- 1Department of Animal Genetics, Breeding and Reproduction, College of Animal Science and Technology, Nanjing Agricultural University, Nanjing, China
- 2Department of Poultry Genetics and Breeding, Nanjing Institute of Animal Husbandry and Poultry Science, Nanjing, China
Introduction: The present study aimed to evaluate the potential of antimicrobial peptide microcin J25 (MccJ25) as a feed additive for pigeons.
Methods: A total of 28-day-old pigeons were allocated to four groups and fed a basal diet (CON) or a basal diet supplemented with 100, 200, or 300 mg/kg MccJ25 (ABP100, ABP200, and ABP300) for 8 weeks.
Results: Dietary MccJ25 supplementation significantly improved survival rates in the ABP200 group compared to the control (p < 0.05). Serum analysis revealed that ABP200 and ABP300 groups exhibited increased levels of total protein (TP), globulin (GLB), immunoglobulin A (IgA), and immunoglobulin G (IgG), alongside reduced aspartate aminotransferase (AST), alanine aminotransferase (ALT), total cholesterol (TC), and triglyceride (TG) concentrations (p < 0.05). Furthermore, MccJ25 supplementation enhanced duodenal maltase and trypsin activity (p < 0.05) and upregulated intestinal and hepatic antioxidant capacity, as evidenced by elevated glutathione peroxidase (GSH-Px) and superoxide dismutase (SOD) activity (p < 0.05). Intestinal morphology improvements were observed in the jejunum and ileum, with increased villus height-to-crypt depth ratios (VH/CD) (p < 0.05). Additionally, ABP200 and ABP300 groups demonstrated significant upregulation of intestinal barrier-related genes occludin (OCLN), claudin 1 (CLDN1), zonula occluden protein 1 (ZO1), mucin 2 (MUC2), superoxide dismutase 1, 2 (SOD1, 2), and catalase (CAT) in duodenum (p < 0.05).
Discussion: These findings indicate that MccJ25 supplementation improves systemic metabolism, enhances antioxidant defenses, strengthens intestinal barrier integrity, and ultimately promotes pigeon health and survival. This study supports the application of MccJ25 as a functional feed additive in poultry production.
1 Introduction
Antibacterial peptides (ABPs), small molecular peptides that are important to innate immune defense system (1, 2), have emerged as promising alternatives to antibiotics in livestock production due to their broad-spectrum antimicrobial activity and low risk of inducing resistance (3, 4). However, the potential effect of ABPs on pigeon production and their possibility of being used as a feed additive product still need to be studied. While studies in poultry (e.g., broilers) have demonstrated that AMPs enhance intestinal barrier function and modulate immune responses (1, 5), their application in pigeon production remains underexplored—a critical gap given the unique digestive physiology and commercial importance of pigeons in the global poultry industry.
Microcin J25 (MccJ25), a bacteriocin produced by Escherichia coli, has garnered attention for its unique stability and efficient antibacterial and anti-inflammatory properties (6). Recent advances highlight the interplay between AMPs and gut microbiota in mediating host health (7), and ABPs have been used as feed additives in livestock production (8, 9). Previous study showed that addition of ABP decreased the number of Escherichia coli in the ileum and cecum, which promoted intestinal health in broilers and then improved the production performance (10). Another study indicated that the addition of ABP to diets significantly reduced the number of coliforms in ileum and cecum and enhanced the humoral immune function, resulting in increased growth performance in weaned piglets (7, 11). It was also showed that dietary supplemented MccJ25 effectively improved performance, attenuated diarrhea and systematic inflammation, enhanced intestinal barrier function, and improved fecal microbiota composition of weaned pigs (12). In pigeons, however, the mechanisms by which AMPs influence gut microbiota composition, redox homeostasis, and systemic immunity remain unclear.
Pigeon meat industry has developed rapidly in recent years and has great market potential (13, 14). Pigeon squabs are first fed by crop milk secreted by their parents, and the digestive system of squabs is hypersensitive during the transition to self-feeding (15, 16). The weight of 28-day old King pigeons was 25.7 times that of the 1-day old, and then reaching their mature weight by day 28 (17). Our previous study found that ABP MccJ25 and improved the antioxidant capacity and production performance of pigeons, which is beneficial to the health of pigeon squabs (18).
This study aimed to evaluate the impact of MccJ25 supplementation on pigeon health and its potential as a feed additive. By integrating serum biochemistry, digestive enzyme profiling, and histopathological analyses, this work provides a comprehensive assessment of MccJ25′s potential as a sustainable feed additive, offering novel insights into AMP applications in niche poultry sectors.
2 Materials and methods
2.1 Ethics statement
This study was approved by the Laboratory Animal Welfare and Ethics Committee of Nanjing Agricultural University, China (Permit number: SYXK-2021-0014).
2.2 Animal experimental design, management and diet
A total of 360 28-day-old American Silver King pigeons (450 ± 10 g body weight) from a genetically homogeneous breeding line (Dongchen Pigeon Industry Co. Ltd., Nanjing, China) were randomly allocated into 4 dietary groups (n = 5 replicates/group). There were 18 pigeons for each replicate, which was divided into 6 cages with 3 pigeons per cage. Pigeons were raised in a ventilated three-layer ladder cage (45 × 40 × 40 cm3) with free access to feed and water. Pigeons were raised in a semi-open dovecote which was ventilated both mechanically and naturally, with an average temperature of 25°C-32°C, relative humidity 55–70% and natural light. The control group (CON) received a basal diet, and the experimental groups (ABP100, ABP200, and ABP300) were fed a basal diet supplemented with 100, 200, or 300 mg/kg MccJ25 (Zhongnong Yingtai Linzhou Biological Co. Ltd., purity: 94.7%) for 8 weeks. The composition and nutrient content of the pigeon diet are shown in Table 1.
