- 1Institute for Parasitology, Center for Infection Medicine, University of Veterinary Medicine Hannover, Hannover, Germany
- 2Research Center for Emerging Infections, and Zoonoses, University of Veterinary Medicine Hannover, Hannover, Germany
- 3Fish Disease Research Unit, Institute for Parasitology, University of Veterinary Medicine Hannover, Hannover, Germany
The current rapidly advancing climate change will affect the transmission of arthropod-borne viruses (arboviruses), mainly through changes in vector populations. Mosquitos of the Culex pipiens complex play a particularly prominent role in virus transmission in central Europe. Factors that contribute to the vector population density and the ability of those vectors to transmit viral pathogens (vector competence) can include nutrition during the larval stages. To test the influence of larval diet on larval survival and adult emergence, as well as vector competence, several diets varying in their nutritional composition were compared using a newly established assay. We tested the effects of 17 diets or diet combinations on the fitness of third-instar larvae of Culex pipiens biotype molestus. Larval survival rates at day 7 ranged from 43.33% to 94.44%. We then selected 3 of the 17 diets (Tetra Pleco, as the routine feed; JBL NovoTab, as the significantly inferior feed; and KG, as the significantly superior feed) and tested the effect of these diets, in combination with Culex Y virus infection, on larval survival rate. All Culex Y virus-infected larvae showed significantly lower larval survival, as well as low pupation and adult emergence rates. However, none of the tested diets in our study had a significant impact on larval survival in combination with viral infection. Furthermore, we were able to correlate several water quality parameters, such as phosphate, nitrate, and ammonium concentration, electrical conductivity, and low O2 saturations, with reduced larval survival. Thus, we were able to demonstrate that Culex Y virus could be a suitable agent to reduce mosquito population density by reducing larval density, pupation rate, and adult emergence rate. When combined with certain water quality parameters, these effects can be further enhanced, leading to a reduced mosquito population density, and reduce the cycle of transmission. Furthermore, we demonstrate, for the first time, the infection of larvae of the mosquito Culex pipiens biotype molestus with a viral pathogen.
1 Introduction
Mosquitos of the Culex pipiens complex are now native to Europe, America, Africa, and Asia, and transmit, among others, the West Nile and Sindbis viruses (1). The medical importance of these viruses justifies the urgent further investigation of possible control measures. One recently introduced strategy to control mosquito populations is the destabilization of populations by the use of natural agents such as entomopathogenic bacteria, fungi, or insect-specific viruses, or a combination of these. Such a strategy enables the amount of insecticides used to be reduced (2).
The mosquito developmental cycle includes an aquatic phase, during which the egg develops into the pupa. During this stage, water quality, nutrient richness, and the presence in the water of microorganisms, including algae, protozoa, bacteria, or viruses, have a great influence on mosquito populations. Nutrition is of particular importance; however, little is known about the nutritional needs of Mosquitos. Most studies on nutrition, and its effect on larval and adult body traits, have been conducted using Aedes aegypti L (3–5). Based on current knowledge, mosquito larvae appear to be able to adapt to their environment in terms of nutrient sources, feeding on microorganisms such as bacteria, protozoa, algae, and small metazoans, as well as non-living materials such as dust and organic detritus, depending on the abundance of food sources in the habitat (6, 7). In addition, cases of cannibalism, within and between larval instars (8), and necrophagy (9) have regularly been observed.
The nutritional intake of mosquito larvae can be divided into macronutrients and micronutrients, which serve energetic and non-energetic purposes, respectively. From an analytical point of view, the main nutritional requirements for mosquito larvae are carbohydrates and proteins, with fats playing a minor role (10). Malnourishment leads to delayed larval development, low energetic reserves in adults, reduced body size, reduced fecundity, and reduced adult emergence (11). Prior to pupation, larvae need to accumulate sufficient caloric reserves, and these are positively correlated with weight. Only larvae reaching the critical mass (defined as the body mass at which 50% of larvae pupate) can develop into pupae. This critical mass depends on several factors, such as temperature, species (12), and nutritional resources (13). Cx. tarsalis larvae show increased development time, decreased pupation and emergence rates, and smaller adult female body size under nutritional stress caused by food deprivation. Ae. aegypti larvae show a similar response to nutritional stress (14), and, in Anopheles darlingi larvae fed ground fish food, higher quantities were associated with a higher survival rate and faster development (15). In contrast, in An. gambiae and An. coluzzii larvae fed Tetramin® baby fish food, higher amounts led to rapid development but a lower survival rate (16). This inconsistency shows that not only availability of nutritional resources, but also larval competition (17) and water quality (18), have an impact on larval survival and pupation and emergence rates.
Carbohydrates, in addition to their described effects on larval growth, pupation, survival, flight, and egg production (19), are also associated with vector competence for Plasmodium in Anopheles Mosquitos by modifying mosquito–microbiota interactions (20). The term microbiota is used to describe the microbial populations living in contact with the body epithelia. The microbiota is composed of bacteria, protists, yeasts, and viruses, which are required for mosquito development (21). Advancements in high-throughput sequencing and metagenomics and intensified mosquito surveillance have led to the discovery of not only new arboviruses, but also a large number of insect-specific viruses (ISVs) that are a part of the virobiota of Mosquitos. In contrast to arboviruses, ISVs cannot be transmitted to vertebrates and are restricted to arthropods. The main route of transmission of ISVs is thought to be vertically, through transovarial infection of offspring, which is supported by laboratory data (22, 23). Other transmission routes include transovum transmission, venereal transmission, and transmission to adult mosquitoes during nectar feeding on infected plants (24) or to mosquitos during the early stages of development during feeding on infected material present in the aquatic habitat (25). ISVs are of particular research interest, as data suggest that these viruses could function as a tool for vector control: it has been shown that, for example, the Espirito Santo virus (ESV)—also an ISV—inhibits the replication of dengue virus (DENV) in Ae. aegypti (26). In addition, this effect has been observed with different combinations of ISVs and arboviruses (27, 28).
This raises the question of whether or not the nutrition state of mosquito larvae has an impact on the interaction of the mosquito with ISVs and, if so, how this interaction affects larval survival, pupation, and adult emergence. To the best of our knowledge, no study has yet focused on the impact of larval diet on the susceptibility to virus infection of Mosquitos at the larval stage. To tackle this question, we investigated the effect of different laboratory larval diets on survival and pupation and adult emergence rates, as well as the impact of those diets on survival in combination with the ESV-related virus Culex Y virus (CYV; both genus Entomobirnavirus, family Birnaviridae), in Cx. pipiens biotype molestus Mosquitos. CYV was identified in 2010 in free-living Cx. pipiens Mosquitos (29) as well as in persistently infected mosquito cell cultures (30). It was shown that this ISV is not mosquito specific under laboratory conditions, as it also replicates in Drosophila melanogaster, but it does not replicate in honeybees and locusts, or in mammalian cells (BHK-21 and A549). Furthermore, the virus has been found to replicate in the reproductive organs of adult Cx. pipiens biotype molestus Mosquitos but, interestingly, no vertical transmission to the offspring occurs. Persistence in free-living Mosquitos is therefore presumed to be by horizontal transmission (31). Consequently, this study will also investigate the replication of the virus in the larval stage. This is the first described attempt to deliver a viral pathogen by injection into larval stages of Cx. pipiens biotype molestus.
2 Material and methods
2.1 Mosquito colony
All experiments were conducted using a cross-bred strain of Cx. pipiens biotype molestus (Forskal, 1775) from Langenlehsten and Wendland [described in (32)]. The strain was maintained in climate-controlled chambers at 25–26°C, 45%–65% RH, with a photoperiod of 16:8 (light–dark; separated by 1 hour of crepuscular periods; daylight 1,600 lx). Plastic basins (37 cm × 30 cm × 7 cm) filled with dechlorinated tap water were used to rear larvae until hatching, and larvae were fed ad libitum with Tetra Pleco fish food (Tetra Werke, Melle, Germany). Hatched adults were released into BugDorm-1 rearing cages (30 cm × 30 cm × 30 cm). They were fed ad libitum with 8% (weight/volume) fructose solution supplemented with 0.5 g/L 4-aminobenzoic acid, and once a week with a 4:1 mixture of animal blood and an 8% fructose solution after 24 h starvation.
Mosquito colonies were naturally infected with Wolbachia, and tested negative for Togaviridae, Phleboviridae, and Flaviviridae (data not shown). Primer sets and thermic conditions for amplification of the four PCR assays have been published previously (33–36).
2.2 Diets
The diets tested in this study included fish, cat, and dog food, as well as corn flour (Table 1). Apart from maize flour, no diet listed the carbohydrate content (Table 2). Therefore, we calculated the carbohydrate content from the other available values as follows:
If the moisture content was not specified, a standardized content of 12% was assumed, as the Food and Drug Administration of the USA (FDA) defines a dry food as food consisting of approximately 88%–90% dry matter (37). Crude proteins (ranging from 7.7% for corn meal up to 45% for algae wafers) and carbohydrates (ranging from 17.10% for algae wafers to 74.0% for corn meal) were the two constituents present in the greatest proportions in all tested food types. Crude fat (ranging from 0.8% to 20.0%), crude ash (ranging from 2.5% to 17.0%), and crude fiber (ranging from 0.82% to 7.0%) accounted for lower proportions of all food types (Tables 1, S1, S2).
All diets were ground to a fine powder with a mortar and pestle and mixed with dechlorinated tap water to produce a liquid diet with a concentration of 0.05 mg/µL. Larval diets and rearing water were not sterilized before the experiments (treatment groups and control groups) in order to assess the imported microbial impact (water quality) in addition to the nutritive effect.
