- 1State Key Laboratory of Forage Breeding-by-Design and Utilization, National Engineering Laboratory for Pollution Control and Waste Utilization in Livestock and Poultry Production, and Hunan Provincial Key Laboratory of Animal Nutritional Physiology and Metabolic Process, Institute of Subtropical Agriculture, Chinese Academy of Sciences, Changsha, China
- 2Dairy Department, National Research Centre, Giza, Egypt
- 3College of Animal Science, Guizhou University, Guiyang, China
- 4College of Advanced Agricultural Sciences, University of Chinese Academy of Sciences, Beijing, China
Introduction: The present study explored the immune response, milk production and health status of mastitis-infected lactating cows fed diets supplemented with Macleaya cordata extract.
Methods: Twenty-four Holstein and Jersey cows were equally assigned to two experimental groups: the first group was fed a control diet (control), and the second experimental group was fed a control diet plus Macleaya extract at 8 g/head/d (Macleaya). The experiment was conducted for 60 days. The daily milk yield was recorded, and the milk samples were analyzed for total solids, fat, protein, and lactose contents.
Results: Blood samples were analyzed for different blood constituents, biochemical parameters, antioxidant capacity and immune indices. Compared with the control, the addition of Macleaya improved immune indices (p < 0.05). No significant differences (p > 0.05) were recorded between the two groups for different rumen liquor parameters, antioxidant capacities, milk yields or compositions. However, supplementing the diet with Macleaya significantly decreased SCC, SAA, and endotoxin.
Conclusion: This study suggested that supplementing the diets of lactating cows with Macleaya extract potentially improved their immune competence without adversely impacting their productive performance.
Introduction
Mastitis in cows is an inflammatory reaction of the mammary gland udder tissue caused by either physical injury or microbial infection. It is regarded as the most prevalent disease that causes financial losses for the dairy industry because of lower milk quality and output (1, 2). On average, the overall failure cost caused by bovine mastitis is projected to be $147 per cow per year, with milk production losses and culling accounting for 11% to 18% of the gross margin per cow per year (3). Seventy percent of the overall losses are due to mammary tissue injury, which causes reduced milk output (4). Clinical, subclinical, and chronic mastitis are the three categories into which bovine mastitis can be divided according to the severity of inflammation. A fever and a red, swollen udder are two obvious signs of a clinical case of bovine mastitis that are simple to identify. The cow milk seems watery, with flakes and clots (5).
In dairy cows, mastitis is caused by bacteria that enter the teat canal and mammary glands and cause inflammation of the udder. These bacteria grow and create toxins that injure milk-secreting tissue, in addition to causing physical damage and chemical irritation. These factors lead to an increase in leukocytes, or somatic cells, in milk, which decreases their amount and degrades the quality of both the milk and its byproducts. Seventy percent of the losses are attributable to injury to the mammary tissue, which results in reduced milk production (4). The incidence of mastitis in a herd can be reduced, but mastitis cannot be completely eradicated. Good husbandry and sanitation techniques, teat dipping after milking, treating mastitis during the nonlactating phase, and culling chronically sick animals are the main components in the management of mastitis (5).
The two main strategies for controlling mastitis are to either reduce the teat’s exposure to possible pathogens or increase the ability of dairy animals to fight infection. Widely prevalent in southern China, Macleaya cordata (MC) is a perennial herb belonging to the group Papaveraceae that is used in traditional Chinese medicine (6). The primary bioactive components of MC extract (MCE) are alkaloids, primarily sanguinarine and chelidonine, which have been shown to have antibacterial (7), anti-inflammatory (8), antitumor (9), and antioxidant properties (10).
In the European Union (EU) and China, sanguinarine derived from MC has been authorized for use as a feed supplement. Research has shown that this substance enhances the immune system and growth performance of livestock (11, 12). The majority of MCE research to date has been conducted on monogastric animals. For example, weaning piglets fed with 50 mg/kg MCE improved growth performance and resulted in a lower rate of diarrhea because the treatment increased the serum IgG concentration, antioxidant activity, and profile of beneficial bacteria in the intestine (13). Yang et al. (14) reported that adding 0.3 g/d MCE to the meals of early-weaned goats reduced the inflammatory response in their lower guts. Adding MCE to the sheep diet enhances their immune system, antioxidant levels, and growth performance (15). To the best of our knowledge, little research has been conducted on the consequences of feeding lactating cows MCE. Consequently, the aim of the current study was to examine the effects of supplementing the diets of lactating cows with dietary Macleaya cordata on the immune response, rumen fermentation, and milk yield and composition.
Materials and methods
All the experimental protocols for the use of animals and the experimental procedures in this study were approved by the Laboratory Animal Welfare and Animal Experimental Ethical Inspection Committee, Institute of Subtropical Agriculture, the Chinese Academy of Sciences, Changsha, China.
Twenty-four Holstein and Jersey cows with similar parities (2 parities), calving dates, daily milk yields, mid-lactation rates and recessive mastitis (somatic cell count (SCC) greater than 200 × 103/mL) were selected for the current experiment. Using a completely randomized block design, the experimental animals were divided into two groups with 12 animals (three Holstein and nine Jersey cows) in each group, and the milk SCCs in the control and experimental groups were 480 × 103/mL and 492 × 103/mL, respectively. The experimental diet was offered to meet their nutrient requirements according to NRC (16) recommendations. The cows in the control group were fed a basic diet, as shown in Table 1 (control), and the cows in the experimental group (Macleaya) were fed a basic diet supplemented with Macleaya extract at 8 g/head/d. The active ingredient of macleaya is benzenoid alkaloids (sanguinarine content of 1.5% and chelidonine content ≥ 0.75%).
The experiment lasted for 60 days, including 10 days for adaptation and 50 days as the experimental phase. The animals were raised in individual pens, fed twice daily (06:00 and 13:30), and had free access to clean drinking water.
Sample collection
Feed sample collection
Approximately 500 g of TMR sample was collected and dried in an oven at 65°C for 48 h. Feed samples were stored at −20°C until further analysis.
Rumen liquor sample collection
Representative samples of rumen liquor were collected from the animals at 60 days of the experiment. The samples were collected from the central rumen at 4 h after the morning feeding through the oral cavity via an oral stomach tube as reported by Wang et al. (17). In summary, the first 150 mL of collected rumen mixture was discarded, and then the remaining 150 mL was collected and filtered through four gauze layers while being continuously exposed to carbon dioxide.
Blood sample collection
On the last day of the experiment, blood samples were collected from the jugular vein of the cows at 4 h post morning feeding. Tubes containing the anticoagulant heparin sodium and a venous blood collection needle were used to collect blood samples from the cows. The tubes of collected blood were inverted gently many times to ensure that the blood sample and anticoagulant were fully mixed. The blood samples were centrifuged for 15 min at 3000× g to quickly separate the plasma. After that, the plasma was separated into sterile 1.5 mL Eppendorf tubes and stored at −80°C.
Milk yield recording and sampling
The daily milk yield of each experimental cow was recorded during the 60-day data collection period. Cows were milked three times daily at 05:30, 13:00, and 19:00 h. Milk samples were collected at 60 days, and morning and afternoon milk samples were stored at 4°C until the evening samples were collected. The samples were subsequently pooled according to morning, afternoon, and evening milk yields.
Sample analysis
Feed sample analysis
The feed samples were analyzed for DM (method 930.15), crude protein (CP; method 954.01) and ether extract (EE; method 920.39) content according to the AOAC official methods (18). Neutral detergent fiber (NDF) content was determined according to the procedure of Van Soest et al. (19) without the use of alpha-amylase but with sodium sulfite. Acid detergent fiber (ADF) was analyzed according to AOAC (18) (method 973.18).
Rumen liquor sample analysis
pH and the concentrations of ammonia nitrogen (NH3-N) and total volatile fatty acid and their proportion (VFA) in the rumen liquor were measured via methods described by Wang et al. (17).
Blood sample analysis
Blood samples were used to analyze biochemical parameters, antioxidant parameters, and immune indices. Blood cells [such as the white blood cell (WBC), neutrophil (Neu), lymphocyte (Lym), monocyte (Mon), eosinophil (Eos), basophilic granulocyte (Bas), red blood cell (RBC), hemoglobin (HGB), hematocrit (HCT), mean corpuscular volume (MCV), mean corpuscular-hemoglobin (MCH), mean corpuscular-hemoglobin concentration (MCHC), red blood cell distribution width variable coefficient (RDW-CV), red blood cell distribution width (RDW-SD), platelet count (PLT), mean platelet volume (MPV), platelet distribution width (PDW), and thrombocytocrit (PCT)], and EDTAK 2 anticoagulant whole blood collected within 2 h were tested via an automatic veterinary blood cell analyzer (BC-5000, Mindray, China), and the specific operation was carried out according to the operation procedure of the automatic veterinary blood cell analyzer.
