- 1Department of Parasitology, Noguchi Memorial Institute of Medical Research, University of Ghana, Legon Accra, Ghana
- 2School of Biodiversity, One Health and Veterinary Medicine, College of Medical, Veterinary and Life Sciences, University of Glasgow, Graham Kerr Building, Glasgow, United Kingdom
Novel ideas for control of mosquito-borne disease include the use of bacterial symbionts to reduce transmission. Bacteria belonging to the family Enterobacteriaceae isolated from mosquito midgut have shown promise in limiting Plasmodium intensity in the Anopheles vector. However, the mechanism of interaction between bacteria and parasite remains unclear. This study aimed at screening bio-products of two bacteria candidates for their anti-Plasmodial effects on mosquito stages of P. falciparum. Enterobacter cloacae and Serratia marcescens were isolated from field-caught Anopheles gambiae s.l. Spent media from liquid cultures of these bacteria were filtered, lyophilized and dissolved in sterile phosphate buffered saline (PBS). The re-dissolved bacterial products were added to gametocytaemic blood meals and fed to An. gambiae mosquitoes via membrane feeders. Control groups were fed on infected blood with or without lyophilized LB medium. The effect of the products on the infection prevalence and intensity of P. falciparum in mosquitoes was assessed by dissecting mosquito midguts and counting oocysts 10-11 days post-infection. S. marcescens bio-products elicited significant reduction in the number of mosquitoes infected (P=4.02 x10-5) with P. falciparum and the oocyst intensity (P<2 x 10-16) than E. cloacae products (P>0.05 for both prevalence and intensity) compared to the control (lyophilized LB medium). These data support the use of bioproducts released by S. marcescens for malaria control based on transmission blocking in the vector.
Introduction
Malaria elimination has been on the agenda for decades and various schemes towards its achievement have been implemented especially in sub-Saharan Africa (1). These strategies have focused primarily on reducing Anopheles vector contact, or Anopheles numbers (2, 3). While the largely chemical-based interventions have led to a significant decline in malaria global burden, the risk of infection and the morbidity and mortality caused by malaria persist (4). Resistance to commonly used insecticides is widespread in the mosquito vector populations which hampers the progressive decline of malaria incidence as reviewed in (5). The paradigm for control and elimination of malaria and other mosquito-borne diseases now includes transmission-blocking mechanisms. Mosquito-endosymbiont interactions have become central to the discovery and development of disease blocking components.
The mosquito midgut is a major bottleneck in the Plasmodium parasite life cycle (6). There is a significant decline in the number of parasites during their traversal of the vector midgut, making the site important for exploiting transmission-blocking potential. Ingested parasites share the mosquito midgut environment with symbiotic bacteria (microbiota) (7, 8). Several of these bacteria have shown significant negative impact on Plasmodium development (reviewed in (9)) making the mosquito microbiota an integral part of vector competence (8, 10). Enterobacter spp. and Serratia marcescens isolated from Anopheles mosquitoes have shown inhibitory results against P. falciparum infection when they are mixed with infected blood and fed to mosquitoes (11, 12).
The anti-parasitic effects of mosquito midgut microbiota is thought to be either through stimulation of the anti-microbial immune responses which are cross-reactive with ingested parasites, or a direct influence on the developing parasites (production of metabolites, enzymes or toxins) (13–15). An. gambiae thioester-containing proteins (TEPs) are particularly involved in phagocytosis of some bacteria (16) and implicated in the destruction of Plasmodium by binding to the parasite surface and eliciting haemocyte encapsulation (17–19). The interaction between mosquito midgut bacteria and parasites could also be direct, through biomolecules that the microbes produce (12, 20). For example, Serratia ureilytica isolated from the midguts of An. sinensis block P. vivax ookinete to oocyst development via lipase activity (15), although this function is not assumed to be ubiquitous among Serratia spp (15, 20).
In this study, we investigate the direct anti-parasitic potential of two bacteria species, E. cloacae and S. marcescens, which are commonly isolated from blood-fed Anopheles mosquitoes. Here, the effect on mosquito stages was examined by feeding bacterial bio-products and P. falciparum to Anopheles gambiae s.s. and assessing the prevalence and intensity of infection.
Materials and methods
Preparation of bacterial spent medium
S. marcescens (Sm) and E. cloacae (Ec) were previously isolated from the midgut of adult female An. gambiae s.l collected from a farm site at Opeibea, Ghana and stored in glycerol at -80°C (21). These were revived by culturing the isolated bacteria species each separately in LB broth overnight at 37°C with shaking at 120rpm and then plating on LB agar to confirm the presence of pure colonies. Bacteria cells were picked and put into culture overnight. Optical density was measured at OD600nm and adjusted to obtain a standardised OD of 0.50-0.55. For each bacterium, 500µL of standardised culture was inoculated into 50mL sterile LB broth. A control tube was set with 50mL sterile LB broth without inoculum. All tubes were incubated as above and retrieved after 8 hours which corresponds to the stationary phase of the two bacteria species (data not shown). Each tube was centrifuged at 18,407 xg to separate the bacteria cells from the spent medium, and supernatant was passed through 0.2µm disc filter to remove cells. The OD of the supernatant pre- and post-filtration was measured and compared to confirm the removal of bacterial cells (Supplementary Table 1). The filtrate was lyophilized by freeze-drying and stored at 4°C until use. Stock concentrations of bioproducts were prepared by dissolving the dry sample in 1X sterile PBS: LB media control (50mg/mL), S. marcescens (150mg/mL), E. cloacae (100mg/mL). Working concentrations of the dissolved products were freshly prepared on the day of mosquito infection experiments by further diluting with sterile 1X PBS and filtering before mixing with the blood meal to get final concentrations.
