- 1College of Animal Science and Technology, Hebei Agricultural University, Baoding, China
- 2College of Food Science and Technology, Hebei Agricultural University, Baoding, China
- 3Hebei Research Institute of Microbiology Co., Ltd., Baoding, China
Zearalenone (ZEA), a prevalent mycotoxin in animal feeds, is known to disrupt normal ovarian development and function due to its estrogenic activity. This study investigates the toxic effects of ZEA on the ovaries of meat rabbits and explores the underlying mechanisms. Ninety healthy 41-day-old Hyla male rabbits were randomly assigned into three groups. The control group received a basal diet, while the experimental groups were fed basal diets supplemented with 300 and 600 μg/kg ZEA, respectively. Each group consisted of 30 replicates, with one rabbit per replicate, and the experimental period lasted 42 days. The results showed that, compared to the control group, the ovarian index was significantly increased in the 600 μg/kg ZEA supplementation group (p < 0.05). In addition, ovarian tissue exhibited pathological changes, including follicular dilatation, thinning of the follicular granulosa, punctate necrosis of granulosa cells, deep stained cytosolic nuclei, and nuclear fragmentation. Compared to the control group, the 600 μg/kg ZEA supplementation group exhibited significantly elevated blood levels of gonadotropin-releasing hormone, luteinizing hormone, estradiol, malondialdehyde (MDA), and interleukin 1β (IL-1β) (p < 0.05). Conversely, total antioxidant power (TAOC) and glutathione peroxidase (GSH-Px) activities were significantly reduced in this group (p < 0.05). The level of MDA in the ovarian tissue of rabbits in the 600 μg/kg ZEA supplementation group was significantly elevated compared to the control group, while the activities of GSH-Px and TAOC were significantly reduced (p < 0.05). Moreover, the expression levels of luteinizing hormone receptor mRNA, heat shock protein 70 mRNA, tumor necrosis factor-α mRNA, and IL-1β mRNA in the ovarian tissue significantly increased, whereas the expression of copper and zinc superoxide dismutase mRNA was significantly decreased compared to the control group (p < 0.05). In conclusion, supplementation with 600 μg/kg ZEA induces oxidative stress and inflammatory responses in the ovaries of meat rabbits by modulating the expression of related genes. These effects disrupt ovarian development, cause pathological changes, and impair the secretion of reproductive hormones. This study is the first to report the toxic effects of ZEA on the ovaries of Hyla rabbits and provides preliminary insights into its underlying mechanisms.
1 Introduction
Zearalenone (ZEA) is a polyketide secondary metabolite primarily produced by Fusarium graminearum (1). It is one of the most prevalent mycotoxins detected in various types of rabbit feeds (2). The heterocyclic phenolic hydroxyl groups in ZEA and its derivatives closely resemble those found in natural estrogens, enabling them to competitively bind to estrogen receptors. This interaction can disrupt the development and function of reproductive organs, resulting in reproductive toxicity (3). According to literature, exposure to ZEA can disrupt the estrous cycle, inhibit the secretion of luteinizing hormone (LH) and estradiol, reduce the number of primary and mature follicles, and ultimately induce ovarian damage in mice (4). Moreover, ZEA exposure has been shown to cause cell cycle arrest in granulosa cells, leading to cellular damage and apoptosis within the ovarian tissue (5). Exposure to ZEA can lower the reproductive ability of female animals by interfering with the neuroendocrine regulatory function of the hypothalamic-pituitary-ovarian axis (HPOA) and inducing abnormal secretion of sex hormones (6). It is a crucial factor contributing to the accelerated atresia of ovarian follicles and the deficiency of luteal function (7, 8). As one of the most harmful mycotoxins in rabbit production, ZEA is not only associated with reproductive toxicity but also exerts organotoxic effects on the liver, kidney, and intestine, in addition to causing immunotoxicity and cytotoxicity (9–11).
One of the mechanisms by which ZEA exerts its toxic effects is through the induction of oxidative stress and damage. Studies have demonstrated that ZEA disrupts the balance between the antioxidant and oxidative systems by causing mitochondrial dysfunction, inducing endoplasmic reticulum stress, and inhibiting the activity of antioxidant enzymes, thereby promoting oxidative stress and damage (9, 12, 13). It has been reported that exposure to ZEA induces mitochondrial damage in mouse liver cells, leading to the overproduction of reactive oxygen species (ROS), which in turn causes oxidative stress and liver damage (12). Additionally, ZEA contributes to injury in chicken embryo fibroblasts by inducing endoplasmic reticulum stress (13). ZEA reduces the activity of antioxidant enzymes and the antioxidant capacity in meat rabbits by inhibiting the expression of some key genes in the nuclear factor E2-related factor 2 (Nrf2)/antioxidant response element (ARE) signaling pathway (9). Furthermore, ZEA can exert toxic effects by triggering inflammatory responses. Many immune cells, including macrophages, B cells, and NK cells, express estrogen receptors on their surface, allowing ZEA to modulate the immune response through estrogen-like activity (14, 15). A previous study reported that exposure to ZEA significantly increased the levels of tumor necrosis factor-α (TNF-α) and interleukin-4 (IL-4) while significantly decreasing interleukin-10 (IL-10) levels in the liver of meat rabbits, leading to inflammatory responses and injuries (9). It is worth noting that the inflammatory response is an important pathway through which ZEA and other environmental estrogens induce apoptosis in ovarian cells and impair ovarian function (16).
The ovary, as a critical reproductive organ in rabbits, is responsible for oocyte production and release, hormone secretion, and other essential physiological functions. It is a target organ for ZEA toxicity, with exposure leading to a range of ovarian disorders, such as granulosa cell damage and apoptosis, reduced ovarian reserve, abnormal ovarian development, and hormone secretion dysfunction, which directly impair the physiological function of the ovary (17–19). This study, for the first time, elucidates the toxic effects of ZEA on the ovaries of Hyla rabbits, along with preliminary insights into the underlying mechanisms involving oxidative stress and inflammatory response. Our findings will provide valuable scientific references for establishing limit standards for ZEA in rabbit production and for the prevention and control of ZEA contamination.
2 Materials and methods
2.1 Experimental materials and basal diets
ZEA, with ≥98% purity, was purchased from TripleBond Scientific Inc. (Guelph, Canada). The basal diets were formulated and prepared based on the nutrient requirements for rabbits as outlined by the NRC (1977), with their composition and nutrient levels shown in Table 1.
Mycotoxin levels (ZEA, aflatoxin B1, and vomitoxin) were measured after the diet preparation using ELISA kits. The ZEA contents in each group were 63.91, 351.08, and 675.32 μg/kg, respectively, while aflatoxin B1 and vomitoxin were undetectable in the diets of all groups. The ELISA kits were purchased from Shenzhen Finder Biotech Co., Ltd. (Shenzhen, China).
2.2 Experimental grouping and feeding management
Ninety healthy Hyla rabbits with similar body weights (1.35 ± 0.08 kg) were randomly divided into three groups, with 30 replicates per group and one rabbit per replicate. The rabbits in the three groups were fed basal diets supplemented with 0, 300, and 600 μg/kg ZEA, respectively. The rabbits were housed in individual cages with ad libitum access to food and water for 42 days.
The experimental protocols were approved by the Animal Care and Use Committee of Hebei Agriculture University (Baoding, China) (Protocol: 2023139).
All animal experiments complied with the ARRIVE guidelines were carried out in accordance with the U.K. Animals (Scientific Procedures) Act, 1986 and associated guidelines, EU Directive 2010/63/EU for animal experiments.
2.3 Measurement indicators and methods
2.3.1 Growth performance and ovarian index
The fasting body weight of rabbits in each group was measured at the beginning and end of the experiment, and the average daily gain (ADG) was calculated. The average daily feed intake (ADFI) was determined by weighing the feed provided to each group, and the feed-to-gain ratio (F:G) was calculated based on ADFI and ADG. The live weight of the rabbits was measured before slaughter. Subsequently, the weight of the ovaries was measured after slaughter to calculate the ovarian index using the following formula:
2.3.2 Morphology and structure of ovarian tissue
Ovarian tissue samples were fixed in a 4% paraformaldehyde solution. Paraffin embedding and hematoxylin-eosin staining (HE) staining of the ovarian tissues were performed following the method reported by Wu et al. (9). A microscope camera system (BA200 Digital) was employed for tissue observation and image acquisition (Motic, Xiamen, China).