The plasma total protein (TP), albumin (ALB), alanine transaminase (ALT), aspartate transaminase (AST), alkaline phosphatase (ALP), gamma glutamyl transpeptidase (GGT), cholinesterase (CHE), direct bilirubin (DBIL), total bilirubin (TBIL), triglyceride (TG), total cholesterol (CHOL), low-density lipoprotein cholesterol (LDL-c), high-density lipoprotein cholesterol (HDL-c), glucose (GLU), amylase (AMS), D-lactic acid (LAC), lactate dehydrogenase (LDH), blood urea nitrogen (BUN), creatinine (CRE), and calcium (Ca) and phosphorus (P) concentrations were measured on a Mindray BS-230 automatic chemistry analyzer (Shenzhen, China) (20).
For the immune indices, the plasma concentrations of interleukin 1β (IL-1β), interleukin 2 (IL-2), interleukin 6 (IL-6), interleukin 8 (IL-8), tumor necrosis factor (TNF-α), immunoglobulin G (IgG), immunoglobulin A (IgA), and immunoglobulin M (IgM) were determined with a Multimode Microplate Reader (Infinite M200 PRO Multimode, Tecan, Männedorf, Switzerland) and a commercial ELISA kit (CUSABIO, Wuhan, China) according to the manufacturer’s protocols.
The samples were analyzed for different antioxidant parameters via assay kits (ELISAs) according to the manufacturers’ instructions. The total antioxidant capacity (TAC), malondialdehyde (MDA), superoxide dismutase (SOD), and glutathione peroxidase (GPx) contents were determined as described by Khattab et al. (21).
Milk sample analysis
Milk samples were analyzed for fat, lactose, protein, nonfat solid (NFS), and total solids (TS) contents via a Foss Milko Scan FT1 Analyzer (Foss Electric A/S, Hillerod, Denmark). The average yield (g/d) of each milk component was calculated for individual animals by multiplying the milk yield by the component concentration (g/kg) of milk. The serum amyloid A (SAA) concentration in milk samples was determined via specific ELISA kits, and the endotoxin concentration was determined with a Multimode Microplate Reader (Infinite M200 PRO Multimode, Tecan, Männedorf, Switzerland) and a commercial ELISA kit (CUSABIO, Wuhan, China) according to the manufacturer’s protocols. Energy-corrected milk (ECM) was calculated according to Sjaunja et al. (22).
Statistical analysis
All the data were subjected to analysis using ANOVA via the MIXED procedure of SAS (version 9.4; SAS Inc.). The fixed effects of breed, treatment, and the interaction effect between breed and treatment were included in the model. The cows represented a random effect. The results are expressed as the means. A p-value < 0.05 was considered to indicate a statistically significant difference.
Results
Rumen fermentation profile
The effects of supplementation of lactating cows’ diets with Macleaya cordata on rumen fermentation profile are presented in Table 2. All values of the different parameters were within the normal range for each parameter (23). The acetate, A/P ratio, isobutyrate, butyrate, and isovalerate concentrations were not significantly greater (p > 0.05) in the Macleaya group than in the control group. Additionally, insignificant (p > 0.05) reductions in the NH3-N, propionate and TVFA concentrations were detected in the Macleaya group compared with those in the control group.
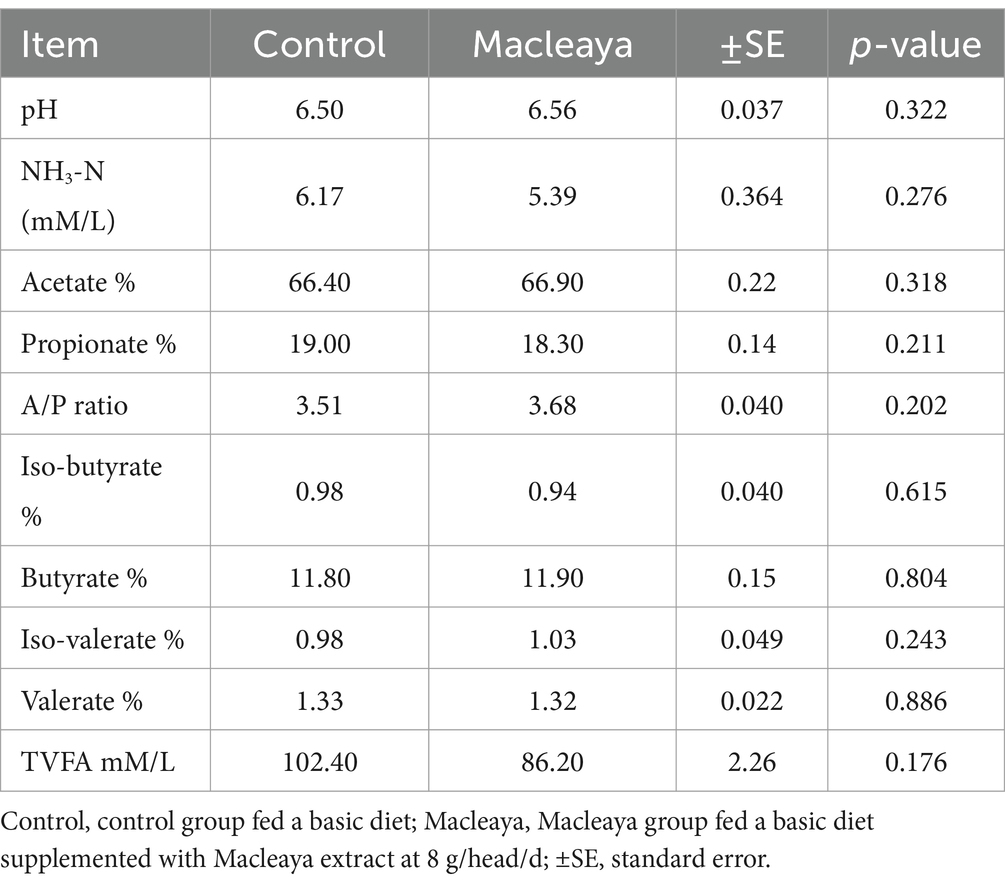
Table 2. Effect of supplementing the diets of lactating cows with Macleaya cordata on ruminal fermentation.
Plasma biochemical values
Table 3 shows the values of the plasma biochemical parameters of the experimental groups. All values of the different parameters were within the normal range of each parameter. No significant differences (p > 0.05) in different plasma biochemical parameters were noted between the experimental groups. Compared with the control group, the Macleaya group tended to decrease (p = 0.09) the concentration of CHE and slightly increased (p > 0.05) the globulin, ALT, AST, GGT, LDH, BUN, CRE, CHOL, HDL-c, amylase, LDL-c and phosphorus concentrations.