2.3 Survival rate
At the end of trial, the number of died pigeons in each replicate was counted and the survival rate was calculated. The formula for survival rate for each repetition is as follows:
2.4 Sample collection
At 84 days of age, one pigeon from each replicate was randomly selected for left wing vein blood collection followed by cervical dislocation. Serum was separated by centrifugation of blood at 4,000 rpm for 15 min at 4°C and stored at −20°C. Duodenal and ileal contents were first collected aseptically, rapidly frozen and stored at −80°C. The intestines were re-moved, the intestinal contents were rinsed with phosphate buffer saline (PBS) and samples of duodenum, jejunum, ileum, jejunal mucosa, and liver were collected partly for intestinal morphology analysis and partly immediately cryopreserved at −80°C for further experiments.
2.5 Laboratory analysis
2.5.1 Serum biochemical parameters
The serum biochemical parameters, including total protein (TP), aspartate ami-notransferase (AST), glucose (GLU), albumin (ALB), alanine aminotransferase (ALT), globulin (GLB), total cholesterol (TC), alkaline phosphatase (ALP) and triglyceride (TG) were measured spectrophotometrically using an automated system (CLS880 analyzer, Nanjing, China). The contents of immunoglobulin A (IgA), immunoglobulin G (IgG), and immunoglobulin M (IgM) in pigeon serum were assayed using commercial chicken elisa kits (Nanjing Aoqing Biotechnology Co., Ltd., CAT, ANG-E32004C, ANG-E32009C, and ANG-E32005C, Nanjing, China).
2.5.2 Digestive enzyme activity
The contents of duodenum in pigeons were collected and were homogenized with ice-cold sodium chloride solution by using an Automatic Sample Rapid Grinding Machine. Then, the homogenization buffer was centrifuged at 3,500 rpm for 10 min at 4°C. The supernatant was used for assaying the enzyme activity of lipase (LPS), amylase (AMS), maltase, trypsin, and chymotrypsin in the duodenum contents of pigeons by using commercial kits (Nanjing Aoqing Biotechnology Co. Ltd., CAT, ZS3151, MZ4302, MZ4638, ZM4288, and ZM4292, respectively, Nanjing, China). The absorbance of each sample was spectrophotometrically measured using a spectrometer against a blank and then the activity was calculated according to the instructions provided by the manufacturer.
2.5.3 Intestinal morphology
Approximately 1-cm intestinal segments (duodenum, jejunum and ileum) were collected and fixed in 4% paraformaldehyde for 24 h. Each sample was dehydrated, cleared and embedded in paraffin and cut at the thickness of 5 μm by using a microtome (LeicaBiosystems, Wetzlar, Germany). Then sections were stained with hematoxylin and eosin (H&E). Five fields of each section were randomly selected for image acquisition under a microscope (OlympusBX50, Tokyo, Japan). The villus height (VH), crypt depth (CD), and the ratio of villus height to crypt depth (V/C) were measured.
2.5.4 Antioxidant indicators
The liver and serum samples were collected from pigeons on day 84 to test the activity of catalase (CAT), glutathione peroxidase (GSH-Px), superoxide dismutase (SOD), total antioxidant capacity (T-AOC), and the content of malonaldehyde (MDA). The level of SOD1 (Cu/Zn-SOD) and SOD2 (Mn-SOD) were measured respectively. Liver samples of approximately 1 g were homogenized with ice-cold sodium chloride solution according to the ratio of mass (g): volume (mL) = 1:9. Then, the homogenization buffer was centrifuged at 3,000 rpm for 10 min at 4°C. The supernatant was used for assaying the antioxidant indices by using commercial kits (Nanjing Aoqing Biotechnology Co. Ltd., CAT, YH1206, YH1267, YH1201, YH1248, and YH1218, respectively, Nanjing, China).
2.5.5 Genes expression
Total RNA was extracted from intestinal, jejunal mucosa, and liver samples with Trizol, and cDNA was synthesized from the RNA using a cDNA synthesis kit (ABM, Richmond, CAN). The expression of target genes was measured using a BlasTaq 2 X qPCR MasterMix (ABM, Richmond, CAN) and a CFX Connect PCR Detection System (Bio-Rad, Hercules, CA, USA) using a reaction program of initial denaturation at 95°C for 3 min and then continued with 40 cycles of 95°C for 15 s and 60°C for 1 min. The primer sequence information of the genes is shown in Table 2. The relative mRNA expression of the target genes were calculated using the 2−ΔΔCT method with β-actin as the control.
2.6 Statistical analysis
Normality of data was confirmed via the Shapiro-Wilk test by using SPSS 20.0 software (SPSS Inc., Chicago, IL, USA). One-way ANOVA with Tukey multiple range test was applied for group comparisons. Survival rate was measured in replicates as the experimental unit, and the experimental unit for the other parameters was the individual bird. The statistical results were expressed as mean ± SEM, with significance set at p < 0.05. The graphs were drawn by using GraphPad Prism 6.0 software (San Diego, CA).
3 Results
3.1 Effect of ABP MccJ25 on survival rate in pigeons
Dietary MccJ25 supplementation significantly improved survival rates (p = 0.045; Table 3). From 28 to 84 days, the survival rate of all ABP groups was obviously increased compared with the control group, and ABP200 group showed the highest survival rate (Table 3).
