- 1Department of Microbiology, Faculty of Veterinary Medicine, Zagazig University, Zagazig, Egypt
- 2Department of Forensic Medicine and Toxicology, Faculty of Veterinary Medicine, Zagazig University, Zagazig, Egypt
- 3Department of Husbandry and Development of Animal Wealth, Faculty of Veterinary Medicine, Menoufia University, Shebin Alkom, Egypt
- 4Department of Pathobiochemistry, Faculty of Pharmacy, Meijo University, Nagoya, Japan
- 5Department of Nutrition and Animal Husbandry, University of Veterinary Medicine and Pharmacy, Košice, Komenského, Slovakia
For enhancing the nutritional characteristics of Moringa oleifera leaves (MOLs), the present research set out to examine the effect of MOLs fermented by Lactobacillus plantarum (MOLF) or MOLs powder (MOLP) on innate immunity defense and resilience to Aeromonas hydrophila challenge in Oreochromis niloticus. A 30-day experiment was conducted with 180 Nile tilapia fingerlings, divided randomly into five equal-sized groups of 36 fingerlings, three replicates per group. The 1st control fish received a basal meal devoid of any supplements. The 2nd MOLP-L and the 3rd MOLP-H fish received basal meals enriched with low and high levels of MOLP (50 g or 100 g/kg diet). The 4th MOLF-L and 5th MOLF-H fish received basal meals enriched with low and high levels of MOLF (50 g or 100 g/kg diet). Ferulic acid, gallic acid, caffeic acid, and p-coumaric acid were the primary phenolic components identified by HPLC in the fermented MOLs. Meanwhile, naringenin, rutin, quercetin, kaempferol, luteolin, apigenin, and catechin were the main flavonoids detected. The results revealed that MOLF dietary supplementation enhanced the immune-related outcomes more significantly (P < 0.05) than MOLP in a dose-related manner. Supplementation of MOLF increased serum nitric oxide and lysozyme levels, phagocytic index, phagocytic %, hepatic superoxide dismutase, and glutathione, yet declined the levels of malondialdehyde more significantly (P < 0.05) than the MOLP. The proinflammatory genes IL1β, TNFα, and IL-2 were significantly (P < 0.0.05) down-regulated. In contrast, the expression of the IL-10 gene was markedly upregulated in the spleen and head kidney (anterior) post A. hydrophila challenge in the MOLF-groups than the MOLP-groups. MOLF-supplemented groups showed a significantly (P < 0.05) enhanced relative proportion of survivorship and survival rates but decreased the A. hydrophila bacterial load (CFU) compared to the MOLP-supplemented groups. In conclusion, our findings have offered new insights into the promising immune-enhancing outcome of MOLF as a dietary supplement for immune augmentation against disease challenges in Nile tilapia.
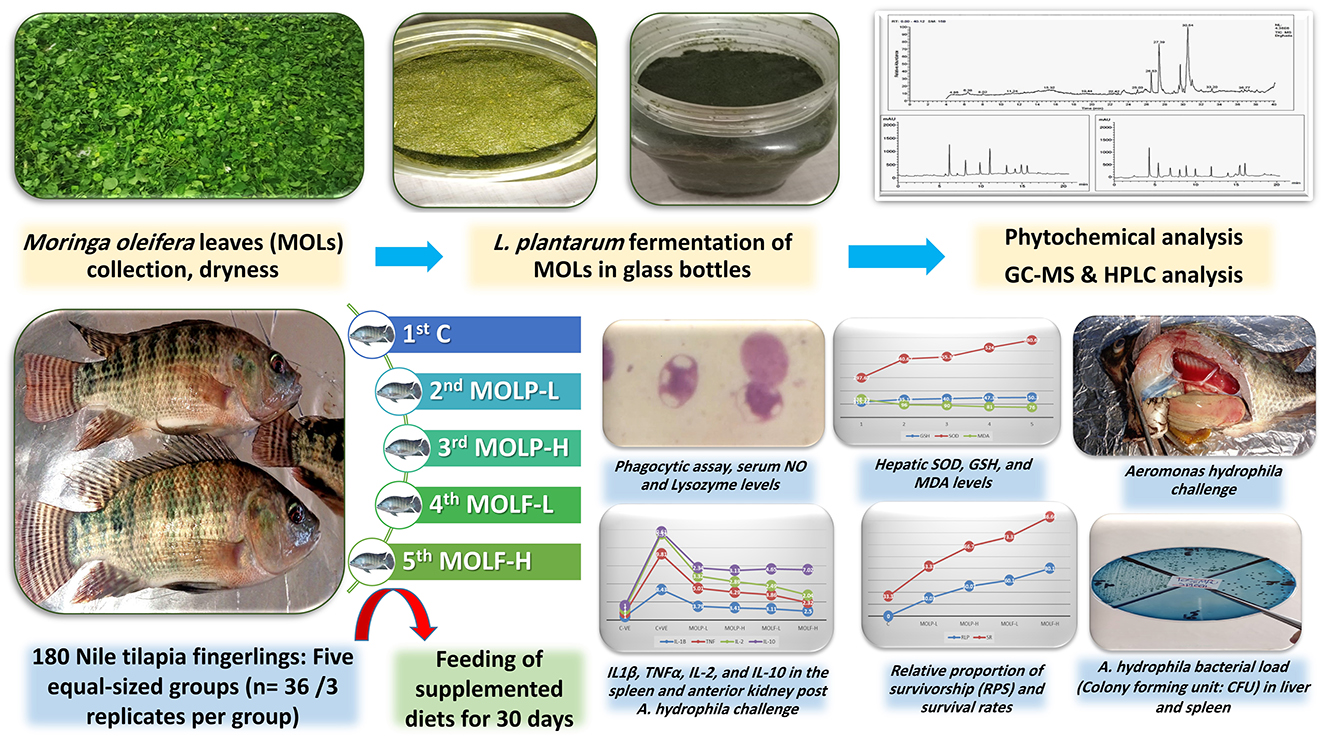
Graphical Abstract. Nutritional supplement of L. plantarum fermented M. oleifera leaves to Nile tilapia feeds could enhance the immune-related responses, antioxidant potential, and mRNA regulation of immunity-related genes, along with enhancing Nile tilapia resistance to A. hydrophila challenge.
1 Introduction
Aquaculture delivers essential nourishment to the human population through high-quality proteins mainly sourced from several fish species (1). Aquatic species, especially fish, are extensively cultivated and significantly alleviate hunger in the human population (2). Nile tilapia (Oreochromis niloticus) constitutes the foundation of the aquaculture industry, yielding significant economic benefits owing to its superior flesh quality, competitive market pricing, elevated survival rate, and efficient feeding practices (3). The Nile tilapia's rapid growth rate, inexpensive production costs, and strong resistance to harsh circumstances make it one of the most significant commercial freshwater fish in aquaculture globally.
According to statistics from the U.S. Department of Agriculture's Foreign Agricultural Service, Egypt is the top producer of Tilapia globally and aquaculture in Africa (4). Outbreaks of bacterial infections, however, pose a significant danger to O. niloticus species and, by extension, Egypt's aquaculture industry, reducing fish production and killing an estimated 80% of the fish stock annually (5). Aeromonas hydrophila (A. hydrophila) is a Gram-negative, highly pathogenic bacterium that may accelerate epidemics and thrive in freshwater fish settings (6). It causes motile Aeromonas septicemia in cultured O. niloticus, which in turn causes bleeding septicemia and high mortality rates (3, 5).
The utilization of herbal remedies has shown a drastic increase in the 20th century, as generally, all officially established medications are natural herbs (7). Moringa oleifera (M. oleifera), sometimes called the “Diamond of Plants”, “Tree of Life,” or “Tree of Wonders,” is a medical and dietary homologous herbal that has substantial health benefits for conditions like cancer, diabetes, hypertension, skin conditions, immunodeficiency, arthritis, and more (8, 9). Due to its capacity to enhance the healthiness, food conversion efficacy, performance during growth, and quality of products of various livestock animals, M. oleifera is extensively grown in nations of Asia and Africa and is preferred by the local population (10). According to research on aquatic animals, feeding MOL's extract may increase immune-related genes. Giant freshwater prawns (Macrobranchium rosenbergii) growth, immune-related gene expression, and resistance to V. anguillarum (11), while a 1% extract enhanced antioxidant activities and resistance to the Photobacterium damselae infection of whiteleg shrimp (Penaeus vannamei) (12). MOLP supplementation at 1.5 to 5% in the diet effectively improved immunity and reduced infection in Nile tilapia (O niloticus) (13). At 20% in the diet, it improved rainbow trout's (Oncorhyncus mykiss) immune parameters and antioxidant activity (14). Additionally, at 15% in the diet, it improved the growth and immune responses of Ctenopharyngodon idella (grass carp) (15). Common carp (Cyprinus carpio) showed enhanced immunity and stress tolerance when mixed with an aqueous extract at a rate of 20 milliliters per kilogram of feed (16). Moringa plant contains phytochemicals such as phenolic acid, flavonoids, carotenoids, alkaloids, tannin, lectin, and terpenoids (17), which have antioxidant, antistress, and immunostimulatory activities in gilthead seabream (Sparus aurata) and Nile tilapia (18–20).
Investigating M. oleifera's main components and bioactivities and outlining its beneficial outcomes in nutritional treatments for animals and humans is extremely important and valuable (21). The miracle tree, or M. oleifera, is a member of the Moringaceae family widely cultivated worldwide because it remains the most nutrient-dense plant ever identified (22). MOLs are fully rich in vitamins C, B, A, E, and D; carotenes and beta-carotenes; minerals like iron and potassium; proteins; essential amino acids; fats; and numerous phytochemical components like alkaloids, terpenes, glucosinolates, flavonoids, phenols, and so on. It is a highly nutritious food with health issues-related significance (23).
M. oleifera has been dubbed a “superfood” and has increased in popularity. Almost every part of the tree is edible or has therapeutic qualities. Despite their nutrient-dense composition and many health advantages, MOLs are abundant in fundamental antinutritional inhibiting and toxic compounds, including alkaloids, tannins, oxalates, saponins, free phytates, and cyanogenetic glycosides. They have comparatively small concentrations of trypsin and amylase inhibitors. The listed antinutrients restrict the biological availability of vital nutrients and impede their digestion and absorption when ingested with nutrient-dense foods (24).
The enhanced usage of M. oleifera requires technical enhancement to guarantee the consumers' safety, improve the bioavailability of nutrients, and reduce production costs for health-promoting M. oleifera formulations (25). Various methods have been widely developed to minimize the impact of intrinsic toxicants and their secondary metabolites. These methods include membrane transformation, treatment with calcium chloride, radiation, exchange of ions, extraction of salty and aquatic contents, solubility differences, enzymatic handling, and microwave handling. Research indicates that food processing techniques such as drying, blanching, de-fatting, and sprouting retain the most significant impact on anti-nutritious agents and toxicants. These techniques improve the food's nutritional qualities and reduce or eliminate the activity of toxic substances through enzyme activity. Another method, heat and soaking treatments, destroyed proteins and resulted in water-soluble nutrients loss (26).
Fermentation is one of several processing methods that have been proposed as a method to improve plant foods' sensory qualities and nutrient delivery capabilities that are degraded by other processing methods (27). The anaerobic degradation of organic molecules by microorganisms and products of microbial origin is known as lactic acid fermentation. Due to this procedure; fermented foods have better texture, taste, and nutritional components (28). Many species of lactic acid bacteria (LAB), such as L. plantarum, L. paracasei, and L. bulgaricus, are known to carry out fermentation. These bacteria have probiotic qualities in addition to their capacity for fermentation (29). Moreover, due to their unique metabolic activity, LABs have antibacterial components. Proteolytic enzymes, bacteriocin synthesis, citrate absorption, metal ion resistance, bacteriophage resistance, polysaccharide biosynthesis, and antibiotics are a few of these (30).
Additionally, Lactobacillus species can degrade a variety of substances originating from plants, including phytates and polyphenols (31), trypsin-inhibiting agents, tannins (32), and oxalates (33). Few studies have documented the dynamic alterations in nutritional and botanical components during fermentation. In contrast, fewer studies have shown the antioxidative capabilities of M. oleifera's food-grade portions throughout the fermentation process.
Nowadays, microbial fermentation is used extensively as one of the fundamental methods for releasing nutrients. This is owing to catabolism throughout the fermentation, which breaks down complex molecules into simpler forms that cells can absorb readily via microbial action. For instance, carbohydrates are converted to glucose, proteins break down into amino acids, and lipids into fatty acids and glycerol (34). Furthermore, fermented substances' biological qualities are enhanced, and novel characteristics are often found (35). The health-harming antinutrients, such as oxalic and phytic acids, are broken down during fermentation. Fermentation can convert a plant's phenolic substances, thereby increasing the bioactivities of such components (36). Several enzymes produced by probiotics during the fermentation accelerate the hydrolysis of glycosidic bonds in some phenolic complexes. The total of free polyphenols could be enhanced by enzymatic hydrolysis (37).
Therefore, the purpose of this study was to investigate the effects of consuming fermented M. oleifera leaves using L. plantaurum or dried M. oleifera leaves powder on phagocytosis, innate immune status, oxidative stress, and immunity-linked gene transcriptional regulations in Nile tilapia, as well as disease resistance against A. hydrophila challenge.
2 Materials and methods
2.1 Collecting, authenticating, and fermenting MOLs
The Egyptian Scientific Society of Moringa, housed at the National Research Center in Dokki, Egypt, was contacted to get MOLs. The leaves were thoroughly rinsed under a stream of water to eliminate any sand or other contaminants. Subsequently, they were left to dry naturally in the open air without exposure to direct sunlight and were regularly rotated to prevent the growth of fungi. After 5 days, a fine powder was formulated by grinding MOLs, which could pass through a screen with a mesh size of 0.15 mm. The leaf meal was securely enclosed in polythene plastic bags, sealed, and stored at ambient temperature until needed.