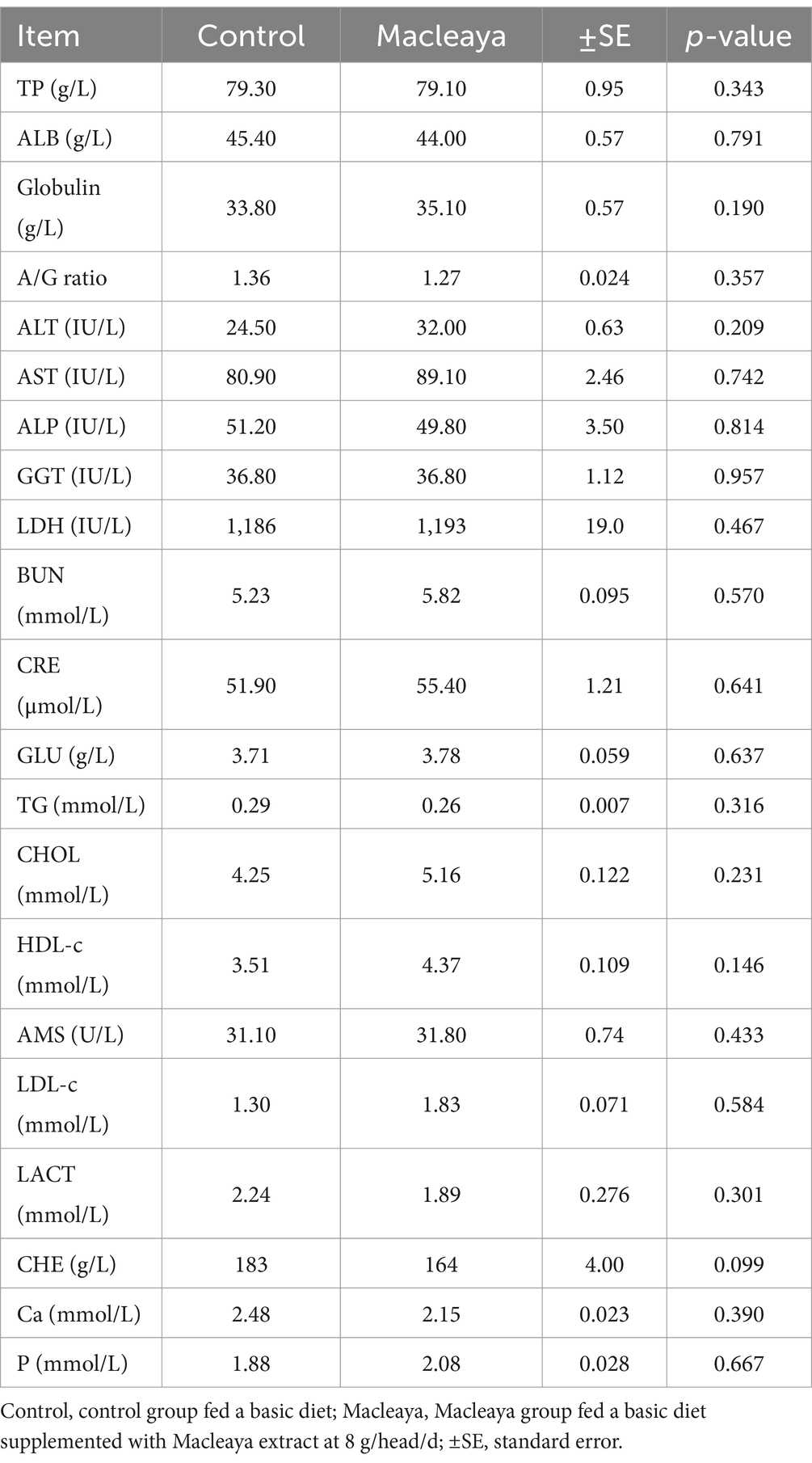
Table 3. Effects of supplementing the diets of lactating cows with Macleaya cordata on plasma biochemical parameters.
Blood constituents
Table 4 shows the values of blood constituents for the experimental groups. No values of different blood constituents were outside the normal range. The white blood cell count, neutrophil count, lymphocyte count, monocyte count, basophil count, RBC count, HGB, HCT, MCHC, RDW-CV, PLT, MPV, and PCT did not significantly differ between the Macleaya and control groups (p > 0.05). Compared with the control group, the Macleaya group presented a significant reduction in Eosinophil. However, compared with the control diet, supplementation with the Macleaya diet significantly increased the MCV, MCH, RDW-SD, and PDW.
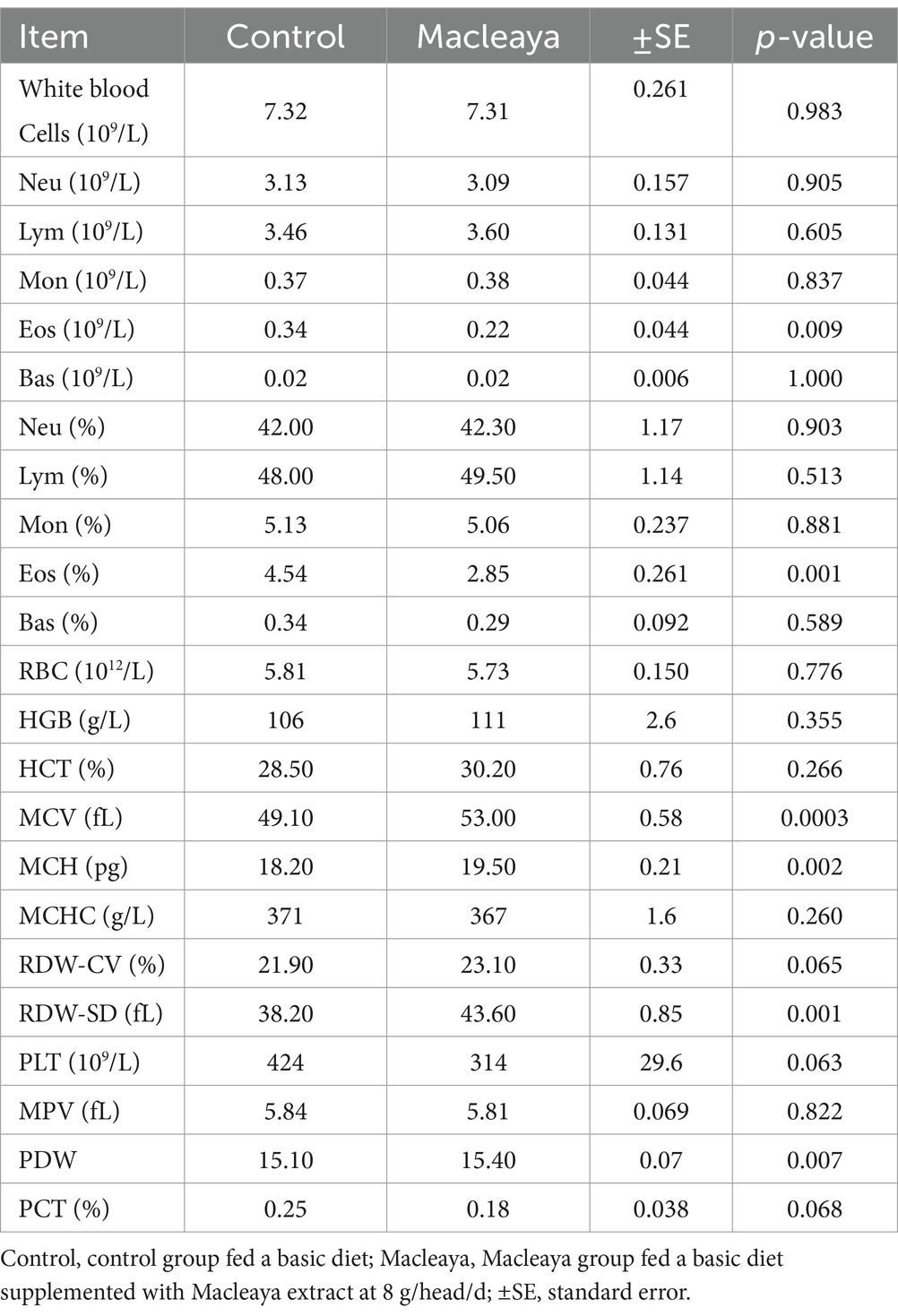
Table 4. Effect of supplementing the diets of lactating cows with Macleaya cordata on blood constituents.
Plasma antioxidant capacity
The antioxidant capacity data are shown in Table 5. The levels of the antioxidant parameters total antioxidant capacity (TAC), superoxide dismutase (SOD), malondialdehyde (MDA) and glutathione peroxidase (GPx) did not significantly (p > 0.05) differ between the Macleaya group and the control group.
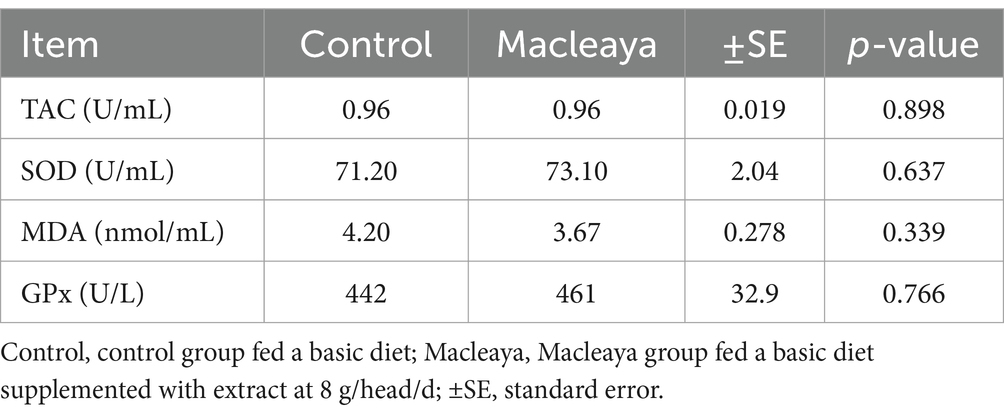
Table 5. Effects of supplementing the diets of lactating cows with Macleaya cordata on plasma antioxidant parameters.
