- 1College of Veterinary Medicine, Yunnan Agricultural University, Kunming, China
- 2Yunnan Animal Science and Veterinary Institute, Kunming, Yunnan, China
- 3Laboratory of Sustainable Agriculture Management, Higher School of Technology Sidi Bennour, Chouaib Doukkali University, El Jadida, Morocco
- 4Higher School of Education and Training, Mohammed I University, Oujda, Morocco
- 5Yunnan Provincial Engineering Research Center of Animal Genetic Resource Conservation and Germplasm Enhancement, Kunming, Yunnan, China
- 6Yunnan Provincial Genebank of Livestock and Poultry Genetic Resources, Kunming, Yunnan, China
Sperm cryopreservation is an approach to preserve sperm cells in liquid nitrogen or other cryogenic media for future use in assisted reproductive technologies, such as in vitro fertilization or artificial insemination. Sperm cryopreservation has been extensively used in the dairy industry and has attained excellent results after artificial insemination. However, for small ruminants the application of sperm cryopreservation is limited, due to the poor quality of frozen semen and special characteristics of the reproductive female tract. In order to improve post-thaw semen quality various cryoprotectants are used. Currently, many types of cryoprotectants, such as permeable organic solvents, sugars, antioxidants, and natural or synthetic ice blockers, have been tested on small ruminants’ sperm cryopreservation. Among them, trehalose; has shown potential acting as an excellent cryoprotectant for semen freezing. While, the exact roles and action mechanisms of trehalose during cryopreservation remain unclear. In this review, we systematically summarized the present usage status, potential action mechanisms, and future application prospects of trehalose in small-ruminant sperm cryopreservation.
1 Introduction
Small ruminants, primarily sheep and goats, significantly contribute to the modern livestock industry and can provide meat, wool, skin, or milk to our society. However, with the fast development of modern intensified agriculture, the genetic diversity of farm animal species is rapidly being reduced in many regions worldwide (1). Semen cryopreservation has been successfully applied to various mammalian species, including humans, domestic animals, and endangered wildlife species. Semen cryopreservation offers multiple advantages to the small ruminant livestock industry through the worldwide distribution of excellent genetic materials through artificial insemination (AI) (2, 3). It is important to take into account that the freezing and thawing process can have a harmful impact on sperm (4, 5). According to current reports, cryopreservation can lead to ice formation, cold shock, chemical effects caused by cryoprotectants, osmotic injury, oxidative injury, and apoptosis (6–8). These factors ultimately damage the structure and physiological function of sperm. Furthermore, these stresses primarily damage the plasma membrane of sperm, resulting in a significant decrease in the viability and fertility of post-thaw sperm (9, 10). In addition, the cryopreservation process causes sublethal cryoinjuries that result in approximately 50% of post-thawed sperm thus losing their viability during cervical artificial insemination with frozen–thawed semen (11).
In sheep, it has been reported that after thawing, 40 to 60% of sperm are still motile, but only 20 to 30% are biologically functional (12, 13). The use of frozen/thawed semen in conventional insemination yields poor fertility in sheep. This may be due to different factors but one of the most important restrictive reasons is considered to be the anatomical structure of the cervix (14–19). Currently, frozen–thawed semen generally attained a better results by intrauterine insemination (19–23). However, this technique needs specific equipment and well-trained technicians. Therefore, to elucidate cryoinjury mechanisms and improve the quality of frozen–thawed ram sperm still remains a great challenge until now.
The success of mammalian sperm cryopreservation is influenced by the species and individual factors (24). In addition, research and advancements in semen cryopreservation techniques continue to focus on enhancing the efficiency and success rates of sperm freezing including the optimization of the cryoprotectant solutions used, development of better freezing and thawing protocols, and application of new approaches to enhance sperm post-thaw survival and functionality (3, 25–28). Among these measures, selecting cryoprotectants is important. Cryoprotectants are substances that help protect sperm cells from damage during the freezing and thawing process (29, 30). Glycerol has been shown to have excellent cryoprotective effects on livestock semen and is now an essential component in the semen freezing extenders (31–33). Furthermore, other cryoprotectants, such as sugars (34–36), antioxidants (37–39), antifreeze proteins (40–42) and synthetic ice blockers (43, 44) have been used for semen cryopreservation. Moreover, among the sugars used in semen cryopreservation is tehalose. Previous investigations have proven that trehalose has beneficial effects on sperm during cryopreservation (45–49). Trehalose has been used for mammalian sperm cryopreservation, and previous studies have shown that it can increase sperm’s tolerance to cryoinjuries (47, 50, 51). Moreover, trehalose may have better cryoprotective effects on small ruminant sperm than other oligosaccharides, such as sucrose (48, 52, 53). However, there are still some disagreements (54, 55). More precisely, the ability of trehalose to preserve the motility of frozen sheep sperm is similar to that of sucrose (54). In another study, the effects of trehalose on frozen goat sperm are not superior to those of other oligosaccharides, including sucrose, maltose, and lactose (35). Also, it should be pointed out that trehalose, unlike glucose and fructose, is unable to pass through the plasma membrane. So, the primary function of trehalose is to protect against extracellular cryodamage (56).
The mechanisms that contribute to the cryoprotective effects of trehalose on mammalian sperm are still unclear, despite the existence of several hypotheses. The purpose of this review is to summarize the current research on trehalose for cryopreservation of small ruminant semen, which includes its current usage status, potential action mechanisms, and future application prospects.
2 The characteristics and potential application of trehalose
Trehalose is a typical non-reducing disaccharide composed of two glucose molecules linked together. It is naturally produced in various organisms, such as plants, fungi, bacteria, and invertebrates (57). Trehalose is known for its unique properties, which make it useful in a variety of applications. Specifically, it can act as a protective agent against stressors, such as desiccation, extreme temperatures, and oxidative damage (58). Recently, trehalose has received a lot of attention in the food and pharmaceutical industries due to its protective properties (59–61). One of the most notable characteristics of trehalose is its capacity to form a protective barrier around cells and biomolecules (57, 62, 63). Trehalose acts as a stabilizer, helping to preserve the structure and function of proteins, enzymes, and other biological molecules (61, 64). It is employed in the pharmaceutical and biotechnology industries to stabilize and maintain the active ingredients in medications, vaccines, and diagnostic kits (65). Furthermore, in the food industry, trehalose is used as a sweetener, flavor enhancer, and stabilizer for various products. Trehalose is a common ingredient in processed foods, baked goods, and beverages (66). It has the potential to enhance the texture, taste, and shelf life of these products. In addition, trehalose has been examined for its potential health benefits. According to some research, trehalose has been reported to have antioxidant and anti-inflammatory properties (67, 68), and it has the potential to be utilized in the prevention or treatment of certain diseases.
Trehalose also has the ability to act as a cryoprotectant, and is able to prevent ice crystal formation as well as maintain cell and tissue structural integrity during cryopreservation processes (47, 69–71). In the context of sperm cryopreservation, trehalose has been investigated as a potential cryoprotectant in many studies (72–75). It has been demonstrated that trehalose protects semen quality parameters from cryodamage (76–78).
Generally, the particularly cryoprotective properties of trehalose make it an intriguing substance for various applications, including sperm cryopreservation. However, it’s worth noting that other cryoprotectants are also commonly used in combination with trehalose or as alternatives, and the choice of cryoprotectants depends on the specific requirements of the cryopreservation protocol. The most effective and standardized protocols for sperm cryopreservation using trehalose as an important cryoprotectant are still being researched and optimized.
3 The application of trehalose in semen cryopreservation of small ruminant
The effects of trehalose on sheep (31, 49, 56, 79, 80) and goat sperm (81–84) cryopreservation have been evaluated. Most researchers support the positive effects of trehalose on sperm during the cryopreservation process. For example, the best results were obtained in sheep semen using tris, citric acid, fructose, egg yolk, glycerol supplemented with 50 or 100 mOsm of trehalose, while the post-thaw sperm quality significantly decreased with 200 and 400 mOsm of trehalose (76). Trehalose confers a greater cryoprotective capacity to the base extender when added up to 100 mOsm. Other studies have also reached similar conclusions (45, 85). In addition, sperm plasma membrane evaluation by ultramicroscopy indicated better cryoprotective effects on sperm frozen in an extender containing trehalose, there was a significant reduction in the number of damaged membranes (45). In an earlier study by our team, it was found that trehalose had superior cryoprotective effects on sheep sperm’s ultrastructure, compared to other cryoprotectants, such as natural or synthetic ice blockers (70).
Cryopreserving sheep semen in an extender with 100 mM trehalose resulted in a decrease in oxidative stress caused by the freezing and thawing process due to the antioxidant proprieties of trehalose (86). In goats, a tris-based extender supplemented with trehalose at 100 mM can improve post-thaw semen characteristics (87). According to an additional study, adding 150 mM trehalose to the tris-citric acid-egg yolk-fructose diluent resulted in higher efficiency for goat sperm cryopreservation due to its improved motility, viability, plasma membrane integrity, and acrosome integrity (81). Moreover, there are still some disagreements regarding trehalose’s cryoprotective properties for sperm (3, 56). Trehalose and sucrose have comparable abilities to maintain the motility of frozen sheep sperm (54). According to a different study, trehalose does not enhance the quality of frozen goat spermatozoa (35). In addition, trehalose was found to have detrimental effects on the post-thaw kinetic sperm parameters when dimethylacetamide was present (88). Besides, the post-thaw motility and morphology were not improved when the trehalose content in the freezing extender was 50 mM or 100 mM (89).
