- 1Physiology, College of Veterinary Medicine, Lincoln Memorial University, Harrogate, TN, United States
- 2KCA Laboratories, Nicholasville, KY, United States
Introduction: This study investigated the pharmacokinetics, safety, and tolerability of a full-spectrum CBD-dominant oil formulated in medium-chain triglycerides (MCT oil) after a single intravenous (IV) administration, a single oral (PO) administration, and multiple oral administrations of CBD at a dose of 2.2 mg/kg in adult male and female beagle dogs.
Methods: The CBD-dominant extract was administered to adult, intact beagle dogs (male n = 4, female n = 2) once intravenously, once orally, and every 12 h orally for 21 days at a dose of 2.2 mg CBD/kg body weight (BW). Blood samples were collected at predetermined times to measure concentrations of serum CBD, 7-hydroxy-CBD (7-OH-CBD), 7-nor-7-carboxy-CBD (7-COOH-CBD), Δ9-tetrahydrocannabinol (Δ9-THC), 11-hydroxy-THC (11-OH-THC), and 11-carboxy-THC (11-COOH-THC). Serum CBD and Δ9-THC concentrations were analyzed to estimate various pharmacokinetic parameters. Selected physical, behavioral, hematologic, and blood chemical measurements were obtained before and during single and repeated dose administrations.
Results: Pharmacokinetics of CBD after IV administration indicated a median (range) systemic clearance (CL) of 7.06 (6.14–10.5) mL/min/kg, a steady-state volume of distribution (Vss) of 2.13 (1.10–2.85) L/kg, and a half-life of 291 (183–508) min. The median (range) extent of systemic availability of CBD after a single oral dose was 31.2 (17.7–35.7)%. Pharmacokinetics of Δ9-THC after IV administration were characterized by a CL of 8.85 (6.88–14.4) mL/min/kg, Vss of 1.98 (1.30–2.30) L/kg, and a half-life of 169 (139–476) min. The extent of systemic availability of Δ9-THC after PO administration was 40.9 (20.5–46.2)%. The test article was well tolerated in all dogs during the study. Although serum alkaline phosphatase concentrations increased during the repeated PO dose study, they remained within normal limits.
Discussion: Both CBD and Δ9-THC were rapidly cleared after IV administration and exhibited extensive volumes of distribution. Comparison of clearance to serum hepatic blood flow estimated the hepatic extraction ratio and extent of first pass metabolism after PO administration, which was confirmed by analyzing the single PO dose pharmacokinetic data. The AUC0−∞ for 7-OH-CBD after single IV compared to single PO dose was not different, suggesting complete absorption of CBD from the formulation in MCT oil when administered with canned dog food.
1 Introduction
Cannabis (Cannabis sativa L.) is an annual herbaceous plant that has been used for various purposes (e.g., fiber, oil, medical, nutrition, ritual or ceremonial, and recreation) for thousands of years (1–3). Cannabis contains over 480 identified substances including more than 100 phytocannabinoids (1, 2), 120 terpenoids, flavonoids, omega-3- and omega-6 fatty acids, alkaloids, and other substances (1, 2, 4–21). Among the phytocannabinoids are the psychoactive (-)-trans-Δ9-tetrahydrocannabinol (Δ9-THC) and the non-psychoactive cannabidiol (CBD) (6, 7). Despite the abundance of potential pharmacologically active substances in C. sativa L., only two cannabinoids have been approved by the US Food and Drug Administration (FDA) for therapeutic use in humans. These FDA-approved products are Epidiolex® (cannabidiol, Greenwich Biosciences, Inc., Carlsbad, CA) and Marinol® (dronabinol, Solvay Pharmaceuticals, Marietta, GA). Furthermore, recent legislation has increased the use of cannabinoids in both humans and animals due to changes in the regulation of hemp.
The Agricultural Improvement Act of 2018 (commonly known as the 2018 Farm Bill) defined hemp as C. sativa L. containing < 0.3 percent Δ9-THC on a dry weight basis and removed hemp and hemp-derived products from Drug Enforcement Administration Schedule I status under the Controlled Substances Act. Thus, the 2018 Farm Bill marked a profound change in the regulation of cannabis in the US, established a pathway for the cultivation and interstate distribution of hemp-derived products that led to a substantial increase in the commercial availability of hemp products formulated for administration to pet animals. Consequently, results of the findings from pharmacokinetic studies investigating the disposition of CBD and its metabolites in dogs and cats have been reported (22–41). However, only one study has included a description of the pharmacokinetics of CBD in the same dogs after both IV and PO administration (42). Therefore, total clearance, volumes of distribution, elimination half-life after IV administration, and the extent of systemic availability after PO administration have not been subsequently determined although more sensitive methods of analysis for CBD and its metabolites have been implemented.
The endocannabinoid system (ECS) is a homeostatic neuroregulatory network of endogenous signaling lipids (endocannabinoids), cannabinoid (CB) and non-cannabinoid G-protein (GP) coupled receptors (e.g., CB1R, CB2R, GPR 55, and GPR119), and enzymes that regulate endocannabinoid biosynthesis and catabolism (43–49). Cultivars of C. sativa L. produce hundreds of biologically active phytocannabinoids, terpenes, and flavonoids (24, 50). Commercially prepared cannabis extracts contain bioactive phytocannabinoids (e.g., Δ9 THC, CBD) marketed as “full-spectrum,” “broad spectrum,” or individual isolates that are promoted for the treatment of chronic osteoarthritis, epilepsy, neuropathic pain, emesis, behavioral abnormalities, and skin diseases (51, 52). Full-spectrum products typically contain the psychoactive cannabinoid Δ9-THC, < 0.3 percent by US law, and non-psychoactive cannabinoids (e.g., CBD, cannabigerol (CBG), cannabichromene (CBC), other phytocannabinoids, and terpenes (51, 53). Broad-spectrum products contain non-psychoactive phytocannabinoids without Δ9-THC whereas isolates contain a single, highly purified phytocannabinoid (e.g., CBD). Differences in product formulation (i.e., phytocannabinoid constituents and their concentrations) are confounded by the interactions of phytocannabinoids with other C. sativa L. constituents including terpenes and flavonoids and by their effects on hepatic metabolism (i.e., CYP induction or inhibition) (54). For example, CBD modulates the effects of Δ9-THC through negative allosteric binding at CB1Rs and inhibition of various CYPs (e.g., CYP1A, CYP2B, CYP2D, and CYP3A) mediated metabolism provoking phytocannabinoid-drug interactions (25, 55–57). The variability in biological effects is due to product type, formulation, dose, route of administration, diet (e.g., food effect, fat source and its concentration), experimental conditions, and the recipient's health status. These factors create a highly complex scenario that necessitates properly designed, product dependent, and species specific pharmacokinetic and pharmacodynamic studies to identify and provide meaningful clinical guidance in their use (24, 26, 39, 41, 52, 58, 59). The primary objectives of this study were to investigate the pharmacokinetics and bioavailability of a full-spectrum Δ9-THC:CBD (1:40, w/w) product after intravenous (IV) and PO administration once and orally twice daily for 21 days. The secondary objective was to monitor selected oxidative metabolites and monitor physical, behavioral, hematologic, and blood chemical variables.
2 Materials and methods
2.1 Animal care and use
All animal procedures were approved by the East Tennessee Clinical Research institutional IACUC committee (PT-21-01). Animals were housed in the AAALAC-accredited facilities at the East Tennessee Research Center and maintained according to the Animal Welfare Act (60) and the Guide for the Care and Use of Laboratory Animals (61).
2.2 Dogs and outcome assessment
Six, purpose-bred, intact, 1–2-year-old beagle dogs (4 males, 2 females) weighing (mean; range) (10.7 kg; 7.5–11.2 kg) were used All dogs were individually housed and underwent a 7-day acclimation period before the study began. Diet, water, environment, and management were maintained throughout the study. A physical examination performed once during the acclimation period included body temperature, thoracic auscultation (heart and respiratory rate), and assessment of major organ systems (see Figure 1). Body weights were recorded on study days −7, −1, 7, 16, 24, and 31 (Figure 1). Body weights measured before each phase were used for dose calculations of CBD. A body condition score (BCS) was assigned on the mornings of study days −7, −1, 7, 16, 24, 31, and 38 using the Purina Body Condition Scoring system (https://www.purinainstitute.com; Figure 1).
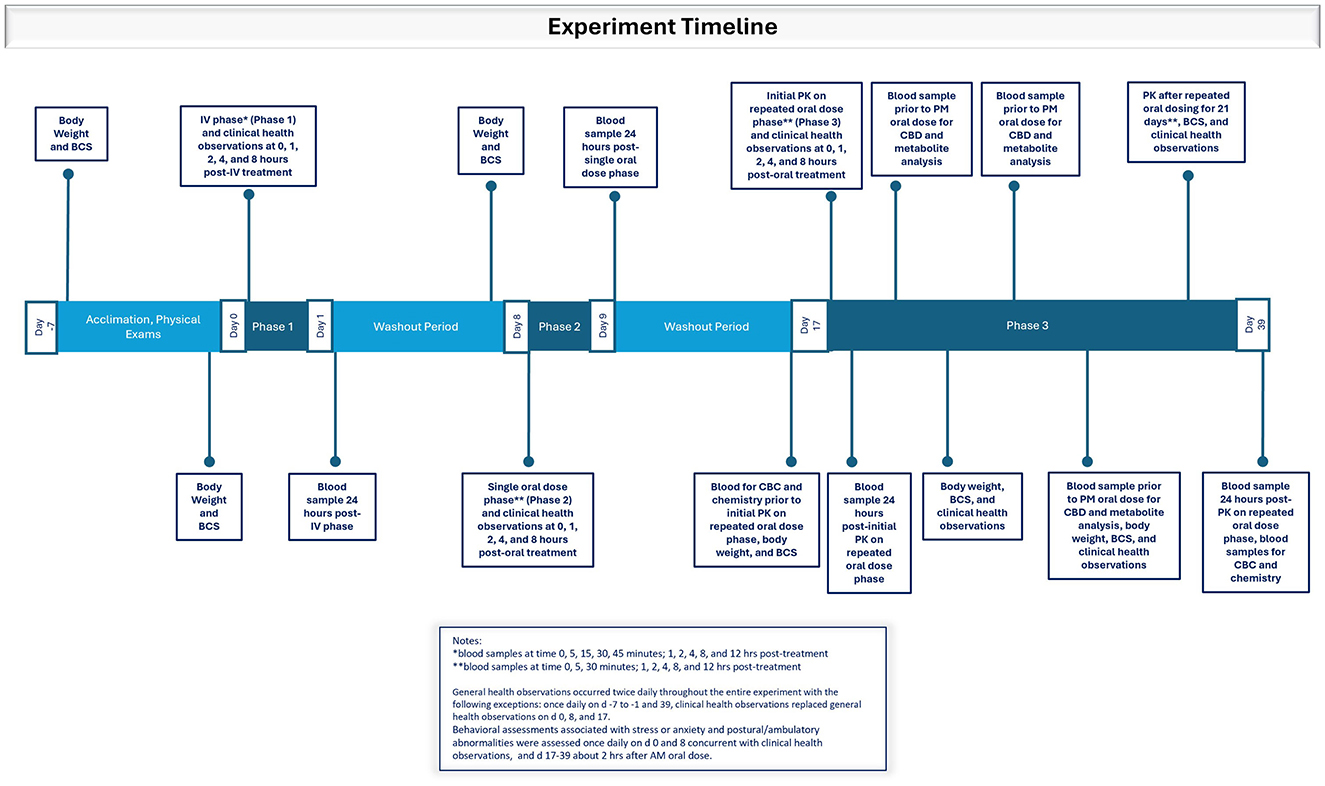
Figure 1. Timeline depicting experimental events during the acclimation period and Phases 1, 2, and 3 in which dogs were dosed with the test article dissolved in ethanol intravenously or orally in a small amount of wet dog food as a single dose or twice daily in an oral dosing regimen.
Blood samples were obtained for a hemogram and blood chemical analysis on days 16 and 39 (Figure 1). Hematologic analysis included total leukocyte count, differential leukocyte count (percentage and absolute), packed cell volume, erythrocyte count, mean corpuscular volume, mean corpuscular hemoglobin, mean corpuscular hemoglobin concentration, hemoglobin concentration, and thrombocyte count. Blood chemical analysis included total protein, albumin, albumin/globulin ratio, globulin, blood urea nitrogen (BUN), BUN/creatinine ratio, creatinine, gamma glutamyl transferase, phosphorus, total bilirubin, alkaline phosphatase, alanine aminotransferase, aspartate aminotransferase, cholesterol, creatine phosphokinase, glucose, lactate dehydrogenase, sodium, potassium, sodium/potassium ratio, calcium, and chloride.
All dogs were fed Purina Pro Plan Adult Complete Essentials Shredded Blend Chicken & Rice dry dog food (minimum 18.2% crude fat, 29.5% crude protein dry matter basis) twice daily during the acclimation period and throughout the study. Dogs were fed immediately after each PO dose in the single and multiple dose phases. Water was available ad libitum.
Approximately 10–20 g of canned dog food1 (minimum 31.8% crude fat, 36.4% crude protein dry matter basis) was fed during Phases 2 and 3 to deliver the test article.
General health observations were recorded twice daily throughout the study with the following exceptions: once daily on study days −7 to −1 and study day 39, and clinical health observations replaced general health observations on study days 0, 8, and 17 (Figure 1). During general health observations, dogs were observed for signs of abnormal health and fecal consistency was scored (7-point scale). During clinical health observations, dogs were observed for signs of salivation, lethargy, tremors, diarrhea or vomiting, and unusual behavior. On days when clinical health observations were recorded, dogs were observed before treatment administration and at 1-, 2-, 4-, and 8-h post-treatment (± 5 min through 4 h; ± 15 min for 8 h post dosing). Abnormal health observations after administration of the test article were considered an adverse event.
Behavioral assessments associated with stress or anxiety and postural/ambulatory abnormalities were assessed once daily on study days 0 and 8 concurrent with clinical health observations, and on study days 17–39 (Phase 3) approximately 2 h after the morning PO dose (Figure 1). Behavioral assessments included hyperactivity, salivation, cowering/fearful posture, pacing, aggression, vocalization, panting, vomiting/atypical elimination, hypervigilance, shaking, and self-trauma or mutilation (e.g., tail biting, nail biting, flank sucking). The assessment scale was 0–5, with 0 = not present and 5 = extensive amount/severe. Postural and ambulatory assessments were assessed on a scale of 0–6, with 0 = no effect and 6 = lies prostrate on the floor.