Mosquito strain
An. gambiae s.s. Kisumu strain mosquitoes (22) were reared under standard conditions of 26°C, 80% relative humidity and a 12:12 hour light:dark cycle. Adult mosquitoes were maintained on 10% glucose with 0.05% para-aminobenzoic acid (PABA) solution, given ad libitum. Fifty (50) 3–5-day old female adults were placed into each of four experimental groups and starved for 24 hours prior to infectious blood feeding at 5-7 days post emergence.
Infection experiments
Plasmodium falciparum clone 3D7 (23) parasites were cultured to produce gametocytes according to standard protocols (24) and used to prepare infectious blood meals. 30µL of re-suspended bacterial product was added to 1.5mL infectious bloodmeal to give a final concentration of 1mg/mL (LB), 2mg/mL (Ec) and 3mg/ml (Sm). These concentrations were previously identified as effective on asynchronous Plasmodium in an in vitro assay (data not shown). A second control was prepared with only infectious blood and without any bio-product (control). The gametocyte density in each blood meal was assessed from Giemsa-stained thin blood films made of the blood meal preparation. The infection experiment was performed with three independent replicates. Mosquitoes were dissected 10-11 days after the infectious feed and the midguts were examined at 400x magnification to establish the number of mosquitoes infected (prevalence) and oocyst number (intensity) in each infected mosquito.
Estimation of mosquito body size
The mosquito body size was measured to allow consideration as a likely determinant of parasite prevalence and intensity. The wing length of the mosquito was measured as an indicator of mosquito body size (25, 26). A wing was removed from each mosquito and the length from the axillary incision to the apical margin was measured using a digital camera imaging system (Moticam 2300) connected to a microscope eyepiece and pre-calibated software (Motic images plus v2.0).
Statistical analyses
Data obtained from this study were analyzed using generalized linear mixed models in R version 4.0.4. Generalized Linear Mixed Models (GLMM) were used to determine the significant factors explaining the differences in prevalence and infection intensity. The mixed models included treatment with re-suspended product, mosquito body size, blood meal gametocyte density, and replicate (n=3) as fixed variables, and individual mosquitoes (mosquitoID) as a random variable. The package DHARMa was used to check the fit of each model and to check for overdispersion (27). The package Effects was used to plot final GLMM model results (28, 29) and the Akaike information criterion (AIC) was used to select the best models.
For analysis of prevalence, the GLMM was fit by maximum likelihood with a binomial error distribution and logit link function using R package lme4 (30). Infection intensity (number of oocysts per mosquito) was modelled using glmmTMB (31) in R with the best fit and most parsimonious model from Poisson, negative binomial, zero-inflated negative binomial and a hurdle model of the zero-inflated negative binomial defined by comparison of AIC.
Results
Effect of bacterial bio-products on infection prevalence
We first determined if LB medium influences the parasite infection of mosquitoes and compared the results from the two experimental controls (Supplementary Tables 2, 3). There was no difference in prevalence of infection between controls with and without re-suspended LB medium (P=0.18). Therefore, we proceeded to compare bacterial bio-product test groups with LB controls alone (Table 1).
The effect of bio-products from Serratia and Enterobacter cultures on mosquito infection prevalence was determined using GLMM with mosquito size, gametocyte density, replicate and bio-product treatment as fixed effects. The best fit model included all fixed effects (Table 2). Bio-product from S. marcescens significantly reduced infection prevalence (P=4.02 x 10-5, estimate -2.818), whereas that from E. cloacae was similar to the LB control (P=0.929) (Figure 1). Infection prevalence correlated positively with mosquito size blood (winglength P=0.0487, estimate = 2.306), which was expected as larger mosquitoes take in larger volumes of blood and thus more gametocytes (Supplementary Figure S1A). Similarly, there was a significant positive correlation between gametocyte density in the blood meal and infection prevalence (P=0.0079, estimate = 0.576) (Supplementary Figure S1A).
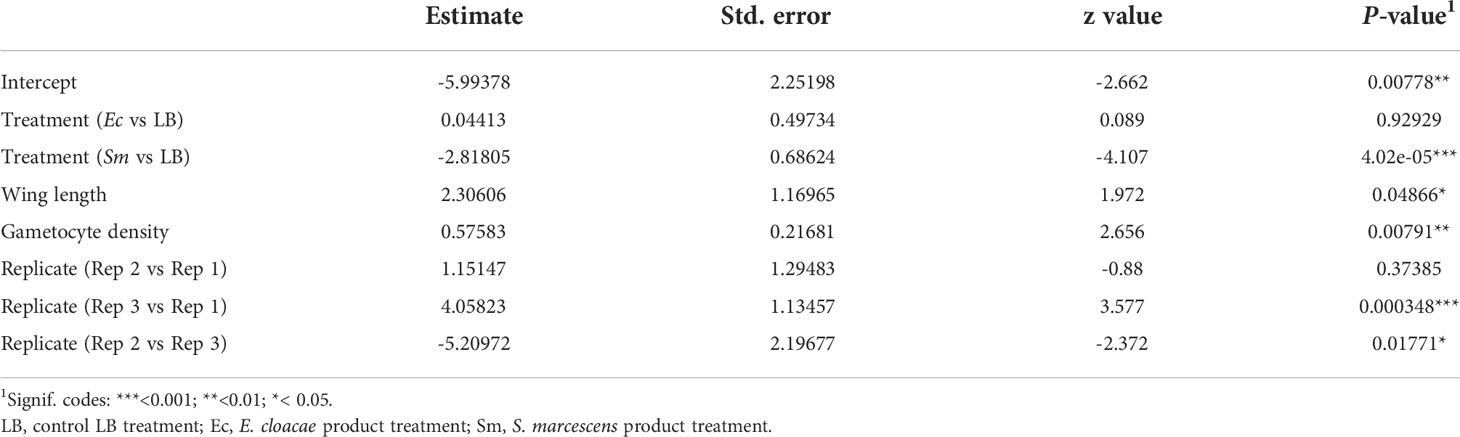
Table 2 Summary of significant variables from a generalised linear mixed model (GLMM) predicting parasite prevalence.
