 kg of trinitrotoluene (TNT), an organic compound used as explosives, which can be life-threatening in case of accidents (Walker, 2018). The above safety concerns should be addressed through further elaboration and testing (van Goeverden et al., 2018).
It is unquestionable and self-evident that passenger comfort is essential for any transport mode (Oborne, 1978; Mclean, 2016). Hyperloop passengers are required to be seated during operation. The digital displays in the pods also have different options; they can control the position, temperature, power and color of the passenger chair (Dorsey, 2018). Hyperloop movement at ultra-HS, could cause discomfort and fear even with a straight path's slightest deviation (Nikitas et al., 2017). Huge curves would be required to prevent braking speed, so it does not cause motion sickness. The experience of acceleration and deceleration would be felt during the start and the end of the trip. The passengers may also feel vibration and jostling (Walker, 2018). The passenger's comfort is also dependent on seat comfort, lavatory access, disability access and entertainment service (Mclean, 2016). In this regard, being seated inside windowless pods may cause claustrophobia for the passengers. Companies want to solve this problem by developing digital “live” solutions which attempt to recreate window scenery (Walker, 2018). VHO, in their prototype, replaced the window area with displays loaded with personalized entertainment (Dorsey, 2018). Though it might make the passengers feel at ease, it will decrease the human connection with nature even further (Walker, 2018).
Environment
Musk's (2013) proposal promises a counteracting effect on the increasing automobiles and air travel by purportedly reducing roadway congestion, fuel consumption and GHG emissions. However, these goals are questionable on various grounds. If hyperloop does not capture adequate ridership from other modes of transport, its impact on the above variables will be minimal (Sideris et al., 2012). Further, land clearing, tunneling or demolition of historical buildings could be required to construct a hyperloop, which will impact the local physical environment (Mclean, 2016). Evidence shows that natural land is aggressively reduced and fragmented in HSR cities due to the socio-economic activities that follow its construction (Zhang et al., 2019). Hyperloop may have similar environmental impacts, indirectly harming surrounding agriculture and wildlife (Sideris et al., 2012). Therefore, it is essential to strengthen natural land management in hyperloop-connected cities (Zhang et al., 2019). Ideally, a hyperloop should only be proposed for corridors where it would cause minimal cutting of trees and demolition of historical buildings.
Where the hyperloop station areas are poorly integrated with other modes of public transport, they will attract more private vehicles giving rise to more parking demand. This is especially so when the stations are to be located on the outskirts, which often lack integration with other public transport connections as well as non-motorized modes (Sideris et al., 2012). According to van Goeverden et al. (2018), the vacuum pumps from the hyperloop may also cause noise. The elevated hyperloop corridor pillars, which are not properly embedded in the urban fabric, may cause visual intrusion. These factors may create a “barrier effect” for the people residing or working in adjacent communities (Sideris et al., 2012). It may cause annoyance and a harmful effect on these people, limiting the possible use of public space. Residents may even prefer to move to other neighborhoods away from the terminal and corridor. Regarding land use, this may lead to development of previous open space, involving problematic opportunity costs (van Goeverden et al., 2018). Therefore, the terminal areas must be planned and integrated well with public transport connections, as well as non-motorized modes.
Urban and Regional Planning and Transport Policy Implications of Hyperloop
Hyperloop Spatial Development Effects at the Regional Level
The high speed of hyperloop will allow it to improve intercity accessibility, as suggested by other operational HS ground transportation impacts (Janić, 2018). Through the hyperloop line, where there is an intermediate station every 500–800 km, passengers would be able to travel long distances for different purposes, such as work or tourism in very reduced time (Musk, 2013). This will change the absolute and relative accessibility of cities at the regional level (Chen et al., 2019). Therefore, long-distance traveling by hyperloop at high speed will bring distinct parts of one country together (Brunello, 2018).
Since hyperloop is a relatively new technology, the development experience of HSR, will be used to explain hyperloop's expected impact on urban development behavior. Various researchers have used modal and empirical-based approaches to investigate HSR's regional spatial development effects (Brotchie, 1991; Chen and Hall, 2011; Yin et al., 2015). They monitored the development change in HSR connected regions with the unconnected ones in Japan (Brotchie, 1991) and the United Kingdom (Chen and Hall, 2011). The result shows a clear relationship between cities connected to HSR and urban development patterns. The connected cities were influenced by HSR through the creation of new location advantages for individuals and firms in terms of private housing, offices and knowledge-intensive activities (Brotchie, 1991; Chen and Hall, 2011). In other words, the location factor created more opportunities and high-level commercial activities that attracted more people from faraway places (Yin et al., 2015). Hence, this transformed the spatial structure and expanded the market and labor potential, which in turn improved the connected city's overall performance in Japan and the United Kingdom (Brotchie, 1991; Chen and Hall, 2011).
HSR connected cities showed an economic advantage as transport hubs that improve the city's status and competitiveness (Pol, 2002; Chen and Hall, 2011; Chen et al., 2019). Improved status leads to economic spillover (Chen and Hall, 2011), which enhances spatial competition and causes an increase in land value, resulting in higher real estate rent and cost of living (Brotchie, 1991; Zhang et al., 2019). Through this, certain people will move to less expensive nearby unconnected cities triggering their growth. In return, this can attract back-office workers to these unconnected cities (Zhang et al., 2019). Conversely, the unconnected cities also experience several challenges, such as unemployment and reduced accessibility (Chen and Hall, 2011). Hence, there is an imbalance between cities connected to HSR and those that are disconnected (Pol, 2002).