2.3 Test article
The test article was prepared in a single batch from a fully characterized “full-spectrum” extract of C. sativa L. at a nominal CBD concentration of 100 mg/mL in medium-chain triglycerides (MCT) oil from coconut oil by a 3rd party-contractor. The test article used in the study was analyzed by an ISO/IEC 17025 accredited commercial laboratory.2 The test article contained the cannabinoids CBD (100 mg/mL), CBC (3.08 mg/mL), Δ9-THC (1.53 mg/mL), CBDV (1.41 mg/mL), CBG (0.334 mg/mL) and CBN (0.107 mg/mL), and the terpenes (-)-alpha-bisabolol (10.6 mg/mL), beta-caryophyllene (5.24 mg/mL), (-)-guaiol (3.18 mg/mL), (-)-caryophyllene oxide (3.02 mg/mL), humulene (2.05 mg/mL), nerolidol (0.67 mg/mL), and limonene (0.48 mg/mL). No acidic cannabinoids were detected above the limit of detection (~0.001%). No heavy metal or solvent residues, mycotoxins, or pesticides were detected in the test article.
The IV dose was prepared for each dog by dissolving the requisite volume of the test article in 70% ethanol and filtering through a 0.22 μm syringe nylon filter immediately before IV administration. The IV dose of CBD (measured in μL of the test article in ethanol) was administered by bolus injection into a non-catheterized cephalic vein.
To prepare the oral CBD dose for each dog at each dose administration, the required volume of the test article (approximately 220 μL per dog per dose) was measured using a high-precision 1,000 μL micropipette. This volume was then dispensed into 10–20 g of canned dog food and thoroughly mixed to ensure even distribution. The time of administration (i.e., the time at which the dog had consumed the entire dose), the dose of CBD, the volume of the test article, and the weight of the canned food were recorded for each dose. The dry food (Purina Pro Plan Adult Complete Essentials Shredded Blend Chicken & Rice) was offered to each dog after it had consumed the medicated canned food.
2.4 Study design
The study design was a non-randomized, unmasked, three-phase study to determine the IV and PO pharmacokinetics of CBD in MCT oil in six beagle dogs. The dose rate of CBD was 2.2 mg/kg at each dose. The same dogs were used in all three phases of the study. Target analytes included CBD, Δ9-THC, 7-OH-CBD, 7-COOH-CBD, 11-OH-THC, and 11-COOH-THC. Dogs were observed twice daily during each treatment phase of the study and were fed to maintain a healthy weight and body condition score throughout the study.
Phase 1: Each dog was administered the test article IV diluted in ethanol. The dose rate of CBD was 2.2 mg/kg. Blood samples were collected from the opposite catheterized cephalic vein at 0, 5, 15, 30, 45 min and 1, 2, 4, 8, and 12 h post-treatment for determination of serum analyte concentrations (Figure 1). Phase 1 was followed by a 7-day washout period.
Phase 2: Each dog was administered the test article PO once in canned dog food as previously described. Blood samples were collected at 0, 5, 30 min and 1, 2, 4, 8, and 12 h after dosing. Phase 2 was followed by a 7-day washout period.
Phase 3: Each dog was administered the test article PO twice daily (every 12 h) for 21 consecutive days in canned dog food as previously described. Blood samples were collected on the first (study day 17) and last (study day 38) days of treatment on the same collection schedule as for Phase 2 (Figure 1). For the evening PO dose on study day 17 and study day 38, the 12-h blood collection occurred immediately before administration of the dose. Blood samples were collected immediately before the PM PO dose on study days 21, 26, and 31 (days 5, 10, and 15 after initiation of Phase 3) for determinations of all target analytes (Figure 1). All dogs were returned to the facility colony after the study (i.e., study day 39) and were managed in compliance with testing facility procedures.
2.5 Blood sample collection and preparation
Intravenous catheters were inserted into a cephalic vein after sedation with IM butorphanol (0.2 mg/kg) before blood collections on study days 0, 8, 17, and 38. The catheters were secured by bandaging, and patency was maintained with heparinized saline flush solution. Elizabethan collars were applied, and the subjects were observed frequently to prevent damage to the catheters. Catheters were removed after collection of the T+12-h sample.
Approximately 3 mL of blood was aspirated from the catheter using a 3-mL syringe, or by direct venipuncture using a 20 ga 1-inch needle (62). The time of each sample collection was recorded on the PK Sample Collection Record.
The blood was dispensed into an evacuated serum tube (red top) containing no anti-coagulants immediately following collection. Each tube remained at room temperature for ~1 h or in a water bath at ~38°C for a similar interval to facilitate clotting. The tubes were centrifuged at approximately 3,600 g for 10 min at room temperature. The serum from each tube was aspirated with disposable pipettes, divided equally between two cryovials, and frozen at −80°C. Collection tubes and cryovials were labeled with the study number, dog I.D., day or date, nominal sampling interval, and sequence (i.e., 1 of 2, 2 of 2). Collection data were recorded on the Serum Processing Record.
2.6 Sample analysis
Serum sample analyses for CBD, Δ9-THC and two metabolites of each were performed by a liquid chromatography-mass spectrometry method developed and validated for pharmacokinetic studies in dogs. The serum concentrations of all analytes are reported as total concentration (i.e., free plus protein-bound concentrations). See the Supplementary material for details of the method.
2.7 Method validation study
The method was validated based on the M10 Bioanalytical Method Validation and Study Sample Analysis Guidance for Industry.3 The study determined the specificity and robustness, the limit of detection (LOD), the limit of quantitation (LOQ), linearity and the linear range, accuracy, repeatability and intermediate precision, and dilution integrity. The results of the method validation study are reported in the Supplementary material.
2.8 Pharmacokinetic analysis
Non-compartmental pharmacokinetic analysis was used to determine the pharmacokinetics of CBD whereas compartmental pharmacokinetic analysis was used to determine the pharmacokinetics of Δ9-THC after single IV and PO because conventional compartmental models could not be fit to the data. Total clearance (CLT), volumes of distribution (compartmental volumes and steady-state volume of distribution), macro- and micro-constants, and half-lives of CBD and Δ9-THC after IV administration and absorption rate, lag time, and extent of systemic availability after PO treatment were determined by compartmental methods. Non-compartmental pharmacokinetic analysis included determination of AUCt using the log-linear trapezoidal method with linear interpolation using pharmacokinetic analysis software (PKSolver, Department of Pharmaceutics, China Pharmaceutical University, E-mail: cGtzb2x2ZXJAY3B1LmVkdS5jbg==). Non-compartmental pharmacokinetic parameters for CBD and metabolites included the maximum serum concentration (Cmax), time at which Cmax was observed (Tmax), lambda z (λz), AUC0-t, and terminal half-life (t1/2). Cmax and Tmax were determined from model calculations or non-compartmental model analysis.
Based on a previous report for estimating the hepatic extraction ratio ER for CBD in dogs (42), the hepatic extraction ratio ER for each dog was estimated from its CLT (assuming that CLT represents hepatic clearance since hepatic metabolism of CBD is extensive and renal clearance of CBD is negligible), its hematocrit (HCT), and an estimate of liver blood flow in dogs (i.e., 30–45 mL/min/kg) (63, 64). The hepatic serum flow rates were estimated by multiplying the hepatic blood flow by (1-HCT) where HCT was the average of two determinations for each dog. The CLT determined for each dog was then divided by the hepatic serum flow to obtain the estimate of the hepatic ER for each dog.
Serum concentrations of 11-OH-THC, and 11-nor-9-COOH-THC were less than LOQ in all samples collected after Treatments 1 and 2. Serum concentrations of CBD, 7-OH-CBD, 7-COOH-CBD, and Δ9-THC were above the LOQ, and are reported. AUC values and half-lives of metabolites were calculated where sufficient data were available.
The accumulation ratio AR during the multiple dose PO trial was estimated using AUC0 − 12h data from the first dose and the next to last dose in the multiple dosing regimen as follows (65, 66):
2.9 Statistical analysis
Statistical analyses were performed using statistical analysis software4 to determine statistical significance (p ≤ 0.05).
Selected complete blood count and serum chemistry test results measured immediately before the first dose and immediately after the last dose in Phase 3 were analyzed using two-tailed paired t-tests. Clearances in female and male dogs were compared using the two-tailed unpaired t-test. AUC values of CBD for 12 h after the first and last dose and ALP concentrations before and after the multiple dosing regimen (Phase 3) were compared by the two-tailed paired t-test to determine significance. The normality of residuals was assessed by the Shapiro-Wilk and the Kolmogorov-Smirnov tests.
A Student's t-test for paired samples was performed to assess differences between pharmacokinetic parameter estimates after PO and IV administration, and the Kolmogorov–Smirnov test was used to determine normality of the data. A non-parametric Wilcoxon signed-rank test for paired samples was used for Tmax, which is not a continuous variable, and was not normally distributed.
Serum concentrations of analytes collected at different times during Phase 3 were compared using repeated measures one-way ANOVA with multiple comparisons using Tukey's test to correct for multiple comparisons. Mean differences were considered statistically significant at P ≤ 0.05.
3 Results
No differences in body weight or body condition scores were observed during the study. The mean body weight on day −2 was 10.7 kg and on day 34 was 10.6 kg. All dogs consumed their food, including canned food mixed with the test article in Phases 2 and 3.
3.1 Hemogram and serum chemistry
The hemogram and serum biochemistry values were within normal ranges on day 16 (before starting Phase 3) and on day 39 (end of Phase 3). Alkaline phosphatase (ALP) was elevated (P = 0.0016) in all dogs at the end of Phase 3 compared to the day before the start of Phase 3 (Figure 2) although all values remained within the reference range. The ALP ranged from 29–51 U/L with a median value of 36.5 U/L on the day before the start of Phase 3 (i.e., day 16). At the end of Phase 3 (i.e., day 39) it ranged from 45–76 U/L, with a median value of 58 U/L. The mean and the 95% confidence interval for the increase in ALP were 22.2 U/L and 13.0–31.3 U/L, respectively.
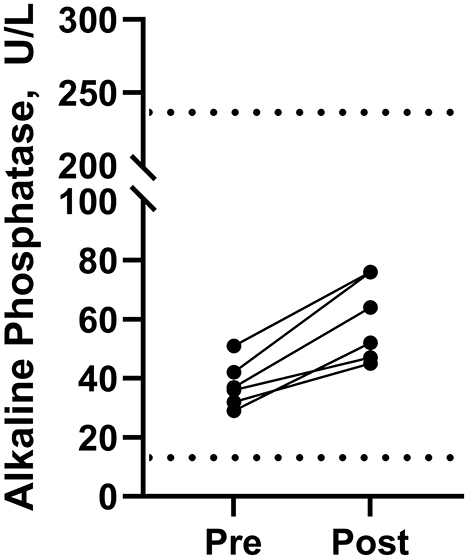
Figure 2. Serum alkaline phosphatase concentrations in beagle dogs immediately before (Pre) and immediately after the last dose (Post) in Phase 3 (2.2 mg of cannabidiol orally every 12 h). Normal limits for alkaline phosphatase in dogs are shown by the dashed lines (····) at 13 and 240 U/L.
3.2 Body condition, behavioral, and adverse events
The mean body condition score was 5.2 on study day −1 and 5.1 on study day 38. The test article produced no effects on postural and ambulatory measurements as all were assigned a score of 0. Some changes in behavior were noted during the study. Mild salivation was reported in three dogs in Phase 1, 1 and 2 h after IV injection and one dog 4 h after IV injection. Salivation was noted in one dog in Phase 2, 1 h after PO dosing, but no other behavioral changes were reported at 2, 4, or 8 h after PO dosing in Phase 2. A transient period of pacing was noted in one dog in Phase 3, 8 h after PO dosing but no other behavioral changes were reported at 1, 2, or 4 h after dosing.
Seven adverse events involving observation of mucus in feces, unformed feces, and regurgitation or vomiting were recorded during the study. On the day after the IV injection, blood and mucus were observed in the feces of one dog and mucus was observed in the feces of two dogs. On study day 9, 2 days after the single PO dose in Phase 2, unformed fecal matter was noted in one dog. On study day 17, on the first day of dosing in Phase 3, one dog regurgitated 1 h after dosing. During the repeated PO dose study, one dog vomited on the 9th day and one dog produced unformed feces on the 12th day.
3.3 Pharmacokinetic study
3.3.1 Serum CBD, Δ9-THC, and metabolites after single dose intravenous administration of the test article–Phase 1
Serum concentrations of CBD, 7-OH-CBD, 7-COOH-CBD, Δ9- THC, 11-OH-THC, and 11-nor-COOH-THC after IV administration of CBD at a dose of 2.2 mg/kg BW were determined by a validated LC-MS/MS method. CBD and 7-COOH-CBD were detected in all samples collected after IV dosing, and 7-OH-CBD was detected in all samples except for 2 of 6 samples collected 24 h after IV dosing. Furthermore, Δ9-THC was detected in serum samples from all dogs and most samples except samples collected at 5 min and 12 and 24 h after IV dosing (which contained Δ9-THC). 11-OH-THC was quantified (LOQ 0.2 ng/mL) in all samples collected through 8–24 h after IV dosing, and 11-nor-9-COOH-THC was not detected (LOQ 1 ng/mL) in any sample collected after IV dosing of the test article.
Plots of log serum concentrations of CBD, 7-OH-CBD, and 7-COOH-CBD vs. time after single IV administration of CBD at a dose of 2.2 mg/kg BW are reported in Figure 3. Furthermore, plots of log serum concentrations of Δ9-THC and 11-OH-THC vs. time after single IV administration of 54 μg/kg BW Δ9-THC are reported in Figure 4.
