- 1Tropical Crops Genetic Resources Research Institute, Chinese Academy of Tropical Agricultural Sciences, Haikou, China
- 2Hainan Xuhuai Technology Co. Ltd., Haikou, China
The milk provided by sows plays an essential role in the growth and development of the piglets. This study aimed to explore the effects of Angelica sinensis extracts on milk composition, immune function, milk-derived hormones, and related gene expression of lactating sows. Forty-eight sows were randomly allocated into four groups, with six replicates and two sows per replicate. The control group (CON) was fed basal experimental diets, and the experimental groups were fed 400, 600, and 800 mg/kg Angelica sinensis extracts in powder (AS1, AS2, and AS3, respectively). Compared to the CON, (1) the lactoprotein and milk fat contents were higher (p < 0.05) in AS1, AS2, and AS3 at 12 and 24 h, and on the 7th day; (2) the IgA, IgG, and IL-2 levels were higher (p < 0.05), and the TNF-α levels were lower (p < 0.05) in AS1, AS2, and AS3 at 12 and 24 h; the IgG, IgM, and IL-2 levels were higher (p < 0.05) in AS1, AS2, and AS3 on the 7th day; (3) the progesterone, prolactin, growth hormone, and insulin-like growth factor-1 contents were higher (p < 0.05) in AS1, AS2, and AS3 at 12 and 24 h; the growth hormone and insulin-like growth factor-1 contents were higher (p < 0.05) in AS2 and AS3 on the 7th day; (4) the relative expressions of PRLP, LALBA, AKT1, FASN, GLUT1, and CSN2 in mammary tissue were higher (p < 0.05) in groups treated with Angelica sinensis extracts; (5) the serum IgA, IgM, IL-6, growth hormone, insulin, and insulin-like growth factor-1 contents were higher (p < 0.05) in AS2 and AS3 in piglets; and (6) the relative expressions of PRLP and IL-10 in umbilical cord were higher (p < 0.05) in groups treated with Angelica sinensis extracts. Incorporating 600 mg/kg of Angelica sinensis extracts into the diets of lactating Wuzhishan sows elicited differential responses regarding milk composition, immune function, hormonal profiles, and associated gene expression across distinct postpartum periods.
1 Introduction
The quality of the sow’s milk largely determines the growth and survival rates of the piglets it feeds (1, 2). The immunoglobulin fractions present in colostrum, particularly secretory IgA and IgG subclasses, confer passive humoral immunity to neonatal swine through pathogen-neutralizing mechanisms. At the same time, the multifaceted bioactive components of mature lactation, including oligosaccharides, lactoferrin, and growth factors, orchestrate gastrointestinal ontogeny by enhancing intestinal barrier function, modulating immune homeostasis, and facilitating the establishment of commensal microbiota colonization resistance (3–5). In addition, colostrum is rich in lactose and lipids, which provide newborn piglets with the necessary energy to maintain their thermal homeostasis (5). Periparturient dietary supplementation with immunonutritional agents, including conjugated linoleic acid (CLA), marine-derived omega-3 polyunsaturated fatty acids (n-3 PUFAs), phytogenic bioactive compounds, probiotic microbial consortia, and yeast-derived mannoprotein complexes, has been shown to epigenetically modulate lactogenic programming in sows, resulting in quantifiable enhancements of mammary secretory output through increased immunoglobulin titers, optimized fatty acid profiles, and amplified synthesis of antimicrobial factors in colostrum and transitional milk (6, 7).
Angelica sinensis is a commonly used Chinese herbal medicine, known for its effects in tonifying and activating blood, regulating menstruation, relieving pain, enhancing immunity, reducing inflammation, providing analgesic effects, acting as a laxative, and promoting digestion (8, 9). Zhao et al. found that Angelica sinensis improved placental structure, attenuated inflammatory response, and modulated placental angiogenesis and growth factor receptors in sows (10). Wu et al. reported that Angelica sinensis improved the intestinal floras and short-chain fatty acids of cecal contents in laying chickens (11). Tian et al. found that Angelica sinensis polysaccharide attenuated LPS-induced inflammation response of primary dairy cow claw dermal cells via nuclear factor kappa-B (NF-κB) and mitogen-activated protein kinase (MAPK) signaling pathways (12). Duan et al. found that Chinese herbal complexes containing Angelica sinensis added to the periparturient sow diet significantly augmented antioxidant capacity in both sows and offspring piglets (13). Currently, there are relatively few studies related to the study of Angelica sinensis on milk composition and immune and hormonal indicators in milk of local sows. This study posits that dietary supplementation with Angelica sinensis extracts enhances lactogenic performance in Wuzhishan sows through dose-dependent modulation of milk composition, immune function, milk-derived hormones, and related gene expression.
The Wuzhishan pig is a small breed native to the Wuzhishan region of Hainan Province in China (14). It is characterized by a compact, firm body, a small and slightly long head, a straight and long snout, a pointed mouth, and a prominent muzzle protrusion. The pig also has small, thin ears, a peach-shaped appearance close to the neck, and a rat-like head (15, 16). The objective of this study was to (a) explore the effects of Angelica sinensis extracts on the milk composition, immune function, milk-derived hormones, and related gene expression of lactating sows; (b) conduct a preliminary investigation of the action mechanism of Angelica sinensis extracts on the lactation ability of sows; and (c) explore the valuable addition of Angelica sinensis extracts in the feed of gestating and lactating sows.
2 Materials and methods
2.1 Experiment material
Gestating and lactating Wuzhishan sows, with an average body weight of 33.00 ± 2.00 kg, were primiparous, had the same genetic background, and shared similar birthing date.
Angelica sinensis (AS) extracts were purchased from Shaanxi Baichuan Biotechnology Co., Ltd. The active ingredient contents of the AS extracts were tested by the liquid chromatograph and mass spectrometer. The Angelica sinensis polysaccharide contents of AS extracts were 12.45%, and the ferulic acid content was 0.06%.
Milk immunoglobulin and serum biochemical kits were purchased from Shanghai Sangon Biotechnology Co., Ltd., Shanghai, China.
2.2 Experiment design and sample collection
The added amount of Angelica sinensis extract was a single influencing factor in this study. Forty-eight Wuzhishan sows were randomly allocated into four groups, with six replicates and two sows per replicate. The basal experimental diets for gestating and lactating were corn-soybean meal-type diets formulated according to the NCR (2012) standards. The composition of the basal experimental diets and nutritional levels is shown in Table 1. The control group (CON) was fed basal experimental diets, and the experimental groups were fed 400, 600, and 800 mg/kg Angelica sinensis extracts in powder form (AS1, AS2, and AS3, respectively) according to recommended usage. The experiment started on day 107 of gestation and ended on day 7 after delivery. Sows were guaranteed free access to feed and water during the trial.