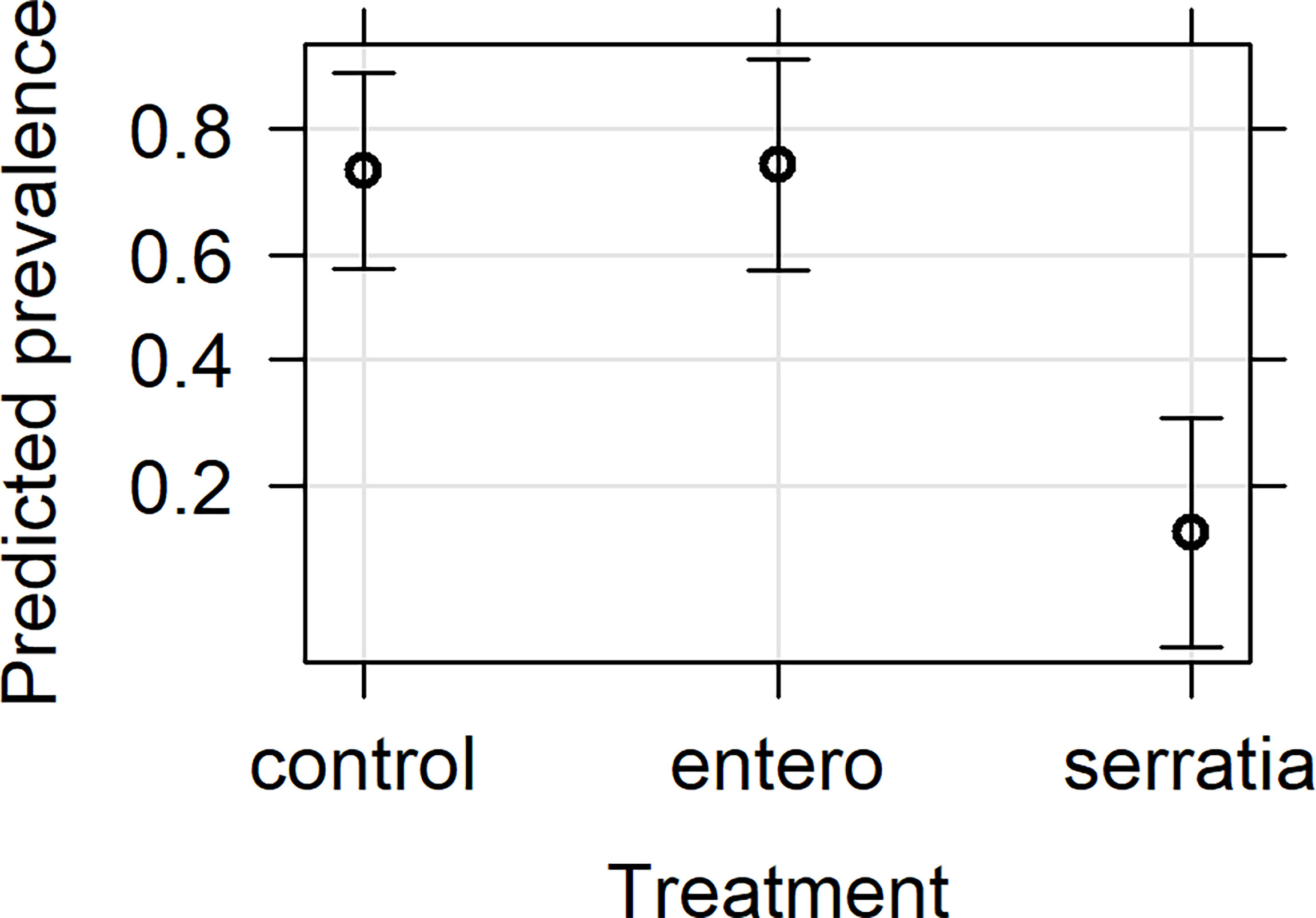
Figure 1 Effects plot of the generalised linear mixed model for infection prevalence following addition of bacterial bio-product to infected blood meal. The model included the fixed effects of treatment, mosquito wing length, gametocyte density in the blood meal and replicate, with the random effect of mosquito. The predicted infection prevalence from the fitted model is shown with 95% confidence intervals predicted from the model.
Effect of bacterial bio-products on oocyst intensity
The effect of E. cloacae and S. marcescens bio-product on oocyst numbers was determined by dissecting mosquitoes 10-11 days after ingesting infectious blood meal and counting oocysts (Table 1; Figure 2). Infection intensity (number of oocysts per mosquito) was modelled using a generalised linear mixed model in R, using package glmmTMB (31). The best fit model was a zero-inflated negative binomial model (nbinom2: variance is modelled as µ(1+ µ/ϕ)), and included the fixed effects of bio-product treatment and replicate, and mosquitoID as a random effect (Table 3). The mosquito wing length (P=0.34) and gametocyte density in the blood meal (P=0.53) were not significant predictors of oocyst intensity. As previously observed for infection prevalence, bio-product from S. marcescens significantly reduced oocyst intensity (P<2 x 10-16, estimate -3.0428) whereas that from E. cloacae did not (P=0.053) (Figure 3).
