- 1Department of Parasitology and Zoology, University of Veterinary Medicine, Budapest, Hungary
- 2HUN-REN-UVMB Climate Change: New Blood-Sucking Parasites and Vector-Borne Pathogens Research Group, Budapest, Hungary
- 3Seashore Environment and Fauna, Tarifa, Spain
- 4Plant Protection Institute, Centre for Agricultural Research, Budapest, Hungary
- 5Department of Plant Sciences, Albert Kázmér Faculty of Mosonmagyaróvár, Széchenyi István University, Mosonmagyaróvár, Hungary
Introduction: Whales, dolphins, and porpoises are susceptible to infections by protozoan and metazoan parasites.
Methods: In this study, tissue samples, as well as flatworms and roundworms, were collected from a common bottlenose dolphin (Tursiops truncatus), three short-beaked common dolphins (Delphinus delphis), two striped dolphins (Stenella coeruleoalba), a harbor porpoise (Phocoena phocoena), a long-finned pilot whale (Globicephala melas), and a fin whale (Balaenoptera physalus). These samples were molecularly analyzed.
Results: In one D. delphis, Toxoplasma gondii was detected in multiple organs, including the cerebellum. The cysts of the tapeworms Clistobothrium delphini and Clistobothrium grimaldii were identified in G. melas. Flukes collected from D. delphis belong to Brachycladium atlanticum, while those removed from S. coeruleoalba probably represent a new species. Four species of lungworms were also identified: Halocercus delphini in S. coeruleoalba, Halocercus sp. in T. truncatus, Stenurus globicephalae in G. melas, and a potentially new Pharurus sp. in P. phocoena.
Conclusion: These findings show, to the best of our knowledge, for the first time, the presence of T. gondii DNA in D. delphis. The cerebellum of the animal was Toxoplasma-infected, which might be relevant to inadvertent stranding. In this study, new genetic markers were sequenced for several helminth parasites of marine mammals, possibly including undescribed species.
Introduction
Cetaceans (Artiodactyla: Cetacea), including whales, dolphins, and porpoises, are streamlined aquatic mammals that spend their whole life in water. All of them are carnivorous, taking either many small preys by bulk filter-feeding (parvorder Mysticeti: baleen whales) or larger prey by echolocation-assisted hunting (parvorder Odontoceti: dolphins, porpoises, toothed whales) (1). Cetaceans include the largest animals of all extinct and extant species and range through all oceans and into some rivers (1).
Cetaceans living under sea conditions represent an integral part of marine ecosystems. Many of their representatives are highly protected, or even critically endangered species. This makes it especially important to study pathogenic microorganisms (viruses, bacteria, and protozoa) as well as parasitic worms they might carry because some of these severely impact their health status (2). This is especially true in an era of climate change when seawater is becoming increasingly contaminated with land-derived microorganisms, such as waterborne bacteria and parasites, that reach the marine environment from freshwaters on the continental mainland due to flooding (3, 4). The most relevant examples of marine or land-derived pathogens affecting cetaceans are those that may underlie their frequently enigmatic stranding, a phenomenon that represents a global problem but also affects the ecosystems of the Mediterranean Sea (5).
At the same time, cetaceans also play a significant role in the epidemiology of those pathogens that have high veterinary-medical significance from the point of view of a broader range of mammals (6), even human beings. The latter is well-exemplified by Toxoplasma gondii (7). Although in most countries with maritime boundaries, marine mammals are usually not caught or directly used for human consumption, they can still participate in the natural transmission cycle of zoonotic bacteria (8) and protozoan parasites (9).
Cetaceans of the Mediterranean Sea include at least six endangered species, and two others categorized as vulnerable (10). Until the era of molecular methods, studies on their pathogenic microbiota were frequently based on serological tests, which are also used nowadays (11). However, detecting systemic antibodies is not always informative on the active status of infection and frequently precludes the organ-specific evaluation of potential causes. Another shortcoming of available data is relevant to groups of protozoan parasites in the marine environment that may cause neurological diseases [e.g., Acanthamoeba: (12)], but for which marine mammals are seldom if ever tested. Last but not least, molecular studies on the helminth fauna of cetaceans appear to show an increasing tendency, with potentially new species also discovered in the marine environment surrounding continental Europe [(e.g., 13)]. Despite this, only a minority of worm species associated with cetaceans were barcoded, and only some of them have broadly accessible sequence data in GenBank (e.g., among lung-associated nematodes, only 4 out of the 13 Halocercus spp.).
The present study was initiated to contribute to our knowledge in the above context. Tissue samples were obtained from various organs of nine individuals of six cetacean species that were found dead in the coastal area of the Strait of Gibraltar (southern Spain). These samples were examined with polymerase chain reaction (PCR) and sequencing for the presence of protozoan parasites known to cause parasitemia and neurologic diseases in marine and/or other mammals, such as Acanthamoeba and cystogenic coccidia. In addition, the worms recovered during pathological examination, representing all three major groups (flukes, cestodes, and nematodes), were evaluated taxonomically with molecular-phylogenetic analyses.
Materials and methods
Sample collection
Tissue and parasite samples were collected from stranded and dead cetaceans [a common bottlenose dolphin (Tursiops truncatus), three short-beaked common dolphins (Delphinus delphis), two striped dolphins (Stenella coeruleoalba), a harbor porpoise (Phocoena phocoena), a long-finned pilot whale (Globicephala melas), and a fin whale (Balaenoptera physalus)] during official necropsies regularly conducted in Algeciras, Spain, between August and December 2022. The necropsies were led by the veterinary team of the NGO Seashore Ambiental at the facilities of the Center for Management of Marine Environment (CEGMA), which belongs to Clearwater Marine Aquarium Sea (CMASEA), Andalusian Regional Government (AMAYA), and is responsible for managing and coordinating the stranding network in Andalusia through its Marine Environment Program.
A sampling protocol and pre-numbered tube sampling system were implemented in the fieldwork. All relevant data were recorded according to tube numbers, including place and date of finding, the name of cetacean species, and any observable information regarding age, sex, and general condition of the individual. The organ location of parasites and the parasite load were also documented, and pictures of lesions and parasites were made on the premises.
Tissue samples were taken from the muscle, lungs, liver, spleen, blood, medulla, cerebellum, and encephalon.
Nematodes and flukes were placed into 96% pure ethanol. Because of varying degrees of autolysis, their morphological identification was not attempted. Native tissue samples (removed with sterile scalpel blades or scissors from the middle of the relevant organ) were placed into empty 2 mL or 9 mL prelabelled screw-cap Sarstedt tubes (Sarstedt, Nümbrecht, Germany), depending on the size of the sample. The tissue samples were frozen at −20°C until use, but the worms soaked in ethanol were stored at room temperature. In this way, 31 tissue and 17 parasite samples were collected from 9 cetacean individuals (Supplementary Table S1).
DNA extraction and molecular analyses
DNA was extracted using the QIAamp DNA Mini Kit (QIAGEN, Hilden, Germany), following the manufacturer’s instructions. Extraction controls (tissue lysis buffer) were processed with each set of samples to monitor cross-contamination.
PCR reactions are summarized according to primers and cycling conditions in Supplementary Table S2. The reaction components were included in a volume of 25 μL, containing 1 U (stock 5 U/μl) HotStarTaq Plus DNA Polymerase, 2.5 μL of 10 × CoralLoad reaction buffer (including 15 mM MgCl2), 0.5 μL of PCR nucleotide Mix (stock 10 mM), 0.5 μL of each primer (stock 50 μM), 15.8 μL of ddH2O, and 5 μL of template DNA. In all PCRs, a non-template reaction mixture served as the negative control. The extraction controls and negative controls remained PCR-negative in all tests.