2.3 Infection of larvae
Larvae were infected at the third- and fourth-instar stages. Larval stage was determined based on size and thorax color. If the thorax was dense and opaque, the larvae were classified as L4 instars (L4) and, if the thorax was more translucent and narrower, the larvae were classified as L3 instars (L3). Third-/fourth-instar larvae were immobilized by cold prior to injection. First, larvae were placed in ice-cold, non-sterilized, dechlorinated tap water (approximately 4°C). After 3–5 minutes, the larvae were transferred onto the lid of a closed glass Petri dish containing ice-cold water to maintain cold immobilization during injection. The excess water was reduced to a minimum with paper strips to optimize immobilization during injection. The larvae were oriented with their dorsal side upwards and injected using a Nanoject II micro-injector (Drummond Scientific) with 23.0 nL (1.2 × 105 RNA copies per 23.0 nL) of CYV in Schneider’s Drosophila Medium or with Schneider’s Drosophila Medium alone as a control. The CYV injection solution used was either the CYV isolate (C6/36 cell line) of Franzke et al. (2018) (30). Furthermore, glass capillary-pricked and non-treated control groups were generated. For an improved survival rate of the larvae after injection, we introduced the capillary cranially into the thoracic segment (Figure 1).
2.4 Growth kinetics of CYV in larvae
At 0, 3, and 5 days post injection (dpi), animals were characterized as larvae, pupae, or adults and frozen at –18°C. To ensure that data were comparable, larvae that had already developed into pupae or adults were excluded. Up to five larvae per time point (L3, n = 45; and L4, n = 36) were tested individually for CYV RNA copy numbers using real-time quantitative PCR (RT-qPCR). Larvae were homogenized in 500 µL Schneider’s Drosophila Medium (PAN-Biotech GmbH, Aidenbach, Germany) using a TissueLyser II (Qiagen, Hilden, Germany) and two 5-mm steel beads (Isometall, Pleidelsheim, Germany), and crushed for 30 s at 30 oscillations/s. The viral RNA was isolated using a QIAamp Viral RNA Kit (Qiagen, Hilden, Germany) in accordance with the manufacturer’s instructions.
CYV infections were validated by RT-qPCR with CYV-specific primers [forward: OSM_318 (5′-CCAGAGAATGTGAAGAGA-3′); reverse: OSM_319 (5′-CGTTGTTAAGGAAGACTC-3′); probe: OSM_320 (FAM-CACAAGAGGATACACAAGCAGCG-BHQ1)] in a 10 µL reaction volume using a Luna® Universal Probe One-Step RT-qPCR Kit (New England Biolabs, Ipswich, MA, USA), in accordance with the manufacturer’s instruction, and an AriaMx Real-time PCR System (Agilent). The thermal cycler profile was as follows: reverse transcription at 55°C for 10 minutes followed by an initial denaturation at 95°C for 1 minute, and 40 cycles of 95°C for 10 s and 54°C for 30 s. Viral RNA copies were quantified using a standard dilution series of a CYV-specific RNA fragment that was produced from a PCR product generated by a SuperScript III One-Step RT-PCR Platinum Taq DNA Polymerase Kit (Thermo Fisher) and the CYV-specific primers OSM_321 (5′-GGCAATGTTCGGGCTCATC-3′) and OSM_322 (5′-CGACAGTGCAGGAGTAGGGG-3′). PCR was performed in a PeqSTAR/Peqlab cycler with the following thermal profile: 1 minute at 60°C and 45 minutes at 50°C, followed by 2 minutes at 95°C for heat inactivation of the reverse transcriptase. This was followed by 40 cycles of 94°C (15 s), 59°C (30 s), and 68°C (30 s). A final elongation step was included in the PCR program (68°C, 7 min). The resulting PCR product was then purified with NucleoSpin Gel and a PCR clean-up kit (Macherey & Nagel), in accordance with the manual. For transcription, a second PCR was performed, during which a T7 promoter was added to the PCR product. For this purpose, a T7 promoter was added to the standard OSM_321 forward primer: OSB_728 (5′-TAATACGACTCACTATAGGGGGCAATGTTC-3′). The RNA concentration was measured, and the number of RNA copies/mL calculated.
2.5 Larval diet assay
In this assay, we analyzed the impact of larval nutrition on survival rate, pupation rate, and the emerging adult rate. For each diet, five L3 larvae and 10 mL of stale tap water were placed into each well of a six-well plate (CytoOne® STARLAB International). Larvae were fed with liquid diets from solid feed tablets that form a final concentration of 0.05 mg/μL only when combined with water. All liquid diets were mixed vigorously by repeated inversion before the mixture was pipetted into the different wells to ensure that each well received the same concentration of the diet. Assays were run in climate-controlled chambers using identical parameters as described above for mosquito colony keeping. Larval survival was checked daily by carefully poking at the bodies with a curved dissection needle. Dead larvae were removed. Pupated larvae were transferred into a Drosophila tube until emergence (Figure 2). Feed levels were not adjusted for decreasing larval density (by pupation/mortality) during the course of the experiment, to keep the parameters for the water quality analysis stable. As the food was provided in excess, no difference should be created by the loss of larvae during the course of the experiment.
2.5.1 Screening of 16 diets in untreated larvae
We screened 16 different diets for larval survival after the administration of a single 10-mg dose (200 µL of diet at a concentration of 0.05 mg/µL) at the beginning of the assay (all diets are presented in Table 1). A pilot experiment lasting 7 days was carried out to evaluate the initial survival rates of larvae fed each of the diets. In total, each diet was tested with 90 larvae: three replicates, each of 30 larvae. The larvae used in the different replicates of this assay were from at least two different generations. Pure water served as a negative control and Tetra Pleco fish food tablets as a positive control.
2.5.2 Partial replacement of Tetra Pleco tablets with corn flour in untreated larvae
We tested the effect of partial replacement of the Tetra Pleco diet with corn flour. In these experiments, larvae received 10 mg of Tetra Pleco diet on day 0 and 5 mg of corn flour on days 3 and 6. As a control, we included experiments without food as negative controls, and experiments with 10 mg of Tetra Pleco on day 0 and 5 mg of Tetra Pleco on days 3 and 6 as positive controls. Each diet was tested with 90 larvae: three replicates, each of 30 larvae. The experiments ran over 7 days and the larval survival rate was evaluated daily.
2.5.3 Refined testing of three diets in CYV-infected larvae
Based on the results of the experiment described in section 2.5.1, we performed a further, more refined, test using Tetra Pleco as a positive control, KG as a means of improving the larval survival rate, JBL Novo Tab as a means of reducing the larval survival rate, and water as a negative control. Larval diet assays were conducted here with four different groups: (a) CYV-injected larvae, (b) Schneider’s medium-injected larvae, (c) glass capillary-pricked larvae, and (d) untreated larvae. Injections and pricking were performed as described above. For each treatment group and each food type, 45 larvae were tested in three separated replicates. Larval survival until 7 dpi was analyzed as validation of the experiment described in section 2.5.1. In the refined testing, we also monitored the pupation rate and the adult emergence rate of each surviving larva. In order to prevent undersupply resulting from the fact that the experiment lasted longer than the experiment described in section 2.5.1, as well as a deterioration of the water quality owing to an oversupply of feed, a higher frequency and lower quantity of feed was selected here: every day until pupation, a 5-mg dose (100 µL of a diet at a concentration of 0.05 mg/µL) of the diets was added to every well. The CYV infection was validated for a representative 11% of the virus-infected animals.
2.6 Water quality analysis
Phosphate, nitrate, and ammonium concentrations were analyzed by using commercially available Spectroquant® kits (Merck KGaA, Darmstadt, Germany; phosphate kit 1.14848, nitrate kit 1.14773, ammonium kit 1.14752) photometrically with the Spectroquant® Move 100. For the sake of simplicity, we will use ammonium as a term for the ammonium/ammonia present in the water in a dissociation equilibrium. To determine the total hardness of the water, the sera gH-Test (sera GmbH, Heinsberg, Germany) was used in accordance with the manual. Oxygen and pH levels were measured with the relevant probes, and electrical conductivity was measured with an HQ40d two-channel multimeter (Hach Lange GmbH, Berlin Germany). All parameters were measured twice for each named diet in this experiment: on day 0, i.e., before the larvae were placed in water, and on day 7 after the larvae were reared in water.
2.7 Statistical analysis
All statistical analyses were performed using Prism 9 Version 9.4.0 (San Diego, CA, USA, www.graphpad.com). To compare larval survival between the different diets, Kaplan–Meier survival curves were generated and significance levels between curves were determined using the log-rank test. Pupation and emergence percentages were first tested for normality using the Shapiro–Wilk test. The dataset was then examined for significant differences between the various groups through t-tests with Welch’s correction. The same procedure was applied to all other parametric data. Non-parametric data were first tested for significant differences with the Kruskal–Wallis test and then compared pairwise using the Mann–Whitney test (non-parametric t-test) if significant differences were found.
3 Results
3.1 Growth kinetics of CYV in larvae
We were able to demonstrate a significant replication of CYV RNA in Cx. pipiens biotype molestus larvae after injection (Figure 1). On the day of injection (0 dpi), a mean RNA copy number of 9.69 × 103 was detected in L3 larvae, and of 8.96 × 103 in L4 larvae. At 3 dpi, the mean RNA copy number of L3 larvae tested increased to 5.57 × 107 and that of L4 larvae increased to 9.64 × 107. At 5 dpi, L3 larvae tested showed a slightly increased RNA copy number, of 1.02 × 108, and L4 larvae showed an increased RNA copy number, of 1.14 ×108. Overall, RNA copy numbers did not differ significantly between the two larval stages (Figure 3).