Immune indices
The immune indices of the different experimental animal groups are listed in Table 6. The results revealed significant decreases in the levels of IL-1β (p = 0.020), IL-6 (p = 0.0004), IL-8 (p = 0.008), and TNF-α (p = 0.007) in the Macleaya group compared with those in the control group. However, ceruloplasmin (p = 0.015) was greater in the Macleaya group than in the control group. IL-2, heptoglobin, IgA, IgG, and IgM levels were not significantly different (p > 0.05) between the experimental groups.
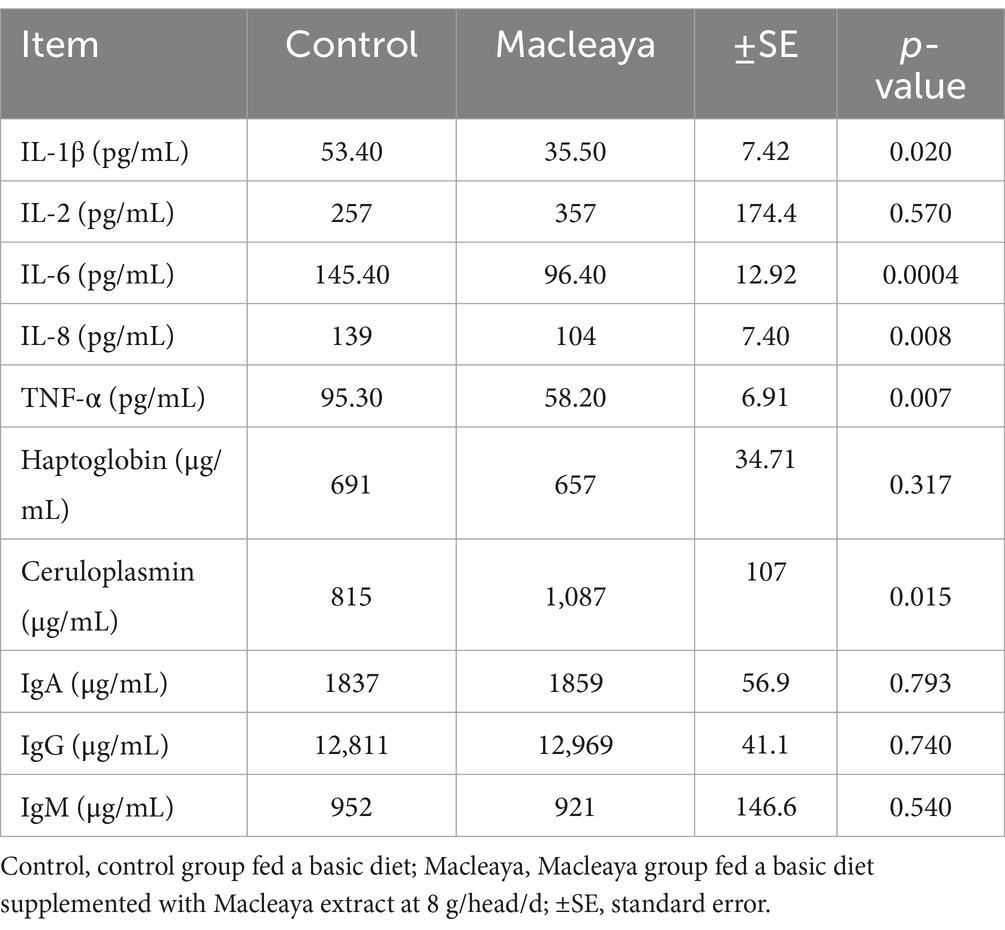
Table 6. Effects of supplementing the diets of lactating cows with Macleaya cordata on immune indices.
Milk yield and composition
The milk yield did not significantly (p > 0.05) differ between the Macleaya and control groups (Table 7). However, the ECM was significantly greater in Macleaya than in the control (p = 0.0203). Additionally, no significant differences in pH, milk total solids content, milk fat content, milk protein content, milk lactose content, or milk NFS were recorded between the experimental groups. The same trend was observed for milk total solids yield, milk fat yield, milk protein yield, milk lactose yield, and milk NFS yield.
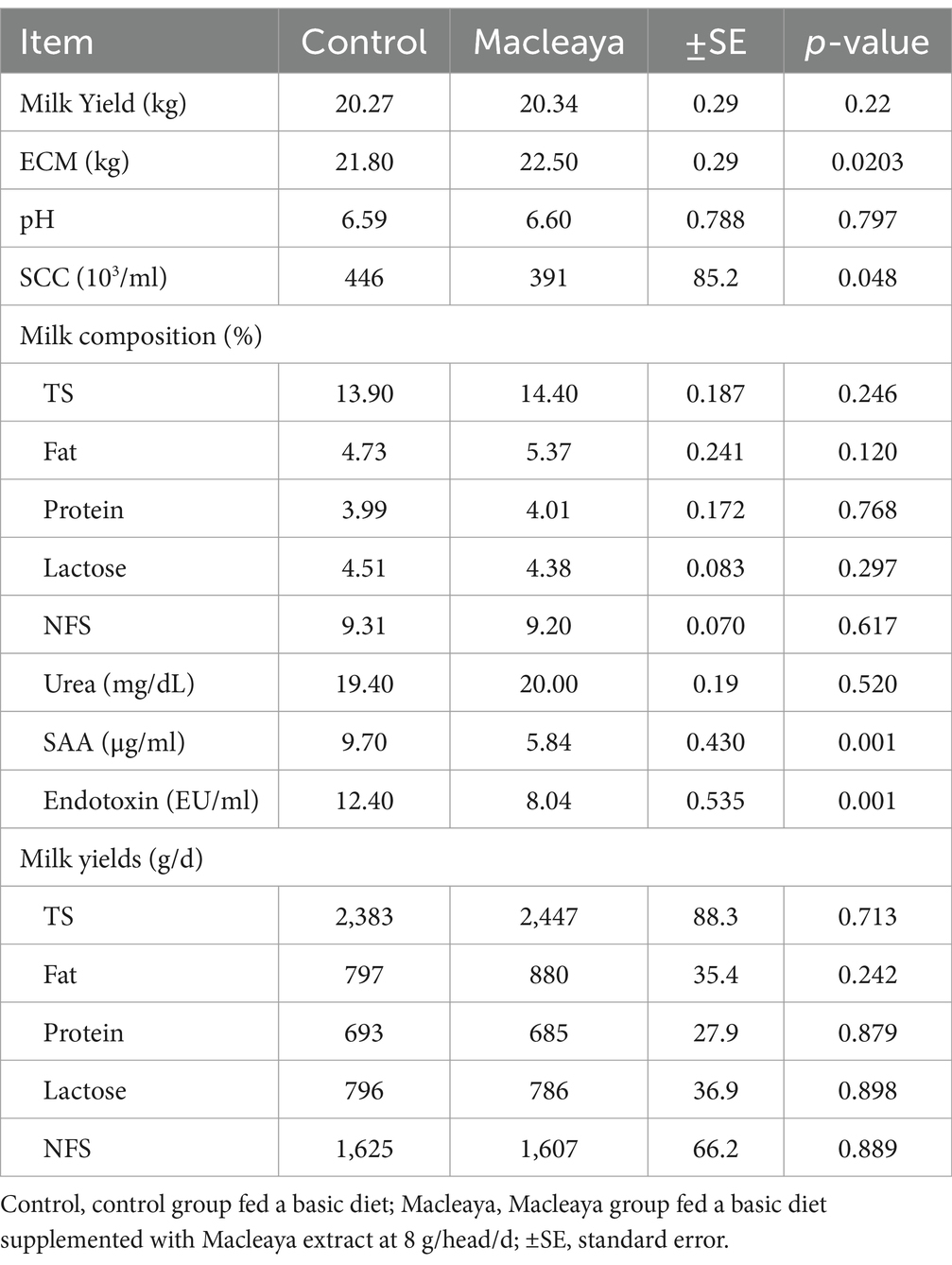
Table 7. Effect of supplementing the diets of lactating cows with Macleaya cordata on milk yield and composition.
Compared with the control diet, the diets supplemented with Macleaya significantly decreased the SCC (p = 0.048), SAA (p = 0.001) and endotoxin content (p = 0.001).
Discussion
The most expensive disease that affects dairy cattle is still mastitis. When the udder becomes inflamed and germs infiltrate the teat canal and mammary glands, dairy cows develop mastitis. These bacteria grow and release toxins, in addition to physical stress and chemical irritants, which harm milk-secreting tissue.