3.2 Effect of ABP MccJ25 on biochemical and immunological parameters in pigeon serum
The impact of ABP treatment on the serum biochemical parameter of pigeons is presented in Table 4. Compared with the control group, ABP200 and ABP300 groups significantly increased the level of TP and GLB in pigeon serum (Table 4). ABP groups significantly reduced the level of AST and TG compared with the control group (Table 4), and the level of ALT and total TC were remarkably lower in the ABP200 and ABP300 groups than that of the control group (Table 4). But ALP and GLU showed no remarkable difference after the supplement of ABP (Table 4). The level of IgA and IgG in pigeon serum was significantly higher in all ABP groups than in the control group (Table 4). In addition, the content of IgM in pigeon serum was also increased in ABP groups, and it was significantly higher in ABP200 group than that of the control group (Table 4).
3.3 Effect of ABP MccJ25 on the digestive enzyme activity in pigeon duodenum
Compared with the control group, the activity of maltase and trypsin in pigeon duodenal contents was significantly increased in ABP groups (Table 5), and chymotrypsin activity was also significantly increased in ABP200 and ABP300 groups (Table 5). In addition, AMS activity in the ABP200 group and LPS activity in the ABP300 group were significantly higher than control group (Table 5).
3.4 Effect of ABP MccJ25 on the intestinal morphology and barrier function in pigeons
The intestinal villi of pigeons in ABP groups showed complete structure in morphology and high density compared with the control group (Figure 1). Villus height (VH) significantly increased by 15.4% (p < 0.001), V/C ratio by 12.7% in duodenum; VH (+10.8%) and V/C ratio (+11.3%) (p < 0.01) in jejunum; VH (+8.2%) and V/C ratio (+11.1%) (p < 0.05) in ileum by diet supplement with ABP200 (Table 6).
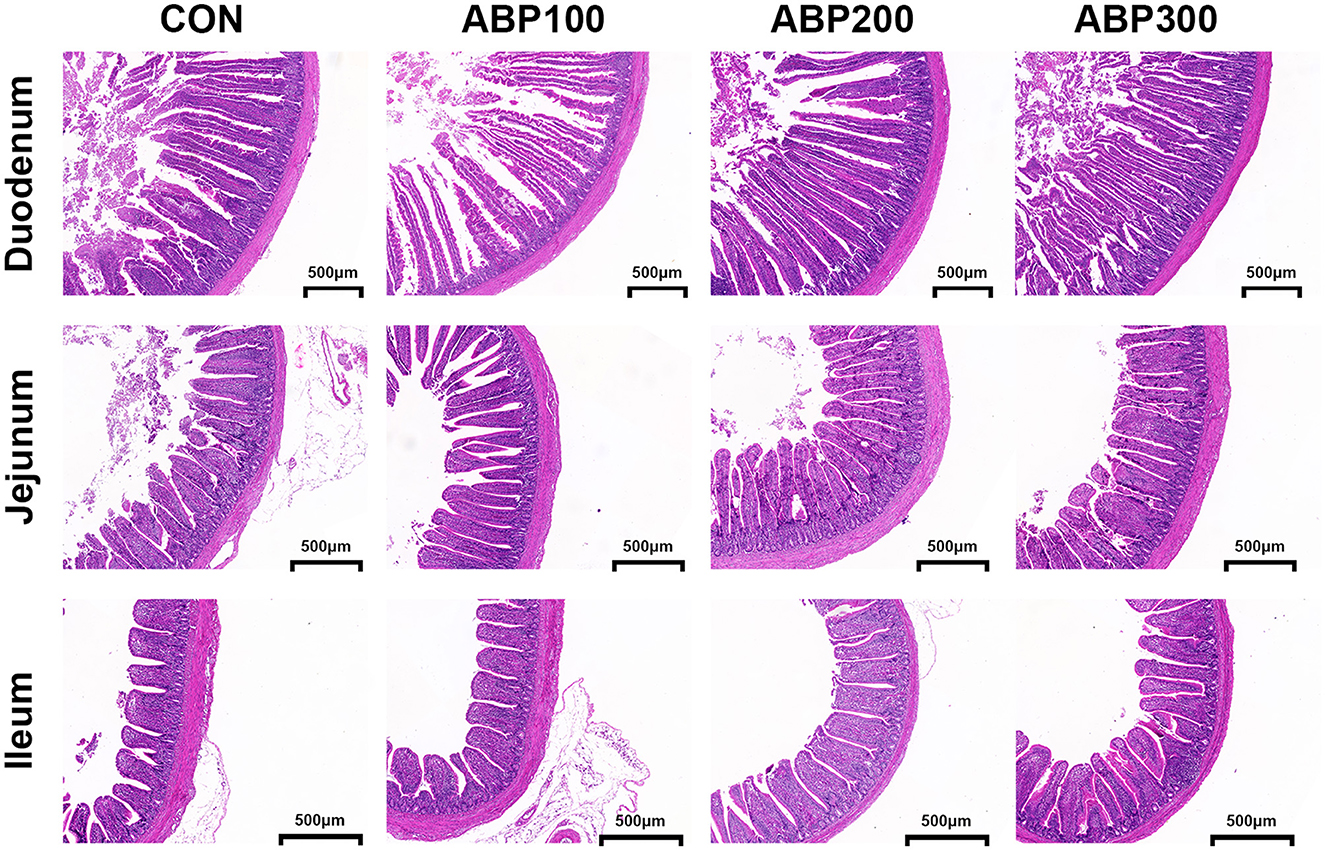
Figure 1. Intestinal morphology of 84-day-old pigeons. The morphology of intestinal villi was observed by hematoxylin and eosin (H&E) staining. Scale bar, 500 μm.