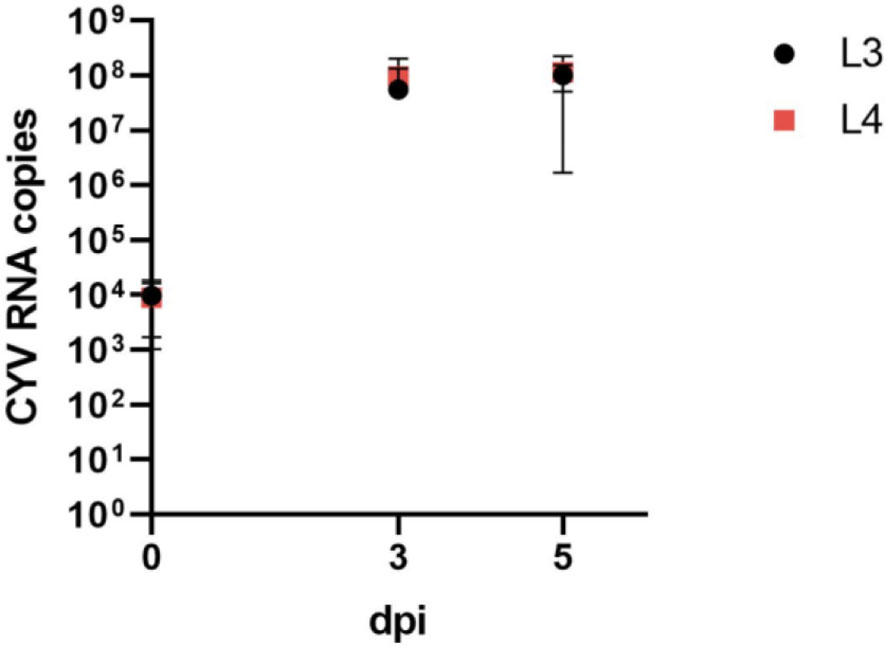
Figure 3 Comparison of viral growth as determined by mean (here shown with standard deviation) RNA copy numbers of Culex Y virus (CYV) in L3 instar (L3) and L4 instar (L4) larvae. Larvae were tested on the day of injection (0 dpi) and on 3 dpi and 5 dpi. RNA copy numbers refer to one tested larva. Black, L3 larvae; red, L4 larvae. dpi, days post-injection.
3.2 Larval diet assay
3.2.1 Screening of 16 diets in untreated larvae
Of the 16 analyzed diets, five (KG, KB, FG, adhesive tablets green, and Bosch Adult with Lamb & Rice) resulted in a significantly better larval survival rate than the positive control, Tetra Pleco (Table 2). Notably, all five diets that enhanced larval survival belonged to the fish food type group. Seven diets were associated with significantly lower survival rate than Tetra Pleco, all dog or cat foods (Happy Dog Profi-Line Basic, Happy Dog Profi-Line High Energy, and Happy Cat Minkas Hairball Control Poultry) and four fish food tablets (algae wafers, JBL NovoTab, Teich-Sticks Premium Color, and AWA’s TEI-KO-Mix Spezial) (Table 3).
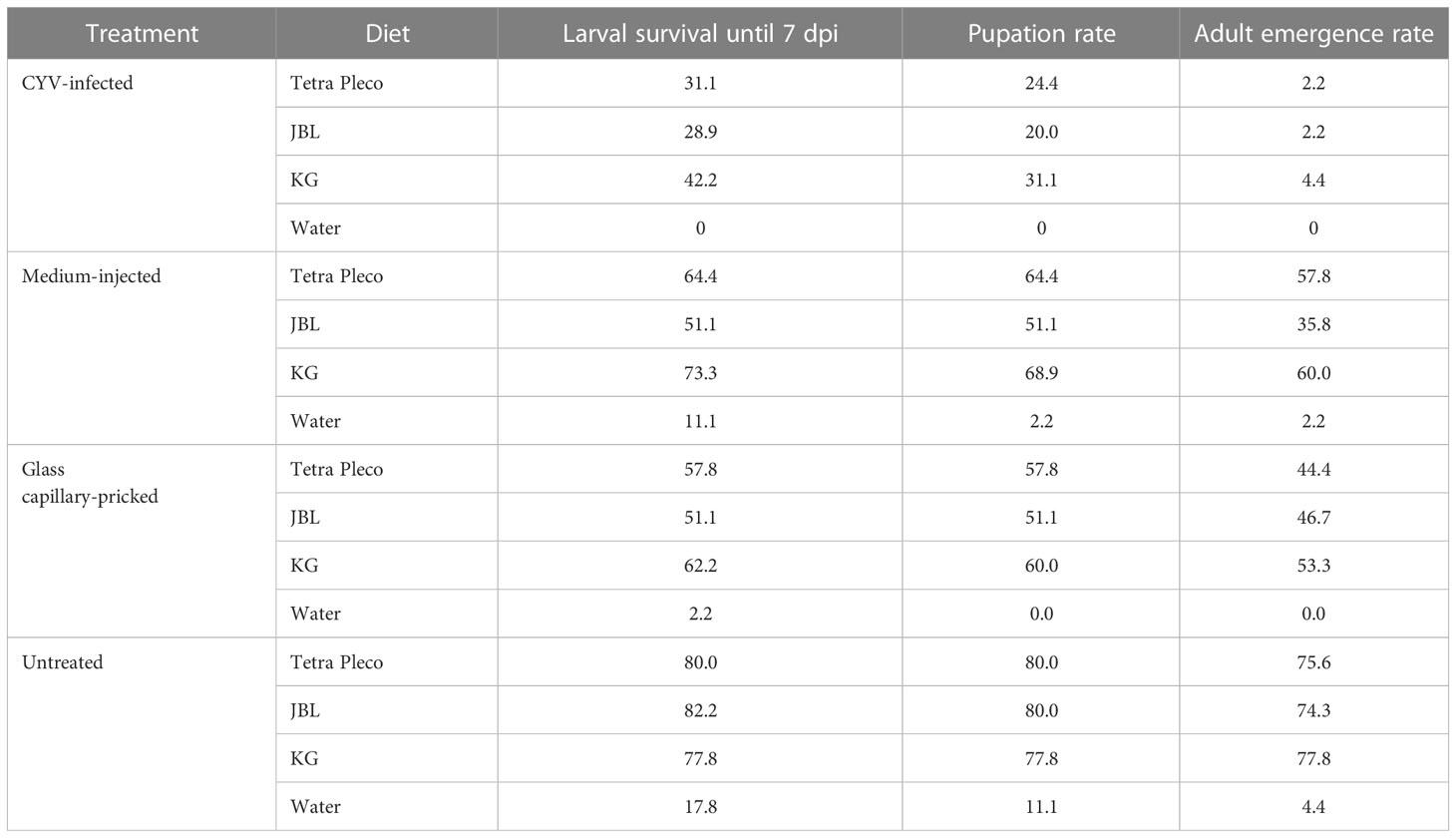
Table 2 Results of the refined larval diet test in Culex Y virus (CYV)-infected larvae and the control groups (medium-injected, glass capillary-pricked, and untreated larvae).
3.2.2 Partial replacement of Tetra Pleco tablets with corn flour in untreated larvae
In a second experiment, we tested if providing larvae with corn flour on days 3 and 6 in addition to an initial feed with Tetra Pleco on day 0 increased larval survival. In this experimental set, feeding the Tetra Pleco diet on day 0 resulted in 74.4% larval survival at 7 dpi; the addition of corn flour on days 3 and 6 increased larval survival to 90% at 7 dpi (log-rank test; p = 0.0090).
3.2.3 Refined testing of three diets in CYV-infected larvae
Next we infected larvae with CYV and compared their survival, pupation, and adult emergence rates under three different diets: Tetra Pleco as a positive control, JBL NovoTab as a significantly inferior feed, and KG as a significantly superior feed. Pricking showed a significant effect on larval survival, pupation, and adult emergence rates. The survival rate of glass capillary-pricked larvae was reduced to 57.0% (p<0.05; Welch’s t-test), pupation rate to 56.3% (p<0.01; Welch’s t-test), and adult emergence rate to 75.6% (p<0.01; Welch’s t-test) compared with untreated larvae (mean of all feeding groups; Table 2; Figure 4). In contrast, although a trend toward poorer tolerability of the treatment was seen, no significant differences between medium-injected larvae and the untreated control larvae were detected. The CYV-infected larvae showed the lowest larval survival rates (mean survival of 34.1% for all food groups excluding the water group). These results were significant compared with the medium-injected group (mean survival rate of 62.9% for all foods) (Welch’s t-test, p< 0.05), the glass capillary-pricked group (mean survival rate of 57% for all foods excluding the water group) (Welch’s t-test, p<0.05), and the untreated group (mean survival rate of 80% for all foods excluding the water group) (Welch’s t-test, p< 0.01) (results summarized in Table 2; Figure 4). In addition, pupation rate and adult emergence rate were significantly lower in the CYV-injected group than in all other groups. In particular, the CYV-injected larvae showed an adult emergence rate of 3%, which was significantly lower than the 75.6% observed in the untreated group (Welch’s t-test, p< 0.001).
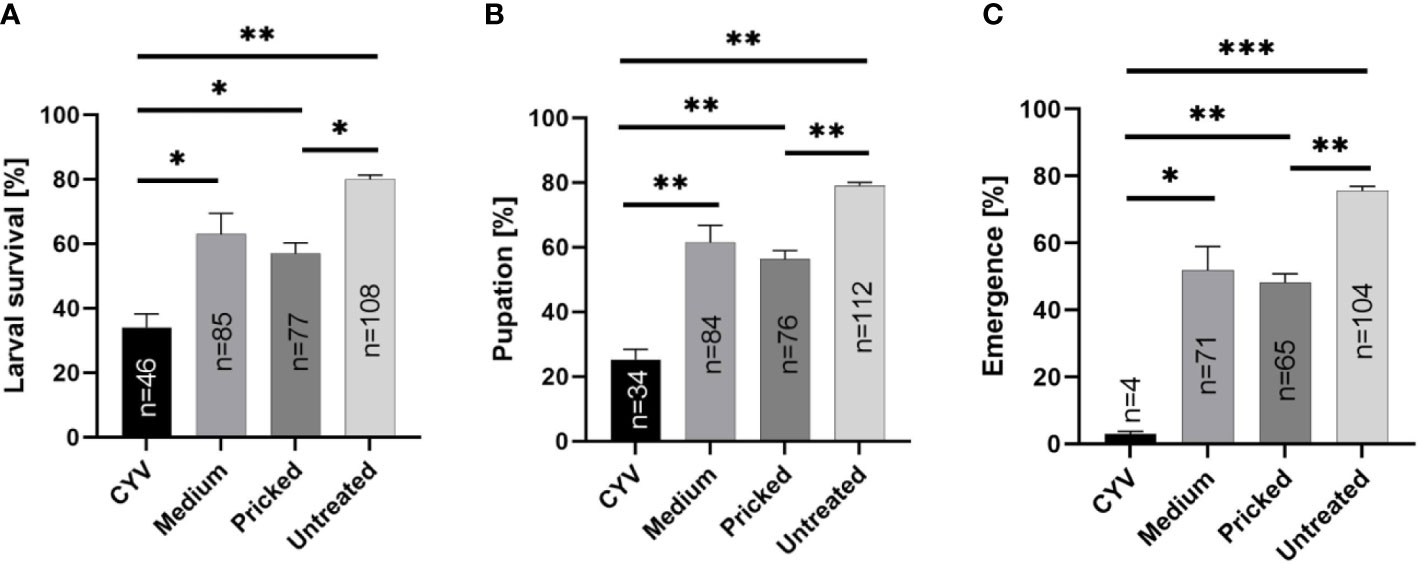
Figure 4 Analyses of the impact of Culex Y virus (CYV) infection compared with injection of medium, glass capillary pricking, and no treatment, independent of the diet (excluding the water control). Asterisks show significant p-values (*p<0.05; **p<0.01) for (A) larval survival rate until 7 dpi, (B) pupation rate, and (C) adult emergence rate, as well as the number (n) of animals reaching each developmental stage. The proportion of mosquitoes shown is normalized by setting of treatment.
