- 1Department of Clinical Microbiology, College of Health Sciences, Kwame Nkrumah University of Science and Technology, Kumasi, Ghana
- 2School of Public Health, College of Health Sciences, Kwame Nkrumah University of Science and Technology, Kumasi, Ghana
- 3Department of Theoretical and Applied Biology, College of Science, Kwame Nkrumah University of Science and Technology (KNUST), Kumasi, Ghana
- 4Biomedical and Public Health Research Unit, Council for Scientific and Industrial Research (CSIR), Water Research Institute, Accra, Ghana
- 5Department of Theoretical and Applied Biology, Kwame Nkrumah University of Science and Technology (KNUST), Kumasi, Ghana
Introduction: Recent surge of Anopheles resistance to major classes of World Health Organization (WHO)-approved insecticides globally necessitates the need for information about local malaria vector populations. It is believed that insecticide efficacy loss may lead to operational failure of control interventions and an increase in malaria infection transmission. We investigated the susceptibility levels of malaria vectors to all classes of WHO-approved vector control insecticides and described the dynamics of malaria transmission in a peri-urban setting.
Methods: Fit 3–5-day-old adults that emerged from Anopheles larvae collected from several different sites in the study area were subjected to the WHO bioassay for detecting insecticide resistance. The knockdown resistance gene (kdr) mutations within the vector populations were detected using PCR. Entomological inoculation rates were determined using the human landing catch technique and Plasmodium falciparum circumsporozoite ELISA.
Results: The malaria vectors from the study area were resistant to all classes of insecticides tested. Out of the 284 Anopheles complex specimen assayed for the resistance study, 265 (93.30%) were identified as Anopheles gambiae s.s. The kdr gene was detected in 90% of the Anopheles gambiae s.s. assayed. In an area where Anopheles coluzzii resistance to insecticides had never been reported, the kdr gene was detected in 78% of the Anopheles coluzzii sampled. The entomological inoculation rate (EIR) for the dry season was 1.44 ib/m/n, whereas the EIR for the rainy season was 2.69 ib/m/n.
Conclusions: This study provides information on the high parasite inoculation rate and insecticide resistance of malaria vectors in a peri-urban community, which is critical in the development of an insecticide resistance management program for the community.
Background
The sub-Saharan African continent has seen a 22% reduction in malaria incidence between 2010 and 2018 (1). Malaria incidence reduction gains have been attributed to the scaled-up provision of vector control interventions (2). However, recent malaria data provided by the World Health Organization (WHO) show that targets for reductions in the death and disease caused by malaria may have stalled due to the rapid emergence of malaria vector control insecticide resistance (3). Two main mechanisms associated with malaria vector control insecticide resistance are metabolic detoxification of insecticides and insecticide target site insensitivity (4). Target site insensitivity is caused by gene mutation of target proteins that interact with vector control insecticides. The commonest target site insensitive mechanisms are the acetylcholinesterase G119S mutation and the voltage-gated sodium channel L1014F mutation that causes resistance to pyrethroids (5). Insecticides produced from the carbamate and organophosphate organochemical class act irreversibly by inactivating the essential enzyme acetylcholinesterase, which is utilized for nerve function in mosquitoes (6). The insecticidal effect of pyrethroids and organochlorines is due to the insecticide chemical binding to the sodium ion channel of the vector. These chemicals act on the gating properties of the vector and keep it open (7). Knockdown resistance (kdr) occurs when the amino acid sequences in voltage-gated sodium channels of the vector get mutated, resulting in the reduction of channel sensitivity to pyrethroid and organochlorine insecticides.
The pyrethroid insecticide class, because of its effectiveness and low toxicity to man, is the most commonly used insecticide class in several vector control programs. It has seen an increase in vector resistance from 71% in the year 2010 to 81% by the end of the year 2016 (3). Between 2010 and 2016, organochlorine insecticide resistance was confirmed in 80% of the WHO member countries (3). More than 50% of the WHO member countries reported insecticide resistance to the carbamate and organophosphate insecticide classes (3). The spread of insecticide resistance is worsened by the use of the same organochemicals in controlling malaria vectors and agricultural pests (8–10). As a result, there is an increased selection pressure on malaria vector populations that do not possess the knockdown resistance (kdr) gene.
In Ghana, the dominant species responsible for the transmission of the disease are Anopheles gambiae s.s, Anopheles coluzzii, Anopheles funestus, and Anopheles arabiensis (8). The key feature that makes these species efficient malaria vectors are their resting behavior and blood source preference. These behavioral differences affect the vectorial capacity, suitability, and effectiveness of vector control interventions. To effectively control these vectors and ultimately reduce disease transmission rates, the establishment of an efficient vector species and behavior database is imperative (6, 11). This will ensure that information on the distribution of vectors, trends in insecticide susceptibility, and transmission rates are factored in national malaria control program decision-making processes. Although several works on vector resistance have been undertaken in Ghana [e.g., (6, 12, 13), just to name a few], the need to describe vector abundance and vector distribution and monitor the changing trends of resistance is still very important. All of these studies were conducted in urban settings with Anopheles speciation in some areas. However, all-inclusive data of vector abundance, speciation, local malaria transmission dynamics, phenotypic and genotypic resistance to insecticides in peri-urban areas are yet to be documented in the Ashanti region of Ghana. We therefore investigated the susceptibility of malaria vectors to all classes of WHO-approved vector control insecticides and those expressed by entomological inoculation rates (EIR), the transmission rates of malaria in a peri-urban setting in the Ashanti region of Ghana.