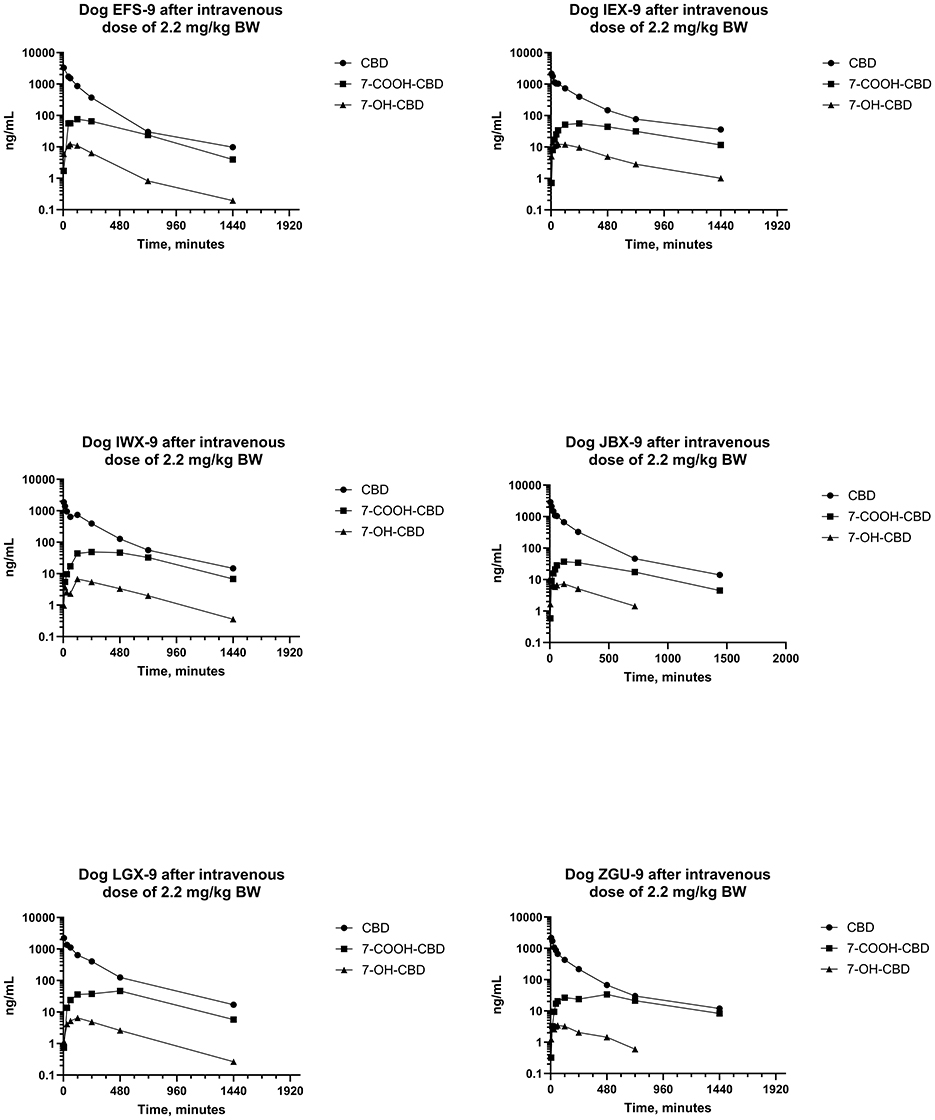
Figure 3. Plots of log serum concentrations of CBD, 7-OH-CBD, and 7-COOH-CBD after a single intravenous dose of CBD to six beagle dogs at a dose of 2.2 mg of CBD per kg BW.
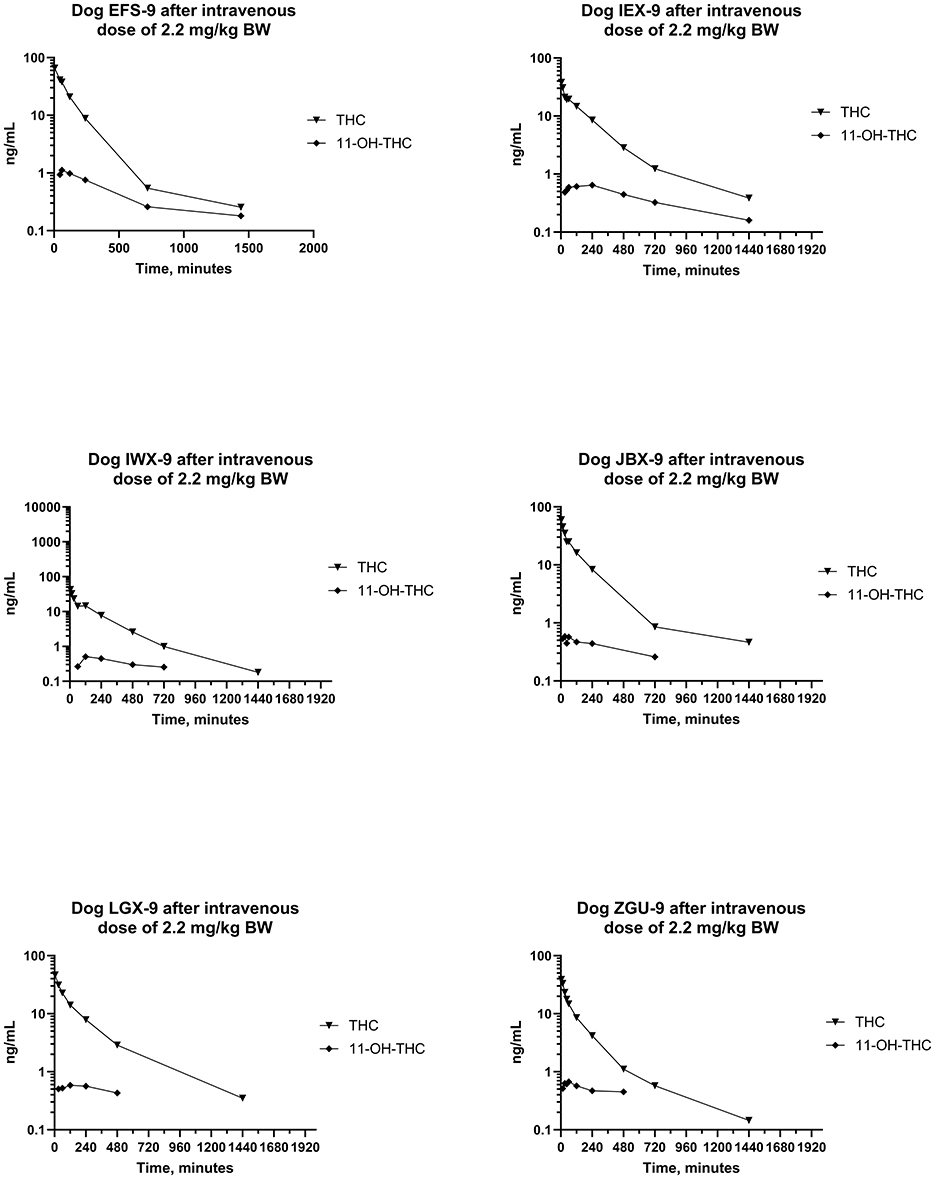
Figure 4. Plots of log serum concentration of Δ9-THC and 11-OH-THC after a single intravenous dose of the test article at a 2.2 mg of CBD per kg and 54 μg of Δ9-THC per kg BW.
Pharmacokinetic parameter estimates [median (range)] for CBD after IV administration are reported in Table 1. The disposition of CBD in beagle dogs after an IV dose of CBD at 2.2 mg/kg BW was characterized by a CLT of 7.06 (6.14–10.5) mL/min/kg BW, Vss of 2,130 (1,102–2,851) mL/kg BW, and terminal t1/2 of 291 (183–508) min. Maximum serum CBD concentrations ranged from 1,869–3,294 ng/mL, and CBD was still quantifiable 24 h after dosing. The MRT for CBD was 277 (179–429) min.
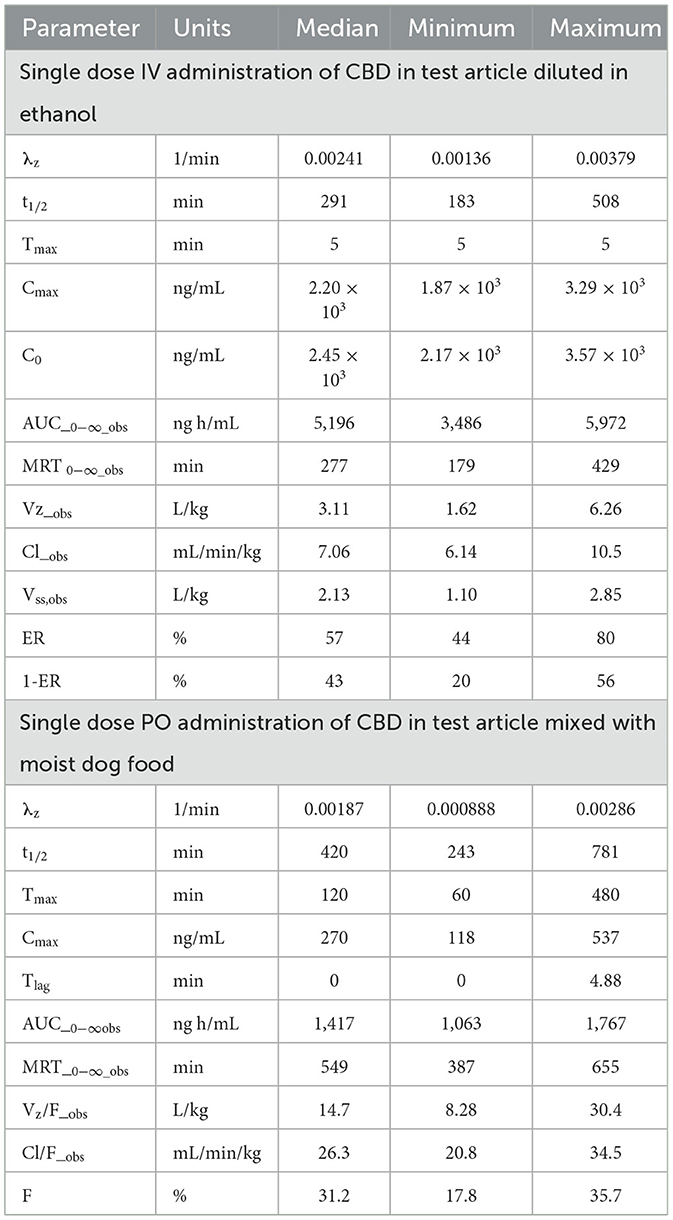
Table 1. Summary of non-compartmental pharmacokinetic parameter estimates after IV and PO administration of CBD at a dose of 2.2 mg/kg of body weight to beagle dogs (n = 6).
Pharmacokinetic parameter estimates [median (range)] for Δ9-THC after IV administration of Δ9-THC in a “full-spectrum” formulation dosed at 54 μg/kg BW are reported in Table 2. The disposition of Δ9-THC in beagle dogs after an IV dose of 54 μg/kg BW was characterized by a CLT of 8.85 (6.88–14.4) mL/min/kg BW, Vss of 1,977 (1,304–2,305) mL/kg BW, and terminal t1/2 of 169 (139–476) min. The mean CLT in females (8.32 mL/min/kg) was not different from that in males (7.05 mL/min/kg) (P = 0.424).
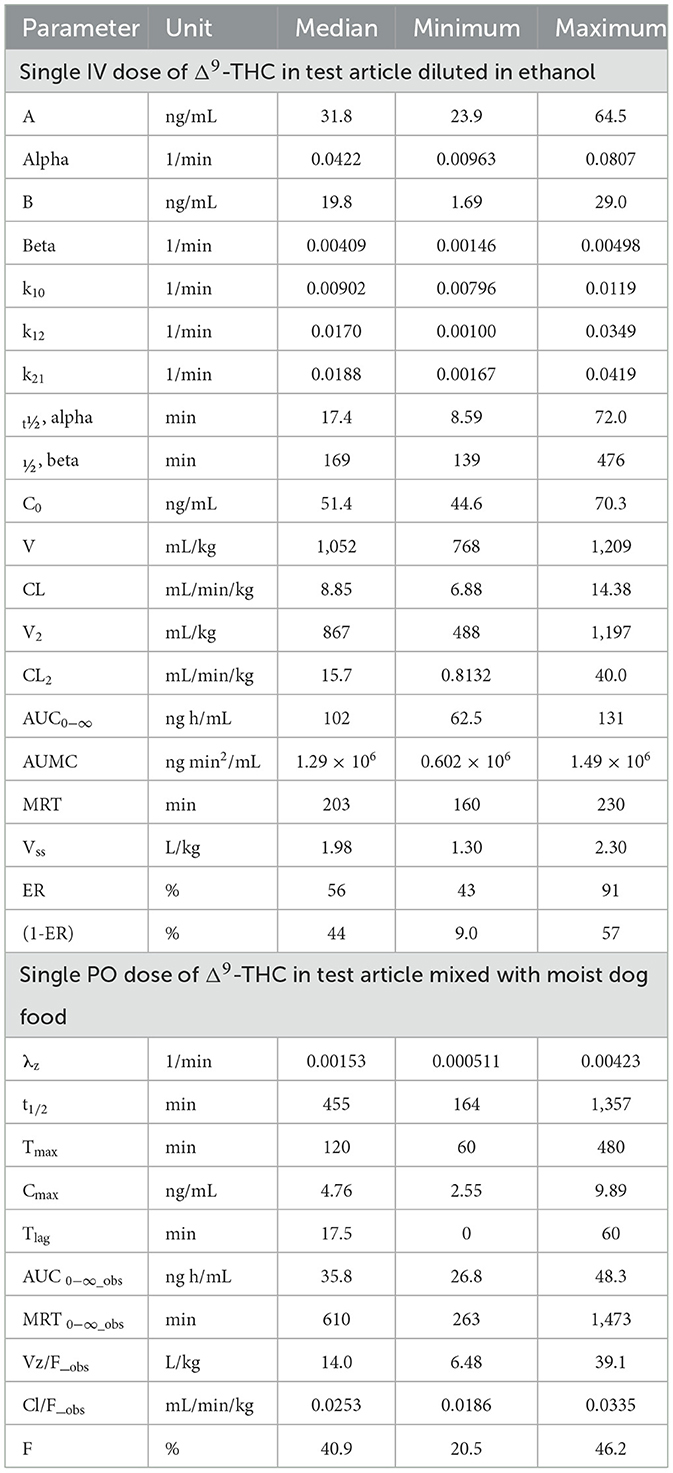
Table 2. Summary of two-compartment pharmacokinetic parameter estimates for Δ9-THC after IV administration and non-compartmental pharmacokinetic parameter estimates after PO administration to beagle dogs as a single dose of 54 μg/kg body weight.
Maximum serum Δ9-THC (i.e., C0) ranged from 44.6–70.3 ng/mL and Δ9-THC was still measurable 24 h after dosing. The MRT for Δ9-THC was 203 (160–230) min. Serum concentrations of 11-OH-THC were detected through 8–24 h after IV dosing of Δ9-THC in the “full-spectrum” formulation (see Figure 4).
3.3.2 Serum CBD, Δ9-THC, and metabolites after single dose oral administration–Phase 2
Serum concentrations of CBD, 7-OH-CBD, and 7-COOH-CBD after single PO administration of CBD at 2.2 mg/kg BW are reported in Figure 5 and pharmacokinetic parameter estimates are reported in Table 1. Maximum CBD ranged from 118–537 ng/mL (median 270 ng/mL) and were observed from 60–480 min (median 120 min) after dosing. The systemic availability of CBD ranged from 17.8%−35.7% (median 31.1%). CBD metabolites were quantifiable in all samples, 7-COOH-CBD concentrations were less than those of CBD but were greater than those of 7-OH-CBD at all sampling times, and the terminal concentrations of CBD and its metabolites decreased in parallel.
