- 1National Research Center for Wildlife Borne Diseases, Institute of Zoology, Chinese Academy of Sciences, Beijing, China
- 2College of Agriculture, Ningxia University, Yinchuan, China
- 3State Key Laboratory of Plateau Ecology and Agriculture, Qinghai University, Xining, China
- 4College of Life Sciences, University of Chinese Academy of Sciences, Beijing, China
- 5College of Animal Science, Anhui Science and Technology University, Chuzhou, China
- 6Animal Disease Prevention and Control Center of Yushu, Yushu, China
- 7Chinese Academy of Inspection and Quarantine, Beijing, China
Tick-borne diseases (TBDs) can cause serious economic losses and are very important to animal and public health. To date, research on TBDs has been limited in Qinghai-Tibet Plateau, China. This epidemiological investigation was conducted to evaluate the distribution and risk factors of Anaplasma spp. and Ehrlichia chaffeensis in livestock in Qinghai. A total of 566 blood samples, including 330 yaks (Bos grunniens) and 236 Tibetan sheep (Ovis aries) were screened. Results showed that A. bovis (33.3%, 110/330) and A. phagocytophilum (29.4%, 97/330) were most prevalent in yaks, followed by A. ovis (1.2%, 4/330), A. capra (0.6%, 2/330), and E. chaffeensis (0.6%, 2/330). While A. ovis (80.9%, 191/236) and A. bovis (5.1%, 12/236) infection was identified in Tibetan sheep. To our knowledge, it is the first time that A. capra and E. chaffeensis have been detected in yaks in China. Apart from that, we also found that co-infection of A. bovis and A. phagocytophilum is common in yaks (28.2%, 93/330). For triple co-infection, two yaks were infected with A. bovis, A. phagocytophilum, and A. capra, and two yaks were infected with A. bovis, A. phagocytophilum, and E. chaffeensis. Risk analysis shows that infection with A. bovis, A. phagocytophilum, and A. ovis was related to region and altitude. This study provides new data on the prevalence of Anaplasma spp. and E. chaffeensis in Qinghai, China, which may help to develop new strategies for active responding to these pathogens.
Introduction
Anaplasmosis and ehrlichiosis are important diseases caused by tick-borne pathogens, which result in additional economic losses to livestock (1, 2). To date, seven Anaplasma species have been identified, including A. bovis, A. phagocytophilum, A. centrale, A. platys, A. marginale, A. ovis, and A. capra (3, 4). A. bovis parasitizes monocytes and macrophages of ruminants and small mammals (5). A. phagocytophilum infects neutrophils of humans and animals, and causing human granulocytic anaplasmosis (HGA), tick-borne fever in ruminants, and canine and equine granulocytic anaplasmosis (5). A. centrale and A. marginale mainly infect erythrocytes of cattle, while A. ovis primarily infect small ruminant animals such as sheep and goats. (6). A. platys mainly infect canine platelets and cause cyclic thrombocytopenia in dogs (6). A. capra is an emerging pathogen, which can infect ruminants and humans (7). In addition, as a member of the Ehrlichia family, Ehrlichia chaffeensis can cause human monocytic ehrlichiosis (HME) (8), and ehrlichiosis in animals (9).
Over the past several decades, the Anaplasma and Ehrlichia infections are very common in many countries (3, 10–12). A. bovis is mainly distributed in Africa, Asia, and South America, and cattle are considered the primary hosts (6). Similarly, A. ovis is the leading cause of anaplasmosis in small ruminants, which is widely distributed around the world (13). Recently, A. phagocytophilum, A. capra, and E. chaffeensis have received much attention for their potential threats to public health (7, 14). A. phagocytophilum has been detected in sheep, cattle, Capreolus pygargus, goats, and humans in different areas of China (15–18). E. chaffeensis infections are very common in the United States, with an annual rate of 4.46 cases/1,000,000 population (19). For A. capra, it was initially isolated from goats and humans in China (7). Subsequently, it was found in many countries (20, 21).
Qinghai is the source of the Yangtze River, the Yellow River, and the Lancang River, located in the northeast of Qinghai-Tibet Plateau and northwest of China with an average altitude of more than 3,000 meters. The complicated topographic features and changeable climate bless the region with advantageous conditions of rich natural resources. Tibetan sheep (Ovis aries) and yaks (Bos grunniens) are the main domestic animals in Qinghai and an important source of life and income for herders. Ixodid tick infestation in livestock is a common and severe problem, and more than 25 tick species in six genera have been reported in Qinghai (22, 23). However, information about tick-borne diseases (TBDs) in the region has been limited. Therefore, to better understand the situation of TBDs in Qinghai, China, a molecular epidemiologic study was conducted investigating exposure to Anaplasma spp. and E. chaffeensis in domestic animals across the area.
Materials and Methods
Blood Sample Collection of Yaks and Tibetan Sheep
A total of 566 blood samples of yaks (n = 330) and Tibetan sheep (n = 236) were collected using random sampling from six sampling sites in Maqin (35°2′38″N, 99°12′5″E; altitude 3,877 m), Dari (33°43′4″N, 99°38′2″E; altitude 4,130 m), and Banma (32°43′24″N, 100°42′41″E; altitude 3,864 m) of Guoluo Tibetan Autonomous Prefecture (GL), and Yushu (32°51′18″N, 96°48′57″E; altitude 4,317 m), Zhiduo (33°37′5″N, 95°58′51″E; altitude 4,177 m) and Qumalai (34°10′15″N, 95°49′57″E; altitude 4,279 m) of Yushu Tibetan Autonomous Prefecture (YS) during June 2020 in Qinghai, China (Figure 1). GL and YS are similar in altitude and climate, and both belong to the continental climate of the plateau. Except for about 400 Tibetan sheep in Maqin, the number of yaks and Tibetan sheep in other sampling sites is between 100 and 200. All animals adopt a free grazing system. Ticks and Melophagus ovinus and their bites can be seen in Tibetan sheep, while ticks are rarely found on yaks.