Glass bottles measuring 1,000 mL were used. About 200 g of powdered MOLs were introduced into the bottles and then processed for 20 min under a sterile environment at 121°C. Afterward, the powder was cooled, and 108 CFU/g of probiotic stock L. plantarum was inoculated into the powder. The medium's water content was based on the powders' absorption capacity. The mixture was homogenized in a sterile condition using a sterile glass rod. For 5 days, the fermentation process was done at 37°C (38).
2.2 Gas chromatography-mass spectrometry (GC-MS) analysis of fermented M. oleifera leaves
According to the protocol described by (39), a Trace GC-TSQ mass spectrometer (Thermo Scientific, Austin, TX, USA) equipped with a direct capillary column TG-5MS (0.25 mm × 30 m × 0.25 μm film thickness), the key compositions of the MOLF fine powder were examined. The column oven temperature was initially held at 50°C and then increased by the rate of 5°C/min to 250°C held for 2 min, then increased to the final temperature of 300°C by 30°C/min and held for 2 mins. The temperature in the MS transfer line and injector was maintained at 260°C and 270°C, respectively. Helium was employed as the carrier gas, and the flow rate was maintained at 1 mL/min. A 1 μL diluted sample was mechanically inoculated with 4 mins delayed solvent using a split-method Autosampler AS1300 paired with GC. In the full scan mode, electron ionization mass spectra were obtained spanning the m/z 50–650 range using an ionization voltage of 70 eV, covering. Around 200°C was used to heat the ion source. The GC components were identified by matching their mass spectra with those found in the WILEY 09 and NIST 14 databases (https://www.sisweb.com/software/ms/wiley.htm).
2.3 High-performance liquid chromatography (HPLC) quantitative analysis of the fermented M. Oleifera leaves extract of polyphenols
The polyphenols were measured according to the protocol described by (40, 41). Using HPLC equipment (Agilent 1100 series, USA) with an auto-sampling injector, solvent degasser, two LC-pump (series 1100), ChemStation software, and a UV/Vis detector (set at 250 nm for phenolic acids and 360 nm for flavonoids), we were able to analyze these chemicals. The particle size was 4.60 mm * 250 mm i.d., five μm, and the column used was an Eclipse C18. Two solvents were used in a gradient mobile phase to extract the phenolic components. Solvent A was methanol, while solvent B was a 1:2 acetic acid and water mixture. During the initial 3 mins of the gradient procedure, the concentration was maintained at 100% B. Following this, 50% eluent A was used for 5 mins. The concentration of A was raised to 80% for the next 2 mins and then decreased to 50% for the next 5 min. The wavelength for detection is 250 nm. The mobile phase was A, acetonitrile, and B, 0.2% (v/v) formic acid in the water, was employed for the flavonoid isolation using an isocratic elution (70:30) method. The separation was carried out with a solvent rate flow of 1 mL per min at 25°C five μL was the volume of every injection.
2.4 Ethical approval
Following the guidelines set out by the National Institutes of Health, the Zagazig University EAURC (Ethics of Animal Use in Research Committee) approved the experimental protocol, approval number ZU-IACUC/2/F/80/2024.
2.5 Fish management condition
A total number of 180 Nile tilapia fingerlings obtained from the Fish Research Center, Zagazig University, were utilized in the current investigation. The fish had a mean weight of 30 ± 3 g. This experiment was performed at Zagazig University's Faculty of Veterinary Medicine in the Fish Diseases and Management Department. The fish were grown up and acclimatized to the lab environment for 14 days. Tilapia was maintained in aquariums made of glass (30 × 40 × 80 × cm) containing 75 L of dechlorinated water. The laboratory photoperiod was at 12 h of light and 12 h of night. Daily at 8:00 am, following the elimination of accumulated fish excreta and cleaning, water was partially substituted for each tank with clean, dechlorinated water. Throughout the study period, the water-related parameters were followed those needed by tilapia for development (42). The water quality kept up at (a temperature of 26.50 ± 0.37 °C, pH of 7.67 ± 0.38, total ammonia nitrogen of 0.11 ± 0.01 mg/L, and dissolved oxygen of 6.80 ± 0.31 mg/L).
2.6 Experimental diets preparation and experimental protocol
Isonitrogenous and isocaloric basal diets were formulated according to the requirements outlined in the Nutrient Requirements of Fish (43). Formulated experimental diets were prepared to meet ' 'fish's nutritional requirements as shown in Table 1. The chemical components and nutritional needs of each basal diet were formulated according to the guidelines (44). The macro-Kjeldahl technique, petroleum ether, and a muffle furnace were employed to determine the crude proteins, crude lipids, and total ash contents of the prepared fish meals utilized in this study. The duration of the experiment spanned 30 days. Throughout the experiment, every day at 9:00 am, 12:00 pm, and 4:00 pm, the fish were given meals that were equal to 3% of their body weight according to the protocol (45). After the fish were weighed, they were randomly divided into five groups for the experiment. With 10 fish in each replication and 3 replicates totaling 30 fish, the experiment was conducted in triplicate. The 1st control set received basal meals devoid of supplements. The 2nd and 3rd sets received a basal meal supplemented with MOLP at 50 g and 100 g/kg diet, respectively, for 30 days. The 4th and 5th sets received basal meals supplemented with MOLF at 50 g and 100 g/kg diet for 30 days. For each diet, ingredients were thoroughly mixed, and then for each kg of diet, 100 mL of water was added. Using a standard kitchen blender, the last formulated paste of each diet was mixed. Then diets were made into pellets with the lab pellet instrument and allowed to dry for 1 day before storage in hygienic, sterile plastic containers at 4°C till usage.
2.7 Sampling
Following the 30-day experimental study, six fishes were selected at random from every set. Then, blood was collected from every group in a sterile manner from the caudal vein in clean and dry tubes containing heparin for evaluation of the phagocytosis assay. Another blood sample was taken in clean tubes without anticoagulant, and then for 15 min, blood was centrifuged at 3,599 × g rpm for sera separations. Then collected serum samples were maintained at−20°C and used for the investigation of the innate immunity responses. Spleen and anterior kidney specimens were dissected from 3 fish/replicates from all experimental tilapia groups on the 5th days post A. hydrophila challenge and immediately kept in TRIzol reagent at −80°C till the molecular investigation. Liver tissue samples were obtained, isolated, rinsed with physiological saline, and subsequently homogenized and centrifuged at 4°C for 15 min at 664 × g. The resulting supernatants were then utilized to estimate oxidative stress-linked markers.
2.8 Phagocytosis assay
Phagocytic activity was done according to the designated routine (46, 47), with particular adjustments. In a sterile plastic tube, after adding one mL of the heat-inactivated C. glabrata to one mL of the calibrated live leukocyte solutions (leukocytes in RPMI 1640 and 5% of collected Tilapia sera). Then the tubes were kept in the incubator humidified with CO2 5% and incubated for 30 min at 27°C. After centrifuging the tubes for 5 min at 2,500 rpm, the supernatants were collected using a Pasteur pipette, leaving a tiny portion used to resuspend the sediment. Leishman's stain was applied after slide smears were made from the deposit and allowed to air dry.
Phagocytic cells in 10 microscopic fields were counted randomly using the oil immersion lens under a light microscope. The number of phagocytic cells engulfing yeast cells and the number of yeast cells inside each phagocytic cell was counted. The following equations were applied to measure the phagocytic activity. Phagocytic percentage (Ph %) = (count of phagocytes engulfing yeast cells/total count of phagocytic cells) × 100. Phagocytic index (PhI) = (total count of engulfed yeast/count of phagocytic cells engulfing yeast cells) ×100.
2.9 Quantification of serum and hepatic biochemical indicators
The colorimetric determination method was used to measure the superoxide dismutase (SOD) enzyme, reduced glutathione (GSH), and malondialdehyde (MDA) using commercial diagnostic colorimetric kits provided by Bio-diagnostic Co. LTD, Egypt, with the following catalog No. SD 25 21, GR 25 11, and MD 25 29, correspondingly.
2.10 A. hydrophila challenge test
At the Fish Diseases and Management Laboratory, Faculty of Veterinary Medicine, Zagazig University, moribund fish were used to cultivate A. hydrophila. Liver and spleen tissue samples were collected under strict sterile conditions. After spreading inoculums onto plates of tryptic soya agar (TSA; Difco, Detroit, MI), plates were maintained at 37°C for 1 day. Next, from each plate, one colony was selected to be cultivated in novel fresh plates of Rimler-Shotts agar media (RS; Difco, Detroit, MI). Following the ' 'manufacturer's instructions, the isolates were classified using the standard examination of their morphological characteristics, biochemical responses, an API 20NE test kit (bioM'erieux, Marcy l′Etoile, France), and the VITEK® C15 automated system for identifying bacteria (BioMerieux Inc., France). Fifteen fish from each set were inoculated through the peritoneum with 0.2 mL of virulent A. hydrophila (24-h-old culture, 1 × 108 CFU) once the 30-day experimental period had ended. The selected dose for A. hydrophila is equivalent to the median lethal dose (LD50) established (48) for the same bacterial species. The LD50 was 2.8 × 108 CFU/mL. A sub-lethal dose was used in the A. hydrophila challenge test. The challenged fish were examined every day for the following 14 days to report any behavior alterations, clinical observations, and mortalities. The mean mortalities in all fish groups were estimated to determine the relative percent survival (RPS) (49). The clinically ill and recently deceased fish were used to re-isolate and confirm A. hydrophila employing biochemical characters, gram stain, and colony characterization. The bacterial “isolates” chemical properties were examined with the VITEK® compact (BioM'erieux).
2.11 qRT-PCR of immunity-linked genes in spleen and anterior kidney
The transcriptional levels of immune-linked genes il1β, TNF-α, il-2, and il-10 were investigated in the spleen and anterior kidney of Nile tilapia. The total RNA was extracted by the use of the QIAamp RNeasy Mini kit (Cat. No. 51304; Qiagen, Hilden, Germany). The levels of RNA were determined at 260 nm using a Spectrostar NanoDrop TM 2000 spectrophotometer (Cat. No. ND-2000; Thermo Fisher, Santa Clara, CA, USA). The quality of the RNA was also assessed using this spectrophotometer. The Stratagene MX3005P RT-PCR equipment (Cat. No. PF1457N; Thermo Fisher, Santa Clara, CA, USA) performed using a single-stage RT-qPCR replication in triplets. This was done using a Quanti Tect SYBR Green RT-PCR Kit (Cat. No. 204243; Qiagen, Hilden, Germany). Melting curve monitoring was used to validate the PCR amplifications. The gene responsible for housekeeping, specifically glyceraldehyde-3-phosphate dehydrogenase (Gapdh), was used as an in-house guide to standardize the levels of regulation for the transcripts. The primer sequences used in RT-qPCR tests are listed in Table 2. The 2ΔΔCT technique was employed to evaluate the proportional mRNA regulation results of the investigated genes (50).
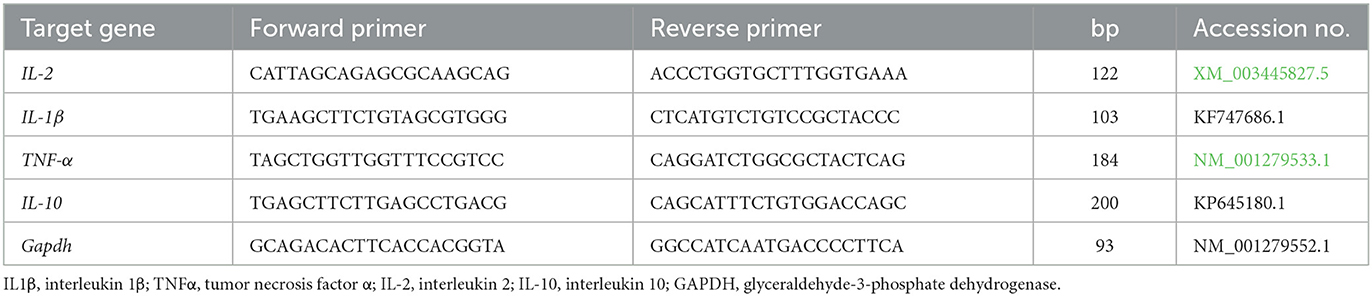
Table 2. Primers sequences, accession number, and product size for the quantitative RT-PCR for the analyzed genes in the spleen and anterior kidney tissues.
2.12 Statistical analysis
A version of IBM SPSS Statistics, version 21 (IBM; Armonk, New York, USA), was used to analyze the data obtained. One-way analysis of variance (ANOVA) was performed to determine the statistical differences within groups. Subsequently, Tukey's multiple range post hoc test was applied for comparisons between pairs. The mean values ± standard error (SE) were used to represent the data for each group. Significance was considered at a threshold of P < 0.05. The clinical signs and autopsy lesions were estimated by semi-quantitative methods: “0 = absence of lesion, 1 = mild, 2 = moderate, and 3 = severe alterations”. The Kaplan-Meier model was performed to determine the specific survival probability. Mortalities were assigned as 1 and survivors as 0, detected every 24 h. Variations between the curves of MOLP or MOLF-exposed groups were tested for significance using the Log-rank Test, with a significance level of P < 0.05. Data visualization was also done using GraphPad Prism version 8 (GraphPad Software, San Diego, CA, USA).