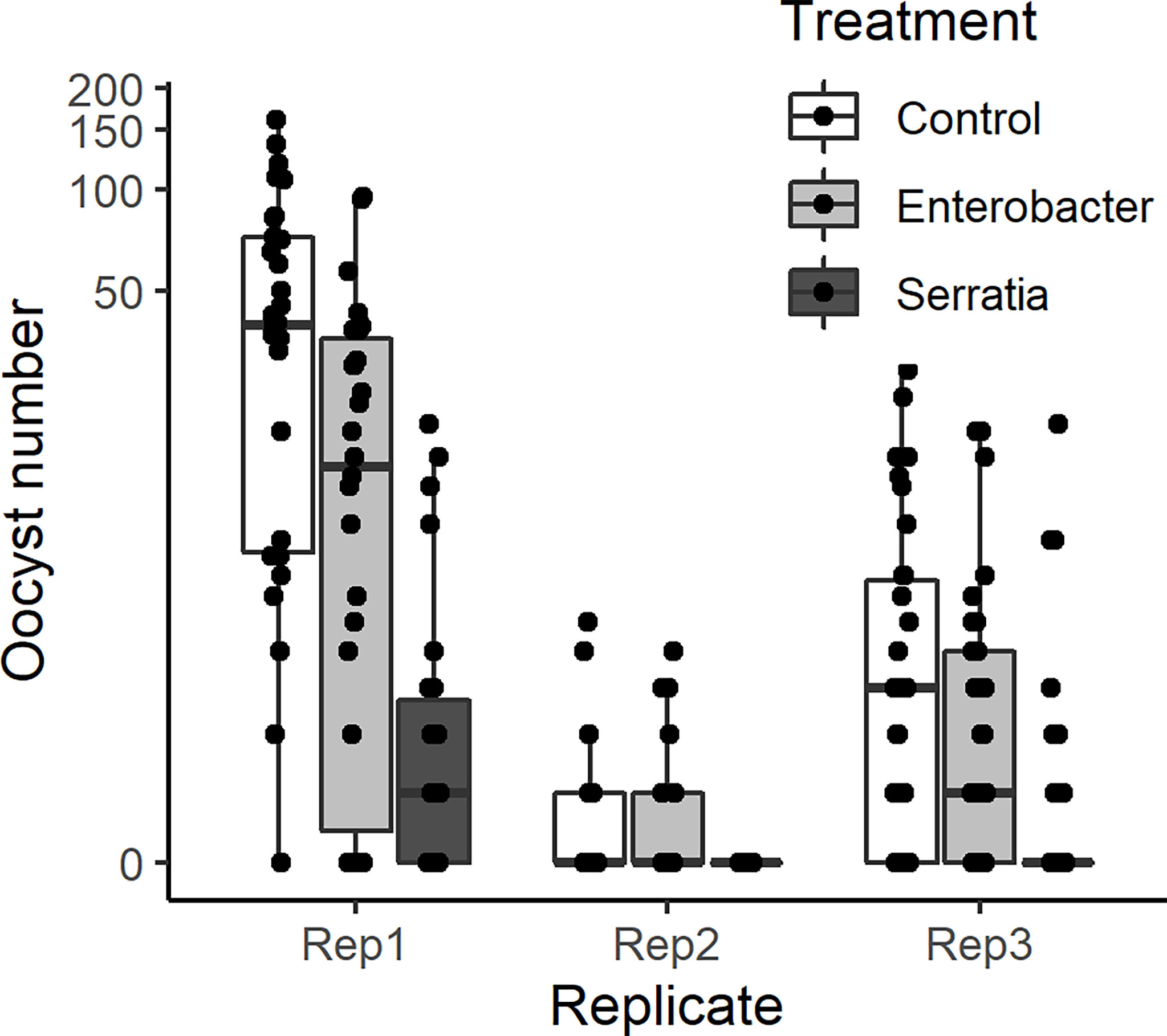
Figure 2 Intensity of infection (oocyst numbers) in mosquitoes fed with blood meal containing lyophilized medium from Enterobacter (Ec), Serratia (Sm) and Control (LB). Individual mosquito data points are shown as black dots. Boxes represent the 25th and 75th percentiles, with the median as a solid line. The whiskers represent the largest and smallest value no further than 1.5 x the interquartile range.
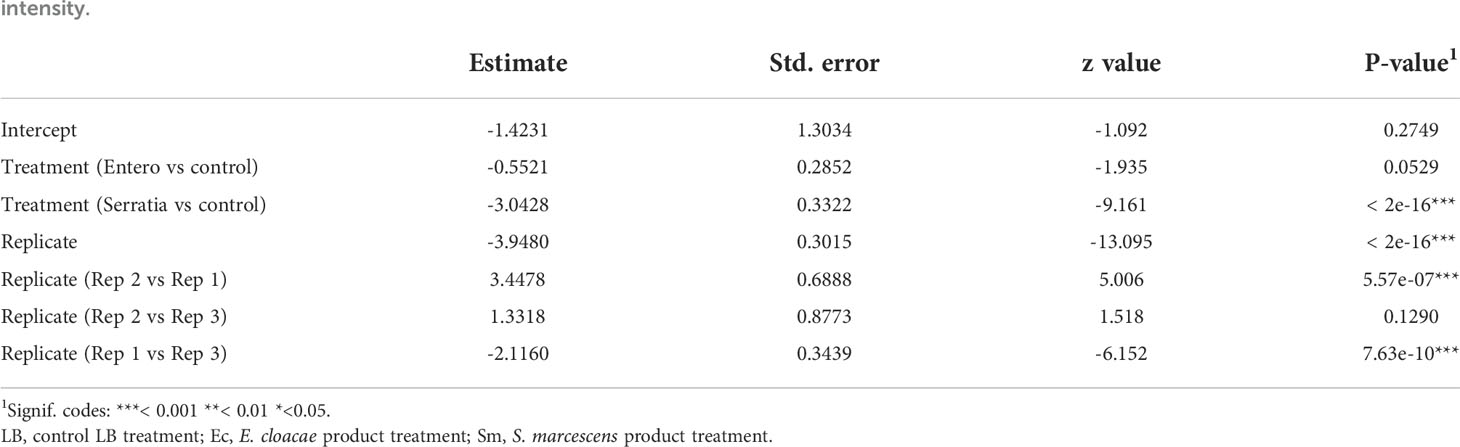
Table 3 Summary of significant variables from a zero-inflated negative binomial generalised linear mixed model (GLMM) predicting oocyst intensity.