ABP groups significantly up-regulated the mRNA expression level of the OCLN, CLDN1, ZO-1 and MUC2 in pigeon duodenum compared with control group (Figure 2). In pigeon jejunum, ABP groups obviously up-regulated the mRNA expression level of the ZO-1 and MUC2 compared with control group, and ABP200 and ABP300 groups significantly increased the mRNA expression of OCLN (Figure 2). In pigeon ileum, compared with control group, the mRNA expression of the OCLN, CLDN1 and ZO-1 was significantly increased in the ABP200 and ABP300 groups (Figure 2). In pigeon jejunal mucosa, compared with the control group, ABP200 and ABP300 groups significantly up-regulated the mRNA expression level of the CLDN1, ZO-1 and MUC2 (Figure 2).
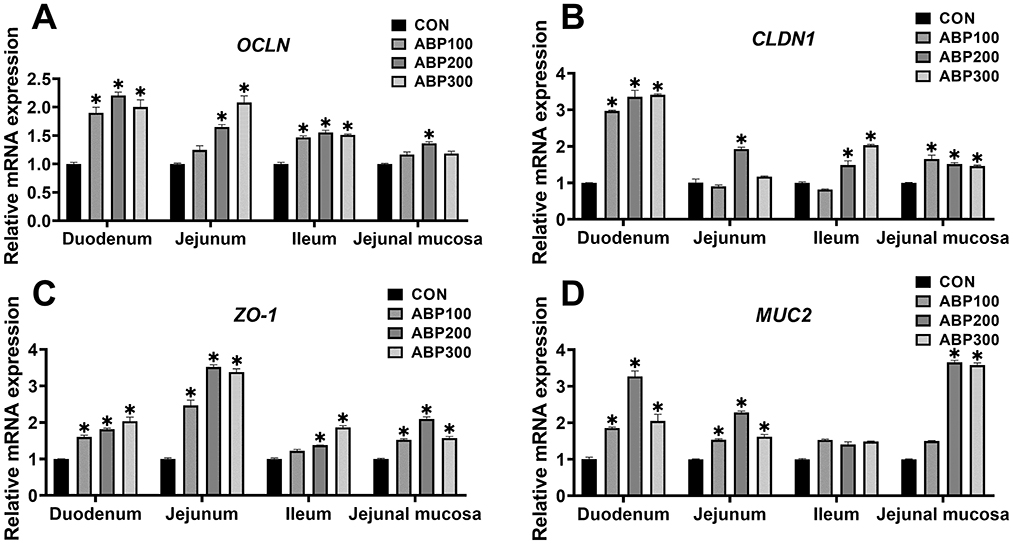
Figure 2. Relative mRNA expression of intestinal barrier-related genes in 84-day-old pigeons. The mRNA expression of tight junction genes OCLN (A), CLDN1 (B), ZO-1 (C) and MUC2 (D) was measurted by qRT-PCR. OCLN, Occludin; CLDN1, Claudin 1; ZO-1, Zonula occluden protein 1; MUC2, Mucin 2. Data are presented as mean ± SEM. ns, p > 0.05; *p < 0.05 vs. CON.
3.5 Effect of ABP MccJ25 on the antioxidant capacity in pigeons
In pigeon serum, the activity of T-AOC and GSH-PX was remarkably increased and MDA content was dramatically decreased in ABP200 and ABP300 groups compared with the control group (Table 7). The activity of SOD in ABP200 group was significantly higher than control group (Table 7). In pigeon liver, ABP200 and ABP300 groups notably enhanced the activity of GSH-PX, SOD and CAT in pigeons compared with the control group, but the content of MDA in ABP200 group was significantly lower than the control group (Table 7).
Compared with the control group, the ABP200 and ABP300 groups significantly up-regulated the mRNA expression level of the SOD1, SOD2 and CAT in pigeon duo-denum, jejunum and liver (Figure 3). The mRNA expression of the SOD1 and CAT was significantly increased in the ABP200 and ABP300 groups in pigeon ileum compared with the control group (Figure 3). In pigeon jejunal mucosa, ABP200 and ABP300 groups significantly increased the mRNA expression of SOD1 and SOD2 compared with the control group, and the mRNA expression of CAT was also significantly increased in ABP200 group (Figure 3).
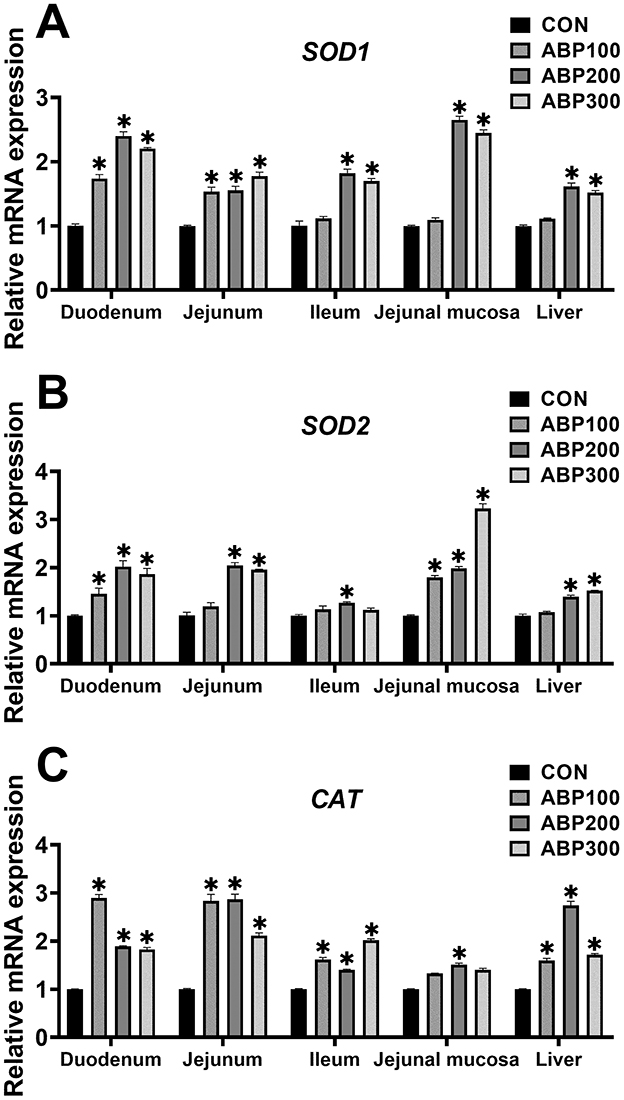
Figure 3. Relative mRNA expression of antioxidant relative genes in intestinal and liver in 84-day-old pigeons. The mRNA expression of the antioxidant genes SOD1 (A), SOD2 (B), and CAT (C). SOD1, Superoxide dismutase 1; SOD2, Superoxide dismutase 2; CAT, Catalase. Data are presented as mean ± SEM (n = 5). ns, p > 0.05; *p < 0.05 vs.CON.