2.3.3 Serum and ovarian tissue indicators
At the end of the experimental period, eight rabbits with body weights close to the group average were selected from each group for blood collection via cardiac puncture after 12 h of fasting. Blood samples were collected into vacuum tubes and centrifuged at 3,000 × g for 10 min at 4°C to separate the serum. After blood sampling, the rabbits were sacrificed by manual cervical dislocation, and ovarian tissue samples were collected. Both serum and ovarian samples were stored at −80°C for subsequent analysis. The activity of superoxide dismutase (SOD) and the levels of TNF-α, interleukin-1β (IL-1β), IL-4, interleukin-6 (IL-6), IL-10, gonadotropin-releasing hormone (GnRH), follicle-stimulating hormone (FSH), LH, estradiol, and progesterone were measured using ELISA kits obtained from Jiangsu Meimian Biotechnology Co., Ltd. (Yancheng, Chian) and Shanghai COIBO Biotechnology Co., Ltd. (Shanghai, China). The activities of TAOC and GSH-Px were measured using a colorimetric method, while CAT activity was determined via visible light detection. Furthermore, the MDA content was assessed using thiobarbituric acid method. All assay kits were purchased from Nanjing Jiancheng Bioengineering Institute (Nanjing, China).
2.3.4 Expression levels of ovary-related genes
Ovarian tissue samples (100 mg) were collected, and the relative expression levels of follicle-stimulating hormone receptor (FSHR), luteinizing hormone receptor (LHR), heat shock protein 70 (HSP70), GSH-Px, copper and zinc superoxide dismutase (Cu/Zn-SOD), manganese superoxide dismutase (Mn-SOD), TNF-α, IL-1β, IL-4, IL-6, IL-10, and glyceraldehyde-3-phosphate dehydrogenase (GAPDH) were analyzed using qRT-PCR. Primers were designed and synthesized based on gene sequences available in GenBank (Table 2). Total RNA was extracted using a Trizol kit following the manufacturer’s instructions (Invitrogen, Massachusetts, United States), and RNA concentration was measured using a NanoDrop Lite spectrophotometer (Thermo, Massachusetts, United States). Next, cDNA was synthesized using a reverse transcription kit according to the provided protocol (Vazyme, Nanjing, China). qRT-PCR premixes were prepared using a qRT-PCR kit (Vazyme, Nanjing, China), quantitative primers, and cDNA templates. GAPDH was used as the internal reference gene, and all the experiments were performed in triplicate. The expression levels of the target genes were calculated using the method.
2.4 Statistical analysis
Excel 2021 and SPSS 26.0 were used for the statistical analysis of the data. One-way analysis of variance (ANOVA) was used to test for significant differences between the groups, and Duncan’s method was employed for multiple comparisons. A p-value less than 0.05 (p < 0.05) was considered indicative of a significant difference.
3 Results
3.1 Growth performance and ovarian index
The ovarian index of the group supplemented with 600 μg/kg ZEA was significantly higher than that of the control group (p < 0.05) (Table 3). There were no significant differences in initial body weight, final body weight, ADG, ADFI, and F/G among all groups (p > 0.05).
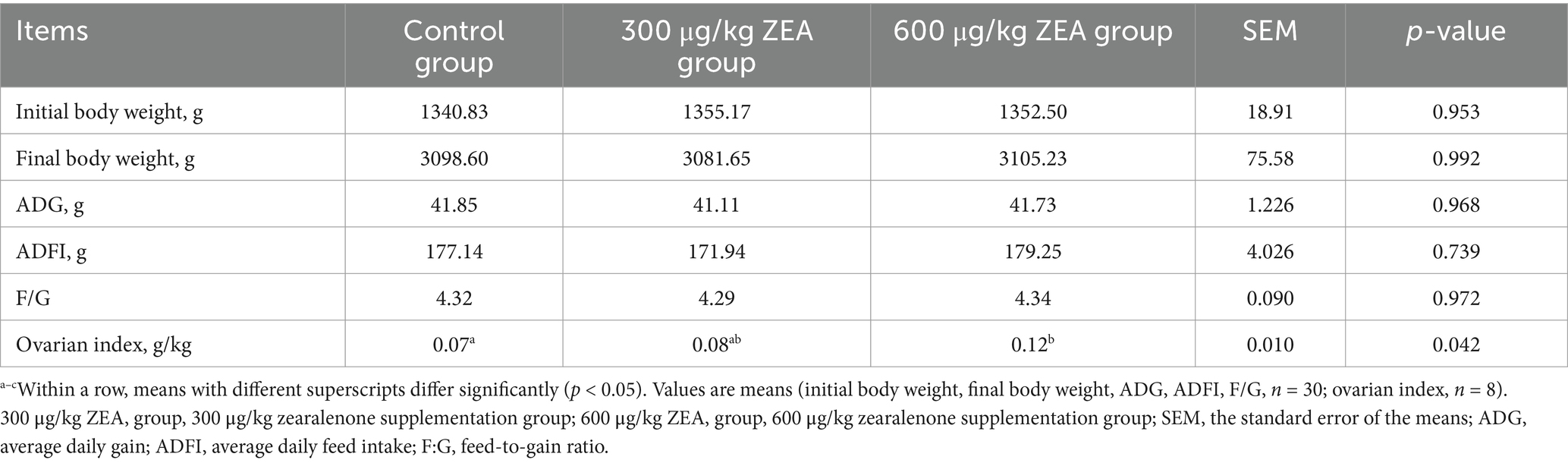
Table 3. Effect of zearalenone-contaminated diet on the growth performance and ovarian index of meat rabbits.
3.2 Structure and morphology of ovarian tissue
In the control group, the ovarian tissue of rabbits exhibited a substantial number of follicles at various developmental stages, including primordial and primary follicles (Figure 1). The follicles displayed regular morphology, with no evidence of interstitial proliferation, necrosis, or inflammatory cell infiltration (A). Compared to the control group, no significant pathological changes or follicular changes were observed in the 300 μg/kg ZEA supplementation group (B). However, in the 600 μg/kg ZEA supplementation group, the follicles of meat rabbits exhibited dilation, thinning of the granulosa layer, punctate necrosis of granulosa cells, and shrinkage, deep staining, and fragmentation of granulosa cell nuclei compared to the control group (C).