3 Results
3.1 GC-MS investigation of fermented MOLs
The GC-MS evaluation employed the screening of phytochemical contents. The compounds of MOLF, their respective durations of retention, and the proportion of the overall peak area that they constitute are presented in Table 3. Figure 1A displays the GC chromatogram that the GC-MS produced. In all, 33 signals have been determined. The principal components (which Area % is more than 1) of the fermented MOLs were (9E,12E)-9,12-(32.70%); octadecadienoyl chloride hexadecanoic acid (21.39%); methyl 9-octadecenoate (9.28%); 8,11,14-eicosatrienoic acid,(Z,Z,Z)- (3.02%); cyclopropaneoctanoic acid, 2-[[2-[(2-ethylcyclopropyl)methyl] cyclopropyl]methyl]-, methyl ester (2.42%); aspidospermidin-17-OL,1-acetyl-16-methoxy- (1.45%); 2-hexadecen-1-OL,3,7,11,15 tetramethyl-,[R-[R*,R* (E)]]- (1.89%); 9-octadecenoic acid (Z)-,methyl ester (1.41%); panaxjapyne A (1.36%); 9,12,15-octadecatrienoic acid,2-(acetyloxy)-1 [(acetyloxy) methyl] ethyl ester, (Z,Z,Z)- (1.31%); 1-dodecaNol,3,7,11-trimethyl- (1.31%); 2H-oxecin-2-one,3,4,7,8,9,10- hexahydro-4-hydroxy-10-methyl-,[4S-(4R*, 5E,10S*)]-(1.25%); octadecanoic acid,9,10-epoxy-18-(trimethylsiloxy)-, methyl ester, CIS- (1.24%); 9-octadecenoic acid (Z)- (1.10%); Arachidonic amide, N-[5-hydroxy-n-pentyl]- (1.07%); and (9E,12E)-9,12-octadecadienoyl chloride (1.02%).
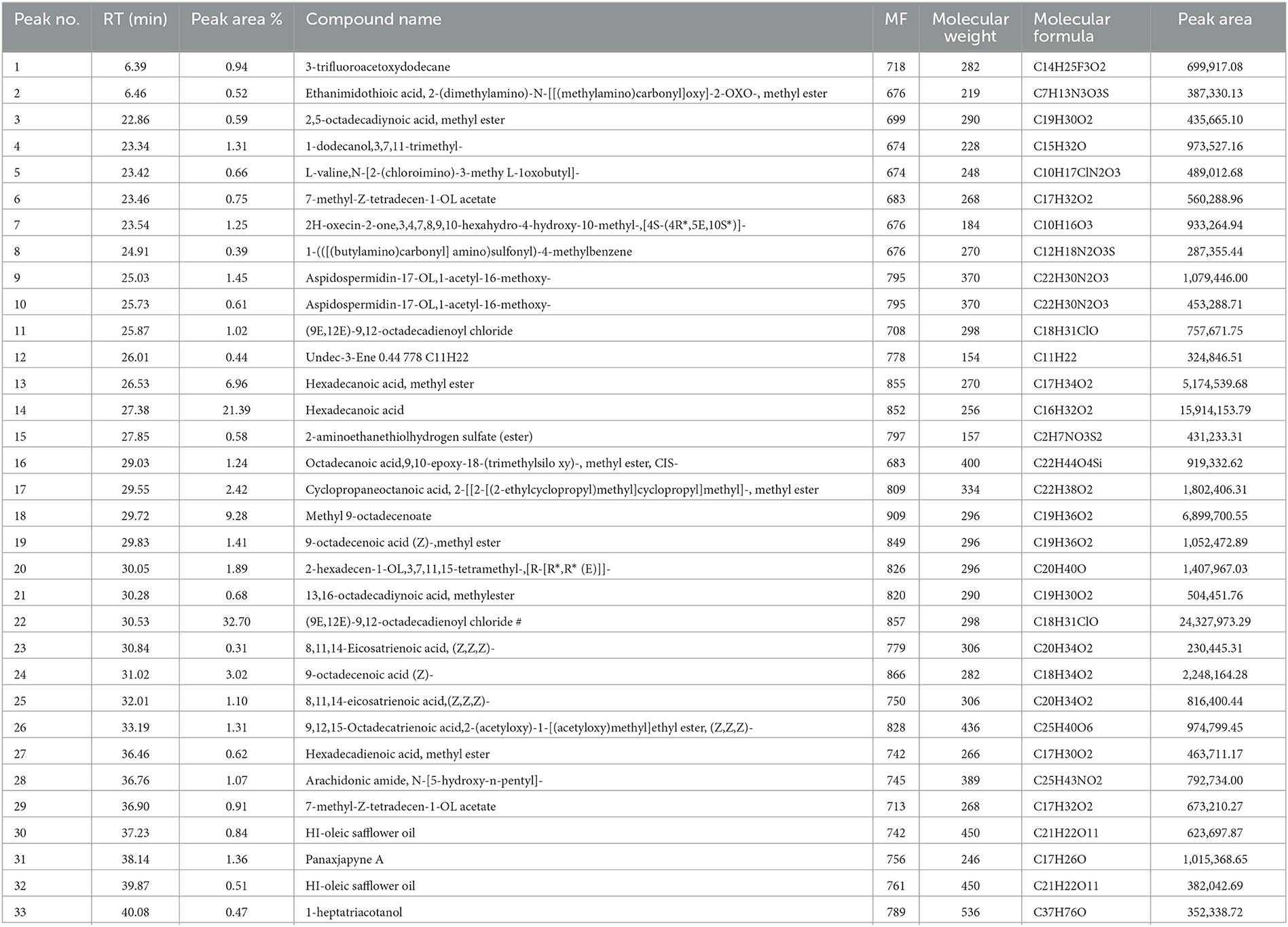
Table 3. List of phytochemical components of fermented Moringa oleifera leaves used in the current study and identified by GC-MS analysis.
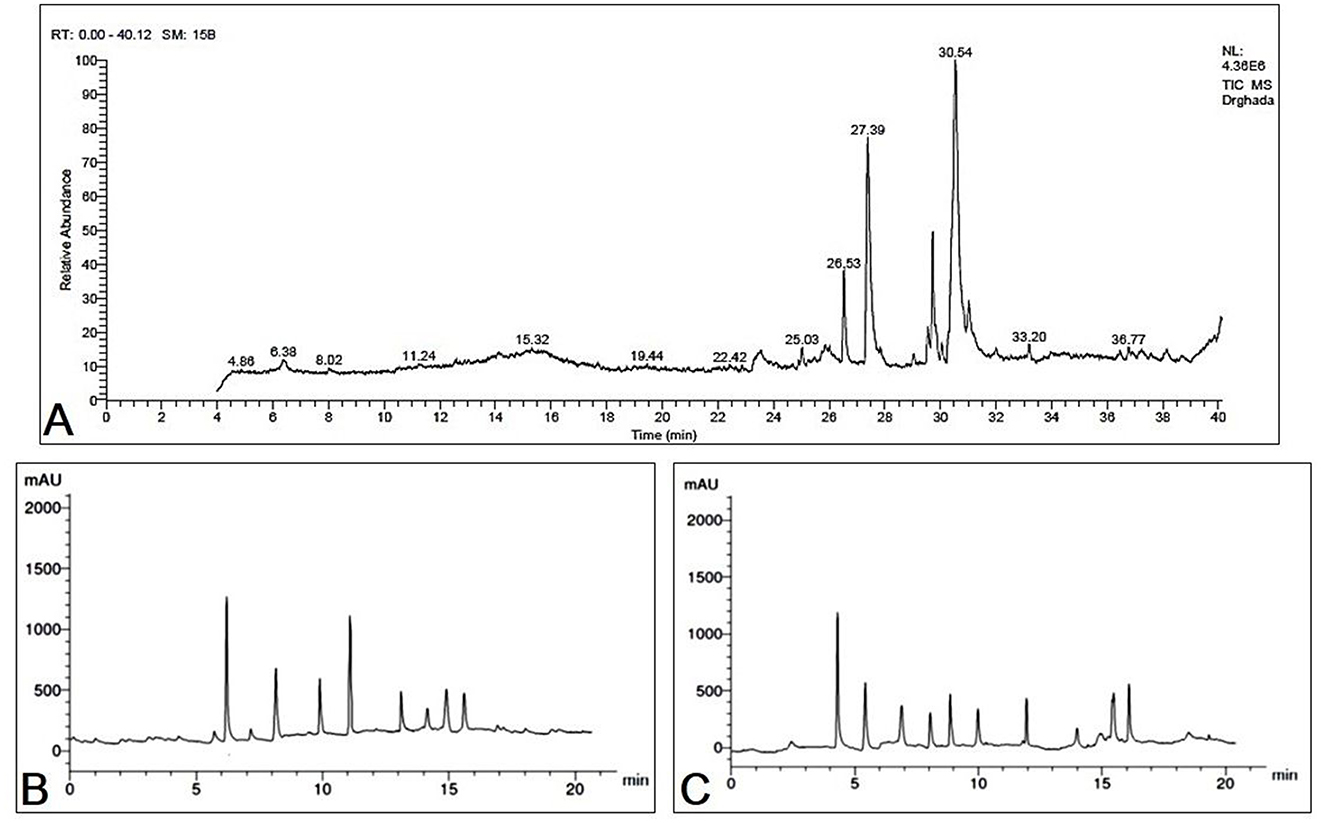
Figure 1. (A) GC-MS chromatogram represents the separated bioactive constituents of fermented Moringa oleifera leaves. HPLC chromatogram represents the separated bioactive constituents of fermented Moringa oleifera leaves ethanolic extract used in the present study. (B) Phenolic compounds, (C) flavonoids.
3.2 HPLC investigation of fermented MOLs phenolic and flavonoid compounds
A whole of 11 components containing phenolic acids and flavonoids were detected and measured by using HPLC in the fermented MOLs ethanolic extract. The main flavonoids and phenolic acids were determined at 425 nm (Table 4, Figures 1B, C). The primary detected phenolic acids were P-Coumaric acid (14.63 μg/mL), caffeic acid (9.42 μg/mL), gallic acid (4.66 μg/mL), ferulic acid (11.63 μg/mL), and catechin (8.62 μg/mL). The significant flavonoids detected by HPLC were naringenin (12.66 μg/mL), rutin (8.52 μg/mL), quercetin (7.45 μg/mL), kaempferol (6.78 μg/mL), luteolin (8.56 μg/mL), and apigenin (7.41 μg/mL).
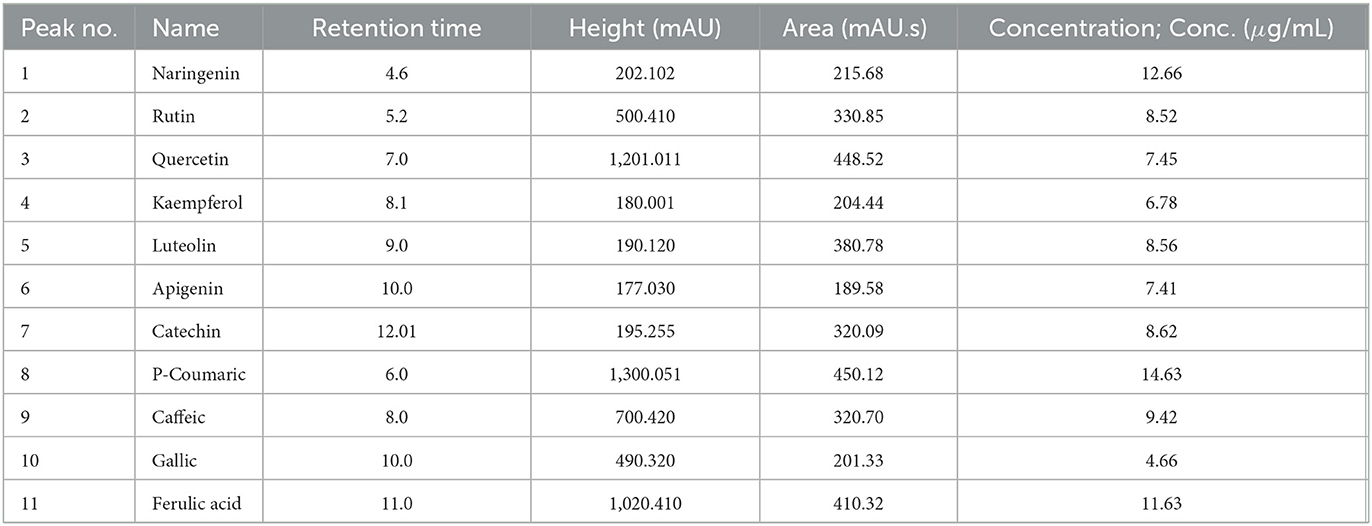
Table 4. List of phytochemical components of fermented Moringa oleifera leaves used in the current study and identified by HPLC analysis.
3.3 Clinical observations and kaplan-meier survival analysis
As shown in Figure 2. The positively infected Nile tilapia showed classic symptoms of A. hydrophila infection between 24 and 120 h post-challenge. Signs included hemorrhagic septicemia, exophthalmia, corneal opacity, fin erosions, ascites, and hemorrhagic congestion of internal organs. Fish moderately exhibited clinical signs in the MOLP-supplemented groups (MOLP at 50 and 100 g/kg diet). At the same time, few observed clinical signs were exhibited by fish in the MOLF-supplemented groups (MOLF at 50 and 100 g/kg diet) compared with the positive C group (challenged with A. hydrophila), which exhibited severe clinical signs and postmortem lesions. Data is shown in Table 5.