Sequencing and phylogenetic analyses
Purification and sequencing of the PCR products were performed by Biomi Ltd. (Gödöllő, Hungary). Quality control and trimming of sequences were performed with the BioEdit program. Obtained sequences were compared to GenBank sequences by the nucleotide BLASTN program.1 Sequences were submitted to GenBank (Table 1).
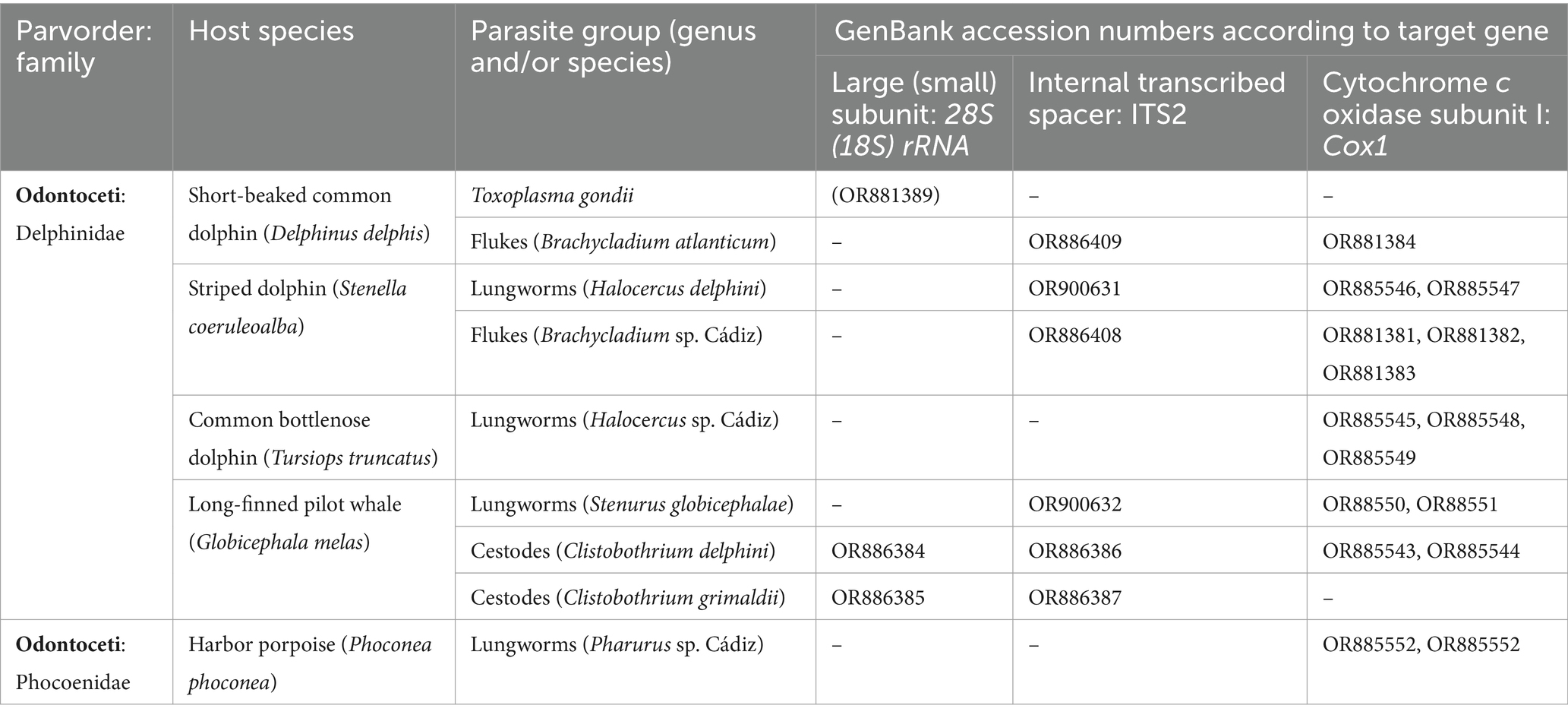
Table 1. Parasite genera and species are shown according to the target gene and GenBank accession numbers of sequences based on which they were identified in this study.
Sequences from other studies (retrieved from GenBank) included in the phylogenetic analyses had approximately or exactly 100% coverage with sequences from this study. Sequence datasets were resampled 1,000 times to generate bootstrap values. Phylogenetic analyses were conducted with the maximum likelihood method with the MEGA version 7.0 software.
Results
Molecular screening and analyses of protozoan parasites
All tissue DNA extracts were negative for Acanthamoeba spp. However, in the general PCR for cystogenic coccidia (Apicomplexa: Sarcocystidae), four samples were positive. These originated from the lungs, muscles, cerebrum, and cerebellum of the same short-beaked common dolphin. In these organs, Toxoplasma gondii was detected with sequencing (Table 1). The amplified part of the small-subunit 18S rRNA gene showed 100% identity with several GenBank sequences, including the highly pathogenic RH strain (e.g., EF472967 and U17349). This was confirmed with Toxoplasma-specific primers of the repeat region in its genome. The liver, spleen, and blood samples of the same animal were PCR-negative.
Molecular analyses of tapeworms (Cestoda: Phyllobothriidae)
Cysts (merocercoids) of cestodes were present in the blubber of a long-finned pilot whale (Figure 1A). The ITS2 sequences of the two larvae were only 97.8% identical to each other, suggesting that they belonged to different species. The longer part of the cox1 gene was also successfully amplified from one of the cysts, and its ITS2 sequence confirmed its closest relationship with the genus Clistobothrium, with 98.6% ITS2 and 89.6% cox1 sequence identity to previously reported sequences (KU724058 and KU987913, respectively) from cape fur seals (Arctocephalus pusillus). Another, shorter (377 bp) part of the cox1 gene showed clustering of this isolate as a sister group to members of the family Phyllobothriidae, including the genus Clistobothrium, although with low support (Supplementary Figure S1).
