- 1Experimental Zooprophylactic Institute of Southern Italy, Naples, Italy
- 2Department of Veterinary Medicine and Animal Production, University of Naples Federico II, Naples, Italy
- 3Department of Microbiology and Food Hygiene, Faculty of Veterinary Medicine, Lorestan University, Khorramabad, Iran
Introduction: Toxin-producing Escherichia coli are gastrointestinal agents found in both animals and humans, potentially leading to mild-to-severe pathogenic outcomes. Therefore, this study aimed to assess the prevalence of toxin-producing E. coli in owned and stray dogs and cats in Southern Italy in order to provide insights into the epidemiology of these zoonotic bacterial infections.
Methods: During necropsy, organ swabs (i.e., intestine, liver, lung, spleen, lymph node, and brain) from dogs and cats were collected and analyzed to isolate E. coli colonies through bacterial culture between 2017 and 2023. The isolated strains were then subjected to biomolecular investigation for pathogenicity factors.
Results: Out of 911 animals, 451 (49.5%) tested positive for E. coli, including 252 (56.1%) dogs and 199 (43.1%) cats. The higher prevalence among dogs was statistical significant (p < 0.01) and associated with a higher risk of infection (OR = 1.69). However, no statistically significant difference in prevalence over the years was found (p = 0.150). At least one virulence factor was detected in 22% of animals, with 12% exhibiting pathogenicity factors (CNF, CDT, LT, and ST) and 10% showing virulence genes (vtx1, vtx2, and eae). Cats were significantly more likely to produce verocytotoxin compared to dogs (p = 0.020, OR = 2.04).
Discussion: These findings suggest a wide circulation of toxin-producing E. coli in dogs and cats in Southern Italy, highlighting the importance of routine screening for these agents to ensure animal welfare and public health.
1 Introduction
Escherichia coli is a Gram-negative bacterium commonly found in the intestines of various species, including humans, dogs, and cats (1).
A wide variety of animal species, particularly cattle, serve as reservoirs of E. coli infections, hosting the microorganisms in their large intestines. Thus, animal-derived food products represent one of the primary sources of infection in humans (2).
Although the majority of E. coli strains are part of the normal gut flora and typically do not cause health issues (3), some strains can acquire virulence factors that make them pathogenic, enabling them to invade extraintestinal sites (4). These pathogenic strains of E. coli can be classified into several pathotypes: enterotoxigenic E. coli (ETEC), enteropathogenic E. coli (EPEC), verocytotoxin-producing E. coli (VTEC), and necrotoxigenic E. coli (NTEC), all of which are associated with infections in both animals and humans (5). NTEC strains are known for producing a cytotoxic necrotizing factor (CNF), which induces significant damage to host cells. CNF influences regulatory factors of the cellular cytoskeleton, triggering a cascade of events leading to cellular dysfunction and death (6). NTEC have been isolated from animals and humans, suggesting possible zoonotic transmission (7, 8).
The pathogenicity of VTEC strains is closely related to the production of exotoxins called verocytotoxin (vtx), a family of Shiga-like toxins causing haemorrhagic colitis and hemolytic uremic syndrome (HUS) (9). For instance, individual VTEC strains produce one or both toxins—vtx1 and vtx2—with the latter being associated with more severe disease forms (9). Enterotoxigenic E. coli (ETEC) is one of the leading causes of traveler’s diarrhea. Its virulence is characterized by the presence of specific adhesins and enterotoxins. ETEC toxins are divided into two groups: heat-stable enterotoxins (STI, STII) and heat-labile enterotoxins (LTI, LTII), both of which are associated with watery diarrhea and gastrointestinal illness (10).
Enteropathogenic E. coli (EPEC) is known to cause characteristic lesions, named attaching and effacing (A/E) lesions, which result in the effacement of microvilli and the formation of actin pedestals (11–13).
Therefore, this study aimed to investigate the prevalence of toxin-producing E. coli in dogs and cats in Southern Italy between 2017 and 2023, in order to assess the circulation of these agents and their potential health implications for animals and humans.