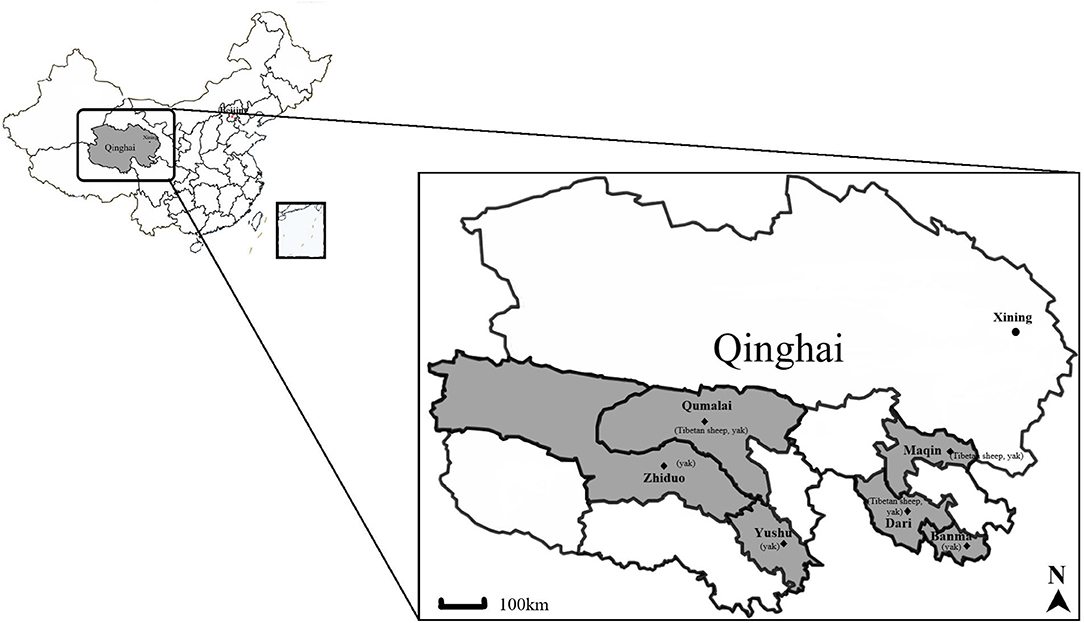
Figure 1. Map of the Qinghai Province, showing the sampling sites and animals included. The yak and Tibetan sheep samples were collected at six sites indicated by ♦. The figure was generated and modified using DITUHUI (https://g.dituhui.com).
Extraction and Quantification of DNA
According to the manufacturer's operation manual, genomic DNA was extracted from 200 uL whole blood samples by the TIANamp Genomic DNA kit (TIANGEN biotech, Beijing). The concentration of the extracted DNA was detected by NanoDrop 2,000 (Thermo Fisher Scientific, USA) and then stored at −20°C for pathogens detection.
Detection of Anaplasma spp. and E. chaffeensis
Conventional PCR or nested PCR was used to screen for Anaplasma spp. and E. chaffeensis in extracted DNA. Nested PCRs were employed to detect A. bovis, A. phagocytophilum, A. centrale, A. platys, and E. chaffeensis based on 16S rRNA gene. Conventional PCR based on the msp4 genes was employed to detect A. marginale and A. ovis, while 16S rRNA gene for detection of A. capra. PCR primers and cycling conditions used in this study, as shown in Table 1. The DNAs extracted from the whole blood of Tibetan sheep and yaks infected with A. bovis, A. phagocytophilum, A. ovis, A. capra, and E. chaffeensis that had been verified by sequencing, were used as a positive control for corresponding PCR reactions; double-distilled water was used as a negative control. The PCR products were detected by 1.5% agarose gel electrophoresis with M5 Hipure Next III Gelred (Mei5 Biotechnology Co., Ltd., Beijing, China) stained.
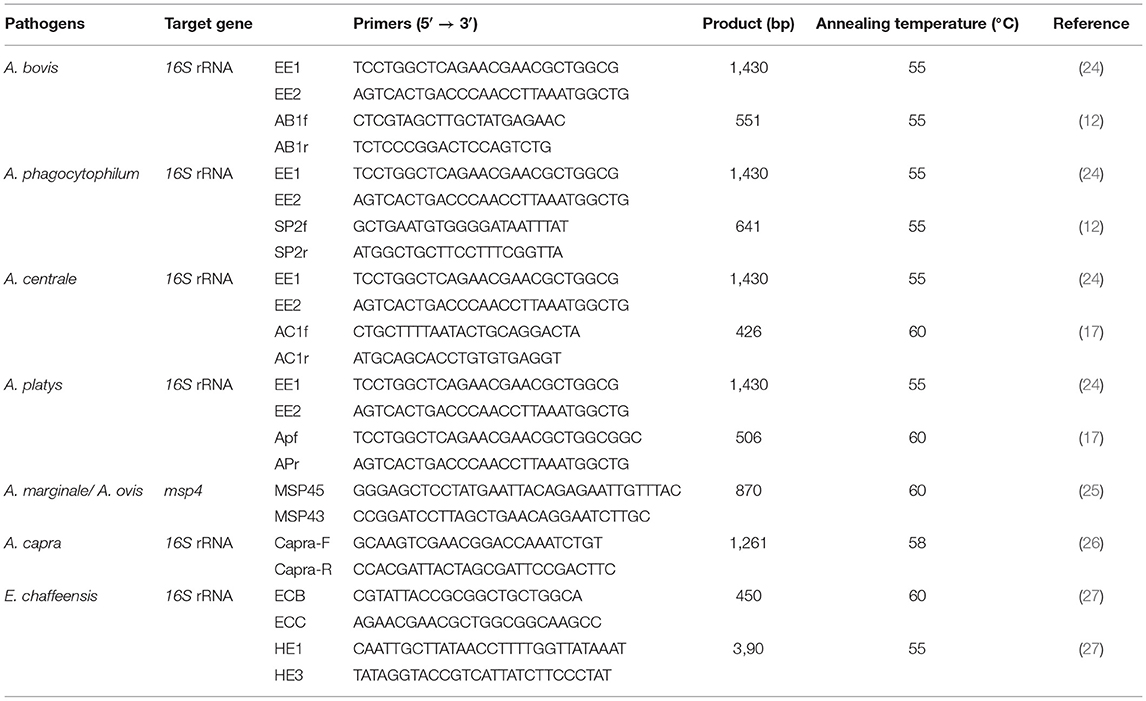
Table 1. Primers used in this study to detect Anaplasma spp. and E. chaffeensis in Tibetan sheep and yaks in Qinghai, China.
Sequencing and Phylogenetic Analysis
PCR products of all positive samples for Anaplasma spp. and E. chaffeensis randomly selected from each sampling site were sequenced by BGI (Beijing, China). The sequence obtained by BGI sequencing was submitted to NCBI for BLASTn search (https://blast.ncbi.nlm.nih.gov/Blast.cgi), and sequence alignment and analysis. The representative nucleotide sequences of this study have been deposited in the GenBank database. Phylogenetic trees were constructed using the neighbor-joining method executed with the p-distance model in MEGA X. Bootstrap values were assessed with 1,000 bootstrap replicates (28, 29).
Statistical Analysis
The data were grouped into four variables according to animal species, gender, sampling sites, and the altitude of sampling sites. The chi-square test was used to calculate the difference of infection rate in SPSS 25.0 software in each group. When p < 0.05, the difference was significant.