Hyperloop is expected to have similar spatial development impacts along its corridor. Therefore, the new location advantage of cities connected to hyperloop result in considerable disparity with the non-connected ones (Zhang et al., 2019). Also, the unemployment in unconnected cities will accelerate migration to the connected ones, which would in turn restrain the development of peripheral cities (Hall, 2009). This tends to contradict Musk's (2013) statement that hyperloops will make cities less crowded as people will be able to travel further away for work. Rather, the previously mentioned impacts of hyperloop suggested by HSR experience, such as gentrification and unemployment, envisage a mixed picture. Hyperloop seems to facilitate both decentralization and concentration in hyperloop-connected cities.
Musk's proposal states that hyperloop should have a maximum of three or no intermediate stops to access the full potential of its speed (Musk, 2013). The trade-off between accessibility and speed risks concentrating services on profitable or major cities (Garmendia et al., 2012; Brunello, 2018). Intermediate cities bypassed in the vicinity of the hyperloop corridor will experience reduced service levels and limited access, which will reduce their ability to generate substantial traffic, causing a marginalization risk for these cities (Brunello, 2018). This can drain the economic activities of intermediate cities, causing an overall negative impact called the “backwash effect” (Berg and Pol, 1998), seen for example in Nagoya, Japan along the Tokyo-Osaka HSR line (Kamada, 1980). In this process, core cities are drawn closer, while distant places tend to get more remote. Hall (2009) refers to this as “peripheralization of the periphery.” These factors are most visible at the regional level. This is because different parts of the same region experiencing various levels of accessibility are measured, thus exacerbating their relations (Brunello, 2018). However, according to Chen and Hall (2011), these challenges can be avoided by integrating unconnected cities with connected ones through other modes of transport, such as metro or other conventional rail systems. This will improve inter-regional and intra-regional accessibility.
It is clear from the above evidence, that hyperloop can reconstruct the political and regional economic structure by redistributing socio-economic activities (Zhang et al., 2019). However, it is essential to know if the development opportunity would differ based on the type of city connected to the hyperloop, again drawing on HSR experiences. This can be summarized as follow.
Major Cities (First-Tier Cities, International Service Cities)
First-tier cities are already dominant cities; connection to HSR will enlarge their area of influence and they would tend to become even more competitive (Cervero and Bernick, 1996; Moyano et al., 2019).
Intermediate Cities (Second and Third-Tier Cities, Cities in Transition)
According to Cervero and Bernick (1996), intermediate cities connected to HSR have a more significant development impact than the major cities. Second tier and third-tier cities can overcome isolation, improve their location advantages and attract business activities. They will also significantly attract and increase tourism (Zhang et al., 2019).
Pol (2002) and Chen et al. (2019) agree and state that HSR improved the primacy for “international service cities” and visibility of “cities in transition” (Sideris et al., 2012). Hence, cities show a generative economic growth. However, Hall (2009, p. 68) argues that HSR “will be the maker of some cities but the breaker of others.” The catalyzing or facilitating role of cities due to connection to HSR brings changes that lead to improving their position in the urban hierarchy. However, this could also lead to unwanted side effects for cities already existing higher in the national and continental hierarchy, such as loss of activities. Instead, this causes the redistribution of the economy. Therefore, cities need to meet preconditions for economic growth and renewal to achieve positive economic growth (Pol, 2002).
As suggested by HSR, the development opportunity and growth associated with hyperloop are expected to differ similarly based on the type of city, major or intermediate, connected to the hyperloop. Hyperloop-connected major cities may become more competitive, whereas intermediate cities may attract more tourists. However, this catalyzing role of these cities connected due to hyperloop will redistribute the economic activity of regions.
To examine the expected regional structural effects of hyperloop it is important to take the results of before and after development effects of cities connected to HSR to provide the anticipated hyperloop behavior. Garmendia et al. (2008) and Preston and Wall (2008) have compared this in Ciudad in Spain and Ashford in England. Their findings show that HSR can aid isolated, intermediate cities to attract housing investment from within the region. Post-HSR connection, both cities increased their spatial polarizing capacity and increased their population. HSR facilitated the development of small cities as specialized sub-centers of metropolitan areas with high-level office development. That way, people do not need to commute to the central or inner areas of the metropolis (Yin et al., 2015). Hyperloop can most likely be expected to facilitate similar development changes as HSR.
Indirect Effects of Hyperloop at the Urban Level
Hyperloop terminals and urban development should reinforce each other. Their terminals must be developed in synergy with urban dynamics with “cities in transition” and “international service cities.” This section discusses the development of hyperloop in synergy with urban dynamics, using HSR impacts as a general guide to expected hyperloop impacts.
Muller (2004) findings show that London's and Paris's urban development patterns were more noticeable after the construction of their metro systems. The connection between the city centre and its periphery improved. Hence, transport infrastructure and urban development are largely path dependent. Similarly, the urban development patterns in hyperloop-connected cities will help determine the conditions for its performance, good or bad. The synergy between HSR and urban dynamics is shown below in Lille, France and Amsterdam, The Netherlands.
HSR Attracted by Declining Regions
The city of Lille was an industrial centre that also included a university and a traditional historical centre. In the 1960s, due to the industrial downturn, there was high unemployment and an increasing gap between social and spatial segregation. When the plan for HSR was drawn up, the rail operators were skeptical of using Lille as an intermediate stop between Paris and Brussels due to the expenses, potential financial loss, less direct route and loss of time. However, the construction of HSR in the centre of Lille proved to be successful. The station was constructed in a manner complementing the existing centre to maintain balance and avoid competition. It led to the revitalization of the city centre, which improved its economy. The station gained new facilities, attracted offices, commercial areas, cultural establishments, public facilities, housing and public spaces (Bertolini, 2000). Therefore, HSR benefited from Lille's economy by reinforcing Lille's regional economic activities and its commercial centre.