4 Discussion
Antimicrobial peptides (AMPs) represent a promising alternative to antibiotics in livestock production, with demonstrated efficacy in enhancing gut health, immune function, and oxidative homeostasis across species (19–21). Pigeons are susceptible to bacterial infections in the early stages of growth, leading to a low survival rate, therefore, this study aimed to investigate the potential application of ABP MccJ25 in young pigeons. The percent study revealed that MccJ25 supplementation at 200 mg/kg exerted significant effects on intestinal barrier reinforcement, redox balance modulation, and humoral immunity enhancement, then improved survival rates in pigeons.
The improved intestinal morphology (villus height, V/C ratio) and upregulated tight junction proteins (OCLN, CLDN1, ZO-1) observed here are consistent with AMP-mediated gut protection mechanisms reported in poultry (10, 22, 23). It has been shown that the addition of ABPs in the diet remarkably increased the VH of jejunum, improved the V/C of duodenum and ileum, and significantly increased the expression of the intestinal tight junction protein genes (ZO-1, CLDN3, and MUC2) in broilers (24). Notably, the enhanced MUC2 expression suggests MccJ25 promotes mucin secretion, a critical component of the mucosal barrier against pathogens (25). The improved intestinal morphology and barrier function may be related to the intestinal flora, and ABP Plectasin significantly reduced the number of Escherichia coli (E. coli) in the ileum of yellow-feathered chickens, improved intestinal morphology, and significantly increased the expression of barrier genes ZO-1 and CLDN3 in the ileum (26). Similarly, this study found that ABP MccJ25 significantly increased the VH, decreased the V/C of small intestine, improved the intestinal morphology in pigeons and was conducive to intestinal absorption of nutrients, which may be the reason for the increase of its duodenal digestive enzyme activity. Recent studies highlight that polysaccharide-induced microbiota modulation alleviates oxidative organ damage by restoring microbial balance (27), while a study identified host-microbe interactions as key determinants of intestinal barrier integrity in swine (28). Although our study did not directly profile gut microbiota, the improved digestive enzyme activity (maltase, trypsin) and reduced systemic inflammation (lower AST/ALT) imply MccJ25 may exert prebiotic-like effects by suppressing pathogenic bacteria (e.g., E. coli) while favoring commensal species—a mechanism previously observed with plectasin in poultry (26). Future metagenomic analyses, as proposed by Huang et al. (28), could clarify MccJ25′s microbiota-specific impacts in pigeons.
Serum biochemical indicators indicate the nutritional metabolism and health status of the animal (29–31). Previous study showed that serum biochemical indicators were improved by the addition of ABPs in broilers (32). Similar results was obtained in this study that the addition ABP MccJ25 significantly increased the level of TP, ALB and GLB in pigeon serum, indicating that protein metabolism was vigorous and the degree of protein digestion and utilization was improved. It has been shown that ABPs significantly reduced TC content in the serum of weaned piglets (33), which potentially via AMP-mediated lipid regulation (34). Our observation of reduced serum TC/TG levels in MccJ25-supplemented pigeons further supports this result. It has been shown that ABPs elevated the activity of intestinal AMS, LPS and trypsin in broilers (10). This study showed that ABP MccJ25 increased the activity of duodenal AMS, maltase and LPS in pigeons and promoted the absorption and utilization of nutrients in the duodenum.
Serum AST and ALT reflect liver function or liver damage (35). In our study, ABP MccJ25 showed a significant decrease in the level of AST and ALT in pigeon serum, suggesting a protective effect of ABP MccJ25 on the liver. Immunoglobulins (IgG, IgA, IgM) are important components of the humoral immune system (10, 36). Previous studies found that ABP Plectasin significantly increased the levels of IgG and IgM in serum of broilers and weaned piglets (11). The use of ABP pratt and full-tide promotes the development of immune organs such as the bursa and thymus in broilers, and has the effect of preventing diseases and reducing mortality in broilers (24). Additionally, the elevated immunoglobulins (IgA, IgG, IgM) corroborate findings by Chen et al. (37). The present results were similar to the previous results, where ABP MccJ25 significantly increased the levels of IgA, IgG and IgM in pigeon serum, enhanced their humoral immune function, and increased the survival rate of the pigeons. This result corroborated the immune function of ABPs (2, 4, 20).