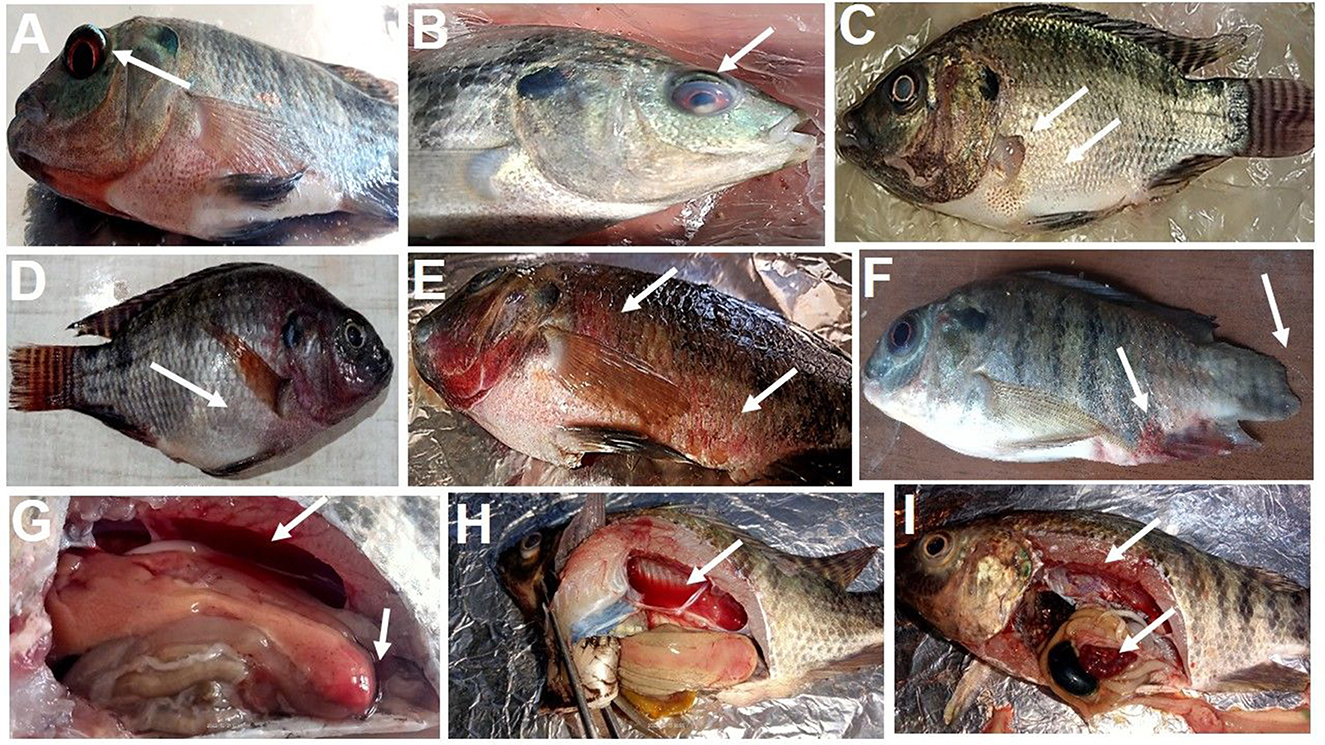
Figure 2. Clinical signs observed in tilapia during experimental infection by A. hydrophila. (A) exophthalmos, (B) corneal opacity, (C) fin rot, abdominal distention, (D) abdominal distention, ascites, (E) hemorrhagic septicemia, (F) fin erosion and hemorrhagic septicemia at the base of the fins and the anus, (G) enlarged yellowish and friable liver with hyperemic intestine filled with yellowish watery fluid, (H) hemorrhagic fluid in the abdominal cavity with enlarged yellowish friable liver, (I) enlarged gall bladder, congestion of all internal organs.
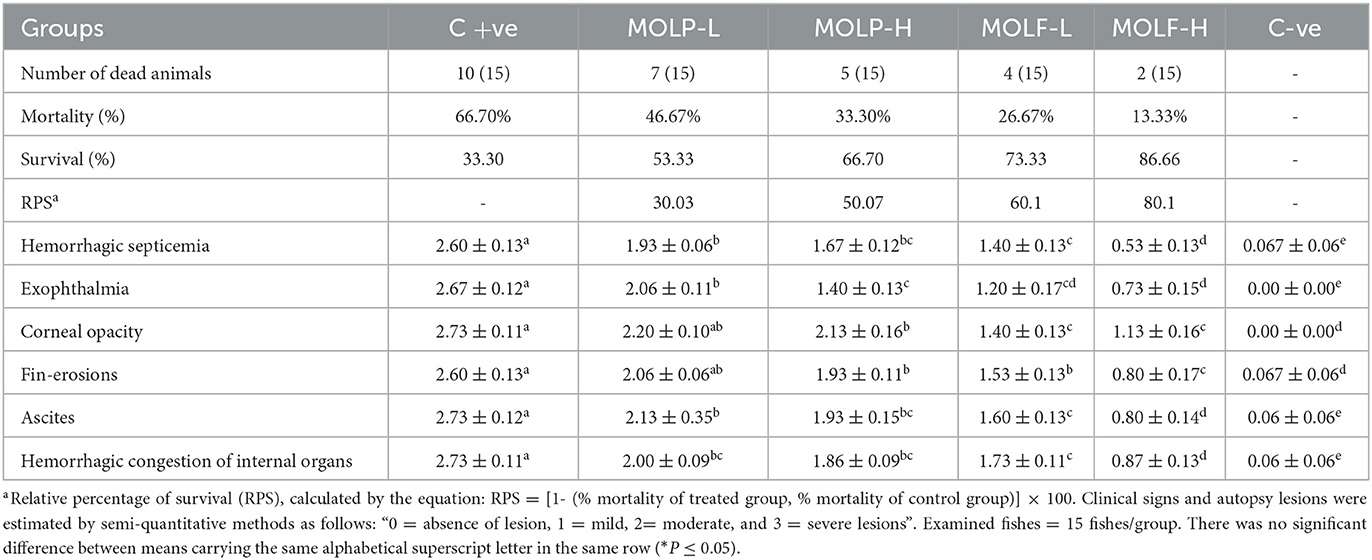
Table 5. RPS of Nile tilapia infected with A. hydrophila and fed a diet supplemented with different doses of dried or fermented Moringa oleifera leaves.
The obtained results of the Kaplan-Meier survival analysis are shown in Figure 3, are displayed that Nile tilapia fed a diet supplemented with MOLF (50 and 100 g/kg diet) displayed considerably reduced mortalities (P < 0.05) than those of the C tilapia when challenged with A. hydrophila. Tilapia in the control set exhibited a mortality rate of 66.7% compared to the (46.67%, 33.3%. 26.67%, and 13.33%) in the MOLP-L, MOLP-H, MOLF-L, and MOLF-H groups respectively. Table 5 shows the RPS of fish challenged with A. hydrophila and fed MOLP or MOLF-supplemented diets at (50 and 100 g/kg diet). There were dose-related effects with RPS of 30.00, 50.00, 60.00, and 80.00% of the groups fed diets supplemented with MOLP-L, MOLP-H, MOLF-L, and MOLF-H (50 and 100 mg/kg diet) when compared to control Nile tilapia.
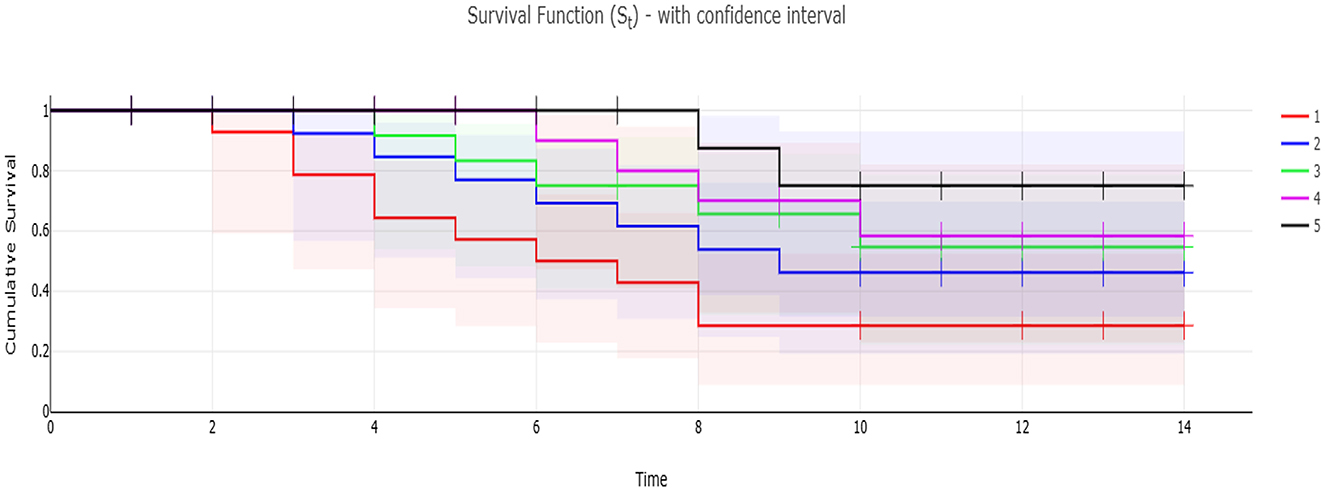
Figure 3. Kaplan-Meier survival analysis of Nile tilapia fed dietary supplementation of −ve control group, MOLP-H, MOLF-L, MOLP-H, and MOLP-L for 30 days and then challenged with A. hydrophila for 14 days. Data are shown as the mean ± SEM (n = 15 fish/group, P < 0.05). Line –Survival rate (St) per time. Confidence interval—added the Confidence interval areas to the line, with confidence level of (1 – α). S.E area—present St ± 1*S.E, the S.E is the area below and above the line. S.E error bars—present St ± 1*S.E, the S.E is presented as error bar. 1, −ve control group; 2, MOLP-L; 3, MOLP-H; 4, MOLF-L; 5, MOLF-H.
3.4 Effect of MOLF or MOLP on immune and phagocytosis assay of Nile tilapia
The obtained results of NO and LYZ serum levels, phagocytic activities, and PhI of fishes fed MOLP or MOLF for a 30-day experimental period are shown in Figure 4. Significantly (P < 0.05), Nile tilapia-fed high-and low-fermented MOLs showed substantially higher NO serum levels (47.53 ± 1.59 and 30.44 ± 1.72) in the MOLF-H and MOLF-L, respectively, when matched with tilapia fed high-and low-dried MOLs (19.91 ± 0.70 and 15.95 ± 1.03), respectively, in the MOLP-H and MOLP-L, which were all markedly (P < 0.05) higher than the C group (10.26 ± 0.06). Nile tilapia fed high-and low-fermented MOLs and high and low-dried MOLs showed significantly (P < 0.05) higher LYZ serum levels (263 ± 4.93, 252.66 ± 3.1, 246 ± 5.01, and 237.83 ± 2.67) in the MOLF-H, MOLF-L, MOLP-H, and MOLP-L groups, correspondingly, when matched with the C tilapia (156 ± 4.64). The variances in the serum LYZ levels within tilapia sets fed MOLP-H, MOLP-L, MOLF-H, and MOLF-L were non-significant.
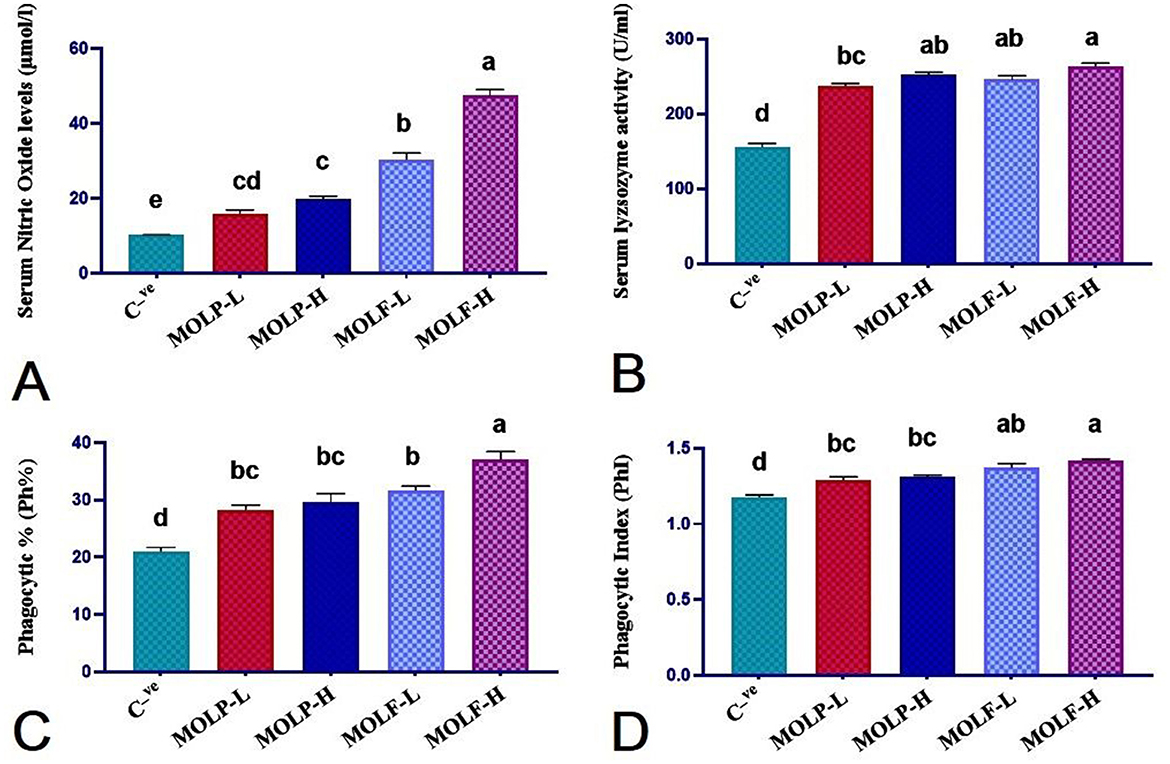
Figure 4. Effect of dietary supplementation of MOLF-H, MOLF-L, MOLP-H, and MOLP-L for 30 days on (A) Serum nitric oxide level (μmol/L), (B) Serum lysozyme activity (unit/mL), (C) Phagocytic percent (%), (D) Phagocytic index of Nile tilapia (O. niloticus). Data are shown as the mean ± SEM. n = 6 samples/group. Bars with different letters are significantly different at (P < 0.05).