Results
Prevalence of Anaplasma spp. and E. chaffeensis in Tibetan Sheep and Yaks
This study identified four Anaplasma species and E. chaffeensis from Tibetan sheep and yaks (Table 2). Of the 566 samples tested, 50% (283/566) were positive for at least one pathogen. The infection rates of A. bovis and A. ovis were 33.3% and 1.2% in yaks, 5.1% and 80.9% in Tibetan sheep. The infection rates of A. phagocytophilum, A. capra, and E. chaffeensis were 29.4%, 0.6%, and 0.6% in yaks, respectively. This is the first time that A. capra and E. chaffeensis have been detected in yaks in China. Interestingly, we noticed A. ovis infection in yaks and A. bovis in Tibetan sheep. The most common co-infection was A. bovis and A. phagocytophilum, with an infection rate of 28.2% (93/330) in yaks. For co-infection with three pathogens, the infection rate of A. bovis, A. phagocytophilum, and A. capra was 0.6% (2/330), and the infection rate of A. bovis, A. phagocytophilum, and E. chaffeensis was 0.6% (2/330) (Table 2). No co-infections by two or more pathogens were detected in Tibetan sheep.
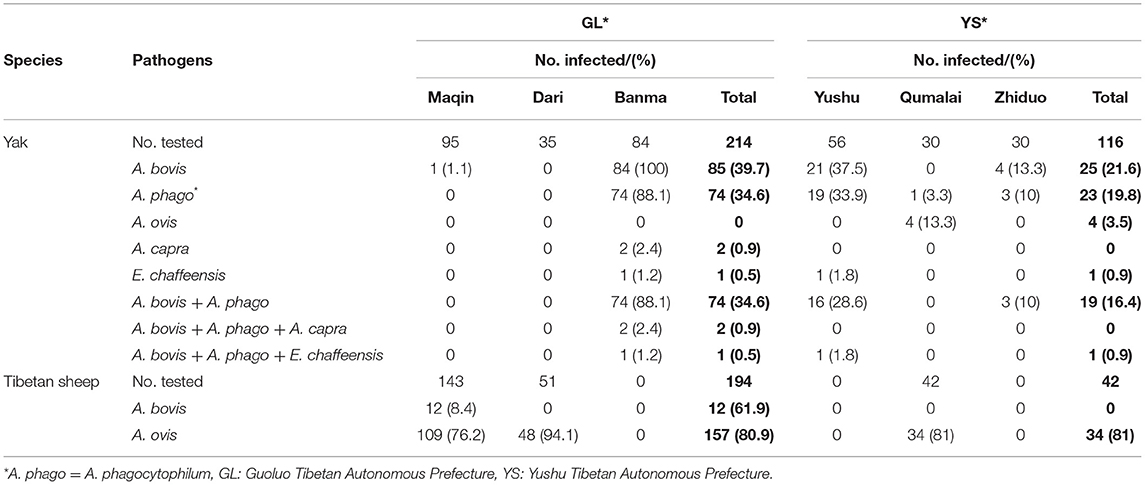
Table 2. The prevalence of Anaplasma spp. and E. chaffeensis in Tibetan sheep and yaks in Qinghai, China.
Sequencing and Phylogenetic Analysis
In the current study, 15 representative sequences were obtained and submitted to GenBank (Table 3). We compared and analyzed the partial 16S rRNA gene sequences of A. bovis, A. phagocytophilum, A. capra, and E. chaffeensis obtained from blood samples of Tibetan sheep and yaks. BLASTn analysis of the 16S rRNA gene showed that the Anaplasma spp. obtained in this study had 99.04–100% identities to either of A. bovis, A. phagocytophilum, A. capra, and E. chaffeensis sequences, respectively. The E. chaffeensis sequences (MW048788, MW048789) from yaks were 99.44–100% identical to E. chaffeensis isolated from goats (KX505292) in China. Phylogenetic analysis of 16S rRNA gene sequences confirmed A. bovis, A. phagocytophilum, A. capra, and E. chaffeensis in this study (Figures 2A,B, 3A,B). Additionally, we analyzed the msp4 genomic region of three A. ovis (MZ231113-MZ231115) obtained in this study. The results showed that the three sequences were consistent with the homology of the Iranian A. ovis (MH790273). A. ovis were classified as A. ovis msp4 Genotypes II based on T366C470 (25). Phylogenetic analysis of msp4 gene sequences confirmed the identity of A. ovis in this study (Figure 4).
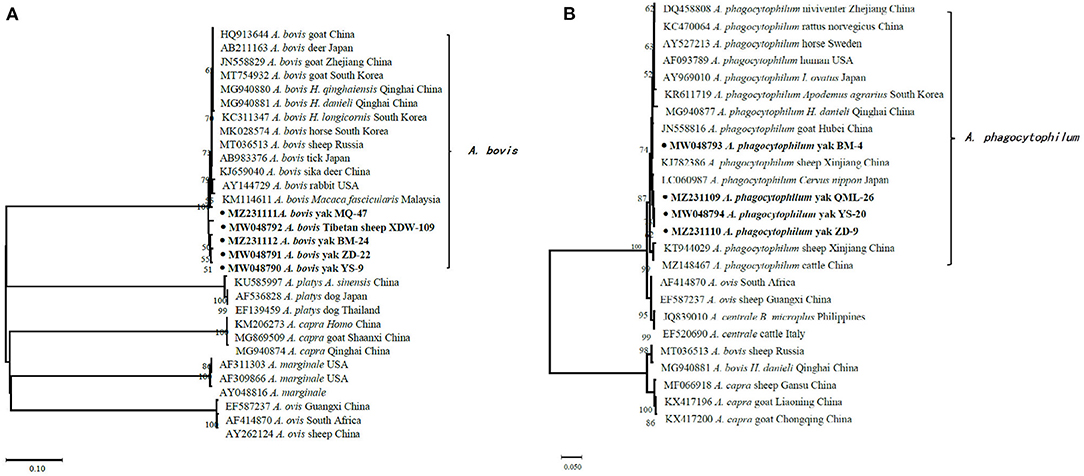
Figure 2. Phylogenetic trees were constructed based on partial sequences of the 16S rRNA genes of A. bovis (A) and A. phagocytophilum (B). Phylogenetic trees were con-structed by the neighbor-joining method with the p-distance model using the MEGA X software, and the bootstrap test was assessed with 1,000 replicates. The species identified in this study are indicated by • and highlighted in bold.