2 Materials and methods
2.1 Study area and sampling
From January 2017 to December 2023, veterinary practitioners in the Calabria and Campania regions (Southern Italy) collected carcasses of owned and stray dogs and cats after road accidents or apparent natural causes in accordance with National Laws (D.lgs. no. 194/2008 and 32/2021) by the Italian Ministry of Health were collected. All carcasses were delivered to the Animal Health Department of the Experimental Zooprophylactic Institute of Southern Italy (Naples, Italy) for a complete necropsy examination. The carcasses were dissected under sterile conditions, and cotton swabs were collected after cauterization with a spatula on the surface of the organ. Swabs were performed on organs displaying characteristic lesions, or in their absence, on target organs such as the intestine, liver, spleen, lung, and brain.
2.2 Cultures
For bacteriological examination, all the organ swabs were analyzed under a laminar flow hood on a plate of no-salt Agar McConkey (MCSS) and a plate of Tryptone Soya Agar +5% Blood (TSA), which were incubated aerobically at 37°C for 24 h. For intestinal swabs, sowing was carried out after preparing graduated dilutions in the ratio 1:10 in sterile peptone saline solution up to dilution 104 to obtain isolated colonies on the culture medium. The diluted solution (10 μL) was transferred to a sterile loop and sown on the solid medium, aerobically incubated at 37°C. After 24 h, Gram staining, as well as catalase and oxidase tests, were performed on both usual and questionable colonies. The suspicious colonies obtained by Gram staining and catalase/oxidase tests were biochemically confirmed at the genus/species level using the Vitek® 2 COMPACT automated system (Biomérieux, Marcy-l’Étoile, France). All E. coli colonies that tested positive for biochemical analysis were then subjected to molecular characterization to identify the presence of any genes encoding pathogenic factors.
2.3 DNA extraction and quantification
DNA was extracted from each E. coli strain using a boiling protocol (14) in which a single colony was suspended in 200 μL of water at 100°C for 10 min and pelleted with cellular debris. To assess the analytical sensitivity of the PCR assays, the DNA concentration of samples was determined by biophotometry BioPhotometer plus (Eppendorf AG, Hamburg, Germany).
2.4 PCR protocols
Virulence genes (i.e., vtx1, vtx2, and eae) were assessed by multiplex PCR in a total volume of 40 μL containing 25 μL HotStarTaq DNA Polymerase, 1 μL of each primer, 9 μL of Q solution (Qiagen, Hilden, Germany), and 10 μL of DNA template. The primer concentration was 0.2 μM, and the amplification conditions included an initial denaturation at 95°C for 15 min, followed by 35 cycles of 1 min of denaturation at 95°C, 2 min of annealing at 65°C for the first 10 cycles, decrementing to 60°C by cycle 15, and 1.5 min of elongation at 72°C incrementing to 2.5 min from cycles 25 to 35.
For CDT and CNF, an end-point PCR was run in a total volume of 50 μL containing 25 μL of Master mix Promega, 3 μL of each primer, and 2 μL of DNA template. The primer concentration was 0.6 μM, and amplification conditions included an initial denaturation step at 94°C for 2 min, followed by 30 cycles, consisting of 30 s of denaturation at 94°C, 1 min of annealing at 52°C, and 2 min of elongation at 72°C, with a final extension step of 5 min at 72°C.
For LT and ST, an end-point PCR was run in a total volume of 25 μL containing 12.5 μL of HotStarTaq DNA Polymerase (Qiagen, Hilden, Germany), 1.25 μL of each primer, and 3 μL of DNA template. The primer concentration was 0.5 μM, and the amplification conditions included an initial denaturation step at 95°C for 15 min, followed by 35 cycles of 1.5 min of denaturation at 94°C, 1.5 min of annealing at 60°C, and 1.5 min of elongation at 72°C, with a final extension step of 7 min at 72°C. The amplicons obtained were visualized by automated capillary electrophoresis with QIAxcel instrument (Qiagen, Hilden, Germany).
All details of the primers used in this study are listed in Table 1 (15–19).