We then analyzed our dataset for potential differences between the three different diets regarding the survival rate of CYV-infected larvae, as well as the pupation and adult emergence rates. We did not observe significant differences in any of these parameters between the Tetra Pleco, JBL NovoTab, and KG diets, indicating that food type does not influence survival and fitness after CYV infection in the tested setting (Tables 3, S3, S4; Figure 4).
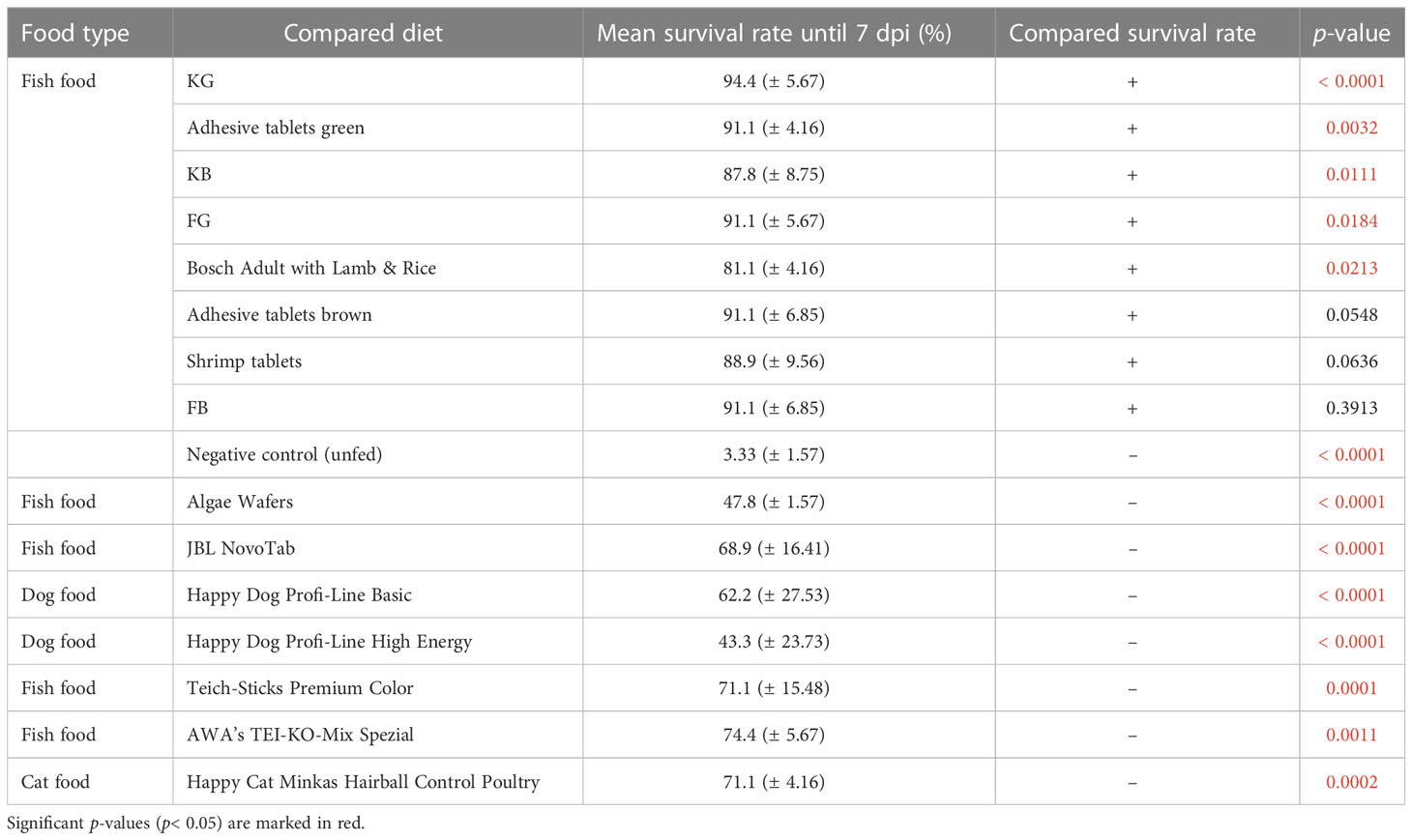
Table 3 Performance of the test diets compared with Tetra Pleco (positive control), which resulted in mean 80% (± 5.44%) larval survival rate.
3.3 Water quality analysis
Finally, we analyzed water quality parameters in different diet regimes to find possible correlations between food source, water quality, and larval survival. Water samples were taken from each diet group (Tetra Pleco tablets, brown adhesive tablets, FB, KG, JBL NovoTab, and corn flour mixed with Tetra Pleco tablets in a 1:1 ratio), and from the no food group as negative control, and phosphate, nitrate, and ammonium concentration, water hardness, O2 saturation, pH, and electrical conductivity were measured (Figure 5). For all tested diets except the negative control we observed a decrease in the mean phosphate content from day 0 to day 7. The highest phosphate concentration on day 0 (7.9 mg/L) was found in the KG diet experiment and the lowest value was in the negative control (< 0.01 mg/L). Nitrate content decreased in water samples from all tested diets except for the negative control, which had an initial nitrate concentration of< 2.2 mg/L. On day 0, we observed similar nitrate concentrations of, on average, 31 mg/L in water samples from all tested diets, except KG, which had a concentration of 52.9 mg/L. After 7 days we detected a large decrease in nitrate concentration (of approximately 7.3 mg/L) in water samples from all tested diets except for the diet JBL NovoTab, which showed a nitrate concentration of 17.85 ± 3.75 mg/L. The ammonium/ammonia concentration increased in water samples from all diet groups, with the highest concentrations after 7 days being in the JBL NovoTab (86.0 ± 48.0 mg/L) and KG (75.5 ± 9.5 mg/L) groups, and the lowest concentration after 7 days being in the negative control group (1.39 ± 0.17 mg/L). The total water hardness showed a decrease after 7 days of approximately 1°dH, with the exception of the water samples taken from the FB and adhesive brown tablet diets, which declined by 3–3.5°dH. From day 0 to day 7, an overall increase in O2 content was measured in all water samples. In particular, a significant increase in O2 saturation, from 88.2% ± 0.7% to 121.5% ± 1.5%, was seen in the water taken from the Tetra Pleco diet. We observed a decline in O2 saturation in the water samples from the diet in which Tetra Pleco was partially replaced with corn flour, and in those from the JBL NovoTab diet. In the samples from JBL NovoTabs diet the O2-saturation was 5.65% ± 1.35%. pH increased from approximately 7 to 8–9 in water samples from all diets except the negative control. In the negative control, pH remained relatively constant. Electrical conductivity increased on average for most the diets. The largest increase was found in water samples from the JBL NovoTabs diet, with a final value on day 7 of 1,560 ± 527 µS/cm.