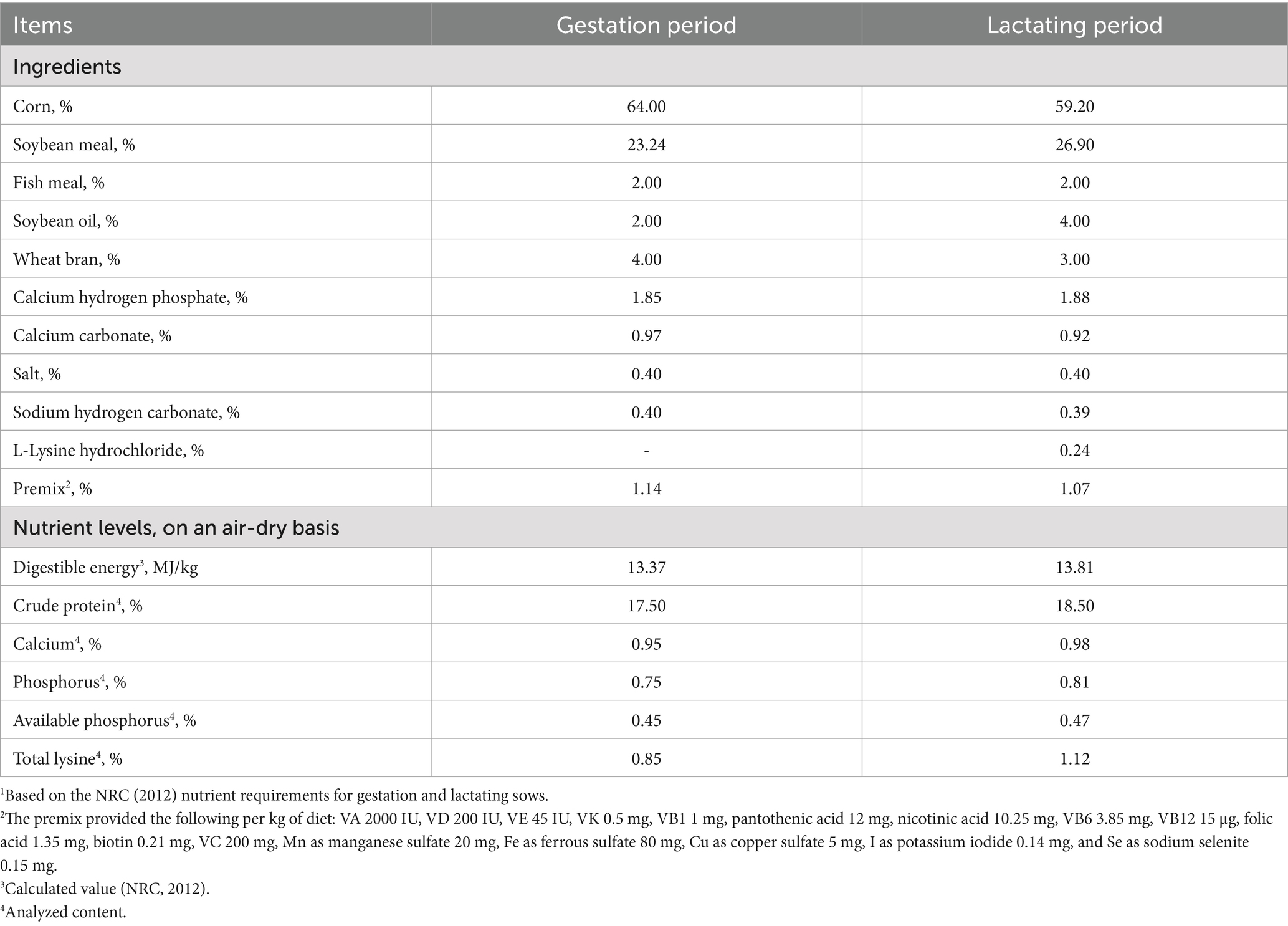
Table 1. Composition (kg/100 kg) of the basal experimental diets1 for gestating and lactating sows.
Approximately 30 ml of colostrum were collected from the front, middle, and rear nipples of the sows in the 0, 12, and 24 h and on the 7th day after the farrowing ended and then mixed well and stored at –20°C until further testing. Four milliliters of lidocaine hydrochloride were injected into the third left papilla of the sows for local anesthesia. A total of 2–4 g of mammary tissue samples were collected in a 2 ml cryopreservative tube using a live sampling gun (BARD® MAGNUM®, MG1522, USA) and disposable sampling needles (C.R. Bard. Inc., Covington, GA, USA) after the farrowing ended, and approximately 2 cm of the umbilical cord was collected approximately 6–8 cm from the piglet’s abdomen. The mammary and umbilical cord tissue samples were stored at −80°C for further use. After birth, two piglets per replicate were randomly selected for approximately 3 ml of marginal ear vein blood collection. After resting the blood for 15 min, it was centrifuged at 3000 rpm for 15 min to obtain the serum, which was divided into Eppendorf tubes and stored at −20°C for testing.
2.3 Milk components
The lactoprotein content, lactose, milk fat, and solids-not-fat in milk were quantified via Fourier-transform near-infrared spectroscopy using a commercially calibrated Milk-Scan 134 A/B system (Beijing LiO Biotechnology Co., China) with wavelength calibration against bovine milk reference standards (ISO 9622:2013). Pre-analytical processing involved refrigerated differential centrifugation (3,000 × g, 20 min, 4°C) to remove cellular debris and lipid stratification prior to spectral acquisition in triplicate (λ = 850–1,050 nm, resolution 8 cm−1).
2.4 Immune function and milk-derived hormone levels
Serum immunophenotypic parameters (IgA, IgG, and IgM), pro−/anti-inflammatory cytokines (IL-2, IL-6, and TNF-α), and metabolic hormones, including growth hormone (GH), insulin, and insulin-like growth factor-1 (IGF-1), were quantified via standardized enzyme-linked immunosorbent assays (ELISAs) using commercially available kits (Shanghai Sangon Biotechnology Co., Ltd., China), with all procedures conducted in triplicate under quality-controlled conditions (inter-assay CV < 8.0%, intra-assay CV < 5.0%) according to ISO 17025-certified manufacturer protocols (Cat. Nos. SS-ELISA-001 to 009).