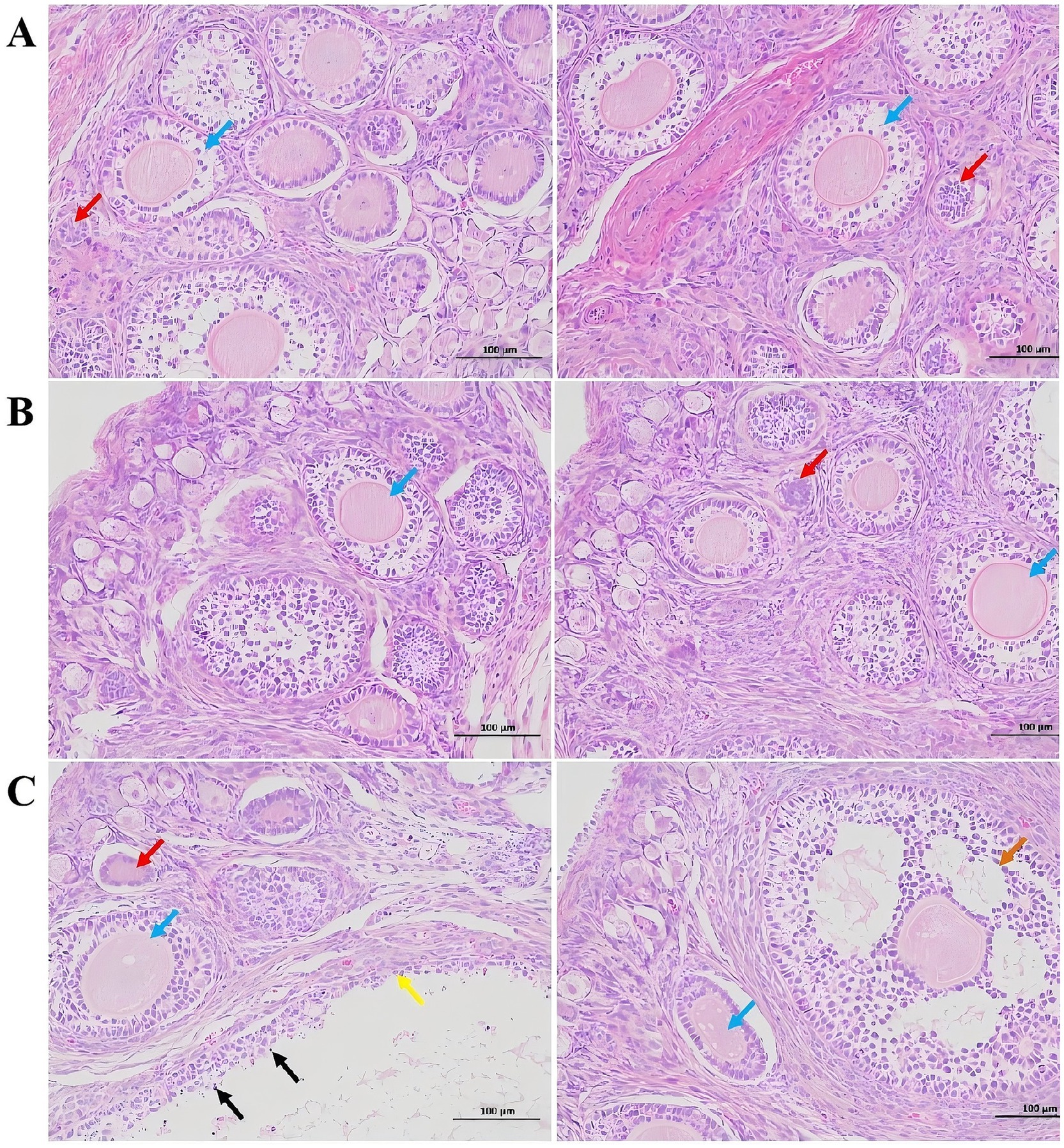
Figure 1. Effect of zearalenone-contaminated diet on the structure and morphology of the ovarian tissues of meat rabbits. (A) (Control group), (B) (300 μg/kg ZEA supplementation group), and (C) (600 μg/kg ZEA supplementation group) illustrate the ovarian tissues at 200× magnification and scales of 100 μm for the control group and experimental groups, respectively (n = 8). In these images, red arrows indicate primordial follicles; blue arrows indicate primary follicles; orange arrows indicate secondary follicles; yellow arrows indicate follicular dilation; and black arrows indicate punctate necrosis of granulosa cells.
3.3 Serum indicators
3.3.1 Serum reproductive hormone indicators
Compared to the control group, the blood levels of GnRH and E2 were significantly elevated in meat rabbits from the 600 μg/kg ZEA supplementation group (p < 0.05) (Table 4). Additionally, the blood LH levels in the 600 μg/kg ZEA supplementation group were significantly higher than those in both the control group and the 300 μg/kg ZEA supplementation group (p < 0.05).
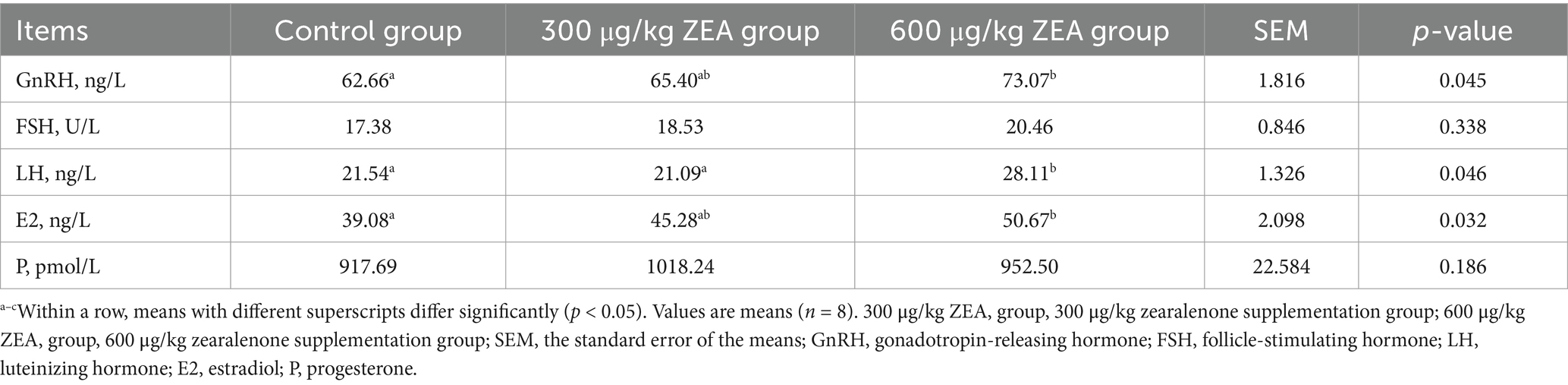
Table 4. Effects of zearalenone-contaminated diet on serum reproductive hormone levels in meat rabbits.
3.3.2 Serum antioxidant indicators
Compared to the control group, the activities of TAOC and GSH-Px in the blood of meat rabbits in the 600 μg/kg ZEA supplementation group were significantly reduced, while the MDA level was significantly elevated (p < 0.05) (Table 5).
3.3.3 Serum inflammatory indicators
Furthermore, the blood IL-1β levels in meat rabbits in the 600 μg/kg ZEA supplementation group were significantly elevated compared to the control group and the 300 μg/kg ZEA supplementation group (p < 0.05) (Table 6).
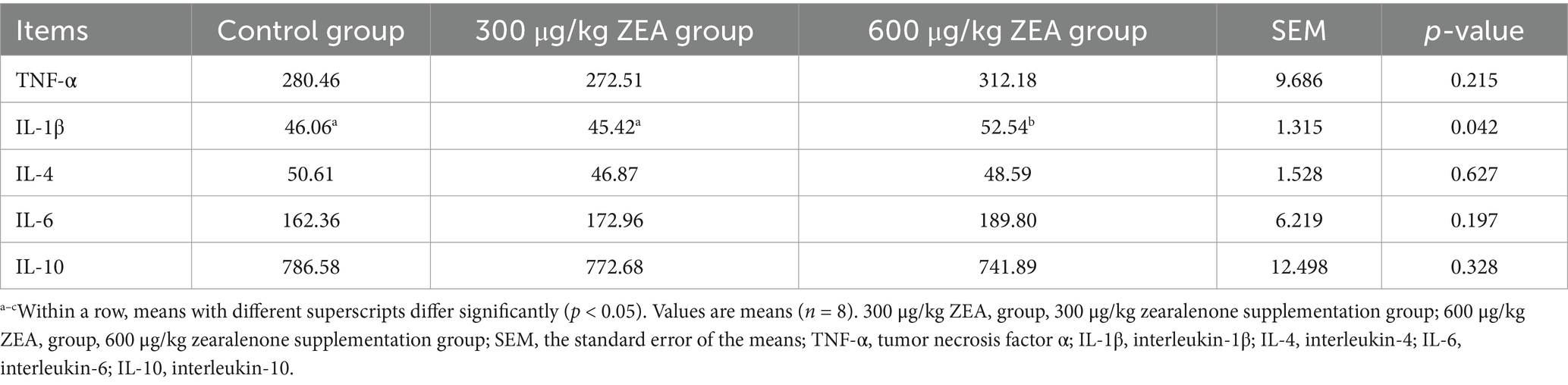
Table 6. Effects of zearalenone-contaminated diet on serum inflammation indicators in meat rabbits (pg/mL).
3.4 Ovarian indicators
3.4.1 Ovarian antioxidant indicators
TAOC levels in the ovaries of meat rabbits in the 600 μg/kg ZEA supplementation group were significantly reduced compared to the control group and 300 μg/kg ZEA supplementation group (p < 0.05) (Table 7). In addition, compared to the 300 μg/kg ZEA supplementation group, GSH-Px activity in the ovaries of meat rabbits in the 600 μg/kg ZEA supplementation group was significantly lower, while the MDA level was markedly increased (p < 0.05).