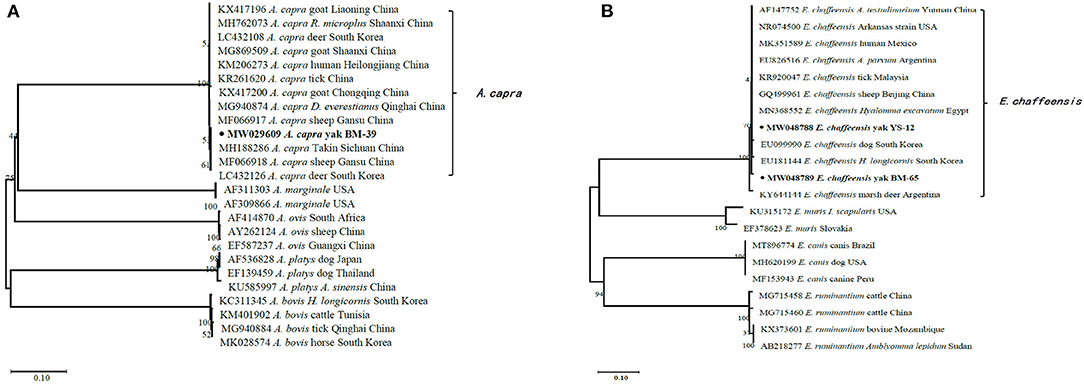
Figure 3. Phylogenetic trees were constructed based on partial sequences of the 16S rRNA genes of A. capra (A) and E. chaffeensis (B). Phylogenetic trees were constructed by the neighbor-joining method with the p-distance model using the MEGA X software, and the bootstrap test was assessed with 1,000 replicates. The species identified in this study are indicated by • and highlighted in bold.
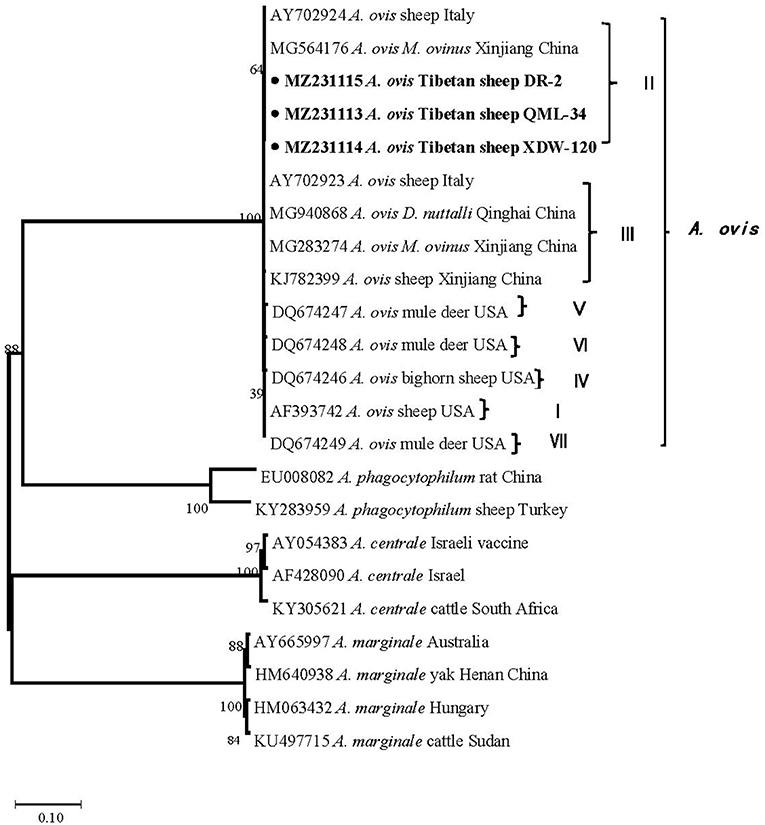
Figure 4. Phylogenetic trees were constructed based on the msp4 genes of A. ovis. Phylogenetic trees were constructed by the neighbor-joining method with the p-distance model using the MEGA X software, and the bootstrap test was assessed with 1,000 replicates. The species identified in this study are indicated by • and highlighted in bold.
Risk Factors of Tibetan Sheep and Yaks Infected With Anaplasma spp. and E. chaffeensis
These factors include animal species, gender, sampling sites, and altitude of sampling sites, which were used as variables for statistical analysis of the infection patterns of Anaplasma spp. and E. chaffeensis. The results indicate that the prevalence of Anaplasma spp. and E. chaffeensis in female animals was similar to that of male animals (P >0.05). The infection rates of A. bovis, A. phagocytophilum, and A. ovis in yaks in GL and YS were 39.7 and 21.6% (P = 0.001), 34.6 and 19.8% (P = 0.006), 0 and 3.5% (P = 0.005), respectively. In addition, the infection rate of A. bovis and A. phagocytophilum below 4,000 m was significantly higher than those above 4,000 m (P = 0.000). In Tibetan sheep, the infection rate of A. ovis above 4,000 m was higher than that below 4,000 m (P = 0.022) (Table 4).
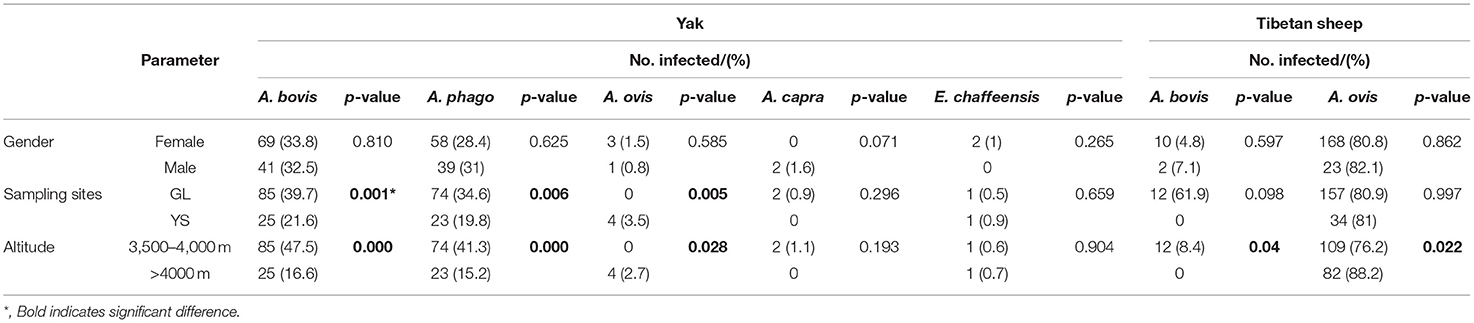
Table 4. Patterns of Anaplssasma spp. and E. chaffeensis prevalence in the yaks and Tibetan sheep, grouped by animal species, gender, sampling sites, and the altitude of sampling sites.
Discussion
In the present study, Anaplasma spp. and E. chaffeensis were investigated in domestic animals in Qinghai, China. Four Anaplasma species (A. bovis, A. phagocytophilum, A. ovis, and A. capra) and E. chaffeensis were identified in Tibetan sheep and yaks. Among them, E. chaffeensis and A. capra were detected in yaks for the first time in China.