Nile tilapia fed high and low fermented MOLs and high and low dried MOLs showed significantly (P < 0.05) higher phagocytic % (37.00 ± 1.46, 31.66 ± 0.76, 29.66 ± 1.47 and 28.33 ± 0.76) in the MOLF-H, MOLF-L, MOLP-H, and MOLP-L groups, respectively when matched with the C tilapia (21.00 ± 0.73). The variances in the phagocytic % within tilapia sets fed MOLP-H, MOLP-L, and MOLF-L were non-significant (P < 0.217). Only the tilapia-fed MOLF-H was significantly (P < 0.0.05) higher in phagocytic % than other supplemented groups.
Nile tilapia fed high and low fermented MOLs and high and low dried MOLs showed significantly (P < 0.05) higher PhI (1.42 ± 0.009, 1.37 ± 0.029, 1.31 ± 0.013, and 1.29 ± 0.023) in the MOLF-H, MOLF-L, MOLP-H, and MOLP-L groups, respectively, when matched with the C tilapia (1.176 ± 0.012). The variances in the PhI between tilapia sets fed MOLP-H, MOLP-L, MOLF-H, and MOLF-L were non-significant.
3.5 Effect of MOLF or MOLP on hepatic oxidative stress biomarkers of Nile tilapia
As presented in Figure 5, a marked (P < 0.05) increment of the GSH level was evident in the liver tissues of Nile tilapia fed high-and low-fermented and high-and low-dried MOLs-supplemented diets (150.30 ± 1.92, 147.36 ± 0.95, 140.70 ± 1.84, and 135.18 ± 1.30) in the MOLF-H, MOLF-L, MOLP-H, and MOLP-L groups, correspondingly, when matched with the C tilapia (115.46 ± 1.59). Supplementation of MOLF markedly (P < 0.05) elevated the GSH content more than the MOLP. Similarly, a marked (P < 0.05) increment of the SOD activities was evident in the liver tissues of Nile tilapia fed high-and low-fermented and high-and low-dried MOLs-supplemented diets (580.66 ± 3.1, 524 ± 3.28, 455.3 ± 6.75, and 440 ± 4.74), respectively, in the MOLF-H, MOLF-L, MOLP-H, and MOLP-L groups when matched with the C tilapia (297.66 ± 3.74). Supplementation of MOLF significantly (P < 0.05) elevated the GSH content and SOD activities more than the MOLP. Meanwhile, a marked decline of MDA concentration (P < 0.05) was evident in the liver tissues of Nile tilapia fed high and low fermented and high and low dried MOLs-supplemented diets (76.00 ± 2.19, 81.00 ± 1.82, 90 ± 3.28, and 96.00 ± 2.19) in the MOLF-H, MOLF-L, MOLP-H, and MOLP-L groups, correspondingly, when matched with the C group (138.33 ± 1.47). Supplementation of MOLF significantly (P < 0.05) reduced the MDA concentration compared to the MOLP.
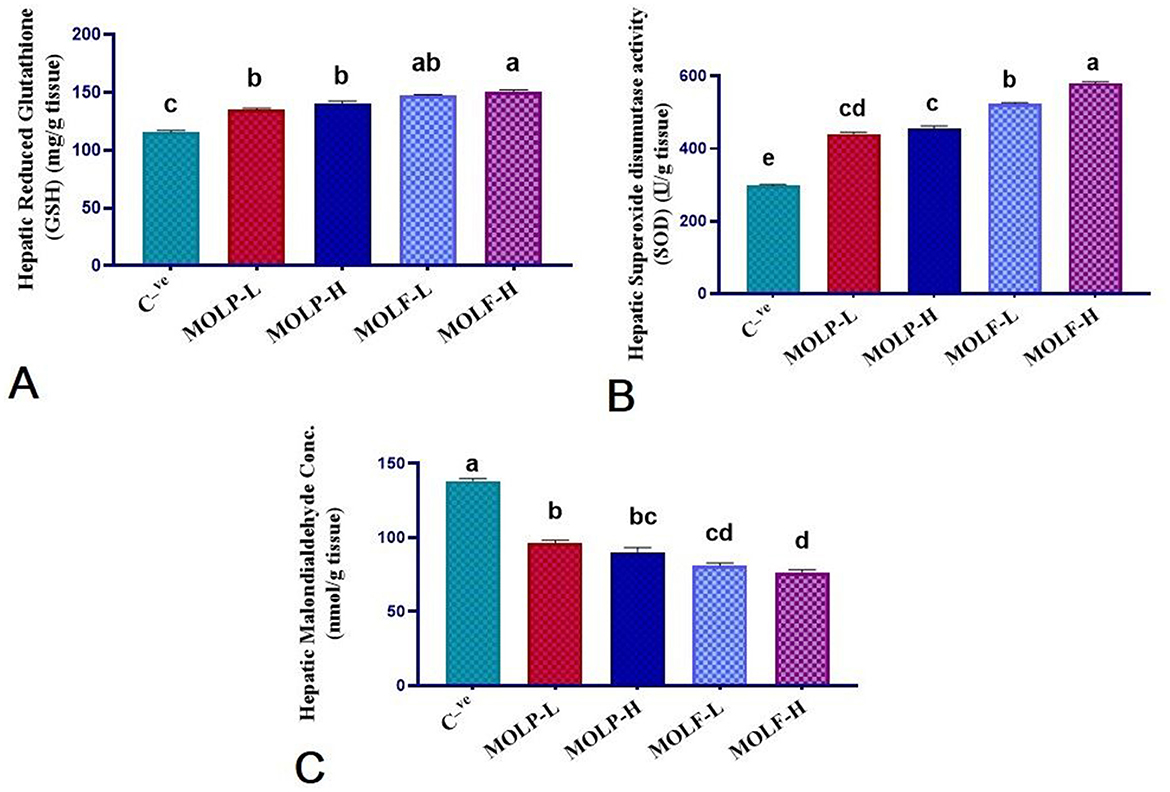
Figure 5. Effect of dietary supplementation of MOLF-H, MOLF-L, MOLP-H, and MOLP-L for 30 days on (A) reduced glutathione (GSH) (mg/g tissue), (B) superoxide dismutase (SOD) activity (u/g tissue), (C) malondialdehyde (MDA) (nmol/g tissue) of Nile tilapia (O. niloticus). Data are shown as the mean ± SEM. n = 6 samples/group. Bars with different letters are significantly different at (P < 0.05).
3.6 Effect of MOLF or MOLP on the mRNA expression of immune-linked genes in the spleen and head kidney of Nile tilapia
As shown in Figures 6, 7, immune functioning-related genes, including ILL-β, TNF-α, and IL-2, were significantly (P < 0.05) upregulated; meanwhile, anti-inflammatory gene IL-10 was significantly (P < 0.05) downregulated in the spleen and head of the kidney of Nile tilapia positive C group (+ve Control infected with A. hydrophila without any diet supplements) compared with the negative C group (non-infected Nile tilapia). On the contrary, a substantial (P < 0.05) correction of the analyzed genes was verified in the Nile tilapia-fed diets supplemented with MOLF and MOLP at high and low doses compared with the positive control group. Of note, notably, Tilapia-fed MOLF-supplemented diets exhibited a marked (P < 0.05) restoration in the mRNA regulation of the analyzed genes in the spleen and anterior kidney compared to the tilapia-fed MOLP-supplemented diets.
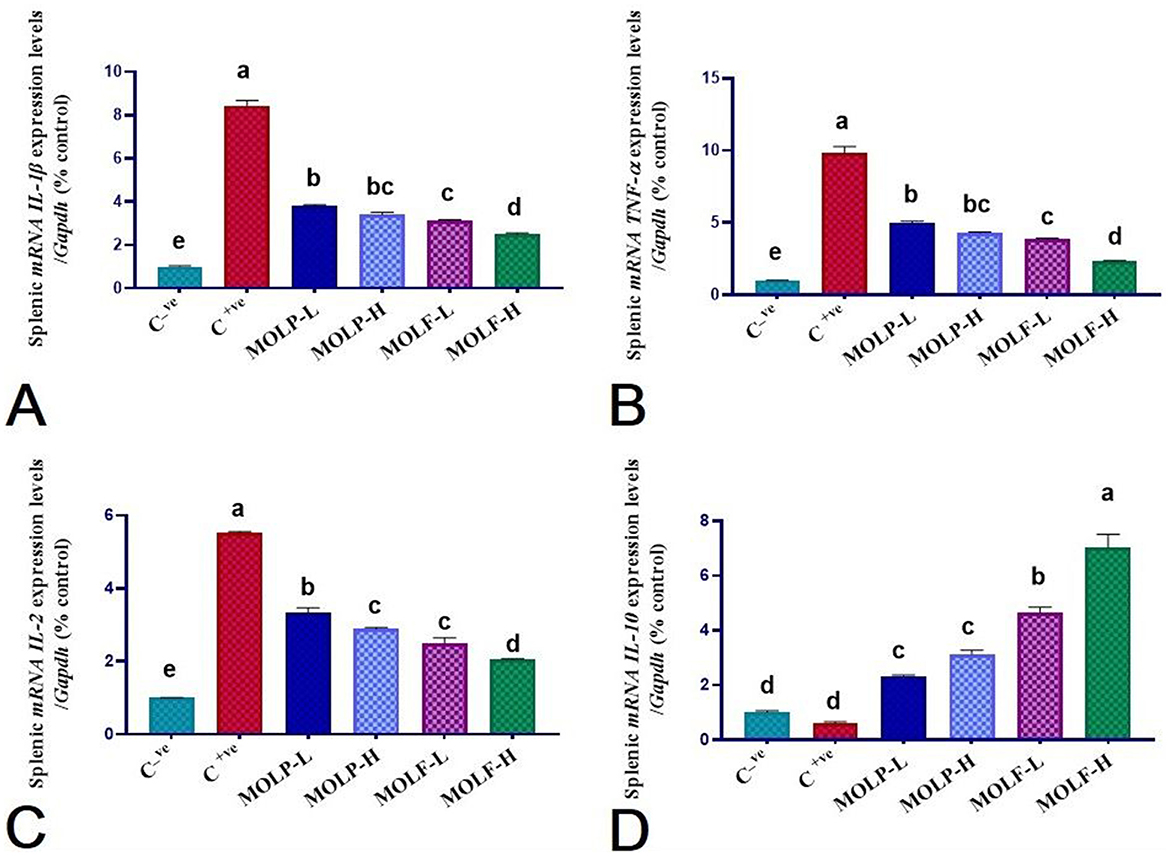
Figure 6. Effect of dietary supplementation of MOLF-H, MOLF-L, MOLP-H, and MOLP-L for 30 days on relative m RNA expression of (A) Spleen IL-1β, (B) Spleen TNF-α, (C) Spleen IL-2 and (D) Spleen IL-10 of Nile tilapia (O. niloticus). Data are shown as the mean ± SEM. n=3 samples/group. Bars with different letters are significantly different at (P < 0.05).
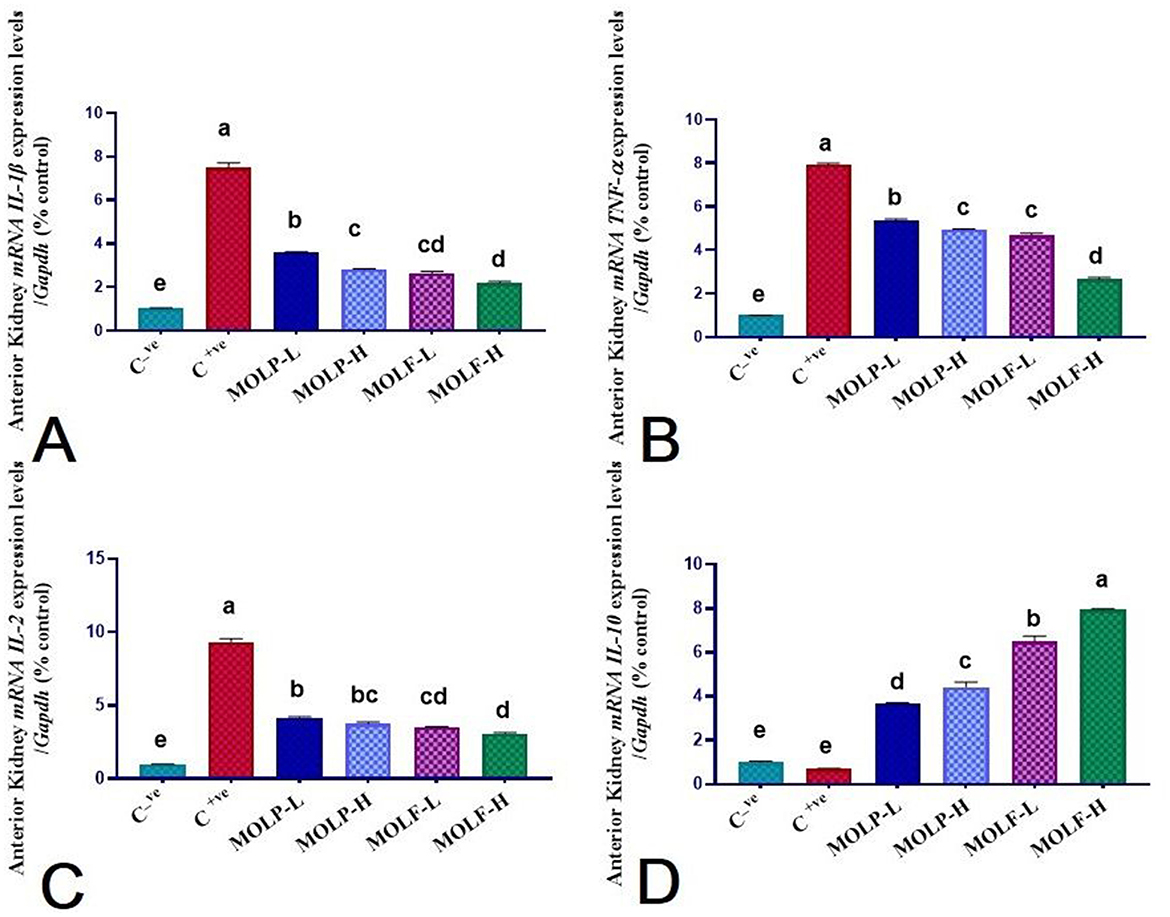
Figure 7. Effect of dietary supplementation of MOLF-H, MOLF-L, MOLP-H, and MOLP-L for 30 days on relative m RNA expression of (A) Anterior kidney IL-1β, (B) Anterior kidney TNF-α, (C) Anterior kidney IL-2, and (D) Anterior kidney IL-10 of Nile tilapia (O. niloticus). Data are shown as the mean±SEM. n = 3 samples/group. Bars with different letters are significantly different at (P < 0.05).