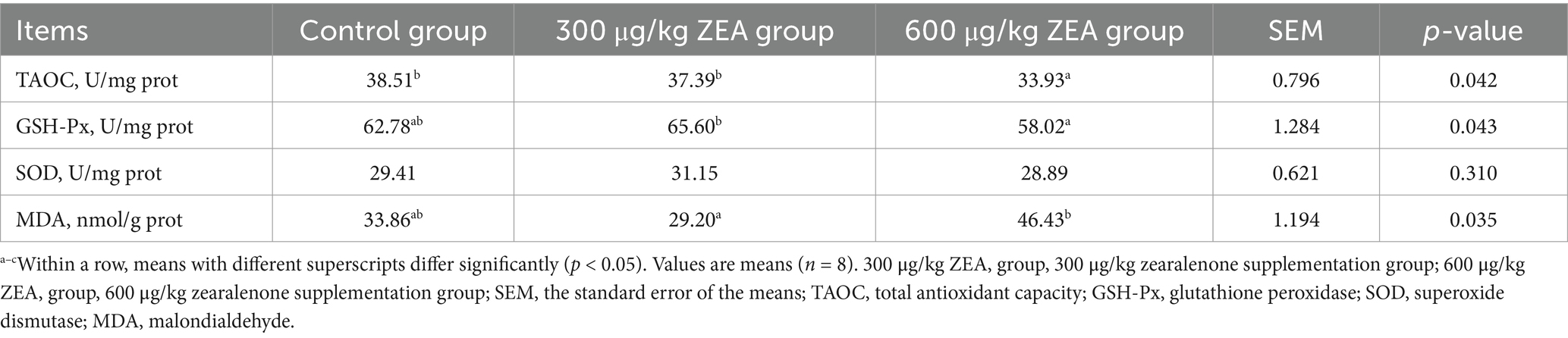
Table 7. Effects of zearalenone-contaminated diet on ovarian antioxidant indicators in meat rabbits.
3.4.2 Ovarian inflammatory indicators
Compared to the control group and the 300 μg/kg ZEA supplementation group, the TNF-α level in the ovaries of meat rabbits was significantly elevated in the 600 μg/kg ZEA supplementation group (p < 0.05) (Table 8).
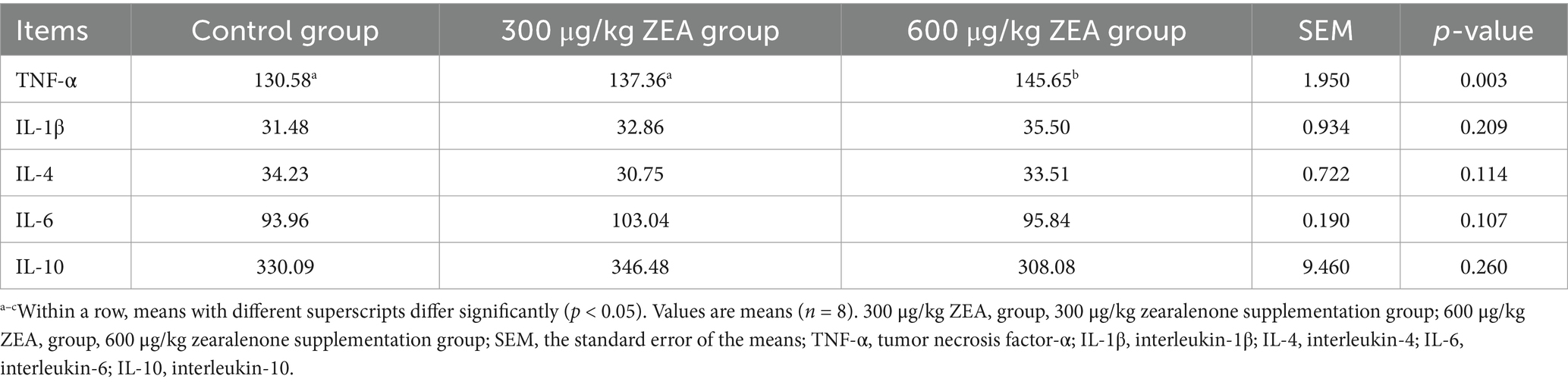
Table 8. Effects of zearalenone-contaminated diet on ovarian inflammatory indicators in meat rabbits (pg/mg prot).
3.5 Expression level of ovarian-related genes
3.5.1 Expression of ovarian FSHR, LHR, HSP70, and antioxidant-related genes
As shown in Figure 2, compared to the control group, the abundance of LHR mRNA (B) was significantly increased, while Cu/Zn-SOD mRNA (E) abundance was significantly decreased in the ovaries of meat rabbits in the 600 μg/kg ZEA supplementation group (p < 0.05). Moreover, the abundance of HSP70 mRNA (C) in the ovaries of meat rabbits in the 600 μg/kg ZEA supplementation group was significantly higher compared to both the control group and the 300 μg/kg ZEA supplementation group (p < 0.05).
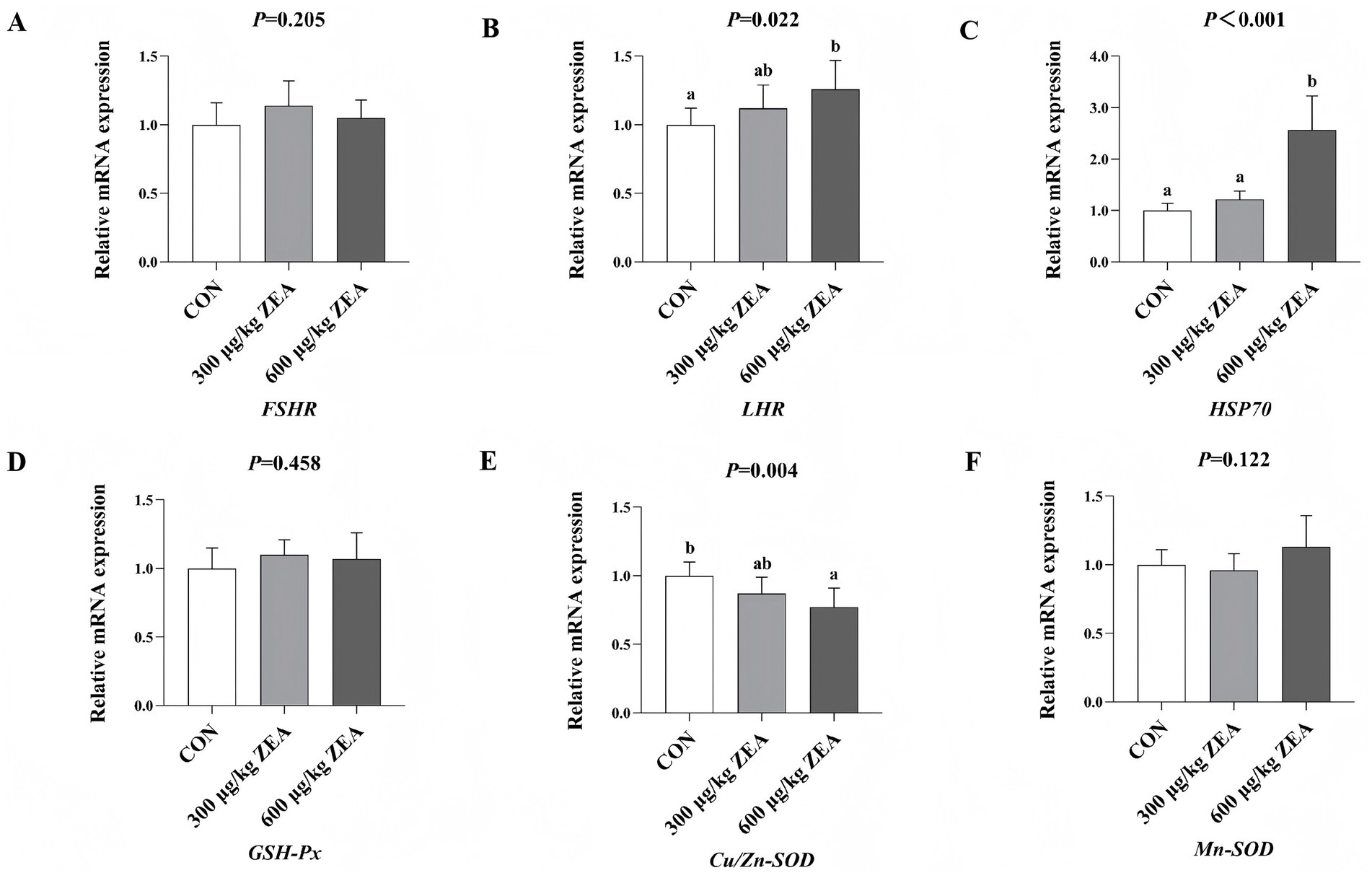
Figure 2. Effects of zearalenone-contaminated diet on the relative expression level of FSHR, LHR, HSP70, and antioxidant-related genes in the ovary of meat rabbits. Bars represent the means ± standard deviation (SD) (n = 8). Above the bar, no letter or the same letter means no significant difference (p > 0.05), while different letters indicate a significant difference (p < 0.05). CON, control group; 300 μg/kg ZEA, 300 μg/kg ZEA supplementation group; 600 μg/kg ZEA, 600 μg/kg ZEA supplementation group; FSHR, follicle-stimulating hormone receptor (A); LHR, luteinizing hormone receptor (B); HSP70, heat shock protein 70 (C); GSH-Px, glutathione peroxidase (D); Cu/Zn-SOD, copper and zinc superoxide dismutase (E), Mn-SOD, manganese superoxide dismutase (F).