Methods
Study Site
The Ashanti region, located in the south of Ghana, is known for its natural resources and agricultural activities. The region is divided into 43 administrative districts, out of which 18 are municipalities. The area for this study was Apromase which is located within the Ejisu-Juaben municipality of the region (Figure 1). Apromase is a peri-urban community where extensive irrigated vegetable farming is done and is also close (11 km) to the Kumasi metropolis, the regional capital that has a high demand for agricultural products. Apromase is located in the transitional forest zone with a minimum mean temperature of 22.4°C and a maximum mean temperature of 31.2°C. The rainy season starts in April and ends in October where it coincides with the start of the dry season which ends in March. The mean annual rainfall of the area is 121.3 mm (15).
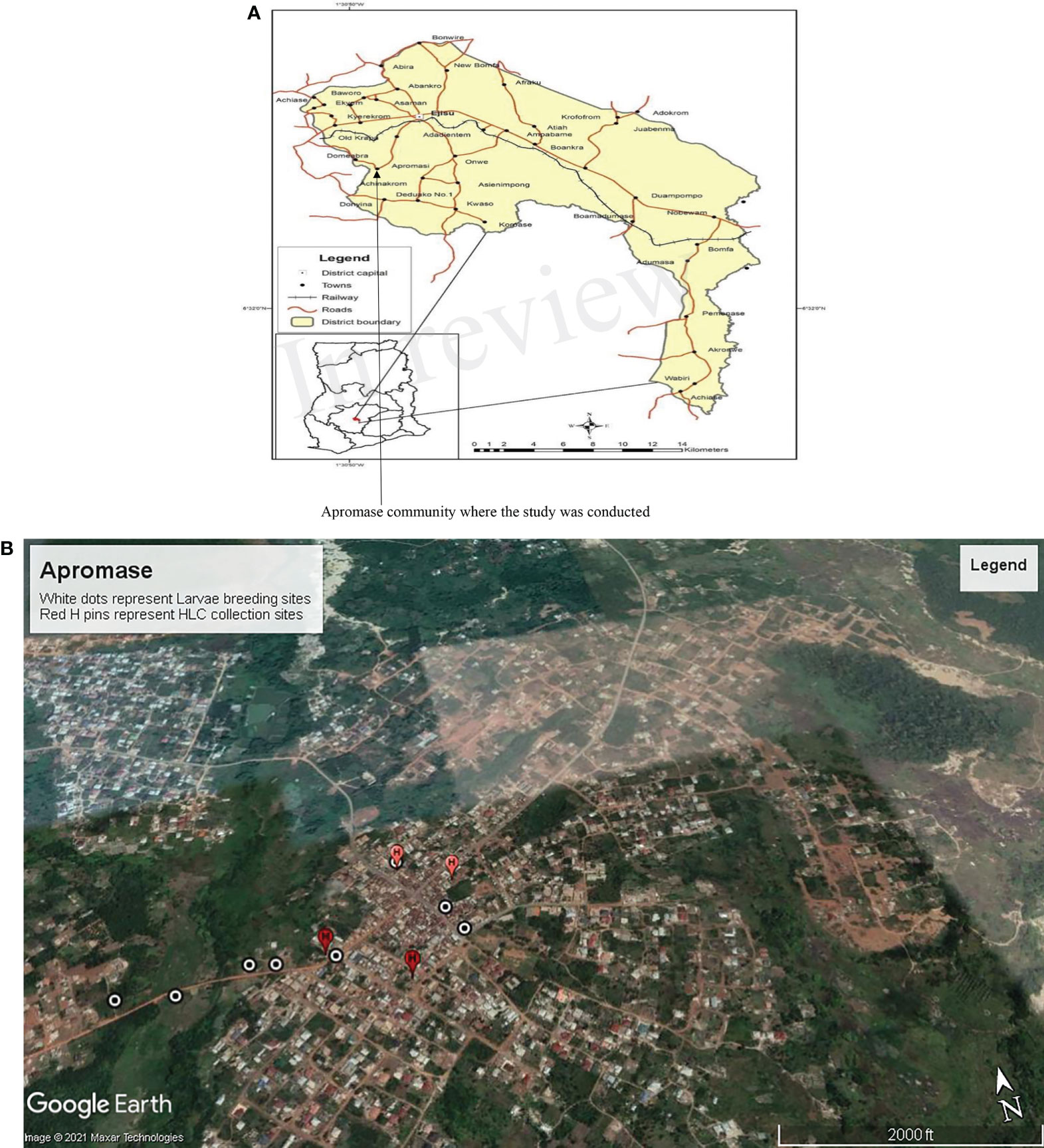
Figure 1 (A) A map of the Ejisu-Juaben Municipality showing Apromase. Source: Ejisu-Juaben Municipal Assembly (14). (B) Anopheles larvae and adult vector collection sites.
The human landing catch (HLC) and larval collections were performed at different sites with some sites overlapping each other. The larval collection sites were selected along the transect of road puddles and water bodies, whereas the HLC were sited closer to houses. This notwithstanding, the distances between HLC sites and larval collections did not exceed 1 km. The sites were thus selected to ensure that houses were within the flight range of emerging mosquitoes. Thus, we can expect that the adult mosquitoes collected are a reflection of larval collection sites. The larval collection sites were a minimum of 100 m apart, and the HLC sites are a minimum of 200 m apart. Larval breeding sites 1 and 12 were 1.47 km apart. HLC site 1 and HLC site 4 are 410 m apart (Figure 1).
Collection of Adult Mosquitoes
The human landing catch (HLC) technique was used to collect adult mosquitoes from four randomly selected houses in the study area. Two trained consenting volunteer adults (one seated indoor and the other outdoor) collected the mosquitoes and were each given a prophylactic dose of doxycycline 100®. The study data were collected for four consecutive nights in December 2018 for the dry season and in April 2019 for the rainy season. Indoor and outdoor mosquito collections were performed simultaneously from 06:00 p.m. to 06:00 a.m. each night (16).