2.5 RNA extraction and quantitative analysis of mRNA with real-time PCR
The mammary and umbilical cord tissue samples of soybean size were taken, and RNA was extracted according to the instructions of the animal tissue RNA extraction kit. The quality of RNA was assessed by a 2% agarose gel electrophoresis. An ultra-microspectrophotometer (NanoPhotometer, Implen, Germany) was used to evaluate the total RNA concentration and purity (A260/A280 ratio). The primer sequences are shown in Table 2, and the primers corresponding to the sow gene sequence were synthesized using Sangon (Shanghai, China). The cDNA was synthesized using the PrimeScript® RT regent kit with the gDNA Eraser (TaKaRa, Dalian, China) reverse transcription kit. DNA was removed from the sample using a reaction system as 2-μl 5 × gDNA Eraser Buffer, 1 μl gDNA Eraser, and 1 μg RNA and then replenished the volume to 10 μl of RNase Free ddH2O (1). The system of reverse transcription was 10 μl reaction liquid, 1 μl RT Primer Mix, 4 μl RNase-free ddH2O, 4-μl 5 × PrimeScript® Buffer 2, and PrimeScript® RT Enzyme MixI. The reaction procedure was maintained at 37°C constant temperature for 15 min, 85°C constant temperature for 5 s, and 4°C stored briefly. The obtained cDNA was stored at −20°C (17). The housekeeping gene of glyceraldehyde-3-phosphate dehydrogenase (GAPDH) was used. The relative mRNA levels of prolactin receptor (PRLP), alpha-lactalbumin (LALBA), serine/threonine kinases (AKT1), beta-1,4-galactosyltransferase 1 (β4GALT1), fatty acid synthase (FASN), glucose transporter (GLUT1), beta-casein (CSN2), interleukin-10 (IL-10), and tumor necrosis factor-α (TNF-α) were calculated using the 2-ΔΔCt method.
2.6 Statistical analysis
Statistical analyses were conducted using SPSS 29.0 statistics software (NY, USA). The Kolmogorov–Smirnov test was used to check if all data in this study followed a normal distribution. Data were expressed as mean ± SEM. Statistical comparisons of different treatments were performed using the one-way analysis of variance (ANOVA). The test results of all analyses were considered significant at a p-value of < 0.05.
3 Result
3.1 Effects of Angelica sinensis extracts on milk components of lactating sows
The lactoprotein and solids-not-fat contents in AS2 and AS3 were higher (p < 0.05) than in the CON at 0 h. The lactoprotein and milk fat contents were higher (p < 0.05) in groups treated with Angelica sinensis extracts than in the CON, and they were higher (p < 0.05) in AS2 and AS3 than in SA1 at 12 h. The lactoprotein, lactose, and milk fat contents were higher (p < 0.05) in groups treated with Angelica sinensis extracts compared to the CON, and the lactoprotein and milk fat contents were higher (p < 0.05) in AS2 and AS3 than in AS1 at 24 h. The lactoprotein, milk fat, and solids-not-fat contents were higher (p < 0.05) in groups treated with Angelica sinensis extracts than in the CON, and the lactoprotein contents were higher (p < 0.05) in AS2 and AS3 than in AS1 on the 7th day after delivery (Table 3).
3.2 Effects of Angelica sinensis extracts on milk immune indexes of lactating sows
The IgA and IL-2 levels were higher (p < 0.05) in the groups treated with Angelica sinensis extracts, the IgM levels were higher (p < 0.05) in AS2 and AS3, and the TNF-α levels were lower (p < 0.05) in AS1, AS2, and AS3 compared to the CON at 0 h. The IgA, IgG, and IL-2 levels were higher (p < 0.05) in AS1, AS2, and AS3, the IgM levels were higher (p < 0.05) in AS2 and AS3, the TNF-α levels were lower (p < 0.05) in groups treated with Angelica sinensis extracts compared to the CON, and the IgA, IgM, and IL-2 levels were higher (p < 0.05) in AS2 and AS3 than in AS1 at 12 h. The IgA, IgG, IgM, and IL-2 levels were higher (p < 0.05), the TNF-α levels were lower (p < 0.05) in groups treated with Angelica sinensis extracts compared to the CON, and the IgA and IgG levels were higher (p < 0.05) in AS2 and AS3 than in AS1 at 24 h. The IgG, IgM, and IL-2 levels were higher (p < 0.05) in AS1, AS2, and AS3 compared to the CON, and the IgG levels were higher (p < 0.05) in AS2 and AS3 than in AS1 on the 7th day after delivery (Table 4).
3.3 Effects of Angelica sinensis extracts on milk hormone levels of lactating sows
The progesterone, prolactin, and insulin contents were higher (p < 0.05) in AS2 and AS3 than in the CON at 0 h. The progesterone, prolactin, growth hormone, and insulin-like growth factor-1 contents were higher (p < 0.05) in AS1, AS2, and AS3, the insulin contents were higher (p < 0.05) in AS2 and AS3 than in the CON, and the progesterone contents were higher (p < 0.05) in AS2 and AS3 than in AS1 at 12 h. The progesterone, prolactin, insulin, and insulin-like growth factor-1 contents were higher (p < 0.05) in groups treated with Angelica sinensis extracts than in the CON, and the prolactin, growth hormone, and insulin contents were higher (p < 0.05) in AS2 and AS3 than in AS1 at 24 h. The growth hormone and insulin-like growth factor-1 contents were higher (p < 0.05) in AS2 and AS3 compared to the CON, and the growth hormone contents were higher (p < 0.05) in AS2 and AS3 than in AS1 on the 7th day after delivery (Table 5).
3.4 Effect of Angelica sinensis extracts on mammary tissue gene expression of lactating sows
The relative expressions of PRLP, LALBA, AKT1, FASN, GLUT1, and CSN2 were higher (p < 0.05) in groups treated with Angelica sinensis extracts than in the CON, the relative expressions of LALBA, FASN, and CSN2 were higher (p < 0.05) in AS2 and AS3 than in AS1, and the relative expressions of FASN were higher (p < 0.05) in AS3 than in AS2. The relative expressions of β4GALT1 were higher (p < 0.05) in AS2 and AS3 than in the CON and AS1 (Figure 1).