In some studies, trehalose and other cryoprotectants were used together to enhance the cryoprotective effects of trehalose on frozen sperm. The addition of a combination of oleic acid and trehalose concentrations to a Tris-based extender can improve the post-thaw quality of ram semen (74). Moreover, the combined addition of fetuin and trehalose to the tris-based extenders can lower the overall glycerol usage concentration to 3%, reducing the harmful effects of glycerol and improving the quality of cryopreserved ram sperm (77). Likewise, the post-thaw of ram sperm was improved with 60 mM trehalose (79). Besides, it was observed that there was a positive synergic impact of iodixanol and trehalose on cryosurvival of semen (90). When the combination of antipain (10 μM) and trehalose (30 and 60 mM) was included, they conferred an extraordinary cryosurvival capacity (91). In a previous study, in a soybean lecithin-based extender, a combination of 100 mM trehalose and 5% glycerol was the best combination to realize a better post-thaw quality of ram sperm (80). In goats, supplementation of 200 nM MitoQ alone or along with 150 mM trehalose to semen extender can improve the quality of cryopreserved sperm, which may be related to improved antioxidant enzymatic defense and mitochondrial activity and reduced DNA fragmentation (78). Another investigation suggests that adding 3 mM and 6 mM pentoxifylline or 50 mM and 70 mM trehalose reduces the damage caused by cooling and cryopreservation processes (83). Furthermore, freezing goat sperm in a trehalose-egg yolk extender with a sufficient concentration of sodium dodecyl sulfate greatly increased the sperm’s ability to freeze (92). We can summarize that the effect of trehalose depends on the concentration, extenders, breed, species and cryopreservation protocols. Table 1 summarizes the effects of trehalose, alone or combined with other substances, on semen cryopreservation in small ruminants.
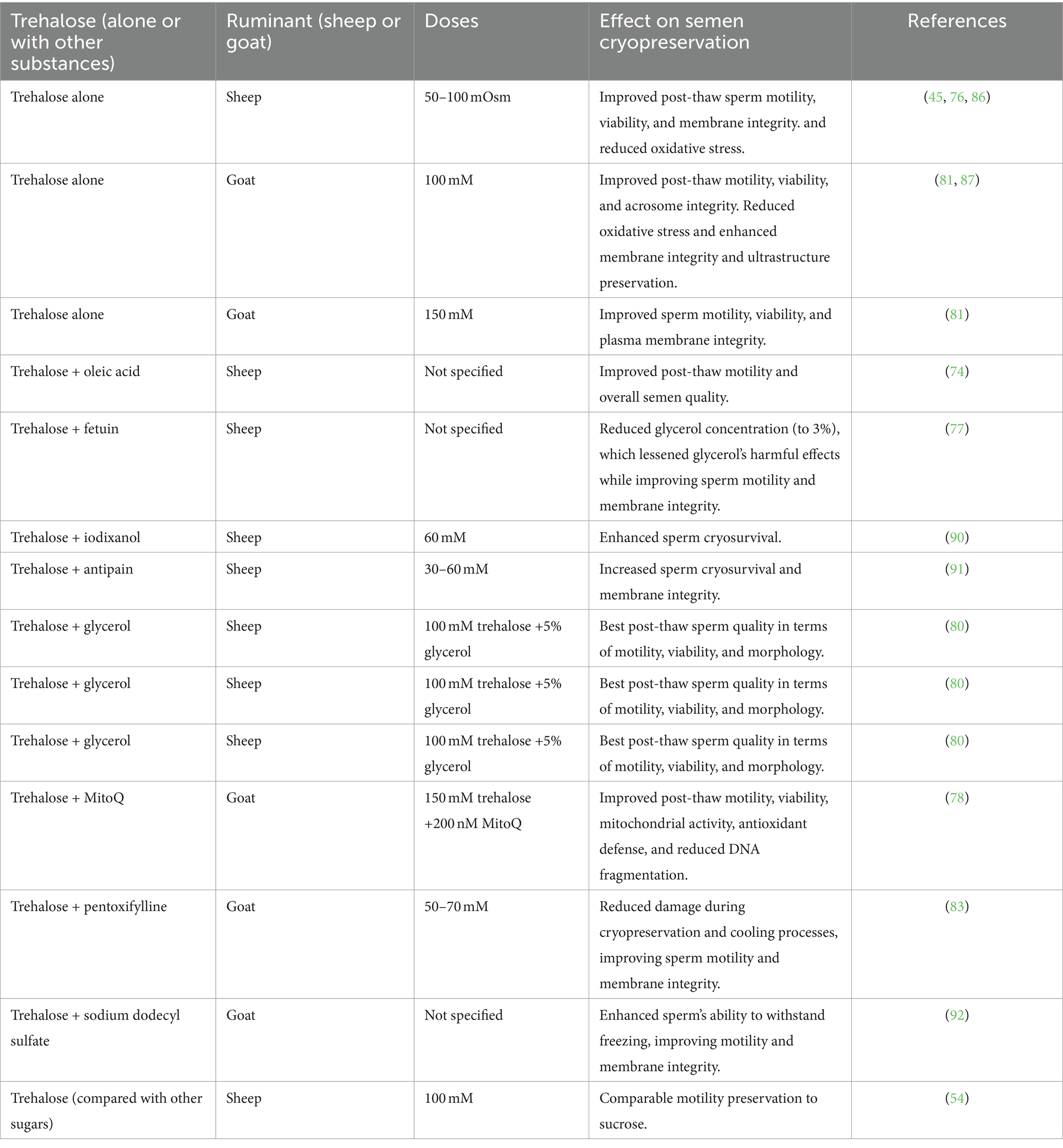
Table 1. Effects of trehalose (alone or in combination) on semen parameters in small ruminants during cryopreservation.
4 The role of trehalose as an antioxidant in semen cryopreservation of small ruminant
Trehalose, a non-reducing disaccharide, has gained prominence in cryobiology due to its multifaceted protective properties, particularly as an antioxidant during semen cryopreservation (93, 94). The cryopreservation process induces osmotic stress and causes the generation of excessive reactive oxygen species (ROS), leading to oxidative stress, which is a major cause of sperm damage (95).
Trehalose’s antioxidant effect stems from its ability to scavenge free radicals, thereby preventing ROS accumulation. It stabilizes cell membranes by forming a glass-like structure around phospholipid bilayers during freezing, which preserves the integrity of the sperm membrane against cold shock and osmotic stress (96). Studies have demonstrated that trehalose significantly reduces the levels of malondialdehyde a marker of lipid peroxidation, thereby maintaining the integrity of sperm membrane lipids. Additionally, trehalose prevents mitochondrial dysfunction, which is a key source of endogenous ROS during cryopreservation, ensuring higher post-thaw ATP levels and energy metabolism (78). Furthermore, trehalose has been shown to modulate the activity of antioxidant enzymes such as superoxide dismutase (SOD) and glutathione peroxidase (GPx) during cryopreservation (50). By maintaining these enzymatic defenses, trehalose reduces oxidative damage to sperm DNA and proteins, resulting in improved sperm chromatin integrity and lower levels of DNA fragmentation post-thaw (47). Furthermore, its antioxidant properties protect the sperm membrane against the attacks enacted by free radical to ROS (86). Recently it has been demonstrated that trehalose can protect sperm from oxidative stress by enhancing antioxidant capacity (83). Moreover, lower doses of trehalose reduce lipid peroxidation and protect the spermatozoa (84).
The inclusion of trehalose in cryopreservation extender has been widely reported to enhance antioxidant activity, decrees the oxidative stress and improve the sperm motility and membrane integrity during sperm storage (45, 97).
5 The proposed mechanisms of action of trehalose during cryopreservation of semen
Different from monosaccharides including fructose and glucose, trehalose, acting as a sugar, cannot permeate the plasma membrane. As such, its primary role is that of an extracellular cryoprotectant (98, 99). The report by Crowe et al. (100) suggested that trehalose needs to be present on both sides of the plasma membrane in order to have the best protective effects. To address this issue, several technologies have been developed, including microinjection of trehalose into cells, transfection to express trehalose in mammalian cells and thermotropic lipid phase transition (101–104). Research is still needed to determine whether these technologies could introduce trehalose into sperm cryopreservation. Furthermore, the exact mechanism for the effect of trehalose on semen cryopreservation remains unclear.