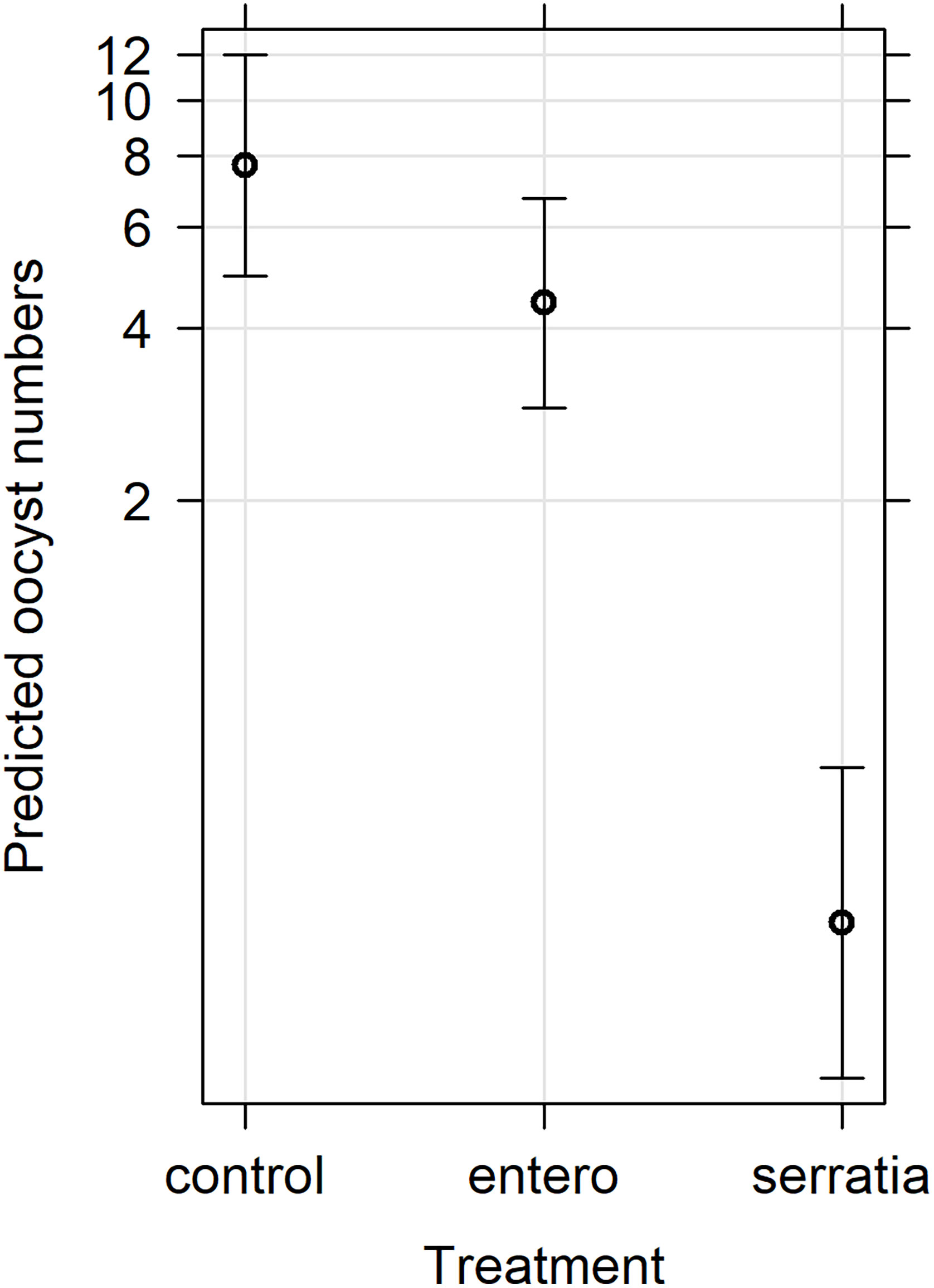
Figure 3 Effects plot of the generalised linear mixed model for oocyst numbers, with fixed effects of treatment and replicate, and the random effect of mosquito. The predicted oocyst numbers are shown with 95% confidence intervals predicted from the model.
Discussion
This study investigated the impact of aseptic bacterial products released into in vitro culture media on the mosquito stages of P. falciparum. Bacteria symbionts associated with mosquitoes are being intensively researched for their anti-parasitic and/or vector population suppression properties for new control methods. The two species of bacteria Enterobacter cloacae and Serratia marcescens were isolated from field-collected An. gambiae mosquitoes from Ghana. Serratia and Enterobacter are common bacterial species found in Anopheles mosquitoes, proliferate especially after blood meals (32, 33) and their impact on Plasmodium has been reported (11, 12, 20). These previous studies used live bacteria which were either re-introduced into mosquitoes prior to infectious blood meals or mixed with the blood meal containing the parasites (7, 11). Here we investigated if these bacteria release anti-parasitic products which can influence parasite development in mosquitoes in the absence of the bacterial cells. The material released into culture medium by the S. marcescens isolate was shown to reduce significantly both the prevalence and infection intensity of P. falciparum in An. gambiae mosquitoes. The blocking effect with S. marcescens product was observed in all experimental replicates with different infection prevalence and intensity. At lower prevalence in replicate 2, where infection prevalence in the control was 36%, S. marcescens product completely blocked mosquito infection. Despite the difference in the parasite infection levels obtained in the replicates, all three showed similar bacterial-mediated oocyst reduction effects. Variation in parasite intensity is a natural phenomenon and within the limits of this study, we have shown that the microbial effect may not be dependent on the parasite infection intensity. Although this is promising for control, additional studies will, however, be required to confirm these results.