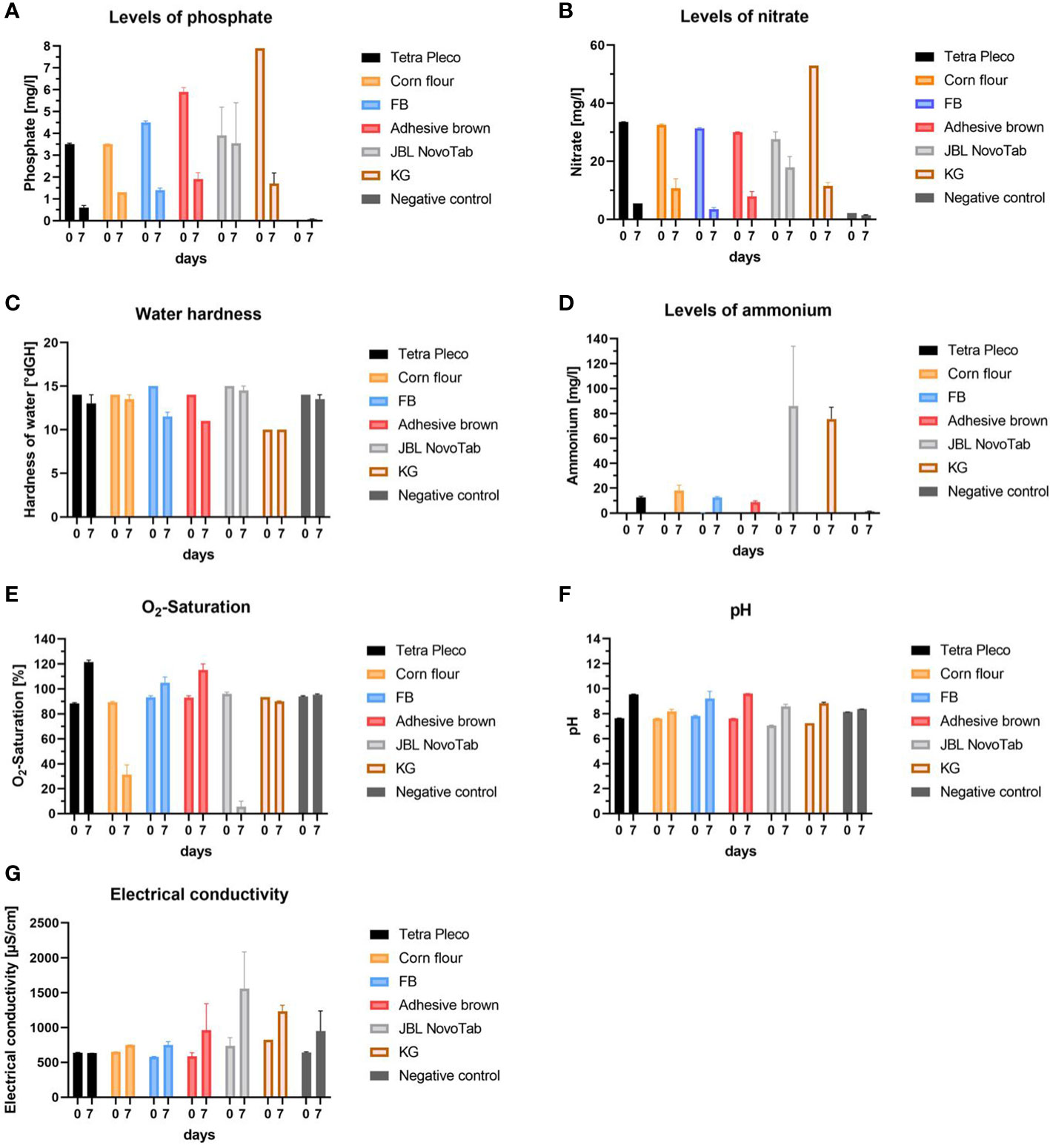
Figure 5 Water quality parameters in rearing waters using different diets on day 0 after diet application and day 7 after rearing larvae on those diets. The investigated diets are Tetra Pleco, a Tetra Pleco–corn flour mixture, FB adhesive brown tablets, JBL NovoTab, and water (control). (A) Phosphate concentration (mg/L), (B) nitrate concentration (mg/L), (C) water hardness (°dH), (D) ammonium concentration (mg/L), (E) O2 saturation of rearing water (%), (F) pH-value, and (G) electrical conductivity (µS/cm).
4 Discussion
Various strategies to control the mosquito population, and thereby also reduce the transmission of arboviruses and other mosquito-borne pathogens, have been explored in recent years. ISVs, discovered as a by-product of microbiome sequencing projects, have been discussed as a novel method for vector-borne disease prevention. Replication of ISVs is restricted to the arthropod vectors, and they have been observed to have an impact on vectors and disease transmission, including interfering with arbovirus replication (38) and reducing population size by increasing mortality or reducing fecundity (39, 40). Furthermore, nutrient status is an important factor affecting mosquito population density and stability has long been demonstrated (3, 41, 42). However, the impact of nutrition status on ISV infections in Mosquitos has not yet been elucidated.
In this study, we investigate the ability of the ISV Culex Y virus (CYV), a natural virus of Cx. pipiens complex Mosquitos (43), to infect larval stages and the impact of such an infection on survival, pupation, and adult emergence. To the best of our knowledge, very little information exists on the viral infection of juvenile stages of Mosquitos. Larval injections have been described in the literature for fungal (44) and bacterial (45) infections, or as a means to produce knock-downs by dsRNA injection (46).
Few studies in the past have described the injection of viral particles into mosquito larvae. When this has been conducted, the goal has always been to examine transstadial transmission, in which case L4 larvae were usually infected and the derived adults examined for infection (47), or to determine the rate of infection of larvae (48). We could not find any study that measured viral replication kinetics after injection while Mosquitos were still in the larval stage. This may be related to the fact that this is possible only when infecting early larval stages (early L4 or, preferably, L3); otherwise development to the pupal stage is too rapid and thus there is only a very short incubation time in which to study virus replication at the larval stage. Therefore, early/young L4 or younger (L3) must be medium-injected and not the old/bigger L4. In this study, we found a large increase in viral RNA copies within the first 3 days post-injection, from 9.69 × 103 to 5.57 × 107 in L3 instars and from 8.96 × 103 to 9.64 × 107 in L4 instars, i.e., by four log levels. Interestingly, these results exceed by far the replication rates of CYV in adult Mosquitos of the same breed after injection, in which an increase in RNA copies of only two log levels was found over the same period of time (from 1.01 × 104 on the day of infection to 1.42 × 106 on the third day) (31). It is not clear why viral replication appears to be far more efficient in the juvenile stage. Generally, a higher infection resistance in juvenile stages to ensure reproduction would be an evolutionary advantage. However, the opposite was shown in many studies across various taxa (49), including insects. A theoretical study analyzing juvenile susceptibility and other life-history traits came to the conclusion that enhanced juvenile susceptibility is strongest for animals with short or long lifespans and low or high probabilities of reaching the adult stage (49). The nature of enhanced susceptibility might be due to low response in an innate immune system or missing priming in adaptive immune systems. In the case of insects, a missing inducible response or less efficient RNAi in juvenile stages could contribute to enhanced infection susceptibility. For example, Vago, an antiviral gene strongly induced by Drosophila C virus infection in adult Drosophila is not expressed in the larvae (50). In addition, in Ae. aegypti Mosquitos, relative expression of RNAi components and Vago is lower in larvae than in pupae or adult stages. Thus, a low immune response paired with the described suppression of RNAi by CYV V3 protein (51, 52) might favor high virus RNA replication in L3/4 larvae. However, the current data showing enhanced juvenile susceptibility are based on artificial infections. Therefore, in further studies it will be of interest to also test an oral application of CYV, as natural biological barriers such as the midgut barrier would no longer be bypassed. In addition, earlier larval stages (L1 or L2) could be infected by this method, thereby facilitating the use of CYV infection in vector control. It has been shown that ISVs can be ingested orally by larvae in aquatic environments, replicated there, and even transmitted to the next stages, as was the case, for example, for the recently discovered Yichang virus (YCV), a Mesonivirus belonging to the ISVs (53). Rearing water containing 103 pfu/mL YCV resulted in infected Cx. pipiens quinquefasciatus adults (RNA detection at 8 dpi). This study also confirmed the impact of water quality on larval susceptibility to viral infections: it was shown that the YCV infection rates of Mosquitos were higher in sewage than in clean water, and that this can be correlated with divalent cations or the pH of the rearing water (53). Further studies also identified water quality as a crucial factor in the infectivity of mosquito larvae: some divalent cations, such as magnesium, enhanced transmission by around 30%, while other divalent cations, such as calcium, inhibited transmission of Cx. restuans Cypovirus (CrCPV; dsRNA virus) to Cx. quinquefasciatus larvae (54, 55). These divalent cations have also been discussed for further viral oral infectability, for example in transmitting nucleopolyhedrovirus (UrsaNPV, DNA virus) to Uranotaenia sapphirina mosquito larvae (56) or Zika virus (ZIKV, ssRNA virus) to Aedes mosquito larvae (57). Next we analyzed the impact of different diet regimes on larval survival, with and without CYV infection. To date, it has been established that different diets have an impact on the susceptibility of adult Mosquitos to viral infections (58, 59). For example, low sucrose concentration in the meals of adult Mosquitos led to an increased rate of transmission of West Nile virus in those Mosquitos (58). In our study, 17 different laboratory larval diets or diet combinations were screened for the fitness parameters of larval survival, pupation rate, and adult emergence rate. The content levels of the two most important energetic nutrients, carbohydrates and protein (4), varied considerably between the tested diets (Table 3). We found five diets that increased larval survival and seven that lowered larval survival. However, we did not observe a correlation between macronutrients and larval survival rate. Therefore, it can be assumed that the micronutrient content of diets (which is not always fully specified) (Table S2) or the quality of macronutrients could play an as yet undefined role in the observed differences in larval survival. Since it has been demonstrated for Ae. aegypti larvae that the main source of calories is carbohydrates (4), we tested if the supplementation of Tetra Pleco fish tablets (the positive control diet) with corn flour, a high-carbohydrate food, would increase the larval survival rate. The supplementation with corn flour clearly increased the larval survival rate in our assay.