The genus Anaplasma are widely distributed in domestic animals, wild animals, ticks, and other vectors (23, 30–32). This study found relatively high A. ovis infection rates of 76.2, 94.1, and 81.3% in Tibetan sheep in three sampling sites, Maqin, Dari, and Qumalai, respectively, which is higher than in sheep in Xinjiang (40.5%) (16) and Gansu (5.7%) (33), and Tibetan sheep in northeast Qinghai (58%) (34). An explanation for higher infection rates of A. ovis in this area could be the bites of ticks and other arthropods. Ticks and M. ovinus were found in Tibetan sheep in Maqin and Dari, and data on that M. ovinus carried A. ovis has been reported in our previous study (31). In addition, we carried out the comparative analysis and phylogenetic analysis of the msp4 gene sequence of A. ovis (25). The results showed that the A. ovis strains isolated from Tibetan sheep were identical to those isolated in M. ovinus in our previous study (31). Whereas, the A. ovis isolated from Dermacentor nuttalli in Qinghai by Han et al. (23) belongs to genotypes III, which is in the same clade as those obtained from sheep in Italy (Figure 4) (25). Genotypes II and III were also isolated from M. ovinus in Xinjiang by Zhao et al. (35). Remarkably, an A. ovis variant was reported in humans (36), indicating that this agent has zoonotic potential. Taken together, there are two A. ovis genotypes prevalent in domestic animals in northwest China, and arthropods (including M. ovinus and ticks) may be the main vectors of A. ovis.
A. phagocytophilum and A. bovis are frequently detected in ruminants around the world. This study confirms that both A. phagocytophilum and A. bovis can infect yaks. The infection rate of A. phagocytophilum in yaks (29.4%) in this study was higher than that reported in sheep (9.9%), dairy cattle (12%), and white yaks (5.3%) in other areas of China (1, 13, 37), and lower than that in C. pygargus (33.3%) from Heilongjiang China (17). Since the first case of HGA, caused by A. phagocytophilum, was reported in Anhui, China (38), HGA has been reported in the USA, Europe, Africa, and Asia (11, 39, 40). For A. bovis, the infection rate in yaks (33.3%) was higher than that in cattle (4.8%) and white yaks (6.2%) from China (16, 37), cattle (1.0%) from South Korea (20). Recent studies have shown that climate, altitude, longitude, latitude, season, tick bites, contact with wild animals, and feeding methods are important factors affecting Anaplasma infection (41). Previous reports have shown that Haemaphysalis qinghaiensis, Dermacentor abaensis, D. nuttalli, and Dermacentor silvarum are common ectoparasites among grazing livestock in high altitude areas (2,800 to 4,300 m), and the risk of tick bites with Anaplasma spp. was related to altitude and tick species (23). Our results also showed that the risk of infection with Anaplasma spp. in Tibetan sheep and yaks is mainly related to altitude and sampling sites. Furthermore, all animals in this study adopted a free grazing system, which increased the risk of domestic animals being exposed to ticks.
A. capra is a novel Anaplasma species that emerged in recent years. The novel species was first found in goats and then in sheep (30), C. pygargus (17), dogs (42), and ticks (23) in China. In addition, A. capra has also been detected in goats, cattle, and Hydropotes inermis argyropus in South Korea (32, 43), cattle in Malaysia (10), and Cervus elaphus and Rucervus duvaucelii in France (21). In 2015, it was isolated from the blood samples of patients with a history of tick bites in northeastern China (7). Subsequently, Peng et al. (44) confirmed the ability of A. capra to infect human erythrocytes, HL-60 and TF-1, and further confirmed its zoonotic characteristics. In this study, we detected A. capra DNA in yaks in China for the first time. In Qinghai, H. qinghaiensis is the most dominant tick species infected with A. capra, followed by D. abaensis and D. nuttalli (23). The above evidence suggests that A. capra is widely distributed and could infect a wide range of hosts.
Ehrlichia species include E. chaffeensis, E. canis, E. ewingii, E. equi, E. muris, and E. ruminantium. These species have been detected in many ticks in China, for instance, Amblyomma testudinarium, Haemaphysalis yeni, Haemaphysalis longicornis, Ixodes sinensis, D. silvarum, Rhipicephalus sanguineus, and Rhipicephalus microplus (45–48). In previous studies, E. canis infection was detected in Cervus nippon in Gansu (49), and high infection rates of E. canis and E. chaffeensis were reported in dogs, cattle, sheep, goats, donkeys, and humans in Xinjiang (9, 18, 50). Ehrlichia species were also detected in birds and small mammals in other parts of China (51, 52). In the current study, the prevalence rate of E. chaffeensis was 0.61%. We present the first report of Ehrlichia infection caused by E. chaffeensis in yaks in China. However, it is unclear which ticks are responsible for the pathogen. Therefore, further study is needed to determine the vector or reservoir host for this pathogen.
Moreover, mixed-infection is also an important issue that would need to be considered in livestock. The present study results illustrate that mixed infection of A. phagocytophilum and A. bovis are very common in yaks in Qinghai. Co-infection involving three Anaplasma species of A. bovis, A. phagocytophilum, and A. capra was also observed in two yaks in this study. In addition, we found that two yaks were co-infected with A. bovis, A. phagocytophilum and E. chaffeensis. Currently, A. phagocytophilum, A. capra, and E. chaffeensis have been recognized as causative agents of human infection. Mixed-infection of tick-borne pathogens has also been observed in animals in other countries and regions (1, 30, 34, 53). Above all, co-infection of tick-borne pathogens emphasizes the need for differential diagnosis of these pathogens in animal hosts and humans to improve the prevention and control of TBDs.
Notably, all pathogens were detected from apparently healthy animals in this study, consistent with other studies (54–56). This indicates that the appearance of clinical symptoms is mainly dependent on the pathogenicity of these pathogens strains and the breed or species of the infected animals (54). Alternatively, these animals have previously been infected with these pathogens and developed immunity against these pathogens (56). Further research is necessary to confirm these speculations.
In conclusion, we investigated the epidemic situation of the TBDs in yaks and Tibetan sheep in Qinghai province, China, and confirmed that Tibetan sheep and yaks could be infected with A. bovis, A. phagocytophilum, A. ovis, A. capra, and E. chaffeensis. This is the first report of A. capra and E. chaffeensis infection in yaks in China. These pathogens could pose a significant threat to livestock and human health. Thus, future studies should focus more on systematically assessing these pathogens' threats to veterinary and public health.
Data Availability Statement
The datasets presented in this study can be found in online repositories. The names of the repository/repositories and accession number(s) can be found in the article/supplementary material.