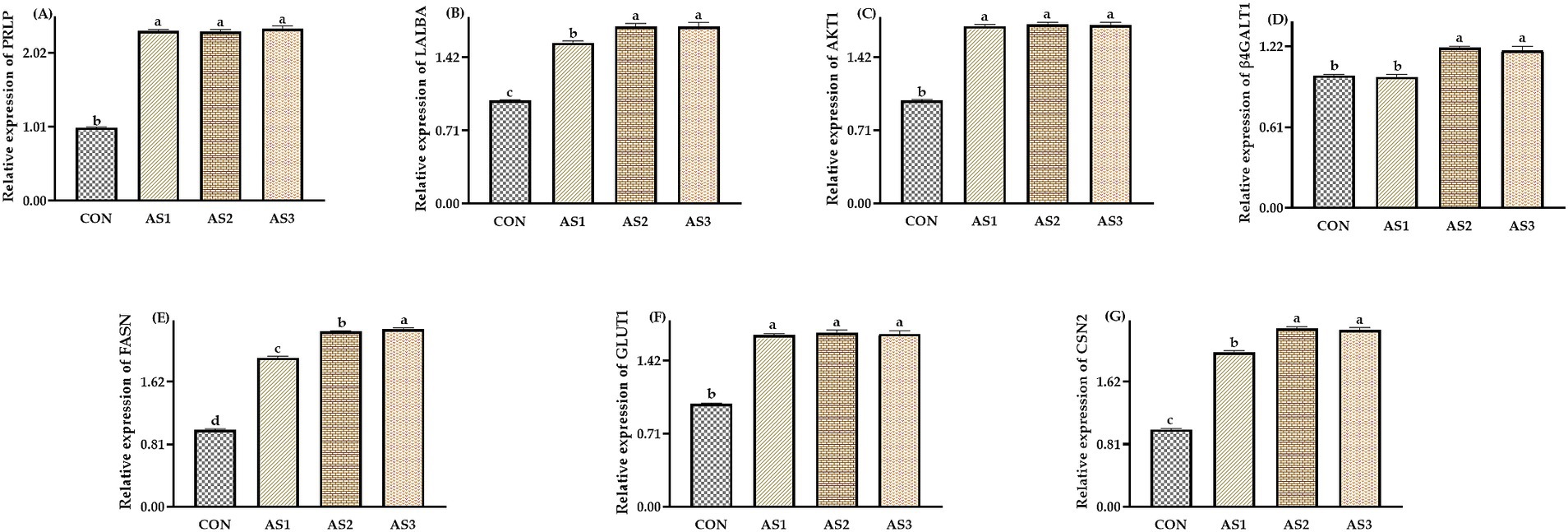
Figure 1. Effects of Angelica sinensis extract on mammary tissue gene expression of lactating sows. (A) The data of relative expression levels of PRLP (prolactin receptor) in the CON, AS1, AS2, and AS3 were 1.00 ± 0.01b, 2.32 ± 0.02a, 2.31 ± 0.02a, and 2.35 ± 0.04a, individually, p = 0.0207; (B) the data of relative expression levels of LALBA (alpha-lactalbumin) in the CON, AS1, AS2, and AS3 were 1.00 ± 0.01c, 1.56 ± 0.02b, 1.72 ± 0.03a, and 1.72 ± 0.04a, individually, p = 0.0397; (C) the data of relative expression levels of AKT1 (serine/threonine kinases) in the CON, AS1, AS2, and AS3 were 1.00 ± 0.01b, 1.72 ± 0.02a, 1.74 ± 0.02a, and 1.73 ± 0.03a, individually, p = 0.0486; (D) the data of relative expression levels of β4GALT1 (beta-1,4-galactosyltransferase 1) in the CON, AS1, AS2, and AS3 were 1.00 ± 0.01b, 0.99 ± 0.02b, 1.21 ± 0.01a, and 1.19 ± 0.03a, individually, p = 0.0424; (E) the data of relative expression levels of FASN (fatty acid synthase) in the CON, AS1, AS2, and AS3 were 1.00 ± 0.01d, 1.93 ± 0.02c, 2.27 ± 0.01b, and 2.30 ± 0.02a, individually, p = 0.0364; (F) the data of relative expression levels of GLUT1 (glucose transporter) in the CON, AS1, AS2, and AS3 were 1.00 ± 0.01b, 1.67 ± 0.01a, 1.69 ± 0.03a, and 1.68 ± 0.03a, individually, p = 0.0268; (G) the data of relative expression levels of CSN2 (beta-casein) in the CON, AS1, AS2, and AS3 were 1.00 ± 0.01c, 2.00 ± 0.02b, 2.31 ± 0.02a, and 2.29 ± 0.03a, individually, p = 0.0237. a,b,c,dValues with different small letter superscripts in the same column mean a significant difference (p < 0.05).
3.5 Effects of Angelica sinensis extracts on immune indexes of piglets
The IgA, IgM, and IL-6 levels were higher (p < 0.05) in groups treated with Angelica sinensis extracts, the IL-2 levels were higher (p < 0.05) in AS2 and AS3 compared to the CON, and the IgM and IL-6 levels were higher (p < 0.05) in AS2 and AS3 than in AS1 in serum of piglets (Table 6).
3.6 Effects of Angelica sinensis extracts on serum hormone levels of piglets
The growth hormone, insulin, and insulin-like growth factor-1 contents were higher (p < 0.05) in groups treated with Angelica sinensis extracts than in the CON, and the insulin and insulin-like growth factor-1 contents were higher (p < 0.05) in AS2 and AS3 than in AS1 in serum of piglets (Table 7).
3.7 Effect of Angelica sinensis extracts on umbilical cord gene expression of piglets
The relative expressions of PRLP and IL-10 were higher (p < 0.05) in groups treated with Angelica sinensis extracts than in the CON (Figure 2).

Figure 2. Effects of Angelica sinensis extract on umbilical cord gene expression of piglets. (A) The data of relative expression levels of PRLP (prolactin receptor) in the CON, AS1, AS2, and AS3 were 1.00 ± 0.01b, 1.15 ± 0.02a, 1.13 ± 0.06a, and 1.16 ± 0.04a, individually, p = 0.0367; (B) the data of relative expression levels of IL-10 (interleukin-10) in the CON, AS1, AS2, and AS3 were 1.00 ± 0.01b, 1.21 ± 0.01a, 1.22 ± 0.02a, and 1.20 ± 0.04a, individually, p = 0.0457; (C) the data of relative expression levels of TNF-α (tumor necrosis factor-α) in CON, AS1, AS2, and AS3 were 1.00 ± 0.01, 1.00 ± 0.02, 1.01 ± 0.03, and 0.99 ± 0.03, individually, p = 0.0926. a,bValues with different small letter superscripts in the same column mean a significant difference (p < 0.05).
4 Discussion
Porcine lactation secretions serve as the gold standard nutritional matrix for neonatal swine development, delivering essential macronutrient profiles for somatic growth while simultaneously incorporating a spectrum of immunobiological factors, including immunoglobulin complexes, cytokine networks, and microbiota-shaping oligosaccharides, that orchestrate immune ontogeny, gastrointestinal barrier maturation, and symbiotic microbial colonization critical for porcine neonate viability (1, 18). Colostrum is the milk produced by mammals in the first few days after delivery, and it contains more proteins, antibodies, vitamins, and minerals than milk (3, 19). Lactoprotein is divided into casein and whey protein, with the former being the most abundant and the latter being soluble, easily digestible, and absorbable (20). Lactose is the main carbohydrate in milk, which can provide animal energy and help absorb mineral elements such as calcium, iron, and zinc (21, 22). Milk fat contains various fatty acids, which can give energy and supply fat-soluble vitamins and beneficial fatty acids such as conjugated linoleic acid for animals (20, 23). Solids-not-fat refers to the substances remaining in milk after removing water and fat, including proteins, lactose, minerals, vitamins, and other substances (20, 24). Chao et al. found that Angelica sinensis extracts have been used to promote breast milk secretion (25). No studies are reporting Angelica sinensis effect on animal milk composition. In the present study, Angelica sinensis extract improved lactose, milk fat, lactoprotein, and solids-not-fat contents of sows at different times after farrowing to varying degrees. The different degrees of improvement in milk composition imply that Angelica sinensis has a specific improvement performance on the lactation performance of sows, which may be attributed to the presence of specific bioactive compounds in Angelica sinensis, which are capable of augmenting the sows’ innate immune function. Consequently, such enhancement facilitates the optimization of the compositional profile and concentration of bioactive constituents within the sows’ milk (26).