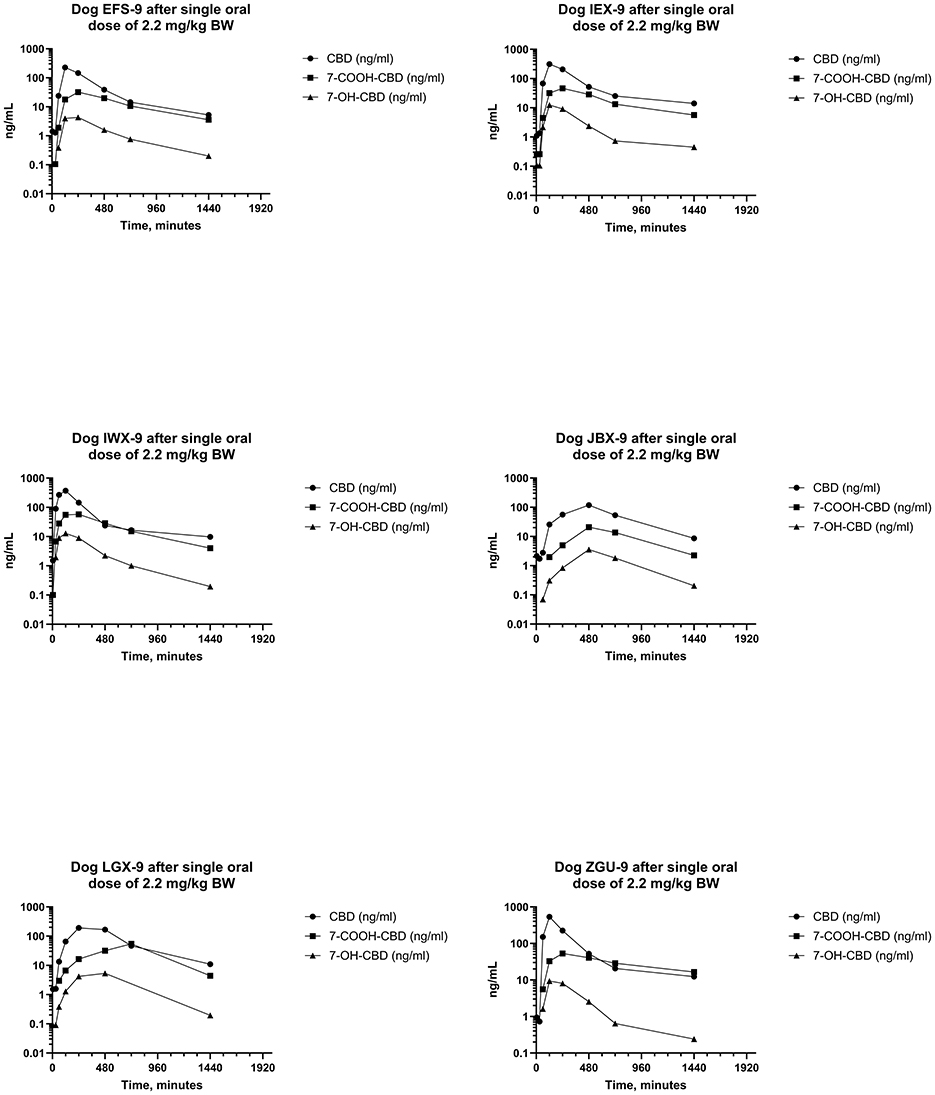
Figure 5. Plots of log serum concentrations of CBD, 7-OH-CBD, and 7-COOH-CBD after a single oral dose of CBD to six beagle dogs at a dose of 2.2 mg of CBD per kg BWt.
Serum concentrations of Δ9-THC, after a single PO dose of Δ9-THC at a dose of 54 μg/kg BW are reported in Figure 6. Pharmacokinetic parameter estimates of CBD after IV and PO dosing are compared in Table 1. Selected pharmacokinetic parameter estimates for CBD, 7-OH-CBD, and 7-COOH CBD after IV and PO dosing are reported in Table 3. Serum concentrations of Δ9-THC were determined in all dogs after a PO dose of the test article, but 11-OH-THC and 11-COOH-THC were not quantifiable except that 11-OH-THC was detected in one dog from 2 to 24 h.
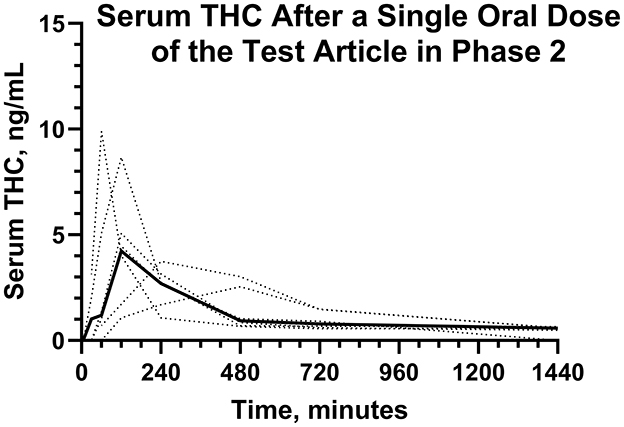
Figure 6. Individual (···) and average (—) serum Δ9-THC concentrations after a single dose of the test article in Phase 2.
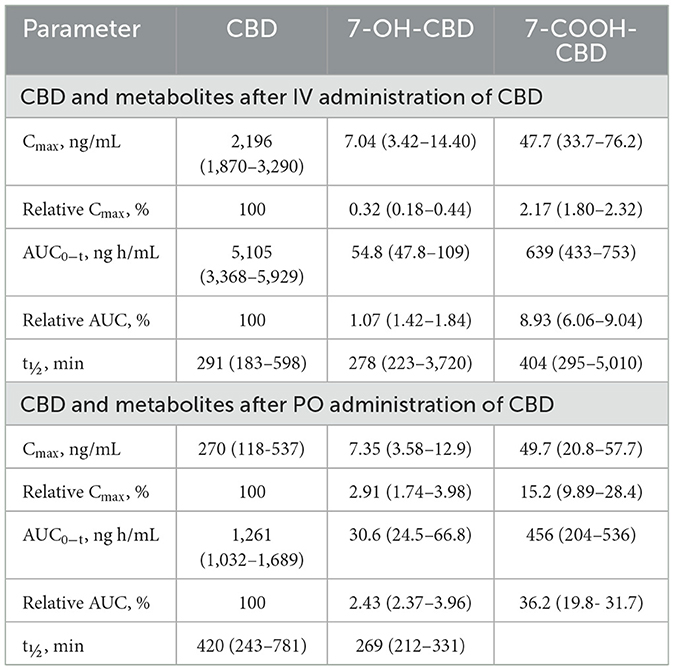
Table 3. Median (range) Cmax and AUC0 − t for CBD, 7-OH-CBD, and 7-COOH-CBD after single dose IV and PO CBD at 2.2 mg/kg body weight.
3.3.3 Serum CBD, Δ9-THC, and metabolites after repeated dose oral administration–Phase 3
Serum concentrations of CBD, 7-OH-CBD, and 7-COOH-CBD after the first and next to last PO dose of CBD at 2.2 mg/kg BW every 12 h for 21 days are reported in Figures 7, 8. Serum concentrations of Δ9-THC after the first and next to last dose are reported in Figure 9.
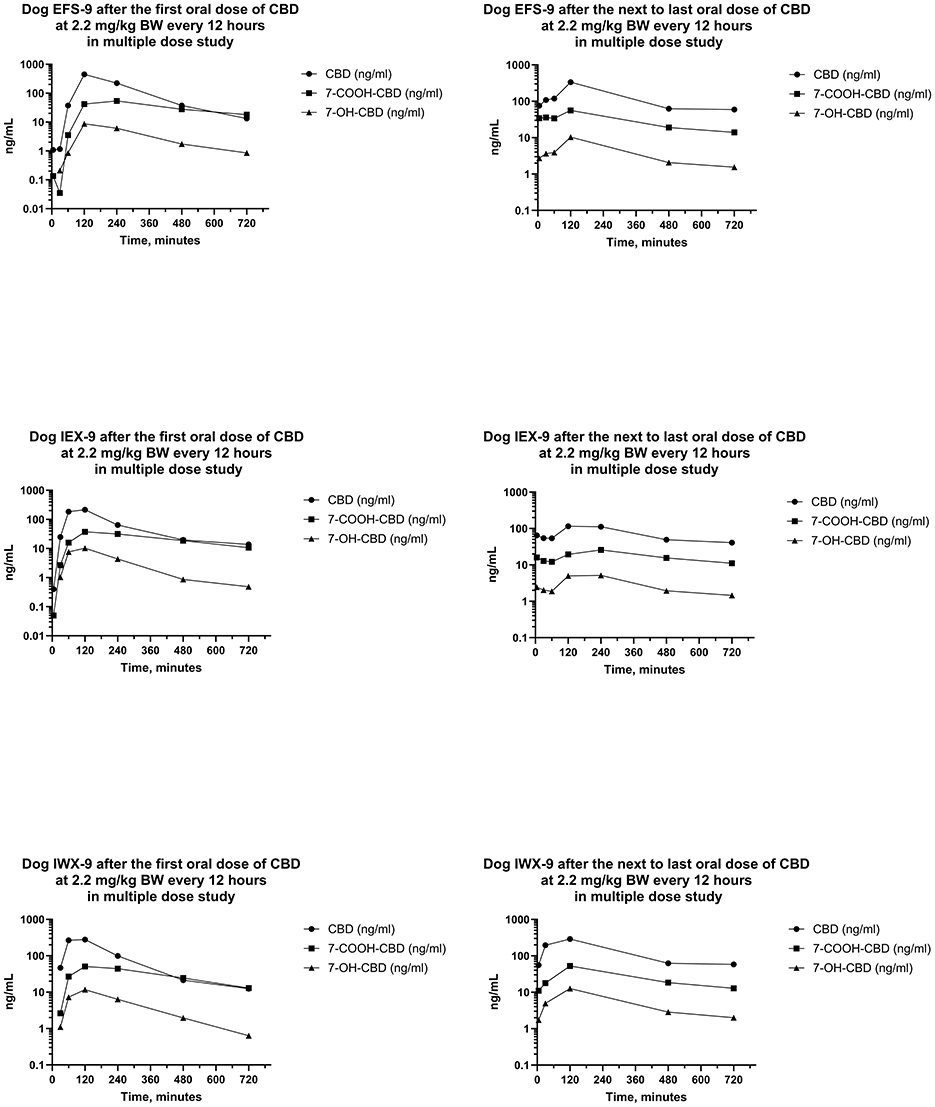
Figure 7. Plots of log serum concentrations of CBD, 7-OH-CBD, and 7-COOH-CBD after the first and last oral dose of CBD to three beagle dogs (EFS-9, IEX-9, and IWX-9) at 2.2 mg of CBD per kg BW every 12 h.
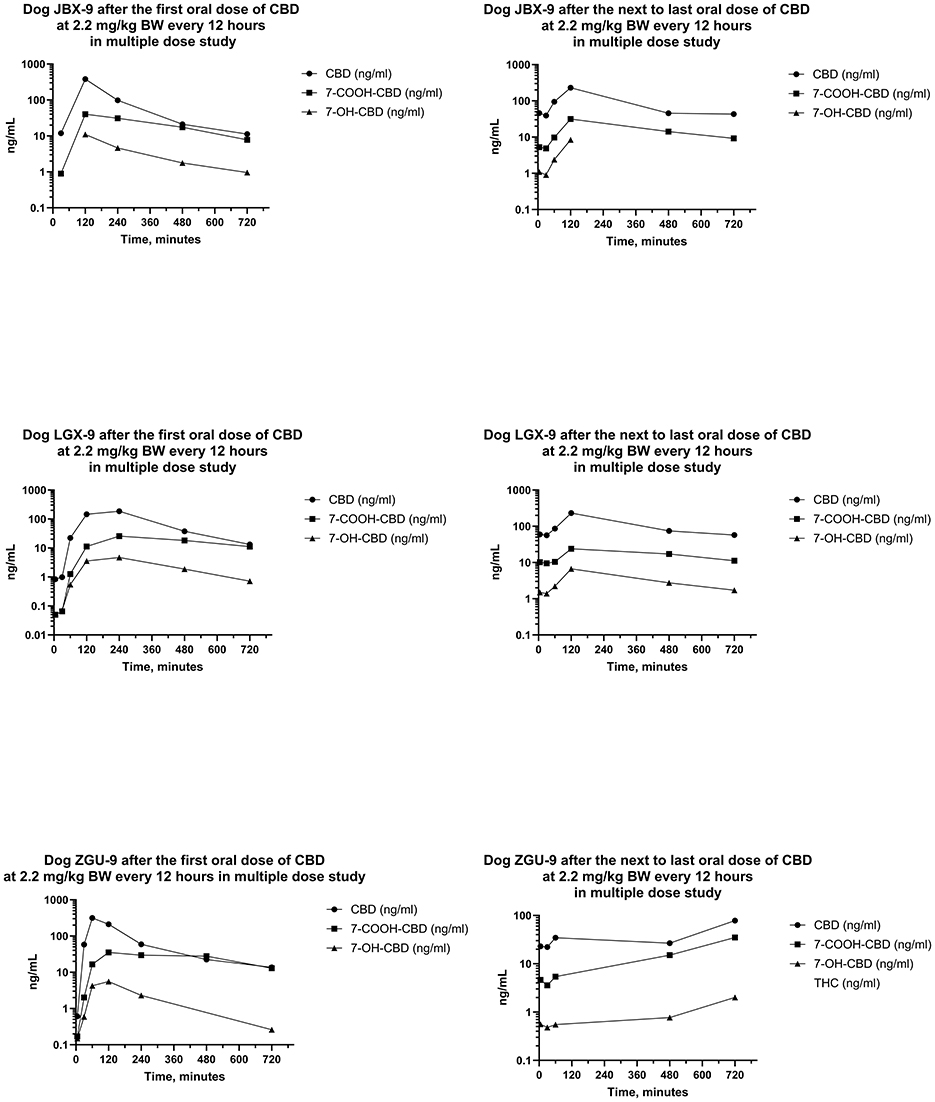
Figure 8. Plots of log serum concentrations of CBD, 7-OH-CBD, and 7-COOH-CBD after the first and last oral dose of CBD to three Beagle dogs (JBX-9, LGX-9, and ZGU-9) at 2.2 mg of CBD per kg BW every 12 h.