At present, numerous hypotheses were recommended along with the enhancement of extracellular vitrification formation, prohibiting intracellular ice formation, stabilization of liquid crystalline within the plasma membrane, linkage with phospholipids and reorganization of the plasma membrane, enhancement of membrane fluidity, antioxidant activity, reduced apoptosis, etc. (Figure 1). At first, an excessive glass transition temperature (Tg) is a crucial function of trehalose (105). The Tg of trehalose (−30°C) is a considerably higher than that of different conventional cryoprotectants, which include ethylene glycol (−8°C) and glycerol (−65° C) (106). Therefore, the presence of trehalose in the media may also make contributions to extracellular vitrification formation and decrease in ice crystal production. Secondly, trehalose can increase extracellular osmotic pressure, cause cell dehydration and decrease the formation of lethal intracellular ice (9). Thirdly, trehalose might also additionally update the water shell of macromolecules with the aid of hydrogen bonding and keep away from cryodamage resulting from the freezing and thawing process, in line with the “water replacement hypothesis (Figure 2) (57, 58). Crowe et al. (58) believed that owing to its ability to replace the water shell of macromolecules, trehalose may prevent injury during freezing or drying. As a replacement for the water molecule, trehalose can link membranes or other macromolecules by hydrogen bonding, which is thought to be a necessary condition for reducing the liquid crystalline to gel phase transition temperature (107, 108). Many researchers have mentioned the stabilization mechanism of trehalose in frozen or lyophilized organic cells (109, 110). As a substitute for the water molecule, trehalose can hyperlink membranes or different macromolecules through hydrogen bonding, which may be a notion to be an important circumstance for lowering the liquid crystalline to gel segment transition temperature (58, 111). In addition, the supplementation of trehalose can enhance plasma membrane fluidity of sperm (97). Moreover, trehalose can also link with plasma membrane phospholipids, reorganize plasma membrane, and make sperm survive through the freezing and thawing process (112, 113). It can be integrated into the plasma membrane and prohibit the excessive dehydration of sperm during the cryopreservation process, consequently reducing the physical damage caused by abnormal variations of cell volume (114). In addition, the cryoprotective roles of trehalose can also be related to its antioxidant activity. Finally, in ram, trehalose suppresses lysophosphatidylcholine-precipitated acrosome response in sperm, therefore enhancing cryosurvival of sperm (115).
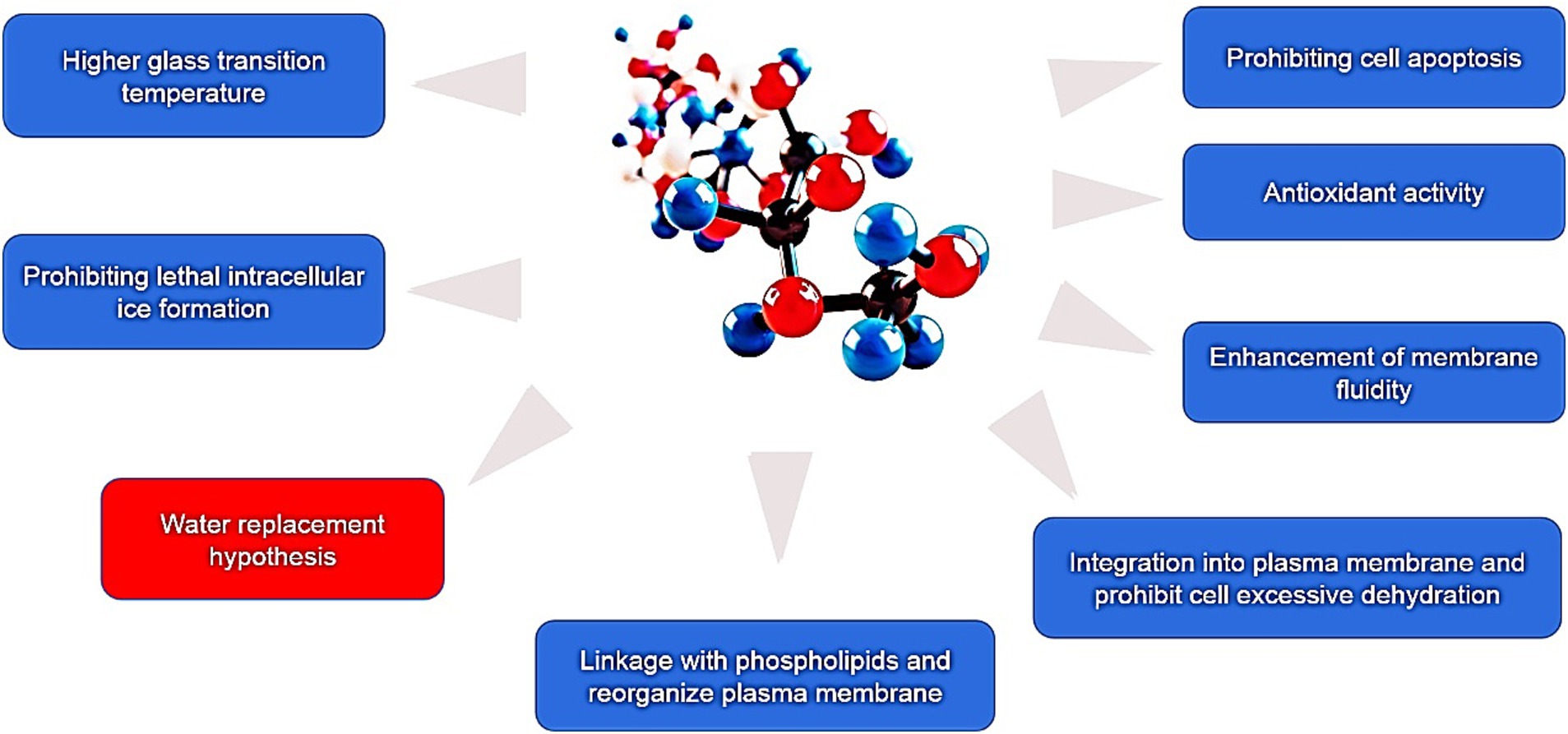
Figure 1. The present cryoprotective hypotheses of trehalose during cryopreservation of mammalian sperm.
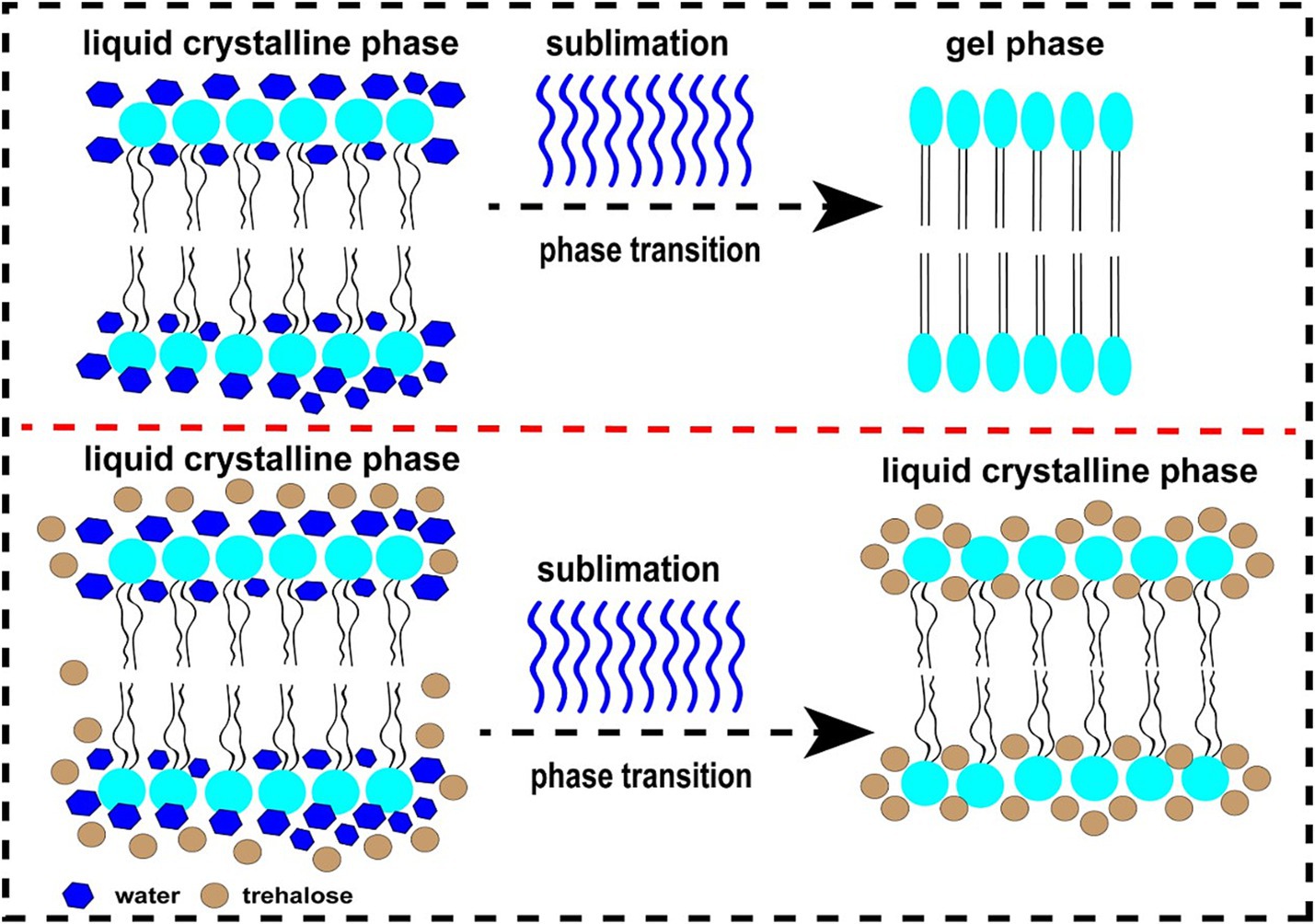
Figure 2. The diagram of water replacement hypothesis. The upper figure represents the changing in sperm plasma membrane from normal liquid crystalline phase to gel phase during freezing in the absence of trehalose. The below figure represents the maintenance of liquid crystalline phase in sperm plasma membrane after freezing in the presence of trehalose.