Immunoglobulins are fundamental components of the animal immune system, predominantly localized in blood, tissue fluids, and exocrine secretions (27). Their principal function is to identify and bind to specific foreign antigens, including bacteria, viruses, and other pathogens, thereby initiating an immune response that either eliminates or neutralizes these invasive entities (27, 28). Interleukins are a class of proteins produced by and acting on a wide range of cells, which are essential mediators of intercellular communication in the immune system and are involved in regulating immune responses and inflammatory processes (29, 30). Tumor necrosis factors (TNFs) constitute a family of multifunctional cytokines that exert critical influence across various biological processes, including immune responses, inflammatory cascades, apoptosis, and tumor surveillance mechanisms (31, 32). Song et al. found that Angelica sinensis radix significantly increased serum immunoglobulin A levels in broiler chickens (33). Zhao et al. found that Angelica sinensis enhanced the chemical and immune barrier by expanding the lysozyme, mucin 2, sIgA, IgG, and IgM in the jejunum of Cobb 500 broilers (34). Wang et al. reported that Angelica sinensis polysaccharide alleviated the decline of white blood cells, lymphocytes, monocytes, neutrophils, and serum IgG and IgM caused by cyclophosphamide in rats (35). Gu et al. reported that Angelica sinensis polysaccharide significantly enhanced lymphocyte proliferation and improved the ratio of CD4 + to CD8 + T cells in mice (36). In this study, the Angelica sinensis extracts improved the milk IgA, IgG, and IgM contents in sows at different times after farrowing to varying degrees, which indicated that Angelica sinensis extract enhanced the immune function of Wuzhishan sows. Gong et al. found that Angelica sinensis significantly increased the expressions of mRNA and protein levels of interleukin-1β, interleukin-6, and tumor necrosis factor in macrophages (RAW 264.7 cells) (37). In this study, the Angelica sinensis extracts did not significantly improve milk levels of interleukin-6 at any period but reduced levels of tumor necrosis factor 24 h after delivery, which was inconsistent with the above study. This may be attributable to the inhibitory effects of specific bioactive compounds present in Angelica sinensis extracts on certain excessive inflammatory responses. Alternatively, this phenomenon could be influenced by the unique physiological or genetic characteristics inherent to the Wuzhishan sow breed.
Progesterone is an essential natural progestin that promotes the development of breast lobules and glands in preparation for lactation (38, 39). Prolactin, a protein hormone predominantly secreted by the anterior pituitary gland, plays a pivotal role in orchestrating the development and growth of the mammary glands (40). It is instrumental in initiating and maintaining lactation (40, 41). O’Cleirigh et al. found Gui Shao Di Huang Wan containing Angelica sinensis upregulated progesterone receptor β in Ishikawa cells (42). Chao et al. found that Angelica sinensis extracts increased milk production. Thus, it can be inferred that Angelica sinensis extracts increased prolactin levels in women (25). Liu et al. reported that Chinese herbs such as Angelica sinensis improved the amenorrhea caused by antipsychotics by boosting prolactin levels in women (43). In this study, the Angelica sinensis extracts enhanced the levels of progesterone and prolactin in the milk of sows at different stages after delivery to various degrees, which was consistent with the results of the above study, suggesting that Angelica sinensis extracts could regulate the lactation performance of Wuzhishan sows by regulating the levels of progesterone and prolactin in the milk. Growth hormone is a protein secreted by the pituitary gland, which plays a vital role in animal growth and development and fat metabolism (44, 45). Insulin, a peptide hormone synthesized and secreted by the pancreatic β-cells, plays a pivotal role in regulating lipid and protein metabolism while also exerting a significant influence on mineral homeostasis (46). Insulin-like growth factors (IGFs) represent a family of multifunctional regulators of cell proliferation that exert a critical influence on cell differentiation, proliferation, and overall growth and development of an organism (45, 46). Lee et al. found that Angelica sinensis increased the longitudinal bone growth rate in pubertal female rats by elevating growth hormone levels (47). Liu et al. reported that Angelica sinensis polysaccharides can improve insulin resistance in the liver by blocking receptors for advanced glycation end products in high-fat-diet and streptozotocin-induced diabetic rats (48). Liu et al. reported that Angelica sinensis improved insulin sensitivity through increased post-receptor insulin signaling mediated by enhancements in insulin receptor substrate-1-associated phosphatidylinositol 3-kinase step and glucose transporter subtype 4 translocations in soleus muscles of animals exhibiting insulin resistance (49). Wang et al. reported that Angelica sinensis polysaccharide effectively improved insulin resistance in high-fat diet-fed mice (50). Lee et al. found that Angelica sinensis increased local insulin-like growth factor-1 expressions in the growth plate in adolescent female rats (47). In this study, Angelica sinensis extracts improved the levels of growth hormone, insulin, and insulin-like growth factor-1 in the milk of sows at different stages after delivery to various degrees and also regulated the levels of all three in the serum of piglets, which was consistent with the results of the above study, indicating that Angelica sinensis extract may exert a regulatory influence on the lactation performance of Wuzhishan sows and the health status of their piglets through the modulation of growth hormone, insulin, and insulin-like growth factor-1 concentrations.
The prolactin receptor (PRLP) gene encodes the prolactin receptor, a transmembrane protein expressed in tissues such as mammary epithelium, liver, and lymphocytes (51). Alpha-lactalbumin (LALBA), encoded by the LALBA gene, interacts with various proteins, peptides, and low-molecular-weight substrates and products (52). Notably, its association with β-1,4-galactosyltransferase forms a catalytic complex that collectively facilitates the enzymatic synthesis of lactose by transferring a galactose moiety to glucose (52, 53). Serine/threonine kinases (AKT1) are a class of proteins involved in various biological processes within cells, including the cell cycle, signaling, and cellular stress responses (54). The beta-1,4-galactosyltransferase 1 (β4GALT1), whose expression is increased during lactation, forms a lactose synthase complex with a-lactoprotein that catalyzes the reaction of uridine diphosphate-galactose with glucose to produce lactose (17). Fatty acid synthase (FASN) encodes a fatty acid synthase, a multi-enzyme complex responsible for synthesizing long-chain fatty acids in cells (55). The glucose transporters encoded by the GLUT1 gene constitute a family of stereospecific transmembrane proteins that facilitate the translocation of glucose across the cell membrane (56). The specific expression patterns of these transporters are pivotal in determining the cell’s glucose uptake rate (56). The beta-casein (CSN2) plays a central role in milk synthesis and can influence the composition and structure of milk proteins and the functional properties and nutritional value of dairy products (57). In this study, relative expressions of PRLP, LALBA, AKT1, FASN, GLUT1 β4GALT1, and CSN2 were higher in mammary tissue in groups treated with Angelica sinensis extracts, which was in general agreement with the above results of milk protein, lactose, milk fat, and solids-not-fat in the milk of sows after delivery, which also implies that Angelica sinensis extract can regulate lactation performance of Wuzhishan sows at the genetic level. The role of interleukin-10 (IL-10) in immune regulation is multifaceted; it plays a role in the maintenance of immune homeostasis as well as a critical role in the pathogenesis of many diseases (58). In this study, the relative expressions of PRLP and IL-10 were higher in the umbilical cord in groups treated with Angelica sinensis extracts, which also implied that Angelica sinensis extract could modulate the immune function of Wuzhishan sows at the gene level and potentially influence the immune function of piglets.