2.5 Statistical analysis
Exact binomial 95% confidence intervals (CIs) were established for the total proportions of infection found herein. The exact Fisher’s test was used to assess statistical differences in infection rates between animal species and collection year; p-values less than 0.05 were considered statistically significant. Odds ratio (OR) values were calculated to assess the risk of infection according to animal species. Statistical analyses were performed using the online software Epitools - Epidemiological Calculators (20).
3 Results
A total number of 911 animals were collected and examined in this study, including 449 dogs and 462 cats. Out of 911 animals, 451 (i.e., 49.5, 95% CI: 46.3–52.7) tested positive for E. coli, including 252 (56.1%) that were dogs and 199 (43.1%) that were cats, respectively. The higher prevalence of dogs found herein was statistically significant (p < 0.01), combined with a higher risk of infection (OR = 1.69). There was no significant statistical difference in prevalence across the years (p = 0.150) (Table 2).
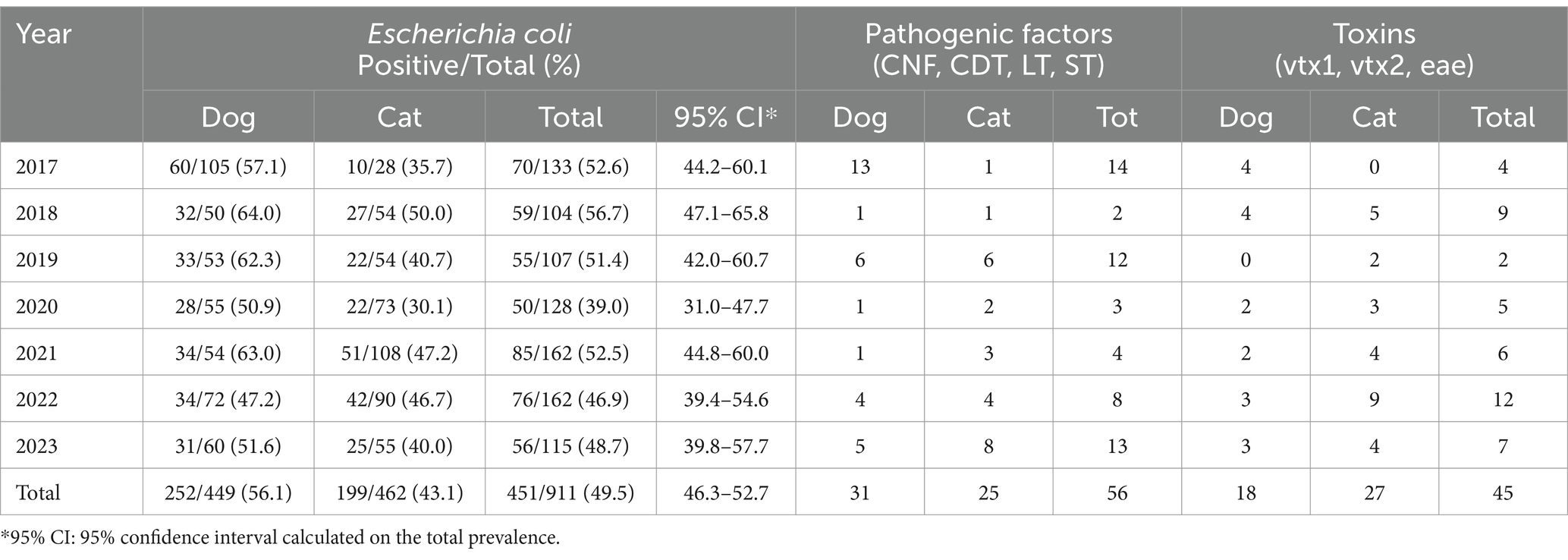
Table 2. Positivity of dogs and cats to Escherichia coli infection and related pathogenic factors and toxins in this study.