These factors lead to an increase in leukocytes, or somatic cells, in milk, which decreases its volume and has a negative effect on the quality of milk and its byproducts (5). Mastitis in dairy cattle can be decreased by monitoring somatic cell levels and quickly diagnosing and treating the condition.
As previously stated, the emergence of antibiotic-resistant pathogen strains has become a significant obstacle in antibiotic treatment, and if the use of antibiotics as the primary treatment strategy is still in place, its efficacy is restricted (24, 25). Moreover, the milk industry is being pressured to use fewer antimicrobial medications due to growing concerns about antibiotic resistance in public health issues. Consequently, it is necessary to look for alternatives to antibiotic therapy, particularly those originating from natural sources such as plants and animals (26–28). Researchers are becoming more interested in the use of plants to treat bovine mastitis since they are rich sources of chemicals for traditional medicine. The benefit of plant-derived chemicals over antibiotics is that they do not cause resistance, even after extended use. The low toxicity of chemicals originating from plants is another benefit (2).
Chinese people have long utilized plants of this genus for their diverse medicinal properties. Traditionally, the entire plant of Macleaya cordata has been used as a popular medicine to cure inflamed wounds and stings and to temporarily reduce muscular discomfort. M. cordata leaves are often used in traditional Chinese medicine to treat rheumatism, scabies, bruises, ulcers, and other skin conditions (29). Both Macleaya species are used primarily for the treatment of inflammation and certain skin conditions. Currently, Trichomonas vaginalis, rheumatoid arthritis, wounds, and other conditions are treated with M. cordata in traditional Chinese medicine (30). The anticancer, antiseptic, anti-inflammatory, and insecticidal properties of several extracts and isolated parts of the Macleaya species have been assessed (31). According to previous studies (32–35), quaternary benzo[c]phenanthridine alkaloids are the main bioactive substances. Numerous pathophysiological mechanisms that respond to tissue injury and the host’s defense against invasive microorganisms depend on inflammation. An increase in cytokines and nitric oxide production is indicative of inflammation. Numerous investigations have indicated that the Macleaya species has an anti-inflammatory effect (36). According to Vrba et al. (37), the anti-inflammatory properties of sanguinarine and chelerythrine may also be linked to their capacity to prevent the production of superoxide radicals by phagocyte NADPH oxidase. However, research on the direct effects of Macleaya extracts on mastitis in dairy cows is limited. These findings suggest that Macleaya has potential effects on oxidative stress control and that it has immune-boosting capabilities, antioxidant properties, antibacterial effects, and anti-inflammatory properties. However, the specific impact on mastitis remains to be fully explored.
The current study showed no noticeable effect of Macleaya cordata extract on rumen fermentation profile of cows. These findings might be due to the bioactive alkaloids in Macleaya cordata, such as sanguinarine and chelerythrine, which known for their antimicrobial and anti-inflammatory properties (38). While these compounds may affect certain microbial populations, they might not target the specific microbes involved in producing key fermentation metabolites (e.g., VFAs, NH3-N) (39). Also, the rumen microbiome is remarkably adaptable and robust.
Leucocyte settling is the mammary gland’s main defensive function during an inflammatory response. These typically have fewer than 200,000 cells per milliliter of milk. The cell count increases when mastitis is present, mostly due to leukocyte infiltration. Inflamed neutrophils and macrophages eliminate cellular aggregates and phagocytize ingested bacteria (40, 41). An aspect of nonspecific resistance, the acute phase response is characterized by a rapid rise in protein synthesis, which is known to be a hallmark of inflammation in animals (42, 43).
The findings of the present study revealed that the addition of macleaya extract to the diet decreased the IL-1β, IL-6 and IL-8 levels. A reduction in the IL-1β, IL-6 and IL-8 levels in cows fed Macleaya extract may indicate the resolution of inflammation or successful treatment for mastitis. Cows with naturally occurring and experimentally induced mastitis, as well as those injected with bacterial LPS, have been shown to have relatively high levels of IL-6 in their blood and milk (44, 45). The fact that IL-6 may stay in the bloodstream longer than other proinflammatory cytokines is a very significant benefit (46).
Both clinical and subclinical diseases may develop from mastitis. According to recent research, the host’s reaction during the early stages of illness, including the impacts of cytokines released, influences the consequences of infection (47–49). Small molecule proteins called cytokines are crucial for intercellular communication. Numerous processes, including cell differentiation, proliferation, remodeling, degeneration, regeneration, and even cell death, are either driven or hindered by cytokines. The SCC and levels of generated cytokines (interleukin (IL)-1, IL-2, IL-4, IL-5, IL-6, IL-8, IL-10, and IL-12) in milk increase in both naturally occurring and experimentally induced mastitis (50). One of the cytokine families that is most commonly represented is the IL-1 family (51). Through a number of signaling pathways, IL-1 family cytokines interact with immune competent tissue cells (52), which mostly carry out inflammatory, autoimmune, fracture-initiating, and mitogenic tasks. Interleukin-1 alpha (IL-1α) locally regulates first-line defense mediators in experimental acute bovine mastitis, including prostaglandin (PG-F2α) and leukotriene (LT-B4), indicating its participation in early inflammation (53). T and B lymphocytes, eosinophils, basophils, mast cells, plasma cells, and epithelial cells are the primary producers of IL-4 in the mammary glands of cows (54, 55). These cells also serve as the foundation for type 2 immunity (56). IL-1 has an indirect effect on immunity by interacting with other messenger molecules through a number of signaling pathways (52). Macrophages, lymphocytes, monocytes, endothelial cells, and fibroblasts in the mammary gland generate IL-1. Furthermore, IL-1 promotes the synthesis of TNF-α, IL-6, IL-8, and IL-12 in addition to its own (55).
Numerous experimental investigations have demonstrated the importance of inflammatory components in the initial inflammatory response. Upon identifying microbial components, bovine mammary epithelial cells can trigger an innate immune response through the release of proinflammatory cytokines (IL-1β, TNF-α, IL-6, and IL-8) and antimicrobial molecules (cathelicidin and defensins) (57, 58). The primary source of the proinflammatory cytokine tumor necrosis factor (TNF)-α is produced by macrophages. It may carry out a variety of tasks, including inducing inflammatory responses and encouraging the manufacture of other cytokines, regulating essential cell activities, and preserving tissue homeostasis, depending on where it is released and which receptor it binds to (59–61). The acute-phase cytokine TNF-α is generated in the early phases of infection (62, 63). The TNF-α concentration significantly decreased as the cows recovered (64). Consequently, the significance of TNF-α can extend beyond its proinflammatory function. However, with endotoxin infusion, the TNF-α concentration only slightly increased (65). TNF-α levels in serum are undetectable, but they increase in milk when the effects of intramammarily administered lipopolysaccharide on the acute-phase response in early and late lactation are examined (66).
Serum amyloid A, an apoprotein that is a component of high-density lipoprotein, is an acute phase protein (APP) (67). SAA functions by preventing platelet aggregation, phagocytosis, and the proliferation of lymphocytes and endothelial cells. Additionally, it promotes the production of prostaglandins and metalloproteinases as well as the migration of neutrophils and monocytes (68). Tumor necrosis factor-α, IL-1α, and IL-6 impact the transcription of SAA mRNAs (SAA1 and SAA2 isoforms), primarily in hepatocytes (69). Research on mastitis-affected cows has demonstrated that acute mammary infections cause a large (>200-fold) increase in SAA concentrations. In cows, SAA is thought to be the most sensitive APP, but it is not correlated with subclinical mastitis (70).
A variety of humoral and cellular immune response mechanisms interact through the transmission of stimulatory or inhibitory cytokine signals. An especially significant function is played by interleukin-6, which is thought to be the greatest stimulant of APP synthesis and secretion (46). Numerous cell types, including lymphocytes, monocytes, macrophages, endothelial cells, epithelial cells, and fibroblasts, express this cytokine, which is stimulated by bacteria. The concentrations of haptoglobin, ceruloplasmin, and transferrin are influenced by interleukin-6. Furthermore, it plays a role in T-cell antigen recognition activation, cytotoxic T-cell differentiation, and B-cell differentiation into distinct classes of Ig-releasing cells by acting as a stimulant (71).
Conclusion
The findings of the present study suggested that supplementing lactating cows infected with mastitis improved immune indices without any adverse effects on rumen fermentation, blood chemistry, antioxidant capacity, and milk yield or milk composition. Additionally, further experiments are urged to examine the impacts of different doses of these supplements on ruminal microbial diversity and animal productive performance.