When the oxidative balance of the body is broken, it will cause oxidative stress in the organism, resulting in cell and tissue damage, which leads to a variety of diseases (38). SOD, GSH-PX, and CAT synergistically eliminate free radicals and peroxides to reduce oxidative damage (13). MDA is lipid peroxide produced by the metabolism of free radicals in the body (39), and its content is inversely proportional to the health status of the body. Consistent with previous studies, the results showed that ABP groups led to significant increases in T-AOC, GSH-Px, SOD, and CAT levels, while reducing MDA content in both pigeon serum and liver, and enhanced the oxidative defense system and scavenging capacity of the body against oxidative factors. It has been shown that ABPs improved the intestinal flora and showed resistance to bacterial infections, and also significantly increased the expression of intestinal antioxidant genes SOD1 and CAT to enhance the antioxidant capacity of broiler chickens (20). It was also found in this study, suggesting the enhanced antioxidant capacity of ABPs in pigeons. While the potential influence of confounding factors such as genetic variability and environmental conditions on experimental outcomes was existed, future studies will delineate microbiome differences or epigenetic modifications induced by these factors. Despite these limitations, our study provided robust support for the causality inferred between MccJ25 supplementation and the observed phenotypic improvements.
The survival rate improvement (6.7% increase in ABP200 vs. CON) holds significant economic potential for pigeon farming. ABP MccJ25 indirect health promotion via gut-liver axis modulation. Notably, the absence of adverse effects at 300 mg/kg contrasts with reports of high-dose AMP toxicity in mice (40, 41), suggesting pigeons may tolerate higher doses for therapeutic applications. However, pigeon-specific microbiota interactions, long-term safety, and comparative efficacy with structurally distinct AMPs (e.g., cLFchimera) warrant further investigation. Future studies should integrate multi-omics approaches to decode host-microbe crosstalk and clarify structure-function relationships in avian species.
5 Conclusion
The present study demonstrated that dietary supplementation with ABP MccJ25 improved serum biochemical indices, elevated intestinal digestive enzyme activity and strengthened systemic antioxidant capacity and ameliorated intestinal barrier integrity, thereby improved survival rates in pigeons. These results collectively highlight the roles of ABP MccJ25 in promoting intestinal health. Based on its dose-dependent efficacy, the inclusion of 200 mg/kg MccJ25 in diets is recommended as a sustainable strategy for improving productivity in pigeons. This investigation provides both theoretical and practical foundations for adopting ABP MccJ25 additives as alternatives in the pigeon industry.
Data availability statement
The original contributions presented in the study are included in the article/supplementary material, further inquiries can be directed to the corresponding author.
Ethics statement
The animal studies were approved by the Laboratory Animal Welfare and Ethics Committee of Nanjing Agricultural University, China. The studies were conducted in accordance with the local legislation and institutional requirements. Written informed consent was obtained from the owners for the participation of their animals in this study.
Author contributions
HC: Conceptualization, Investigation, Writing – original draft. YL: Resources, Writing – review & editing. XZ: Investigation, Writing – review & editing. FL: Formal analysis, Writing – review & editing. ML: Methodology, Writing – review & editing. JZ: Software, Writing – review & editing. HH: Formal analysis, Writing – review & editing. QM: Software, Writing – review & editing. MY: Conceptualization, Methodology, Writing – review & editing.
Funding
The author(s) declare that financial support was received for the research and/or publication of this article. This work was supported by the National Natural Science Foundation of China (32372825) and the Rural Revitalization of Industrial Development Fund of Nanjing Luhe, China (No. HY0075).
Acknowledgments
Thanks to the assistance of Shanjin Xu and Zaixu Pan from Nanjing Dongchen Pigeon Industry Co., Ltd. (Nanjing, China).
Conflict of interest
The authors declare that the research was conducted in the absence of any commercial or financial relationships that could be construed as a potential conflict of interest.
Generative AI statement
The author(s) declare that no Gen AI was used in the creation of this manuscript.
Publisher's note
All claims expressed in this article are solely those of the authors and do not necessarily represent those of their affiliated organizations, or those of the publisher, the editors and the reviewers. Any product that may be evaluated in this article, or claim that may be made by its manufacturer, is not guaranteed or endorsed by the publisher.
References
1. Zhang QY, Yan ZB, Meng YM, Hong XY, Shao G, Ma JJ, et al. Antimicrobial peptides: mechanism of action, sctivity and clinical potential. Military Med Res. (2021) 8:48. doi: 10.1186/s40779-021-00343-2
2. Talapko J, Meštrović T, Juzbašić M, Tomas M, Erić S, Horvat Aleksijević L, et al. Antimicrobial peptides—mechanisms of action, antimicrobial effects and clinical applications. Antibiotics. (2022) 11:1417. doi: 10.3390/antibiotics11101417