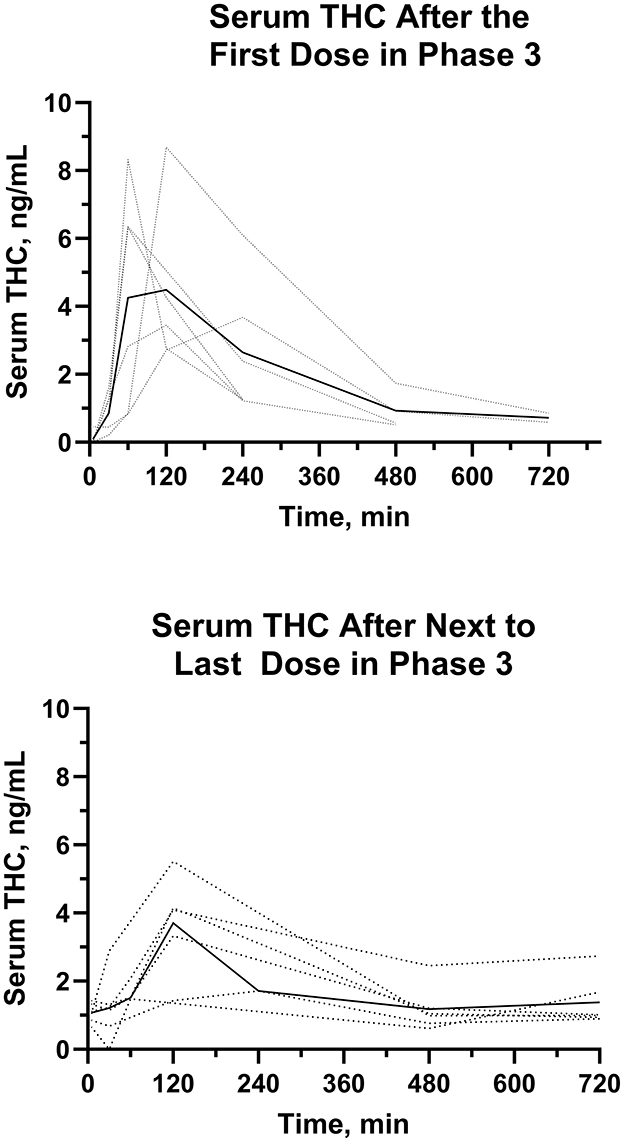
Figure 9. Individual (···) and average (—)Δ9-THC serum concentrations after the first and next to last dose in the multiple dose oral trial (Phase 3).
Serum concentrations of CBD, 7-OH-CBD, 7-COOH-CBD, and Δ9-THC after the first dose were like those in the single dose PO study. Serum concentrations of 11-OH-THC and 11-nor-9-COOH-THC were quantifiable in some samples after the first dose, but all concentrations were < 1 ng/mL. Serum concentrations of CBD, 7-COOH-CBD, 7-OH-CBD, and Δ9-THC were present in all samples collected for 12 h following dose administration after the 20th day, and 11-OH-THC was present at concentrations < 1 ng/mL in three of six samples.
Median (range) serum CBD concentrations in Phase 3 in samples collected 12 h after the first dose on day 1, and before dosing on days 5, 10, 15, and 21 were 13.3 (11.2–13.8), 143 (89.9–181), 127 (72.2–268), 109 (100–185), and 57.8 (41.1–78.3) ng/mL, respectively. Serum concentrations of CBD at 12 h after the first dose were lower than CBD concentrations 12 h after doses on days 5, 10, 15, and 21. Furthermore, serum CBD concentrations 12 h after the first dose of the day on day 21 were less than those collected 12 h after dosing on days 5 and 15. CBD concentrations on days 5, 10, and 15 after the beginning of the multiple dose study were not different.
The AUCs for CBD from the time of dosing through 12 h were determined after the first and the next to last dose during twice daily PO dosing of CBD for 21 days. The median (range) of the AUCs for 12 h after the first and next to last doses were 932 (723–1,408) ng min/mL and 1,193 (448–1,548) ng min/mL, respectively. The median (range) of the ARs was 0.880 (0.632–1.85). Furthermore, the median (range) of the serum CBD Cmax was 298 (185–450) ng/mL and 230 (78–336) ng/mL, respectively, and did not differ between the first and next to last dose. On the other hand, the ratio of the t1/2 after the first dose to that after the last dose was 0.502 (0.436–0.540) indicating that it was about twice as long after twice daily dosing for 3 weeks than it was at the start of the multiple dosing regimen.
The AUC0 − 12h for serum Δ9-THC from the time of dose administration through 12 hours were determined after the first and the next to last dose during twice daily PO dosing for 21 days. The median (range) AUC0 − 12h were 21.0 (12.6–40.7) ng min/mL min and 22.4 (7.7–26.7) ng min/mL, after the first and next to last dose, respectively. The AUCs of Δ9-THC after the first and next to last dose were not different. The median (range) AR were 0.855 (0.574–1.26). The median (range) serum Δ9-THC Cmax values after the first PO dose were higher (P = 0.01) than that after the next to last PO dose.
4 Discussion
To the authors' knowledge, this is the first study since the late 1980s including both IV and PO administration of CBD to the same dogs (42, 67). Serum CBD, 7-OH-CBD, 7-COOH-CBD, Δ9-THC, 11-OH-THC, and 11-COOH-THC were determined with a validated, tandem LC-MSn method using stable isotope-labeled internal standards for each analyte. The method used in this study was more sensitive and included more target analytes than the HPLC-UV methods used in earlier studies (22, 27–29, 31, 34, 37, 41, 52, 68–72). Serum concentrations of Δ9-THC, 11-OH-THC, 11-COOH-THC, 7-OH-CBD, and 7-COOH-CBD were targeted in all phases of the study for a more complete characterization of the pharmacokinetics of orally administered CBD and Δ9-THC in dogs.
This study is the first to report CBD and two of its metabolites, as well as Δ9-THC and two of its metabolites, in serum samples from beagle dogs administered the test article via IV administration. The results of pharmacokinetic analysis after IV administration of the test article permitted estimation of the systemic availability of CBD after PO administration. These results indicated that the extent of systemic availability was consistent with estimates of ER obtained from the analysis of the corresponding IV data. The AUC data for 7-OH-CBD after IV and PO administration of CBD did not differ, thereby providing evidence that absorption of CBD from the gastrointestinal tract after PO dosing was complete (73). The t1/2 values of CBD, 7-OH-CBD, and 7-COOH-CBD were similar, indicating that the t1/2 values of metabolites are formation rate-limited.
Results of the pharmacokinetic investigations of CBD and Δ9-THC after IV and single PO doses are reported (Tables 1–3). Results of the pharmacokinetic analysis of IV CBD indicated CLT near estimates of liver blood flow after estimating serum hepatic flow from the HCT for each dog. Estimates of the Vss are consistent with extensive distribution of CBD and other cannabinoids. The median (range) of t½ after IV dosing was 291 (183–508) min, reflecting the effect of the large volume of distribution despite the rapid clearance. The disposition of Δ9-THC after IV administration was characterized by similar pharmacokinetic parameters.
Pharmacokinetic analysis of data from IV administration of the test article permitted estimation of the systemic availability of CBD and Δ9-THC after PO administration. These results indicated that estimates of the extent of systemic availability were consistent with estimates of ER and 1-ER obtained from the analysis of the corresponding IV data by equating CLT of each substance to hepatic serum flow since CBD does not partition into erythrocytes (42). The systemic availability for CBD based on the estimate of 1-ER was 43% compared to the measured oral bioavailability of 31%. The systemic availability of Δ9-THC was estimated to be 44% based on an estimate of 1-ER under the same assumptions (64) and the measured availability was 41%. Although estimates of E (and 1-ER) were based on measurements of CLT and HCT for each dog, the calculations require determination of hepatic blood flow which was not available for study dogs and therefore, estimated (30–45 mL/min/kg) (63, 64). Use of the published estimate of hepatic blood flow instead of actual hepatic blood flow in each dog contributed to the uncertainty in these calculations. Nevertheless, the estimates are in reasonable agreement with measurements based on comparisons of the AUC values after PO and IV dosing. In addition to the pharmacokinetic parameter estimates for CBD, the current study reports that AUC data for 7-OH-CBD after IV and PO administration of CBD did not differ, thereby providing evidence that absorption of CBD from the gastrointestinal tract after PO dosing was complete and was not limited by the poor aqueous solubility of CBD (73).
Plasma CBD concentrations in earlier studies were determined by HPLC-UV methods, in which the reported LOD was 25 ng/mL (42, 67, 69, 74–76). The pharmacokinetics of CBD in one frequently cited study were determined after single IV doses of about 45 and 90 mg to dogs weighing between 16 and 24 kg (~2.25 and 4.5 mg/kg, respectively) and a single PO dose of 180 mg (~9 mg/kg) to each of six mixed-breed dogs. The disposition of CBD was well characterized after IV administration, but serum metabolites of CBD were not targeted, and CBD was not detected in three of the six dogs after PO administration because the plasma concentrations were too low to be quantified. The results of that study indicated multiexponential disposition of CBD after IV administration, rapid distribution of CBD from plasma to tissues, rapid clearance approximately equal to hepatic plasma flow, large volumes of distribution, high ER of 0.74, dose-dependent AUC, and extensive first-pass metabolism after PO administration (42). The CLT was rapid at 17.3 L/h and 15.9 L/h, respectively (approximately 14.4 mL/min/kg and 13.3 mL/min/kg, respectively (42). Furthermore, the Vss values were 5.65 and 5.85 L/kg, respectively, the volumes of distribution beta (Vdβ) were 8.4 and 10.5 L/kg, respectively, and the terminal t½ values were 6.8 h and 9.3 h, respectively (42).
Results from a companion study of the urinary excretion of CBD and its metabolites indicated that CBD is extensively metabolized, and that renal clearance of CBD is a minor route of elimination since only 0.013% of the administered dose was recovered from the urine. These findings lead to the conclusion that the CLT is due to hepatic clearance (42, 67). Furthermore, the total amount of CBD and CBD-related substances recovered in the urine accounted for < 2% of the administered dose (67) indicating that metabolites are eliminated by other routes like biliary excretion or that a portion of the dose was eliminated in the feces. The metabolites identified in urine collected after oral dosing of CBD result from oxidation to mono-, di-, and tri-hydroxy and acid metabolites (67, 75, 76). Several of the alkyl side chain oxidized metabolites are subsequently conjugated with glucose and excreted in the urine (67, 75, 76).
The conclusions of other studies of the disposition of PO CBD in dogs attribute low systemic availability to poor absorption or extensive first-pass metabolism without any direct evidence. These studies did not acquire IV data and therefore could not estimate the extent of systemic availability from AUC after PO and IV administration. The results of the current study indicate modest first-pass metabolism of PO CBD in dogs, but do not show any evidence for poor or incomplete absorption. Peak serum CBD concentrations after PO administration were observed at 120 min (range 60–480) indicating relatively rapid absorption. Comparison of AUC values for CBD after PO and IV administration indicated that median (range) of the systemic availability was 40.9 (20.5–46.2)%, and a comparison (Table 3) of the AUCs for 7-OH-CBD after IV and PO administration of CBD indicated complete absorption of the dose in this study. Since the test article included CBD in MCT oil and was fed with canned dog food containing fats, the systemic availability of the CBD may have been improved relative to other studies because losses of undissolved CBD in the feces were unlikely. Results of studies of the disposition of CBD in dogs (and other species) indicate a substantial first-pass effect after PO administration and are consistent with predictions for highly lipophilic substances like CBD and other cannabinoids because lipophilicity and related factors determine, or have substantial influence on pharmacokinetic properties (77–79).
Natural cannabinoids are rapidly distributed into highly vascularized organs (e.g., lung, heart, brain, and liver) but are more slowly distributed into less vascularized tissues (e.g., adipose tissue) (80). The rapid distribution of CBD is reflected in the rapid reduction in CBD serum concentrations after IV administration in this study (Figure 3) and those in an earlier study (42). The extensive distribution of CBD into highly vascularized tissues and its subsequent partitioning into less vascular tissue results in a large fraction of the administered dose residing in tissues outside the blood. Furthermore, highly lipophilic tissues like adipose tissue have a large capacity for storing CBD from which it slowly partitions back into the blood as blood concentrations decrease. The partitioning of CBD into such tissues contributes to the large volumes of distribution that have been reported in this study (Table 1) and a previous study (42). The median (range) of Vss in this study in beagle dogs was 2.13 (1.10–2.85) L/kg and the mean (SD) Vss from the previous study was 5.65 ± 2.1 L/kg after a dose of 45 mg IV (42). Large volumes of distribution characterize the disposition of CBD in other species as well. In a study of older horses, the mean (SD) Vss of CBD was 5.5 ± 1.5 L/kg, indicating extensive distribution in this species (81). Furthermore, in pigs, the mean (SD) Vss was 3.3 (±0.29) L/kg (82). In humans, the volume of distribution of CBD after IV administration of its deuterium- labeled analog was reported as 2,520 L (approximately 36 L/kg, assuming a BW of 70 kg) (83). The extensive distribution of CBD and correspondingly large volumes of distribution are largely a consequence of the high lipophilicity of CBD.
Metabolism of CBD in dogs is extensive and only small amounts of CBD are excreted unchanged in the urine, so CLT is attributed largely or exclusively to hepatic clearance (74–76). After the CLT estimates based on plasma measurements in the previous IV study were converted to blood clearance, the plasma clearance estimates [i.e., 20.3 and 19.5 mL/min/kg, respectively (42)] were compared to the liver blood flow reported for dogs (63) to estimate the hepatic ER for CBD. The ER estimate of 0.74 (42) in that study predicted that approximately 26% of an oral dose of CBD to dogs escapes first-pass metabolism and reaches the systemic circulation unchanged (42). Due to the limitations of the analytical methodology (i.e., HPLC-UV with a reported lower limit of detection (LOD) for CBD of 25 ng/mL (69), CBD was not quantifiable after PO administration of 180 mg in three of the six dogs (42). The extent of systemic availability in the remaining three dogs was reported to be 13%, 13%, and 19% (42). These estimates are in reasonable agreement with the estimate of 26% from the prediction based on the ER and may have been lower due to assay limitations (42).
Pharmacokinetic parameter estimates after IV CBD at 2.2 mg/kg in the present study are reported (Table 1). Our findings and those from an earlier study using purified CBD in dogs are similar (42). In both cases, the disposition of CBD was characterized by non-compartmental modeling. The dose of 2.2 mg/kg was near the dose of 2.25 mg/kg in the earlier study, but the sensitivity and specificity of the CBD analysis in serum were improved by LC-MSn analysis in the current study, thereby resulting in the measurement of serum CBD, Δ9-THC, and oxidative metabolites at lower concentrations than were achievable by the HPLC-UV method used previously. CBD concentrations decreased rapidly during the first 4 h after IV administration, then declined at a slower rate characterized by a median (range) t1/2 of 291 (183–508) min compared to 420 (294–888) min in the previous study (42). The rapid decline in CBD concentrations after dosing in the present study did not appear as pronounced as that reported in the previous study (42, 69) because sampling was less frequent during this period.
Comparisons of the plasma or serum clearance of CBD to the liver blood flow in the previous (42)and current study of IV CBD in dogs indicate that hepatic clearance is likely flow-rate dependent. Therefore, variability in liver blood flow rates will affect hepatic clearance more than variability in the free fraction and intrinsic metabolic activity (i.e., fu·CLint where fu is the fraction unbound and CLint is the intrinsic clearance) (64, 84–88). The estimate of ER from the present study is important as it suggests that the hepatic clearance of CBD in dogs is likely flow rate-limited. Consequently, it is unlikely to be significantly impacted by changes in plasma protein binding and intrinsic hepatic metabolic clearance (88). Since the extent of systemic availability after PO administration of a high extraction ratio drug depends on 1-ER, small changes in ER can result in differences in systemic availability. This variability is likely responsible for the inconsistent systemic availability of orally administered CBD, as reported in this and other studies of the disposition of CBD in dogs (27–29, 31, 34, 37, 39, 41, 52, 72, 89, 90).