As of now, the omics innovations technologies have been utilized within the inquiry about small ruminants’ sperm. In understanding with the show reports, the cryopreservation process can modify the abundance of transcripts (116–122), proteins (6, 123–127), metabolites (128–130) and lipids (131). In a few scattered thoughts, about, analysts endeavored to investigate the cryoprotective components of trehalose on little ruminant sperm amid the cryopreservation handle from the viewpoint of transcripts, proteins, and metabolites. In a previous study, the group used electrophoresis technology to assess the relationship between the presence of protein in ram plasma and the quality of semen frozen with 5% glycerol or 100 mM trehalose (132). A total of 26 bands were identified in ram’s seminal plasma. In another study, a total of 1,269 proteins were identified using the isobaric tag for relative and absolute quantification (iTRAQ) strategy combined with parallel reaction monitoring (PRM) Among them, 21 differentially expressed proteins were identified. These proteins were involved in oxidoreductase activity, stress response, and catabolic processes, which may be associated with the cryoprotective effects of trehalose (56). Regarding changes in sperm metabolites after freezing in the presence of trehalose, a research group used GC–MS-based metabolomics to investigate the effects of trehalose on goat sperm (85). 48 different metabolites were found. L-isoleucine, L-leucine, L-threonine, and dihydroxyacetone are synthesized through this pathway, including valine, leucine, and isoleucine biosynthesis, glycerolipid metabolism, and aminoacylRNA biosynthesis (85). For this reason, they thought that trehalose played an important role in changing the amino acid and glycerol metabolism processes in sperm (35). Recently, the use of the RNA sequencing (RNA-Seq) approach to investigate the consequences of the cryopreservation procedure on mammalian sperm transcript profiles became a hot spot (133–135). To the best of our knowledge there are no published reports to elucidate the effects of trehalose on the post-thaw small ruminant sperm based on the changes of sperm-derived RNAs. According to our unpublished research, storage conditions significantly alter the transcription profiles of sheep sperm. However, no mRNA had different expression levels between the control group and the trehalose group. Therefore, we initially hypothesized that the inhibitory effect of trehalose might be unrelated to transcriptomic changes of sperm during storage. However, this result requires further investigation.
6 The future application prospects of trehalose in semen cryopreservation
Although there are still ongoing debates, most studies support the positive effect of trehalose on small ruminant spermatozoa during storage (45, 47, 79). It should be noted that the cryoprotective effect of trehalose may depend on certain factors such as the species, breed, and composition of the extenders used (3). Moreover, the mechanism underlying this protective function is not yet fully clear. According to current studies, the use of omics technologies, including genomics, transcriptomics, proteomics and metabolomics, may be the best solution to investigate the mechanism of trehalose utilization. In particular, changes in the structure of RNA molecules such as mRNA, lncRNA, circRNA and microRNA may be an important mechanism explaining the functional role of trehalose (133, 136–139). Semen quality may also be associated with sperm fertility (102, 140, 141) and may give us some clues about the role of trehalose. However, the limitations of this study are related to how we can obtain useful information from already published studies, since most of the current studies on spermatozoa research include basic bioinformatic analysis. Nevertheless, some results are completely speculative and do not stand up to scrutiny. However, according to the “water exchange hypothesis” theory, most of the major trehalose must enter the cell to be effective in the inhibition process (57, 58). Finally, the mechanism of trehalose loading in yeast is complex. The actual effect of trehalose on sperm quality remains to be confirmed.
7 Conclusion
Trehalose has demonstrated beneficial effects during cryopreservation of small ruminant sperm according to the most current investigations. However, some disputes about the effects of trehalose on sperm characteristics after the cryopreservation process still exist. Currently, some hypotheses, such as the water replacement hypothesis, enhancement of membrane fluidity, and prevention of ice formation, have been proposed to explain the possible functional roles of trehalose during semen cryopreservation. However, the real cryoprotective mechanism of trehalose still needs to be determined.
At present, the rapid development of omics technologies including transcriptomics, proteomics, and metabolomics may provide new opportunities for elucidating the cryoprotective roles of trehalose. In addition, according to the “water replacement hypothesis,” trehalose should be present in cells to ensure its optimally protective effects on frozen cells. However, how to load trehalose into sperm is a difficult task that may influence the actual action effects of trehalose. Finally, the cryoprotective function of trehalose on sperm still needs verification by artificial insemination in the field.
Author contributions
BJ: Writing – original draft. LA: Writing – original draft, Writing – review & editing. CLi: Writing – review & editing. JL: Writing – review & editing. CLv: Writing – review & editing. GW: Conceptualization, Funding acquisition, Investigation, Project administration, Resources, Validation, Writing – review & editing. GQ: Conceptualization, Funding acquisition, Investigation, Project administration, Resources, Supervision, Validation, Visualization, Writing – original draft, Writing – review & editing.
Funding
The author(s) declare that financial support was received for the research, authorship, and/or publication of this article. This paper is funded by Yunnan Province Basic Research Project (Grant No. 202301AS070005), the Earmarked Fund for China Agriculture Research System (Grant No. CARS-39-08), and Yunnan International Joint Laboratory of Conservation and Innovative Utilization of Sheep Germplasm Resources (Grant No. 202403AP140017).
Acknowledgments
The authors would like to thank Prof. Badaoui Bouabid from the Biodiversity, Ecology and Genome Laboratory, Department of Biology, Faculty of Science, Mohammed V University in Rabat Morocco; Pr Uchebuchi Ike Osuagwuha (Department of Theriogenology, College of Veterinary Medicine, Michael Okpara University of Agriculture, Umudike, Nigeria), and Pr Anass Benmoula (Department of Life Sciences, Polydisciplinary Faculty of Larache, Abdelmalek Essaadi University, 745 BP, 92004 Larache, Morocco), for theirs assistance with the English editing of the manuscript.
Conflict of interest
The authors declare that the research was conducted in the absence of any commercial or financial relationships that could be construed as a potential conflict of interest.
Publisher’s note
All claims expressed in this article are solely those of the authors and do not necessarily represent those of their affiliated organizations, or those of the publisher, the editors and the reviewers. Any product that may be evaluated in this article, or claim that may be made by its manufacturer, is not guaranteed or endorsed by the publisher.
References
1. Mara, L, Casu, S, Carta, A, and Dattena, M. Cryobanking of farm animal gametes and embryos as a means of conserving livestock genetics. Anim Reprod Sci. (2013) 138:25–38. doi: 10.1016/j.anireprosci.2013.02.006
2. Bailey, JL, Bilodeau, JF, and Cormier, N. Semen cryopreservation in domestic animals: a damaging and capacitating phenomenon. J Androl. (2000) 21:1–7. doi: 10.1002/j.1939-4640.2000.tb03268.x
3. Lv, C, Wu, G, Hong, Q, and Quan, G. Spermatozoa cryopreservation: state of art and future in small ruminants. Biopreserv Biobank. (2019) 17:171–82. doi: 10.1089/bio.2018.0113
4. Hai, E, Li, B, Zhang, J, and Zhang, J. Sperm freezing damage: the role of regulated cell death. Cell Death Discov. (2024) 10:239–13. doi: 10.1038/s41420-024-02013-3
5. Khan, IM, Cao, Z, Liu, H, Khan, A, Rahman, SU, Khan, MZ, et al. Impact of cryopreservation on spermatozoa freeze-thawed traits and relevance OMICS to assess sperm Cryo-tolerance in farm animals. Front Vet Sci. (2021) 8:609180. doi: 10.3389/fvets.2021.609180
6. Hezavehei, M, Sharafi, M, Kouchesfahani, HM, Henkel, R, Agarwal, A, Esmaeili, V, et al. Sperm cryopreservation: A review on current molecular cryobiology and advanced approaches. Reprod Biomed Online. (2018) 37:327–39. doi: 10.1016/j.rbmo.2018.05.012
7. Morris, GJ, Faszer, K, Green, JE, Draper, D, Grout, BWW, and Fonseca, F. Rapidly cooled horse spermatozoa: loss of viability is due to osmotic imbalance during thawing, not intracellular ice formation. Theriogenology. (2007) 68:804–12. doi: 10.1016/j.theriogenology.2007.06.009