5 Conclusion
This study examined the impact of incorporating Angelica sinensis extracts into the diet of Wuzhishan sows and revealed significant enhancements in milk composition, immune function, hormone levels, and associated gene expression across various postpartum periods. Notably, the group administered 600 mg/kg of Angelica sinensis extracts demonstrated superior performance in several critical areas: increased lactoprotein, lactose, milk fat, and solids-not-fat contents in milk; boosted levels of key immune indices, including IgA, IgG, and IgM; modulated the concentrations of vital hormones, such as prolactin and growth hormone; and upregulated the expression of pivotal genes in mammary tissue, namely, PRLP, LALBA, and CSN2. These results suggest that a dose of 600 mg/kg of Angelica sinensis extracts represents the optimal concentration for augmenting the lactation performance and overall health of Wuzhishan sows, thereby offering a robust theoretical foundation for its integration into Wuzhishan sow production protocols.
Data availability statement
The raw data supporting the conclusions of this article will be made available by the authors, without undue reservation.
Ethics statement
The animal study was approved by All procedures involving care and management were approved by the Institutional Animal Care and Use Committee of the Chinese Academy of Tropical Agricultural Sciences (Approval No: CATAS-20240315-21). The study was conducted in accordance with the local legislation and institutional requirements.
Author contributions
HW: Data curation, Formal analysis, Software, Writing – original draft, Writing – review & editing. XY: Data curation, Software, Writing – original draft, Writing – review & editing. XZ: Data curation, Methodology, Software, Writing – original draft. WP: Methodology, Resources, Software, Writing – original draft. FJ: Methodology, Project administration, Software, Writing – original draft. QS: Methodology, Software, Writing – original draft. RL: Funding acquisition, Resources, Software, Supervision, Writing – original draft, Writing – review & editing.
Funding
The author(s) declare that financial support was received for the research and/or publication of this article. This study was supported by the National Key Research and Development Program of China (No. 2024YFE0214700), the Central Public-interest Scientific Institution Basal Research Fund (No. 1630032024020), the Hainan Modern Agricultural Production Research System (HNARS-02-G03), and the China Scholarship Council (CSC) National Public Graduate Program for Building Highly Qualified Universities.
Conflict of interest
QS was employed by Hainan Xuhuai Technology Co. Ltd.
The remaining authors declare that the research was conducted in the absence of any commercial or financial relationships that could be construed as a potential conflict of interest.
Generative AI statement
The author(s) declare that no Gen AI was used in the creation of this manuscript.
Publisher’s note
All claims expressed in this article are solely those of the authors and do not necessarily represent those of their affiliated organizations, or those of the publisher, the editors and the reviewers. Any product that may be evaluated in this article, or claim that may be made by its manufacturer, is not guaranteed or endorsed by the publisher.
References
1. Xu, C, Xie, J, Ji, F, Peng, W, Song, Y, Diao, X, et al. Supplementation of dietary semen vaccariae extracts to lactating sow diets: effects on the production performance, milk components, and gene expression related to mammogenesis. Front Vet Sci. (2023) 10:1284552. doi: 10.3389/fvets.2023.1284552
2. Hu, P, Yang, H, Lv, B, Zhao, D, Wang, J, and Zhu, W. Dynamic changes of fatty acids and minerals in sow milk during lactation. J Anim Physiol Anim Nutr. (2019) 103:603–11. doi: 10.1111/jpn.13040
3. Puppel, K, Gołębiewski, M, Grodkowski, G, Slósarz, J, Kunowska-Slósarz, M, Solarczyk, P, et al. Composition and factors affecting quality of bovine colostrum: a review. Animals. (2019) 9:1070. doi: 10.3390/ani9121070
4. Kessler, EC, Bruckmaier, RM, and Gross, JJ. Comparative estimation of colostrum quality by brix refractometry in bovine, caprine, and ovine colostrum. J Dairy Sci. (2021) 104:2438–44. doi: 10.3168/jds.2020-19020
5. Godden, SM, Lombard, JE, and Woolums, AR. Colostrum management for dairy calves. Vet Clin Food Anim Pract. (2019) 35:535–56. doi: 10.1016/j.cvfa.2019.07.005
6. Sandrucci, A, Bava, L, Tamburini, A, Gislon, G, and Zucali, M. Management practices and milk quality in dairy goat farms in northern Italy. Ital J Anim Sci. (2019) 18:1–12. doi: 10.1080/1828051X.2018.1466664
7. Jaguezeski, AM, Perin, G, Bottari, NB, Wagner, R, Fagundes, MB, Schetinger, MRC, et al. Addition of curcumin to the diet of dairy sheep improves health, performance and milk quality. Anim Feed Sci Technol. (2018) 246:144–57. doi: 10.1016/j.anifeedsci.2018.10.010
8. Nai, J, Zhang, C, Shao, H, Li, B, Li, H, Gao, L, et al. Extraction, structure, pharmacological activities and drug carrier applications of Angelica sinensis polysaccharide. Int J Biol Macromol. (2021) 183:2337–53. doi: 10.1016/j.ijbiomac.2021.05.213
9. Lu, C, Liu, M, Shang, W, Yuan, Y, Li, M, Deng, X, et al. Knowledge mapping of Angelica sinensis (Oliv.) Diels (Danggui) research: a scientometric study. Front Pharmacol. (2020) 11:294. doi: 10.3389/fphar.2020.00294
10. Zhao, L, Zhang, J, He, J, Guo, M, Wu, H, Ma, X, et al. Network pharmacology analysis of the regulatory effects and mechanisms of ALAE on sow reproduction in vivo and in vitro. J Ethnopharmacol. (2024) 334:118525. doi: 10.1016/j.jep.2024.118525
11. Wu, F, Ji, P, Hu, Y, Li, C, and He, J. Study on the hepatoprotective effect mechanism of polysaccharides from charred angelica sinensis on the layer chickens based on the detection of the intestinal floras and short-chain fatty acids of cecal contents and association analysis. Vet Sci. (2023) 10:224. doi: 10.3390/vetsci10030224