HSR Attracted by Active, Lively Stations
In 1990, Amsterdam experienced an increase in population growth due to an increase in the global economy. The growth led to the requirement of new economic spaces. Therefore, banks, business and residential developments started to move to the edge of the city (Salet and Majoor, 2005). Due to these changes, Amsterdam Zuidas (the southern part of Amsterdam), became the new focal point. In 1997, plans were drawn to construct a HSR station at Zuidas. However, the operators feared that connecting Zuidas would elevate its area in the urban hierarchy and create an imbalance with the rest of the city (Bruinsma, 2009). After Amsterdam Zuidas' connection to HSR, it encouraged balanced development throughout the city. People saw the need to change the monocentric structure of the city and adopt the multi-nuclei (polycentric) model, which led to high quality, mixed-use development throughout the city (Salet and Majoor, 2005).
As seen from the above, these two cities achieved synergy between HSR and their own urban dynamics and witnessed a positive development impact. Similarly, hyperloop connected cities, with and without advantages, may reinforce urban development and growth on an urban level. However, there are various challenges involved.
Hyperloop may cause rapid urbanization that will accelerate the rate of urban expansion and decrease cultivated land (Zhang et al., 2019). According to Brenner (1999), a new transport system is a significant driver in influencing urban form. Evidence of other operational modes of HS ground transportation shows the rapid change in land use patterns due to station development or redevelopment. Studies also show a connection between the land value of housing property and railway infrastructure. For example, the areas within a walking distance of 10 min to the station (about 800 meters) have distinctly higher land value due to the attractiveness of retail activity in the area which in turn is driven by the improved mostly pedestrian-based “movement economy.” On the other hand, certain factors can bring down value, such as noise and crime effects often associated with railway stations. However, the impact of the first may be more significant than the latter (Yin et al., 2015; Zhang et al., 2019). An example of this can be seen in the Frankfurt central station area, Germany (Möbert, 2018; FAZ, 2020). Flyvbjerg (2012) agrees and further states that urban megaprojects are usually poorly integrated with the rest of the city's planning. Only certain parts of the city, mainly the areas around the station, receive special planning. Therefore, the build-out of space by hyperloop will not be uniform throughout the city. It will create an imbalance between cities and their hinterlands. The serviced areas will improve their status, modernity and value (Chen et al., 2019). The result will tend to be gentrification around the station area, causing displacement of lower income populations and an influx of higher income people (Sideris et al., 2012). Therefore, hyperloop may facilitate polarization and segregation between station areas and other parts of the city. The differential increase in accessibility accompanied by hyperloop may lead to spatial inequality and centralization (Chen et al., 2019).
According to Musk (2013), hyperloop will mitigate the relocation of household and economic activities from moving outwards within a city, i.e., less urban sprawl. However, as suggested above, hyperloop might cause gentrification which may further encourage sprawl, since many people may move toward the periphery where land is more affordable compared to station areas. Further, the potentially rapid and extensive development in cities due to hyperloop, may cause various challenges, termed broadly as the “urban disease.” These include weakly built physical environments, loss of urban and environmental quality and traffic congestion (Sideris et al., 2012).
Micro Effects of Stations on the Urban Development Patterns of Adjusting Neighborhoods
HS ground transportation contributes to the identity of the surrounding area. Their stations' functions are like that of a traditional railway station area (Yin et al., 2015). Zhong et al. (2014) defines the station area as the development perimeter and the zone of influence based on accessibility levels. It is vital for a city and its inhabitants to link the station's catchment area with the transport network (Zemp et al., 2011). The catchment area for a HSR terminal is much larger than that of traditional rail stations. Its catchment area falls in the radius range of 5–40 km, depending on the feeder system (Zhong et al., 2014). As various researchers have used HS ground transportation's catchment area as the minimum of 5 km (Murakami and Cervero, 2010; Yin et al., 2015), this research assumes the same. The hyperloop can be reached within 15 min by using typical complementary transport modes such as trams, metro, suburban rail and buses with an average speed of 20 km/h, a typical urban bus speed average speed (Kenworthy and Laube, 2001). However, the hyperloop station's accessibility in the immediate station area catchment of 5 km and distances higher than 5 km would generate different development effects in these areas. As mentioned earlier, there are no operational examples of hyperloop to define these differences clearly. Therefore, HSR is used to suggest these differences. Yin et al. (2015) divided the area around HSR stations into three zones, primary, secondary and tertiary development zones (Table 3).
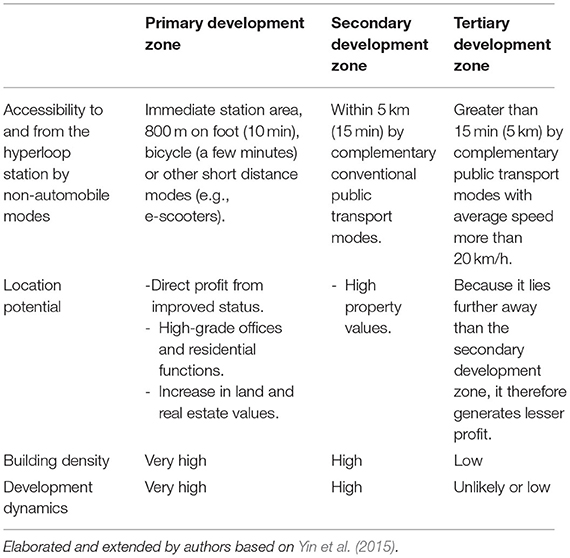
Table 3. Likely development effects of a hyperloop station based on the respective development zones.
The primary zone witnesses the most significant number and magnitude of effects due to direct contact with the station area. This zone consists of the highest land prices, high-grade offices and residential functions because of its proximity to the station, which improves its status, leading to high levels of dense development. The secondary zone is reachable within 15 min from the station via complementary conventional modes of public transport such as metro, tram, suburban rail, BRT or in many cases urban buses, provided they can operate at an average speed of 20 km/h or more. The development, density of buildings and land value are still high but less compared to the immediate station area. The tertiary zone is less likely to see development due to its furthest proximity to the station (Yin et al., 2015). As suggested by the above HSR impacts, the hyperloop terminal is expected to induce similar development patterns.