8. Ozimic, S, Ban-Frangez, H, and Stimpfel, M. Sperm cryopreservation today: approaches, efficiency, and pitfalls. Curr Issues Mol Biol. (2023) 45:4716–34. doi: 10.3390/cimb45060300
9. Holt, WV . Fundamental aspects of sperm cryobiology: the importance of species and individual differences. Theriogenology. (2000) 53:47–58. doi: 10.1016/s0093-691x(99)00239-3
10. Watson, PF . Recent developments and concepts in the cryopreservation of spermatozoa and the assessment of their post-thawing function. Reprod Fertil Dev. (1995) 7:871–91. doi: 10.1071/rd9950871
11. Sharafi, M, Borghei-Rad, SM, Hezavehei, M, Shahverdi, A, and Benson, JD. Cryopreservation of semen in domestic animals: A review of current challenges, applications, and prospective strategies. Animals (Basel). (2022) 12:3271. doi: 10.3390/ani12233271
12. Jia, B, Larbi, A, Lv, C, Liang, J, Xiang, D, Zhang, B, et al. Identification and validation of ram sperm proteins associated with cryoinjuries caused by the cryopreservation process. Theriogenology. (2022) 184:191–203. doi: 10.1016/j.theriogenology.2022.03.015
13. Watson, PF . The causes of reduced fertility with cryopreserved semen. Anim Reprod Sci. (2000) 60–61:481–92. doi: 10.1016/s0378-4320(00)00099-3
14. Barbas, JP, and Mascarenhas, RD. Cryopreservation of domestic animal sperm cells. Cell Tissue Bank. (2009) 10:49–62. doi: 10.1007/s10561-008-9081-4
15. Brinsko, SP, and Varner, DD. Artificial insemination and preservation of semen. Vet Clin N Am Equine Pract. (1992) 8:205–18. doi: 10.1016/S0749-0739(17)30476-5
16. Gillan, L, Maxwell, WMC, and Evans, G. Preservation and evaluation of semen for artificial insemination. Reprod Fertil Dev. (2004) 16:447–54. doi: 10.1071/RD04034
17. Kaabi, M, Alvarez, M, Anel, E, Chamorro, CA, Boixo, JC, de Paz, P, et al. Influence of breed and age on morphometry and depth of inseminating catheter penetration in the ewe cervix: a postmortem study. Theriogenology. (2006) 66:1876–83. doi: 10.1016/j.theriogenology.2006.04.039
18. Menchaca, A, and Rubianes, E. New treatments associated with timed artificial insemination in small ruminants. Reprod Fertil Dev. (2004) 16:403–13. doi: 10.1071/RD04037
19. Pau, S, Falchi, L, Ledda, M, Bogliolo, L, Ariu, F, and Zedda, MT. Surgery on cervical folds for transcervical intrauterine artificial insemination with frozen-thawed semen enhances pregnancy rates in the sheep. Theriogenology. (2019) 126:28–35. doi: 10.1016/j.theriogenology.2018.11.019
20. Lambo, C, Grahn, R, Lyons, L, Bateman, H, Newsom, J, and Swanson, W. Comparative fertility of freshly collected vs frozen-thawed semen with laparoscopic Oviductal artificial insemination in domestic cats. Reprod Domest Anim. (2012) 47:284–8. doi: 10.1111/rda.12038
21. Liang, J, Larbi, A, Lv, C, Ali, S, Wu, G, and Quan, G. Fertility results after exocervical insemination using goat semen cryopreserved with extenders based on egg yolk, skim milk, or soybean lecithin. Reprod Domest Anim. (2023) 58:431–42. doi: 10.1111/rda.14304
22. Pool, KR, Rickard, JP, Tumeth, E, and de Graaf, SP. Treatment of rams with melatonin implants in the non-breeding season improves post-thaw sperm progressive motility and DNA integrity. Anim Reprod Sci. (2020) 221:106579. doi: 10.1016/j.anireprosci.2020.106579
23. Yeste, M, Rodríguez-Gil, JE, and Bonet, S. Artificial insemination with frozen-thawed boar sperm. Mol Reprod Dev. (2017) 84:802–13. doi: 10.1002/mrd.22840
24. Yánez-Ortiz, I, Catalán, J, Rodríguez-Gil, JE, Miró, J, and Yeste, M. Advances in sperm cryopreservation in farm animals: cattle, horse, pig and sheep. Anim Reprod Sci. (2022) 246:106904. doi: 10.1016/j.anireprosci.2021.106904
25. Choong, C, and Wales, R. The use of various diluents for deep-freezing bull spermatozoa. Aust J Bio Sci. (1963) 16:896. doi: 10.1071/BI9630896
26. Ezzati, M, Shanehbandi, D, Hamdi, K, Rahbar, S, and Pashaiasl, M. Influence of cryopreservation on structure and function of mammalian spermatozoa: an overview. Cell Tissue Bank. (2020) 21:1–15. doi: 10.1007/s10561-019-09797-0
27. Peris-Frau, P, Martín-Maestro, A, Iniesta-Cuerda, M, Sánchez-Ajofrín, I, Mateos-Hernández, L, Garde, JJ, et al. Freezing-thawing procedures remodel the proteome of ram sperm before and after in vitro capacitation. Int J Mol Sci. (2019) 20:E4596. doi: 10.3390/ijms20184596
28. Purdy, PH . A review on goat sperm cryopreservation. Small Rumin Res. (2006) 63:215–25. doi: 10.1016/j.smallrumres.2005.02.015
29. Bhattacharya, S . Cryoprotectants and their usage in cryopreservation process. In: Cryopreservation biotechnology in biomedical and biological sciences. London, UK: IntechOpen (2018) 7–19.
30. Rittschof, D . A brief review of current strategies and advances in cryopreservation techniques. AIBM. (2023) 17:001–3. doi: 10.19080/AIBM.2024.17.555978
31. Bohlool, Z, Mohammadi, M, Mehr, MR-A, Hossein-Zadeh, NG, Bohlool, Z, Mohammadi, M, et al. Effect of different concentrations of trehalose and glycerol on the freezability of ram semen using soybean lecithin-based diluents. Anim Prod Sci. (2014) 55:666–71. doi: 10.1071/AN13431
32. Najafi, A, Daghigh-Kia, H, Dodaran, HV, Mehdipour, M, and Alvarez-Rodriguez, M. Ethylene glycol, but not DMSO, could replace glycerol inclusion in soybean lecithin-based extenders in ram sperm cryopreservation. Anim Reprod Sci. (2017) 177:35–41. doi: 10.1016/j.anireprosci.2016.12.004
33. Silva, ECB, Cajueiro, JFP, Silva, SV, Vidal, AH, Soares, PC, and Guerra, MMP. In vitro evaluation of ram sperm frozen with glycerol, ethylene glycol or acetamide. Anim Reprod Sci. (2012) 132:155–8. doi: 10.1016/j.anireprosci.2012.05.014
34. Naing, SW, Wahid, H, Mohd Azam, K, Rosnina, Y, Zuki, AB, Kazhal, S, et al. Effect of sugars on characteristics of Boer goat semen after cryopreservation. Anim Reprod Sci. (2010) 122:23–8. doi: 10.1016/j.anireprosci.2010.06.006
35. Quan, GB, Hong, QH, Lan, ZG, Yang, HY, and Wu, SS. Comparison of the effect of various disaccharides on frozen goat spermatozoa. Biopreserv Biobank. (2012) 10:439–45. doi: 10.1089/bio.2012.0013
36. Yildiz, C, Kaya, A, Aksoy, M, and Tekeli, T. Influence of sugar supplementation of the extender on motility, viability and acrosomal integrity of dog spermatozoa during freezing. Theriogenology. (2000) 54:579–85. doi: 10.1016/S0093-691X(00)00373-3
37. Al-Mutary, MG . Use of antioxidants to augment semen efficiency during liquid storage and cryopreservation in livestock animals: A review. J King Saud Univ. (2021) 33:101226. doi: 10.1016/j.jksus.2020.10.023
38. Lv, C, Larbi, A, Wu, G, Hong, Q, and Quan, G. Improving the quality of cryopreserved goat semen with a commercial bull extender supplemented with resveratrol. Anim Reprod Sci. (2019) 208:106127. doi: 10.1016/j.anireprosci.2019.106127
39. Zou, J, Wei, L, Li, D, Zhang, Y, Wang, G, Zhang, L, et al. Effect of glutathione on sperm quality in Guanzhong dairy goat sperm during cryopreservation. Front Vet Sci. (2021) 8:771440. doi: 10.3389/fvets.2021.771440
40. Lv, C, Larbi, A, Memon, S, Liang, J, Fu, X, Wu, G, et al. The effects of antifreeze protein III supplementation on the Cryosurvival of goat spermatozoa during cryopreservation. Biopreserv Biobank. (2021) 19:298–305. doi: 10.1089/bio.2020.0140
41. Masuda, Y, Kheawkanha, T, Nagahama, A, Kawasaki, K, Konno, T, Yamanaka, K, et al. Antifreeze protein type III addition to freezing extender comprehensively improves post-thaw sperm properties in Okinawan native Agu pig. Anim Reprod Sci. (2023) 252:107232. doi: 10.1016/j.anireprosci.2023.107232
42. Monteiro, MM, de Mello Seal, DC, de Souza, JH, Trevisan, M, Arruda, LCP, Silva, SV, et al. Effect of antifreeze protein type III on frozen/thawed of spermatozoa recover from goat epididymis. Res Vet Sci. (2023) 154:108–12. doi: 10.1016/j.rvsc.2022.12.006
43. Quan, GB, Li, DJ, Ma, Y, Zhu, L, Lv, CR, and Hong, QH. Cryopreservation of ram spermatozoa in the presence of cyclohexanhexol-derived synthetic ice blocker. Small Rumin Res. (2015) 123:110–7. doi: 10.1016/j.smallrumres.2014.11.007
44. Quan, GB, Wu, SS, Lan, ZG, Yang, HY, Shao, QY, and Hong, QH. The effects of 1,4-cyclohexanediol on frozen ram spermatozoa. Cryo Letters. (2013) 34:217–27.
45. Aisen, E, Quintana, M, Medina, V, Morello, H, and Venturino, A. Ultramicroscopic and biochemical changes in ram spermatozoa cryopreserved with trehalose-based hypertonic extenders. Cryobiology. (2005) 50:239–49. doi: 10.1016/j.cryobiol.2005.02.002
46. El-Badry, DA, Abo El-Maaty, AM, and El Sisy, GA. The effect of Trehalose supplementation of INRA-82 extender on quality and fertility of cooled and frozen-thawed stallion spermatozoa. J Equine Vet. (2017) 48:86–92. doi: 10.1016/j.jevs.2016.08.020
47. Öztürk, AE, Bodu, M, Bucak, MN, Ağır, V, Özcan, A, Keskin, N, et al. The synergistic effect of trehalose and low concentrations of cryoprotectants can improve post-thaw ram sperm parameters. Cryobiology. (2020) 95:157–63. doi: 10.1016/j.cryobiol.2020.03.008
48. Quan, GB, Hong, QH, Hong, QY, Yang, HY, and Wu, SS. The effects of trehalose and sucrose on frozen spermatozoa of Yunnan semi-fine wool sheep during a non-mating season. Cryo Letters. (2012) 33:307–17.