In addition to the nutrient content of the different diets, the diets’ effect on water quality could be a factor in larval survival. Thus, we tested the influence of water quality from a selection of diets in a panel of water quality assays: the Tetra Pleco diet as a representative control; two diets showing no significant differences in larval survival rate to Tetra Pleco (adhesive brown tablets and FB); one diet showing a lower survival rate than the control (JBL NovoTab); and the diet in which Tetra Pleco was partially replaced with corn flour. Our records showed high similarities in water quality for the diets Tetra Pleco, adhesive brown tablet, and FB regarding all investigated parameters. In contrast, a clear difference in water quality could be demonstrated after 7 days of JBL NovoTab feeding: phosphate, nitrate, and ammonium/ammonia concentrations and electrical conductivity were all higher than with the other tested diets, whereas dissolved oxygen values were much lower. Decreases in nitrate and phosphate and an increase in dissolved oxygen may be related to algae growth, as microalgae consume nitrate and phosphate for growth and produce oxygen through photosynthesis (60–62). The role of dissolved oxygen in larval physiology has not yet been conclusively clarified. It was long thought to have no effect, as larvae do not rely on filtering oxygen through gills or through diffusion but instead are metapneustic (having a respiratory apparatus in the anus) and can primarily breathe atmospheric oxygen through their siphon (63). However, a study by Silberbush et al., in 2015, provided the first experimental evidence that lower dissolved oxygen levels reduce larval survival and delay development (64). Our results confirm this observation, as larvae fed on the JBL diet showed the lowest dissolved oxygen and the lowest larval survival rate after 7 days. In water, ammonium and ammonia are in a dissociation equilibrium, the distribution of which depends on temperature and pH (65). We observed that ammonium/ammonia levels increased with time for all tested diets. This phenomenon is in large part caused by the excretions of live larvae (66). It is known that high ammonium levels result in a higher larval mortality and a slower development of larvae (67), although they are able to tolerate relatively high concentrations of ammonium (68). This is exemplified by the survival rate and ammonium/ammonia content in larvae fed the JBL NovoTab diet. JBL NovoTab had the highest measured ammonium/ammonia and lowest survival rate of all diets selected for water quality monitoring. For all diets, we recorded a water hardness of 14–15°dGH. This value dropped to varying degrees for all diets. High water hardness can impact mosquito larvae by affecting their pupation rate, resulting in lower emergence of adults (69). We recorded for all diets a water hardness of 14–15°dGH on day 0, followed by an average decrease of 1.8°dGH for all tested diets. The decline in water hardness (dissolved minerals primarily comprising calcium and magnesium) can likely be attributed to the growth of algae, as these organisms consume the minerals during growth (70, 71). This algal growth was also observed macroscopically. The pH of aquatic mosquito habitats and its fluctuation has a great impact on larvae survivorship (72). This parameter has an influence on the balance of body fluid ionic composition (73). A study investigating Cx. pipiens quinquefasciatus showed that the fastest development was observed at pH 7.0 and the slowest at 4 pH or above 9 pH (69). Our study indicated that pH increases independent of diet and reaches values above 9 after 7 days, except in the case of the diet in which Tetra Pleco was partially replaced with a corn flour. Electrical conductivity, which is a measure to a substance’s or medium’s ability to conduct electricity (74), is influenced by the total dissolved salts, other inorganic chemicals, and also by temperature (75). Electrical conductivity can affect larval development as well as adult body size, and an electrical conductivity greater than 368 µS/cm generally leads to delayed development and a smaller observed body size (76). Our water analyses showed high values for all diets (>539 µS/cm on day 0 and >631 µS/cm on day 7), indicating high amounts of salts and other inorganic chemicals. Taken together, several water quality parameters differed between the tested diets and could contribute to the observed differences in larval survival.
Next we tested three selected diets and measured their impact on larval survival in CYV-infected larvae and control larvae. We selected Tetra Pleco as the positive control; KG, which showed significantly enhanced larval survival rates in our first experiments; and JBL NovoTab, which showed significantly reduced larval survival rates in our first experiments. In the first experimental setup, a preliminary screening of many feed types was performed over 7 days. We found that most larvae did not reach the pupal stage after feeding in this period. In a more detailed setup, with fewer feed types and a longer experiment period, we excluded the possibility of underfeeding (which is a risk in one-time large feedings) without severely affecting water quality by feeding small amounts of food each day. Under this regime, we observed differences of around 14% between larval survival rates in the untreated control groups (comparing the results reported in sections 3.2.1 and 3.2.2), which can be explained by cannibalism and by conducting the two experiments at different times of year (part A in spring/summer and part B in fall), which could have indirectly had an impact on larval fitness, as the laboratory strain tested may still have some seasonality owing to its relatively young age (established in 2012–2013). Regarding the treatment of larvae, we observed that the supposedly more invasive Schneider’s medium injection presented a trend toward higher fitness rates (larval survival, pupation rate, and emergene rates) than the pricked larvae across all diets. The induced injury is similar in both groups because both pricks are performed with glass capillaries; thus, the difference cannot be explained by different wound sizes, but can be explained by the fact that the injected medium probably brings more nutritive advantages than it does physical disadvantages. In addition, the possible withdrawal due to leakage of body fluid during the prick process plays a role in the capillary prick group, so that this group has a disadvantage compared to the other groups tested. However, the effects on larval fitness caused exclusively by CYV cannot be precisely determined in this study because neither the larval feed nor the rearing water was sterilized prior to the experiment, and thus other pathogens such as bacteria or fungi may have played a direct or indirect role in the infection experiments. Nevertheless, the infection of larvae with CYV had a major impact on larval fitness. Compared with all other control groups, significantly lower rates of larval survival, pupation, and emergence were detected in the CYV-infected larvae. The proportion of CYV-infected larvae developing into adults was consistently below 5%; in contrast, in the control groups, this figure ranged from 48.1% to 75.6%. These results suggest that, owing to high larval mortality, CYV might not be transmitted vertically, as is described in various other ISVs (77–80). However, covert infections, as described in, for example, Cx. pipiens quinquefasciatus and Baculovirus, can lead to lower larval mortality, and so to a restricted but possible vertical transmission (81). Similar covert CYV infection in the adult Cx. pipiens biotype molestus, for example in the ovaries, could lead to a reduced pathogenic effect or infection in following generations.
Infection through water, as CYV is a member of the Birnaviridae family (29, 82) and thus stable in water (83), or through ingestion of infected larvae cadavers is also conceivable. For the dipteran ISV brevidensovirus 1, it has already been shown that stability and infectivity remain stable in water for more than one year (84, 85). Further studies will have to be conducted to validate the infectivity of CYV in dead larvae, as well as in water bodies. Regarding the impact of larval diet on the survival of CYV-infected larvae, none of the tested diets showed a significant difference. Nevertheless, we observed a trend toward KG diet improving mosquito fitness across all stages compared with Tetra Pleco, and JBL NovoTab showed the worst results for these parameters. This indicates that there might be small differences in nutrient availability between the three diets that have slight effects on larval survival under viral infection. However, since the differences were small and variation between experiments was observed, this could also be a random effect.
In conclusion, we were able to demonstrate that CYV can infect Cx pipiens biotype molestus larvae, replicating in large numbers and reducing the fitness parameters of larval survival rate, pupation rate, and adult emergence rate. This profound impact could open new perspectives for vector control by using CYV feed in aquatic habitats, similar to the use of Bacillus thuringiensis israelensis (Bti) in vector control (86). Furthermore, we verified that the effects of CYV infection were not influenced by the diet regime of the larvae, indicating broad applications for CYV for mosquito population control.
Data availability statement
The original contributions presented in the study are included in the article/Supplementary Material. Further inquiries can be directed to the corresponding authors.
Author contributions
Conceptualization, FH, MH-H, PN, and VJ-S; methodology, FH, MH-H, PN, and FT; formal analysis, MH-H and PN; investigation, PN, FH, and MH-H; resources, FH, SB, and VJ-S; data curation, FH MH-H, and PN; writing—original draft preparation, FH and MH-H; writing—review and editing FH, MH-H, PN, FT, VJ-S, and SB. All authors contributed to the article and approved the submitted version.
Funding
This research received funding from BMBF—Zoonosenplattform (01Kl1911). This Open Access publication was funded by the Deutsche Forschungsgemeinschaft (DFG, German Research Foundation) - 491094227 "Open Access Publication Funding" and the University of Veterinary Medicine Hannover, Foundation.
Acknowledgments
We would like to thank Prof. Dieter Steinhagen for his support in this project.
Conflict of interest
The authors declare that the research was conducted in the absence of any commercial or financial relationships that could be construed as a potential conflict of interest.
Publisher’s note
All claims expressed in this article are solely those of the authors and do not necessarily represent those of their affiliated organizations, or those of the publisher, the editors and the reviewers. Any product that may be evaluated in this article, or claim that may be made by its manufacturer, is not guaranteed or endorsed by the publisher.
Supplementary material
The Supplementary Material for this article can be found online at: https://www.frontiersin.org/articles/10.3389/fitd.2023.1107857/full#supplementary-material
References
1. Brugman VA, Hernández-Triana LM, Medlock JM, Fooks AR, Carpenter S, Johnson N. The role of culex pipiens L.(Diptera: Culicidae) in virus transmission in Europe. Int J Environ Res Public Health (2018) 15:389. doi: 10.3390/ijerph15020389
2. Scholte E-J, Knols BG, Samson RA, Takken W. Entomopathogenic fungi for mosquito control: a review. J Insect Sci (2004) 4:19. doi: 10.1093/jis/4.1.19
3. Souza RS, Virginio F, Riback TIS, Suesdek L, Barufi JB, Genta FA. Microorganism-based larval diets affect mosquito development, size and nutritional reserves in the yellow fever mosquito aedes aegypti (Diptera: Culicidae). Front Physiol (2019) 10:152. doi: 10.3389/fphys.2019.00152
4. van Schoor T, Kelly ET, Tam N, Attardo GM. Impacts of dietary nutritional composition on larval development and adult body composition in the yellow fever mosquito (Aedes aegypti). Insects (2020) 11:535. doi: 10.3390/insects11080535
5. MacLeod HJ, Dimopoulos G, Short SM. Larval diet abundance influences size and composition of the midgut microbiota of aedes aegypti Mosquitos. Front Microbiol (2021) 12:645362. doi: 10.3389/fmicb.2021.645362
6. Walker ED, Olds EJ, Merritt RW. Gut content analysis of mosquito larvae (Diptera: Culicidae) using DAPI stain and epifluorescence microscopy. J Med Entomol (1988) 25:551–4. doi: 10.1093/jmedent/25.6.551
7. Timmermann S, Briegel H. Effect of plant, fungal and animal diets on mosquito development, in: Proceedings of Proceedings of the 9th International Symposium on Insect-Plant Relationships Entomol Exp Appl (Zürich, Switzerland: Kluwer Academic Publishers) (1996) 173–6.