At least one virulence factor was detected in 22% of the animals (i.e., 12.4% for pathogenicity factors CNF, CDT, LT, ST, and 10.0% for virulence genes vtx1, vtx2, and eae). For CNF, CDT, LT, and ST, there was no significant difference between dogs and cats found (p = 1.680); however, cats were significantly more susceptible to verocytotoxins than dogs (p = 0.020, OR = 2.04).
Out of 252 dogs and 199 cats positive for E. coli infections, 31 dogs (i.e., 12.3, 95% CI: 8.8–16.9) and 25 cats (12.6, 95% CI: 8.7–17.9) had shown positivity for at least one toxin (CNF, CDT, LT, and ST); regarding verocytotoxins (vtx1, vtx2, eae), 18 dogs (7.1, 95% CI: 4.6–11.0) and 27 cats (i.e., 13.6, 95% CI: 9.5–19.0).
4 Discussion
The high prevalence of E. coli in dogs and cats in this study indicates a wide circulation of the infection in both owned and stray animals in Southern Italy, underlying the importance of routine screening for this bacterium to guarantee animal welfare and public health. The screening could be relevant mainly in rural/peri-urban areas with abundant phenomena of free-ranging animals, where stray dogs and cats may overlap with livestock and other companion animals. Indeed, dogs and cats remain under-diagnosed, and given their close proximity to other animals and humans in urban/peri-urban areas, the transmission of the microorganism is expected (1). In this regard, Ahmed et al. (21) highlights the role of companion animals in the spread of STEC strains in the environment. Contacts between animals and their related owners may facilitate the transmission of these strains to humans, as E. coli can transfer virulence genes via a horizontal transmission pathway (22, 23). For instance, a recent case of haemolytic uremic syndrome has been ascertained in a child who lived with a cat that tested positive for verocytotoxin-producing E. coli but showed no signs/symptoms, indicating that this pet was a reservoir of infection for humans (24). Dogs had a higher prevalence of E. coli than cats (56.1% vs 43.1%, p < 0.01), suggesting potentially important differences in the role each species plays as a carrier/reservoir of bacteria that require further investigations.
The absence of a significant statistical difference in prevalence through the years (p = 0.150) suggests that the stable circulation of E. coli in the study area.
Regarding toxin-producing E. coli, very few is known in the literature about dogs and cats (25). The present study highlights a high percentage (i.e., 22%) of animals harboring toxin-producing E. coli, indicating the potential role of companion animals in the spread of these zoonotic agents. The risk of infection should be considered, especially when dogs and cats share the same environment with children and immunosuppressed individuals who are more susceptible to contracting the disease in its severe form (26).
The high prevalence of both pathogenicity factor genes (12.4%) and virulence genes (10.0%) is particularly noteworthy as these genes are linked to significant gastrointestinal diseases in humans (5, 6), such as hemorrhagic colitis and haemolytic-uremic syndrome (6).
In particular, the positivity for E. coli toxins (CNF, CDT, LT, and ST) occurs similarly in dogs (12.3%) and cats (12.6%), whereas verocytotoxins (vtx1, vtx2, and eae) are found in these animals with different prevalence values (7.1% dogs and 13.6% cats). These findings suggest that whereas dogs have a higher prevalence of E. coli infection, cats have a higher incidence of virulence factors/verocytotoxins. These findings are supported by studies in the literature, which show that the prevalence of toxin-producing E. coli in dogs ranges from 2.9 to 12.3% in dogs and 13.8 to 23.1% in cats (25).
The findings that cats are significantly more suitable for verocytotoxin production than dogs (p = 0.020, OR = 2.04) suggest that these felids may act as the main reservoir for toxin-producing E. coli (27). However, this hypothesis deserves insights focused on the ecological factors of cats (e.g., dietary habits, environmental exposure, and gut microbiota) that may be involved in the prevalence between cats and dogs (28).
In this context, multicentre studies examining seasonal fluctuations in prevalence or exploring regional differences across broader geographic areas would provide more comprehensive insights.
Finally, our data suggest that epidemiological investigations should be routinized with a holistic approach considering the role of pets such as dogs and cats in the multiple routes of transmission, contamination, and infection by E. coli.