49. Uysal, O, and Bucak, MN. The role of different trehalose concentrations and cooling rates in freezing of ram semen. Ankara Univ Vet Fak Derg. (2009) 56:99–103. doi: 10.1501/Vetfak_0000002176
50. Anjos, C, Santos, AL, Duarte, D, Matias, D, and Cabrita, E. Effect of Trehalose and sucrose in post-thaw quality of Crassostrea angulata sperm. Front Physiol. (2021) 12:749735. doi: 10.3389/fphys.2021.749735
51. Zhu, Z, Fan, X, Pan, Y, Lu, Y, and Zeng, W. Trehalose improves rabbit sperm quality during cryopreservation. Cryobiology. (2017) 75:45–51. doi: 10.1016/j.cryobiol.2017.02.006
52. Nur, Z, Zik, B, Ustuner, B, Sagirkaya, H, and Ozguden, CG. Effects of different cryoprotective agents on ram sperm morphology and DNAintegrity. Theriogenology. (2010) 73:1267–75. doi: 10.1016/j.theriogenology.2009.12.007
53. Schulz, M, Risopatrón, J, Matus, G, Pineda, E, Rojas, C, Isachenko, V, et al. Trehalose sustains a higher post-thaw sperm motility than sucrose in vitrified human sperm. Andrologia. (2017) 49:e12757. doi: 10.1111/and.12757
54. Molinia, FC, Evans, G, Quintana Casares, PI, and Maxwell, WMC. Effect of monosaccharides and disaccharides in Tris-based diluents on motility, acrosome integrity and fertility of pellet frozen ram spermatozoa. Anim Reprod Sci. (1994) 36:113–22. doi: 10.1016/0378-4320(94)90058-2
55. Pérez-Marín, CC, Requena, FD, Arando, A, Ortiz-Villalón, S, Requena, F, and Agüera, EI. Effect of trehalose- and sucrose-based extenders on equine sperm quality after vitrification: preliminary results. Cryobiology. (2018) 80:62–9. doi: 10.1016/j.cryobiol.2017.12.002
56. Jia, B, Memon, S, Liang, J, Lv, C, Hong, Q, Wu, G, et al. Trehalose modifies the protein profile of ram spermatozoa during cryopreservation. Theriogenology. (2021) 171:21–9. doi: 10.1016/j.theriogenology.2021.05.004
57. Crowe, JH, and Crowe, LM. Preservation of mammalian cells—learning nature’s tricks. Nat Biotechnol. (2000) 18:145–6. doi: 10.1038/72580
58. Crowe, JH, Crowe, LM, Oliver, AE, Tsvetkova, N, Wolkers, W, and Tablin, F. The trehalose myth revisited: introduction to a symposium on stabilization of cells in the dry state. Cryobiology. (2001) 43:89–105. doi: 10.1006/cryo.2001.2353
59. Alrosan, M, Almajwal, AM, Al-Qaisi, A, Gammoh, S, Alu’datt, MH, Al Qudsi, FR, et al. Evaluation of digestibility, solubility, and surface properties of trehalose-conjugated quinoa proteins prepared via pH shifting technique. Food Chem X. (2024) 22:101397. doi: 10.1016/j.fochx.2024.101397
60. Chen, A, and Gibney, PA. Dietary Trehalose as a bioactive nutrient. Nutrients. (2023) 15:1393. doi: 10.3390/nu15061393
61. O’Neill, MK, Piligian, BF, Olson, CD, Woodruff, PJ, and Swarts, BM. Tailoring trehalose for biomedical and biotechnological applications. Pure Appl Chem. (2017) 89:1223–49. doi: 10.1515/pac-2016-1025
62. Jain, NK, and Roy, I. Effect of trehalose on protein structure. Protein Sci. (2009) 18:24–36. doi: 10.1002/pro.3
63. Vinciguerra, D, Gelb, MB, and Maynard, HD. Synthesis and application of Trehalose materials. JACS Au. (2022) 2:1561–87. doi: 10.1021/jacsau.2c00309
64. Li, J, Wang, H, Wang, L, Yu, D, and Zhang, X. Stabilization effects of saccharides in protein formulations: A review of sucrose, trehalose, cyclodextrins and dextrans. Eur J Pharm Sci. (2024) 192:106625. doi: 10.1016/j.ejps.2023.106625
65. Liu, Y, Zhang, S, Wang, S, Zhang, C, Su, X, Guo, L, et al. Screening and stability evaluation of freeze-dried protective agents for a live recombinant pseudorabies virus vaccine. Vaccines (Basel). (2024) 12:65. doi: 10.3390/vaccines12010065
66. Chen, A, Tapia, H, Goddard, JM, and Gibney, PA. Trehalose and its applications in the food industry. Compr Rev Food Sci Food Saf. (2022) 21:5004–37. doi: 10.1111/1541-4337.13048
67. Bastin, AR, Nazari-Robati, M, Sadeghi, H, Doustimotlagh, AH, and Sadeghi, A. Trehalose and N-acetyl cysteine alleviate inflammatory cytokine production and oxidative stress in LPS-stimulated human peripheral blood mononuclear cells. Immunol Investig. (2022) 51:963–79. doi: 10.1080/08820139.2021.1891095
68. Yu, S, Park, H, and Kim, W. Trehalose inhibits inflammatory responses through mitochondrial reprogramming in RAW 264.7 macrophages. Antioxidants. (2023) 12:1166. doi: 10.3390/antiox12061166
69. Hu, Y, Liu, X, Liu, F, Xie, J, Zhu, Q, and Tan, S. Trehalose in biomedical cryopreservation–properties, mechanisms, delivery methods, applications, benefits, and problems. ACS Biomater Sci Eng. (2023) 9:1190–204. doi: 10.1021/acsbiomaterials.2c01225
70. Liang, J, Lv, C, Yang, H, Zhang, Y, Raza, SHA, Wu, G, et al. Ultrastructural modification of ram sperm frozen with Cyclohexanediol and Trehalose. Biopreserv Biobank. (2022) 20:348–56. doi: 10.1089/bio.2021.0120
71. Zhang, T-Y, Tan, P-C, Xie, Y, Zhang, X-J, Zhang, P-Q, Gao, Y-M, et al. The combination of trehalose and glycerol: an effective and non-toxic recipe for cryopreservation of human adipose-derived stem cells. Stem Cell Res Ther. (2020) 11:460. doi: 10.1186/s13287-020-01969-0
72. El-Sheshtawy, RI, Sisy, GA, and El-Nattat, WS. Effects of different concentrations of sucrose or trehalose on the post-thawing quality of cattle bull semen. Asian Pac J Reprod. (2015) 4:26–31. doi: 10.1016/S2305-0500(14)60053-1
73. Gholami, D, Sharafi, M, Esmaeili, V, Nadri, T, Alaei, L, Riazi, G, et al. Beneficial effects of trehalose and gentiobiose on human sperm cryopreservation. PLoS One. (2023) 18:e0271210. doi: 10.1371/journal.pone.0271210
74. Soltani, L . Role of oleic acid and trehalose on frozen-thawed ram semen. Cryo Letters. (2023) 44:343–51. doi: 10.54680/fr23610110712
75. Zhao, J, Xiao, G, Zhu, W, Fang, D, Li, N, Han, C, et al. Trehalose addition to a Tris-fructose egg yolk extender on quality of ram sperm preserved at 0 °C. Rev Bras Zootec. (2020) 49:e20200061. doi: 10.37496/rbz4920200061
76. Aisen, EG, Medina, VH, and Venturino, A. Cryopreservation and post-thawed fertility of ram semen frozen in different trehalose concentrations. Theriogenology. (2002) 57:1801–8. doi: 10.1016/s0093-691x(02)00653-2
77. Bucak, MN, Akalın, PP, Keskin, N, Bodu, M, Öztürk, AE, İli, P, et al. Combination of fetuin and trehalose in presence of low glycerol has beneficial effects on freeze-thawed ram spermatozoa. Andrology. (2021) 9:1000–9. doi: 10.1111/andr.12974
78. Rezaei, A, Bahmani, HR, Mafakheri, S, Farshad, A, Nazari, P, and Masoudi, R. Protective effects of different doses of MitoQ separately and combined with trehalose on oxidative stress and sperm function of cryopreserved Markhoz goat semen. Cryobiology. (2023) 110:36–43. doi: 10.1016/j.cryobiol.2022.12.019
79. Bucak, MN, Keskin, N, Ili, P, Bodu, M, Akalın, PP, Öztürk, AE, et al. Decreasing glycerol content by co-supplementation of trehalose and taxifolin hydrate in ram semen extender: microscopic, oxidative stress, and gene expression analyses. Cryobiology. (2020) 96:19–29. doi: 10.1016/j.cryobiol.2020.09.001
80. Najafi, A, Zhandi, M, Towhidi, A, Sharafi, M, Akbari Sharif, A, Khodaei Motlagh, M, et al. Trehalose and glycerol have a dose-dependent synergistic effect on the post-thawing quality of ram semen cryopreserved in a soybean lecithin-based extender. Cryobiology. (2013) 66:275–82. doi: 10.1016/j.cryobiol.2013.03.002
81. Kamal, MM, Alam, ME, Das, SK, MostS, Y, Ahmed, S, MstA, R, et al. Effects of glucose and trehalose on tris-citric acid-egg yolk-fructose diluents for semen cryopreservation in goat. J Adv Vet Anim Res. (2023) 10:169–77. doi: 10.5455/javar.2023.j666