3.7 Effect of MOLF or MOLP supplements on the bacterial load (CFU) in the spleen and liver of Nile tilapia
The data obtained declared that dietary supplements of fermented and dried MOLs to Nile tilapia significantly decreased the total bacterial count CFU of the A. hydrophila in the spleen and liver tissues compared to those of the C tilapia. Data of the A. hydrophila CFU per g tissue among all groups is shown in Figure 8. A significant (P < 0.05) decline of the A. hydrophila CFU was evident in the spleen tissues of Nile tilapia that received high-and low-fermented and high-and low-dried MOLs-supplemented diets (13.88 ± 0.45, 15.18 ± 0.21, 17.13 ± 0.41, and 18.34 ± 0.11) respectively, in the MOLF-H, MOLF-L, MOLP-H, and MOLP-L groups compared with the positive C tilapia (infected with A. hydrophila) (20.19 ± 0.11). Supplementation of MOLF considerably (P < 0.05) declined the A. hydrophila CFU in the spleen more than the MOLP. Similarly, a significant (P < 0.05) decline of the A. hydrophila CFU was evident in the liver tissues of Nile tilapia fed high-and low-fermented and high-and low-dried MOLs-supplemented diets (13.89 ± 0.76, 15.75 ± 0.32, 17.78 ± 0.22, and 18.72 ± 0.58), respectively, in the MOLF-H, MOLF-L, MOLP-H, and MOLP-L groups than the positive C tilapia group (infected with A. hydrophila) (21.24 ± 0.043). Supplementation of MOLF considerably (P < 0.05) declined the A. hydrophila CFU in the liver more than the MOLP.
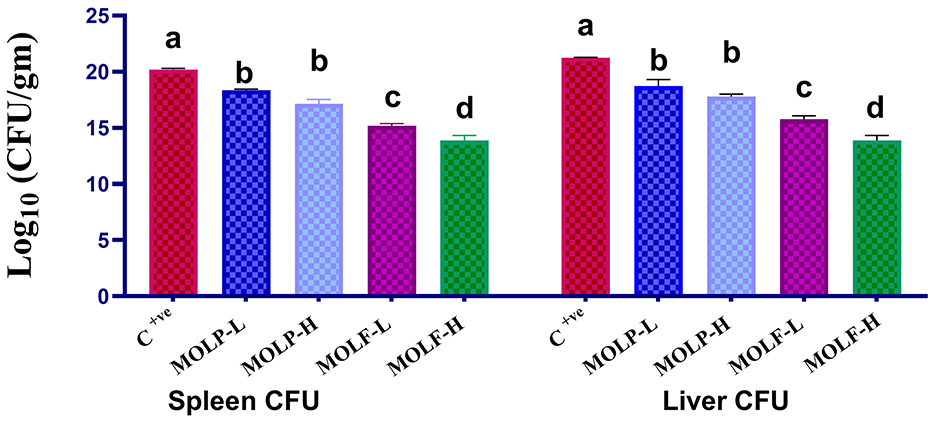
Figure 8. Effect of dietary supplementation of MOLF-H, MOLF-L, MOLP-H, and MOLP-L for 30 days on bacteriological colony count (CFU/g) of the challenged Nile tilapia fish (O. niloticus) spleen and liver. Data are shown as the mean ± SEM. n = 3 samples/group for each organ. Bars with different letters are significantly different at (P < 0.05).
4 Discussion
Using dietary nutrient additives as novel instruments for improving immune functioning and lowering disease incidences is considered safe, non-toxic, economical, and environmentally beneficial. Several nutritional immunostimulants are utilized to increase aquaculture output (51). Immunostimulant feed supplements upregulate immune-linked genes besides enhancing cellular and humoral immune responses in blood and head-kidney (52), as well as the immune reactivity against infections in aquatic species (53). In this study, authors set out to investigate the effect of lactic fermentation in enhancing the nutritious and phytochemical characteristics of MOLs and innate immune activities of O. niloticus, thereby alleviating their tolerance vs. the A. hydrophila challenge.
Our obtained results of the MOLF HPLC analysis revealed a significantly high content of the phenolic and flavonoid components. Current results showed that L.plantarum fermentation enriched the polyphenols and flavonoid synthesis in MOLs through different enzymatic reactions (54). The HPLC analysis results of fermented M. oleifera leaves showed several flavonoids (naringenin, rutin, quercetin, kaempferol, luteolin, apigenin, and catechin) in addition to phenolic compounds (p-coumaric, caffeic, gallic, and ferulic acids) that were significantly amplified by fermentation. In addition, we detected that the fermented MOL has kaempferol, which could not be identified in the raw MOLP. Our results suggested that L. plantarum fermentation improved the biological properties of MOL by increasing phytochemical production. Many studies reported that catechin, ellagic, kaempferol, and quercetin may overcome inflammatory reactions (55, 56). Catechin displayed an essential anti-inflammatory role via regulating the ferroptosis pathway (57). Moreover, kaempferol, quercetin, and ellagic notably decreased inflammation using reduced expression of proinflammatory cytokines and production of NO (58, 59). Therefore, its higher polyphenol concentration may amplify MOLF's anti-inflammatory benefits. One possible use for L. plantarum-fermented MOLs is as an addition to feed. In comparison to MOLP, we propose that MOLF is more active.
Regarding the effect of fermented MOLs, the current study results exhibited that high and low levels of MOLF significantly enhanced NO and LYZ and the phagocytic efficacy and PhI of Nile tilapia. The obtained data are in agreement with (20, 60). MOLP at a level of 15% was more effective than 10% and 5% in enhancing serum LYZ activity in guppy fish (20). Similarly, incorporating MOLP into the diet of Nile tilapia at 5% levels was more effective than at 1.5% levels (13). The phenolic compounds detected in MOLs, including quercetin, kaempferol, flavonoids, and anthocyanins, are linked to enhanced NO levels (61). Furthermore, the phytochemical content of Moringa leaves, such as carotenoids, minerals, vitamins, moringinine, alkaloids, flavonoids, sterols, phytoestrogens, and caffeoylquinic acids, may contribute to these observed effects (38, 62).
Oxidative stress occurs when pro-oxidant and antioxidant levels are imbalanced. The resultant ROS can induce oxidative damage in macromolecules, including proteins, nucleic acids, and lipids. The present study revealed that GSH, SOD, and MDA were altered as biomarkers of oxidative damage. MOLs significantly increased GSH content and SOD activities but lessened MDA concentrations in the liver tissue of Nile tilapia-fed fermented MOLs more significantly than dried MOLs powder. These results are aligned with the previous report (60, 63). MOL's relative abundance of polyphenols (phenyl propanoids, phenolic acids, favonoids, and tannins) directly enhances the antioxidant response of O. niloticus by acting as hydrogen donors that stabilize free radicals generated by cells through electron donation or acceptance (64). The potent antioxidant activity of Moringa species is attributed to their abundant phenolic content, which functions as an antioxidant molecule by stabilizing free radicals produced within a cell by either receiving or giving electrons (65). The findings of the current study may be attributed to the presence of vitamin C, carotenoids, tocopherols, flavonoids, and other phenolic substances in M. oleifera (66). The presence of glucosinolates, which carry a benzyl-glycoside and undergo enzymatic hydrolysis to isothiocyanates, thiocyanates, or nitriles, further boosts the antioxidant activity in MOLs (67, 68).
Feeding Megalobrama amblycephala juveniles with 2.2% and 4.4% of FMOL for 8 weeks enhanced antioxidant activities in the liver and downregulated the transcriptional levels of inflammatory response-linked genes, including NF-kβ and TLR-4 thus enhancing hepatic anti-inflammatory response (63). Leaves of M. oleifera possess high contents of flavonoids and phenolic compounds, including quercetin, as well as high content of ascorbic acid and vitamin A. Dietary supplements with M. oleifera improved the antioxidant potentials of aquatic animals, including Nile tilapia (13, 69). Similarly, MOLF significantly increased the GSH content in the liver of tilapia fed on MOLF-H and MOLF-L. GSH scavenges ROS to relieve oxidative stress directly (70). The data obtained in this study revealed that MOLF enhanced the antioxidant capacity in the liver of O. niloticus, which was more significant in MOLF dietary supplements than in raw MOLP.
The immunological response of Nile tilapia to Aeromonas infections has been previously studied using several traditional immune indicators, such as leukocyte counts, red blood cell counts, LYZ (71), and immunoglobulin M (3). Nevertheless, the molecular mechanisms that mediate the early innate immune response remain largely unknown. Gene expression analysis is useful for examining host-pathogen interactions and locating crucial cellular functions during infection.
This knowledge can facilitate the creation of efficient control methods for bacterial infections in tilapia farming. The study employed a local strain of A. hydrophila to infect Nile tilapia, and within the first 120 h after the challenge, the proportional regulations of specific genes (IL-1β, TNF-α, IL-2, and IL-10) involved in early innate immunity reactions in the spleen and anterior kidney were examined. These genes could determine the critical elements required to successfully combat A. hydrophila infection, a leading cause of disease-related mortality outbreaks on Egyptian Tilapia farms, by describing and learning more about the complex dynamics of host-pathogen interactions during the early stages of infection. The outcomes of the current protocol exhibited that supplementation of MOLF meaningfully upregulated the IL-1β, TNF-α, and IL-2 and downregulated the IL-10 transcriptional expression levels in the spleen and anterior kidney of Nile tilapia post A. hydrophila infection. Parallel results were exhibited where the INF-γ, IL-10, and TNF-α genes were significantly upregulated in Nile tilapia spleen supplemented with MOLs (72). In contrast, the IL-1β gene regulation was markedly downregulated in the tilapia that received MOLF-and MOLP-supplemented diets.
The key factors for inflammatory responses are macrophages (73). Macrophages produce proinflammatory and inflammatory intermediaries, including IL-1β, TNF-α, IL-6, NO, iNOS, and ROS, prompting cellular injury (37). In the present study, MOLF significantly downregulated IL-1β, TNF-α, and IL-2 expression in Nile tilapia” 'spleen and anterior kidney compared with the positively infected fish groups. Fermentation could increase anti-inflammatory potentials by transforming the phenolic combinations (36). MOLF showed high contents of catechin and quercetin, which have anti-inflammatory capabilities via modulation of IL-1β, TNF-α, and NO (74). So, such components may strengthen the anti-inflammatory effects of MOLF. Inflammation is associated with high cellular ROS levels that modulate the proinflammatory cytokines via transcriptional upregulation of inflammation-associated genes (75). Our results showed that A. hydrophila challenge induced oxidative stress, which can be markedly alleviated by MOLF dietary supplementation. Kaempferol is a powerful flavonoid that could decrease ROS production (76). In our study, kaempferol was in the MOLF-fermented L. plantarum. In contrast, the ameliorating effect of MOLF on oxidative stress may be attributed to the ' 'MOLF's kaempferol content.
A key modulator of the adaptive immune response to microbial infections, IL-1β is the initial proinflammatory cytokine produced. When a disease (or damage) occurs, IL-1β sets off a series of events that produce systemic and local reactions that result in inflammation, allowing the host to react to infections quickly. The increase or downregulation of the expression of additional effector molecules mediates the actions of IL-1β. These signaling pathways activate transcription factors, which cause the synthesis of cell adhesion molecules, chemokines, cytokines, and acute-phase proteins (77, 78). Following IP injection challenges with A. hydrophila and Pseudomonas fluorescens, the proportional IL-1β gene regulation outcomes in Nile tilapia in different organs were assessed. It was discovered that the infected fish had considerably higher levels of this gene than the uninfected controls (79).
Contrary to our results, fermented moringa significantly decreased TNF-α and IL-1β after A. hydrophila infection (60). They suggested that this was due to the ability of polysaccharides and flavonoids to regulate macrophage activity, normalizing the proinflammatory cytokine expression levels in gibel carp. Increased MOL levels in diets reduced the expression of TNF-α and IL-1β mRNA in the skin and gills of S. aurata (80). This decrease may be linked to MOLs' inhibitory effects on the phosphorylation of inhibitor kβ protein and mitogen-activated protein kinases (81). Feeding Nile tilapia with 0.5% or 1% methanolic extract of M. oleifera seeds decreased the TNF-α and increased the IL-1β expression in the liver (82).
5 Conclusion
The combined results from the current work advocate that the nutritional supplement of L. plantarum fermented M. oleifera leaves to Nile tilapia feeds could enhance the immune-related responses, antioxidant potential, and mRNA regulation of immunity-related genes, along with enhancing Nile tilapia” 'resistance to A. hydrophila challenge. This might be mediated mainly via the acid fermentation process that increases the amounts of health-enhancing active components in leaves of M. oleifera, decreases toxicological antinutritional factors, and improves the antioxidant status. Notably, according to this study, fermented MOLF exhibited more significant potential for health benefits in the fight against infections than the raw MOLP, and concurrent supplementation of fermented M. oleifera leaves markedly exhibited anti-inflammatory potentials against the detrimental impacts of A. hydrophila infection of Nile tilapia. Further study of the immunostimulants effects of MOLF against immune-toxic chemicals in aquaculture as well as their neuroprotective potentials in long term studies are recommended.