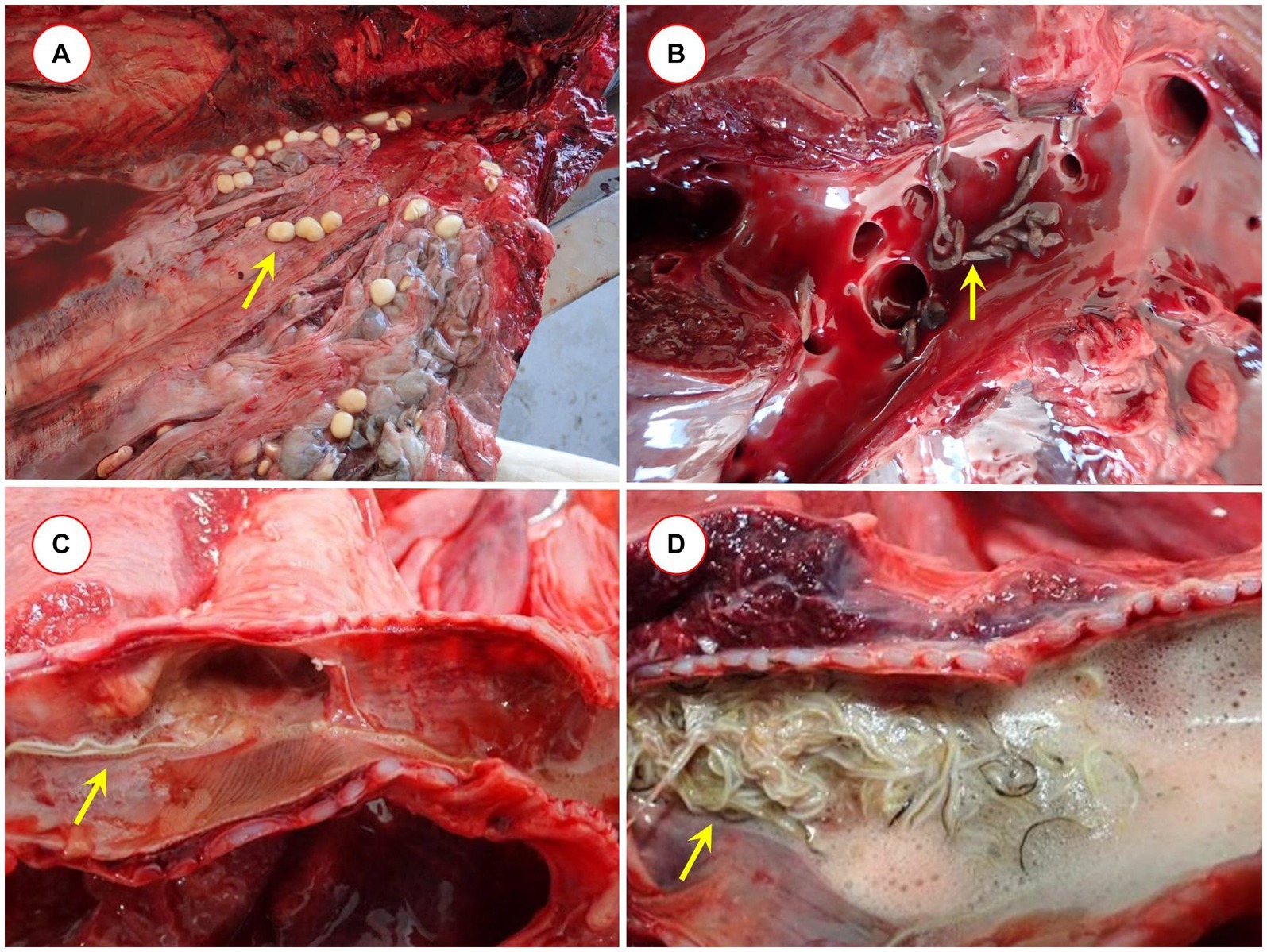
Figure 1. Pathological findings: (A) Clistobothrium sp. in the blubber of Globicephala melas, (B) Brachycladium sp. in the hepatic ducts of Stenella coeruleoalba, and (C) and (D) Halocercus sp. in the respiratory passages of Tursiops truncatus.
A 1280 bp-long fragment of the 28S rRNA gene was also successfully amplified from both cysts. One of them, the same as above, showed 100% sequence identity to Clistobothrium delphini from a striped dolphin reported previously from Spain (AY741600). The other had 100% sequence identity to Clistobothrium grimaldii from A. pusillus sampled in Japan (LC718556). Based on the 28S rRNA gene, all three Clistobothrium species included in the phylogenetic analysis formed a monophyletic clade, in which the clustering of C. delphini and C. grimaldii was well-supported (with 93%: Figure 2). The ITS2 or cox1 sequences of these tapeworm species were not available in GenBank for comparison.
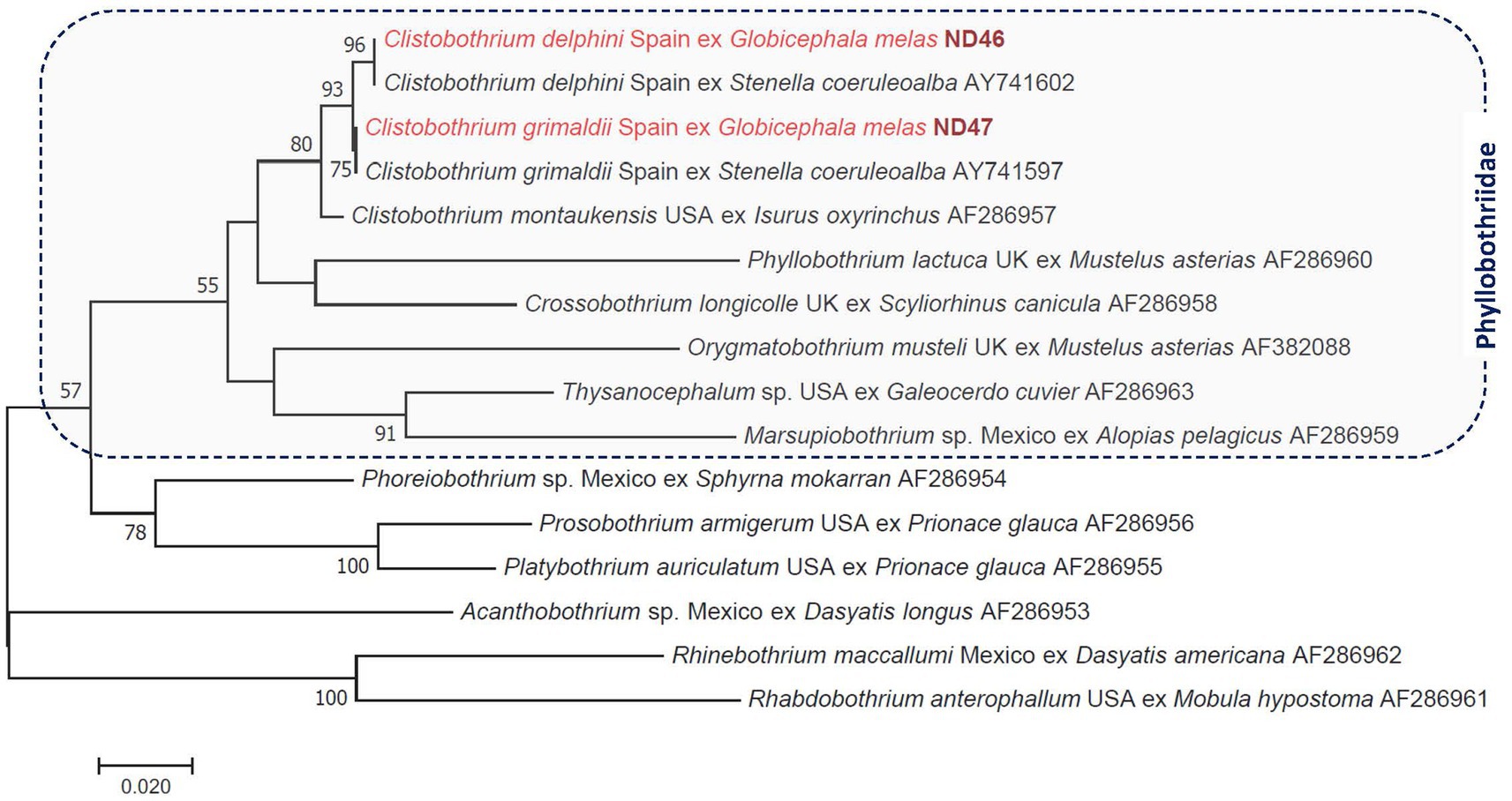
Figure 2. Phylogenetic tree of tapeworms (Cestoda: Phyllobothriidae, Onchobothriidae, Rhinebothriidae) based on the 28S rRNA gene. In each row of sequences, after the helminth species name, the isolation source (host), the country or region of origin, and the GenBank accession number are shown. Sequences from this study are indicated with red fonts and bold, maroon accession numbers. The evolutionary history was inferred by using the maximum likelihood method based on the general time-reversible model. The tree is drawn to scale, with branch lengths measured in the number of substitutions per site. The analysis involved 16 nucleotide sequences. All positions containing gaps and missing data were eliminated. There were a total of 639 positions in the final dataset.