Certain preconditions are essential and will influence the intensity of development in the zones mentioned above, such as strength of the regional economy, the existing image of the city, integration of the HS transport mode with other modes of transport, location of the station, mixed-use development in the station area and balanced embedding of the new transportation in the existing urban fabric (Jong, 2007; Walker, 2018). Good car accessibility in more peripheral locations would also be influential in certain circumstances, but generally in denser or more central locations, non-automobile modes are preferred due to their spatial efficiency (cars require high road and parking capacity near stations) and their ability to deliver large number of potential patrons.
HS ground transportation's effects on long-term spatial development are complex processes, which are rarely isolated and less quantifiable. The development effects include the revitalization and new development of neighborhoods around the station area. Examples can be seen in Amsterdam Zuidas in the Netherlands or Lisbon East in Portugal. These station areas showed an improvement and increase in trade, socio-cultural facilities, new residences, creation of public spaces and the quality of the urban districts through green areas (Peters, 2009; Vale, 2015).
Another positive effect is the regeneration and revitalization of formerly derelict areas. An example of such an area is the mall at the HSR station in Euralille, Lille (France). HSR might also aid in defining new city cores and commercial centres, as can be seen at Shin-Osaka in Japan. Some cases of HSR also showed the redevelopment of formerly brownfield sites and railway properties such as Stratford in England. Another visible effect is the combination of convention centres, retail and entertainment facilities in new architectural landmarks and new, attractive and significant buildings such as in Berlin, Germany and Lille, France (Sideris et al., 2012).
It can be concluded that hyperloop may act as a catalyst for new commercial and residential development and facilitate urban regeneration. This would generally involve enormous economic spinoffs. However, these impacts differ depending on context and circumstances. Specific operational modes of HS ground transportation experiences in the past have also shown an opposite impact. For example, Berlin post-HSR showed no signs of new development or redevelopment around its station area. Berlin is Germany's poorest large city with the lowest GDP per capita (Kenworthy, 2013). Other similar examples are Tours station in France, Ashford station in England and the Ebbsfleet International HSR station, also in England. These stations have done little to regenerate the areas around them, which so far mainly witness the building of park-and-ride facilities (Hall, 2009).
In effect, station-adjacent neighborhoods of hyperloop may or may not act as catalysts for desirable patterns of development and growth, as suggested by the diversity of HSR impacts. Therefore, hyperloop may have varied impacts on the local context. However, it is mostly generative, as suggested by most HSR experiences. Any hyperloop service and its associated terminals may cause an increase in government subsidies and opportunity costs because of forgone other services caused by the enormous likely expenditure for hyperloop. The loss of public goodwill then becomes a potential problem and the political cost of foregoing the development of other essential infrastructure, which may be more beneficial to a particular area, is a problem for everyone concerned (Sideris et al., 2012). The hyperloop station area would be expected to have a high population density. A high population or dense area, however, usually confronts institutional barriers in its creation, which can sometimes thwart its fruition (Taylor et al., 2016). There is a risk of urban fragmentation of the station area through developing into a separate “island” in terms of spatial and functional development, ownership, control, scale and architecture (Trip, 2004; Sideris et al., 2012). The urban planning and urban design of the station should be done carefully and with maximum public participation to complement its surroundings and mitigate these unfavorable conditions.
Integration Between Hyperloop and Urban Transport Networks
Different modes of transport are elements that contribute to the making of the entire transport system in an urban area. Each element must contribute to bringing its passengers within the access point of other elements for most trips (Yin et al., 2015). The interaction potentials between urban nodes in the transportation network are measured using accessibility, accessibility depending essentially on the proximity between nodes, their relative size or attraction potential and the quality of the transport connections between those nodes, which results in greater or lesser travel “friction” (Black, 1981). Hence, accessibility is of critical importance to the transport network (Yang et al., 2018). Secure integration of hyperloop with other modes of transport will prove to be crucial to its overall performance in terms of its system, passengers and the region served by its lines. This integration will enable hyperloop lines to link with regional services, which could then operate in harmony with the hyperloop through connections and matching timetable. This should ideally avoid disorderly competition, encourage a sensible distribution of occupancy, and stimulate cooperation among operators. More passengers would be able to reach hyperloop stations, increasing its spatial penetration (Yin et al., 2015) while simultaneously enhancing use of sustainable modes of transport connecting to it.
The hyperloop should ideally involve a seamless physical integration between the connection modes and the transfer station (Sideris et al., 2012), the aim of a connector being to reduce “transfer resistance” (Yin et al., 2015). HS ground transportation relies on integration with rail and bus (and non-motorized modes for proximity to stations) to provide a door-to-door service (Berg and Pol, 1998), using timetable matching and integrated ticketing (Hall, 2009), which will also aid in reducing “transfer resistance." On the other hand, weak integration will cause low passenger volume, which indirectly works in favor of cars and in the long term, lowers the development impact on the stations' primary and secondary development zones. This causes delays and connection disruption during rush hours and affects the area's development potential (Yin et al., 2015).
Evidence also shows HS ground transportation location poses challenges for other modes. According to Walker (2018), locating the hyperloop station in the city center would prove to be more beneficial. This is because hyperloops may be able to provide an opportunity to use the already available infrastructure (metro or other rail) to help develop their stations or terminals (Sideris et al., 2012). This would save terminal costs, travel and waiting time of passengers and ease of integration with other modes of transport (Taylor et al., 2016). For instance, Taiwan started constructing HSR in 2000, which was operational from 2007 (Kao and Lin, 2007). The construction of HSR stations in Taiwan is mostly on the outskirts of urban areas with no integration of public transport, leading to poor performance due to a decline in passenger requirements and timetable matching. Although the government aimed to integrate public transport with the existing HSR stations and strengthen it, it was not easy to achieve (Cheng, 2010) due to technical issues, operational challenges, civil engineering and financial challenges (Sideris et al., 2012). On the other hand, the HSR in Japan was successful due to integration, reinforcement and improvement of public transport services with it, such as narrow-gauge rail lines, bus and ferry (Okabe, 1980).