Data availability statement
The raw data supporting the conclusions of this article will be made available by the authors, without undue reservation.
Ethics statement
Ethical approval was not required for the studies involving animals in accordance with the local legislation and institutional requirements because. The ethical approval was not required since the work was done on deceased animals. Written informed consent was not obtained from the owners for the participation of their animals in this study because the work was done on animals that arrived to the Experimental Zooprophylactic Institute of Southern Italy after death within a routinary necropsy examination.
Author contributions
GDL: Conceptualization, Investigation, Writing – original draft. GC: Conceptualization, Data curation, Investigation, Methodology, Writing – review & editing. LB: Writing – review & editing. PI: Data curation, Methodology, Writing – review & editing. NR: Data curation, Methodology, Writing – review & editing. FDP: Data curation, Methodology, Writing – review & editing. OV: Data curation, Methodology, Writing – review & editing. AG: Data curation, Methodology, Writing – review & editing. CA: Data curation, Methodology, Writing – review & editing. FA: Data curation, Methodology, Writing – review & editing. MP: Formal analysis, Writing – review & editing. GS: Writing – review & editing. PK: Formal analysis, Writing – review & editing. ND'A: Formal analysis, Writing – review & editing. GF: Resources, Supervision, Writing – review & editing. MGL: Project administration, Resources, Writing – review & editing.
Funding
The author(s) declare that financial support was received for the research and/or publication of this article. This work was supported by the project entitled “Antibiotico resistenza il ruolo della avifauna selvatica (ARAS)” (grant no. IZS PB 01/23 RC) funded by the Italian Ministry of Health.
Conflict of interest
The authors declare that the research was conducted in the absence of any commercial or financial relationships that could be construed as a potential conflict of interest.
Generative AI statement
The authors declare that no Gen AI was used in the creation of this manuscript.
Publisher’s note
All claims expressed in this article are solely those of the authors and do not necessarily represent those of their affiliated organizations, or those of the publisher, the editors and the reviewers. Any product that may be evaluated in this article, or claim that may be made by its manufacturer, is not guaranteed or endorsed by the publisher.
References
1. Quinn, PJ, Markey, BK, Carter, ME, Donnelly, WJ, and Leonard, FC. Veterinary microbiology and microbiology disease. Oxford: Blackwell Science (2002).
2. World Health Organization. E. coli (2024) Available online at:https://www.who.int/news-room/fact-sheets/detail/e-coli#:~:text=It%20is%20transmitted%20to%20humans,contaminated%20raw%20vegetables%20and%20sprouts [Accessed October 13, 2024).
3. Leimbach, A, Hacker, J, and Dobrindt, U. E. coli as an all-rounder: the thin line between commensalism and pathogenicity. Curr Top Microbiol Immunol. (2013) 358:3–32. doi: 10.1007/82_2012_303
4. Yun, CS, Moon, BY, Hwang, MH, Lee, SK, Ku, BK, and Lee, K. Characterization of the pathogenicity of extraintestinal pathogenic Escherichia coli isolates from pneumonia-infected lung samples of dogs and cats in South Korea. Sci Rep. (2023) 13:5575. doi: 10.1038/s41598-023-32287-z
5. Borriello, G, Lucibelli, MG, De Carlo, E, Auriemma, C, Cozza, D, Ascione, G, et al. Characterization of enterotoxigenic E. coli (ETEC), Shiga-toxin producing E. coli (STEC) and necrotoxigenic E. coli (NTEC) isolated from diarrhoeic Mediterranean water buffalo calves (Bubalus bubalis). Res Vet Sci. (2012) 93:18–22. doi: 10.1016/j.rvsc.2011.05.009
6. Kadhum, HJ, Ball, HJ, Oswald, E, and Rowe, MT. Characteristics of cytotoxic necrotizing factor and cytolethal distending toxin producing Escherichia coli strains isolated from meat samples in Northern Ireland. Food Microbiol. (2006) 23:491–7. doi: 10.1016/j.fm.2005.07.003
7. Blanco, J, Blanco, M, and Blanco, JE. Virulence factors of necrotizing Escherichia coli strains isolated from animals. J Med Microbiol. (1990) 33:177–82.