Ethics Statement
The Animal Ethics Committee of the Institute of Zoology, Chinese Academy of Sciences approved the procedures of collecting blood samples from Tibetan sheep and yaks, and obtained the livestock owner's consent. Written informed consent for participation was not obtained from the owners because all the samples in this study were collected by local veterinarians during the daily epidemic surveillance.
Author Contributions
YW: investigation, conceptualization, methodology, data curation, visualization, writing—original draft, and writing—review & editing. QZ: investigation, methodology, visualization, writing—original draft, and writing—review & editing. YL: investigation, methodology, data curation, and funding acquisition. SH: investigation, methodology, and writing—original draft. BW, GY, PZ, ZY, and HZ: investigation and methodology. YS, XH, and JC: investigation. HH: investigation, visualization, supervision, validation, writing—review & editing, and funding acquisition. All authors contributed to the article and approved the submitted version.
Funding
This work was supported by the Regular Assistance Project of the International Department of the Ministry of Science and Technology of China (KY201904013), the Chinese Academy of Sciences (CZBZX-1), National Forestry, and Grassland Administration, China.
Conflict of Interest
The authors declare that the research was conducted in the absence of any commercial or financial relationships that could be construed as a potential conflict of interest.
Publisher's Note
All claims expressed in this article are solely those of the authors and do not necessarily represent those of their affiliated organizations, or those of the publisher, the editors and the reviewers. Any product that may be evaluated in this article, or claim that may be made by its manufacturer, is not guaranteed or endorsed by the publisher.
Acknowledgments
We want to express our heartfelt thanks to all the herdsmen and all the Guoluo and Yushu Animal Disease Prevention and Control Center staff for their strong support for this study, enabling us to smoothly carry out the research.
References
1. Li Y, Galon EM, Guo Q, Rizk MA, Moumouni PFA, Liu M. et al. Molecular detection and identification of Babesia spp, Theileria spp, and Anaplasma spp in sheep from border regions, northwestern China. Front Vet Sci. (2020) 7:630. doi: 10.3389/fvets.2020.00630
2. Von Fricken ME, Voorhees MA, Koehler JW, Asbun C, Lam B, Qurollo B, et al. Molecular characteristics of Rickettsia in ticks collected along the southern border of mongolia. Pathogens. (2020) 9:943. doi: 10.3390/pathogens9110943
3. Liu Z, Ma M, Wang Z, Wang J, Peng Y, Li Y, et al. Molecular survey and genetic identification of Anaplasma species in goats from central and southern China. Appl Environ Microbiol. (2012) 78:464–70. doi: 10.1128/AEM.06848-11
4. Yang J, Liu Z, Niu Q, Mukhtar MU, Guan G, Liu G, et al. A novel genotype of “Anaplasma capra” in wildlife and its phylogenetic relationship with the human genotypes. Emerg Microbes Infect. (2018) 7:210. doi: 10.1038/s41426-018-0212-0
5. Dumler JS, Barbet AF, Bekker CP, Dasch GA, Palmer GH, Ray SC, et al. Reorganization of genera in the families rickettsiaceae and anaplasmataceae in the order rickettsiales: unification of some species of Ehrlichia with Anaplasma, Cowdria with Ehrlichia and Ehrlichia with Neorickettsia, descriptions of six new species combinations and designation of Ehrlichia equi and 'HGE agent' as subjective synonyms of Ehrlichia phagocytophila. Int J Syst Evol Microbiol. (2001) 51:2145–65. doi: 10.1099/00207713-51-6-2145
6. Battilani M, de Arcangeli S, Balboni A, Dondi F. Genetic diversity and molecular epidemiology of Anaplasma. Infect Genet Evol. (2017) 49:195–211. doi: 10.1016/j.meegid.2017.01.021
7. Li H, Zheng Y, Ma L, Jia N, Jiang B, Jiang R, et al. Human infection with a novel tick-borne Anaplasma species in China: a surveillance study. Lancet Infect Dis. (2015) 15:663–70. doi: 10.1016/S1473-3099(15)70051-4
8. Peng SH, Yang SL, Ho YN, Chen HF, Shu PY. Human case of Ehrlichia chaffeensis infection, Taiwan. Emerg Infect Dis. (2019) 25:2141–3. doi: 10.3201/eid2511.190665
9. Chahan B, Jian Z, Xuan X, Sato Y, Kabeya H, Tuchiya K, et al. Serological evidence of infection of Anaplasma and Ehrlichia in domestic animals in xinjiang uygur autonomous region area, China. Vet Parasitol. (2005) 134:273–8. doi: 10.1016/j.vetpar.2005.07.024
10. Koh FX, Panchadcharam C, Sitam FT, Tay ST. Molecular investigation of Anaplasma spp. in domestic and wildlife animals in Peninsular Malaysia. Vet Parasitol Reg Stud Reports. (2018) 13:141–7. doi: 10.1016/j.vprsr.2018.05.006
11. Rodino KG, Theel ES, Pritt BS. Tick-borne diseases in the United States. Clin Chem. (2020) 66:537–48. doi: 10.1093/clinchem/hvaa040
12. Kawahara M, Rikihisa Y, Lin Q, Isogai E, Tahara K, Itagaki A, et al. Novel genetic variants of Anaplasma phagocytophilum, Anaplasma bovis, Anaplasma centrale, and a novel Ehrlichia sp. in wild deer and ticks on two major islands in Japan. Appl Environ Microbiol. (2006) 72:1102–9. doi: 10.1128/AEM.72.2.1102-1109.2006
13. Yan Y, Jiang Y, Tao D, Zhao A, Qi M, Ning C. Molecular detection of Anaplasma spp. in dairy cattle in southern Xinjiang, China. Vet Parasitol Reg Stud Reports. (2020) 20:100406. doi: 10.1016/j.vprsr.2020.100406
14. Dahlgren FS, Mandel EJ, Krebs JW, Massung RF, McQuiston JH. Increasing incidence of Ehrlichia chaffeensis and Anaplasma phagocytophilum in the United States, 2000-2007. Am J Trop Med Hyg. (2011) 85:124–31. doi: 10.4269/ajtmh.2011.10-0613