Locating HS ground transportation in the city centre unfortunately also poses a few challenges. It will have higher costs associated with land acquisition than peripheral locations (Taylor et al., 2016). It might also be a challenge to create additional capacity for a hyperloop station in the already existing transport system located in centres. In these situations, underground construction may be a solution. However, governments tend to rule this out due to high costs (Yin et al., 2015). Therefore, locating a hyperloop station on the periphery is cheaper in construction and land costs but also characterized by poor or low public transport accessibility, and more expensive, though easier car accessibility. City centre locations would prove to be more beneficial through careful planning to avoid the earlier mentioned challenges.
Even though hyperloop would compete with air travel, integration of it with airports will provide a complementary service (Walker, 2018). It could provide fast, easy access to airports and enlarge its service area in terms of intercontinental flights. HSR experience of this has been witnessed by Lyon-Satolas airport in France. There was a massive decline in the volume of passengers initially at the airport after the HSR began in 1981. However, after the construction of the HSR terminal at the existing airport there was improved accessibility to an enlarged radius of 400 km. This, in return, encouraged intercontinental flights. By 1996, Lyon-Satolas surpassed its international traffic mark of 50% (Yin et al., 2015). In the current situation of potentially devastating global climate change, however, increased air travel must also be seriously questioned because of its huge CO2 emissions and other impacts in the upper atmosphere.
In conclusion to the integration of hyperloop with other transport networks, companies envisage, strong planning of inter-modality. To meet the demand of passengers' needs and transfers, any hyperloop station should provide secure, good access and consistent punctual departures and arrivals through reasonable timetables and suitable places for boarding, disembarking and waiting. The stations should also provide excellent spatial orientation and design for passengers to change between modes. Passengers also require information and related services such as ticketing in waiting areas and beyond (Zemp et al., 2011). A great deal has been posited in this section about the abilities of the hyperloop. In the case of achievement, the hard evidence of success or the best way to proceed is much less clear. Hyperloop proposals need to address issues specific to it and from the experience of other HS ground transportation. Real-world demonstrations would be required to overcome the potential issues related to safety, economics, and passenger comfort (Walker, 2018).
Conclusions
This paper set out to answer two key questions: What are the key technical, environmental, economic and human considerations in assessing the potential application of hyperloop technology in a location? What are its likely urban and regional planning and transport policy implications? Musk's ideal hyperloop is said to reach ultra-high speeds of around 1,200 km/h, though evidence in the literature suggests more likely speeds of around 500 km/h due to the practical realities of engineering in different locations coupled with limits of passenger comfort. It will have a relatively low carrying capacity per departure due to each pod having only 28 seated passengers. Theoretical peak hour capacity of 3,360 passengers depends on 30 s headways which, for safety reasons, may need to be 80 s, reducing peak capacity to 1,260 passengers. Furthermore, evidence suggests that companies have underestimated the costs involved with the system. These and other technical, environmental, and human considerations were questioned throughout the paper and for the most part, the positive claims for hyperloop are disputed or at least suggested to be overstated.
Such problems could lead to loss of public goodwill and political legitimacy on the part of governments seeking to install hyperloop without a very thoroughgoing investigation of its implications for specific corridors and station locations. A key problem appears to be that it will likely only be affordable for travel by higher income populations which can add to an already large societal gap and accelerate further inequity. In human terms, traveling at an ultra-high speed with no windows in the pods detracts from hyperloop's comfort level and acceptability for people, notwithstanding any efforts at virtual entertainment. Additionally, hyperloop's terminals may attract parking lots, visual and noise pollution if not carefully planned to maximize interconnections via conventional public transport, bicycles and walking and with genuine public participation.
Based on diverse experience with conventional HSR, hyperloop may cause barrier effects and limit the use of urban space for communities, in particular green space, if the possible densification and land use change impulses from land value/real estate price increases around terminals are not properly planned with the broader community good in mind. There are also potential impacts on near-city and within-city agriculture, which are discussed in the paper and need to be considered, especially in rapidly industrializing cities where agriculture and other food production in urban hinterlands is still very important. Broadly speaking, the available evidence would tend to suggest that there are more sustainable approaches to regional and urban mobility that need to be thoroughly investigated and comprehensively compared to hyperloop, in environmental, economic and social terms, before decisions are taken to seriously consider hyperloop.
This is especially true when considering the potential urban and regional planning and transport policy implications, which are very diverse, as elaborated in detail in the paper. On a regional, urban and micro level, it is anticipated that hyperloop provides the possibility of broad transformations very like HSR experiences around the world, some of which have been good, some bad and some cases where there has been little effect, depending on the broader economic, political and planning circumstances. Specific cases are explained in the paper. For example, like HSR, hyperloop may bring distinct parts of the city together, yet the imbalance that could be generated between hyperloop-connected and unconnected cities is a critical finding to be examined by authorities and the operating companies. Musk's statement that the hyperloop will make cities less crowded is a double-edged sword. This claim could be true regarding certain aspects while untrue for others, as it would likely facilitate both decentralization and concentration at the same time.
In the likely event that hyperloop would be financed through government subsidies, just like most HSR-projects, opportunity costs are almost certain to occur. This will very likely result in the absorption of capital funds essential for other critical transport infrastructure projects such as BRT, metro or better walking and cycling facilities. This makes it essential to perform a comprehensive comparative analysis of the benefits vs. costs of investment in hyperloop vs. other crucial infrastructure needs in any specific area or transport corridor. Therefore, a wide range of highly skilled and specialized people are required to keep the issues mentioned in this paper in close consideration to legitimately and confidently assess, in any given situation, this so far real-world unproven technology.