3.5.2 Expression of ovarian inflammatory response-related genes
Compared to the control group, the abundance of TNF-α mRNA (A) in the ovaries of meat rabbits in the 600 μg/kg ZEA supplementation group was significantly increased (p < 0.05) (Figure 3). Additionally, the abundance of IL-1β mRNA (B) in the ovaries of meat rabbits in the 600 μg/kg ZEA supplementation group was significantly increased compared to both the control group and 300 μg/kg ZEA supplementation group.
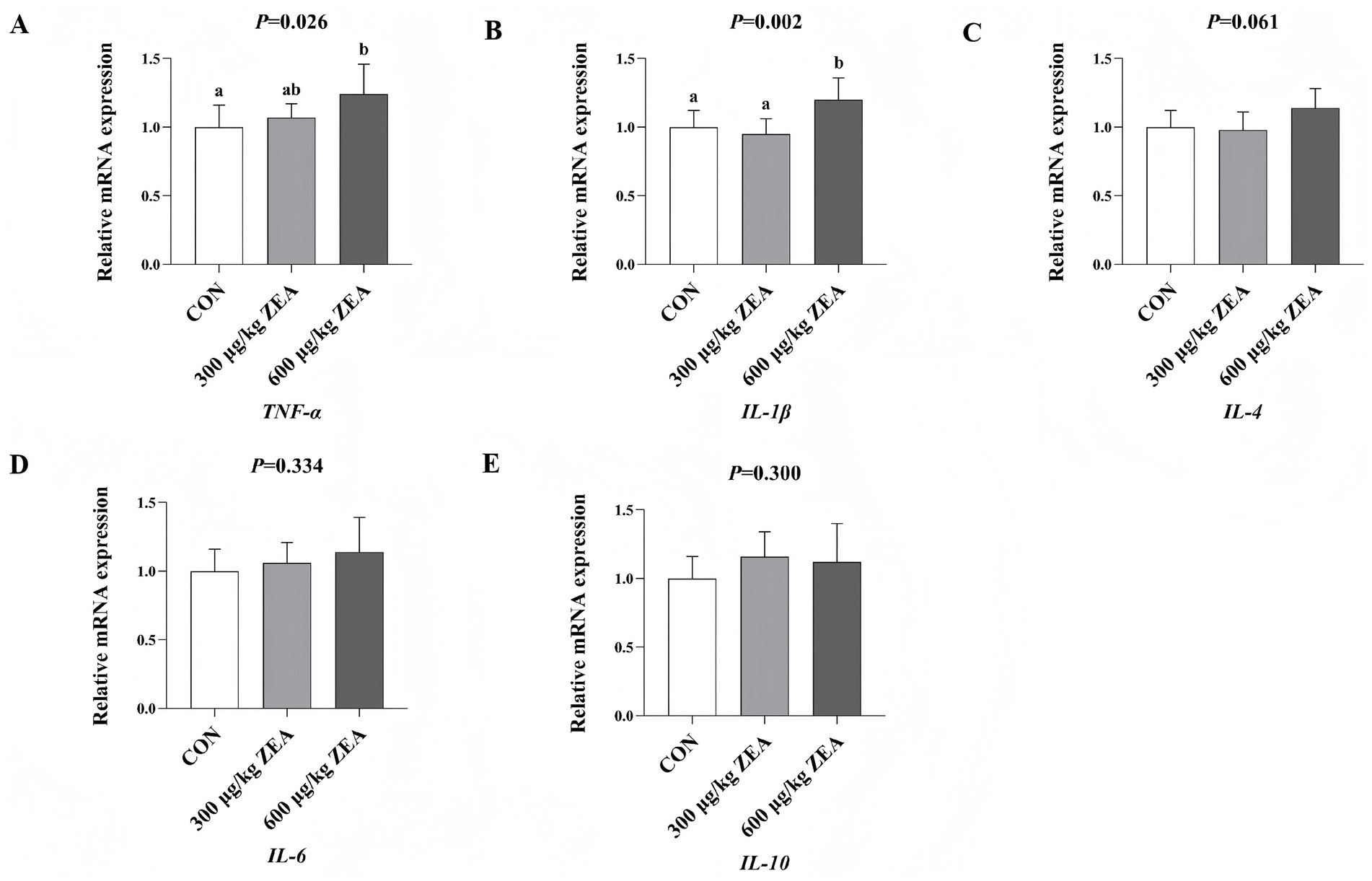
Figure 3. Effects of zearalenone-contaminated diet on the relative expression level of inflammatory response-related genes in the ovary of meat rabbits. Bars represent the means ± standard deviation (SD) (n = 8). Above the bar, no letter or the same letter means no significant difference (p > 0.05), while different letters indicate a significant difference (p < 0.05). CON, control group; 300 μg/kg ZEA, 300 μg/kg ZEA supplementation group; 600 μg/kg ZEA, 600 μg/kg ZEA supplementation group; TNF-α, tumor necrosis factor-α (A); IL-1β, interleukin-1β (B); IL-4, interleukin-4 (C); IL-6, interleukin-6 (D); IL-10, interleukin-10 (E).
4 Discussion
ZEA is a highly non-polar, fat-soluble compound that is primarily absorbed in the intestinal tract upon entering the organism. After a series of metabolic reactions, such as monohydroxylation, dehydroreduction, and conjugation in the liver and gallbladder, ZEA’s metabolites and derivatives are distributed to various tissues and organs throughout the body, including the ovaries, uterus, liver, and intestines after reabsorption in the intestinal tract (20–22). It is well known that ZEA can cause oxidative stress and apoptosis in hepatocytes and enterocytes by inducing mitochondrial damage, triggering endoplasmic reticulum stress, and inhibiting antioxidant enzymes. These effects disrupt the organism’s ability to digest, absorb, and metabolize nutrients, ultimately impacting its growth and development (23, 24). However, several studies have reported that ZEA does not significantly affect the growth performance of animals. For example, Wu et al. (9) indicated that feeding rabbits a diet supplemented with 600 μg/kg ZEA for 28 days induced inflammatory response and oxidative stress in the liver but had no significant impact on ADG, ADFI, and F/G. Similarly, Reddy et al. (25) reported that feeding 42-day-old boars a diet supplemented with 800 μg/kg ZEA for 28 days did not significantly affect the ADG, ADFI, and F/G. Peillod et al. (26) found that feeding 84-day-old male ducks a diet supplemented with 500 μg/kg ZEA for 12 days exhibited no significant effect on total feed intake, ADG, and feed conversion ratio. In this experiment, no significant differences in initial body weight, final body weight, ADG, ADFI, and F/G were observed among the groups, indicating that ZEA had no significant effect on the growth performance of meat rabbits, which is partially consistent with the findings reported above. The lack of significant effects may be attributed to the dose of ZEA and the duration of the experiment. In addition, ZEA toxicity mainly targets the reproductive system rather than the digestive system, with reproductive toxicity generally being more severe than growth-related toxicity.