Molecular analyses of flukes (Trematoda: Brachycladiidae)
Flukes were collected from two species of dolphins (Figure 1B). The fluke found on the peritoneum and in the liver of a short-beaked common dolphin was molecularly identified as Brachycladium atlanticum, on account of its 100% ITS2 sequence identity to this species (FJ211250). The cox1 sequence of B. atlanticum was 89.5% identical to that of B. goliath (NC_029757) and was hitherto not available, but was submitted from this study to GenBank.
In the case of flukes from the pylorus, gallbladder, and hepatic ducts of a striped dolphin (n = 3), the cox1 gene sequences indicated slight intraspecific genetic variation and only 87–87.5% sequence identity to the most closely related species, Brachycladium goliath (NC_029757). The ITS2 sequences of these worms were identical to each other and most similar to that of B. goliath, meaning 98% identity to the corresponding sequence (KR703279) of this species. The phylogenetic analyses of concatenated genes confirmed these results, and the species from S. coeruleoalba (provisionally called here Brachycladium sp. Cádiz) clustered separately from both B. goliath and B. atlanticum, with moderately high (78%) support (Figure 3).
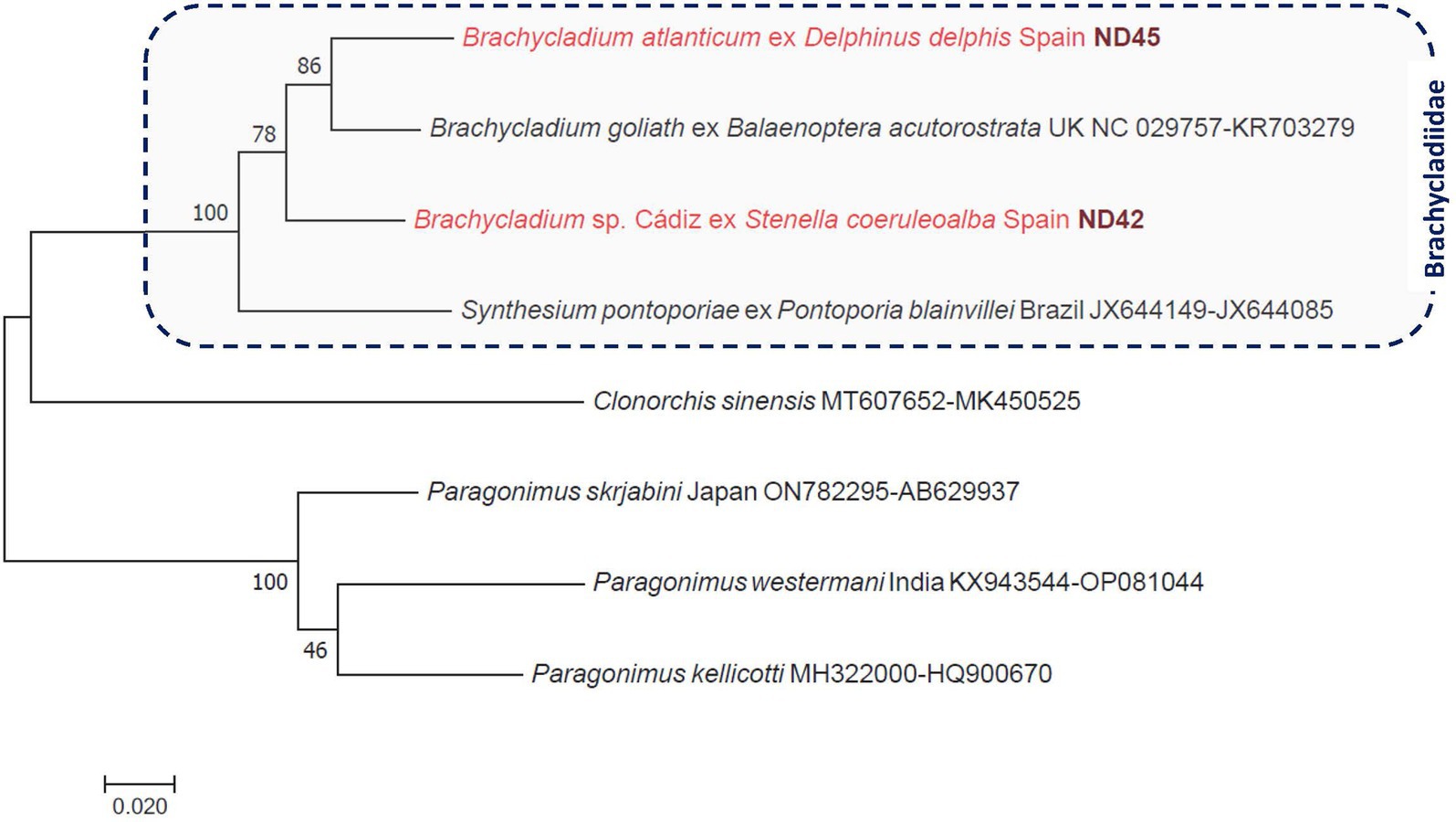
Figure 3. Phylogenetic tree of digenetic flukes (Trematoda: Brachycladiidae, Opisthorchiidae, Paragonimidae) based on concatenated cox1 and ITS2 sequences. In each row of sequences, after the helminth species name, the isolation source (host), the country or region of origin, and the GenBank accession number are shown. Sequences from this study are indicated with red fonts and bold, maroon accession numbers. The evolutionary history was inferred by using the maximum likelihood method based on the general time-reversible model. The tree is drawn to scale, with branch lengths measured in the number of substitutions per site. The analysis involved eight nucleotide sequences. All positions containing gaps and missing data were eliminated. There were a total of 811 positions in the final dataset.
Molecular analyses of lungworms (Nematoda: Pseudaliidae)
Lungworms were found in the respiratory tracts of four cetacean species (Figures 1C,D): S. coeruleoalba, T. truncatus, G. melas, and P. phocoena. In S. coeruleoalba, two genetic variants of Halocercus delphini were identified, according to their 96.6–99.2% cox1 sequence identity to a formerly reported sequence of this species (OQ200459). In T. truncatus, a different species (provisionally named Halocercus sp. Cádiz) showed only 93.9–94.4% cox1 sequence identity to the most closely related species, Halocercus pingi (OQ200469). From the pterygoid sinus of G. melas, Stenurus globicephalae was identified, based on its 99.1–99.4% cox1 sequence identity to this species (OQ200456), and in P. phocoena, a Pharurus species (tentatively called Pharurus sp. Cádiz), which showed the highest but only 90.2–90.6% cox1 sequence identity to Phanurus sunameri (OQ200467).
In the case of the lungworms from S. coeruleoalba and G. melas, ITS2 sequences confirmed the above species, with 100% identity to H. delphini (syn. Skrjabinalius guevarai: MN747502) and S. globicephalae (FJ787303). Based on the clustering of Halocercus sp. Cádiz and Pharurus sp. Cádiz sequences in the cox1 phylogenetic tree, these are species different from those already reported in GenBank, with high (99%) or moderately low (60%) support (Figure 4).