Based on the findings of this paper, we conclude that there exist many positive claims about hyperloop, mainly by its proponents, but for each one of these claims there are counter-claims, mainly by experts in the rail field, which cast severe doubt on the viability of hyperloop both as a technology and as a tool in helping to reshape urban regions to reduce the time and space separation between cities. The review of existing literature in this paper has attempted to systematically bring together both sides of the story and to compare the claims of each. Based on this approach, we conclude that the counter-messaging about hyperloop is convincing and therefore warrants a high degree of caution in considering it for deployment anywhere.
The other clear message is that it would be foolhardy for any government to commit to building a commercial hyperloop line or system without much more thorough testing of prototype systems in different conditions. Accordingly, the development and construction costs of any hyperloop in the foreseeable future should be borne to the largest degree by the proponents of the system, not by the governments to whom hyperloop is being marketed. Over-commitment to hyperloop now by any government, especially financially, would not be prudent.
Data Availability Statement
The original contributions presented in the study are included in the article/supplementary material, further inquiries can be directed to the corresponding author/s.
Author Contributions
SP and JK were involved in the writing of the original manuscript and its continual review and improvement. Both authors contributed to the article and approved the submitted version.
Conflict of Interest
The authors declare that the research was conducted in the absence of any commercial or financial relationships that could be construed as a potential conflict of interest.
Publisher's Note
All claims expressed in this article are solely those of the authors and do not necessarily represent those of their affiliated organizations, or those of the publisher, the editors and the reviewers. Any product that may be evaluated in this article, or claim that may be made by its manufacturer, is not guaranteed or endorsed by the publisher.
References
Arabian Business, (2016). Abu Dhabi-Dubai Hyperloop Route Said to Cost $4.8bn. Available online at: https://www.arabianbusiness.com/abu-dhabi-dubai-hyperloop-route-said-cost-4-8bn-650603.html (accessed January 7, 2020).
Berg, L., and Pol, P. (1998). The European High-Speed Train and Urban Development: Experience in fourteen European and Urban Regions. Ashgate: Aldershot.
Bertolini, L. (2000). Planning in the borderless city: A conceptualization and an application to the case of station area redevelopment. Town Plan. Rev. 71, 455–475. doi: 10.3828/tpr.71.4.2883q121q1671185
Black, J. (1981). Urban Transport Planning: Theory and Practice. London: Routledge Library Editions.
Brenner, N. (1999). Globalization as reterritorialisation: The re-scaling of urban governance in the European Union. Urban Stud. 36, 431–451. doi: 10.1080/0042098993466
Brotchie, J. (1991). “Fast rail networks and socio-economic impacts,” in Cities of the 21st Century: New Technologies and Spatial Systems, eds J. Brotchie, M. Batty, P. Hall, and P. Newton (Longman: Cheshire).
Bruinsma, F. (2009). The impact of railway station development on urban dynamics: a review of the Amsterdam south axis project. Built Environ. 35, 107–121. doi: 10.2148/benv.35.1.107
Brunello, L. (2018). High-Speed Rail and Access Transit Networks. Cham: Springer. doi: 10.1007/978-3-319-61415-1
Caulfield, B., Rock, S., and Ahern, A. (2014). “Equity and fairness in transport planning: the state of play,” in 93rd Annual Meeting of the Transportation Research Board (Washington, DC: Transportation Research Board).
Cervero, R., and Bernick, M. (1996). High-Speed Rail and Development of California's Central Valley. Berkeley: University of California at Berkeley, Institute of Urban and Regional Development.
Ch2m (2018). Ultra-High-Speed Ground Transportation Study. Washington: Washington Department of Transportation.
Chen, C., and Hall, P. (2011). The impacts of high-speed trains on British economic geography: A study of the UK's InterCity 125/225 and its effects. J. Transport Geography 19, 689–704. doi: 10.1016/j.jtrangeo.2010.08.010
Chen, C., Loukaitou-Sideris, A., De Ureña, J., and Vickerman, R. (2019). Spatial short and long-term implications and planning challenges of high-speed rail: A literature review framework for the special issue. Eur. Plan. Stud. 27, 415–433. doi: 10.1080/09654313.2018.1562658
Chen, X., Tang, F., Huang, Z., and Wang, G. (2007). High-speed maglev noise impacts on residents: A case study in Shanghai. Transport. Res. Part D: Trans. Environ. 12, 437–448. doi: 10.1016/j.trd.2007.05.006
Cheng, Y. (2010). High-speed rail in Taiwan: New experience and issues for future development. Transport Policy 17, 51–63. doi: 10.1016/j.tranpol.2009.10.009
Cohn, S. (2019). Hyperloop and Climate Change. Available online at: https://www.hyperlooppartnership.org/single-post/2019/03/12/Hyperloop-and-Climate-Change (accessed July 15, 2020).
Dorsey, B. (2018). Inside a Hyperloop Pod With First-Class Seats, Mood Lighting. Available online at: https://thepointsguy.com/news/step-inside-a-real-hyperloop-pod-with-first-class-seats-and-mood-lighting/ (accessed October 28, 2020).
Edwards, L. K. (1965). High-speed tube transportation. Sci. Am. 213, 30–40 doi: 10.1038/scientificamerican0865-30
FAZ (2020). Vor Köln und Hamburg: Frankfurter Hauptbahnhof mit den meisten Straftaten. Available online at: https://www.faz.net/aktuell/rhein-main/frankfurt/frankfurter-hauptbahnhof-mit-den-meisten-straftaten-16668149.html (accessed October 2, 2020).