Captured mosquitoes were placed carefully in labeled paper cups with net coverings. A wet cotton wad was placed over the tops of the cups to maintain the mosquitoes at an appropriate humidity during transportation to the insectary of the Department of Clinical Microbiology at the Kwame Nkrumah University of Science and Technology (KNUST) in Kumasi, Ghana. The mosquitoes were morphologically identified, dissected, and preserved dry on silica gels in 1.5-ml Eppendorf tubes.
The HLC and larval collections were performed at different sites as indicated on the Google Earth map with some overlaps. The larval collection sites were along the transect of road puddles and water bodies. However, HLC essentially has to be close to human dwellings, but the distance between HLC and larval collections did not exceed 1 km. Thus, we can expect emerging mosquitoes to fly to where humans sleep for which the HLC must be representative. The larval collection sites are a minimum 100 m apart, and the HLC sites are a minimum 200 m apart. Larval breeding site 1 and breeding site 12 are 1.47 km apart. HLC site 1 and HLC site 4 are 410 m apart.
Determination of Parasite Infection Rate
Sandwich ELISA was conducted on the head and thorax of randomly selected wild-caught Anopheles vectors. The presence of Plasmodium falciparum circumsporozoite protein (PfCSP) in the Anopheles vectors was done following the protocol of Wirtz et al. (17).
Mosquito Larval Collection for Insecticide Susceptibility Bioassay
Anopheles mosquito larvae were collected from breeding sites identified during larval habitat surveys in the community.
They were then transported to the insectary of the Department of Clinical Microbiology at KNUST in Kumasi, Ghana, where they were raised to adulthood.
The collection bowls were kept at temperatures of 25 ± 2°C. Pupated larvae were then collected into plastic cups using Pasteur pipettes and placed in mosquito cages for the emergence of their adult forms which were fed with 10% sucrose solution.
Insecticide Susceptibility Testing Using WHO Bioassay Tubes
Eight (8) WHO-approved insecticide-impregnated papers from four organochemical classes approved for public health use were procured from the Universiti Sains Malaysia, Penang. These were pyrethroids (0.05% deltamethrin, 0.15% cyfluthrin), organophosphates (5.0% malathion, 0.25% pirimiphos-methyl), carbamates (0.1% propoxur, 0.1% bendiocarb), and organochlorines (4.0% dieldrin, 4.0% DDT). Using the WHO insecticide bioassay protocol (18), 3–5-day-old non-blood-fed female Anopheles mosquitoes were used for the bioassay with eight replicates consisting of 20 mosquitoes per tube and a control with paper impregnated with mineral oil. At the end of the 60-min exposure period, the Anopheles mosquitoes were transferred into holding tubes, and a wad of cotton soaked with 10% sucrose solution was placed on top of it for mortality rates to be checked after 24 h. After the 24-h post-exposure period, each exposure tube was examined for dead or alive mosquitoes. Corresponding values for each tube were scored using the WHO insecticide monitoring bioassay guideline (18).
Extraction of Genomic DNA and Identification of Anopheles gambiae Complex Sibling Species
Using the Chelex protocol of Musapa et al. (19), DNA was extracted from dead and alive Anopheles mosquitoes from the WHO resistance bioassay. Restriction fragment length polymorphism polymerase chain reaction (PCR-RFLP) as described by Fanello et al. (20) was used to characterize the Anopheles gambiae complex.
Detection of Knockdown Resistance (kdr) Gene in the Anopheles gambiae Complex
As described by Martinez-Torres et al. (21), primers AgD1 (ATA GAT TCC CCG ACC ATG) and AgD3 (AAT TTG CAT TAC TTA CGA CA) were used to detect the 195-bp fragment of the resistant allele whereas AgD2 (AGA CAA GGA TGA TGA ACC) and AgD4 (CTG TAG TGA TAG GAA ATT TA) were used to detect the 137-bp fragment of the susceptible allele. As a control measure, a common 293-bp fragment was amplified with primers AgD1 and AgD2. The polymerase chain reaction (PCR) was carried out using a PTC 100™ thermal cycler (MJ Research Inc., Watertown, MA, USA) with an initial step of 3 min at 94°C followed by 40 cycles, each consisting of 30 s denaturation at 94°C, 30 s annealing at 53°C (for resistant allele and control fragment detection) or 48°C (susceptible allele detection), and 30 s extension at 72°C; the final cycle products were extended for 5 min at 72°C. Fragments were run through an ethidium bromide 2% agarose gel.
Data Analysis
Data were entered into Microsoft Excel 2016. Graphs and tables were drawn using Microsoft Excel 2016. Sporozoite rates were calculated as the proportion of dissected mosquitoes, which were ELISA positive for PfCSP. The entomological inoculation rates were calculated as the product of the human biting rate and the sporozoite rate.
The WHO criteria for insecticide susceptibility assay were used to define resistance levels. A population was assumed resistant when the mortality rate 24 h after exposure to impregnated insecticide papers was less than 98%. It was scored as susceptible when the mortality rate was equal to or greater than 98%. Th formula of Abbot was applied when the mosquitoes in the control setup experienced mortalities less than 10%. The knockdown resistance gene frequency was calculated using the Hardy–Weinberg formula. Mortality rates were defined as very low when the mortality rate was below 50%, low when the mortality rate was between 50% and 80%, and high when the mortality rate was above 80%.
Results
Biting Pressure of Malaria Vectors
A total of 1,333 Anopheles gambiae s.l. vectors were collected during the study period, as shown in Table 1. The indoor biting rate per night was 78.6 bites per man per night (b/m/n) and 88 b/m/n for the outdoor biting rate. The human biting rate per night for the rainy season was 117 b/m/n and 49.6 b/m/n for the dry season. The overall human biting rate was 83.3 b/m/n.