8. Caprioli, A, Morabito, S, Brugère, H, and Oswald, E. Enterohaemorrhagic Escherichia coli: emerging issues on virulence and modes of transmission. Vet Res. (2005) 36:289–311. doi: 10.1051/vetres:2005002
9. Karmali, MA. Infection by verocytotoxin-producing Escherichia coli. Clin Microbiol Rev. (1989) 2:15–38. doi: 10.1128/CMR.2.1.15
10. Kolenda, R, Burdukiewicz, M, and Schierack, P. A systematic review and meta-analysis of the epidemiology of pathogenic Escherichia coli of calves and the role of calves as reservoirs for human pathogenic E. coli. Front Cell Infect Microbiol. (2015) 5:23. doi: 10.3389/fcimb.2015.00023
11. Hua, Y, Bai, X, Zhang, J, Jernberg, C, Chromek, M, Hansson, S, et al. Molecular characteristics of EAE-positive clinical Shiga toxin-producing Escherichia coli in Sweden. Emerg Microbes Infect. (2020) 9:2562–70. doi: 10.1080/22221751.2020.1850182
12. Luo, Y, Frey, EA, Pfuetzner, RA, Creagh, AL, Knoechel, DG, Haynes, CA, et al. Crystal structure of enteropathogenic Escherichia coli intimin-receptor complex. Nature. (2000) 405:1073–7. doi: 10.1038/35016618
13. Oswald, E, Schmidt, H, Morabito, S, Karch, SH, Marchès, O, and Caprioli, A. Typing of intimin genes in human and animal enterohemorrhagic and enteropathogenic Escherichia coli: characterization of a new intimin variant. Infect Immun. (2000) 68:64–71. doi: 10.1128/IAI.68.1.64-71.2000
14. Queipo-Ortuño, MI, De Dios, CJ, Macias, M, Bravo, MJ, and Morata, P. Preparation of bacterial DNA template by boiling and effect of immunoglobulin gas an inhibitor in real-time PCR for serum samples from patients with brucellosis. Clin Vaccine Immunol. (2008) 15:293–6. doi: 10.1128/CVI.00270-07
15. Paton, AW, and Paton, JC. Detection and characterization of Shiga toxigenic Escherichia coli by using multiplex PCR assays for stx1, stx2, eaeA, enterohemorrhagic E. coli hlyA, rfb O111, and rfb O157. J Clin Microbiol. (1998) 36:598–602. doi: 10.1128/JCM.36.2.598-602.1998
16. Toth, I, Hérault, F, Beutin, L, and Oswald, E. Production of cytolethal distending toxins by pathogenic Escherichia coli strains isolated from human and animal sources: establishment of the existence of a new cdt variant (type IV). J Clin Microbiol. (2003) 41:4285–91. doi: 10.1128/JCM.41.9.4285-4291.2003
17. Nüesch-Inderbinen, M, Stevens, MJA, Cernela, N, Müller, A, Biggel, M, and Stephan, R. Distribution of virulence factors, antimicrobial resistance genes and phylogenetic relatedness among Shiga toxin-producing Escherichia coli serogroup O91 from human infections. Int J Med Microbiol. (2021) 311:151541. doi: 10.1016/j.ijmm.2021.151541
18. Vereecke, N, Van Hoorde, S, Sperling, D, Theuns, S, Devriendt, B, and Cox, E. Virotyping and genetic antimicrobial susceptibility testing of porcine ETEC/STEC strains and associated plasmid types. Front Microbiol. (2023) 14:1139312. doi: 10.3389/fmicb.2023.1139312
19. Nishikawa, Y, Zhou, Z, Hase, A, Ogasawara, J, Kitase, T, Abe, N, et al. Diarrheagenic Escherichia coli isolated from stools of sporadic cases of diarrheal illness in Osaka City, Japan between 1997 and 2000: prevalence of enteroaggregative E. coli heat-stable enterotoxin 1 gene-possessing E. coli. Jpn J Infect Dis. (2002) 55:183–90. doi: 10.7883/yoken.JJID.2002.183
20. Sergeant ESG. Epitools: Epidemiological Calculators (2018) Available online at: https://epitools.ausvet.com.au/. [Accessed December 10, 2024].