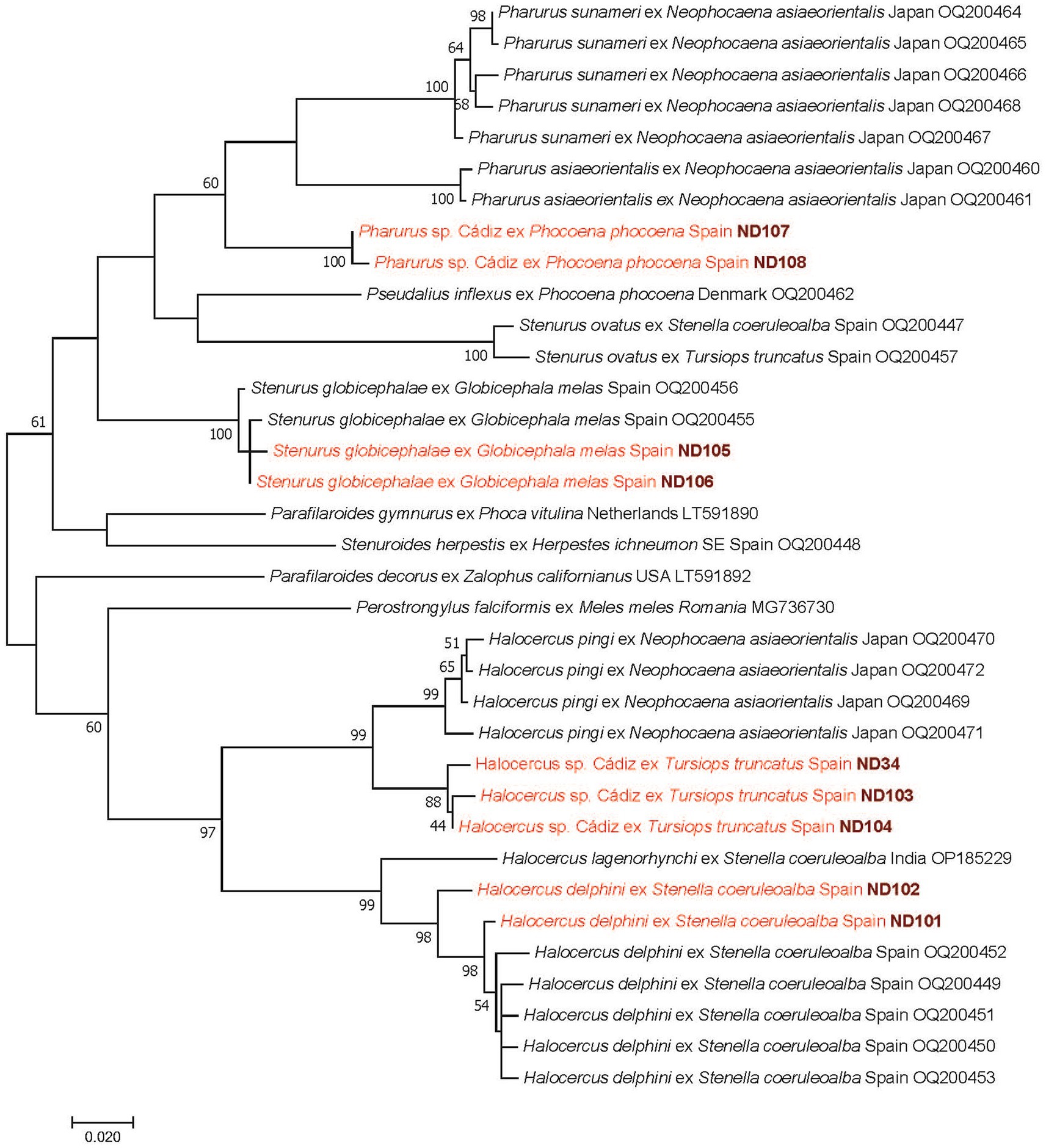
Figure 4. Phylogenetic tree of roundworms (Nematoda: Pseudaliidae) based on the cox1 gene. In each row of sequences, after the helminth species name, the isolation source (host), the country or region of origin, and the GenBank accession number are shown. Sequences from this study are indicated with red fonts and bold, maroon accession numbers. The evolutionary history was inferred by using the maximum likelihood method based on the Hasegawa–Kishino–Yano model. The tree is drawn to scale, with branch lengths measured in the number of substitutions per site. The analysis involved 35 nucleotide sequences. All positions containing gaps and missing data were eliminated. There were a total of 561 positions in the final dataset.
Discussion
Despite the limited sample size in this study, molecular data were obtained for the first time on several species of endoparasites that infect cetaceans in the Western Mediterranean region. Albeit less important in this context, the negative results of this study will also likely encourage further investigations with a similar aim. For instance, no Acanthamoeba DNA was detected in the central nervous system, although (A) these opportunistic parasites occur in the marine environment (12), (B) infection can be acquired from contaminated water, and (C) several members of the order Artiodactyla (which includes infraorder Cetacea) are known to be susceptible to these protozoa (14). Similarly, among cystogenic coccidia (Apicomplexa: Sarcocystidae), no evidence was found for any Sarcocystis species in the muscle or nervous system of marine mammals examined in this study, although recently a fatal Sarcocystis infection was reported from an Atlantic spotted dolphin (Stenella frontalis) (2).
However, the general PCR for cystogenic coccidia revealed the presence of T. gondii DNA in multiple organs of D. delphis. Considering that all previous studies reporting Toxoplasma-infection of this cetacean were based on immunological (serological) methods [in Europe: (15, 16)], to the best of our knowledge, this is the first molecular evidence to confirm T. gondii in D. delphis in a worldwide context. Disseminated toxoplasmosis was reported to cause stranding among cetaceans, both in Europe (17) and in other parts of the globe (18). In addition, PCR-positivity of the cerebrum and cerebellum also attests that this condition may have played a role in the stranding of the relevant animal, as the latter part of the CNS is particularly important in coordination and maintaining balance (19).
Among marine mammals, cetaceans have a higher prevalence of infection with T. gondii in the region of Europe than pinnipeds [14.8% vs. 2.8%: (11)]. For dolphins, the most likely source of acquiring Toxoplasma is probably not direct waterborne exposure [as they drink little or no water (6)], but rather via their prey items, most notably fish, which are frequent carriers of this protozoan parasite [prevalence in Europe: 21.8%: (11)]. Importantly, based on the present results, which indicated multi-organ involvement, and according to previous studies reporting high seroprevalence of toxoplasmosis in D. delphis (15, 16), this dolphin species should rank among highly Toxoplasma-susceptible dolphins, which hitherto included the common bottlenose dolphin, the striped dolphin in the Mediterranean Sea, and many other marine ecosystems worldwide (7).
Among phyllobothriidian cestodes, C. delphini and C. grimaldii were identified in the blubber of a long-finned pilot whale. These tapeworm species are known to occur in this and other cetacean species in marine habitats surrounding Spain (20), frequently infecting the same host individual simultaneously (21), as also observed here. Both cestode species have a relatively broad host range and may reach high (exceeding 70%) prevalence among dolphins and whales in the Mediterranean and North Atlantic regions (22). In the life cycle of C. delphini, cetaceans are the second intermediate host, and sharks play the final host role (23).
This is the first report on the molecular analysis of the ITS2 sequence of C. delphini and C. grimaldii and of two parts of the cox1 gene in the case of the former species. As shown here based on sequences of two genetic markers (Figure 2; Supplementary Figure S1), C. delphini and C. grimaldii are phylogenetically closely related to the member(s) of this genus from pinnipeds (24) and form a monophyletic clade with other Clistobothrium species, as already reported based on shorter 28S rRNA sequences (21), further justifying their taxonomic placement in this genus instead of Phyllobothrium (25). Together with other members of the family Phyllobothriidae, C. delphini belonged to a sister group of the clade that includes Proteocephalidae, as previously reported based on other nuclear genetic markers (26).