As shown in Figure 2, Anopheles gambiae s.l. biting commenced at dusk (06:00 p.m.) and continued till dawn (06:00 a.m.). Peak biting activity of the vector for both indoor and outdoor collection was observed between the hours of 01:00 a.m.–02:00 a.m. and 23:00 p.m.–00:00 a.m., respectively.
Sporozoite Infection Rates
Of the 580 Anopheles gambiae mosquitoes assayed for Plasmodium falciparum circumsporozoite protein (PfCSP), 15 were found to be positive for PfCSP leading to a sporozoite infectivity rate of 0.026, as shown in Table 2.
The distribution of PfCSP positive of malaria vectors from Apromase showed that infective bites occurred throughout the evening reaching peak levels between 22:00 p.m. and 03:00 a.m. The overall EIR of malaria vector species collected from indoor and outdoor collections was 2.16 infective bites per man per night (ib/m/n). The EIR for the dry and rainy seasons was 1.44 ib/m/n and 2.69 ib/m/n. A monthly estimated EIR (estEIR) of 64.8 ib/m/n was observed in the community (Table 3).
Insecticide Susceptibility Assay
All Anopheles gambiae s.l. from Apromase were resistant to all classes of insecticide tested. The insecticide 0.15% Cyfluthrin (Pyrethroids) had the least knockdown percentage after 60 min at 18.75% whereas the insecticide 0.25% pirimiphos-methyl (organophosphate) had the highest knockdown percentage after 60 min at 46.87%. Overall, less than 50% of the assayed mosquitoes were knocked down at the 60th-min mark (Figure 3).
Mortality Rates of Female Anopheles Mosquitoes
The mortality rates of female Anopheles mosquitoes exposed to the pyrethroid (31%–44%), organochlorine (24%–27%), and carbamate (25%–54%) organochemical classes were very low (Table 4). Relatively higher mortalities (56%–61%) were recorded for the organophosphate insecticide class.
Molecular Characterization of Anopheles gambiae Complex and Detection of Knockdown Resistance (kdr) Gene
Of the 1,280 used for the insecticide resistance bioassay, 284 (251 alive, 33 dead) were used for molecular speciation of the Anopheles gambiae complex and for the detection of the knockdown resistance (kdr) target-site mutation. Two hundred and sixty-five (93.3%) were Anopheles gambiae s.s. while the remainder were Anopheles coluzzii. Overall, 255 (89%) of the assayed Anopheles gambiae population from Apromase had the kdr gene. The kdr gene was found in 240 (90.55%) of the assayed Anopheles gambiae s.s. and 15 (78.94%) of the Anopheles coluzzii (Table 5).

Table 5 The distribution of the knockdown resistance (kdr) gene in female Anopheles mosquitoes assayed.
The kdr gene was found in 249 (99.20%) of the female Anopheles vectors that were alive 24 h after exposure to insecticides (Table 6).

Table 6 The distribution of the knockdown resistance (kdr) gene in alive female Anopheles mosquitoes from susceptibility bioassay.
The kdr gene was found in six (18.18%) of the female Anopheles vectors that were dead 24 h after exposure to insecticides (Table 7).

Table 7 The distribution of the knockdown resistance (kdr) gene in dead female Anopheles mosquitoes from susceptibility bioassay.
Discussion
A better understanding of the composition, host preferences, and vectorial capacity of disease vectors is essential for the implementation of integrated vector control interventions. This study was carried out to investigate the diversity, density, parasite inoculation rates, and insecticide susceptibility profile of malaria vectors in Apromase, Ghana.
More than 93% of the malaria vectors collected from the Apromase community were identified as Anopheles gambiae s.s., similar to species ratios reported in previous studies in the country (6, 22). The high proportion of Anopheles gambiae s.s. in a community which falls within the rain forest zone of Ghana was not surprising given previous observations, suggesting that Anopheles gambiae s.s. is more adapted to breeding in temporary pools and rain forest areas (8, 23). As envisaged, the Anopheles coluzzii species which is usually found in paddy fields (24) was less than 7% as in studies (4, 25).
The biting rate of a vector in a given locality is one of the key indices that influence disease transmission (26). In a study conducted in Ghana (13), only Anopheles coluzzii with a high entomological inoculation rate (EIR) and anthropomorphism was found to contribute to malaria transmission. The appearance of Anopheles coluzzii in the metropolis where they were not previously observed (27) has significant implications for malaria transmission in the region. This assertion however requires studies to be done over an extended period on a larger sampling scale to fully appreciate localized entomological data. This ultimately should assist in the regional and national application of stringent malaria control policies, particularly in the event of high Leu-Phe knockdown resistance gene mutations, which is the main mechanism of pyrethroid insecticide resistance in Anopheles coluzzii malaria vectors.
Klinkenberg et al. (28) observed that insecticide resistance and transmission of malaria was not solely a rural phenomenon given that levels of disease transmission in urban and peri-urban areas with numerous irrigated farms were similar to rural areas. The elevated Anopheles population is therefore very much expected in urban and peri-urban settings, thus buttressing literature (4, 6, 27) that irrigated farms were the chief culprits in populating urban and peri-urban settings with resistant vectors. Based on the WHO criteria for characterizing insecticide resistance, this study found evidence of insecticide resistance in the vector population in a peri-urban setting to all the tested insecticides. Bioassay results showed high levels of resistance of the malaria vector species to various organochemicals most significantly to the pyrethroid organochemical which happens to be the most effective class approved for the production of long-lasting insecticide bed nets. The Anopheles vector developing genotypic and phenotypic resistance to organochemicals approved for vector control has been linked to the scaled-up coverage of ITNs, IRS, and agricultural practices (8, 9). In Ghana, it is estimated that over 80% of cash crop and vegetable farmers utilize organochemicals that are reformulated in the production of insecticides for pest control chemicals (6, 29) primarily due to their effective curative action (30). During pesticide applications, residues of these organochemicals seep into surrounding breeding pools, thus constantly exposing the vector larval stage to the control chemicals. In addition to ITNs, consumer products such as mosquito insecticide coils, aerosol sprays, and repellent creams are used in various homes (31). In all these consumer products, pyrethroid is the commonly used organochemical. This may be an auxiliary factor to the rapid buildup of resistance in local vectors.