The estimate of hepatic extraction ratio provided an estimate of the extent of first-pass metabolism that agreed with the direct determinations based on AUC comparisons.
The metabolism of CBD has been investigated in mice (91–96), rats (74, 80, 94, 97), dogs (67, 74–76), cats (94), gerbils (94), guinea pigs (94), rabbits (94), hamsters (94), horses (81, 98–101), pigs (82), sheep, cattle (102), and humans (74, 103–106). Numerous oxidative and conjugated metabolites of CBD have been identified from urine after PO and IV administration to dogs (67, 71, 74–76), but this study is the first in which metabolites of CBD have been determined in serum after PO and IV doses. Serum concentrations of 7-OH-CBD and 7-COOH-CBD were determined by LC-MSn and the resulting data were subjected to non-compartmental pharmacokinetic analysis. Serum concentrations and AUC of 7-OH-CBD were lower than those of 7-COOH-CBD and CBD in all samples after IV and PO administration of CBD (Figures 3, 5). Similarly, serum concentrations and AUC of 7-COOH-CBD were lower than those of CBD in all samples after IV and PO administration. The Cmax values for serum 7-OH-CBD were observed somewhat earlier than those of 7-COOH-CBD suggesting that 7-OH-CBD was formed before 7-COOH-CBD and t1/2s of CBD, 7-OH-CBD, and 7-COOH-CBD were similar, indicating that the half-lives of metabolites are formation rate-limited (107–109). This conclusion is supported by the observation that the “ratio plot” (i.e., C7 − OH − CBDt/CCBD and C7 − COOH/CCBD) vs. time after administration increased, as was previously described for formation rate-limited elimination (110).
In dogs and humans, CBD is sequentially metabolized to 7-OH-CBD, the major active metabolite, and then to 7-COOH-CBD, an inactive metabolite (106). The current finding for relative Cmax and AUC values for CBD, 7-COOH-CBD, and 7-OH-CBD contrast with those from studies of PO CBD in humans in which serum 7-COOH-CBD concentrations are higher than those of both CBD and 7-OH-CBD (106). 7-COOH-CBD is a metabolite in horses where it attains higher plasma concentrations than CBD and 7-OH-CBD and has a longer t1/2 than CBD. Consequently, plasma 7-COOH-CBD is detectable after CBD has become undetectable in horses (81, 100, 101, 111, 112).
Pharmacokinetic parameter estimates for Δ9-THC after IV administration to beagle dogs from the present study were compared to those reported previously after normalizing volumes of distributions and clearances based on body weights of the dogs (64). Although a highly specific and sensitive LC-MSn method was used to determine Δ9-THC in the present study, the use of 14C-labeled Δ9-THC, a larger dose, and more frequent and prolonged sampling in the previous study allowed detection of Δ9-THC and its metabolites for an extended period after dose administration. The model used to describe these data used five exponential terms, including one that described the disposition of Δ9-THC at lower concentrations and for a longer period than was possible in the present study. Consequently, the magnitudes of the half-lives and volumes of distributions were substantially different from the values reported in Table 2. For example, the mean Vc in the present study was 1,007 mL/kg whereas in the earlier study it was only 94.7 mL/kg. Furthermore, the median (interquartile range) of the β-phase t1/2 of Δ9-THC in the present study was 170 (141–253) min whereas it was 11,820 min (197 h) in the earlier study. On the other hand, the median (interquartile range) for CLT in the present study was 8.52 (7.77–10.1) mL/min/kg and 8.85 (7.85–11.0) mL/min/kg from the earlier study. These values were not different.
Differences in pharmacologic responses of dogs and humans from exposure to Δ9-THC have been hypothesized to be due to differences in its metabolism (113) and differences in the metabolites excreted in urine (74). Serum concentrations of 11-OH-THC, a pharmacologically active metabolite of Δ9-THC were present after IV, but not after PO, administration in this study (Figure 4). This metabolite was present in the earliest samples collected, reflecting its rapid formation in dogs (64, 114). Serum concentrations of 11-OH-THC were always less than those of Δ9-THC and the ratio of serum concentration of Δ9-THC to that of 11-OH-THC decreased with time. Therefore, this finding suggests that this metabolite may contribute to the effects of CBD after IV exposure or dosing to dog.
The test article was well tolerated when administered as a single IV dose, a single PO dose, and repeated PO doses every 12 h for 21 days to beagle dogs (4 males, 2 females) weighing (median; range) 10.7 kg; 7.5–11.2 kg. Occasional salivation and mucus in the feces, unformed feces, and regurgitation or vomiting were observed in some dogs during different phases of the study. These results are consistent with other studies in which CBD isolates and full-spectrum CBD products have been administered in single or multiple PO doses to beagles and mixed-breed dogs (34, 58, 71).
An increase in ALP within the normal range was noted between the first and last doses after repeated PO administration of CBD at a daily dose of 4.4 mg/kg (2.2 mg/kg every 12 h) in this study (Figure 2). This finding is consistent with previous reports of elevated ALP after repeated PO administration of CBD to dogs at daily doses of 1–4 mg/kg (29), 1–12 mg/kg (31, 70), 4 mg/kg (71), 5 and 10 mg/kg (115), 5 mg/kg (116), 5–20 mg/kg (40), and 10–20 mg/kg (32). A previous investigation into the effects of CBD on hematologic and biochemical parameters in dogs after PO administration of CBD at 4 mg/kg daily for 6 months found that ALP was not elevated at the end of 2 weeks, but was elevated at the end of 4, 10, 18, and 26 weeks after which the ALP returned to pre-study values within the 4-week washout period (71). After daily dosing of CBD at 4 mg/kg, mean ALP concentrations were 98.0, 139, 145, 135, and 136 U/L after 2, 4, 10, 18, and 26 weeks, respectively (71). Furthermore, the ALP concentrations at 4, 10, 18, and 26 weeks were greater than the upper limit of the clinically normal range (i.e., 131 U/L) for the test method that was used (71). In addition to the elevated ALP concentrations, bone alkaline phosphate (BALP) concentrations were also elevated and strongly correlated with the ALP concentrations suggesting that the increased ALP was partially due to increased BALP (71). Although increased ALP is usually considered an indicator of dose-dependent drug-induced liver injury, the underlying pathophysiology associated with its rise during chronic treatment with CBD in dogs is unknown (117).
Limitations of this study include lack of a placebo group, small number of subjects, and the short duration of the repeated oral dose study period. The small number of subjects reduced the statistical power, and the short duration of the repeated dose study may have precluded determination of effects arising during longer treatment. Furthermore, since the study used purpose-bred, 1–2 year old, beagle dogs, the effects, if any, of breed, age, neutering, and sizes of the dogs were not investigated.
In conclusion, the disposition of CBD and Δ9-THC in beagle dogs is characterized by rapid and extensive hepatic metabolism resulting in a substantial first-pass effect after oral administration. Both CBD and Δ9-THC are highly lipophilic substances that are characterized by large Vss (~2 L/kg), due to distribution into tissues such as fat from which they are slowly released. The test article was well tolerated when administered as a single IV dose, a single PO dose, and repeated PO doses every 12 h for 21 days to beagle dogs. Serum concentrations in the current study were determined by a sensitive and specific LC-MSn method in contrast to earlier studies of the disposition of CBD in dogs after IV and PO administration (42, 67, 69). Serum concentrations of Δ9-THC, 11-OH-THC, 11-COOH-THC, 7-OH-CBD, and 7-COOH-CBD were determined in the current study for a more complete characterization of the pharmacokinetics of orally administered CBD in contrast to other studies (23, 26–28, 31, 33, 34, 37, 39–41, 52, 72, 118–120). Both CBD and Δ9-THC are subject to sequential first-pass metabolism to oxidative metabolites. Furthermore, we have investigated the pharmacokinetics of CBD and Δ9-THC after IV administration to the same dogs and characterized the pharmacokinetics of both. We postulate that the CLT of both CBD and Δ9-THC in dogs is due to hepatic metabolism, that hepatic clearance is blood flow-rate dependent, and that the hepatic ER predicts the extent of systemic availability of both CBD and Δ9-THC after PO administration when fed with moist dog food.
Data availability statement
The raw data supporting the conclusions of this article will be made available by the authors, without undue reservation.
Ethics statement
The animal study was approved by the East Tennessee Clinical Research Institutional Animal Care and Use Committee. The study was conducted in accordance with the local legislation and institutional requirements.
Author contributions
SK-M: Conceptualization, Data curation, Investigation, Methodology, Project administration, Resources, Supervision, Writing – review & editing. RS: Conceptualization, Formal analysis, Investigation, Methodology, Project administration, Supervision, Validation, Writing – review & editing. WM: Conceptualization, Formal analysis, Funding acquisition, Methodology, Validation, Writing – original draft, Writing – review & editing.
Funding
The author(s) declare that financial support was received for the research and/or publication of this article.
Acknowledgments
We thank Jamie Gaynor, DVM and Peak Therapeutics CBD for supporting this project. We thank Jasper van Heemst for performing the cannabinoid analyses at KCA Laboratories in Nicholasville, Kentucky.
Conflict of interest
The authors declare that this study received funding from Peak Therapeutics CBD. Peak Therapeutics CBD provided the test article at no cost and funded the animal studies, staff support, equipment, clinical pathology, disposable materials, and serum cannabinoid analyses by KCA Laboratories, and publication costs.
Generative AI statement
The author(s) declare that no Gen AI was used in the creation of this manuscript.
Publisher's note
All claims expressed in this article are solely those of the authors and do not necessarily represent those of their affiliated organizations, or those of the publisher, the editors and the reviewers. Any product that may be evaluated in this article, or claim that may be made by its manufacturer, is not guaranteed or endorsed by the publisher.
Supplementary material
The Supplementary Material for this article can be found online at: https://www.frontiersin.org/articles/10.3389/fvets.2025.1556975/full#supplementary-material
Abbreviations
CBD, Cannabidiol; 7-OH-CBD, 7-Hydroxycannabidiol; 7-COOH-CBD, 7-nor-7-carboxycannabidiol; Δ9-THC, (-)-trans-Δ9-tetrahydrocannabinol; 11-OH-THC, 11-Hydroxytetrahydrocannabinol; 11-COOH-THC, 11-nor-9-Carboxytetrahydrocannabinol; BW, Body weight; MCT, Medium chain triglycerides; CBG, Cannabigerol; CBC, Cannabichromene; CBDV, Cannabidivarin; AUC, Area under the serum concentration vs. time curve; AR, Accumulation ratio; ER, Extraction ratio; MRT, Mean residence time; fu, Fraction unbound; CLint, Intrinsic clearance; t1/2, Half-life; HCT, Hematocrit; CLT, Total clearance; Vss, Volume of distribution at steady-state; Vc, Volume of the central compartment; t1/2, Half-life.
Footnotes
1. ^Purina One® SmartBlend® Chicken & Brown Rice Entrée.
2. ^Kaycha Labs, 879 Federal Blvd., Denver, CO, USA.
3. ^https://www.fda.gov/media/162903/download
4. ^GraphPad Prism version 8.3.1 for Windows, GraphPad Software, San Diego, California USA (https://www.graphpad.com).