Flyvbjerg, B. (2005). Machiavellian megaprojects. Antipode 37, 18–22. doi: 10.1111/j.0066-4812.2005.00471.x
Flyvbjerg, B. (2012). Why mass media matter to planning research. J. Plan. Educ. Res. 32, 169–181. doi: 10.1177/0739456X12441950
Garmendia, M., De Ureña, J., Ribalaygua, C., Leal, J., and Coronado, J. (2008). Urban residential development in isolated small cities that are partially integrated in metropolitan areas by high speed train. Eur. Urban Regional Stud. 15, 249–264. doi: 10.1177/0969776408090415
Garmendia, M., Ribalaygua, C., and Ureña, J. (2012). High speed rail: implication for cities. Cities 29, 26–31. doi: 10.1016/j.cities.2012.06.005
Hall, P. (2009). Magic carpets and seamless webs: opportunities and constraints for high-speed trains in Europe. Built Environ. 35, 59–69. doi: 10.2148/benv.35.1.59
Janić, M. (2018). Multicriteria evaluation of the high-speed rail, trans rapid maglev and hyperloop systems. Transport. Syst. Technol. 4, 5–31. doi: 10.17816/transsyst2018445-31
Janzen, R. (2017). Trans pod ultra-high-speed tube transportation: dynamics of vehicles and infrastructure. Proc. Eng. 199, 8–17. doi: 10.1016/j.proeng.2017.09.142
Jong, M. (2007). Attractiveness of HST Locations. Master thesis. Urban Planning Universiteit van Amsterdam, Amsterdam.
Kamada, M. (1980). “Achievements and future problems of the shinkansen,” in The Shinkansen High-Speed Rail Network of Japan, eds A. Straszak and R. Touch (Pergamon Press).
Kao, T. C., and Lin, C. K. (2007). “Taiwan high speed rail & its impact to regional development,” in Proceedings of the 4th Asian Civil Engineering Coordinating Council (ACECC) International Conference (Taipei, Taiwan).
Kenworthy, J. (2013). Decoupling urban car use and metropolitan GDP growth. World Transport Policy Pract. 19, 7–21
Kenworthy, J. (2021). “Urban structure and its impact on mobility patterns: reducing automobile dependence through polycentrism,” in The Palgrave Encyclopedia of Urban and Regional Futures, ed R. Brears (Cham: Palgrave Springer Nature). doi: 10.1007/978-3-030-51812-7_167-1
Kenworthy, J., and Laube, F. (2001), Millennium Cities Database for Sustainable Transport (database prepared for the UITP), International Association of Public Transport (UITP), Brussels.
Mclean, N. (2016). Comparative Analysis of the Hyperloop Against High-Speed Rail for Commuting Between Sydney, Canberra and Melbourne. Honours Thesis, School of Mechanical and Mining Engineering. The University of Queensland.
Möbert (2018). The German Housing Market in 2018. Available online at: https://www.dbresearch.com/PROD/RPS_EN-PROD/PROD0000000000460528/The_German_housing_market_in_2018.pdf?undefined&realload=EJ0CAaxMWugRNa1yt4fvRJ3R4okEzzRNdoDIUOI97FTiVUQJvjLE32NzNtjYLDaaODjAhfm9Glw1qXjBINO2Q==(accessed November 26, 2020).
Monbiot, G. (2015). Embracing Revolution on Climate Change and Neoliberalism | Letters. Available online at: https://www.theguardian.com/environment/2019/apr/26/embracing-revolution-on-climate-change-and-neoliberalism (accessed February 6, 2020).
Moyano, A., Rivas, A., and Coronado, J. (2019). Business and tourism high-speed rail same-day trips: factors influencing the efficiency of high-speed rail links for Spanish cities. Eur. Plan. Stud. 27, 533–554. doi: 10.1080/09654313.2018.1562657
Muller, P. O. (2004). “Transportation and urban form-stages in the spatial evolution of the American metropolis,” in The Geography of Urban Transportation, eds S. Hanson and G. Giuliano (New York: The Guilford Press).
Murakami, J., and Cervero, R. (2010). California High-Speed Rail and Economic Development: Station-Area Market Profiles and Public Policy Responses. Berkeley: Mimeo, University of California Transportation Centre.
Musk, E. (2013). Hyperloop Alpha. Available online at: https://www.spacex.com/sites/spacex/files/hyperloop_alpha.pdf (accessed February 6, 2020).
Nikitas, A., Kougias, I., Alyavina, E., and Njoya Tchouamou, E. (2017). How can autonomous and connected vehicles, electromobility, BRT, hyperloop, shared use mobility and mobility as a service shape transport futures for the context of smart cities? Urban Sci. 36,40036. doi: 10.3390/urbansci1040036
Oborne, D. (1978). Passenger comfort — an overview. Appl. Ergo. 9, 131–136. doi: 10.1016/0003-6870(78)90002-9
Okabe, S. (1980). “Impact of the sanyo shinkansen on local communities,” in The Shinkansen High-Speed Rail Network of Japan, eds A. Straszak and R. Touch (Belgium: Pergamon Press).
Özbek, R., and Çodur, M. (2021). Comparison of hyperloop and existing transport vehicles in terms of security and costs. Modern Transport. Syst. Technol. 7, 5–29. doi: 10.17816/transsyst2021735-29
Park, D. (2019). Incheon airport maglev line. Przeglad Elektrotechniczny 1, 3–5. doi: 10.15199/48.2019.06.01
Pérez, M. (2019). HYPERLOOP: An Analysis of Its Fit in the European Union. Postgraduate. Politechnico Di Milano.
Peters, D. (2009). The renaissance of inner-city rail station areas: a key element in contemporary urban restructuring dynamics. Crit. Plan. 16, 163–185. Available online at: https://www.researchgate.net/publication/228385956_The_renaissance_of_inner-city_rail_station_areas_a_key_element_in_contemporary_urban_restructuring_dynamics
Pol, P. (2002). A Renaissance of Stations, Railways and Cities: Economic Effects, Development Strategies and Organizational Issues of European High-Speed-Train Stations. Delft University Press, Netherlands.