All transmission reduction activity observed in this study occurred in mosquitoes with intact natural microbiota, suggesting a direct effect on the parasites, rather than through enhanced triggering of mosquito immune responses. It is also possible that the lyophilate could augment the mosquito immune response to the naturally occurring bacteria, or could affect their growth, resulting in lower infection levels. Many studies have focused on the innate immune system because as mosquitoes take in a blood meal, there is an increase in the microbial community in the midgut (12, 34, 35), which activate signalling pathways such as the Immune Deficiency (IMD) and Toll pathways, and reduce Plasmodium numbers in the midgut (10, 36). Experiments to assess bacterial effects on mosquito physiological processes and Plasmodium development often use aseptic mosquitoes that were cleared of residing bacteria by introduction of antibiotics through sugar-feeding (7, 12, 37, 38) or through creating axenic mosquitoes (39, 40). Using this aseptic procedure, an isolate of S. marcescens from a laboratory colony of An. gambiae effected reduction in P. falciparum infection intensity (12). A similar impact on P. berghei infection intensity was observed with S. marcescens adapted from field-caught mosquitoes in Burkina Faso (20). However the absence of competing natural bacteria in this experimental system may result in higher colony forming units of re-introduced bacteria. In vitro development of P. berghei ookinetes was reduced in the presence of S. marcescens (12, 20) but also with supernatants from bacterial liquid cultures (12), suggesting that secreted factors were involved in direct killing of ookinetes and oocysts. A related species, S. ureilytica, has been shown to produce an antimalarial lipase which can kill the parasite at the gametocyte and ookinete stages. Immunofluorescence assays revealed the lipase was detected on P. berghei ookinetes, suggesting a direct killing mechanism (15). Our study confirms the anti-Plasmodial activity of secreted products from S. marcescens and further shows their effectiveness without clearing or reducing the host microbial community. Further studies will however be required to demonstrate whether the anti-parasite mechanism of S. marcescens is solely due to the direct action of bacterial secreted products on the parasites (7, 41), or if recognition of S. marcescens in the midgut by the mosquito immune system and subsequent enhancement of antibacterial effectors also contributes to killing parasites.
We did not observe any significant transmission blocking activity with bioproducts from Enterobacter cloacae. Previous studies demonstrated that Enterobacter (Esp_Z) isolated from An. arabiensis in Zambia negatively affected ookinete development, but the anti-parasitic effect was reduced when the bacteria were heat-inactivated before re-introduction into mosquitoes (11). Filtered fresh Esp_Z culture supernatant was able to reduce P. berghei ookinete formation in vitro, and the authors concluded that parasite inhibition was mediated by production of reactive oxygen species (ROS), a short-lived bacterial-produced molecule. The lack of anti-parasite activity with E. cloacae culture supernatant in the current study could be because ROS may have been depleted at our 8-hour harvesting of bacterial bio-product or destroyed during the lyophilization process. Therefore, further investigations will be needed to determine if collection of supernatant at different time points (growth stages) of culture will impact on Plasmodium development, and/or if this E. cloacae isolate from An. gambiae s.l mosquitoes collected from Ghana produces ROS, as reported for Esp_Z (11).
Data availability statement
The original contributions presented in the study are included in the article/Supplementary Material. Further inquiries can be directed to the corresponding authors.
Author contributions
JA, LR-C conceptualized the study. EA, JA, LR-C designed the methodology. EA, PU, SP performed the experiments. EA, LR-C analyzed the data. EA wrote the first draft of the manuscript. All authors reviewed, edited and approved the final manuscript.
Funding
EA was supported by the University of Glasgow’s GCRF Small Grants Fund, an initiative supported by an allocation of Global Challenges Research Fund from the Scottish Funding Council under agreement SFC/AN/18/2020. This research was funded in part, by the Wellcome Trust [Grant number: 220737/Z/20/Z] to JA. PU was supported by the Tertiary Education Trust Fund (TETFund) of Nigeria.
Acknowledgments
We acknowledge the technical assistance of Elizabeth Peat, Dorothy Armstrong and Angela McBride, and thank Oyindamola Ekuntuyi for assistance with the laboratory work at the University of Glasgow.
Conflict of interest
The authors declare that the research was conducted in the absence of any commercial or financial relationships that could be construed as a potential conflict of interest.
Publisher’s note
All claims expressed in this article are solely those of the authors and do not necessarily represent those of their affiliated organizations, or those of the publisher, the editors and the reviewers. Any product that may be evaluated in this article, or claim that may be made by its manufacturer, is not guaranteed or endorsed by the publisher.