3. Zhu Y, Hao W, Wang X, Ouyang J, Deng X, Yu H, et al. Antimicrobial peptides, conventional antibiotics, and their synergistic utility for the treatment of drug-resistant infections. Med Res Rev. (2022) 42:1377–422. doi: 10.1002/med.21879
4. Luo Y, Song Y. Mechanism of antimicrobial peptides: antimicrobial, anti-inflammatory and antibiofilm activities. Int J Mol Sci. (2021) 22:11401. doi: 10.3390/ijms222111401
5. Daneshmand A, Kermanshahi H, Sekhavati MH, Javadmanesh A, Ahmadian M, Alizadeh M, et al. Effects of clfchimera peptide on intestinal morphology, integrity, microbiota, and immune cells in broiler chickens challenged with necrotic enteritis. Sci Rep. (2020) 10:17704. doi: 10.1038/s41598-020-74754-x
6. Yu H Shang L Yang G Dai Z Zeng X and Qiao S. Biosynthetic microcin J25 exerts strong antibacterial, anti-inflammatory activities, low cytotoxicity without increasing drug-resistance to bacteria target. Front Immunol. (2022) 13:811378. doi: 10.3389/fimmu.2022.811378
7. Yoon JH, Ingale SL, Kim JS, Kim KH, Lee SH, Park YK, et al. Effects of dietary supplementation of synthetic antimicrobial peptide-A3 and P5 on growth performance, apparent total tract digestibility of nutrients, fecal and intestinal microflora and intestinal morphology in weanling pigs. Livest Sci. (2014) 159:53–60. doi: 10.1016/j.livsci.2013.10.025
8. Silveira RF, Roque-Borda CA, Vicente EF. Antimicrobial peptides as a feed additive alternative to animal production, food aafety and public health implications: an overview. Anim Nutr. (2021) 7:896–904. doi: 10.1016/j.aninu.2021.01.004
9. Patyra E, Kwiatek K. Insect meals and insect antimicrobial peptides as an alternative for antibiotics and growth promoters in livestock production. Pathogens. (2023) 12:854. doi: 10.3390/pathogens12060854
10. Ma JL, Zhao LH, Sun DD, Zhang J, Guo YP, Zhang ZQ, et al. Effects of dietary supplementation of recombinant plectasin on growth performance, intestinal health and innate immunity response in broilers. Probiotics Antimicro. (2020) 12:214–23. doi: 10.1007/s12602-019-9515-2
11. Yuan W, Jin H, Ren Z, Deng J, Zuo Z, Wang Y, et al. Effects of antibacterial peptide on humoral immunity in weaned piglets. Food Agric Immunol. (2015) 26:682–9. doi: 10.1080/09540105.2015.1007448
12. Yu H, Ding X, Shang L, Zeng X, Liu H, Li N, et al. Protective ability of biogenic antimicrobial peptide microcin J25 against enterotoxigenic escherichia coli-induced intestinal epithelial dysfunction and inflammatory responses ipec-J2 cells. Front Cell Infect Mi. (2018) 8:242. doi: 10.3389/fcimb.2018.00242
13. Jiang SG, Pan NX, Chen MJ, Wang XQ, Yan HC, Gao CQ. Effects of dietary supplementation with dl-methionine and dl-methionyl-dl-methionine in breeding pigeons on the carcass characteristics, meat quality and antioxidant activity of squabs. Antioxidants. (2019) 8:435. doi: 10.3390/antiox8100435
14. Chang L, Tang Q, Zhang R, Fu S, Mu C, Shen X, et al. Evaluation of meat quality of local pigeon varieties in China. Animals. (2023) 13:1291. doi: 10.3390/ani13081291
15. Zhang R, Ma H, Han P, Li Y, Sun Y, Yuan J, et al. Effects of feed systems on growth performance, carcass characteristics, organ index, and serum biochemical parameters of pigeon. Poult Sci. (2022) 101:102224. doi: 10.1016/j.psj.2022.102224
16. Jin CL, He YA, Jiang SG, Wang XQ, Yan HC, Tan HZ, et al. Chemical composition of pigeon crop milk and factors affecting its production: a review. Poult Sci. (2023) 102:102681. doi: 10.1016/j.psj.2023.102681
17. Gao CQ, Yang JX, Chen MX, Yan HC, Wang XQ. Growth curves and age-related changes in carcass characteristics, organs, serum parameters, and intestinal transporter gene expression in domestic pigeon (columba livia). Poult Sci. (2016) 95:867–77. doi: 10.3382/ps/pev443
18. Cao H, Lu YL, Zhang XY, Zhou J, Li F, Pan ZX, et al. Effects of antibacterial peptide microcin J25 on growth performance, antioxidant capacity, intestinal barrier function and intestinal microbiota in pigeon squabs. Ital J Anim Sci. (2024) 23:427–39. doi: 10.1080/1828051X.2024.2326119
19. Aslam B, Wang W, Arshad MI, Khurshid M, Muzammil S, Rasool MH, et al. Antibiotic resistance: a rundown of a global crisis. Infect Drug Resistance. (2018) 11:1645–58. doi: 10.2147/IDR.S173867
20. Zhu CL, Bai YL, Xia XJ, Zhang M, Wu XL, Wu YD, et al. Effects of the antimicrobial peptide mastoparan X on the performance, permeability and microbiota populations of broiler chickens. Animals. (2022) 12:16. doi: 10.3390/ani12243462
21. Bondad-Reantaso MG, MacKinnon B, Karunasagar I, Fridman S, Alday-Sanz V, Brun E, et al. Review of alternatives to antibiotic use in aquaculture. Rev Aquac. (2023) 15:1421–51. doi: 10.1111/raq.12786
22. Cheng F, Qiao Z, Liang G, Li J, Qiao Y, Yun S, et al. Polysaccharide from sparassis latifolia alleviates intestinal barrier dysfunction in mice exposed to lead. Int J Biol Macromol. (2023) 253:127615. doi: 10.1016/j.ijbiomac.2023.127615
23. Tian Y, Zhang R, Li G, Zeng T, Chen L, Xu W, et al. Microbial fermented feed affects flavor amino acids and yolk trimethylamine of duck eggs via cecal microbiota-yolk metabolites crosstalk. Food Chem. (2024) 430:137008. doi: 10.1016/j.foodchem.2023.137008