12. Tian, M, Li, K, Liu, R, Du, J, Zou, D, and Ma, Y. Angelica polysaccharide attenuates LPS-induced inflammation response of primary dairy cow claw dermal cells via NF-κB and MAPK signaling pathways. BMC Vet Res. (2021) 17:248. doi: 10.1186/s12917-021-02952-4
13. Duan, X, Wang, X, Li, Z, Liu, C, Bao, Y, Shi, W, et al. Effects of supplemental feeding of Chinese herbal mixtures to perinatal sows on antioxidant capacity and gut microbiota of sows and their offspring piglets. Front Microbiol. (2024) 15:1459188. doi: 10.3389/fmicb.2024.1459188
14. Niu, M, Liu, Y, Xiang, L, Zhao, Y, Yuan, J, Jia, Y, et al. Long-term case study of a Wuzhishan miniature pig with diabetes. Anim Model Exp Med. (2020) 3:22–31. doi: 10.1002/ame2.12098
15. Shao, M, Wang, Z, He, Y, Tan, Z, and Zhang, J. Fecal microbial composition and functional diversity of Wuzhishan pigs at different growth stages. AMB Express. (2021) 11:88. doi: 10.1186/s13568-021-01249-x
16. Hou, G, Wang, D, Guan, S, Zeng, H, Huang, X, and Ma, Y. Associated analysis of single nucleotide polymorphisms of IGF2 gene's exon 8 with growth traits in Wuzhishan pig. Mol Biol Rep. (2010) 37:497–500. doi: 10.1007/s11033-009-9681-5
17. Wu, H, Xu, C, Wang, J, Hu, C, Ji, F, Xie, J, et al. Effects of dietary probiotics and acidifiers on the production performance, colostrum components, serum antioxidant activity and hormone levels, and gene expression in mammary tissue of lactating sows. Animals. (2023) 13:1536. doi: 10.3390/ani13091536
18. Juthamanee, P, and Tummaruk, P. Factors associated with colostrum consumption in neonatal piglets. Livest Sci. (2021) 251:104630. doi: 10.1016/j.livsci.2021.104630
19. Inoue, R, and Tsukahara, T. Composition and physiological functions of the porcine colostrum. Anim Sci J. (2021) 92:e13618. doi: 10.1111/asj.13618
20. Goulding, DA, Fox, PF, and O’Mahony, JA. Milk proteins: An overview. Milk Prot. Amsterdam, The Netherlands: Elsevier. (2020) 21–98. doi: 10.1016/B978-0-12-815251-5.00002-5
21. Rao, PS, Singh, P, Sharma, V, and Arora, S. Traditional analytical approaches for lactose residues determination in lactose hydrolysed milks: a review. LWT. (2021) 151:112069. doi: 10.1016/j.lwt.2021.112069
22. Ohlsson, JA, Johansson, M, Hansson, H, Abrahamson, A, Byberg, L, Smedman, A, et al. Lactose, glucose and galactose content in milk, fermented milk and lactose-free milk products. Int Dairy J. (2017) 73:151–4. doi: 10.1016/j.idairyj.2017.06.004
23. Hageman, JH, Danielsen, M, Nieuwenhuizen, AG, Feitsma, AL, and Dalsgaard, TK. Comparison of bovine milk fat and vegetable fat for infant formula: implications for infant health. Int Dairy J. (2019) 92:37–49. doi: 10.1016/j.idairyj.2019.01.005
24. Liu, Q, Guo, W, He, H, and Zhu, X. Effect of solids-not-fat content on dielectric properties of skim milk. Int J Food Sci Technol. (2018) 53:2560–6. doi: 10.1111/ijfs.13895
25. Chao, J, Ko, CY, Lin, CY, Tomoji, M, Huang, CH, Chiang, HC, et al. Ethnobotanical survey of natural galactagogues prescribed in traditional chinese medicine pharmacies in Taiwan. Front Pharmacol. (2021) 11:625869. doi: 10.3389/fphar.2020.625869
26. Liu, L, Chen, D, Yu, B, Luo, Y, Huang, Z, Zheng, P, et al. Influences of selenium-enriched yeast on growth performance, immune function, and antioxidant capacity in weaned pigs exposure to oxidative stress. Biomed Res Int. (2021) 2021:5533210. doi: 10.1155/2021/5533210
27. Ulfman, LH, Leusen, JHW, Savelkoul, HFJ, Warner, JO, and van Neerven, RJJ. Effects of bovine immunoglobulins on immune function, allergy, and infection. Front Nutr. (2018) 5:52. doi: 10.3389/fnut.2018.00052
28. Guo, Y, Tian, X, Wang, X, and Xiao, Z. Adverse effects of immunoglobulin therapy. Front Immunol. (2018) 9:1299. doi: 10.3389/fimmu.2018.01299
29. Briukhovetska, D, Dörr, J, Endres, S, Libby, P, Dinarello, CA, and Kobold, S. Interleukins in cancer: from biology to therapy. Nat Rev Cancer. (2021) 21:481–99. doi: 10.1038/s41568-021-00363-z
30. Boskabadi, H, and Zakerihamidi, M. Evaluate the diagnosis of neonatal sepsis by measuring interleukins: a systematic review. Pediatr Neonatol. (2018) 59:329–38. doi: 10.1016/j.pedneo.2017.10.004
31. Salomon, BL, Leclerc, M, Tosello, J, Ronin, E, Piaggio, E, and Cohen, JL. Tumor necrosis factor α and regulatory T cells in oncoimmunology. Front Immunol. (2018) 9:444. doi: 10.3389/fimmu.2018.00444
32. Alijotas-Reig, J, Esteve-Valverde, E, Ferrer-Oliveras, R, Llurba, E, and Maria, GJ. Tumor necrosis factor-alpha and pregnancy: focus on biologics. An updated and comprehensive review. Clin Rev Allerg Immunol. (2017) 53:40–53. doi: 10.1007/s12016-016-8596-x
33. Song, J, Ji, B, Peng, YN, Ye, Z, Wang, ZY, Zhao, TW, et al. Effects of supplementation with Angelica sinensis radix and its powder formulation on growth performance, serum antioxidant capacity, immune response and intestinal microflora of broiler chickens. J Anim Feed Sci. (2024) 33:504–16. doi: 10.22358/jafs/189181/2024
34. Zhao, X, Hao, S, Zhang, J, Yao, Y, Li, L, Sun, L, et al. Aerial parts of Angelica sinensis supplementation for improved broiler growth and intestinal health. Poult Sci. (2024) 103:103473. doi: 10.1016/j.psj.2024.103473
35. Wang, YL, Shu, XH, Zhang, X, Liu, YB, Zhang, YJ, Lv, T, et al. Effects of two polysaccharides from traditional Chinese medicines on rat immune function. Front Vet Sci. (2021) 8:703956. doi: 10.3389/fvets.2021.703956
36. Gu, P, Liu, Z, Sun, Y, Ou, N, Hu, Y, Liu, J, et al. Angelica sinensis polysaccharide encapsulated into PLGA nanoparticles as a vaccine delivery and adjuvant system for ovalbumin to promote immune responses. Int J Pharm. (2019) 554:72–80. doi: 10.1016/j.ijpharm.2018.11.008