Effective regulation of agro-pesticide use and management of insecticide resistance will be critical in avoiding, delaying the development of resistance, or regaining susceptibility in vectors to improve public health. An expansive integrated vector control program targeting the different life stages of the malaria vector is belated and should be executed with knowledge on local vectorial competence.
Assessment of vectorial competence is usually achieved through detecting circumsporozoite in the salivary glands of the vector. Sporozoite infectivity assay results of this study showed that high Plasmodium falciparum sporozoite rates in Apromase were similar to other infectivity rates reported across the subregion (13, 32, 33). The malaria vector is abundant in urban, peri-urban, and rural areas of Ghana. Changes in the behavior of the vector and how it affects disease transmission in these settings particularly peri-urban areas however remain poorly understood. The hourly biting pattern of the vector in Apromase showed biting intensifying from 09:00 p.m. to 04:00 a.m. Hourly distribution of sporozoite-positive bites of Anopheles in the coastal savannah of Ghana (13) revealed that infective Anopheles bites usually occurred after 21:00 and peaked at 04.00 a.m. The hourly biting activities with peaks after midnight and declining toward dawn are typical for the malaria vector and have been described extensively in several areas (13, 34). Anopheles feeding and resting behavior is of great importance with respect for the effectiveness of vector control interventions. Malaria vectors collected indoor in this study were marginally below that of the outdoor collection unlike previous studies (32, 34) that reported strong endophilic behavior of the Anopheles vector. The vector altering its feeding location is not unheard of.
They have been documented to alter, depending on the season and location, their feeding behavior (34, 35). In the Northern parts of Ghana, where there is a distinct difference in weather conditions as compared to the middle and southern parts of the country, Koram et al. (36) showed that vectors readily sought hosts outdoors in when inhabitants dwelled or slept outside due to the higher temperatures.
This present study did not characterize the distribution of infectivity with regard to the hour of the night, but the reports of Tchouassi et al. and Badu et al. (13, 32) indicate that the majority of the Anopheles gambiae infective bites occur between the hours of 12:00 a.m. and 04:00 a.m., which coincides with peak biting densities of the vectors observed in this study. This has an important epidemiologic consequence as many of the inhabitants are usually out of bed by dawn. It has been reported by Tuno et al. (34) that Anopheles frequently sought their hosts outdoors and that peak time for biting had changed from midnight to early hours of the night, hence overlapping with outdoor human activity (37). These changes seemed to have coincided with the scale-up distribution of ITNs, indicating that exophilic behaviors may be influenced by insecticides (38). Comparable studies (13) reported that the biting behavior of Anopheles had changed to the early hours of the evening (06:00 p.m.–09:00 p.m.) because people in Ghana generally went to sleep under LLINs after 09:00 p.m. According to Okwa et al. (39), the sporozoite rate of Anopheles gambiae in the forest zone of Nigeria was 2.33%. This is comparable to the estimated sporozoite rate of 2.59% that we obtained. The biting behavior of the vector in the community therefore necessitates a review control strategy as some vectors may feed on inhabitants when they are active and unprotected outdoors (40).
The entomological inoculation rate (EIR) is a favored tool used for assessing malaria transmission intensity and endemicity based on the estimated exposure of people to Plasmodium falciparum-infected vectors. A review of EIR estimates across the African continent (41) found marked heterogeneity in malaria risk. The review showed that EIR estimates mostly from rural areas ranged from 0 to 884 infectious bites per person annually whereas EIRs from urban settings ranged from 0 to 43 infectious bites per person annually. This study shows that malaria transmission is generally high in the forest zone of Ghana and that inhabitants of Apromase, a peri-urban community with reduced insecticide efficacy, could be exposed to 788 infective bites in a year. The annual estimated EIR of this study (788.4), although higher than EIRs reported by Appawu (42) in the coastal forest zone of Ghana (21.9) and Owusu-Agyei (43) in the forest savannah transitional zone (269), is comparable to EIRs reported in Afanamanso (866), a rural farming community in the forest zone by Abonuusum (44). Ghana is one of 11 countries described by the WHO as high malaria burden countries. The country saw an 8% increase in malaria cases in 2018 compared to 2017 (45). With no or significantly reduced protection from vector bites due to insecticide resistance, the rate of disease transmission in the community and the country at large will continue to climb. It is therefore to be reasonably feared that the use of insecticides and other vector control tools may not be effective in preventing malaria transmission in areas with such high transmission rate.
The WHO insecticide susceptibility bioassays provided information on a limited scale to infer the presence but not the intensity of insecticide resistance in the community. A wider study with a larger sample size focusing on intensity bioassays is needed to better inform the vector profile in the region.
Limitations of the Study
This study did not include clinical malaria cases or parasite prevalence in the study community. Parasite prevalence would have indicated malaria burden in the study area. However, the study reports man biting rates (host–vector contact), sporozoite rates (proportion of vector-carrying parasites), and the EIR which indicates the number of infectious bites an individual receives in a given period. Beier et al. (46), using data from 31 sites in Africa, observed a linear relationship (r2 = 0.71) between annual EIRs. 5, 15, and 200 infective bites per year had levels of P. falciparum prevalence exceeding 40%, 50%, and 80%, respectively. Thus, EIR is a good indicator of transmission intensity upon which vector control can be benchmarked.