The ovaries serve both reproductive and endocrine functions and are among the major target organs for ZEA-induced reproductive toxicity. Dai et al. (27) investigated the effects of dietary ZEA supplementation in 28-day-old piglets and found that feeding a diet containing 1,040 μg/kg ZEA for 35 days enhanced the autocrine action of growth hormone-releasing peptide, upregulated gene expression in the porcine ovary, and stimulated the development of the ovary and ovarian follicles. Yang et al. (28) supplemented the diets of 25-day-old piglets with 500, 1,000, and 1,500 μg/kg ZEA for 35 days and found that all three doses promoted ovarian development. They further demonstrated that the activation of the estrogen receptors (ERs)/glycogen synthase kinase (GSK-3β)-dependent Wnt inhibitory factor-1 (WIF-1)/β-catenin signaling pathway by ZEA played a critical role in this promotive effect. In this study, the ovarian index of meat rabbits in the 600 μg/kg ZEA supplementation group was significantly elevated, suggesting enhanced ovarian development. This finding partially aligns with the results reported above. Given that there are limited reports on the effects of ZEA on ovarian development in rabbits, further research is needed to determine whether ZEA promotes development through the pathways mentioned above. Inflammatory factors, particularly those involving the production of tumor necrosis factor (TNF), are key contributors to ovarian cell apoptosis in mammals induced by environmental estrogens like ZEA (16). Moreover, ZEA disrupts the balance between oxidative and antioxidant systems, leading to oxidative stress and cellular injury, which are crucial pathways through which ZEA induces ovarian cell apoptosis and damages ovarian tissue (29). In this experiment, pathological changes observed in the ovaries of meat rabbits in the 600 μg/kg ZEA supplementation group, including granulosa cell necrosis and nuclear fragmentation, were associated with ZEA-induced inflammatory response, as evidenced by significant increases in TNF-α levels and gene expression in the ovaries, and oxidative stress, indicated by significantly elevated MDA levels in blood and ovaries.
GnRH, a neurohormone secreted by the hypothalamus, regulates the secretion of gonadotropins by the pituitary gland and has a significant reproduction regulatory effect (30). Yang et al. (31) reported that administering 5 mg/kg of ZEA to 15-day-old rats for 5 days significantly increased the expression levels of GnRH mRNA and protein in the hypothalamus by mediating the kisspeptin-GPR54-GnRH pathway. Additionally, significant increases in serum FSH, LH, and E2 levels, as well as uterine weight, were observed. Similar to the findings of Yang et al. (31) and Kriszt et al. (32) demonstrated that administering 10 mg/kg ZEA to 18-day-old rats for 10 days activated kisspeptin neurons in the periventricular nucleus of the anterior lateral ventricle of the hypothalamus, which in turn stimulated the expression of GnRH mRNA, resulting in increased uterine weights and a higher number of follicles in the ovarian sinusoids. In this study, serum GnRH levels were significantly elevated in meat rabbits in the 600 μg/kg ZEA supplementation group. This finding indicates that ZEA exerts a regulatory effect on GnRH in meat rabbits, partially consistent with previous reports; however, the specific underlying regulatory mechanisms remain unidentified. GnRH interacts with its receptors in the pituitary gland via the pituitary portal system, regulating the secretion of FSH and LH (33). In this study, serum LH levels were significantly elevated in meat rabbits in the 600 μg/kg ZEA supplementation group, a response likely associated with the increased GnRH levels. Zheng et al. (33) demonstrated that ZEA and its metabolites inhibit the synthesis and secretion of LH by modulating the GPR30-G-AC/cAMP-PKA-ERK-LHX3-miR-7-FOS pathway in the porcine pituitary. Similarly, Yuan et al. (34) reported a comparable effect on LH levels in 25-week-old laying hens fed diets supplemented with 0.75 mg/kg ZEA for 35 days. Zheng et al. (33) and Yuan et al. (34) reported contrasting results, which may be attributed to species-specific differences in sensitivity to ZEA. However, the precise regulatory mechanisms underlying these differences have not yet been elucidated. A previous study found that LH interacts with the LHR receptor in the ovary to regulate E2 secretion (33). In this study, the expression of LHR mRNA and serum E2 levels were significantly elevated in the ovaries of rabbits in the 600 μg/kg ZEA supplementation group. These changes were associated with the increased serum LH levels and the impact of ZEA-induced oxidative stress and inflammatory responses on ovarian endocrine function. The results of this experiment suggest that ZEA exposure may increase the level of GnRH by stimulating the hypothalamus. GnRH can act on the GnRH receptors in the pituitary gland, raising the level of LH, which further acts on the LHR in the ovaries, increasing the level of E2, thereby interfering with the neuroendocrine function of the HPOA.
ZEA disrupts the balance between oxidative and antioxidant systems in the body, leading to oxidative stress and tissue injury, which contribute to various toxic effects (33, 35–37). This study found that supplementation with 600 μg/kg ZEA significantly reduced TAOC in rabbit ovaries by downregulating the expression of the antioxidant enzyme regulatory gene Cu/Zn-SOD mRNA. Meanwhile, the expression of HSP70 mRNA and serum MDA levels were significantly elevated in the ovaries of rabbits supplemented with ZEA, suggesting that ZEA impacts the antioxidant system of meat rabbits by regulating the expression of antioxidant enzyme-related genes and the activity of antioxidant enzymes, thereby inducing oxidative stress to a certain extent. According to Wu et al. (9), ZEA inhibits SOD and GSH-Px activities in rabbits through the Nrf2/ARE signaling pathway, leading to increased MDA levels and consequent oxidative stress and injury (9). These findings are consistent with some of the results observed in this experiment. Oxidative stress is closely linked to inflammatory responses. ZEA and its metabolites can stimulate electron leakage from the respiratory chain, leading to an increase in free radicals. Subsequently, excessive free radicals can trigger an inflammatory response through the modal or non-modal receptor pathways (38). For example, ZEA can activate ROS-mediated NLRP3 inflammatory vesicles, which in turn promote the activation of the inflammatory cytokine IL-1β via cysteine-dependent aspartic protein hydrolase 1 (39). In this study, supplementation with 600 μg/kg ZEA significantly elevated the TNF-α level by increasing the abundance of TNF-α mRNA in the rabbit ovary. Furthermore, both the expression of IL-1β mRNA in the ovary and serum IL-1β levels were significantly increased, suggesting the induction of an inflammatory response. These findings align with those reported by Wu et al. (9), who observed similar effects following the addition of ZEA to rabbit diets. The oxidative stress and inflammatory response induced by ZEA in this study were directly linked to ovarian damage and dysfunction. Based on the data from this study, we summarized the toxic effects and mechanisms of zearalenone exposure on rabbits in the form of a schematic diagram (Figure 4).
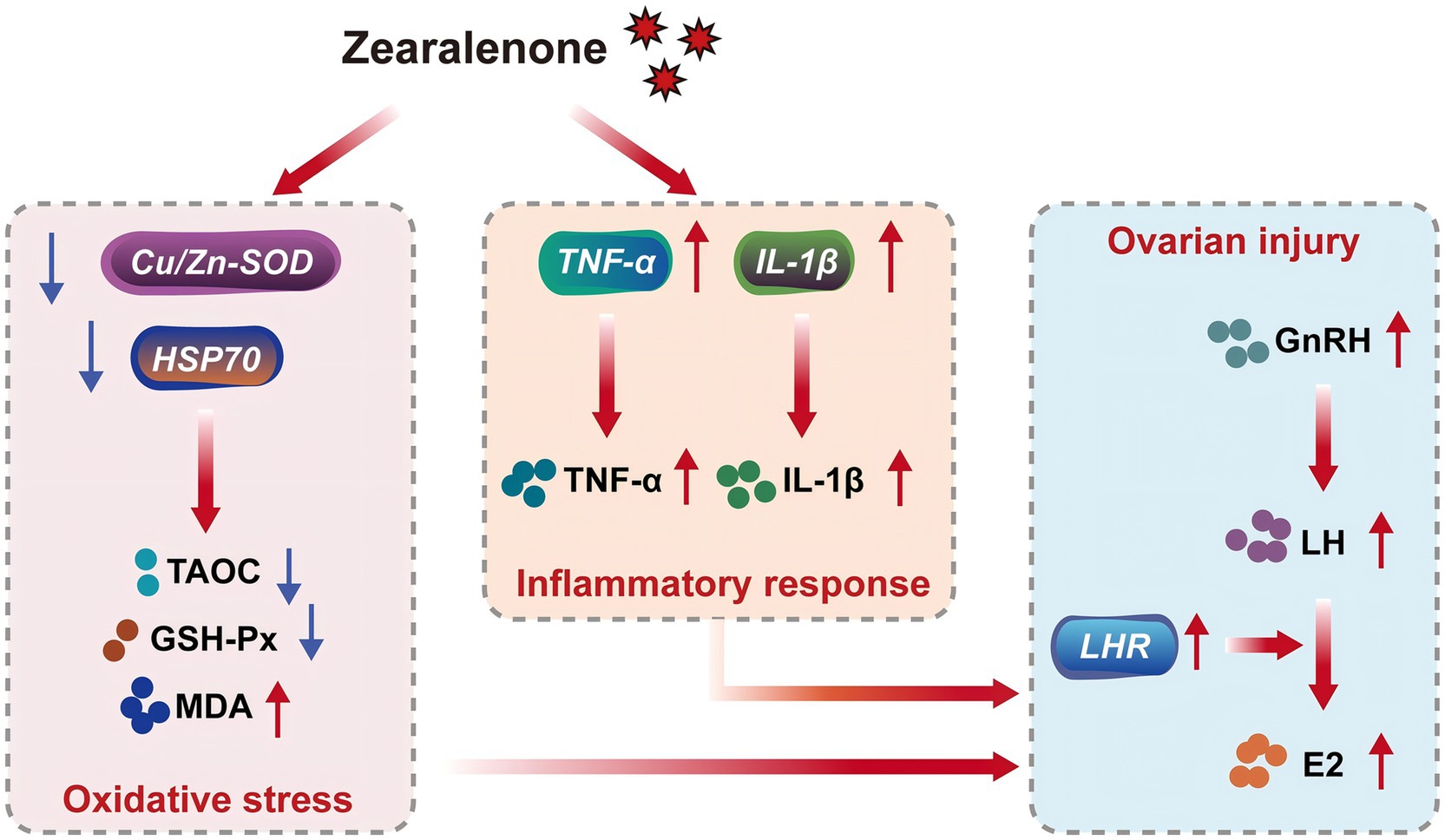
Figure 4. Toxicity and mechanism of zearalenone exposure in meat rabbits. Cu/Zn-SOD, copper and zinc superoxide dismutase; HSP70, heat shock protein 70; TAOC, total antioxidant capacity; GSH-Px, glutathione peroxidase; MDA, malondialdehyde; TNF-α, tumor necrosis factor-α; IL-1β, interleukin-1β; GnRH, gonadotropin-releasing hormone; LH, luteinizing hormone; LHR, luteinizing hormone receptor; E2, estradiol.