Data availability statement
The original contributions presented in the study are included in the article/supplementary material, further inquiries can be directed to the corresponding authors.
Ethics statement
The animal studies were approved by the Laboratory Animal Welfare and Animal Experimental Ethical Inspection Committee, Institute of Subtropical Agriculture, the Chinese Academy of Sciences, Changsha, China. The studies were conducted in accordance with the local legislation and institutional requirements. Written informed consent was obtained from the owners for the participation of their animals in this study.
Author contributions
MK: Writing – original draft, Writing – review & editing. LH: Data curation, Formal analysis, Methodology, Writing – review & editing. TS: Conceptualization, Funding acquisition, Project administration, Writing – review & editing. YQ: Data curation, Formal analysis, Methodology, Writing – review & editing. LY: Data curation, Investigation, Writing – review & editing. TZ: Funding acquisition, Project administration, Writing – review & editing. LQ: Formal analysis, Methodology, Writing – review & editing.
Funding
The author(s) declare that financial support was received for the research and/or publication of this article. This research was funded by grants from the Ministry of Science and Technology of China (2023YFD1301701), the Chinese Academy of Sciences President’s International Fellowship Initiative (2024PVA0033), and the Technical System of the Herbivore Industry in Hunan Province.
Conflict of interest
The authors declare that the research was conducted in the absence of any commercial or financial relationships that could be construed as a potential conflict of interest.
Generative AI statement
The authors declare that no Gen AI was used in the creation of this manuscript.
Publisher’s note
All claims expressed in this article are solely those of the authors and do not necessarily represent those of their affiliated organizations, or those of the publisher, the editors and the reviewers. Any product that may be evaluated in this article, or claim that may be made by its manufacturer, is not guaranteed or endorsed by the publisher.
References
1. Gomes, F, and Henriques, M. Control of bovine mastitis: old and recent therapeutic approaches. Curr Microbiol. (2016) 72:377–82. doi: 10.1007/s00284-015-0958-8
2. Cheng, WN, and Han, SG. Bovine mastitis: risk factors, therapeutic strategies, and alternative treatments — a review. Asian Australas J Anim Sci. (2020) 33:1699–713. doi: 10.5713/ajas.20.0156
3. Hogeveen, H, Steeneveld, W, and Wolf, CA. Production diseases reduce the efficiency of dairy production: a review of the results, methods, and approaches regarding the economics of mastitis. Ann Rev Resour Econ. (2019) 11:289–312. doi: 10.1146/annurev-resource-100518-093954
4. Zhao, X, and Lacasse, P. Mammary tissue damage during bovine mastitis: causes and control1. J Anim Sci. (2008) 86:57–65.
5. Khan, MZ, and Khan, A. Basic facts of mastitis in dairy animals: a review. Pak Vet J. (2006) 26:204–8.
6. Chen, J, Liu, Z, Liu, Y, Zhang, X, and Zeng, J. Preliminary investigations on the pathogenesis-related protein expression profile of the medicinal herb Macleaya cordata and anti-bacterial properties of recombinant proteins. Phytochemistry. (2021) 184:112667. doi: 10.1016/j.phytochem.2021.112667
7. Arora, D, and Sharma, A. A review on phytochemical and pharmacological potential of genus Chelidonium. Pharm J. (2013) 5:184–90. doi: 10.1016/j.phcgj.2013.07.006
8. Abbaspour, H, Saeidi-Sar, S, Afshari, H, and Abdel-Wahhab, MA. Tolerance of mycorrhiza infected pistachio (Pistacia vera L.) seedling to drought stress under glasshouse conditions. J Plant Physiol. (2012) 169:704–9. doi: 10.1016/j.jplph.2012.01.014
9. Sun, M, Liu, C, Nadiminty, N, Lou, W, Zhu, Y, Yang, J, et al. Inhibition of Stat3 activation by Sanguinarine suppresses prostate Cancer cell growth and invasion. Prostate. (2014) 72:82–9. doi: 10.1002/pros.21409
10. Bavarsadi, M, Mahdavi, AH, Ansari-Mahyari, S, and Jahanian, E. Effects of different levels of sanguinarine on antioxidant indices, immunological responses, ileal microbial counts and jejunal morphology of laying hens fed diets with different levels of crude protein. J Anim Physiol Anim Nutr. (2017) 101:936–48. doi: 10.1111/jpn.12528
11. Guo, S, Lei, J, Liu, L, Qu, X, Li, P, Liu, X, et al. Effects of Macleaya cordata extract on laying performance, egg quality, and serum indices in Xuefeng black-bone chicken. Poult Sci. (2021) 100:101031. doi: 10.1016/j.psj.2021.101031
12. Kantas, D, Papatsiros, VG, Tassis, PD, Athanasiou, LV, and Tzika, ED. The effect of a natural feed additive (Macleaya cordata), containing sanguinarine, on the performance and health status of weaning pigs. Anim Sci J. (2015) 86:92–8. doi: 10.1111/asj.12240
13. Chen, J, Kang, B, Yao, K, Fu, C, and Zhao, Y. Effects of dietary Macleaya cordata extract on growth performance, immune responses, antioxidant capacity, and intestinal development in weaned piglets. J Appl Anim Res. (2019) 47:349–56. doi: 10.1080/09712119.2019.1636800
14. Yang, C, Cheng, Y, Li, X, Li, H, Yan, Q, He, Z, et al. Effects of dietary Macleaya cordata extract inclusion on transcriptomes and inflammatory response in the lower gut of early weaned goats. Anim Feed Sci Technol. (2021) 272:114792. doi: 10.1016/j.anifeedsci.2020.114792
15. Jiao, P, Hu, G, Liang, G, Chen, M, An, N, Wang, Z, et al. Dietary supplementation with Macleaya cordata extract inclusion affects growth performance, rumen fermentation, bacterial communities, and immune responses of weaned lambs. Anim Feed Sci Technol. (2021) 282:115127–9. doi: 10.1016/j.anifeedsci.2021.115127
16. NRC. Nutrient requirements of dairy cattle: 2001. Washington, D.C.: National Academies Press (2001).
17. Wang, Z, Zhao, Y, Lan, X, He, J, Wan, F, Shen, W, et al. Tannic acid supplementation in the diet of Holstein bulls: impacts on production performance, physiological and immunological characteristics, and ruminal microbiota. Front Nutr. (2022) 9:1–15. doi: 10.3389/fnut.2022.1066074
18. AOAC. Official methods of analysis of AOAC International. AOAC (2016). 3172. Available online at: https://www.techstreet.com/standards/official-methods-of-analysis-of-aoac-international-20th-edition-2016?product_id=1937367 (Accessed June 07, 2023)
19. Van Soest, PJ, Robertson, JB, and Lewis, BA. Methods for dietary Fiber, neutral detergent Fiber, and nonstarch polysaccharides in relation to animal nutrition. J Dairy Sci. (1991) 74:3583–97. doi: 10.3168/jds.S0022-0302(91)78551-2
20. Wu, Z, Yang, Y, Wang, B, Gebeyew, K, Tang, S, Han, X, et al. Blood metabolites and Faecal microbial communities in nonpregnant and early gestation ewes in highly cold areas. Biology. (2023) 12:1–16. doi: 10.3390/biology12111436
21. Khattab, MSA, Matloup, OH, Hassan, AA, El-Hendawy, NM, El-Zaiat, HM, and Sallam, SMA. Lactating buffalos’ productive performance, ruminal kinetics, nutrients digestibility and oxidative status as response to supplementing diets with alpinia galanga. Anim Biotechnol. (2022) 34:3363–70. doi: 10.1080/10495398.2022.2146588
22. Sjaunja, L, Baevre, L, Junkkarinen, L, and Pedersen, J. A Nordic proposal for an energy Correctec Milk (ECM) formula. In: Proceedings of the 27th Bienal Session of the International Committee for Animal Recording (ICAR) (1990)156–157.