21. Ahmed, W, Gyawali, P, and Toze, S. Quantitative PCR measurements of Escherichia coli including Shiga toxin-producing E. coli (STEC) in animal feces and environmental waters. Environ Sci Technol. (2015) 49:3084–90. doi: 10.1021/es505477n
22. Rumi, MV, Irino, K, Deza, N, Huguet, MJ, and Bentancor, AB. First isolation in Argentina of a highly virulent Shiga toxin-producing Escherichia coli O145:NM from a domestic cat. J Infect Dev Ctries. (2012) 6:358–63. doi: 10.3855/jidc.2225
23. Vračar, V, Potkonjak, A, Spasojević Kosić, L, Lalošević, V, and Kozoderović, G. First molecular detection of shiga toxin-producing Escherichia coli in dogs from Serbia: a potential threat to human health? Vet Ital. (2023) 59:1–6. doi: 10.12834/VetIt.2569.18469.2
24. Das, S, Kabir, A, Chouhan, CS, Shahid, MAH, Habib, T, Rahman, M, et al. Domestic cats are potential reservoirs of multidrug-resistant human enteropathogenic E. coli strains in Bangladesh. Saudi J Biol Sci. (2023) 30:103786. doi: 10.1016/j.sjbs.2023.103786
25. Galarce, N, Escobar, B, Sánchez, F, Paredes-Osses, E, Alegría-Morán, R, and Borie, C. Virulence genes, Shiga toxin subtypes, Serogroups, and clonal relationship of Shiga toxin-producing Escherichia Coli strains isolated from livestock and companion animals. Animals (Basel). (2019) 9:733. doi: 10.3390/ani9100733
26. Kreiniz, N, Abu-Ahmad, A, Golan-Shany, O, Paz, A, and Tadmor, T. Shiga-like toxin-producing Escherichia coli inducing diarrhea in patients with multiple myeloma diagnosed by biofire PCR-film array. Acta Haematol. (2019) 142:187–9. doi: 10.1159/000498906
27. Caliman, MW, and Marin, JM. Virulence genes in Escherichia coli isolated from feces and urine of cats. Arq Bras Med Vet Zootec. (2014) 66:1287–90. doi: 10.1590/1678-6694
Keywords: cat, dog, Escherichia coli, Italy, public health, toxin
Citation: De Luca G, Costantini G, Borrelli L, Izzo P, Riccone N, Del Piano F, Valvini O, Gallo A, Auriemma C, Alfano F, Paone M, Sgroi G, Khademi P, D’Alessio N, Fusco G and Lucibelli MG (2025) Toxin-producing Escherichia coli: a long-term retrospective study in dogs and cats between 2017 and 2023 in Italy. Front. Vet. Sci. 12:1557445. doi: 10.3389/fvets.2025.1557445
Edited by:
Victoria J. Brookes, Sydney School of Veterinary Science, AustraliaReviewed by:
Joycee Jogi, Nanaji Deshmukh Veterinary Science University, IndiaSwati Sahay, Birsa Agricultural University, India
Copyright © 2025 De Luca, Costantini, Borrelli, Izzo, Riccone, Del Piano, Valvini, Gallo, Auriemma, Alfano, Paone, Sgroi, Khademi, D’Alessio, Fusco and Lucibelli. This is an open-access article distributed under the terms of the Creative Commons Attribution License (CC BY). The use, distribution or reproduction in other forums is permitted, provided the original author(s) and the copyright owner(s) are credited and that the original publication in this journal is cited, in accordance with accepted academic practice. No use, distribution or reproduction is permitted which does not comply with these terms.
*Correspondence: Giovanni Sgroi, Z2lvdmFubmkuc2dyb2lAaXpzbXBvcnRpY2kuaXQ=