References
1. Hazekamp A, Fischedick JT, Díez ML, Lubbe A, Ruhaak RL. Chemistry of cannabis. In: Liu H-W, Mander L, , editors. Comprehensive Natural Products II. Oxford: Elsevier (2010). p. 1033–84. doi: 10.1016/B978-008045382-8.00091-5
2. ElSohly MA, Gul W. Constituents of Cannabis sativa. In: Pertwee R, , editor. Handbook of Cannabis. Oxford: Oxford University Press (2014). p. 3–22. doi: 10.1093/acprof:oso/9780199662685.003.0001
3. Helcman M, Šmejkal K. Biological activity of Cannabis compounds: a modern approach to the therapy of multiple diseases. Phytochem Rev. (2021) 21:429–70. doi: 10.1007/s11101-021-09777-x
4. Goncalves J, Rosado T, Soares S, Simao AY, Caramelo D, Luis A, et al. Cannabis and its secondary metabolites: their use as therapeutic drugs, toxicological aspects, and analytical determination. Medicines (Basel). (2019) 6:31. doi: 10.3390/medicines6010031
5. Sommano SR, Chittasupho C, Ruksiriwanich W, Jantrawut P. The cannabis terpenes. Molecules. (2020) 25:5792. doi: 10.3390/molecules25245792
6. Lange BM, Zager JJ. Comprehensive inventory of cannabinoids in Cannabis sativa L: Can we connect genotype and chemotype? Phytochem Rev. (2021) 21:1273–313. doi: 10.1007/s11101-021-09780-2
7. Hanus LO, Meyer SM, Munoz E, Taglialatela-Scafati O, Appendino G. Phytocannabinoids: a unified critical inventory. Nat Prod Rep. (2016) 33:1357–92. doi: 10.1039/C6NP00074F
8. Goncalves ECD, Baldasso GM, Bicca MA, Paes RS, Capasso R, Dutra RC. Terpenoids, cannabimimetic ligands, beyond the cannabis plant. Molecules. (2020) 25:1567. doi: 10.3390/molecules25071567
9. Radwan MM, Chandra S, Gul S, ElSohly MA. Cannabinoids, phenolics, terpenes and alkaloids of cannabis. Molecules. (2021) 26:2774. doi: 10.3390/molecules26092774
10. Pollastro F, Minassi A, Fresu LG. Cannabis phenolics and their bioactivities. Curr Med Chem. (2018) 25:1160–85. doi: 10.2174/0929867324666170810164636
11. Erridge S, Mangal N, Salazar O, Pacchetti B, Sodergren MH. Cannflavins - from plant to patient: a scoping review. Fitoterapia. (2020) 146:104712. doi: 10.1016/j.fitote.2020.104712
12. Thomas BF, ElSohly MA. Biosynthesis and pharmacology of phytocannabinoids and related chemical constituents. In: Thomas BF, ElSohly MA, , editors. The Analytical Chemistry of Cannabis. New York: Elsevier (2016). p. 27–41. doi: 10.1016/B978-0-12-804646-3.00002-3
13. Vanhoenacker G, Van Rompaey P, De Keukeleire D, Sandra P. Chemotaxonomic features associated with flavonoids of cannabinoid-free cannabis (Cannabis sativa subsp. sativa L.) in relation to hops (Humulus lupulus L.). Nat Prod Lett. (2002) 16:57–63. doi: 10.1080/1057563029001/4863
14. Beleggia R, Menga V, Fulvio F, Fares C, Trono D. Effect of genotype, year, and their interaction on the accumulation of bioactive compounds and the antioxidant activity in industrial hemp (Cannabis sativa L.) inflorescences. Int J Mol Sci. (2023) 24:8969. doi: 10.3390/ijms24108969
15. Ross SA, ElSohly MA, Sultana GN, Mehmedic Z, Hossain CF, Chandra S. Flavonoid glycosides and cannabinoids from the pollen of Cannabis sativa L. Phytochem Anal. (2005) 16:45–8. doi: 10.1002/pca.809
16. Clark MN, Bohm BA. Flavonoid variation in Cannabis L. Botanical. J Linnean Soc. (1979) 79:249–57. doi: 10.1111/j.1095-8339.1979.tb01517.x
17. Pellati F, Brighenti V, Sperlea J, Marchetti L, Bertelli D, Benvenuti S. New methods for the comprehensive analysis of bioactive compounds in Cannabis sativa L. (hemp). Molecules. (2018) 23:2639. doi: 10.3390/molecules23102639
18. Gertsch J, Pertwee RG, Di Marzo V. Phytocannabinoids beyond the Cannabis plant - do they exist? Br J Pharmacol. (2010) 160:523–9. doi: 10.1111/j.1476-5381.2010.00745.x
19. Flores-Sanchez IJ, Verpoorte R. PKS activities and biosynthesis of cannabinoids and flavonoids in Cannabis sativa L. plants Plant. Cell Physiol. (2008) 49:1767–82. doi: 10.1093/pcp/pcn150
20. Flores-Sanchez IJ, Verpoorte R. Secondary metabolism in cannabis. Phytochem Rev. (2008) 7:615–39. doi: 10.1007/s11101-008-9094-4
21. Jin D, Dai K, Xie Z, Chen J. Secondary metabolites profiled in cannabis inflorescences, leaves, stem barks, and roots for medicinal purposes. Sci Rep. (2020) 10:3309. doi: 10.1038/s41598-020-60172-6
22. Boothe DM, Cruz-Espindola C, Gillette R, Strunk R, Warnner C. Cannabidiol disposition after single oral dosing in fasted and fed dogs. 2020 ACVIM Forum On Demand Research Abstract Program. J Vet Intern Med. (2020) 15:2830–989. doi: 10.1111/jvim.15904
23. Coelho MPRC, Leme FDOP, Moreira FA, Branco SEMT, Melo MM, de Melo EG. Current review of hemp-based medicines in dogs. J Vet Pharmacol Ther. (2021) 44:870–82. doi: 10.1111/jvp.13016
24. De Briyne N, Holmes D, Sandler I, Stiles E, Szymanski D, Moody S, et al. Cannabis, cannabidiol oils and tetrahydrocannabinol-what do veterinarians need to know? Animals (Basel). (2021) 11:892. doi: 10.3390/ani11030892
25. Morris EM, Kitts-Morgan SE, Spangler DM, McLeod KR, Costa JHC, Harmon DL. The impact of feeding cannabidiol (CBD) containing treats on canine response to a noise-induced fear response test. Front Vet Sci. (2020) 7:569565. doi: 10.3389/fvets.2020.569565
26. Chicoine A, Illing K, Vuong S, Pinto KR, Alcorn J, Cosford K. Pharmacokinetic and safety evaluation of various oral doses of a novel 1:20 THC:CBD cannabis herbal extract in dogs. Front Vet Sci. (2020) 7:583404. doi: 10.3389/fvets.2020.583404
27. Lebkowska-Wieruszewska B, Stefanelli F, Chericoni S, Owen H, Poapolathep A, Lisowski A, et al. Pharmacokinetics of Bedrocan(R), a cannabis oil extract, in fasting and fed dogs: an explorative study. Res Vet Sci. (2019) 123:26–8. doi: 10.1016/j.rvsc.2018.12.003
28. Fernandez-Trapero M, Perez-Diaz C, Espejo-Porras F, de Lago E, Fernandez-Ruiz J. Pharmacokinetics of Sativex(R) in dogs: towards a potential cannabinoid-based therapy for canine disorders. Biomolecules. (2020) 10:279. doi: 10.3390/biom10020279
29. Gamble LJ, Boesch JM, Frye CW, Schwark WS, Mann S, Wolfe L, et al. Pharmacokinetics, safety, and clinical efficacy of cannabidiol treatment in osteoarthritic dogs. Front Vet Sci. (2018) 5:165. doi: 10.3389/fvets.2018.00165
30. McGrath S, Bartner LR, Rao S, Packer RA, Gustafson DL. Randomized blinded controlled clinical trial to assess the effect of oral cannabidiol administration in addition to conventional antiepileptic treatment on seizure frequency in dogs with intractable idiopathic epilepsy. J Am Vet Med Assoc. (2019) 254:1301–8. doi: 10.2460/javma.254.11.1301
31. Vaughn DM, Paulionis LJ, Kulpa JE. Randomized, placebo-controlled, 28-day safety and pharmacokinetics evaluation of repeated oral cannabidiol administration in healthy dogs. Am J Vet Res. (2021) 82:405–16. doi: 10.2460/ajvr.82.5.405
32. McGrath S, Bartner LR, Rao S, Kogan LR, Hellyer PW. A report of adverse effects associated with the administration of cannabidiol in healthy dogs. Vet Med. (2018) 1:6–8.
33. Amstutz K, Schwark WS, Zakharov A, Gomez B, Lyubimov A, Ellis K, et al. Single dose and chronic oral administration of cannabigerol and cannabigerolic acid-rich hemp extract in fed and fasted dogs: physiological effect and pharmacokinetic evaluation. J Vet Pharmacol Ther. (2022) 45:245–54. doi: 10.1111/jvp.13048
34. Deabold KA, Schwark WS, Wolf L, Wakshlag JJ. Single-dose pharmacokinetics and preliminary safety assessment with use of CBD-rich hemp nutraceutical in healthy dogs and cats. Animals (Basel). (2019) 9:832. doi: 10.3390/ani9100832
35. Feng W, Qin C, Abdelrazig S, Bai Z, Raji M, Darwish R, et al. Vegetable oils composition affects the intestinal lymphatic transport and systemic bioavailability of co-administered lipophilic drug cannabidiol. Int J Pharm. (2022) 624:121947. doi: 10.1016/j.ijpharm.2022.121947
36. Della Rocca G, Di Salvo A. Hemp in veterinary medicine: from feed to drug. Front Vet Sci. (2020) 7:387. doi: 10.3389/fvets.2020.00387
37. Polidoro D, Temmerman R, Devreese M, Charalambous M, Ham LV, Cornelis I, et al. Pharmacokinetics of cannabidiol following intranasal, intrarectal, and oral administration in healthy dogs. Front Vet Sci. (2022) 9:899940. doi: 10.3389/fvets.2022.899940
38. Corsato Alvarenga I, Panickar KS, Hess H, McGrath S. Scientific validation of cannabidiol for management of dog and cat diseases. Annu Rev Anim Biosci. (2023) 11:227–46. doi: 10.1146/annurev-animal-081122-070236
39. Wakshlag JJ, Schwark WS, Deabold KA, Talsma BN, Cital S, Lyubimov A, et al. Pharmacokinetics of cannabidiol, cannabidiolic acid, delta9-tetrahydrocannabinol, tetrahydrocannabinolic acid and related metabolites in canine serum after dosing with three oral forms of hemp extract. Front Vet Sci. (2020) 7:505. doi: 10.3389/fvets.2020.00505
40. Doran CE, McGrath S, Bartner LR, Thomas B, Cribb AE, Gustafson DL. Drug-drug interaction between cannabidiol and phenobarbital in healthy dogs. Am J Vet Res. (2021) 83:86–94. doi: 10.2460/ajvr.21.08.0120
41. Bartner LR, McGrath S, Rao S, Hyatt LK, Wittenburg LA. Pharmacokinetics of cannabidiol administered by 3 delivery methods at 2 different dosages to healthy dogs. Can J Vet Res. (2018) 82:178–83.
42. Samara E, Bialer M, Mechoulam R. Pharmacokinetics of cannabidiol in dogs. Drug Metab Dispos. (1988) 16:469–72. doi: 10.1016/S0090-9556(25)06962-4
43. Zou S, Kumar U. cannabinoid receptors and the endocannabinoid system: signaling and function in the central nervous system. Int J Mol Sci. (2018) 19:833. doi: 10.3390/ijms19030833
44. Crocq MA. History of cannabis and the endocannabinoid system. Dialogues Clin Neurosci. (2020) 22:223–8. doi: 10.31887/DCNS.2020.22.3/mcrocq
45. Lu HC, Mackie K. Review of the endocannabinoid system. Biol Psychiatry Cogn Neurosci Neuroimaging. (2021) 6:607–15. doi: 10.1016/j.bpsc.2020.07.016
46. Di Marzo V. The endocannabinoidome as a substrate for noneuphoric phytocannabinoid action and gut microbiome dysfunction in neuropsychiatric disorders. Dialogues Clin Neurosci. (2020) 22:259–69. doi: 10.31887/DCNS.2020.22.3/vdimarzo
47. Pertwee R. The evidence for the existence of cannabinoid receptors. Gen Pharmacol. (1993) 24:811–24. doi: 10.1016/0306-3623(93)90154-P
48. Thomas A, Stevenson LA, Wease KN, Price MR, Baillie G, Ross RA, et al. Evidence that the plant cannabinoid Delta9-tetrahydrocannabivarin is a cannabinoid CB1 and CB2 receptor antagonist. Br J Pharmacol. (2005) 146:917–26. doi: 10.1038/sj.bjp.0706414
49. Pertwee RG. GPR55: a new member of the cannabinoid receptor clan? Br J Pharmacol. (2007) 152:984–6. doi: 10.1038/sj.bjp.0707464
50. Morales P, Hurst DP, Reggio PH. Molecular targets of the phytocannabinoids: a complex picture. Prog Chem Org Nat Prod. (2017) 103:103–31. doi: 10.1007/978-3-319-45541-9_4
51. Berthold EC, Kamble SH, Kanumuri SRR, Kuntz MA, Senetra AS, Chiang YH, et al. Comparative pharmacokinetics of commercially available cannabidiol isolate, broad-spectrum, and full-spectrum products. Eur J Drug Metab Pharmacokinet. (2023) 48:427–35. doi: 10.1007/s13318-023-00839-3
52. Di Salvo A, Conti MB, Della Rocca G. Pharmacokinetics, efficacy, and safety of cannabidiol in dogs: an update of current knowledge. Front Vet Sci. (2023) 10:1204526. doi: 10.3389/fvets.2023.1204526
53. Martinez V, Iriondo De-Hond A, Borrelli F, Capasso R, Del Castillo MD, Abalo R. Cannabidiol and other non-psychoactive cannabinoids for prevention and treatment of gastrointestinal disorders: useful nutraceuticals? Int J Mol Sci. (2020) 21:3067. doi: 10.3390/ijms21093067
54. Campos AC, Moreira FA, Gomes FV, Del Bel EA, Guimaraes FS. Multiple mechanisms involved in the large-spectrum therapeutic potential of cannabidiol in psychiatric disorders. Philos Trans R Soc Lond B Biol Sci. (2012) 367:3364–78. doi: 10.1098/rstb.2011.0389
55. Lucas CJ, Galettis P, Schneider J. The pharmacokinetics and the pharmacodynamics of cannabinoids. Br J Clin Pharmacol. (2018) 84:2477–82. doi: 10.1111/bcp.13710
56. Schwark WS, Wakshlag JJ. A One Health perspective on comparative cannabidiol and cannabidiolic acid pharmacokinetics and biotransformation in humans and domestic animals. Am J Vet Res. (2023) 84:31. doi: 10.2460/ajvr.23.02.0031
57. Court MH, Mealey KL, Burke NS, Jimenez TP, Zhu Z, Wakshlag JJ. Cannabidiol and cannabidiolic acid: Preliminary in vitro evaluation of metabolism and drug-drug interactions involving canine cytochrome P-450, UDP-glucuronosyltransferase, and P-glycoprotein. J Vet Pharmacol Ther. (2024) 47:1–13. doi: 10.1111/jvp.13403
58. Whalley BJ, Lin H, Bell L, Hill T, Patel A, Gray RA, et al. Species-specific susceptibility to cannabis-induced convulsions. Br J Pharmacol. (2019) 176:1506–23. doi: 10.1111/bph.14165
59. Anand U, Pacchetti B, Anand P, Sodergren MH. Cannabis-based medicines and pain: a review of potential synergistic and entourage effects. Pain Manag. (2021) 11:395–403. doi: 10.2217/pmt-2020-0110
61. Committee Committee for the Update of the Guide for the Care and Use of Laboratory Animals Institute for Laboratory Animal Research Division Division on Earth and Life Studies and National Research Council. Guide for the Care and Use of Laboratory Animals. 8th Edn. Washington, DC: National Academies Press (2010).
62. Barr CA, Gianotti G, Graffeo CE, Drobatz KJ, Silverstein DC. Effect of blood collection by the push-pull technique from an indwelling catheter vs. direct venipuncture on venous blood gas values before and after administration of alfaxalone or propofol in dogs. J Am Vet Med Assoc. (2017) 251:1166–74. doi: 10.2460/javma.251.10.1166
63. Altman PL, Dittmer DS. Biological Data Book. 2 ed. Bethesda, MD: Federation of American Societies of Experimental Biology (1974).