Flukes (Digenea: Brachycladiidae) collected from the peritoneum and liver of a short-beaked common dolphin were molecularly identified as B. atlanticum, whereas those removed from the pylorus, gallbladder, and hepatic ducts of a striped dolphin were phylogenetically shown to belong to a different, probably new species (Brachycladium sp. Cádiz). The genus Brachycladium currently comprises nine species, of which five occur in the Pacific Ocean and five in the Atlantic Ocean (B. goliath in both oceans); but only B. atlanticum has been reported so far in the Mediterranean Sea (27). So far, only two Brachycladium species have been reported in the European region: B. atlanticum in both D. delphis and S. coeruleoalba (28, 29) and B. goliath in minke whale (Balaenoptera acutorostrata) in the Northeast Atlantic (30). Therefore, the findings of the present study indicate one more species indigenous to the Mediterranean Sea (probably also in marine environments surrounding Europe).
The life cycle of Brachycladium spp. is not completely known (30), but most likely fish act as second intermediate hosts, similar to some other members of the Brachycladiidae associated with pinnipeds (31). Although in other, geographically distant marine ecosystems, unidentified Brachycladium species were reported (29), to the best of our knowledge, this is the first finding of a third species of this genus in the Mediterranean region. This potentially new species is genetically most closely related to B. goliath, in contrast to B. nipponicum, which is closer related to B. atlanticum (29). Regarding the clinicopathological significance of these species and others with similar tissue tropism, trematodes of dolphins associated with the liver are regarded as potential causes of stranding (32).
Among the lungworms (Nematoda: Pseudaliidae) that were found in four cetacean species in this study, Halocercus species were only identified in two species of dolphins (Delphinidae: Delphininae). These mammals play a final host role in the life cycle and probably acquire Halocercus infection from fish intermediate hosts (33). However, depending on the species, prenatal infection might also be possible (34).
Regarding the host range of these lungworms, among the Western Mediterranean dolphins analyzed here, H. delphini was present in S. coeruleoalba and a potentially new species (Halocercus sp. Cádiz) in T. truncatus. Considering other studies involving the latter dolphin species in Europe, either H. delphini (20) or no Halocercus sp. were found (22). Since the lungworm species reported here in T. truncatus was most closely related to H. pingi, it is a species different from the one discovered recently in orca (Orcinus orca) in Northern Europe (13).
In a geographical context, three Halocercus species were reported to occur along the Spanish Atlantic coastline: H. delphini in D. delphis and T. truncatus, and H. invaginatus in P. phocoena, as well as an unidentified Halocercus sp. in S. coeruleoalba (20). More recently, H. delphini was shown to have a broader host range along the Mediterranean coastline of Spain, including not only D. delphis and T. truncatus but also five Stenella spp., all from Delphininae (35).
Accepting that the genus Halocercus consists of 12 species (36), because Skrjabinalius cryptocephalus deserves its own genus and Skrjabinalius guevarai is a synonym of H. delphini (37), so far there have been five species reported in Europe (38, 39). Since H. pingi occurs in the Pacific Ocean (40), this is the first report of a Halocercus sp. genetically most closely related to this species in the Western Palearctic.
In the present study, from the pterygoid sinus of G. melas (Odontoceti: Delphinidae: Globicephalinae), Stenurus globicephalae was identified. This parasite–host association was already reported along the Spanish Atlantic coast (20), and later this pseudaliid worm turned out to have a much broader host range in the Western Mediterranean, including six species of five genera from the subfamily Globicephalinae (35). In addition, in a P. phocoena from this study, a Pharurus species (tentatively called Pharurus sp. Cádiz) was demonstrated, which was molecularly and phylogenetically most closely related to Phanurus sunameri [OQ200467: (40)]. Considering that hitherto nematodes of this genus were not known to infect harbor porpoises (41), this is the first report of a lungworm species from this cetacean that, based on two genetic markers, aligns with Phanurus species and belongs to their phylogenetic group.
Taken together, the phylogenetic relationships of the four lungworm species found in this study confirm (A) previously reported relative host-specificity of pseudaliid worms, particularly among Delphinidae (35), and (B) that Halocercus vs. Stenurus and Pharurus species belong to two sister groups (40). In addition, (C) clustering of these genera also attests that the family Pseudaliidae is not monophyletic unless including Parafilaroides and Perostrongylus, as proposed earlier (40). Importantly, pseudaliid lungworms of cetaceans (especially if they occur in cranial sinuses) are thought to play a role in the stranding of infected animals (42).
Conclusion
In this study, first-time molecular evidence was obtained on the organ-specific location of T. gondii in D. delphis. Molecular-phylogenetic analyses of this study revealed an unexpected diversity of helminth parasites in cetaceans of the Western Mediterranean, including hitherto not-yet-analyzed genetic markers of long-known species (as exemplified by the cestode C. delphini) and possibly even undescribed (new) species (among flukes and lungworms in the genera Brachycladium and Halocercus, respectively). These results will substantially contribute to understanding host–parasite interactions and eco-epidemiological risks that might threaten cetacean populations in the Mediterranean Sea or Northeast Atlantic region.
Data availability statement
The sequences obtained during this study are deposited in GenBank under the accession numbers listed in Table 1. All other relevant data are included in the manuscript and the references or are available upon request by the corresponding author.
Ethics statement
This study was conducted according to relevant national and international guidelines. Ethical approval was not considered necessary because the animals were not handled alive. Samples were taken with the NGO Seashore Ambiental, on animals found stranded on the beach along the coasts of Andalusia, Spain. Usage of the samples for scientific purposes and publication of the results were granted by an agreement between Seashore Ambiental, Spain, and the University of Veterinary Medicine, Hungary (Registration number PART/9635-1/2023). The transportation of the samples (from Spain to Hungary) was carried out by the NGO Seashore Ambiental. Importation and research were approved the Pest County Government Office, Hungary (certificate No.: PE-06/KTF/33276-8/2023). CITES permit was not required because transportation took place within the EU (thus avoiding articles Nos. 4 and 5). Complying with article No. 8, the samples were only used for pre-defined research purposes in the context of preserving and protecting all involved species.
Author contributions
NS: Writing – review & editing, Writing – original draft, Resources, Project administration, Methodology, Investigation, Funding acquisition, Data curation, Conceptualization. GK: Project administration, Writing – review & editing, Writing – original draft, Supervision, Methodology, Investigation, Funding acquisition, Formal analysis, Data curation, Conceptualization. CF-M: Writing – review & editing, Writing – original draft, Validation, Project administration, Methodology, Investigation, Data curation. AC: Validation, Project administration, Writing – review & editing, Writing – original draft, Supervision, Methodology, Investigation. NT: Investigation, Data curation, Writing – review & editing, Writing – original draft, Methodology. JK: Methodology, Writing – review & editing, Writing – original draft, Visualization, Software. SH: Writing – original draft.
Funding
The author(s) declare financial support was received for the research, authorship, and/or publication of this article. Molecular work was funded by the Office for Supported Research Groups, Hungarian Research Network (HUN-REN), Hungary (project no. 1500107).
Acknowledgments
The authors would like to thank the “Consejería de Sostenibilidad, Medio Ambiente y Economía Azul” and the “Agencia de Medio Ambiente y Agua” of the “Junta de Andalucía” for allowing the use of their facilities and for granting authorization to use the samples in this study.
Conflict of interest
The authors declare that the research was conducted in the absence of any commercial or financial relationships that could be construed as a potential conflict of interest.