Data availability statement
The original contributions presented in the study are included in the article/supplementary material, further inquiries can be directed to the corresponding authors.
Ethics statement
Following the guidelines set out by the National Institutes of Health, the Zagazig University EAURC (Ethics of Animal Use in Research Committee) has approved the experimental protocol, approval number ZU-IACUC/2/F/80/2024. The study was conducted in accordance with the local legislation and institutional requirements.
Author contributions
GA: Conceptualization, Data curation, Formal analysis, Investigation, Methodology, Resources, Software, Writing – original draft, Writing – review & editing. E-SE-N: Conceptualization, Investigation, Methodology, Resources, Supervision, Software, Validation, Visualization, Writing – review & editing. HA: Conceptualization, Data curation, Formal analysis, Investigation, Methodology, Resources, Supervision, Validation, Visualization, Writing – original draft. EA-Z: Conceptualization, Data curation, Formal analysis, Investigation, Methodology, Resources, Software, Validation, Visualization, Writing – original draft. IR: Conceptualization, Data curation, Formal analysis, Funding acquisition, Project administration, Validation, Writing – review & editing. FZ: Conceptualization, Data curation, Funding acquisition, Investigation, Project administration, Resources, Software, Validation, Writing – review & editing. GE: Conceptualization, Investigation, Methodology, Software, Supervision, Validation, Writing – review & editing.
Funding
The author(s) declare that financial support was received for the research and/or publication of this article. This work was supported by the Slovak grants VEGA no. 1/0162/23 and KEGA no. 011UVLF-4/2024.
Conflict of interest
The authors declare that the research was conducted in the absence of any commercial or financial relationships that could be construed as a potential conflict of interest.
Generative AI statement
The author(s) declare that no Gen AI was used in the creation of this manuscript.
Publisher's note
All claims expressed in this article are solely those of the authors and do not necessarily represent those of their affiliated organizations, or those of the publisher, the editors and the reviewers. Any product that may be evaluated in this article, or claim that may be made by its manufacturer, is not guaranteed or endorsed by the publisher.
References
1. Khan SR, Iqbal R, Hussain R, Ali M, Khalid M, Nazish N, et al. Broccoli partially lowers oxidative stress, histopathological lesions and enhances antioxidant profile of mono sex tilapia exposed to zinc oxide nanoparticles. Pakist Vet J. (2024) 44:2. doi: 10.29261/pakvetj/2024.159
2. Alsulami MN, El-Saadony MT. The enhancing effect of bacterial zinc nanoparticles on performance, immune response, and microbial load of Nile tilapia (Oreochromis niloticus) by reducing the infection by trichodina heterodentata. Pakist Vet J. (2024) 44:3. doi: 10.29261/pakvetj/2024.243
3. El-Magd MA, El-Said KS, El-Semlawy AA, Tanekhy M, Afifi M, Mohamed TM. Association of MHC IIA polymorphisms with disease resistance in Aeromonas hydrophila-challenged Nile tilapia. Dev Comp Immunol. (2019) 96:126–34. doi: 10.1016/j.dci.2019.03.002
4. USDA. U.S. Department of Agriculture Foreign Agricultural Service. Egypt: An Overview of the Aquaculture Industry in Egypt. (2022). Available online at: https://www.fas.usda.gov/
5. Aboyadak I, Ali N, Goda A, Aboelgalagel W, Salam A. Molecular detection of Aeromonas hydrophila as the main cause of outbreak in tilapia farms in Egypt. J Aquac Mar Biol. (2015) 2:2–5. doi: 10.15406/jamb.2015.02.00045
6. Beaz-Hidalgo R, Figueras M. Aeromonas Spp. whole genomes and virulence factors implicated in fish disease. J Fish Dis. (2013) 36:371–88. doi: 10.1111/jfd.12025
7. Ekor M. The growing use of herbal medicines: issues relating to adverse reactions and challenges in monitoring safety. Front Pharmacol. (2014) 4:177. doi: 10.3389/fphar.2013.00177
8. Dantas DL, da Costa Alves M, Dantas GMS, Campos ARN, de Santana RAC, Soares JKB, et al. Supplementation with Moringa oleifera lam leaf and seed flour during the pregnancy and lactation period of wistar rats: maternal evaluation of initial and adult neurobehavioral development of the rat progeny. J Ethnopharmacol. (2024) 325:117904. doi: 10.1016/j.jep.2024.117904
9. Saleemi MK, Raza A, Khatoon A, Zubair M, Xu Y, Murtaza B, et al. Toxic effects of aflatoxin B1 on hematobiochemical and histopathological parameters of juvenile white leghorn male birds and their amelioration with vitamin E and Moringa oleifera. Pakistan Vet J. (2023) 43:3. doi: 10.29261/pakvetj/2023.053
10. Yuniati N, Kusumiyati K, Mubarok S, Nurhadi B. The role of moringa leaf extract as a plant biostimulant in improving the quality of agricultural products. Plants. (2022) 11:2186. doi: 10.3390/plants11172186
11. Kaleo IV, Gao Q, Liu B, Sun C, Zhou Q, Zhang H, et al. Effects of Moringa oleifera leaf extract on growth performance, physiological and immune response, and related immune gene expression of macrobrachium rosenbergii with vibrio anguillarum and ammonia stress. Fish Shellfish Immunol. (2019) 89:603–13. doi: 10.1016/j.fsi.2019.03.039
12. Akbary P, Gholamhosseini A, Ali M, Jahanbakhshi A, Tavabe KR, Kuchaksaraei BS, et al. Dietary administration of Moringa oleifera extract enhances growth, fatty acid composition, antioxidant activity and resistance of shrimp litopenaeus vannamei against photobacterium damselae. Agricult Nat Res. (2021) 55:161–70. doi: 10.34044/j.anres.2021.55.2.01
13. Abd El-Gawad EA, El Asely AM, Soror EI, Abbass AA, Austin B. Effect of dietary Moringa oleifera leaf on the immune response and control of Aeromonas hydrophila infection in Nile tilapia (Oreochromis niloticus) fry. Aquacult Int. (2020) 28:389–402. doi: 10.1007/s10499-019-00469-0
14. Labh SN. Expression of Immune Genes and Stress Enzyme Profiles of Rainbow Trout (Oncorhynchus mykiss) fed Moringa oleifera Leaf Meal (MLM). New Delhi: Global environment & Social Association (2020). Available online at: https://www.gesa.org.in/journals.php
15. Faheem M, Khaliq S, Mustafa N, Rani S, Lone KP. Dietary Moringa oleferia leaf meal induce growth, innate immunity and cytokine expression in grass carp, ctenopharyngodon idella. Aquacult Nutr. (2020) 26:1164–72. doi: 10.1111/anu.13073
16. Khalil F, Korni FM. Evaluation of Moringa oleifera leaves and their aqueous extract in improving growth, immunity and mitigating effect of stress on common carp (Cyprinus carpio) Fingerlings. Aquatic Sci Eng. (2017) 32:170–7. doi: 10.18864/TJAS201715
17. Abdel-Latif HM, Abdel-Daim MM, Shukry M, Nowosad J, Kucharczyk D. Benefits and applications of Moringa oleifera as a plant protein source in aquafeed: a review. Aquaculture. (2022) 547:737369. doi: 10.1016/j.aquaculture.2021.737369
18. Elgendy MY, Awad ES, Darwish DA, Ibrahim TB, Soliman WS, Kenawy AM, et al. Investigations on the influence of Moringa oleifera on the growth, haematology, immunity and disease resistance in Oreochromis niloticus with special reference to the analysis of antioxidant activities by page electrophoresis. Aquac Res. (2021) 52:4983–95. doi: 10.1111/are.15370
19. García-Beltrán JM, Mansour AT, Alsaqufi AS, Ali HM, Esteban MÁ. Effects of aqueous and ethanolic leaf extracts from drumstick tree (Moringa oleifera) on gilthead seabream (Sparus Aurata L) leucocytes, and their cytotoxic, antitumor, bactericidal and antioxidant activities. Fish Shellfish Immunol. (2020) 106:44–55. doi: 10.1016/j.fsi.2020.06.054
20. Helmiati S, Isnansetyo A. The replacement of fish meal with fermented moringa leaves meal and its effect on the immune response of red tilapia (Oreochromis Sp.). In: IOP Conference Series: Earth and Environmental Science. Bristol: IOP Publishing (2021).
21. Noubissi PA, Njilifac Q, Tagne MAF, Fondjo AF, Nguepi MSD, Ngakou JM, et al. Protective effects of Moringa oleifera against acetic acid-induced colitis in rat: inflammatory mediators' inhibition and preservation the colon morphohistology. Pharmacol Res-Natural Prod. (2024) 3:100038. doi: 10.1016/j.prenap.2024.100038
22. Islam Z, Islam SR, Hossen F, Mahtab-ul-Islam K, Hasan MR, Karim R. Moringa oleifera is a prominent source of nutrients with potential health benefits. Int J Food Sci. (2021) 2021:6627265. doi: 10.1155/2021/6627265
23. Peraza-Juan M, Espinoza-Sánchez EA, Lara-Reyes JA, Méndez-Zamora G, Rascón-Cruz Q, Garcia-Zambrano EA, et al. Characterization of seed storage protein of Moringa oleifera and analyses of their bioactive peptide's nutraceutical properties. Acta Scientiarum Polonorum Technologia Alimentaria. (2022) 21:311–20. doi: 10.17306/J.AFS.1063
24. Shi H, Yang E, Li Y, Chen X, Zhang J. Effect of solid-state fermentation on nutritional quality of leaf flour of the drumstick tree (Moringa oleifera Lam). Front Bioeng Biotechnol. (2021) 9:626628. doi: 10.3389/fbioe.2021.626628
25. Saucedo-Pompa S, Torres-Castillo J, Castro-López C, Rojas R, Sánchez-Alejo E, Ngangyo-Heya M, et al. Moringa plants: bioactive compounds and promising applications in food products. Food Res Int. (2018) 111:438–50. doi: 10.1016/j.foodres.2018.05.062
26. Bezie A. The effect of different heat treatment on the nutritional value of milk and milk products and shelf-life of milk products. A review. J Dairy Vet Sci. (2019) 11:555822. doi: 10.19080/JDVS.2019.11.555822
27. Perveen S, Akhtar S, Qamar M, Saeed W, Suleman R, Younis M, et al. The effect of Lactiplantibacillus plantarum fermentation and blanching on microbial population, nutrients, anti-nutrients and antioxidant properties of fresh and dried mature Moringa oleifera leaves. J Agricult Food Res. (2024) 18:101366. doi: 10.1016/j.jafr.2024.101366
28. Rakhmanova A, Khan ZA, Shah K. A mini review fermentation and preservation: role of lactic acid bacteria. MOJ Food Process Technol. (2018) 6:414–7. doi: 10.15406/mojfpt.2018.06.00197
29. Park S, Ji Y, Park H, Lee K, Park H, Beck BR, et al. Evaluation of functional properties of lactobacilli isolated from Korean white kimchi. Food Control. (2016) 69:5–12. doi: 10.1016/j.foodcont.2016.04.037
30. Izuddin WI, Loh TC, Samsudin AA, Foo HL. In vitro study of postbiotics from lactobacillus plantarum Rg14 on rumen fermentation and microbial population. Revista Brasileira de Zootecnia. (2018) 47:e20170255. doi: 10.1590/rbz4720170255
31. Mendoza-Avendaño C, Meza-Gordillo R, Ovando-Chacón S, Luján-Hidalgo M, Ruiz-Cabrera M, Grajales-Lagunes A, et al. Evaluation of bioactive and anti-nutritional compounds during soy milk fermentation with lactobacillus plantarum bal-03-ittg and lactobacillus fermentum Bal-21-Ittg. Revista Mexicana de Ingeniería Química. (2019) 18:967–78. doi: 10.24275/uam/izt/dcbi/revmexingquim/2019v18n3/Mendoza
32. Terefe ZK, Omwamba MN, Nduko JM. Effect of solid state fermentation on proximate composition, antinutritional factors and in vitro protein digestibility of maize flour. Food Sci Nutr. (2021) 9:6343–52. doi: 10.1002/fsn3.2599
33. Valdez-González FJ, Gutiérrez-Dorado R, García-Ulloa M, Cuevas-Rodríguez BL, Rodríguez-González H. Effect of fermented, hardened, and dehulled of chickpea (Cicer arietinum) meals in digestibility and antinutrients in diets for tilapia (Oreochromis niloticus). Spanish J Agricult Res. (2018) 16:e0605. doi: 10.5424/sjar/2018161-11830
34. Sharma R, Garg P, Kumar P, Bhatia SK, Kulshrestha S. Microbial fermentation and its role in quality improvement of fermented foods. Fermentation. (2020) 6:106. doi: 10.3390/fermentation6040106
35. Nkhata SG, Ayua E, Kamau EH, Shingiro JB. Fermentation and germination improve nutritional value of cereals and legumes through activation of endogenous enzymes. Food Sci Nutr. (2018) 6:2446–58. doi: 10.1002/fsn3.846
36. Le B, Anh PTN, Yang SH. Enhancement of the anti-inflammatory effect of mustard kimchi on raw 2647 macrophages by the Lactobacillus plantarum fermentation-mediated generation of phenolic compound derivatives. Foods. (2020) 9:181. doi: 10.3390/foods9020181
37. Lee MR, Kim JE, Park JJ, Choi JY, Song BR, Choi YW, et al. Protective role of fermented mulberry leave extract in LPS-induced inflammation and autophagy of Raw264.7 macrophage cells. Mol Med Reports. (2020) 22:4685–95. doi: 10.3892/mmr.2020.11563
38. Thierry NN, Léopold TN, Didier M, Moses FMC. Effect of Pure Culture Fermentation on Biochemical Composition of Moringa oleifera Lam Leaves Powders. (2013). Available online at: https://medcraveonline.com/
39. Abd El-Kareem MS, Rabbih MAEF, Selim ETM. Elsherbiny EAE, El-Khateeb AY. Application of GC/EIMS in combination with semi-empirical calculations for identification and investigation of some volatile components in basil essential oil. Int J Analyt Mass Spectromet Chromatog. (2016) 4:14–25. doi: 10.4236/ijamsc.2016.41002
40. Kuntić V, Pejić N, Ivković B, Vujić Z, Ilić K, Mićić S, et al. Isocratic Rp-Hplc method for rutin determination in solid oral dosage forms. J Pharm Biomed Anal. (2007) 43:718–21. doi: 10.1016/j.jpba.2006.07.019
41. Lin Y-L, Juan I-M, Chen Y-L, Liang Y-C, Lin J-K. Composition of polyphenols in fresh tea leaves and associations of their oxygen-radical-absorbing capacity with antiproliferative actions in fibroblast cells. J Agric Food Chem. (1996) 44:1387–94. doi: 10.1021/jf950652k
42. Boyd CE, Tucker CS. Pond Aquaculture Water Quality Management. Cham: Springer Science & Business Media (2012).
43. NRC NRC. Nutrient Requirements of Fish and Shrimp. Washington, DC: National Academies Press (2011).
44. Horwitz W, Latimer G. AOAC Official Methods of Analysis of Aoac International. Gaithersburg: AOAC International. (2005).