5 Conclusion
In conclusion, supplementation with 600 μg/kg ZEA induces oxidative stress and inflammatory response by modulating the abundance of Cu/Zn-SOD mRNA, TNF-α mRNA, and IL-1β mRNA in the ovaries of meat rabbits. This disruption affects ovarian development, leading to ovarian damage and abnormal estradiol secretion. In contrast, 300 μg/kg ZEA did not have a significant effect on ovarian development or secretion in rabbits.
Data availability statement
The original contributions presented in the study are included in the article/supplementary material, further inquiries can be directed to the corresponding author.
Ethics statement
The animal study was approved by the Animal Care and Use Committee of Hebei Agriculture University (Baoding, China) (Protocol: 2023139). All animal experiments complied with the ARRIVE guidelines were carried out in accordance with the U.K. Animals (Scientific Procedures) Act, 1986 and associated guidelines, EU Directive 2010/63/EU for animal experiments. The study was conducted in accordance with the local legislation and institutional requirements.
Author contributions
FWu: Investigation, Project administration, Resources, Validation, Writing – original draft, Writing – review & editing. FWa: Investigation, Writing – original draft. ZT: Investigation, Writing – original draft. XY: Data curation, Resources, Writing – original draft. YL: Data curation, Writing – original draft. MZ: Data curation, Writing – original draft. SL: Validation, Writing – original draft. SH: Validation, Writing – original draft. BC: Funding acquisition, Writing – original draft, Writing – review & editing.
Funding
The author(s) declare that financial support was received for the research and/or publication of this article. This research was funded by Hebei Agricultural University Introduced a Talent Research Project (YJ2024023), Research Project on Basic Scientific Research Business Expenses for Provincial Universities in Hebei Province (KY2024045), and the Modern Agriculture Industry Technology System of Rabbit (CARS-43-B-2).
Conflict of interest
ZT was employed Hebei Research Institute of Microbiology Co., Ltd.
The remaining authors declare that the research was conducted in the absence of any commercial or financial relationships that could be construed as a potential conflict of interest.
Generative AI statement
The authors declare that no Gen AI was used in the creation of this manuscript.
Publisher’s note
All claims expressed in this article are solely those of the authors and do not necessarily represent those of their affiliated organizations, or those of the publisher, the editors and the reviewers. Any product that may be evaluated in this article, or claim that may be made by its manufacturer, is not guaranteed or endorsed by the publisher.
References
1. Rai, A, Das, M, and Tripathi, A. Occurrence and toxicity of a fusarium mycotoxin, zearalenone. Crit Rev Food Sci Nutr. (2020) 60:2710–29. doi: 10.1080/10408398.2019.1655388
2. Knutsen, HK, Alexander, J, Barregård, L, Bignami, M, Brüschweiler, B, Ceccatelli, S, et al. Risks for animal health related to the presence of zearalenone and its modified forms in feed. EFSA J. (2017) 15:e04851. doi: 10.2903/j.efsa.2017.4851
3. Zhou, J, Zhao, L, Huang, S, Liu, Q, Ao, X, Lei, Y, et al. Zearalenone toxicosis on reproduction as estrogen receptor selective modulator and alleviation of zearalenone biodegradative agent in pregnant sows. J Anim Sci Biotechnol. (2022) 13:36. doi: 10.1186/s40104-022-00686-3
4. Sun, N, Haseeb, A, Sun, P, Zhang, H, Zhong, J, Yin, W, et al. Scutellarin targets Wnt5a against zearalenone-induced apoptosis in mouse granulosa cells in vitro and in vivo. J Hazard Mater. (2024) 464:132917. doi: 10.1016/j.jhazmat.2023.132917
5. Yi, Y, Wan, S, Wang, S, Khan, A, Guo, J, Zheng, X, et al. Scutellarin protects mouse ovarian granulosa cells from injury induced by the toxin zearalenone. Food Funct. (2021) 12:1252–61. doi: 10.1039/d0fo02711a
6. Gajęcka, M, Zielonka, Ł, Babuchowski, A, and Gajęcki, MT. Exposure to low zearalenone doses and changes in the homeostasis and concentrations of endogenous hormones in selected steroid-sensitive tissues in pre-pubertal gilts. Toxins. (2022) 14:790. doi: 10.3390/toxins14110790
7. Wan, B, Huang, L, Jing, C, Li, Y, Jiao, N, Liang, M, et al. Zearalenone promotes follicle development through activating the SIRT1/PGC-1α signaling pathway in the ovaries of weaned gilts. J Anim Sci. (2022) 100:skac058. doi: 10.1093/jas/skac058
8. Shi, Z, Wu, Z, Wang, Z, Liu, T, Xie, T, Liu, N, et al. Protective effects of dietary supplementation of Bacillus subtilis MZ18 against the reproductive toxicity of zearalenone in pregnant rats. Ecotoxicol Environ Saf. (2025) 292:117954. doi: 10.1016/j.ecoenv.2025.117954
9. Wu, F, Wang, F, Tang, Z, Yang, X, Liu, Y, Zhao, M, et al. Quercetagetin alleviates zearalenone-induced liver injury in rabbits through Keap1/Nrf2/ARE signaling pathway. Front Pharmacol. (2023) 14:1271384. doi: 10.3389/fphar.2023.1271384
10. Zhang, FL, Zhu, KX, Wang, JY, Zhang, M, Yan, JM, Liu, QC, et al. Cross-species analysis of transcriptome emphasizes a critical role of TNF-α in mediating MAP2K7/AKT2 signaling in zearalenone-induced apoptosis. J Hazard Mater. (2023) 459:132226. doi: 10.1016/j.jhazmat.2023.132226
11. Pierron, A, Kleber, A, Mayer, E, and Gerner, W. Effect of DON and ZEN and their metabolites DOM-1 and HZEN on B cell proliferation and antibody production. Front Immunol. (2024) 15:1338937. doi: 10.3389/fimmu.2024.1338937
12. Yang, X, Zheng, H, Niu, J, Chen, X, Li, H, Rao, Z, et al. Curcumin alleviates zearalenone-induced liver injury in mice by scavenging reactive oxygen species and inhibiting mitochondrial apoptosis pathway. Ecotoxicol Environ Saf. (2024) 277:116343. doi: 10.1016/j.ecoenv.2024.116343
13. Hu, H, Yu, Q, Zheng, Y, Cui, H, Huang, X, and Zhang, K. Forsythoside A protects against zearalenone-induced cell damage in chicken embryonic fibroblasts via mitigation of endoplasmic reticulum stress. Vet Res Commun. (2024) 48:1659–70. doi: 10.1007/s11259-024-10350-y
14. Igarashi, H, Kouro, T, Yokota, T, Comp, PC, and Kincade, PW. Age and stage dependency of estrogen receptor expression by lymphocyte precursors. Proc Natl Acad Sci USA. (2001) 98:15131–6. doi: 10.1073/pnas.011513098