Conclusion
The findings of this study show plasticity in Anopheles gambiae biting patterns and an increasing vector insecticide resistance in the Apromase community which is a community with no resistance management carried out by state agencies responsible for ensuring compliance and proper use of organochemicals. The formulation of a robust resistance management program that incorporates the changing behavior of the vector is therefore critical as the country presses to eliminate malaria to achieve the sustainable development goals (SDG) 3 and 8.
Data Availability Statement
The original contributions presented in the study are included in the article/Supplementary Material. Further inquiries can be directed to the corresponding author.
Ethics Statement
The studies involving human participants were reviewed and approved by the Committee on Human Research, Publications and Ethics; Kwame Nkrumah University of Science and Technology, School of Medical Sciences, and Komfo Anokye Teaching Hospital. The patients/participants provided their written informed consent to participate in this study.
Author Contributions
YA-B, KB, and SK designed the study. RB critically supervised the study. YA-B, PO, IO-F, and AA carried out the laboratory experiments and sample analysis. YA-B and KB analyzed the data and drafted the manuscript. RB, KB, and SK revised the manuscript for important intellectual content. All authors contributed to the article and approved the submitted version.
Funding
This research was supported by PSOP24-377 Research project, which is part of the EDCTP2 programme supported by the European Union (grant number: CDF1605-2016). The views and opinions of authors expressed herein do not necessarily state or reflect those of EDCTP.
Conflict of Interest
The authors declare that the research was conducted in the absence of any commercial or financial relationships that could be construed as a potential conflict of interest.
Publisher’s Note
All claims expressed in this article are solely those of the authors and do not necessarily represent those of their affiliated organizations, or those of the publisher, the editors and the reviewers. Any product that may be evaluated in this article, or claim that may be made by its manufacturer, is not guaranteed or endorsed by the publisher.
Acknowledgments
We are indebted to Prof. Ellis Owusu-Dabo for providing us with laboratory space. The following reagent was obtained through BEI Resources, NIAID, NIH: Plasmodium falciparum Sporozoite ELISA Reagent Kit, MRA890, contributed by Robert A. Wirtz. We are grateful to Dr. Fredrick Opoku, Rebecca Pwalia, Senyo Botchie, Hubert Ahor, and Priscilla Adjei-Kusi for assisting in the collection of data. Finally, the study team is grateful to all households and vegetable farmers within the study area.
Supplementary Material
The Supplementary Material for this article can be found online at: https://www.frontiersin.org/articles/10.3389/fitd.2021.739771/full#supplementary-material
Abbreviations
AM, ante meridiem; DDT, dichlorodiphenyltrichloroethane; DNA, deoxyribonucleic acid; EIR, entomological inoculation rate; ELISA, enzyme-linked immunosorbent assay; GMT, Greenwich Meridian Time; HLC, human landing catch; IRS, indoor residual spray; ITN, insecticide treated net; KDR, knockdown resistance; MBR, man biting rate; PfCSP, Plasmodium falciparum circumsporozoite protein; PM, post meridiem; SDG, sustainable development goal; SPR, sporozoite rate; WHO, World Health Organization.
References
2. Chanda J, Saili K, Phiri F, Stevenson JC, Mwenda M, Chishimba S, et al. Pyrethroid and Carbamate Resistance in Anopheles Funestus Giles Along Lake Kariba in Southern Zambia. Am J Trop Med Hyg (2020) 103(2 Suppl)90–7. doi: 10.4269/ajtmh.19-0664
3. World Health Organization. World Malaria Report. (2016). pp. 1–186 © World Health Organization 2016
4. Pwalia R, Joannides J, Iddrisu A, Addae C, Acquah-Baidoo D, Obuobi D, et al. High Insecticide Resistance Intensity of Anopheles Gambiae (Sl) and Low Efficacy of Pyrethroid LLINs in Accra, Ghana. Parasites Vectors (2019) 12(1):1–9. doi: 10.1186/s13071-019-3556-y
5. Wondji CS, Dabire RK, Tukur Z, Irving H, Djouaka R, Morgan JC, et al. Identification and Distribution of a GABA Receptor Mutation Conferring Dieldrin Resistance in the Malaria Vector Anopheles Funestus in Africa. JIB (2011) 41(7):484–91. doi: 10.1016/j.ibmb.2011.03.012
6. Baffour-Awuah S, Annan AA, Maiga-Ascofare O, Dieudonné SD, Adjei-Kusi P, Owusu-Dabo E, et al. Insecticide Resistance in Malaria Vectors in Kumasi, Ghana. Parasites Vectors (2016) 9(1):633. doi: 10.1186/s13071-016-1923-5
7. Colovic MB, Krstic DZ, Lazarevic-Pasti TD, Bondzic AM, Vasic VM. Acetylcholinesterase Inhibitors: Pharmacology and Toxicology. JCn (2013) 11(3):315–35. doi: 10.2174/1570159X11311030006
8. Chabi J, Baidoo PK, Datsomor AK, Okyere D, Ablorde A, Iddrisu A, et al. Insecticide Susceptibility of Natural Populations of Anopheles Coluzzii and Anopheles Gambiae (Sensu Stricto) From Okyereko Irrigation Site, Ghana, West Africa. Parasites Vectors (2016) 9(1):1–8. doi: 10.1186/s13071-016-1462-0
9. Akogbeto M, Djouaka R, Noukpo H. Use of Agricultural Insecticides in Benin. Bull la Societe Pathologie Exotique (1990) (2005) 98(5):400–5.