8. Uspensky I, Braun S. Cannibalism in mosquito larvae during microbial larvicide potency tests. Folia Parasitol (2020) 67:1–4. doi: 10.14411/fp.2020.005
9. Mastrantonio V, Crasta G, Urbanelli S, Porretta D. Cannibalism and necrophagy promote a resource loop and benefit larval development in insects of temporary waters. Insects (2021) 12:657. doi: 10.3390/insects12070657
10. Singh KRP, Brown A. Nutritional requirements of aedes aegypti l. J Insect Physiol (1957) 1:199–220. doi: 10.1016/0022-1910(57)90036-7
11. Joy TK, Arik AJ, Corby-Harris V, Johnson AA, Riehle MA. The impact of larval and adult dietary restriction on lifespan, reproduction and growth in the mosquito aedes aegypti. Exp gerontol (2010) 45:685–90. doi: 10.1016/j.exger.2010.04.009
12. Farjana T, Tuno N, Higa Y. Effects of temperature and diet on development and interspecies competition in aedes aegypti and aedes albopictus. Med vet entomol (2012) 26:210–7. doi: 10.1111/j.1365-2915.2011.00971.x
13. Carvajal-Lago L, Ruiz-López MJ, Figuerola J, Martínez-de la Puente J. Implications of diet on mosquito life history traits and pathogen transmission. Environ Res (2021) 195:110893. doi: 10.1016/j.envres.2021.110893
14. Muturi EJ, Alto BW. Larval environmental temperature and insecticide exposure alter aedes aegypti competence for arboviruses. Vector-Borne Zoonotic Dis (2011) 11:1157–63. doi: 10.1089/vbz.2010.0209
15. Araújo M-S, Gil LHS. Larval food quantity affects development time, survival and adult biological traits that influence the vectorial capacity of anopheles darlingi under laboratory conditions. Malaria J (2012) 11:1–9. doi: 10.1186/1475-2875-11-261
16. Vantaux A, Ouattarra I, Lefèvre T, Dabiré KR. Effects of larvicidal and larval nutritional stresses on anopheles gambiae development, survival and competence for plasmodium falciparum. Parasites Vectors (2016) 9:1–11. doi: 10.1186/s13071-016-1514-5
17. Walsh R, Facchinelli L, Ramsey J, Bond J, Gould F. Assessing the impact of density dependence in field populations of aedes aegypti. J Vector Ecol (2011) 36:300–7. doi: 10.1111/j.1948-7134.2011.00170.x
18. Walton WE, Mai K, Nguyen A, Tse R. Evaluation of two management strategies for harvested emergent vegetation on immature mosquito abundance and water quality. J Am Mosq Control Assoc (2020) 36:139–51. doi: 10.2987/20-6913.1
19. Nayar J, Van Handel E. The fuel for sustained mosquito flight. J Insect Physiol (1971) 17:471–81. doi: 10.1016/0022-1910(71)90026-6
20. Wang M, An Y, Gao L, Dong S, Zhou X, Feng Y, et al. Glucose-mediated proliferation of a gut commensal bacterium promotes plasmodium infection by increasing mosquito midgut pH. Cell Rep (2021) 35:108992. doi: 10.1016/j.celrep.2021.108992
21. Linenberg I, Christophides GK, Gendrin M. Larval diet affects mosquito development and permissiveness to plasmodium infection. Sci Rep (2016) 6:1–10. doi: 10.1038/srep38230
22. Bolling BG, Eisen L, Moore CG, Blair CD. Insect-specific flaviviruses from culex Mosquitos in Colorado, with evidence of vertical transmission. Am J Trop Med hygiene (2011) 85:169. doi: 10.4269/ajtmh.2011.10-0474
23. Haddow AD, Guzman H, Popov VL, Wood TG, Widen SG, Haddow AD, et al. First isolation of aedes flavivirus in the Western hemisphere and evidence of vertical transmission in the mosquito aedes (Stegomyia) albopictus (Diptera: Culicidae). Virology (2013) 440:134–9. doi: 10.1016/j.virol.2012.12.008
24. Halbach R, Junglen S, van Rij RP. Mosquito-specific and mosquito-borne viruses: evolution, infection, and host defense. Curr Opin Insect Sci (2017) 22:16–27. doi: 10.1016/j.cois.2017.05.004
25. Roossinck MJ. Plants, viruses and the environment: ecology and mutualism. Virology (2015) 479:271–7. doi: 10.1016/j.virol.2015.03.041
26. White AV, Fan M, Mazzara JM, Roper RL, Richards SL. Mosquito-infecting virus espirito Santo virus inhibits replication and spread of dengue virus. J Med Virol (2021) 93:3362–73. doi: 10.1002/jmv.26686
27. Bolling BG, Olea-Popelka FJ, Eisen L, Moore CG, Blair CD. Transmission dynamics of an insect-specific flavivirus in a naturally infected culex pipiens laboratory colony and effects of co-infection on vector competence for West Nile virus. Virology (2012) 427:90–7. doi: 10.1016/j.virol.2012.02.016
28. Vasilakis N, Tesh RB. Insect-specific viruses and their potential impact on arbovirus transmission. Curr Opin Virol (2015) 15:69–74. doi: 10.1016/j.coviro.2015.08.007
29. Marklewitz M, Gloza-Rausch F, Kurth A, Kummerer BM, Drosten C, Junglen S. First isolation of an entomobirnavirus from free-living insects. J Gen Virol (2012) 93:2431–5. doi: 10.1099/vir.0.045435-0
30. Franzke K, Leggewie M, Sreenu VB, Jansen S, Heitmann A, Welch SR, et al. Detection, infection dynamics and small RNA response against culex y virus in mosquito-derived cells. J Gen Virol (2018) 99:1739–45. doi: 10.1099/jgv.0.001173
31. Heinig-Hartberger M, Hellhammer F, Zöller DD, Dornbusch S, Bergmann S, Vocadlova K, et al. Culex y virus: A native virus of culex species characterized In vivo. Viruses (2023) 15:235. doi: 10.3390/v15010235
32. Leggewie M, Badusche M, Rudolf M, Jansen S, Börstler J, Krumkamp R, et al. Culex pipiens and culex torrentium populations from central Europe are susceptible to West Nile virus infection. One Health (2016) 2:88–94. doi: 10.1016/j.onehlt.2016.04.001
33. Sánchez-Seco MP, Rosario D, Quiroz E, Guzmán G, Tenorio A. A generic nested-RT-PCR followed by sequencing for detection and identification of members of the alphavirus genus. J Virol Methods (2001) 95:153–61. doi: 10.1016/S0166-0934(01)00306-8
34. Lambert AJ, Lanciotti RS. Molecular characterization of medically important viruses of the genus orthobunyavirus. J Gen Virol (2008) 89:2580–5. doi: 10.1099/vir.0.2008/002253-0
35. Johnson N, Wakeley PR, Mansfield KL, McCracken F, Haxton B, Phipps LP, et al. Assessment of a novel real-time pan-flavivirus RT-polymerase chain reaction. Vector-Borne Zoonotic Dis (2010) 10:665–71. doi: 10.1089/vbz.2009.0210
36. Ruang-Areerate T, Kittyapong P, Baimai V, O'Neill SL. Molecular phylogeny of wolbachia endosymbionts in southeast Asian Mosquitos (Diptera: Culicidae) based on wsp gene sequences. J Med Entomol (2003) 40:1–5. doi: 10.1603/0022-2585-40.1.1
37. Administration, U.S.F.D. Pet food labels - general (2022). Available at: https://www.fda.gov/animal-veterinary/animal-health-literacy/pet-food-labels-general.
38. Öhlund P, Lundén H, Blomström A-L. Insect-specific virus evolution and potential effects on vector competence. Virus Genes (2019) 55:127–37. doi: 10.1007/s11262-018-01629-9
39. Johnson RM, Rasgon JL. Densonucleosis viruses (‘densoviruses’) for mosquito and pathogen control. Curr Opin Insect Sci (2018) 28:90–7. doi: 10.1016/j.cois.2018.05.009
40. Ren X, Rasgon JL. Potential for the anopheles gambiae densonucleosis virus to act as an “evolution-proof” biopesticide. J Virol (2010) 84:7726–9. doi: 10.1128/JVI.00631-10
41. Washburn J. Regulatory factors affecting larval mosquito populations in container and pool habitats: implications for biological control. J Am Mosq Control Association-Mosquito News (1995) 11:279–83.
42. Ranasinghe H, Amarasinghe L. Naturally occurring microbiota associated with mosquito breeding habitats and their effects on mosquito larvae. BioMed Res Int (2020) 2020. doi: 10.1155/2020/4065315
43. Marklewitz M, Zirkel F, Rwego IB, Heidemann H, Trippner P, Kurth A, et al. Discovery of a unique novel clade of mosquito-associated bunyaviruses. J Virol (2013) 87:12850–65. doi: 10.1128/JVI.01862-13
44. Bawin T, Boukraa S, Seye F, Raharimalala FN, Zimmer J-Y, Delvigne F, et al. Using micro-injection technique to assess fungal toxicity in mosquito control. Commun Agric Appl Biol Sci (2014) 79:181–5.
45. League GP, Hillyer JF. Functional integration of the circulatory, immune, and respiratory systems in mosquito larvae: pathogen killing in the hemocyte-rich tracheal tufts. BMC Biol (2016) 14:1–18. doi: 10.1186/s12915-016-0305-y
46. Munawar K, Alahmed AM, Khalil SM. Delivery methods for RNAi in mosquito larvae. J Insect Sci (2020) 20:12. doi: 10.1093/jisesa/ieaa074
47. Sudeep A, Mandar P, Ghodke Y, George R, Gokhale M. Vector competence of two Indian populations of culex quinquefasciatus (Diptera: Culicidae) Mosquitos to three West Nile virus strains. J Vector Borne Dis (2015) 52:185.
48. Hasan S, Vago C, Kuhl G. Infection of aedes detritus hal. with mosquito iridescent virus. Bull World Health Organ (1971) 45:268.