64. Garrett ER, Hunt CA. Pharmacokinetics of delta9-tetrahydrocannabinol in dogs. J Pharm Sci. (1977) 66:395–407. doi: 10.1002/jps.2600660322
65. Scheerans C, Heinig R, Mueck W. Proposal for defining the relevance of drug accumulation derived from single dose study data for modified release dosage forms. Biopharm Drug Dispos. (2015) 36:93–103. doi: 10.1002/bdd.1923
66. Meineke I, Gleiter CH. Assessment of drug accumulation in the evaluation of pharmacokinetic data. J Clin Pharmacol. (1998) 38:680–4. doi: 10.1002/j.1552-4604.1998.tb04806.x
67. Samara E, Bialer M, Harvey DJ. Pharmacokinetics of urinary metabolites of cannabidiol in the dog. Biopharm Drug Dispos. (1990) 11:785–95. doi: 10.1002/bdd.2510110906
68. Bookout W, Dziwenka M, Valm K, Kovacs-Nolan J. Safety study of cannabidiol products in healthy dogs. Front Vet Sci. (2024) 11:1349590. doi: 10.3389/fvets.2024.1349590
69. Samara E, Bialer M. Rapid high-performance liquid chromatographic assay with pharmacokinetic applications for monitoring cannabidiol in plasma. J Chromatogr. (1987) 416:370–4. doi: 10.1016/0378-4347(87)80522-4
70. Vaughn D, Kulpa J, Paulionis L. Preliminary investigation of the safety of escalating cannabinoid doses in healthy dogs. Front Vet Sci. (2020) 7:51. doi: 10.3389/fvets.2020.00051
71. Bradley S, Young S, Bakke AM, Holcombe L, Waller D, Hunt A, et al. Long-term daily feeding of cannabidiol is well-tolerated by healthy dogs. Front Vet Sci. (2022) 9:977457. doi: 10.3389/fvets.2022.977457
72. Corsato Alvarenga I, Gustafson D, Banks K, Wilson K, McGrath S. Cannabidiol plasma determination and pharmacokinetics conducted at beginning, middle and end of long-term supplementation of a broad-spectrum hemp oil to healthy adult dogs. Front Vet Sci. (2023) 10:1279926. doi: 10.3389/fvets.2023.1279926
73. Pang KS. Metabolite pharmacokinetics: the area under the curve of metabolite and the fractional rate of metabolism of a drug after different routes of administration for renally and hepatically cleared drugs and metabolites. J Pharmacokinet Biopharm. (1981) 9:477–87. doi: 10.1007/BF01060890
74. Harvey DJ, Samara E, Mechoulam R. Comparative metabolism of cannabidiol in dog, rat and man. Pharmacol Biochem Behav. (1991) 40:523–32. doi: 10.1016/0091-3057(91)90358-9
75. Samara E, Bialer M, Harvey DJ. Identification of glucose conjugates as major urinary metabolites of cannabidiol in the dog. Xenobiotica. (1990) 20:177–83. doi: 10.3109/00498259009047153
76. Samara E, Bialer M, Harvey DJ. Identification of urinary metabolites of cannabidiol in the dog. Drug Metab Dispos. (1990) 18:571–9. doi: 10.1016/S0090-9556(25)08593-9
77. Chandrasekaran B, Abed SN, Al-Attraqchi O, Kuche K, Tekade RK. Computer-Aided Prediction of Pharmacokinetic (ADMET) Properties. In: Tekade RK, , editor. Dosage Form Design Parameters. London: Academic Press (2018). p. 731–55. doi: 10.1016/B978-0-12-814421-3.00021-X
78. Murata Y, Neuhoff S, Rostami-Hodjegan A, Takita H, Al-Majdoub ZM, Ogungbenro K. In vitro to in vivo extrapolation linked to physiologically based pharmacokinetic models for assessing the brain drug disposition. AAPS J. (2022) 24:28. doi: 10.1208/s12248-021-00675-w
79. Miller RR, Madeira M, Wood HB, Geissler WM, Raab CE, Martin IJ. Integrating the impact of lipophilicity on potency and pharmacokinetic parameters enables the use of diverse chemical space during small molecule drug optimization. J Med Chem. (2020) 63:12156–70. doi: 10.1021/acs.jmedchem.9b01813
80. Child RB, Tallon MJ. Cannabidiol (CBD) dosing: plasma pharmacokinetics and effects on accumulation in skeletal muscle, liver and adipose tissue. Nutrients. (2022) 14:2101. doi: 10.3390/nu14102101
81. Turner SE, Knych HK, Adams AA. Pharmacokinetics of cannabidiol in a randomized crossover trial in senior horses. Am J Vet Res. (2022) 83:28. doi: 10.2460/ajvr.22.02.0028
82. Koch N, Jennotte O, Lechanteur A, Deville M, Charlier C, Cardot JM, et al. An intravenous pharmacokinetic study of cannabidiol solutions in piglets through the application of a validated ultra-high-pressure liquid chromatography coupled to tandem mass spectrometry method for the simultaneous quantification of CBD and its carboxylated metabolite in plasma. Pharmaceutics. (2024) 16:140. doi: 10.3390/pharmaceutics16010140
83. Ohlsson A, Lindgren JE, Andersson S, Agurell S, Gillespie H, Hollister LE. Single-dose kinetics of deuterium-labelled cannabidiol in man after smoking and intravenous administration. Biomed Environ Mass Spectrom. (1986) 13:77–83. doi: 10.1002/bms.1200130206
84. Khazaeinia T, Ramsey AA, Tam YK. The effects of exercise on the pharmacokinetics of drugs. J Pharm Pharm Sci. (2000) 3:292–302.
85. Pirttiaho HI, Sotaniemi EA, Pelkonen RO, Pitkanen U, Anttila M, Sundqvist H. Roles of hepatic blood flow and enzyme activity in the kinetics of propranolol and sotalol. Br J Clin Pharmacol. (1980) 9:399–405. doi: 10.1111/j.1365-2125.1980.tb01068.x
86. Pang KS, Rowland M. Hepatic clearance of drugs. III Additional experimental evidence supporting the “well-stirred” model, using metabolite (MEGX) generated from lidocaine under varying hepatic blood flow rates and linear conditions in the perfused rat liver in situ preparation. J Pharmacokinet Biopharm. (1977) 5:681–99. doi: 10.1007/BF01059690
87. Rowland M, Tozer TN. Clinical Pharmacokinetics: Concepts and Applications. Philadelphia: Lippincott Williams & Wilkins. (1995).
88. Cho S, Yoon Y-R. Understanding the pharmacokinetics of prodrug and metabolite. Transl Clin Pharmacol. (2018) 26:1–5. doi: 10.12793/tcp.2018.26.1.1
89. Della Rocca G, Paoletti F, Conti MB, Galarini R, Chiaradia E, Sforna M, et al. Pharmacokinetics of cannabidiol following single oral and oral transmucosal administration in dogs. Front Vet Sci. (2022) 9:1104152. doi: 10.3389/fvets.2022.1104152
90. Lyons C, McEwan K, Munn-Patterson M, Vuong S, Alcorn J, Chicoine A. Pharmacokinetic of two oral doses of a 1:20 THC:CBD cannabis herbal extract in cats. Front Vet Sci. (2024) 11:1352495. doi: 10.3389/fvets.2024.1352495
91. Bolognini D, Rock EM, Cluny NL, Cascio MG, Limebeer CL, Duncan M, et al. Cannabidiolic acid prevents vomiting in Suncus murinus and nausea-induced behaviour in rats by enhancing 5-HT1A receptor activation. Br J Pharmacol. (2013) 168:1456–70. doi: 10.1111/bph.12043
92. Bornheim LM, Grillo MP. Characterization of cytochrome P450 3A inactivation by cannabidiol: possible involvement of cannabidiol-hydroxyquinone as a P450 inactivator. Chem Res Toxicol. (1998) 11:1209–16. doi: 10.1021/tx9800598
93. Mozaffari K, Willette S, Lucker BF, Kovar SE, Holguin FO, Guzman I. The effects of food on cannabidiol bioaccessibility. Molecules. (2021) 26:3573. doi: 10.3390/molecules26123573
94. Harvey DJ, Brown NK. Comparative in vitro metabolism of the cannabinoids. Pharmacol Biochem Behav. (1991) 40:533–40. doi: 10.1016/0091-3057(91)90359-A
95. Harvey DJ, Paton WD. Identification of in vivo liver metabolites of delta 6-tetrahydrocannabinol produced by the mouse. Drug Metab Dispos. (1980) 8:178–86. doi: 10.1016/S0090-9556(25)00250-8
96. Harvey DJ, Martin BR, Paton WD. Identification of the glucuronides of cannabidiol and hydroxycannabidiols in mouse liver. Biochem Pharmacol. (1976) 25:2217–9. doi: 10.1016/0006-2952(76)90137-4
97. Hlozek T, Uttl L, Kaderabek L, Balikova M, Lhotkova E, Horsley RR, et al. Pharmacokinetic and behavioural profile of THC, CBD, and THC+CBD combination after pulmonary, oral, and subcutaneous administration in rats and confirmation of conversion in vivo of CBD to THC. Eur Neuropsychopharmacol. (2017) 27:1223–37. doi: 10.1016/j.euroneuro.2017.10.037
98. Hill E, Byrne W. 13 Detection time following administration of cannabidiol applied as an oral emulsified solution in Thoroughbred horses. J Equine Vet Sci. (2023) 124:104315. doi: 10.1016/j.jevs.2023.104315
99. Turner S, Knych HK, Adams AA. The effects of cannabidiol on immune function and health parameters in senior horses. Vet Immunol Immunopathol. (2023) 257:110549. doi: 10.1016/j.vetimm.2023.110549
100. Eichler F, Pozniak B, Machnik M, Schenk I, Wingender A, Baudisch N, et al. Pharmacokinetic modelling of orally administered cannabidiol and implications for medication control in horses. Front Vet Sci. (2023) 10:1234551. doi: 10.3389/fvets.2023.1234551
101. Ryan D, McKemie DS, Kass PH, Puschner B, Knych HK. Pharmacokinetics and effects on arachidonic acid metabolism of low doses of cannabidiol following oral administration to horses. Drug Test Anal. (2021) 13:1305–17. doi: 10.1002/dta.3028
102. Xu X, Murphy LA. Fast and sensitive LC-MS/MS method for quantification of cannabinoids and their metabolites in plasma of cattle fed hemp. J Sep Sci. (2024) 47:e2300630. doi: 10.1002/jssc.202300630
103. Beers JL, Fu D, Jackson KD. Cytochrome P450-catalyzed metabolism of cannabidiol to the active metabolite 7-hydroxy-cannabidiol. Drug Metab Dispos. (2021) 49:882–91. doi: 10.1124/dmd.120.000350
104. Ujvary I, Hanus L. Human metabolites of cannabidiol: a review on their formation, biological activity, and relevance in therapy. Cannabis Cannabinoid Res. (2016) 1:90–101. doi: 10.1089/can.2015.0012
105. Abbotts KSS, Ewell TR, Butterklee HM, Bomar MC, Akagi N, Dooley GP, et al. Cannabidiol and cannabidiol metabolites: pharmacokinetics, interaction with food, and influence on liver function. Nutrients. (2022) 14:2152. doi: 10.3390/nu14102152
106. Zhang Q, Melchert PW, Markowitz JS. Pharmacokinetic variability of oral cannabidiol and its major metabolites after short-term high-dose exposure in healthy subjects. Med Cannabis Cannabinoids. (2024) 7:1–9. doi: 10.1159/000535726
107. Pang KS, Gillette JR. Theoretical relationships between area under the curve and route of administration of drugs and their precursors for evaluating sites and pathways of metabolism. J Pharm Sci. (1978) 67:703–4. doi: 10.1002/jps.2600670536
108. Pang KS, Gillette JR. Sequential first-pass elimination of a metabolite derived from a precursor. J Pharmacokinet Biopharm. (1979) 7:275–90. doi: 10.1007/BF01060018
109. Pang KS, Gillette JR. Metabolite pharmacokinetics: methods for simultaneous estimates of elimination rate constants of a drug and its metabolite. A commentary. Drug Metab Dispos. (1980) 8:39–43. doi: 10.1016/S0090-9556(25)00218-1
110. Pang KS. A review of metabolite kinetics. J Pharmacokinet Biopharm. (1985) 13:633–62. doi: 10.1007/BF01058905
111. Yocom AF, O'Fallon ES, Gustafson DL, Contino EK. Pharmacokinetics, safety, and synovial fluid concentrations of single- and multiple-dose oral administration of 1 and 3 mg/kg cannabidiol in horses. J Equine Vet Sci. (2022) 113:103933. doi: 10.1016/j.jevs.2022.103933
112. Sanchez de Medina A, Serrano-Rodriguez JM, Diez de Castro E, Garcia-Valverde MT, Saitua A, Becero M, et al. Pharmacokinetics and oral bioavailability of cannabidiol in horses after intravenous and oral administration with oil and micellar formulations. Equine Vet J. (2023) 55:1094–103. doi: 10.1111/evj.13923
113. Joffe M, Joffe D. Comparing the prevalence of tetrahydrocannabinol (THC) toxicities in dogs before and after legalization of cannabis in Canada. Can Vet J. (2020) 61:997–9.
114. Garrett ER. Pharmacokinetics and disposition of delta 9-tetrahydrocannabinol and its metabolites. Adv Biosci. (1978) 22:105–21. doi: 10.1016/B978-0-08-023759-6.50014-7
115. Corsato Alvarenga I, Wilson KM, McGrath S. Tolerability of long-term cannabidiol supplementation to healthy adult dogs. J Vet Intern Med. (2024) 38:326–35. doi: 10.1111/jvim.16949
116. Mejia S, Duerr FM, Griffenhagen G, McGrath S. Evaluation of the effect of cannabidiol on naturally occurring osteoarthritis-associated pain: a pilot study in dogs. J Am Anim Hosp Assoc. (2021) 57:81–90. doi: 10.5326/JAAHA-MS-7119
117. Lo LA, Christiansen A, Eadie L, Strickland JC, Kim DD, Boivin M, et al. Cannabidiol-associated hepatotoxicity: a systematic review and meta-analysis. J Intern Med. (2023) 293:724–52. doi: 10.1111/joim.13627
118. Shilo-Benjamini Y, Lavy E, Yair N, Milgram J, Zilbersheid D, Hod A, et al. Therapeutic efficacy and pharmacokinetics of liposomal-cannabidiol injection: a pilot clinical study in dogs with naturally-occurring osteoarthritis. Front Vet Sci. (2023) 10:1224452. doi: 10.3389/fvets.2023.1224452
119. Tittle D, Wakshlag J, Schwark W, Lyubimov A, Zakharov A, Gomez B. Twenty-four hour and one-week steady state pharmacokinetics of cannabinoids in two formulations of cannabidiol and cannabidiolic acid rich hemp in dogs. Medical Research Archives. (2022) 10:2907. doi: 10.18103/mra.v10i7.2907
120. Sosa-Higareda M, Guzman DS, Knych H, Lyubimov A, Zakharov A, Gomez B, et al. Twice-daily oral administration of a cannabidiol and cannabidiolic acid-rich hemp extract was well tolerated in orange-winged Amazon parrots (Amazona amazonica) and has a favorable pharmacokinetic profile. Am J Vet Res. (2023) 84:197. doi: 10.2460/ajvr.22.11.0197
Keywords: dog, cannabidiol, pharmacokinetics, adverse effects, oral dosing, intravenous dosing
Citation: Kitts-Morgan SE, Sams RA and Muir WW (2025) Pharmacokinetics of cannabidiol, (-)-trans-Δ9-tetrahydrocannabinol, and their oxidative metabolites after intravenous and oral administration of a cannabidiol-dominant full-spectrum hemp product to beagle dogs. Front. Vet. Sci. 12:1556975. doi: 10.3389/fvets.2025.1556975
Received: 07 January 2025; Accepted: 28 February 2025;
Published: 08 April 2025.
Edited by:
Nora Mestorino, National University of La Plata, ArgentinaReviewed by:
Matthias Kohlhauer, École nationale vétérinaire d'Alfort (EnvA), FranceSasithorn Limsuwan, Kasetsart University, Thailand
Copyright © 2025 Kitts-Morgan, Sams and Muir. This is an open-access article distributed under the terms of the Creative Commons Attribution License (CC BY). The use, distribution or reproduction in other forums is permitted, provided the original author(s) and the copyright owner(s) are credited and that the original publication in this journal is cited, in accordance with accepted academic practice. No use, distribution or reproduction is permitted which does not comply with these terms.
*Correspondence: Susanna E. Kitts-Morgan, c3VzYW5uYS5raXR0cy1tb3JnYW5AbG11bmV0LmVkdQ==
†ORCID: Richard A. Sams orcid.org/0000-0002-1613-763X