10. Diabate A, Baldet T, Chandre F, Akoobeto M, Guiguemde TR, Darriet F, et al. The Role of Agricultural Use of Insecticides in Resistance to Pyrethroids in Anopheles Gambiae Sl in Burkina Faso. Am J Trop Med Hyg (2002) 67(6):617–22. doi: 10.4269/ajtmh.2002.67.617
11. Kabula BI, Attah PK, Wilson MD, Boakye DA. Characterization of Anopheles Gambiae Sl and Insecticide Resistance Profile Relative to Physicochemical Properties of Breeding Habitats Within Accra Metropolis, Ghana. Tanzania J Health Res (2011) 13(3):163–87. doi: 10.4314/thrb.v13i3.66915
12. Anto F, Asoala V, Anyorigiya T, Oduro A, Adjuik M, Owusu-Agyei S, et al. Insecticide Resistance Profiles for Malaria Vectors in the Kassena-Nankana District of Ghana. Malaria J (2009) 8(1):81. doi: 10.1186/1475-2875-8-81
13. Tchouassi DP, Quakyi IA, Addison EA, Bosompem KM, Wilson MD, Appawu MA, et al. Characterization of Malaria Transmission by Vector Populations for Improved Interventions During the Dry Season in the Kpone-On-Sea Area of Coastal Ghana. Parasites Vectors (2012) 5(1):212. doi: 10.1186/1756-3305-5-212
14. Wiafe ED, Asamoah GJ. Land-Cover Change Assessment in Ejisu–Juabeng District in Ghana. EJoFS (2018) 6(1):1–8. doi: 10.31195/ejejfs.429741
16. Abeyasingha R, Yapabanadara A, Kusumawathie P, Perera D, Peiris B, Hewavitharane H, et al. Guidelines for Entomological Surveillance of Malaria Vectors in Sri Lanka. (2009). pp. 62–7. Available at: http://amc.health.gov.lk/images/Publication%20Repository/SOP/Revised_Guidelines_for_Entomological_surveillance.pdf Accessed on 20th June 2021.
17. Wirtz RA, Zavala F, Charoenvit Y, Campbell GH, Burkot TR, Schneider I, et al. Comparative Testing of Monoclonal Antibodies Against Plasmodium Falciparum Sporozoites for ELISA Development. Bull World Health Organ (1987) 65(1):39–45.
18. World Health Organization. Test Procedures for Insecticide Resistance Monitoring in Malaria Vector Mosquitoes. (2016). Available at: https://apps.who.int/iris/bitstream/10665/250677/1/9789241511575-eng.pdf © World Health Organization 2016.
19. Musapa M, Kumwenda T, Mkulama M, Chishimba S, Norris DE, Thuma PE, et al. A Simple Chelex Protocol for DNA Extraction From Anopheles Spp. J Vis Exp (2013) (71):3281. doi: 10.3791/3281
20. Fanello C, Santolamazza F, Della Torre A. Simultaneous Identification of Species and Molecular Forms of the Anopheles Gambiae Complex by PCR-RFLP. Med Veterinary Entomol (2002) 16(4):461–4. doi: 10.1046/j.1365-2915.2002.00393.x
21. Martinez-Torres D, Chandre F, Williamson M, Darriet F, Berge JB, Devonshire AL, et al. Molecular Characterization of Pyrethroid Knockdown Resistance (Kdr) in the Major Malaria Vector Anopheles Gambiae Ss. Insect Mol Biol (1998) 7(2):179–84. doi: 10.1046/j.1365-2583.1998.72062.x
22. Hunt RH, Fuseini G, Knowles S, Stiles-Ocran J, Verster R, Kaiser ML, et al. Insecticide Resistance in Malaria Vector Mosquitoes at Four Localities in Ghana, West Africa. Parasites Vectors (2011) 4(1):107. doi: 10.1186/1756-3305-4-107
23. Wondji C, Simard F, Fontenille D. Evidence for Genetic Differentiation Between the Molecular Forms M and S Within the Forest Chromosomal Form of Anopheles Gambiae in an Area of Sympatry. JIMB (2002) 11(1):11–9. doi: 10.1046/j.0962-1075.2001.00306.x
24. Charlwood J, Tomas E, Egyir-Yawson A, Kampango A, Pitts R. Feeding Frequency and Survival of Anopheles Gambiae in a Rice-Growing Area in Ghana. JM Entomol v (2012) 26(3):263–70. doi: 10.1111/j.1365-2915.2011.00987.x
25. Djègbè I, Boussari O, Sidick A, Martin T, Ranson H, Chandre F, et al. Dynamics of Insecticide Resistance in Malaria Vectors in Benin: First Evidence of the Presence of L1014S Kdr Mutation in Anopheles Gambiae From West Africa. Malar J (2011) 10: (1):1–11. doi: 10.1186/1475-2875-10-261
26. Beier JC, Perkins PV, Onyango FK, Gargan TP, Oster CN, Whitmire RE, et al. Characterization of Malaria Transmission by Anopheles (Diptera: Culicidae) in Western Kenya in Preparation for Malaria Vaccine Trials. J Med Entomol (1990) 27(4):570–7. doi: 10.1093/jmedent/27.4.570
27. Afrane YA, Klinkenberg E, Drechsel P, Owusu-Daaku K, Garms R, Kruppa T. Does Irrigated Urban Agriculture Influence the Transmission of Malaria in the City of Kumasi, Ghana? JAt (2004) 89(2):125–34. doi: 10.1016/j.actatropica.2003.06.001
28. Klinkenberg E, McCall P, Wilson MD, Amerasinghe FP, Donnelly MJ. Impact of Urban Agriculture on Malaria Vectors in Accra, Ghana. JMJ (2008) 7(1):1–9. doi: 10.1186/1475-2875-7-151
29. Dinham B. Growing Vegetables in Developing Countries for Local Urban Populations and Export Markets: Problems Confronting Small-Scale Producers. Pest Manage Sci (2003) 59(5):575–82. doi: 10.1002/ps.654
30. Hardy J. Apolipoprotein E in the Genetics and Epidemiology of Alzheimer's Disease. Am J Med Genet (1995) 60(5):456–60. doi: 10.1002/ajmg.1320600519
31. Naing C, Ren WY, Man CY, Fern KP, Qiqi C, Ning CN, et al. Awareness of Dengue and Practice of Dengue Control Among the Semi-Urban Community: A Cross Sectional Survey. J Community Health (2011) 36: (6):1044–9. doi: 10.1007/s10900-011-9407-1
32. Badu K, Brenya RC, Timmann C, Garms R, Kruppa TF. Malaria Transmission Intensity and Dynamics of Clinical Malaria Incidence in a Mountainous Forest Region of Ghana. MWJ (2013) 4:14.