Supplementary material
The Supplementary Material for this article can be found online at: https://www.frontiersin.org/articles/10.3389/fitd.2022.979615/full#supplementary-material
References
1. Cotter C, Sturrock HJ, Hsiang MS, Liu J, Phillips AA, Hwang J, et al. The changing epidemiology of malaria elimination: New strategies for new challenges. Lancet (2013) 382(9895):900–11. doi: 10.1016/S0140-6736(13)60310-4
2. Gachelin G, Garner P, Ferroni E, Verhave JP, Opinel A. Evidence and strategies for malaria prevention and control: A historical analysis. Malar J (2018) 17(1):96. doi: 10.1186/s12936-018-2244-2
3. Lover AA, Baird JK, Gosling R, Price RN. Malaria elimination: Time to target all species. Am J Trop Med Hyg (2018) 99(1):17–23. doi: 10.4269/ajtmh.17-0869
5. Liu N. Insecticide resistance in mosquitoes: Impact, mechanisms, and research directions. Annu Rev Entomol. (2015) 60:537–59. doi: 10.1146/annurev-ento-010814-020828
6. Smith RC, Vega-Rodriguez J, Jacobs-Lorena M. The Plasmodium bottleneck: Malaria parasite losses in the mosquito vector. Mem Inst Oswaldo Cruz (2014) 109(5):644–61. doi: 10.1590/0074-0276130597
7. Dong Y, Manfredini F, Dimopoulos G. Implication of the mosquito midgut microbiota in the defense against malaria parasites. PloS Pathog (2009) 5(5):e1000423. doi: 10.1371/journal.ppat.1000423
8. Minard G, Mavingui P, Moro CV. Diversity and function of bacterial microbiota in the mosquito holobiont. Parasit Vectors (2013) 6:146. doi: 10.1186/1756-3305-6-146
9. Romoli O, Gendrin M. The tripartite interactions between the mosquito, its microbiota and Plasmodium. Parasit Vectors (2018) 11(1):200. doi: 10.1186/s13071-018-2784-x
10. Jupatanakul N, Sim S, Dimopoulos G. The insect microbiome modulates vector competence for arboviruses. Viruses (2014) 6(11):4294–313. doi: 10.3390/v6114294
11. Cirimotich CM, Dong Y, Clayton AM, Sandiford SL, Souza-Neto JA, Mulenga M, et al. Natural microbe-mediated refractoriness to Plasmodium infection in Anopheles gambiae. Science (2011) 332(6031):855–8. doi: 10.1126/science.1201618
12. Bahia AC, Dong Y, Blumberg BJ, Mlambo G, Tripathi A, BenMarzouk-Hidalgo OJ, et al. Exploring Anopheles gut bacteria for Plasmodium blocking activity. Environ Microbiol (2014) 16(9):2980–94. doi: 10.1111/1462-2920.12381
13. Bai L, Wang L, Vega-Rodriguez J, Wang G, Wang S. A gut symbiotic bacterium Serratia marcescens renders mosquito resistance to Plasmodium infection through activation of mosquito immune responses. Front Microbiol (2019) 10:1580. doi: 10.3389/fmicb.2019.01580
14. Pan X, Zhou G, Wu J, Bian G, Lu P, Raikhel AS, et al. Wolbachia induces reactive oxygen species (Ros)-dependent activation of the toll pathway to control Dengue virus in the mosquito Aedes aegypti. Proc Natl Acad Sci U.S.A. (2012) 109(1):E23–31. doi: 10.1073/pnas.1116932108
15. Gao H, Bai L, Jiang Y, Huang W, Wang L, Li S, et al. A natural symbiotic bacterium drives mosquito refractoriness to Plasmodium infection via secretion of an antimalarial lipase. Nat Microbiol (2021) 6(6):806–17. doi: 10.1038/s41564-021-00899-8
16. Povelones M, Waterhouse RM, Kafatos FC, Christophides GK. Leucine-rich repeat protein complex activates mosquito complement in defense against Plasmodium parasites. Science (2009) 324(5924):258–61. doi: 10.1126/science.1171400
17. Blandin S, Shiao SH, Moita LF, Janse CJ, Waters AP, Kafatos FC, et al. Complement-like protein Tep1 is a determinant of vectorial capacity in the malaria vector Anopheles gambiae. Cell (2004) 116(5):661–70. doi: 10.1016/s0092-8674(04)00173-4
18. Shiao SH, Whitten MM, Zachary D, Hoffmann JA, Levashina EA. Fz2 and Cdc42 mediate melanization and actin polymerization but are dispensable for Plasmodium killing in the mosquito midgut. PloS Pathog (2006) 2(12):e133. doi: 10.1371/journal.ppat.0020133
19. Dimopoulos G, Richman A, Muller HM, Kafatos FC. Molecular immune responses of the mosquito Anopheles gambiae to bacteria and malaria parasites. Proc Natl Acad Sci U.S.A. (1997) 94(21):11508–13. doi: 10.1073/pnas.94.21.11508
20. Bando H, Okado K, Guelbeogo WM, Badolo A, Aonuma H, Nelson B, et al. Intra-specific diversity of Serratia marcescens in Anopheles mosquito midgut defines Plasmodium transmission capacity. Sci Rep (2013) 3:1641. doi: 10.1038/srep01641
21. Ezemuoka LC, Akorli EA, Aboagye-Antwi F, Akorli J. Mosquito midgut Enterobacter cloacae and Serratia marcescens affect the fitness of adult female Anopheles gambiae s.l. PloS One (2020) 15(9):e0238931. doi: 10.1371/journal.pone.0238931
22. Shute GT. A method of maintaining colonies of East African strains of Anopheles gambiae. Ann Trop Med Parasitol (1956) 50(1):92–4. doi: 10.1080/00034983.1956.11685743
23. Walliker D, Quakyi IA, Wellems TE, McCutchan TF, Szarfman A, London WT, et al. Genetic analysis of the human malaria parasite Plasmodium falciparum. Science (1987) 236(4809):1661–6. doi: 10.1126/science.3299700
24. Carter R, Ranford-Cartwright L, Alano P. The culture and preparation of gametocytes of Plasmodium falciparum for immunochemical, molecular, and mosquito infectivity studies. Methods Mol Biol (1993) 21:67–88. doi: 10.1385/0-89603-239-6:67
25. Koella JC, Lyimo EO. Variability in the relationship between weight and wing length of Anopheles gambiae (Diptera: Culicidae). J Med Entomol. (1996) 33(2):261–4. doi: 10.1093/jmedent/33.2.261
26. Lyimo EO, Takken W. Effects of adult body size on fecundity and the pre-gravid rate of Anopheles gambiae females in Tanzania. Med Vet Entomol. (1993) 7(4):328–32. doi: 10.1111/j.1365-2915.1993.tb00700.x
27. Hartig F. Dharma: Residual diagnostics for hierarchical (Multi-level / mixed) regression models. 0.4.5. R package (2022). Available at: http://florianhartig.github.io/DHARMa/
28. Fox J. Effect displays in R for generalised linear models. J Stat Softw. (2003) 8(15):1–27. doi: 10.18637/jss.v008.i15