23. Murphy, MR, Baldwin, RL, and Koong, LJ. Estimation of stoichiometric parameters for rumen fermentation of roughage and concentrate diets. J Anim Sci. (1982) 55:411–21. doi: 10.2527/jas1982.552411x
24. Fair, RJ, and Tor, Y. Antibiotics and bacterial resistance in the 21st century. Perspect Med Chem. (2014) 6:25–64. doi: 10.4137/PMC.S14459
25. Suriyasathaporn, W, Chupia, V, Sing-Lah, T, Wongsawan, K, Mektrirat, R, and Chaisri, W. Increases of antibiotic resistance in excessive use of antibiotics in smallholder dairy farms in northern Thailand. Asian Australas J Anim Sci. (2012) 25:1322–8. doi: 10.5713/ajas.2012.12023
26. Yang, WT, Ke, CY, Wu, WT, Lee, RP, and Tseng, YH. Effective treatment of bovine mastitis with intramammary infusion of angelica dahurica and rheum officinale extracts. Evid Based Complement Alternat Med. (2019) 2019:705. doi: 10.1155/2019/7242705
27. Anantasook, N, Wanapat, M, Cherdthong, A, and Gunun, P. Effect of plants containing secondary compounds with palm oil on feed intake, digestibility, microbial protein synthesis and microbial population in dairy cows. Asian Australas J Anim Sci. (2013) 26:820–6. doi: 10.5713/ajas.2012.12689
28. Heikkilä, AM, Liski, E, Pyörälä, S, and Taponen, S. Pathogen-specific production losses in bovine mastitis. J Dairy Sci. (2018) 101:9493–504. doi: 10.3168/jds.2018-14824
29. Lin, L, Liu, YC, Huang, JL, Bin, LX, Qing, ZX, Zeng, JG, et al. Medicinal plants of the genus Macleaya (Macleaya cordata, Macleaya microcarpa): a review of their phytochemistry, pharmacology, and toxicology. Phytother Res. (2018) 32:19–48. doi: 10.1002/ptr.5952
30. Zhou, JX, and Xie, GYX. Encyclopedia of traditional Chinese medicines. Isolat Compound AB. (2011) 1:455. doi: 10.1007/978-3-642-16735-5_1
31. Malikova, J, Zdarilova, A, and Hlobilkova, A. Effects of sanguinarine and chelerythrine on the cell cycle and apoptosis. Biomed Pap Med Fac Univ Palacký Olomouc Czech Repub. (2006) 150:5–12. doi: 10.5507/bp.2006.001
32. Juskiewicz, J, Gruzauskas, R, Zdunczyk, Z, Semaskaite, A, Jankowski, J, Totilas, Z, et al. Effects of dietary addition of Macleaya cordata alkaloid extract on growth performance, caecal indices and breast meat fatty acids profile in male broilers. J Anim Physiol Anim Nutr. (2011) 95:171–8. doi: 10.1111/j.1439-0396.2010.01037.x
33. Kosina, P, Gregorova, J, Gruz, J, Vacek, J, Kolar, M, Vogel, M, et al. Phytochemical and antimicrobial characterization of Macleaya cordata herb. Fitoterapia. (2010) 81:1006–12. doi: 10.1016/j.fitote.2010.06.020
34. Psotova, J, Vecera, R, Zdarilova, A, Anzenbacherova, E, Kosina, P, Svobodova, A, et al. Safety assessment of sanguiritrin, alkaloid fraction of Macleaya cordata, in rats. Vet Med. (2006) 51:145–55. doi: 10.17221/5534-VETMED
35. Zdarilova, A, Vrublova, E, Vostalova, J, Klejdus, B, Stejskal, D, Proskova, J, et al. Natural feed additive of Macleaya cordata: safety assessment in rats a 90-day feeding experiment. Food Chem Toxicol. (2008) 46:3721–6. doi: 10.1016/j.fct.2008.09.054
36. Park, H, Bergeron, E, Senta, H, Guillemette, K, Beauvais, S, Blouin, R, et al. Sanguinarine induces apoptosis of human osteosarcoma cells through the extrinsic and intrinsic pathways. Biochem Biophys Res Commun. (2010) 399:446–51. doi: 10.1016/j.bbrc.2010.07.114
37. Vrba, J, Dvořák, Z, Ulrichová, J, and Modrianský, M. Conventional protein kinase C isoenzymes undergo dephosphorylation in neutrophil-like HL-60 cells treated by chelerythrine or sanguinarine. Cell Biol Toxicol. (2008) 24:39–53. doi: 10.1007/s10565-007-9014-1
38. Liu, Z, Wang, W, Zhang, Z, Sun, L, and Wu, S. Natural antibacterial and Antivirulence alkaloids from Macleaya cordata against methicillin-resistant Staphylococcus aureus. Front Pharmacol. (2022) 13:1–11. doi: 10.3389/fphar.2022.813172
39. Jia, P, Dong, L, Feng, TY, and Yu, DQ. Bacillus subtilis and Macleaya cordata extract regulate the rumen microbiota associated with enteric methane emission in dairy cows. Microbiome. (2023) 11:229. doi: 10.1186/s40168-023-01654-3
40. Niemialtowski, M, Nonnecke, BJ, and Targowski, SP. Phagocytic activity of Milk leukocytes during chronic staphylococcal mastitis. J Dairy Sci. (1988) 71:780–7. doi: 10.3168/jds.S0022-0302(88)79618-6
41. Paape, MJ, Bannerman, DD, Zhao, X, and Lee, J-W. The bovine neutrophil: structure and function in blood and milk. Vet Res. (2003) 34:597–627. doi: 10.1051/vetres:2003024
42. Eckersall, PD, Young, FJ, McComb, C, Hogarth, CJ, Safi, S, Weber, A, et al. Acute phase proteins in serum and milk from dairy cows with clinical mastitis. Vet Rec. (2001) 148:35–41. doi: 10.1136/vr.148.2.35
43. Murata, H, Shimada, N, and Yoshioka, M. Current research on acute phase proteins in veterinary diagnosis: An overview. Vet J. (2004) 168:28–40. doi: 10.1016/S1090-0233(03)00119-9
44. Shuster, DE, Lee, EK, and Kehrli, MEJ. Bacterial growth, inflammatory cytokine production, and neutrophil recruitment during coliform mastitis in cows within ten days after calving, compared with cows at midlactation. Am J Vet Res. (1996) 57:1569–75. doi: 10.2460/ajvr.1996.57.11.1569
45. Hagiwara, K, Yamanaka, H, Hisaeda, K, Taharaguchi, S, Kirisawa, R, and Iwai, H. Concentrations of IL-6 in serum and whey from healthy and mastitic cows. Vet Res Commun. (2001) 25:99–108. doi: 10.1023/a:1006400801305
46. Song, M, and Kellum, JA. Interleukin-6. Crit Care Med. (2005) 33:S463–5. doi: 10.1097/01.CCM.0000186784.62662.A1
47. Alluwaimi, AM. The cytokines of bovine mammary gland: prospects for diagnosis and therapy. Res Vet Sci. (2004) 77:211–22. doi: 10.1016/j.rvsc.2004.04.006
48. Shah, KN, Valand, P, Nauriyal, DS, and Joshi, CG. Immunomodulation of IL-1, IL-6 and IL-8 cytokines by Prosopis juliflora alkaloids during bovine sub-clinical mastitis. 3. Biotech. (2018) 8:409. doi: 10.1007/s13205-018-1438-1
49. Murphy, MP, Niedziela, DA, Leonard, FC, and Keane, OM. The in vitro host cell immune response to bovine-adapted Staphylococcus aureus varies according to bacterial lineage. Sci Rep. (2019) 9:6134–13. doi: 10.1038/s41598-019-42424-2
50. Bannerman, DD. Pathogen-dependent induction of cytokines and other soluble inflammatory mediators during intramammary infection of dairy cows1. J Anim Sci. (2009) 87:10–25. doi: 10.2527/jas.2008-1187
51. Garlanda, C, Dinarello, CA, and Mantovani, A. The Interleukin-1 family: Back to the future. Immunity. (2013) 39:1003–18. doi: 10.1016/j.immuni.2013.11.010
52. Dinarello, CA. Immunological and inflammatory functions of the Interleukin-1 family. Annu Rev Immunol. (2009) 27:519–50. doi: 10.1146/annurev.immunol.021908.132612
53. Piotrowska-Tomala, KK, Siemieniuch, MJ, Szóstek, AZ, Korzekwa, AJ, Woclawek-Potocka, I, Galváo, AM, et al. Lipopolysaccharides, cytokines, and nitric oxide affect secretion of prostaglandins and leukotrienes by bovine mammary gland epithelial cells. Domest Anim Endocrinol. (2012) 43:278–88. doi: 10.1016/j.domaniend.2012.04.005
54. Karo-Atar, D, Bitton, A, Benhar, I, and Munitz, A. Therapeutic targeting of the Interleukin-4/Interleukin-13 signaling pathway: in Allergy and Beyond. BioDrugs. (2018) 32:201–20. doi: 10.1007/s40259-018-0280-7
55. Ezzat Alnakip, M, Quintela-Baluja, M, Böhme, K, Fernández-No, I, Caamaño-Antelo, S, Calo-Mata, P, et al. The immunology of mammary gland of dairy ruminants between healthy and inflammatory conditions. J Vet Med. (2014) 2014:1–31. doi: 10.1155/2014/659801
56. Heeb, LEM, Egholm, C, Impellizzieri, D, Ridder, F, and Boyman, O. Regulation of neutrophils in type 2 immune responses. Curr Opin Immunol. (2018) 54:115–22. doi: 10.1016/j.coi.2018.06.009
57. Boudjellab, N, Chan-Tang, HS, and Zhao, X. Bovine interleukin-1 expression by cultured mammary epithelial cells (MAC-T) and its involvement in the release of MAC-T derived interleukin-8. Comp Biochem Physiol A Mol Integr Physiol. (2000) 127:191–9. doi: 10.1016/S1095-6433(00)00257-9
58. Bronzo, V, Lopreiato, V, Riva, F, Amadori, M, Curone, G, Addis, MF, et al. The role of innate immune response and microbiome in resilience of dairy cattle to disease: the mastitis model. Animals. (2020) 10:1–20. doi: 10.3390/ani10081397
59. Kalliolias, GD, and Ivashkiv, LB. TNF biology, pathogenic mechanisms and emerging therapeutic strategies. Nat Rev Rheumatol. (2016) 12:49–62. doi: 10.1038/nrrheum.2015.169
60. Ahmad, S, Azid, NA, Boer, JC, Lim, J, Chen, X, Plebanski, M, et al. The key role of TNF-TNFR2 interactions in the modulation of allergic inflammation: a review. Front Immunol. (2018) 9:572. doi: 10.3389/fimmu.2018.02572
61. Holbrook, J, Lara-Reyna, S, Jarosz-Griffiths, H, and McDermott, M. Tumour necrosis factor signalling in health and disease. F1000Res. (2019) 8:111. doi: 10.12688/f1000research.17023.1
62. Sordillo, LM, Shafer-Weaver, K, and DeRosa, D. Immunobiology of the mammary gland. J Dairy Sci. (1997) 80:1851–65. doi: 10.3168/jds.S0022-0302(97)76121-6
63. Rainard, P, and Paape, MJ. Sensitization of the bovine mammary gland to Escherichia coli endotoxin. Vet Res. (1997) 28:231–8.