82. Khalili, B, Farshad, A, Zamiri, MJ, Rashidi, A, and Fazeli, P. Effects of sucrose and Trehalose on the Freezability of Markhoz goat spermatozoa. Asian Australas J Anim Sci. (2009) 22:1614–9. doi: 10.5713/ajas.2009.90286
83. Nazari, P, Farshad, A, and Hosseini, Y. Protective effects of Trehalose and Pentoxifylline on goat sperm exposed to chilling-freezing process. Biopreserv Biobank. (2022) 20:540–50. doi: 10.1089/bio.2021.0081
84. Tuncer, PB, Taşdemir, U, Büyükleblebici, S, Özgürtaş, T, Coşkun, E, Erol, H, et al. Effects of different doses of trehalose supplementation in egg yolk extender in frozen–thawed angora buck semen. Small Rumin Res. (2013) 113:383–9. doi: 10.1016/j.smallrumres.2013.04.012
85. Xu, B, Wang, Z, Wang, R, Song, G, Zhang, Y, Su, R, et al. Metabolomics analysis of buck semen cryopreserved with trehalose. Front Genet. (2022) 13:938622. doi: 10.3389/fgene.2022.938622
86. Bucak, MN, Ateşşahin, A, Varişli, O, Yüce, A, Tekin, N, and Akçay, A. The influence of trehalose, taurine, cysteamine and hyaluronan on ram semen microscopic and oxidative stress parameters after freeze-thawing process. Theriogenology. (2007) 67:1060–7. doi: 10.1016/j.theriogenology.2006.12.004
87. Dutta, M, Kadirvel, G, Borah, P, Sinha, S, Ahmed, K, Hazarika, G, et al. Effect of membrane stabilizers on semen quality and sperm membrane protein expression during cryopreservation of goat semen. Cryo Letters. (2023) 44:299–306. doi: 10.54680/fr23510110612
88. Bittencourt, RF, Oba, E, de Almeida Biscarde, CE, Azevedo, HC, Bittencourt, MV, de Menezes, GFO, et al. Dimethylacetamide and trehalose for ram semen cryopreservation. Cryobiology. (2018) 85:1–6. doi: 10.1016/j.cryobiol.2018.10.266
89. Cirit, Ü, Bağiş, H, Demir, K, Agca, C, Pabuccuoğlu, S, Varişli, Ö, et al. Comparison of cryoprotective effects of iodixanol, trehalose and cysteamine on ram semen. Anim Reprod Sci. (2013) 139:38–44. doi: 10.1016/j.anireprosci.2013.03.010
90. Özmen, MF, Cirit, Ü, Arıcı, R, Demir, K, Kurt, D, Pabuccuoğlu, S, et al. Evaluation of synergic effects of iodixanol and trehalose on cryosurvival of electroejaculated ram semen. Andrologia. (2020) 52:e13656. doi: 10.1111/and.13656
91. Akhtarshenas, B, Karami Shabankareh, H, Hajarian, H, Bucak, MN, Abdolmohammadi, AR, and Dashtizad, M. The protease inhibitor antipain has a beneficial synergistic effect with trehalose for ram semen cryopreservation. Reprod Domest Anim. (2018) 53:1359–66. doi: 10.1111/rda.13253
92. Aboagla, EM-E, and Terada, T. Effects of the supplementation of trehalose extender containing egg yolk with sodium dodecyl sulfate on the freezability of goat spermatozoa. Theriogenology. (2004) 62:809–18. doi: 10.1016/j.theriogenology.2003.12.003
93. Izanloo, H, Soleimanzadeh, A, Bucak, MN, Imani, M, and Zhandi, M. The effects of varying concentrations of glutathione and trehalose in improving microscopic and oxidative stress parameters in Turkey semen during liquid storage at 5 °C. Cryobiology. (2021) 101:12–9. doi: 10.1016/j.cryobiol.2021.07.002
94. Hu, J-H, Zan, L-S, Zhao, X-L, Li, Q-W, Jiang, Z-L, Li, Y-K, et al. Effects of trehalose supplementation on semen quality and oxidative stress variables in frozen-thawed bovine semen1. J Anim Sci. (2010) 88:1657–62. doi: 10.2527/jas.2009-2335
95. Len, JS, Koh, WSD, and Tan, S-X. The roles of reactive oxygen species and antioxidants in cryopreservation. Biosci Rep. (2019) 39:BSR20191601. doi: 10.1042/BSR20191601
96. Majzoub, A, and Agarwal, A. Antioxidants in sperm cryopreservation. In: SJ Parekattil, SC Esteves, and A Agarwal, editors. Male infertility: Contemporary clinical approaches, andrology, ART and Antioxidants. Cham: Springer International Publishing (2020). 671–8.
97. Aboagla, EM-E, and Terada, T. Trehalose-enhanced fluidity of the goat sperm membrane and its protection during freezing. Biol Reprod. (2003) 69:1245–50. doi: 10.1095/biolreprod.103.017889
98. Gómez-Fernández, J, Gómez-Izquierdo, E, Tomás, C, Mocé, E, and de Mercado, E. Effect of different monosaccharides and disaccharides on boar sperm quality after cryopreservation. Anim Reprod Sci. (2012) 133:109–16. doi: 10.1016/j.anireprosci.2012.06.010
99. Stanishevskaya, O, Silyukova, Y, Pleshanov, N, and Kurochkin, A. Role of mono- and disaccharide combination in Cryoprotective medium for rooster semen to ensure Cryoresistance of spermatozoa. Molecules. (2021) 26:5920. doi: 10.3390/molecules26195920
100. Crowe, JH, Crowe, LM, Carpenter, JF, Rudolph, AS, Wistrom, CA, Spargo, BJ, et al. Interactions of sugars with membranes. Biochimica et Biophysica Acta (BBA) – reviews on. Biomembranes. (1988) 947:367–84. doi: 10.1016/0304-4157(88)90015-9
101. Abazari, A, Meimetis, LG, Budin, G, Bale, SS, Weissleder, R, and Toner, M. Engineered Trehalose permeable to mammalian cells. PLoS One. (2015) 10:e0130323. doi: 10.1371/journal.pone.0130323
102. Jenkins, TG, Aston, KI, James, ER, and Carrell, DT. Sperm epigenetics in the study of male fertility, offspring health, and potential clinical applications. Syst Biol Reprod Med. (2017) 63:69–76. doi: 10.1080/19396368.2016.1274791
103. Mussauer, H, Sukhorukov, VL, and Zimmermann, U. Trehalose improves survival of electrotransfected mammalian cells. Cytometry. (2001) 45:161–9. doi: 10.1002/1097-0320(20011101)45:3<161::AID-CYTO1159>3.0.CO;2-7
104. Stewart, S, and He, X. Intracellular delivery of Trehalose for cell banking. Langmuir. (2019) 35:7414–22. doi: 10.1021/acs.langmuir.8b02015
105. Cummins, HZ, Zhang, H, Oh, J, Seo, J-A, Kim, HK, Hwang, Y-H, et al. The liquid–glass transition in sugars: relaxation dynamics in trehalose. J Non-Cryst Solids. (2006) 352:4464–74. doi: 10.1016/j.jnoncrysol.2006.02.182
106. Pereira, RM, and Marques, CC. Animal oocyte and embryo cryopreservation. Cell Tissue Bank. (2008) 9:267–77. doi: 10.1007/s10561-008-9075-2
107. Olgenblum, GI, Sapir, L, and Harries, D. Properties of aqueous Trehalose mixtures: glass transition and hydrogen bonding. J Chem Theory Comput. (2020) 16:1249–62. doi: 10.1021/acs.jctc.9b01071
108. Rani, N, Maiti, A, and Daschakraborty, S. Comparative study of molecular mechanisms of sucrose & trehalose mediated protection and stabilization of Escherichia coli lipid membrane during desiccation. Chem Phys Impact. (2024) 8:100645. doi: 10.1016/j.chphi.2024.100645
109. Stanishevskaya, O, Silyukova, Y, Tereshina, V, Ianutsevich, E, Pleshanov, N, Kurochkin, A, et al. Trehalose as a stabilizer of the lipid composition of membranes and the composition of the cytosol of frozen/thawed rooster spermatozoa. Agriculture. (2023) 13:1387. doi: 10.3390/agriculture13071387
110. Sundaramurthi, P, Patapoff, TW, and Suryanarayanan, R. Crystallization of Trehalose in frozen solutions and its phase behavior during drying. Pharm Res. (2010) 27:2374–83. doi: 10.1007/s11095-010-0243-2
111. Luzardo, M d C, Amalfa, F, Nuñez, AM, Díaz, S, Biondi de Lopez, AC, and Disalvo, EA. Effect of trehalose and sucrose on the hydration and dipole potential of lipid bilayers. Biophys J. (2000) 78:2452–8. doi: 10.1016/S0006-3495(00)76789-0
112. Ahmad, E, and Aksoy, M. Trehalose as a cryoprotective agent for the sperm cells: a mini review. (2012) 1:123–129. Available at: https://www.academia.edu/25421641/Trehalose_as_a_Cryoprotective_Agent_for_the_Sperm_Cells_A_Mini_Review (accessed July 18, 2024).