Publisher’s note
All claims expressed in this article are solely those of the authors and do not necessarily represent those of their affiliated organizations, or those of the publisher, the editors and the reviewers. Any product that may be evaluated in this article, or claim that may be made by its manufacturer, is not guaranteed or endorsed by the publisher.
Supplementary material
The Supplementary material for this article can be found online at: https://www.frontiersin.org/articles/10.3389/fvets.2024.1431625/full#supplementary-material
Abbreviations
cox1, Cytochrome c oxidase subunit I; ITS, Internal transcribed spacer; SSU, Small subunit.
Footnotes
References
1. Fordyce, RE. Cetacea (whales, porpoises and dolphins) In: Encyclopedia of life sciences. 1st ed. ed. R. Ewan Fordyce. Chichester: John Wiley & Sons, Ltd, (2001).
2. Balik, SE, Ossiboff, RJ, Stacy, NI, Wellehan, JF, Huguet, EE, and Gallastegui, A. Case report: Sarcocystis speeri, aspergillus fumigatus, and novel Treponema sp. infections in an adult Atlantic spotted dolphin (Stenella frontalis). Front Vet Sci. (2023) 10:1132161. doi: 10.3389/fvets.2023.1132161
3. Guégan, JF. Chlimate change and infectious diseases in the Mediterranean region In: The Mediterranean region under climate change: A scientific update [internet]. eds. Marie-LiseSabrié, E Gibert-Brunet, and T Mourier Mareille: IRD Éditions, (2016).
4. Dupke, S, Buchholz, U, Fastner, J, Förster, C, Frank, C, Lewin, A, et al. Impact of climate change on waterborne infections and intoxications. J Health Monit. (2023) 8:62. doi: 10.25646/11402
5. Cuvertoret-Sanz, M, López-Figueroa, C, Byrne, AO, Canturri, A, Martí-Garcia, B, Pintado, E, et al. Causes of cetacean stranding and death on the Catalonian coast (western Mediterranean Sea), 2012-2019. Dis Aquat Org. (2020) 142:239–53. doi: 10.3354/dao03550
6. Dubey, JP, Zarnke, R, Thomas, NJ, Wong, SK, Van Bonn, W, Briggs, M, et al. Toxoplasma gondii, Neospora caninum, Sarcocystis neurona, and Sarcocystis canis-like infections in marine mammals. Vet Parasitol. (2003) 116:275–96. doi: 10.1016/S0304-4017(03)00263-2
7. Di Guardo, G, and Mazzariol, S. Toxoplasma gondii: clues from stranded dolphins. Vet Pathol. (2013) 50:737. doi: 10.1177/0300985813486816
8. Gardner, BR, Bachmann, NL, Polkinghorne, A, Hufschmid, J, Tadepalli, M, Marenda, M, et al. A novel marine mammal Coxiella burnetii—genome sequencing identifies a new genotype with potential virulence. Pathogens. (2023) 12:893. doi: 10.3390/pathogens12070893
9. Marangi, M, Carlucci, R, Carlino, P, Fanizza, C, Cirelli, G, and Maglietta, R. Dolphins and sea turtles may host zoonotic parasites and pathogenic bacteria as indicators of anthropic pressure in the Gulf of Taranto (northern Ionian Sea, central-eastern Mediterranean Sea). Vet Res Commun. (2022) 46:1157–66. doi: 10.1007/s11259-022-10011-y
10. Reeves, RR, and Notarbartolo di Sciara, G. The status and distribution of cetaceans in the Black Sea and Mediterranean Sea. (2006) [cited 2023. december 18.]; Available at: https://policycommons.net/artifacts/1376036/the-status-and-distribution-of-cetaceans-in-the-black-sea-and-mediterranean-sea/1990298/
11. Ahmadpour, E, Rahimi, MT, Ghojoghi, A, Rezaei, F, Hatam-Nahavandi, K, Oliveira, SMR, et al. Toxoplasma gondii infection in marine animal species, as a potential source of food contamination: a systematic review and Meta-analysis. Acta Parasitol. (2022) 67:592–605. doi: 10.1007/s11686-021-00507-z
12. Mohd Hussain, RH, Abdul Ghani, MK, Khan, NA, Siddiqui, R, and Anuar, TS. Acanthamoeba species isolated from marine water in Malaysia exhibit distinct genotypes and variable physiological properties. J Water Health. (2022) 20:54–67. doi: 10.2166/wh.2021.128
13. Lehnert, K, Boyi, JO, and Siebert, U. Potential new species of pseudaliid lung nematode (Metastrongyloidea) from two stranded neonatal orcas (Orcinus orca) characterized by ITS -2 and COI sequences. Ecol Evol. (2023) 13:e10036. doi: 10.1002/ece3.10036
14. Schuster, FL, and Visvesvara, GS. Free-living amoebae as opportunistic and non-opportunistic pathogens of humans and animals. Int J Parasitol. (2004) 34:1001–27. doi: 10.1016/j.ijpara.2004.06.004
15. Cabezón, O, Resendes, AR, Domingo, M, Raga, JA, Agustí, C, Alegre, F, et al. Seroprevalence of toxoplasma gondii antibodies in wild dolphins from the Spanish Mediterranean coast. J Parasitol. (2004) 90:643–4. doi: 10.1645/GE-257R
16. Forman, D, West, N, Francis, J, and Guy, E. The sero-prevalence of toxoplasma gondii in British marine mammals. Mem Inst Oswaldo Cruz. (2009) 104:296–8. doi: 10.1590/S0074-02762009000200024
17. Fernández-Escobar, M, Giorda, F, Mattioda, V, Audino, T, Di Nocera, F, Lucifora, G, et al. Toxoplasma gondii genetic diversity in Mediterranean dolphins. Pathogens. (2022) 11:909. doi: 10.3390/pathogens11080909
18. Landrau-Giovannetti, N, Waltzek, TB, López-Orozco, N, Su, C, Rotstein, D, Levine, G, et al. Prevalence and genotype of toxoplasma gondii in stranded Hawaiian cetaceans. Dis Aquat Org. (2022) 152:27–36. doi: 10.3354/dao03699
19. Kern, A, Seidel, K, and Oelschläger, HHA. The central vestibular complex in dolphins and humans: functional implications of Deiters’ nucleus. Brain Behav Evol. (2009) 73:102–10. doi: 10.1159/000213646
20. Abollo, E, Lopez, A, Gestal, C, Benavente, P, and Pascual, S. Macroparasites in cetaceans stranded on the northwestern Spanish Atlantic coast. Dis Aquat Org. (1998) 32:227–31. doi: 10.3354/dao032227
21. Agusti, C, Aznar, FJ, Olson, PD, Littlewood, DTJ, Kostadinova, A, and Raga, JA. Morphological and molecular characterization of tetraphyllidean merocercoids (Platyhelminthes: Cestoda) of striped dolphins (Stenella coeruleoalba) from the Western Mediterranean. Parasitology. (2005) 130:461–74. doi: 10.1017/S0031182004006754
22. Terracciano, G, Fichi, G, Comentale, A, Ricci, E, Mancusi, C, and Perrucci, S. Dolphins stranded along the tuscan coastline (Central Italy) of the “pelagos sanctuary”: a parasitological investigation. Pathogens. (2020) 9:612. doi: 10.3390/pathogens9080612
23. Aznar, FJ, Agustí, C, Littlewood, DTJ, Raga, JA, and Olson, PD. Insight into the role of cetaceans in the life cycle of the tetraphyllideans (Platyhelminthes: Cestoda). Int J Parasitol. (2007) 37:243–55. doi: 10.1016/j.ijpara.2006.10.010