37. Gong, G, Zhang, L, Lam, C, Xu, M, Wang, H, Lin, H, et al. Danggui Buxue Tang, an ancient Chinese herbal decoction, protects β-amyloid-induced cell death in cultured cortical neurons. BMC Complement Altern Med. (2019) 19:9. doi: 10.1186/s12906-018-2411-6
38. Di Renzo, GC, Tosto, V, and Tsibizova, V. Progesterone: history, facts, and artifacts. Best Pract Res Clin Obstet. (2020) 69:2–12. doi: 10.1016/j.bpobgyn.2020.07.012
39. Mac Lean, JAII, and Hayashi, K. Progesterone actions and resistance in gynecological disorders. Cells. (2022) 11:647. doi: 10.3390/cells11040647
40. Bernard, V, Young, J, and Binart, N. Prolactin--a pleiotropic factor in health and disease. Nat Rev Endocrinol. (2019) 15:356–65. doi: 10.1038/s41574-019-0194-6
41. Borba, VV, Zandman-Goddard, G, and Shoenfeld, Y. Prolactin and autoimmunity. Front Immunol. (2018) 9:73. doi: 10.3389/fimmu.2018.00073
42. O’Cleirigh, R, and Roslyn, G. Gui Shao Di Huang wan promotes angiogenesis and regulates oestrogen receptor α and progesterone receptor β in vitro bioassays. bioRxiv. (2021):2021–06. doi: 10.1101/2021.06.14.448215
43. Liu, L, Li, H, Tan, G, and Ma, Z. Traditional Chinese herbal medicine in treating amenorrhea caused by antipsychotic drugs: Meta-analysis and systematic review. J Ethnopharmacol. (2022) 289:115044. doi: 10.1016/j.jep.2022.115044
44. Ranke, MB, and Wit, JM. Growth hormone--past, present and future. Nat Rev Endocrinol. (2018) 14:285–300. doi: 10.1038/nrendo.2018.22
45. Takahashi, Y. The role of growth hormone and insulin-like growth factor-I in the liver. Int J Mol Sci. (2017) 18:1447. doi: 10.3390/ijms18071447
46. Yaribeygi, H, Farrokhi, FR, Butler, AE, and Sahebkar, A. Insulin resistance: review of the underlying molecular mechanisms. J Cell Physiol. (2019) 234:8152–61. doi: 10.1002/jcp.27603
47. Lee, D, and Kim, H. Effects of Angelica sinensis root on longitudinal bone growth rate in adolescent female rats. Korean J Herbol. (2017) 32:69–74. doi: 10.6116/kjh.2017.32.1.69
48. Liu, W, Li, Z, Feng, C, Hu, S, Yang, X, Xiao, K, et al. The structures of two polysaccharides from Angelica sinensis and their effects on hepatic insulin resistance through blocking RAGE. Carbohydr Polym. (2022) 280:119001. doi: 10.1016/j.carbpol.2021.119001
49. Liu, IM, Tzeng, TF, and Liou, SS. A Chinese herbal decoction, dang gui bu xue tang, prepared from radix astragali and radix angelicae sinensis, ameliorates insulin resistance induced by a high-fructose diet in rats. Evid Based Complement Alternat Med. (2011) 2011:248231. doi: 10.1093/ecam/nep004
50. Wang, K, Cao, P, Wang, H, Tang, Z, Wang, N, Wang, J, et al. Chronic administration of Angelica sinensis polysaccharide effectively improves fatty liver and glucose homeostasis in high-fat diet-fed mice. Sci Rep. (2016) 6:26229. doi: 10.1038/srep26229
51. Cosenza, G, Iannaccone, M, Auzino, B, Macciotta, NPP, Kovitvadhi, A, Nicolae, I, et al. Remarkable genetic diversity detected at river buffalo prolactin receptor (PRLR) gene and association studies with milk fatty acid composition. Anim Genet. (2018) 49:159–68. doi: 10.1111/age.12645
52. Yang, F, Zhang, M, Rong, Y, Liu, Z, Yang, S, Zhang, W, et al. A novel SNPs in alpha-Lactalbumin gene effects on lactation traits in chinese Holstein dairy cows. Animals. (2020) 10:60. doi: 10.3390/ani10010060
53. Manzoor, S, Nadeem, A, and Javed, M. Polymorphism association and expression analysis of alpha-lactalbumin (LALBA) gene during lactation in Nili Ravi buffalo. Trop Anim Health Prod. (2020) 52:265–71. doi: 10.1007/s11250-019-02010-0
54. Janczarek, M, Vinardell, JM, Lipa, P, and Karaś, M. Hanks-type serine/threonine protein kinases and phosphatases in bacteria: roles in signaling and adaptation to various environments. Int J Mol Sci. (2018) 19:2872. doi: 10.3390/ijms19102872
55. Fhu, CW, and Ali, A. Fatty acid synthase: an emerging target in cancer. Molecules. (2020) 25:3935. doi: 10.3390/molecules25173935
56. Wang, J, Ye, C, Chen, C, Xiong, H, Xie, B, Zhou, J, et al. Glucose transporter GLUT1 expression and clinical outcome in solid tumors: a systematic review and meta-analysis. Oncotarget. (2017) 8:16875–86. doi: 10.18632/oncotarget.15171
57. Al-Amareen, AH, and Jawasreh, KI. Single and combined effects of CSN1S1 and CSN2-casein genes on Awassi sheep milk quantity and quality. Vet World. (2022) 15:435–41. doi: 10.14202/vetworld.2022.435-441
58. Mirjalili, SA, Moghimi, M, Aghili, K, Jafari, M, Abolbaghaei, SM, Neamatzadeh, H, et al. Association of promoter region polymorphisms of interleukin-10 gene with susceptibility to colorectal cancer: a systematic review and meta-analysis. Arq Gastroenterol. (2018) 55:306–13. doi: 10.1590/s0004-2803.201800000-66
Keywords: Angelica sinensis extracts, lactating sows, colostrum composition, immune function, milk hormone levels
Citation: Wu H, Yu X, Zhang X, Peng W, Ji F, Shen Q and Lv R (2025) Supplementation of dietary Angelica sinensis extracts to lactating Wuzhishan sows: effects on milk composition, immune function, milk-derived hormones, and related gene expression. Front. Vet. Sci. 12:1524258. doi: 10.3389/fvets.2025.1524258
Edited by:
Giovanni Buonaiuto, University of Bologna, ItalyReviewed by:
Tarique Hussain, Nuclear Institute for Agriculture and Biology, PakistanWenkui Ma, China Agricultural University, China
Copyright © 2025 Wu, Yu, Zhang, Peng, Ji, Shen and Lv. This is an open-access article distributed under the terms of the Creative Commons Attribution License (CC BY). The use, distribution or reproduction in other forums is permitted, provided the original author(s) and the copyright owner(s) are credited and that the original publication in this journal is cited, in accordance with accepted academic practice. No use, distribution or reproduction is permitted which does not comply with these terms.
*Correspondence: Renlong Lv, bHZyZW5sb25nQGFsaXl1bi5jb20=
†These authors have contributed equally to this work