Preston, J., and Wall, G. (2008). The Ex-ante and Ex-post economic and social impacts of the introduction of high-speed trains in South East England. Plan. Pract. Res. 23, 403–422. doi: 10.1080/02697450802423641
Rajendran, S., and Harper, A. (2020). A simulation-based approach to provide insights on Hyperloop network operations. Transport. Res. Interdisc. Perspect. 4, 1–10. doi: 10.1016/j.trip.2020.100092
Ravenscroft, T. (2019). India Approves Mumbai-Pune Hyperloop In Bid to Build World's First System. Available online at: https://www.dezeen.com/2019/08/07/mumbai-pune-hyperloop-virgin-maharashtra-india/ (accessed September 12, 2020).
Rodrigue, J. (2013). HS2: Mixed Success for High Speed Rail Worldwide. Available online at: https://theconversation.com/hs2-mixed-success-for-high-speed-rail-worldwide-20045 (accessed November 4, 2020).
Salet, W. G. M., and Majoor, S. J. H. (2005). “Reshaping Urbanity in the Amsterdam region,” in Amsterdam Zuidas - European Space, eds W. G. M. Salet and S. J. H. Majoor (Uitgevers: Rotterdam).
Sideris, A. L., Cuff, D., Higgins, T., and Linovski, O. (2012). Impact of high-speed rail stations on local development: a delphi survey. Built Environ. 38, 51–70. doi: 10.2148/benv.38.1.51
Taylor, C., Hyde, D., and Barr, L. (2016). Hyperloop Commercial Feasibility Analysis: High Level Overview. Cambridge, CA: Volpe (US Department of Transport).
Trip, J. J. (2004). “The contribution of HST-related development projects to a competitive Urban climate,” in Paper presented to City Futures Conference (Chicago).
Upbin, B. (2016). We Ran the Numbers on a European Hyperloop — And They Look Fantastic. Available online at: https://hyperloop-one.com/blog/we-ran-the-numbers-on-euro-hyperloop (accessed January 5, 2020).
Vale, D. (2015). Transit-oriented development, integration of land use and transport, and pedestrian accessibility: Combining node-place model with pedestrian shed ratio to evaluate and classify station areas in Lisbon. J. Transport Geography 45, 70–80. doi: 10.1016/j.jtrangeo.2015.04.009
van Goeverden, K., Milakis, D., Janic, M., and Konings, R. (2018). Analysis and modelling of performances of the HL (Hyperloop) transport system. Eur. Transport Res. Rev. 10, 1–17. doi: 10.1186/s12544-018-0312-x
Walker, R. (2018). Hyperloop: Cutting Through the Hype. Available online at: https://trl.co.uk/sites/default/files/Hyperloop%20white%20paper_0.pdf (accessed February 5, 2020).
WSDOT (2018). Ultra-High Speed Ground Transportation Study. Available online at: https://www.wsdot.wa.gov/publications/fulltext/LegReports/17-19/UltraHighSpeedGroundTransportation_FINAL.pdf (accessed October 1, 2021).
Yang, J., Guo, A., Li, X., and Huang, T. (2018). Study of the impact of a high-speed railway opening on China's accessibility pattern and spatial equality. Sustainability 10:2943. doi: 10.3390/su10082943
Yin, M., Bertolini, L., and Duan, J. (2015). The effects of the high-speed railway on urban development: International experience and potential implications for China. Prog. Plan. 98, 1–52. doi: 10.1016/j.progress.2013.11.001
Zemp, S., Stauffacher, M., Lang, D., and Scholz, R. (2011). Generic functions of railway stations - a conceptual basis for the development of common system understanding and assessment criteria. Transport Policy 18,7. doi: 10.1016/j.tranpol.2010.09.007
Zhang, H., Li, X., Liu, X., Chen, Y., Ou, J., Niu, N., et al. (2019). Will the development of a high-speed railway have impacts on land use patterns in China? Ann. Am. Assoc. Geograph. 109, 979–1005. doi: 10.1080/24694452.2018.1500438
Zhong, C., Bel, G., and Warner, M. (2014). High-speed rail accessibility: a comparative analysis of urban access in Los Angeles, San Francisco, Madrid, and Barcelona. Eur. J. Transport Infrastructure Res. 14, 468–488. doi: 10.18757/ejtir.2014.14.4.3048
Keywords: hyperloop, high speed ground transportation, economic factors, environmental impact (EI), urban and transport planning, technical factors, ultra-high speed (UHS), human impact
Citation: Premsagar S and Kenworthy J (2022) A Critical Review of Hyperloop (Ultra-High Speed Rail) Technology: Urban and Transport Planning, Technical, Environmental, Economic, and Human Considerations. Front. Sustain. Cities 4:842245. doi: 10.3389/frsc.2022.842245
Received: 23 December 2021; Accepted: 25 February 2022;
Published: 04 May 2022.
Edited by:
Tiit Tammaru, University of Tartu, EstoniaReviewed by:
Yonn Dierwechter, University of Washington Tacoma, United StatesAnzhelika Antipova, University of Memphis, United States
Copyright © 2022 Premsagar and Kenworthy. This is an open-access article distributed under the terms of the Creative Commons Attribution License (CC BY). The use, distribution or reproduction in other forums is permitted, provided the original author(s) and the copyright owner(s) are credited and that the original publication in this journal is cited, in accordance with accepted academic practice. No use, distribution or reproduction is permitted which does not comply with these terms.
*Correspondence: Jeffrey Kenworthy, ai5rZW53b3J0aHlAY3VydGluLmVkdS5hdQ==