24. Xie Z, Zhao QQ, Wang H, Wen LJ Li W, Zhang XH, et al. Effects of antibacterial peptide combinations on growth performance, intestinal health, and immune function of broiler chickens. Poult Sci. (2020) 99:6481–92. doi: 10.1016/j.psj.2020.08.068
25. Wang Y, Ji X, Zhao M, Li J, Yin H, Jin J, et al. Modulation of tryptophan metabolism via ahr-Il22 pathway mediates the alleviation of dss-induced colitis by chitooligosaccharides with different degrees of polymerization. Carbohyd Polym. (2023) 319:121180. doi: 10.1016/j.carbpol.2023.121180
26. Zhang X, Zhao Q, Wen L, Wu C, Yao Z, Yan Z, et al. The effect of the antimicrobial peptide plectasin on the growth performance, intestinal health, and immune function of yellow-feathered chickens. Front Vet Sci. (2021) 8:688611. doi: 10.3389/fvets.2021.688611
27. Li L, Guo WL, Zhang W, Xu JX, Qian M, Bai WD, et al. Correction: grifola frondosa polysaccharides ameliorate lipid metabolic disorders and gut microbiota dysbiosis in high-fat diet fed rats. Food Funct. (2024) 15:10553. doi: 10.1039/D4FO90096K
28. Huang L, Luo S, Liu S, Jin M, Wang Y, Zong X. Comparative multiomics analyses reveal the breed effect on the colonic host–microbe interactions in pig. iMetaOmics. (2024) 1:e8. doi: 10.1002/imo2.8
29. Song HJ Li WT, Li YF, Zhai B, Guo YJ, Chen Y, et al. Genome-wide association study of 17 serum biochemical indicators in a chicken F2 resource population. BMC Genomics. (2023) 24:12. doi: 10.1186/s12864-023-09206-7
30. Al-Garadi MA, Suliman GM, Hussein EO, Al-Owaimer AN, Swelum AA, Almalamh NA, et al. The effects of betaine and nano-emulsified plant-oil supplementation on growth performance and serum biochemistry indices of heat-stressed broiler chickens. Ital J Anim Sci. (2023) 22:398–406. doi: 10.1080/1828051X.2023.2206417
31. Li L, Guo WL, Zhang W, Xu JX, Qian M, Bai WD, et al. Grifola frondosa polysaccharides ameliorate lipid metabolic disorders and gut microbiota dysbiosis in high-fat diet fed rats. Food Funct. (2019) 10:2560–72. doi: 10.1039/C9FO00075E
32. Sholikin MM, Sadarman S, Irawan A, Prihambodo TR, Qomariyah N, Wahyudi AT, et al. Antimicrobial peptides as an additive in broiler chicken nutrition: a meta-analysis of bird performance, nutrient digestibility and serum metabolites. J Anim Feed Sci. (2021) 30:100–10. doi: 10.22358/jafs/136400/2021
33. Wang JH, Wu CC, Feng J. Effect of dietary antibacterial peptide and zinc-methionine on performance and serum biochemical parameters in piglets. Czech J Anim Sci. (2011) 56:30–6. doi: 10.17221/341/2009-CJAS
34. Chu H, Du C, Yang Y, Feng X, Zhu L, Chen J, et al. Mc-Lr aggravates liver lipid metabolism disorders in obese mice fed a high-fat diet via Pi3k/Akt/Mtor/Srebp1 signaling pathway. Toxins. (2022) 14:833. doi: 10.3390/toxins14120833
35. Tan JJ, Hu RZ, Gong JT, Fang CK Li YL, Liu M, et al. Protection against metabolic associated fatty liver disease by protocatechuic acid. Gut Microbes. (2023) 15:16. doi: 10.1080/19490976.2023.2238959
36. Zhao C, Li H, Gao C, Tian H, Guo Y, Liu G, et al. Moringa oleifera leaf polysaccharide regulates fecal microbiota and colonic transcriptome in calves. Int J Biol Macromol. (2023) 253:127108. doi: 10.1016/j.ijbiomac.2023.127108
37. Chen F, Wang Y, Wang K, Chen J, Jin K, Peng K, et al. Effects of litsea cubeba essential oil on growth performance, blood antioxidation, immune function, apparent digestibility of nutrients, and fecal microflora of pigs. Front Pharmacol. (2023) 14:1166022. doi: 10.3389/fphar.2023.1166022
38. Li QH, Yang SW, Chen F, Guan WT, Zhang SA. Nutritional strategies to alleviate oxidative stress in sows. Anim Nutr. (2022) 9:60–73. doi: 10.1016/j.aninu.2021.10.006
39. Wang Z, Guo L, Ding X, Li F, Xu H, Li S, et al. Supplementation of chestnut tannins in diets can improve meat quality and antioxidative capability in Hu lambs. Meat Sci. (2023) 206:109342. doi: 10.1016/j.meatsci.2023.109342
40. Yu HT, Zhang JQ, Sun MC, Chen H, Shi XM, You FP, et al. Polymeric nanohybrids engineered by chitosan nanoparticles and antimicrobial peptides as novel antimicrobials in food biopreservatives: risk assessment and anti-foodborne pathogen escherichia coli O157:H7 infection by immune regulation. J Agric Food Chem. (2022) 70:12535–49. doi: 10.1021/acs.jafc.2c05308
Keywords: antioxidant capacity, biochemical function, feed additive, microcin J25, pigeon
Citation: Cao H, Lu Y, Zhang X, Li F, Li M, Zhou J, He H, Ma Q and Yu M (2025) Dietary supplementation with antibacterial peptide microcin J25 improved antioxidant capacity and intestinal health of pigeons. Front. Vet. Sci. 12:1550776. doi: 10.3389/fvets.2025.1550776
Received: 24 December 2024; Accepted: 07 April 2025;
Published: 25 April 2025.
Edited by:
Valiollah Palangi, Ege University, TürkiyeReviewed by:
Baseer Ahmad, Muhammad Nawaz Shareef University of Agriculture, PakistanMoyosore Joseph Adegbeye, University of Africa, Nigeria
Copyright © 2025 Cao, Lu, Zhang, Li, Li, Zhou, He, Ma and Yu. This is an open-access article distributed under the terms of the Creative Commons Attribution License (CC BY). The use, distribution or reproduction in other forums is permitted, provided the original author(s) and the copyright owner(s) are credited and that the original publication in this journal is cited, in accordance with accepted academic practice. No use, distribution or reproduction is permitted which does not comply with these terms.
*Correspondence: Minli Yu, eXVtaW5saUBuamF1LmVkdS5jbg==
†These authors have contributed equally to this work