15. Yang J, Liu Z, Guan G, Liu Q, Li Y, Chen Z, et al. Prevalence of Anaplasma phagocytophilum in ruminants, rodents and ticks in Gansu, north-western China. J Med Microbiol. (2013) 62:254–8. doi: 10.1099/jmm.0.046771-0
16. Yang J, Li Y, Liu Z, Liu J, Niu Q, Ren Q, et al. Molecular detection and characterization of Anaplasma spp. in sheep and cattle from Xinjiang, northwest China. Parasit Vectors. (2015) 8:108. doi: 10.1186/s13071-015-0727-3
17. Wang H, Yang J, Mukhtar MU, Liu Z, Zhang M, Wang X. Molecular detection and identification of tick-borne bacteria and protozoans in goats and wild siberian roe deer (Capreolus pygargus) from Heilongjiang Province, northeastern China. Parasit Vectors. (2019) 12:296. doi: 10.1186/s13071-019-3553-1
18. Zhang L, Liu H, Xu B, Zhang Z, Jin Y, Li W, et al. Rural residents in China are at increased risk of exposure to tick-borne pathogens Anaplasma phagocytophilum and Ehrlichia chaffeensis. Biomed Res Int. (2014) 2014:313867. doi: 10.1155/2014/313867
19. Mogg M, Wang HH, Baker A, Derouen Z, Borski J, Grant WE. Increased Incidence of Ehrlichia chaffeensis infections in the United States, 2012 through 2016. Vector Borne Zoonotic Dis. (2020) 20:547–50. doi: 10.1089/vbz.2019.2595
20. Seo MG, Ouh IO, Lee H, Geraldino PJL, Rhee MH, Kwon OD, et al. Differential identification of Anaplasma in cattle and potential of cattle to serve as reservoirs of Anaplasma capra, an emerging tick-borne zoonotic pathogen. Vet Microbiol. (2018) 226:15–22. doi: 10.1016/j.vetmic.2018.10.008
21. Jouglin M, Blanc B, De La Cotte N, Bastian S, Ortiz K, Malandrin L. First detection and molecular identification of the zoonotic Anaplasma capra in deer in France. PLoS ONE. (2019) 14:e0219184. doi: 10.1371/journal.pone.0219184
22. Chen Z, Yang X, Bu F, Yang X, Yang X, Liu J. Ticks (acari: ixodoidea: argasidae, ixodidae) of China. Exp Appl Acarol. (2010) 51:393–404. doi: 10.1007/s10493-010-9335-2
23. Han R, Yang JF, Mukhtar MU, Chen Z, Niu QL, Lin YQ, et al. Molecular detection of Anaplasma infections in ixodid ticks from the qinghai-tibet plateau. Infect Dis Poverty. (2019) 8:1–8. doi: 10.1186/s40249-019-0522-z
24. Barlough JE, Madigan JE, DeRock E, Bigornia L. Nested polymerase chain reaction for detection of Ehrlichia equi genomic DNA in horses and ticks (Ixodes pacificus). Veterinary Parasitology. (1996) 63:319–29. doi: 10.1016/0304-4017(95)00904-3
25. De La Fuente J, Atkinson MW, Naranjo V, de Mera IGF, Mangold AJ, Keating KA, et al. Sequence analysis of the msp4 gene of Anaplasma ovis strains. Vet Microbiol. (2007) 119:375–81. doi: 10.1016/j.vetmic.2006.09.011
26. Yang J, Liu Z, Niu Q, Liu J, Han R, Liu G, et al. Molecular survey and characterization of a novel Anaplasma species closely related to Anaplasma capra in ticks, northwestern China. Parasit Vectors. (2016) 9:603. doi: 10.1186/s13071-016-1886-6
27. Dawson JE, Stallknecht DE, Howerth EW, Warner C, Biggie K, Davidson WR, et al. Susceptibility of white-tailed deer (Odocoileus virginianus) to infection with Ehrlichia chaffeensis, the etiologic agent of human ehrlichiosis. J Clin Microbiol. (1994) 32:2725–8. doi: 10.1128/jcm.32.11.2725-2728.1994
28. Kumar S, Tamura K, Nei M, MEGA. Molecular evolutionary genetics analysis software for microcomputers. Comput Appl Biosci. (1994) 10:189–91. doi: 10.1093/bioinformatics/10.2.189
29. Saitou N, Nei M. The neighbor-joining method: a new method for reconstructing phylogenetic trees. Mol Biol Evol. (1987) 4:406–25. doi: 10.1093/oxfordjournals.molbev.a040454
30. Shi Y, Yang J, Guan G, Liu Z, Luo J, Song M. Molecular investigation of Anaplasma species in sheep from Heilongjiang province, northeast China identified four Anaplasma species and a novel genotype of Anaplasma capra. Parasitol Int. (2020) 76:102072. doi: 10.1016/j.parint.2020.102072
31. Zhang QX, Wang Y, Li Y, Han SY, Wang B, Yuan GH, et al. Vector-borne pathogens with veterinary and public health significance in Melophagus ovinus (sheep ked) from the Qinghai-Tibet Plateau. Pathogens. (2021) 10:249. doi: 10.3390/pathogens10020249
32. Amer S, Kim S, Yun Y, Na KJ. Novel variants of the newly emerged Anaplasma capra from Korean water deer (Hydropotes inermis argyropus) in South Korea. Parasit Vectors. (2019) 12:365. doi: 10.1186/s13071-019-3622-5
33. Yang J, Han R, Niu Q, Liu Z, Guan G, Liu G, et al. Occurrence of four Anaplasma species with veterinary and public health significance in sheep, northwestern China. Ticks Tick Borne Dis. (2018) 9:82–5. doi: 10.1016/j.ttbdis.2017.10.005
34. Li J, Jian Y, Jia L, Galon EM, Benedicto B, Wang G, et al. Molecular characterization of tick-borne bacteria and protozoans in yaks (Bos grunniens), Tibetan sheep (Ovis aries) and Bactrian camels (Camelus bactrianus) in the Qinghai-Tibetan Plateau Area, China. Ticks Tick Borne Dis. (2020) 11:101466. doi: 10.1016/j.ttbdis.2020.101466
35. Zhao L, He B, Li KR, Li F, Zhang LY, Li XQ, et al. First report of Anaplasma ovis in pupal and adult Melophagus ovinus (sheep ked) collected in South Xinjiang, China. Parasit Vectors. (2018) 11:258. doi: 10.1186/s13071-018-2788-6
36. Chochlakis D, Ioannou I, Tselentis Y, Psaroulaki A. Human anaplasmosis and Anaplasma ovis variant. Emerg Infect Dis. (2010) 16:1031–2. doi: 10.3201/eid1606.090175
37. Yang J, Liu Z, Niu Q, Liu J, Guan G, Xie J, et al. First molecular survey and identification of Anaplasma spp. in white yaks (bos grunniens) in China. Parasitology. (2016) 143:686–91. doi: 10.1017/S003118201600041X
38. Zhang L, LY, Ni D, Li Q, Yu Y, Yu XJ, et al. Nosocomial transmission of human granulocytic anaplasmosis in China. JAMA. (2008) 300:2263–70. doi: 10.1001/jama.2008.626