45. Riche M, Haley D, Oetker M, Garbrecht S, Garling D. Effect of feeding frequency on gastric evacuation and the return of appetite in tilapia Oreochromis niloticus (L). Aquaculture. (2004) 234:657–73. doi: 10.1016/j.aquaculture.2003.12.012
46. Lowry V, Farnell M, Ferro P, Swaggerty C, Bahl A, Kogut M. Purified B-glucan as an abiotic feed additive up-regulates the innate immune response in immature chickens against salmonella enterica serovar enteritidis. Int J Food Microbiol. (2005) 98:309–18. doi: 10.1016/j.ijfoodmicro.2004.06.008
47. Sornplang P, Leelavatcharamas V, Soikum C. Heterophil phagocytic activity stimulated by Lactobacillus salivarius L61 and L55 supplementation in broilers with salmonella infection. J Anim Sci. (2015) 28:1657. doi: 10.5713/ajas.15.0359
48. Ashour M, Mabrouk MM, Ayoub HF, El-Feky MM, Zaki SZ, Hoseinifar SH, et al. Effect of dietary seaweed extract supplementation on growth, feed utilization, hematological indices, and non-specific immunity of Nile tilapia, Oreochromis niloticus challenged with Aeromonas hydrophila. J Appl Phycol. (2020) 32:3467–79. doi: 10.1007/s10811-020-02178-1
50. Livak KJ, Schmittgen TD. Analysis of relative gene expression data using real-time quantitative PCR and the 2– Δδct method. Methods. (2001) 25:402–8. doi: 10.1006/meth.2001.1262
51. Dawood MA. Nutritional immunity of fish intestines: important insights for sustainable aquaculture. Rev Aquacult. (2021) 13:642–63. doi: 10.1111/raq.12492
52. Mandujano-Tinoco EA, Sultan E, Ottolenghi A, Gershoni-Yahalom O, Rosental B. Evolution of cellular immunity effector cells; perspective on cytotoxic and phagocytic cellular lineages. Cells. (2021) 10:1853. doi: 10.3390/cells10081853
53. Sakai M, Hikima J-i, Kono T. Fish cytokines: current research and applications. Fish Sci. (2021) 87:1–9. doi: 10.1007/s12562-020-01476-4
54. Chiu HF, Wang HM, Shen YC, Venkatakrishnan K, Wang CK. Anti-inflammatory properties of fermented pine (Pinus morrisonicola Hay) needle on lipopolysaccharide-induced inflammation in raw 2647 macrophage cells. J Food Biochem. (2019) 43:e12994. doi: 10.1111/jfbc.12994
55. Ahmad S, Aqib AI, Ghafoor M, Shoaib M, ul Haq S, Ataya FS, et al. Drug resistance modulation of dairy mrsa through berberine artesunate and quercetin in combination with B-lactams. Pak Vet J. (2024) 44:510–6.
56. Ramaiyulis R, Mairizal M, Salvia S, Fati N, Malvin T. Effects of dietary catechin uncaria gambir extract on growth performance, carcass characteristics, plasma lipids, antioxidant activity and nutrient digestibility in broiler chickens. Int J Vet Sci. (2023) 12:169–74.
57. Kuang W, Yang J, Liu Z, Zeng J, Xia X, Chen X, et al. Catechin mediates ferroptosis to exert an anti-inflammatory effect on raw 2647 cells. Foods. (2022) 11:1572. doi: 10.3390/foods11111572
58. BenSaad LA, Kim KH, Quah CC, Kim WR, Shahimi M. Anti-inflammatory potential of ellagic acid, gallic acid and punicalagin a&b isolated from punica granatum. BMC Complement Altern Med. (2017) 17:1–10. doi: 10.1186/s12906-017-1555-0
59. Nishikawa M, Kada Y, Kimata M, Sakaki T, Ikushiro S. Comparison of metabolism and biological properties among positional isomers of quercetin glucuronide in Lps-and Rankl-challenged Raw264.7 cells. Bioscience, Biotechnology, and Biochemistry. (2022) 86:1670–9. doi: 10.1093/bbb/zbac150
60. Zhang X, Sun Z, Cai J, Wang J, Wang G, Zhu Z. et al. Effects of dietary fish meal replacement by fermented moringa (Moringa oleifera Lam) leaves on growth performance, nonspecific immunity and disease resistance against Aeromonas hydrophila in juvenile gibel carp (Carassius auratus gibelio var cas III). Fish Shellfish Immunol. (2020) 102:430–9. doi: 10.1016/j.fsi.2020.04.051
61. Valavanidis A, Vlahogianni T, Dassenakis M, Scoullos M. Molecular biomarkers of oxidative stress in aquatic organisms in relation to toxic environmental pollutants. Ecotoxicol Environ Saf. (2006) 64:178–89. doi: 10.1016/j.ecoenv.2005.03.013
62. Anwar F, Latif S, Ashraf M, Gilani AH. Moringa oleifera: a food plant with multiple medicinal uses. Phytother Res. (2007) 21:17–25. doi: 10.1002/ptr.2023
63. Jiang W, Qian L, Zhao Y, Lin Y, Yang Y, Shen H, et al. The application of moringa oleifera leaf meal and its fermentation products in the diet of megalobrama amblycephala juveniles. Fermentation. (2023) 9:577. doi: 10.3390/fermentation9060577
64. Sreelatha S, Padma P. Antioxidant activity and total phenolic content of Moringa oleifera leaves in two stages of maturity. Plant Foods Human Nutr. (2009) 64:303–11. doi: 10.1007/s11130-009-0141-0
65. Toppo R, Roy BK, Gora RH, Baxla SL, Kumar P. Hepatoprotective activity of Moringa oleifera against cadmium toxicity in rats. Vet World. (2015) 8:537. doi: 10.14202/vetworld.2015.537-540
66. Pakade V, Cukrowska E, Chimuka L. Comparison of antioxidant activity of Moringa oleifera and selected vegetables in South Africa. S Afr J Sci. (2013) 109:1–5. doi: 10.1590/sajs.2013/1154
67. Bennett RN, Mellon FA, Foidl N, Pratt JH, Dupont MS, Perkins L, et al. Profiling glucosinolates and phenolics in vegetative and reproductive tissues of the multi-purpose trees Moringa oleifera L (horseradish tree) and Moringa Stenopetala L. J Agricult Food Chem. (2003) 51:3546–53. doi: 10.1021/jf0211480
68. Wach A, Pyrzyńska K, Biesaga M. Quercetin content in some food and herbal samples. Food Chem. (2007) 100:699–704. doi: 10.1016/j.foodchem.2005.10.028
69. Monir W, Abdel-Rahman MA, Hassan SE-D, Awad SM. Pomegranate peel and moringa-based diets enhanced biochemical and immune parameters of Nile tilapia against bacterial infection by Aeromonas hydrophila. Microb Pathog. (2020) 145:104202. doi: 10.1016/j.micpath.2020.104202
70. Abou-Zeid SM, Ahmed AI, Awad A, Mohammed WA, Metwally MM, Almeer R, et al. Moringa oleifera ethanolic extract attenuates tilmicosin-induced renal damage in male rats via suppression of oxidative stress, inflammatory injury, and intermediate filament proteins mRNA expression. Biomed Pharmacother. (2021) 133:110997. doi: 10.1016/j.biopha.2020.110997
71. Hardi E, Saptiani G, Kusuma I, Suwinarti W, Nugroho R. Evaluation of traditional plant extracts for innate immune mechanisms and disease resistance against fish bacterial Aeromonas hydrophila and Pseudomonas Sp. In: IOP Conference Series: Earth and Environmental Science. Bristol: IOP Publishing (2018).
72. El-Son MA, Hendam BM, Nofal MI, Abdel-Latif HM. Effects of Moringa oleifera-based diets on growth, immunological responses, liver antioxidant biomarkers and expression of immune-related genes in Nile tilapia (Oreochromis niloticus) raised in hapa-in-pond system. Aquac Res. (2022) 53:4338–52. doi: 10.1111/are.15932
73. Saqib U, Sarkar S, Suk K, Mohammad O, Baig MS, Savai R. Phytochemicals as modulators of M1-M2 macrophages in inflammation. Oncotarget. (2018) 9:17937. doi: 10.18632/oncotarget.24788
74. Yamaguchi M, Levy RM. The combination of catechin, baicalin and B-caryophyllene potentially suppresses the production of inflammatory cytokines in mouse macrophages in vitro. Exp Ther Med. (2019) 17:4312–8. doi: 10.3892/etm.2019.7452
75. Park J, Min J-S, Kim B, Chae U-B, Yun JW, Choi M-S, et al. Mitochondrial ROS govern the LPS-induced pro-inflammatory response in microglia cells by regulating Mapk and Nf-Kb Pathways. Neurosci Lett. (2015) 584:191–6. doi: 10.1016/j.neulet.2014.10.016
76. Huang YB, Lin MW, Chao Y, Huang CT, Tsai YH, Wu PC. Anti-oxidant activity and attenuation of bladder hyperactivity by the flavonoid compound Kaempferol. Int J Urol. (2014) 21:94–8. doi: 10.1111/iju.12179
77. Dan X-M, Zhang T-W, Li Y-W, Li A-X. Immune responses and immune-related gene expression profile in orange-spotted grouper after immunization with cryptocaryon irritans vaccine. Fish Shellfish Immunol. (2013) 34:885–91. doi: 10.1016/j.fsi.2012.12.011
78. Dinarello CA. Overview of the Il-1 family in innate inflammation and acquired immunity. Immunol Rev. (2018) 281:8–27. doi: 10.1111/imr.12621
79. Hal AM, Manal I. Gene Expression and histopathological changes of Nile tilapia (Oreochromis niloticus) infected with Aeromonas hydrophila and pseudomonas fluorescens. Aquaculture. (2020) 526:735392. doi: 10.1016/j.aquaculture.2020.735392
80. Mansour AT, Espinosa C, García-Beltrán JM, Miao L, Ceballos Francisco DC, Alsaqufi AS, et al. Dietary supplementation of drumstick tree, Moringa oleifera, improves mucosal immune response in skin and gills of seabream, sparus aurata, and attenuates the effect of hydrogen peroxide exposure. Fish Physiol Biochem. (2020) 46:981–96. doi: 10.1007/s10695-020-00763-2
81. Praengam K, Muangnoi C, Dawilai S, Awatchanawong M, Tuntipopipat S. Digested Moringa oleifera boiled pod exhibits anti-inflammatory activity in caco-2 cells. J Herbs Spices Med Plants. (2015) 21:148–60. doi: 10.1080/10496475.2014.932880
Keywords: Lactobacillus plantarum-fermentation, Moringa oleifera, Nile tilapia, innate immunity, phagocytosis, immune-related genes, HPLC phytochemical analysis
Citation: Abdelkader GS, El-Naenaeey E-S, Abdallah HM, Abu-Zeid EH, Rehan IF, Zigo F and Elmowalid GA (2025) Immune enhancement and disease resistance against Aeromonas hydrophila infection by dietary Lactobacillus plantarum-fermented Moringa oleifera leaves in Oreochromis niloticus. Front. Vet. Sci. 12:1557671. doi: 10.3389/fvets.2025.1557671
Received: 09 January 2025; Accepted: 17 March 2025;
Published: 25 April 2025.
Edited by:
Ana Couto, University of Porto, PortugalReviewed by:
Aisha Khatoon, University of Agriculture, Faisalabad, PakistanSeyed Pezhman Hosseini Shekarabi, Iranian Fisheries Science Research Institute (IFSRI), Iran
Copyright © 2025 Abdelkader, El-Naenaeey, Abdallah, Abu-Zeid, Rehan, Zigo and Elmowalid. This is an open-access article distributed under the terms of the Creative Commons Attribution License (CC BY). The use, distribution or reproduction in other forums is permitted, provided the original author(s) and the copyright owner(s) are credited and that the original publication in this journal is cited, in accordance with accepted academic practice. No use, distribution or reproduction is permitted which does not comply with these terms.
*Correspondence: Ehsan H. Abu-Zeid, ZWhlbHNoYXJrYXd5QHp1LmVkdS5lZw==; Ibrahim F. Rehan, aWJyYWhpbS5yZWhhbkB2ZXQubWVub2ZpYS5lZHUuZWc=; František Zigo, ZnJhbnRpc2VrLnppZ29AdXZsZi5zaw==