64. Hisaeda, K, Hagiwara, K, Eguchi, J, Yamanaka, H, Kirisawa, R, and Iwai, H. Interferon-γ and tumor necrosis factor-α levels in sera and whey of cattle with naturally occurring coliform mastitis. J Vet Med Sci. (2001) 63:1009–11. doi: 10.1292/jvms.63.1009
65. Perkins, KH, VandeHaar, MJ, Burton, JL, Liesman, JS, Erskine, RJ, and Elsasser, TH. Clinical responses to intramammary endotoxin infusion in dairy cows subjected to feed restriction. J Dairy Sci. (2002) 85:1724–31. doi: 10.3168/jds.S0022-0302(02)74246-X
66. Vitenberga-verza, Z, Pilmane, M, Serstnova, K, Melderis, I, Gontar, Ł, Kochariski, M, et al. Identification of inflammatory and regulatory cytokines IFN-γ-producing cells in the Milk of dairy cows with. Pathogens. (2022) 11:372. doi: 10.3390/pathogens11030372
67. Eklund, KK, Niemi, K, and Kovanen, PT. Immune functions of serum amyloid a. Crit Rev Immunol. (2012) 32:335–48. doi: 10.1615/critrevimmunol.v32.i4.40
68. Hagbard, H, Petersen, JPN, and Heegaard, PMH. Application of acute phase protein measurements in veterinary clinical chemistry. Vet Res. (2004) 35:163–87. doi: 10.1051/vetres
69. Bochniarz, M, Szczubiał, M, Brodzki, P, Krakowski, L, and Dąbrowski, R. Serum amyloid a as an marker of cow’s mastitis caused by Streptococcus sp. Comp Immunol Microbiol Infect Dis. (2020) 72:101498. doi: 10.1016/j.cimid.2020.101498
70. Miglio, A, Moscati, L, Fruganti, G, Pela, M, Scoccia, E, Valiani, A, et al. Use of milk amyloid a in the diagnosis of subclinical mastitis in dairy ewes. J Dairy Res. (2013) 80:496–502. doi: 10.1017/S0022029913000484
71. Bochniarz, M, Zdzisińska, B, Wawron, W, Szczubiał, M, and Dąbrowski, R. Milk and serum IL-4, IL-6, IL-10, and amyloid a concentrations in cows with subclinical mastitis caused by coagulase-negative staphylococci. J Diary Sci. (2017) 100:9674–80. doi: 10.3168/jds.2017-13552
Glossary
ALB - Albumin
ALP - Alkaline phosphatase
ALT - Alanine transaminase
AMS - Amylase
AST - Aspartate transaminase
Bas - Basophilic granulocyte
BUN - Blood urea nitrogen
CHE - Cholinesterase
CHOL - Total cholesterol
CRE - Creatinine
DBIL - Direct bilirubin
ECM - Energy corrected milk
Eos - Eosinophils
GGT - Gamma glutamyl transpeptidase
GLU - Glucose
GPx - Glutathione peroxidase
HCT - Hematocrit
HDL-c - High-density lipoprotein cholesterol
HGB - Hemoglobin
IgA - Immunoglobulin A
IgG - Immunoglobulin G
IgM - Immunoglobulin M
IL-1β - Interleukins 1β
IL-2 - Interleukin 2
IL-6 - Interleukin 6
IL-8 - Interleukin 8
LAC - D-lactic acid
LDH - Lactate ehydrogenase
LDL-c - Low-density lipoprotein cholesterol
Lym - Lymphocytes
MC - Macleaya cordata
MCE - Macleaya cordata extract
MCH - Mean corpuscular-hemoglobin
MCHC - Mean corpuscular-hemoglobin concentration
MCV - Mean corpuscular volume
MDA - Malondialdehyde
Mon - Monocytes
MPV - Mean platelet volume
Neu - Neutrophils
NFS - Milk nonfat solid
PCT - Thrombocytocrit
PDW - Platelet distribution width
PLT - Platelet count
RBC - Red blood cell
RDW-CV - Red blood cell distribution width variable coefficient
RDW-SD - Red blood cell distribution width standard deviation
SCC - Somatic cell count
SOD - Superoxide dismutase
TAC - Total antioxidant capacity
TBIL - Total bilirubin
TG - Triglycerides
TNF-α - Tumor necrosis factor
TP - Plasma total protein
TS - Milk total solids
WBC - White blood cell
Keywords: antioxidant capacity, immune indices, Macleaya cordata, mastitis, milk yield
Citation: Khattab MSA, Haiqiang L, Shaoxun T, Qiongxian Y, Yong L, Zhiliang T and Qi L (2025) Immunological response enhancement in cows with subclinical mastitis fed diet supplemented with Macleaya cordata. Front. Vet. Sci. 12:1503487. doi: 10.3389/fvets.2025.1503487
Edited by:
Sadarman Sadarman, State Islamic University of Sultan Syarif Kasim Riau, IndonesiaReviewed by:
Moyosore Joseph Adegbeye, University of Africa, NigeriaAmirul Faiz Mohd Azmi, Universiti Malaysia Kelantan, Malaysia
Copyright © 2025 Khattab, Haiqiang, Shaoxun, Qiongxian, Yong, Zhiliang and Qi. This is an open-access article distributed under the terms of the Creative Commons Attribution License (CC BY). The use, distribution or reproduction in other forums is permitted, provided the original author(s) and the copyright owner(s) are credited and that the original publication in this journal is cited, in accordance with accepted academic practice. No use, distribution or reproduction is permitted which does not comply with these terms.
*Correspondence: Mostafa S. A. Khattab, bXNha2hhdHRhYkBnbWFpbC5jb20=; bXMua2hhdHRhYkBucmMuc2NpLmVn; Tang Shaoxun, c3h0YW5nQGlzYS5hYy5jbg==; Lu Qi, bHVxaTI1NTY3MjhAMTYzLmNvbQ==
†ORCID: Mostafa S. A. Khattab, https://orcid.org/0000-0002-4688-4580
Tang Shaoxun, https://orcid.org/0000-0001-9343-1341
Yan Qiongxian, https://orcid.org/0000-0003-0035-6819
Zhiliang Tan, https://orcid.org/0000-0002-7483-1478