24. Klotz, D, Hirzmann, J, Bauer, C, Schöne, J, Iseringhausen, M, Wohlsein, P, et al. Subcutaneous merocercoids of Clistobothrium sp. in two cape fur seals (Arctocephalus pusillus pusillus). Int J Parasitol Parasites Wild. (2018) 7:99–105. doi: 10.1016/j.ijppaw.2018.02.003
25. Caira, JN, Jensen, K, Pickering, M, Ruhnke, TR, and Gallagher, KA. Intrigue surrounding the life-cycles of species of Clistobothrium (Cestoda: Phyllobothriidea) parasitising large pelagic sharks. Int J Parasitol. (2020) 50:1043–55. doi: 10.1016/j.ijpara.2020.08.002
26. Caira, JN, Jensen, K, Waeschenbach, A, Olson, PD, and Littlewood, DTJ. Orders out of chaos–molecular phylogenetics reveals the complexity of shark and stingray tapeworm relationships. Int J Parasitol. (2014) 44:55–73. doi: 10.1016/j.ijpara.2013.10.004
27. Fraija-Fernández, N, Aznar, F, Raga, J, Gibson, D, and Fernández, M. A new brachycladiid species (Digenea) from Gervais’ beaked whale Mesoplodon europaeus in North-Western Atlantic waters. Acta Parasitol [Internet]. (2014) 59:510–7. doi: 10.2478/s11686-014-0274-7
28. Mateu, P, Raga, JA, and Aznar, FJ. Host specificity of Oschmarinella rochebruni and Brachycladium atlanticum (Digenea: Brachycladiidae) in five cetacean species from western Mediterranean waters. J Helminthol. (2011) 85:12–9. doi: 10.1017/S0022149X10000180
29. Kim, S, Youn, H, Lee, K, Lee, H, Kim, MJ, Kang, Y, et al. Novel morphological and molecular data for Nasitrema spp.(Digenea: Brachycladiidae) in the east Asian finless porpoise (Neophocaena asiaeorientalis sunameri). Front Mar Sci. (2023) 10:1187451. doi: 10.3389/fmars.2023.1187451
30. Briscoe, AG, Bray, RA, Brabec, J, and Littlewood, DTJ. The mitochondrial genome and ribosomal operon of Brachycladium goliath (Digenea: Brachycladiidae) recovered from a stranded minke whale. Parasitol Int. (2016) 65:271–5. doi: 10.1016/j.parint.2016.02.004
31. Kremnev, G, Gonchar, A, Krapivin, V, Knyazeva, O, and Krupenko, D. First elucidation of the life cycle in the family Brachycladiidae (Digenea), parasites of marine mammals. Int J Parasitol. (2020) 50:997–1009. doi: 10.1016/j.ijpara.2020.05.011
32. Ridgway, SH, and Dailey, MD. Cerebral and cerebellar involvement of trematode parasites in dolphins and their possible role in stranding. J Wildl Dis. (1972) 8:33–43. doi: 10.7589/0090-3558-8.1.33
33. Lehnert, K, von Samson-Himmelstjerna, G, Schaudien, D, Bleidorn, C, Wohlsein, P, and Siebert, U. Transmission of lungworms of harbour porpoises and harbour seals: molecular tools determine potential vertebrate intermediate hosts. Int J Parasitol. (2010) 40:845–53. doi: 10.1016/j.ijpara.2009.12.008
34. Dailey, M, Walsh, M, Odell, D, and Campbell, T. Evidence of prenatal infection in the bottlenose dolphin (Tursiops truncates) with the lungworm Halocercus lagenorhynchi (Nematoda: Pseudaliidae). J Wildl Dis. (1991) 27:164–5. doi: 10.7589/0090-3558-27.1.164
35. Pool, R, Romero-Rubira, C, Raga, JA, Fernández, M, and Aznar, FJ. Determinants of lungworm specificity in five cetacean species in the western Mediterranean. Parasit Vectors. (2021) 14:április 12:196. doi: 10.1186/s13071-021-04629-1
36. de Oliveira Carvalho Demarque, I, FCR, d O, da Silveira, LS, Barbosa, LA, and Ederli, NB. The lungworm, Halocercus brasiliensis (Nematoda: Pseudaliidae), from Guiana dolphins Sotalia guianensis from Brazil with pathological findings. J Parasitol. (2020) 106:254–60. doi: 10.1645/19-77
37. Pool, R, Fernández, M, Chandradeva, N, Raga, JA, and Aznar, FJ. The taxonomic status of Skrjabinalius guevarai Gallego & Selva, 1979 (Nematoda: Pseudaliidae) and the synonymy of Skrjabinalius Delyamure, 1942 and Halocercus Baylis & Daubney, 1925. Syst Parasitol. (2020) 97:389–401. doi: 10.1007/s11230-020-09921-9
38. Gibson, DI, Harris, EA, Bray, RA, Jepson, PD, Kuiken, T, Baker, JR, et al. A survey of the helminth parasites of cetaceans stranded on the coast of England and Wales during the period 1990-1994. J Zool. (1998) 244:563–74. doi: 10.1111/j.1469-7998.1998.tb00061.x
39. Costello, M, Emblow, C, and White, R. European register of marine species: A check-list of the marine species in Europe and a bibliography of guides to their identification In: Collection Patrimoines Naturels. eds. MJ Costello, C Emblow, and R White Paris: Muséum National d’Histoire Naturelle, Institute d’écologie et de gestion de la biodiversité service du patrimoine naturel. (2001). 463.
40. Pool, R, Shiozaki, A, Raga, JA, Fernández, M, and Aznar, FJ. Molecular phylogeny of the Pseudaliidae (Nematoda) and the origin of associations between lungworms and marine mammals. Int J Parasitol Parasites Wildl. (2023) 20:192–202. doi: 10.1016/j.ijppaw.2023.03.002
41. Dzido, J, Rolbiecki, L, Izdebska, JN, Rokicki, J, Kuczkowski, T, and Pawliczka, I. A global checklist of the parasites of the harbor porpoise Phocoena phocoena, a critically-endangered species, including new findings from the Baltic Sea. Int J Parasitol Parasites Wildl. (2021) 15:290–302. doi: 10.1016/j.ijppaw.2021.07.002
Keywords: Brachycladiidae, Cestoda, Nematoda, Phyllobothriidae, Pseudaliidae, Toxoplasma gondii , Trematoda, cetacea
Citation: Specht NR, Keve G, Fernández-Maldonado C, Cerezo Caro A, Takács N, Kontschán J and Hornok S (2024) Molecular investigation of endoparasites of marine mammals (Cetacea: Mysticeti, Odontoceti) in the Western Mediterranean. Front. Vet. Sci. 11:1431625. doi: 10.3389/fvets.2024.1431625
Edited by:
Vikrant Sudan, Guru Angad Dev Veterinary and Animal Sciences University, IndiaReviewed by:
Ranju Manoj, Cornell University, United StatesMarialetizia Palomba, University of Tuscia, Italy
Copyright © 2024 Specht, Keve, Fernández-Maldonado, Cerezo Caro, Takács, Kontschán and Hornok. This is an open-access article distributed under the terms of the Creative Commons Attribution License (CC BY). The use, distribution or reproduction in other forums is permitted, provided the original author(s) and the copyright owner(s) are credited and that the original publication in this journal is cited, in accordance with accepted academic practice. No use, distribution or reproduction is permitted which does not comply with these terms.
*Correspondence: Gergő Keve, a2V2ZS5nZXJnb0B1bml2ZXQuaHU=
†These authors have contributed equally to this work and share first authorship