29. Fox J, Weisberg S. An R companion to applied regression. 3rd ed. Thousand Oaks, CA, USA: Sage (2019).
30. Bates D, Mächler M, Bolker B, Walker S. Fitting linear mixed-effects models using Lme4. J Stat Softw. (2015) 67(1):1–48. doi: 10.18637/jss.v067.i01
31. Brooks ME, Kristensen K, van Benthem KJ, Magnusson A, Berg CW, Nielsen A, et al. GlmmTMB balances speed and flexibility among packages for zero-inflated generalized linear mixed modeling. R J (2017) 9(2):378–400. doi: 10.32614/RJ-2017-066
32. Rani A, Sharma A, Rajagopal R, Adak T, Bhatnagar RK. Bacterial diversity analysis of larvae and adult midgut microflora using culture-dependent and culture-independent methods in lab-reared and field-collected Anopheles stephensi-an Asian malarial vector. BMC Microbiol (2009) 9:96. doi: 10.1186/1471-2180-9-96
33. Gonzalez-Ceron L, Santillan F, Rodriguez MH, Mendez D, Hernandez-Avila JE. Bacteria in midguts of field-collected Anopheles albimanus block Plasmodium vivax sporogonic development. J Med Entomol. (2003) 40(3):371–4. doi: 10.1603/0022-2585-40.3.371
34. Sharma P, Rani J, Chauhan C, Kumari S, Tevatiya S, Das De T, et al. Altered gut microbiota and immunity defines Plasmodium vivax survival in Anopheles stephensi. Front Immunol (2020) 11:609. doi: 10.3389/fimmu.2020.00609
35. Rodgers FH, Gendrin M, Wyer CAS, Christophides GK. Microbiota-induced peritrophic matrix regulates midgut homeostasis and prevents systemic infection of malaria vector mosquitoes. PloS Pathog (2017) 13(5):e1006391. doi: 10.1371/journal.ppat.1006391
36. Saraiva RG, Kang S, Simoes ML, Anglero-Rodriguez YI, Dimopoulos G. Mosquito gut antiparasitic and antiviral immunity. Dev Comp Immunol (2016) 64:53–64. doi: 10.1016/j.dci.2016.01.015
37. Kalappa DM, Subramani PA, Basavanna SK, Ghosh SK, Sundaramurthy V, Uragayala S, et al. Influence of midgut microbiota in Anopheles stephensi on Plasmodium berghei infections. Malar J (2018) 17(1):385. doi: 10.1186/s12936-018-2535-7
38. Ramirez JL, Short SM, Bahia AC, Saraiva RG, Dong Y, Kang S, et al. Chromobacterium Csp_P reduces malaria and Dengue infection in vector mosquitoes and has entomopathogenic and in vitro anti-pathogen activities. PloS Pathog (2014) 10(10):e1004398. doi: 10.1371/journal.ppat.1004398
39. Correa MA, Matusovsky B, Brackney DE, Steven B. Generation of axenic Aedes aegypti demonstrate live bacteria are not required for mosquito development. Nat Commun (2018) 9(1):4464. doi: 10.1038/s41467-018-07014-2
40. Steven B, Hyde J, LaReau JC, Brackney DE. The axenic and gnotobiotic mosquito: Emerging models for microbiome host interactions. Front Microbiol (2021) 12:714222. doi: 10.3389/fmicb.2021.714222
Keywords: mosquito midgut microbiota, bacterial bio-products, Enterobacter cloacae, Serratia marcescens, Anopheles gambiae s.l, Plasmodium falciparum, disease transmission blocking
Citation: Akorli EA, Ubiaru PC, Pradhan S, Akorli J and Ranford-Cartwright L (2022) Bio-products from Serratia marcescens isolated from Ghanaian Anopheles gambiae reduce Plasmodium falciparum burden in vector mosquitoes. Front. Trop. Dis 3:979615. doi: 10.3389/fitd.2022.979615
Received: 27 June 2022; Accepted: 14 September 2022;
Published: 29 September 2022.
Edited by:
Kounbobr Roch Dabiré, Research Institute for Health Science-Direction Régionale de l’Ouest (IRSS-DRO), Burkina FasoReviewed by:
Olivier Gnankine, University of Ouagadougou, Burkina FasoRajnikant Dixit, National Institute of Malaria Research (ICMR), India
Copyright © 2022 Akorli, Ubiaru, Pradhan, Akorli and Ranford-Cartwright. This is an open-access article distributed under the terms of the Creative Commons Attribution License (CC BY). The use, distribution or reproduction in other forums is permitted, provided the original author(s) and the copyright owner(s) are credited and that the original publication in this journal is cited, in accordance with accepted academic practice. No use, distribution or reproduction is permitted which does not comply with these terms.
*Correspondence: Jewelna Akorli, amFrb3JsaUBub2d1Y2hpLnVnLmVkdS5naA==; amV3ZWxuYS5ha29ybGlAZ21haWwuY29t; Lisa Ranford-Cartwright, TGlzYS5SYW5mb3JkLUNhcnR3cmlnaHRAZ2xhc2dvdy5hYy51aw==
†These authors contributed equally to this work and share last authorship