33. Dery DB, Brown C, Asante KP, Adams M, Dosoo D, Amenga-Etego S, et al. Patterns and Seasonality of Malaria Transmission in the Forest-Savannah Transitional Zones of Ghana. Malaria J (2010) 9(1):314. doi: 10.1186/1475-2875-9-314
34. Tuno N, Kjaerandsen J, Badu K, Kruppa TJ. Blood-Feeding Behavior of Anopheles Gambiae and Anopheles Melas in Ghana, Western Africa. Jome (2010) 47(1):28–31. doi: 10.1603/033.047.0104
35. McBride CS, Baier F, Omondi AB, Spitzer SA, Lutomiah J, Sang R, et al. Evolution of Mosquito Preference for Humans Linked to an Odorant Receptor. Nature (2014) 515: (7526):222–7. doi: 10.1038/nature13964
36. Loroño-Pino MA, García-Rejón JE, Machain-Williams C, Gomez-Carro S, Nuñez-Ayala G, del Rosario Nájera-Vázquez M, et al. Towards a Casa Segura: A Consumer Product Study of the Effect of Insecticide-Treated Curtains on Aedes Aegypti and Dengue Virus Infections in the Home. Am J Trop Med Hyg (2013) 89: (2):385–97. doi: 10.4269/ajtmh.12-0772
37. Baffour-Awuah S, Owusu-Dabo E, Kruppa T, Annan A, Nartey R, Dogbe J, et al. Lysinibacillus Sphaericus Biolarvicide, an Efficacious Tool in the Control of Anopheles Gambiae in Kumasi, Ghana. East Afr J Public Health (2014) 11:851–61.
38. Reddy MR, Overgaard HJ, Abaga S, Reddy VP, Caccone A, Kiszewski AE, et al. Outdoor Host Seeking Behaviour of Anopheles Gambiae Mosquitoes Following Initiation of Malaria Vector Control on Bioko Island, Equatorial Guinea. Malar J (2011) 10: (1):1–10. doi: 10.1186/1475-2875-10-184
39. Okwa O, Akinmolayan F, Carter V, Hurd HJ. Transmission Dynamics of Malaria in Nigeria. AoAm (2009) 8(1):1–9. doi: 10.4103/1596-3519.55756
40. Machani MG, Ochomo E, Amimo F, Kosgei J, Munga S, Zhou G, et al. Resting Behaviour of Malaria Vectors in Highland and Lowland Sites of Western Kenya: Implication on Malaria Vector Control Measures. PloS One (2020) 15(2):e0224718. doi: 10.1371/journal.pone.0224718
41. Hay SI, Rogers DJ, Toomer JF, Snow RWJ. Annual Plasmodium Falciparum Entomological Inoculation Rates (EIR) Across Africa: Literature Survey, Internet Access and Review. TotRSoTM Hyg (2000) 94(2):113–27. doi: 10.1016/S0035-9203(00)90246-3
42. Appawu M, Baffoe-Wilmot A, Afari E, Dunyo S, Koram K, Nkrumah F. Malaria Vector Studies in Two Ecological Zones in Southern Ghana. JAE (2001) 9(1):59–65.
43. Owusu-Agyei S, Asante KP, Adjuik M, Adjei G, Awini E, Adams M, et al. Epidemiology of Malaria in the Forest-Savanna Transitional Zone of Ghana. Malar J (2009) 8: (1):1–10. doi: 10.1186/1475-2875-8-220
44. Abonuusum A, Owusu-Daako K, Tannich E, May J, Garms R, Kruppa T. Malaria Transmission in Two Rural Communities in the Forest Zone of Ghana. JPr (2011) 108(6):1465–71. doi: 10.1007/s00436-010-2195-1
45. World Health Organization. World Malaria Report. (2019). pp 1–232. Available at: https://www.who.int/publications-detail-redirect/9789241565721.
Keywords: insecticide resistance, vector, malaria, sporozoite rate, EIR, transmission intensity, Anopheles gambiae (s.l.)
Citation: Akuamoah-Boateng Y, Brenyah RC, Kwarteng SA, Obuam P, Owusu-Frimpong I, Agyapong AK and Badu K (2021) Malaria Transmission, Vector Diversity, and Insecticide Resistance at a Peri-Urban Site in the Forest Zone of Ghana. Front. Trop. Dis 2:739771. doi: 10.3389/fitd.2021.739771
Received: 11 July 2021; Accepted: 24 August 2021;
Published: 17 September 2021.
Edited by:
Baldwyn Torto, International Centre of Insect Physiology and Ecology (ICIPE), KenyaReviewed by:
Jonathan Wee Kent Liew, University of Malaya, MalaysiaThomas Unnasch, University of South Florida, United States
Copyright © 2021 Akuamoah-Boateng, Brenyah, Kwarteng, Obuam, Owusu-Frimpong, Agyapong and Badu. This is an open-access article distributed under the terms of the Creative Commons Attribution License (CC BY). The use, distribution or reproduction in other forums is permitted, provided the original author(s) and the copyright owner(s) are credited and that the original publication in this journal is cited, in accordance with accepted academic practice. No use, distribution or reproduction is permitted which does not comply with these terms.
*Correspondence: Kingsley Badu, a2luZ3NiYWR1QGdtYWlsLmNvbQ==