39. Zhang L, Wang G, Liu Q, Chen C, Li J, Long B, et al. Molecular analysis of Anaplasma phagocytophilum isolated from patients with febrile diseases of unknown etiology in China. PLoS ONE. (2013) 8:e57155. doi: 10.1371/journal.pone.0057155
40. Negi T, Kandari LS, Arunachalam K. Update on prevalence and distribution pattern of tick-borne diseases among humans in India: a review. Parasitol Res. (2021) 120:1523–39. doi: 10.1007/s00436-021-07114-x
41. Noaman V. Epidemiological study on Anaplasma phagocytophilum in cattle: molecular prevalence and risk factors assessment in different ecological zones in Iran. Prev Vet Med. (2020) 183:105118. doi: 10.1016/j.prevetmed.2020.105118
42. Shi K, Li J, Yan Y, Chen Q, Wang K, Zhou Y, et al. Dogs as new hosts for the emerging zoonotic pathogen Anaplasma capra in China. Front Cell Infect Microbiol. (2019) 9:394. doi: 10.3389/fcimb.2019.00394
43. Seo HJ, Jin BC, Kim KH, Yoo MS, Seong KW, Jeong SJ, et al. Molecular detection and phylogenetic analysis of Anaplasma spp. in Korean native goats from ulsan metropolitan city, Korea. Vector Borne Zoonotic Dis. (2019) 19:773–6. doi: 10.1089/vbz.2018.2374
44. Peng Y, Lu C, Yan Y, Song J, Pei Z, Gong P, et al. The novel zoonotic pathogen, Anaplasma capra, infects human erythrocytes, HL-60, and TF-1 cells in vitro. Pathogens. (2021) 10:600. doi: 10.3390/pathogens10050600
45. Hou J, Ling F, Liu Y, Zhang R, Song X, Huang R, et al. A molecular survey of Anaplasma, Ehrlichia, Bartonella and Theileria in ticks collected from southeastern China. Exp Appl Acarol. (2019) 79:125–35. doi: 10.1007/s10493-019-00411-2
46. Chen Z, Liu Q, Liu JQ, Xu BL, Lv S, Xia S, et al. Tick-borne pathogens and associated co-infections in ticks collected from domestic animals in central China. Parasit Vectors. (2014) 7:237. doi: 10.1186/1756-3305-7-237
47. Cao WC, Gao YM, Zhang PH, Zhang XT, Dai QH, Dumler JS, et al. Identification of Ehrlichia chaffeensis by nested PCR in ticks from southern China. J Clin Microbiol. (2000) 38:2778–80. doi: 10.1128/JCM.38.7.2778-2780.2000
48. Dong T, Qu Z, Zhang L. Detection of A phagocytophilum and E chaffeensis in patient and mouse blood and ticks by a duplex real-time PCR assay. PLoS ONE. (2013) 8:e74796. doi: 10.1371/journal.pone.0074796
49. Li Y, Chen Z, Liu Z, Liu J, Yang J, Li Q, et al. Molecular survey of Anaplasma and Ehrlichia of red deer and sika deer in gansu, China in 2013. Transbound Emerg Dis. (2016) 63:e228–36. doi: 10.1111/tbed.12335
50. Mengfan Q, Lixia W, Ying L, Yan R, Kuojun C, Jinsheng Z, et al. Molecular detection and genetic variability of Ehrlichia canis in pet dogs in Xinjiang, China. Vet World. (2020) 13:916–22. doi: 10.14202/vetworld.2020.916-922
51. Yang J, Liu Z, Niu Q, Tian Z, Liu J, Guan G, et al. Tick-borne zoonotic pathogens in birds in Guangxi, Southwest China. Parasit Vectors. (2015) 8:637. doi: 10.1186/s13071-015-1249-8
52. Du CH, Liu HB, Wei R, Jongejan F, Gao ZH, Shao ZT, et al. Investigation on Ehrlichia infection in small mammals and ticks from tengchong, Yunnan Province, Southern China. Vector Borne Zoonotic Dis. (2018) 18:563–6. doi: 10.1089/vbz.2017.2205
53. Miranda EA, Han SW, Cho YK, Choi KS, Chae JS. Co-infection with Anaplasma species and novel genetic variants detected in cattle and goats in the Republic of Korea. Pathogens. (2021) 10:28. doi: 10.3390/pathogens10010028
54. Chatanga E, Kainga H, Maganga E, Hayashida K, Katakura K, Sugimoto C, et al. Molecular identification and genetic characterization of tick-borne pathogens in sheep and goats at two farms in the central and southern regions of Malawi. Ticks Tick Borne Dis. (2021) 12:101629. doi: 10.1016/j.ttbdis.2020.101629
55. Sazmand A, Harl J, Eigner B, Hodzic A, Beck R, Hekmatimoghaddam S, et al. Vector-borne bacteria in blood of camels in Iran: new data and literature review. Comp Immunol Microbiol Infect Dis. (2019) 65:48–53. doi: 10.1016/j.cimid.2019.04.004
56. Ringo AE, Aboge GO, Adjou Moumouni PF, Lee SH, Jirapattharasate C, Liu M, et al. Molecular detection and genetic characterisation of pathogenic Theileria, Anaplasma and Ehrlichia species among apparently healthy sheep in central and western Kenya. Onderstepoort J Vet Res. (2019) 86:e1–8. doi: 10.4102/ojvr.v86i1.1630
Keywords: tick-borne disease, Anaplasma capra, Ehrlichia chaffeensis, Tibetan sheep, yak, Qinghai
Citation: Wang Y, Zhang Q, Han S, Li Y, Wang B, Yuan G, Zhang P, Yang Z, Zhang H, Sun Y, Chen J, Han X and He H (2021) Ehrlichia chaffeensis and Four Anaplasma Species With Veterinary and Public Health Significance Identified in Tibetan Sheep (Ovis aries) and Yaks (Bos grunniens) in Qinghai, China. Front. Vet. Sci. 8:727166. doi: 10.3389/fvets.2021.727166
Received: 18 June 2021; Accepted: 06 September 2021;
Published: 30 September 2021.
Edited by:
Cornelia Silaghi, Friedrich-Loeffler-Institute, GermanyReviewed by:
Giulia Morganti, University of Perugia, ItalySnorre Stuen, Norwegian Veterinary Institute (NVI), Norway
Copyright © 2021 Wang, Zhang, Han, Li, Wang, Yuan, Zhang, Yang, Zhang, Sun, Chen, Han and He. This is an open-access article distributed under the terms of the Creative Commons Attribution License (CC BY). The use, distribution or reproduction in other forums is permitted, provided the original author(s) and the copyright owner(s) are credited and that the original publication in this journal is cited, in accordance with accepted academic practice. No use, distribution or reproduction is permitted which does not comply with these terms.
*Correspondence: Hongxuan He, aGVoeEBpb3ouYWMuY24=
†These authors have contributed equally to this work and share first authorship