113. Jhamb, D, Talluri, TR, Sharma, S, Juneja, R, Nirwan, SS, Yadav, D, et al. Freezability and fertility rates of stallion semen supplemented with Trehalose in lactose extender. J Equine Vet. (2023) 126:104293. doi: 10.1016/j.jevs.2023.104293
114. Cseh, S, Faigl, V, and Amiridis, GS. Semen processing and artificial insemination in health management of small ruminants. Anim Reprod Sci. (2012) 130:187–92. doi: 10.1016/j.anireprosci.2012.01.014
115. Ahmad, E, Naseer, Z, Aksoy, M, Küçük, N, Uçan, U, Serin, I, et al. Trehalose enhances osmotic tolerance and suppresses lysophosphatidylcholine-induced acrosome reaction in ram spermatozoon. Andrologia. (2015) 47:786–92. doi: 10.1111/and.12329
116. Chen, X, Wang, Y, Zhu, H, Hao, H, Zhao, X, Qin, T, et al. Comparative transcript profiling of gene expression of fresh and frozen-thawed bull sperm. Theriogenology. (2015) 83:504–11. doi: 10.1016/j.theriogenology.2014.10.015
117. Ebenezer Samuel King, JP, Sinha, MK, Kumaresan, A, Nag, P, Das Gupta, M, Arul Prakash, M, et al. Cryopreservation process alters the expression of genes involved in pathways associated with the fertility of bull spermatozoa. Front Genet. (2022) 13:1025004. doi: 10.3389/fgene.2022.1025004
118. Kopeika, J, Thornhill, A, and Khalaf, Y. The effect of cryopreservation on the genome of gametes and embryos: principles of cryobiology and critical appraisal of the evidence. Hum Reprod Update. (2015) 21:209–27. doi: 10.1093/humupd/dmu063
119. Nazari, H, Ahmadi, E, Hosseini Fahraji, H, Afzali, A, and Davoodian, N. Cryopreservation and its effects on motility and gene expression patterns and fertilizing potential of bovine epididymal sperm. Vet Med Sci. (2020) 7:127–35. doi: 10.1002/vms3.355
120. Pironcheva, GL, Miteva, K, Vaisberg, C, and Russev, G. Influence of freezing at different temperatures on the transcription activity of buffalo sperm chromatin. Cytobios. (1995) 83:207–10.
121. Riesco, MF, and Robles, V. Cryopreservation causes genetic and epigenetic changes in zebrafish genital ridges. PLoS One. (2013) 8:e67614. doi: 10.1371/journal.pone.0067614
122. Trummer, H, Tucker, K, Young, C, Kaula, N, and Meacham, RB. Effect of storage temperature on sperm cryopreservation. Fertil Steril. (1998) 70:1162–4. doi: 10.1016/S0015-0282(98)00349-5
123. Ali, MA, Qin, Z, Dou, S, Huang, A, Wang, Y, Yuan, X, et al. Cryopreservation induces acetylation of metabolism-related proteins in boar sperm. Int J Mol Sci. (2023) 24:10983. doi: 10.3390/ijms241310983
124. Larbi, A, Li, C, and Quan, G. An updated review on the application of proteomics to explore sperm cryoinjury mechanisms in livestock animals. Anim Reprod Sci. (2024) 263:107441. doi: 10.1016/j.anireprosci.2024.107441
125. Li, P, Hulak, M, Koubek, P, Sulc, M, Dzyuba, B, Boryshpolets, S, et al. Ice-age endurance: the effects of cryopreservation on proteins of sperm of common carp, Cyprinus carpio L. Theriogenology. (2010) 74:413–23. doi: 10.1016/j.theriogenology.2010.02.024
126. Lv, C, Larbi, A, Memon, S, Liang, J, Zhao, X, Shao, Q, et al. The proteomic characterization of ram sperm during cryopreservation analyzed by the two-dimensional electrophoresis coupled with mass spectrometry. Cryobiology. (2020) 97:37–45. doi: 10.1016/j.cryobiol.2020.10.011
127. Nynca, J, Arnold, GJ, Fröhlich, T, and Ciereszko, A. Cryopreservation-induced alterations in protein composition of rainbow trout semen. Proteomics. (2015) 15:2643–54. doi: 10.1002/pmic.201400525
128. Ofosu, J, Nartey, MA, Mo, X, Ye, J, Zhang, Y, Zeng, C, et al. Ram sperm cryopreservation disrupts metabolism of unsaturated fatty acids. Theriogenology. (2023) 204:8–17. doi: 10.1016/j.theriogenology.2023.03.023
129. Yu, J, Wang, Z, Wang, F, Wang, W, Ge, S, Fan, Z, et al. Changes in sperm metabolites of Dezhou donkey after cryopreservation. Reprod Domest Anim. (2022) 57:1593–601. doi: 10.1111/rda.14236
130. Zhang, Y, Liang, H, Liu, Y, Zhao, M, Xu, Q, Liu, Z, et al. Metabolomic analysis and identification of sperm Freezability-related metabolites in boar seminal plasma. Animals (Basel). (2021) 11:1939. doi: 10.3390/ani11071939
131. Xu, B, Wang, R, Wang, Z, Liu, H, Wang, Z, Zhang, W, et al. Evaluation of lipidomic change in goat sperm after cryopreservation. Front Vet Sci. (2022) 9:1004683. doi: 10.3389/fvets.2022.1004683
132. Goularte, KL, Gastal, GDA, Schiavon, RS, Gonçalves, AO, Schneider, JR, Corcini, CD, et al. Association between the presence of protein bands in ram seminal plasma and sperm tolerance to freezing. Anim Reprod Sci. (2014) 146:165–9. doi: 10.1016/j.anireprosci.2014.03.009
133. Dai, D-H, Qazi, IH, Ran, M-X, Liang, K, Zhang, Y, Zhang, M, et al. Exploration of miRNA and mRNA profiles in fresh and frozen-thawed boar sperm by transcriptome and small RNA sequencing. Int J Mol Sci. (2019) 20:802. doi: 10.3390/ijms20040802
134. Fraser, L, Brym, P, Pareek, CS, Mogielnicka-Brzozowska, M, Paukszto, Ł, Jastrzębski, JP, et al. Transcriptome analysis of boar spermatozoa with different freezability using RNA-Seq. Theriogenology. (2020) 142:400–13. doi: 10.1016/j.theriogenology.2019.11.001
135. Qin, Z, Wang, W, Ali, MA, Wang, Y, Zhang, Y, Zhang, M, et al. Transcriptome-wide m6A profiling reveals mRNA post-transcriptional modification of boar sperm during cryopreservation. BMC Genomics. (2021) 22:588. doi: 10.1186/s12864-021-07904-8
136. Fraser, L, Paukszto, Ł, Mańkowska, A, Brym, P, Gilun, P, Jastrzębski, JP, et al. Regulatory potential of long non-coding RNAs (lncRNAs) in boar spermatozoa with good and poor Freezability. Life (Basel). (2020) 10:300. doi: 10.3390/life10110300
137. Kadivar, A, Shams Esfandabadi, N, Dehghani Nazhvani, E, Shirazi, A, and Ahmadi, E. Effects of cryopreservation on stallion sperm protamine messenger RNAs. Reprod Domest Anim. (2020) 55:274–82. doi: 10.1111/rda.13615
138. Ran, M-X, Li, Y, Zhang, Y, Liang, K, Ren, Y-N, Zhang, M, et al. Transcriptome sequencing reveals the differentially expressed lncRNAs and mRNAs involved in cryoinjuries in frozen-thawed Giant panda (Ailuropoda melanoleuca) sperm. Int J Mol Sci. (2018) 19:E3066. doi: 10.3390/ijms19103066
139. Valcarce, DG, Cartón-García, F, Herráez, MP, and Robles, V. Effect of cryopreservation on human sperm messenger RNAs crucial for fertilization and early embryo development. Cryobiology. (2013) 67:84–90. doi: 10.1016/j.cryobiol.2013.05.007
140. Bonde, JPE, Ernst, E, Jensen, TK, Hjollund, NHI, Kolstad, H, Scheike, T, et al. Relation between semen quality and fertility: a population-based study of 430 first-pregnancy planners. Lancet. (1998) 352:1172–7. doi: 10.1016/S0140-6736(97)10514-1
Keywords: semen, small ruminant, cryopreservation, trehalose, cryoinjury
Citation: Jia B, Allai L, Li C, Liang J, Lv C, Wu G and Quan G (2024) A review on the functional roles of trehalose during cryopreservation of small ruminant semen. Front. Vet. Sci. 11:1467242. doi: 10.3389/fvets.2024.1467242
Edited by:
Amal M. Aboelmaaty, National Research Center, EgyptReviewed by:
Wael A. Khalil, Mansoura University, EgyptEjaz Ahmad, Bahauddin Zakariya University, Pakistan
Copyright © 2024 Jia, Allai, Li, Liang, Lv, Wu and Quan. This is an open-access article distributed under the terms of the Creative Commons Attribution License (CC BY). The use, distribution or reproduction in other forums is permitted, provided the original author(s) and the copyright owner(s) are credited and that the original publication in this journal is cited, in accordance with accepted academic practice. No use, distribution or reproduction is permitted which does not comply with these terms.
*Correspondence: Guobo Quan, d2FsdHEyMDAyMDEwOUAxNjMuY29t
†These authors have contributed equally to this work