49. Ashby B, Bruns E. The evolution of juvenile susceptibility to infectious disease. Proc R Soc B (2018) 285:20180844. doi: 10.1098/rspb.2018.0844
50. Dostert C, Jouanguy E, Irving P, Troxler L, Galiana-Arnoux D, Hetru C, et al. The jak-STAT signaling pathway is required but not sufficient for the antiviral response of drosophila. Nat Immunol (2005) 6:946–53. doi: 10.1038/ni1237
51. Vargas V, Cime-Castillo J, Lanz-Mendoza H. Immune priming with inactive dengue virus during the larval stage of aedes aegypti protects against the infection in adult Mosquitos. Sci Rep (2020) 10:1–10. doi: 10.1038/s41598-020-63402-z
52. van Cleef KW, van Mierlo JT, Miesen P, Overheul GJ, Fros JJ, Schuster S, et al. Mosquito and drosophila entomobirnaviruses suppress dsRNA-and siRNA-induced RNAi. Nucleic Acids Res (2014) 42:8732–44. doi: 10.1093/nar/gku528
53. Ye G, Wang Y, Liu X, Dong Q, Cai Q, Yuan Z, et al. Transmission competence of a new mesonivirus, yichang virus, in Mosquitos and its interference with representative flaviviruses. PloS Negl Trop Dis (2020) 14:e0008920. doi: 10.1371/journal.pntd.0008920
54. Green TB, Shapiro A, White S, Rao S, Mertens PP, Carner G, et al. Molecular and biological characterization of a cypovirus from the mosquito culex restuans. J invertebrate Pathol (2006) 91:27–34. doi: 10.1016/j.jip.2005.10.007
55. Shapiro A, Green T, Rao S, White S, Carner G, Mertens PP, et al. Morphological and molecular characterization of a cypovirus (Reoviridae) from the mosquito uranotaenia sapphirina (Diptera: Culicidae). J Virol (2005) 79:9430–8. doi: 10.1128/JVI.79.15.9430-9438.2005
56. Shapiro AM, Becnel JJ, White SE. A nucleopolyhedrovirus from uranotaenia sapphirina (Diptera: Culicidae). J invertebrate Pathol (2004) 86:96–103. doi: 10.1016/j.jip.2004.04.005
57. Du S, Liu Y, Liu J, Zhao J, Champagne C, Tong L, et al. Aedes Mosquitos acquire and transmit zika virus by breeding in contaminated aquatic environments. Nat Commun (2019) 10:1–11. doi: 10.1038/s41467-019-09256-0
58. Vaidyanathan R, Fleisher AE, Minnick SL, Simmons KA, Scott TW. Nutritional stress affects mosquito survival and vector competence for West Nile virus. Vector-Borne Zoonotic Dis (2008) 8:727–32. doi: 10.1089/vbz.2007.0189
59. Westby KM, Muturi EJ, Juliano SA. How do nutritional stress and la crosse virus infection interact? tests for effects on willingness to blood feed and fecundity in aedes albopictus (Diptera: Culicidae). J Med Entomol (2016) 53:166–71. doi: 10.1093/jme/tjv146
60. Fried S, Mackie B, Nothwehr E. Nitrate and phosphate levels positively affect the growth of algae species found in perry pond. Tillers (2003) 4:21–4.
61. Lavajoo F, Thaherizade M, Dehghani M. The absorption of nitrate and phosphate from urban sewage by blue-green algae (Spirolina Platensis)(An alternative medium) as application for removing the pollution. J Appl Sci Environ Manage (2015) 19:353–6. doi: 10.4314/jasem.v19i3.15
62. Li M, Li Y, Zhang Y, Xu Q, Iqbal MS, Xi Y, et al. The significance of phosphorus in algae growth and the subsequent ecological response of consumers. J Freshw Ecol (2022) 37:57–69. doi: 10.1080/02705060.2021.2014365
63. Clements AN. The biology of Mosquitos. Volume 1: development, nutrition and reproduction. Chapman & Hall: Cambridge University Press (1992).
64. Silberbush A, Abramsky Z, Tsurim I. Dissolved oxygen levels affect the survival and developmental period of the mosquito culex pipiens. J Vector Ecol (2015) 40:425–7. doi: 10.1111/jvec.12186
65. Huang J-C, Shang C. Air stripping. In: Advanced physicochemical treatment processes. Springer (2006). p. 47–79.
66. Piermarini PM, Esquivel CJ, Denton JS. Malpighian tubules as novel targets for mosquito control. Int J Environ Res Public Health (2017) 14:111. doi: 10.3390/ijerph14020111
67. Dias AC, Rodrigues MM, Silva AA. Effect of acute and chronic exposure to ammonia on different larval instars of anopheles darlingi (Diptera: Culicidae). J Vector Ecol (2019) 44:112–8. doi: 10.1111/jvec.12335
68. Durant AC, Donini A. Development of aedes aegypti (Diptera: Culicidae) mosquito larvae in high ammonia sewage in septic tanks causes alterations in ammonia excretion, ammonia transporter expression, and osmoregulation. Sci Rep (2019) 9:1–17. doi: 10.1038/s41598-019-54413-6
69. Ukubuiwe AC, Ojianwuna CC, Olayemi IK, Arimoro FO, Ukubuiwe CC. Quantifying the roles of water pH and hardness levels in development and biological fitness indices of culex quinquefasciatus say (Diptera: Culicidae). J Basic Appl Zoo (2020) 81:1–10. doi: 10.1186/s41936-020-0139-6
71. Mathew MM, Khatana K, Vats V, Dhanker R, Kumar R, Dahms H-U, et al. Biological approaches integrating algae and bacteria for the degradation of wastewater contaminants–a review. Front Microbiol (2021) 12. doi: 10.3389/fmicb.2021.801051
72. Yee DA, Kneitel JM, Juliano SA. Environmental correlates of abundances of mosquito species and stages in discarded vehicle tires. J Med Entomol (2010) 47:53–62. doi: 10.1093/jmedent/47.1.53
73. Daily JP, Hitt NP, Smith DR, Snyder CD. Experimental and environmental factors affect spurious detection of ecological thresholds. Ecology (2012) 93:17–23. doi: 10.1890/11-0516.1
74. Oliveira R, Georgieva P, de Azevedo SF. Plant and equipment| instrumentation and process control: Instrumentation. Encyclopedia of Dairy Sciences (UK: Academic Press) (2002) 1392–401.
75. Zareifard MR, Ramaswamy HS, Marcotte M, Karimi Y. Factors influencing electrical conductivity. Ohmic Heating Food Process (2014) 53. doi: 10.1201/b16605
76. Mamai W, Lees RS, Maiga H, Gilles JR. Reusing larval rearing water and its effect on development and quality of anopheles arabiensis Mosquitos. Malaria J (2016) 15:1–10. doi: 10.1186/s12936-016-1227-4
77. Agboli E, Leggewie M, Altinli M, Schnettler E. Mosquito-specific viruses–transmission and interaction. Viruses (2019) 11:873. doi: 10.3390/v11090873
78. Muttis E, Miele SA, Belaich MN, Micieli MV, Becnel JJ, Ghiringhelli PD, et al. First record of a mosquito iridescent virus in culex pipiens L.(Diptera: Culicidae). Arch Virol (2012) 157:1569–71. doi: 10.1007/s00705-012-1302-2
79. Lutomiah JJ, Mwandawiro C, Magambo J, Sang RC. Infection and vertical transmission of kamiti river virus in laboratory bred aedes aegypti Mosquitos. J Insect Sci (2007) 7. doi: 10.1673/031.007.5501
80. Ledermann JP, Suchman EL, Black WC IV, Carlson JO. Infection and pathogenicity of the mosquito densoviruses AeDNV, HeDNV, and APeDNV in aedes aegypti Mosquitos (Diptera: Culicidae). J Economic Entomol (2004) 97:1828–35. doi: 10.1093/jee/97.6.1828
81. Becnel JJ, White SE, Moser BA, Fukuda T, Rotstein MJ, Undeen AH, et al. Epizootiology and transmission of a newly discovered baculovirus from the Mosquitos culex nigripalpus and c. quinquefasciatus. J Gen Virol (2001) 82:275–82. doi: 10.1099/0022-1317-82-2-275
82. Marklewitz M. Diversity and evolutionary origin of the virus family bunyaviridae Vol. 2017. Bonn, Germany: Dissertation, Bonn, Rheinische Friedrich-Wilhelms-Universität Bonn (2016).
83. Delmas B, Mundt E, Vakharia V, Wu J. in Virus taxonomy. (Fauquet CM, Mayo MA, Maniloff J, Desselberger U, Ball LA, editors). Amsterdam: Elsevier.p. 561–9.
84. Buchatsky L. Densonucleosis of bloodsucking Mosquitos. Dis Aquat Organisms (1989) 6:145–50. doi: 10.3354/dao006145
85. Buchatsky L, Filenko O. Certain physico-chemical properties of mosquito densonucleosis virus DNA. Biopolymers Cell (1988) 4:254–8. doi: 10.7124/bc.000234
Keywords: insect-specific virus, CYV, larvae, mosquito, diet, nutrition
Citation: Hellhammer F, Heinig-Hartberger M, Neuhof P, Teitge F, Jung-Schroers V and Becker SC (2023) Impact of different diets on the survival, pupation, and adult emergence of Culex pipiens biotype molestus larvae, and infectability with the insect-specific Culex Y virus. Front. Trop. Dis 4:1107857. doi: 10.3389/fitd.2023.1107857
Received: 25 November 2022; Accepted: 06 February 2023;
Published: 09 March 2023.
Edited by:
Emilie Pondeville, MRC-University of Glasgow Centre For Virus Research (MRC), United KingdomReviewed by:
Ian Patterson, Brock University, CanadaDiwas Srivastava, UPR9022 Réponse immunitaire et développement chez les insectes (RIDI), France
William Louie, University of California, Davis, United States
Copyright © 2023 Hellhammer, Heinig-Hartberger, Neuhof, Teitge, Jung-Schroers and Becker. This is an open-access article distributed under the terms of the Creative Commons Attribution License (CC BY). The use, distribution or reproduction in other forums is permitted, provided the original author(s) and the copyright owner(s) are credited and that the original publication in this journal is cited, in accordance with accepted academic practice. No use, distribution or reproduction is permitted which does not comply with these terms.
*Correspondence: Fanny Hellhammer, fanny.hellhammer@tiho-hannover.de; Stefanie C. Becker, stefanie.becker@tiho-hannover.de
†These authors have contributed equally to this work and share first authorship