15. Curran, EM, Berghaus, LJ, Vernetti, NJ, Saporita, AJ, Lubahn, DB, and Estes, DM. Natural killer cells express estrogen receptor-alpha and estrogen receptor-beta and can respond to estrogen via a non-estrogen receptor-alpha-mediated pathway. Cell Immunol. (2001) 214:12–20. doi: 10.1006/cimm.2002.1886
16. Zhang, FL, Kong, L, Zhao, AH, Ge, W, Yan, ZH, Li, L, et al. Inflammatory cytokines as key players of apoptosis induced by environmental estrogens in the ovary. Environ Res. (2021) 198:111225. doi: 10.1016/j.envres.2021.111225
17. Li, Y, Gao, Y, Yao, D, Li, Z, Wang, J, Zhang, X, et al. Heme oxygenase-1 regulates zearalenone-induced oxidative stress and apoptosis in sheep follicular granulosa cells. Int J Mol Sci. (2024) 25:2578. doi: 10.3390/ijms25052578
18. Roach, CM, Mayorga, EJ, Baumgard, LH, Ross, JW, and Keating, AF. Phenotypic, endocrinological, and metabolic effects of zearalenone exposure and additive effect of heat stress in prepubertal female pigs. J Therm Biol. (2024) 119:103742. doi: 10.1016/j.jtherbio.2023.103742
19. Wu, F, Gao, L, Li, F, Cui, J, Yang, X, Liu, Y, et al. Effects of zearalenone on ovarian development of prepubertal gilts through growth hormone axis. Front Vet Sci. (2022) 9:950063. doi: 10.3389/fvets.2022.950063
20. Buranatragool, K, Poapolathep, S, Isariyodom, S, Imsilp, K, Klangkaew, N, and Poapolathep, A. Dispositions and tissue residue of zearalenone and its metabolites α-zearalenol and β-zearalenol in broilers. Toxicol Rep. (2015) 2:351–6. doi: 10.1016/j.toxrep.2014.12.011
21. Biehl, ML, Prelusky, DB, Koritz, GD, Hartin, KE, Buck, WB, and Trenholm, HL. Biliary excretion and enterohepatic cycling of zearalenone in immature pigs. Toxicol Appl Pharmacol. (1993) 121:152–9. doi: 10.1006/taap.1993.1140
22. Zhang, Q, Huang, L, Leng, B, Li, Y, Jiao, N, Jiang, S, et al. Zearalenone affect the intestinal villi associated with the distribution and the expression of ghrelin and proliferating cell nuclear antigen in weaned gilts. Toxins. (2021) 13:736. doi: 10.3390/toxins13100736
23. Wu, J, Li, J, Wu, Y, Yang, M, Chen, Y, Wang, N, et al. Betulinic acid mitigates zearalenone-induced liver injury by ERS/MAPK/Nrf2 signaling pathways in mice. Food Chem Toxicol. (2023) 177:113811. doi: 10.1016/j.fct.2023.113811
24. Xia, S, Yan, C, Gu, J, Yuan, Y, Zou, H, Liu, Z, et al. Resveratrol alleviates zearalenone-induced intestinal dysfunction in mice through the NF-κB/Nrf2/HO-1 signalling pathway. Foods. (2024) 13:1217. doi: 10.3390/foods13081217
25. Reddy, KE, Song, J, Lee, HJ, Kim, M, Kim, DW, Jung, HJ, et al. Effects of high levels of deoxynivalenol and zearalenone on growth performance, and hematological and immunological parameters in pigs. Toxins. (2018) 10:114. doi: 10.3390/toxins10030114
26. Peillod, C, Laborde, M, Travel, A, Mika, A, Bailly, JD, Cleva, D, et al. Toxic effects of fumonisins, deoxynivalenol and zearalenone alone and in combination in ducks fed the maximum EUTolerated level. Toxins. (2021) 13:152. doi: 10.3390/toxins13020152
27. Dai, M, Jiang, S, Yuan, X, Yang, W, Yang, Z, and Huang, L. Effects of zearalenone-diet on expression of ghrelin and PCNA genes in ovaries of post-weaning piglets. Anim Reprod Sci. (2016) 168:126–37. doi: 10.1016/j.anireprosci.2016.03.006
28. Yang, LJ, Zhou, M, Huang, LB, Yang, WR, Yang, ZB, Jiang, SZ, et al. Zearalenone-promoted follicle growth through modulation of Wnt-1/β-catenin signaling pathway and expression of estrogen receptor genes in ovaries of postweaning piglets. J Agric Food Chem. (2018) 66:7899–906. doi: 10.1021/acs.jafc.8b02101
29. Wu, J, Li, J, Liu, Y, Liao, X, Wu, D, Chen, Y, et al. Tannic acid repair of zearalenone-induced damage by regulating the death receptor and mitochondrial apoptosis signaling pathway in mice. Environ Pollut. (2021) 287:117557. doi: 10.1016/j.envpol.2021.117557
30. Kaprara, A, and Huhtaniemi, IT. The hypothalamus-pituitary-gonad axis: tales of mice and men. Metabolism. (2018) 86:3–17. doi: 10.1016/j.metabol.2017.11.018
31. Yang, R, Wang, YM, Zhang, L, Zhao, ZM, Zhao, J, and Peng, SQ. Prepubertal exposure to an oestrogenic mycotoxin zearalenone induces central precocious puberty in immature female rats through the mechanism of premature activation of hypothalamic kisspeptin-GPR54 signaling. Mol Cell Endocrinol. (2016) 437:62–74. doi: 10.1016/j.mce.2016.08.012
32. Kriszt, R, Winkler, Z, Polyák, Á, Kuti, D, Molnár, C, Hrabovszky, E, et al. Xenoestrogens ethinyl estradiol and zearalenone cause precocious puberty in female rats via central kisspeptin signaling. Endocrinology. (2015) 156:3996–4007. doi: 10.1210/en.2015-1330
33. Zheng, W, Feng, N, Wang, Y, Noll, L, Xu, S, Liu, X, et al. Effects of zearalenone and its derivatives on the synthesis and secretion of mammalian sex steroid hormones: a review. Food Chem Toxicol. (2019) 126:262–76. doi: 10.1016/j.fct.2019.02.031
34. Yuan, T, Li, J, Wang, Y, Li, M, Yang, A, Ren, C, et al. Effects of zearalenone on production performance, egg quality, ovarian function and gut microbiota of laying hens. Toxins. (2022) 14:653. doi: 10.3390/toxins14100653
35. Zheng, W, Pan, S, Wang, G, Wang, YJ, Liu, Q, Gu, J, et al. Zearalenone impairs the male reproductive system functions via inducing structural and functional alterations of sertoli cells. Environ Toxicol Pharmacol. (2016) 42:146–55. doi: 10.1016/j.etap.2016.01.013
36. Zheng, W, Wang, B, Li, X, Wang, T, Zou, H, Gu, J, et al. Zearalenone promotes cell proliferation or causes cell death. Toxins. (2018) 10:184. doi: 10.3390/toxins10050184
37. Tatay, E, Espín, S, García-Fernández, AJ, and Ruiz, MJ. Oxidative damage and disturbance of antioxidant capacity by zearalenone and its metabolites in human cells. Toxicol In Vitro. (2017) 45:334–9. doi: 10.1016/j.tiv.2017.04.026
38. Gill, R, Tsung, A, and Billiar, T. Linking oxidative stress to inflammation: toll-like receptors. Free Radic Biol Med. (2010) 48:1121–32. doi: 10.1016/j.freeradbiomed.2010.01.006
Keywords: zearalenone, meat rabbit, ovary, oxidative stress, inflammatory response, reproductive hormone
Citation: Wu F, Wang F, Tang Z, Yang X, Liu Y, Zhao M, Liu S, Han S and Chen B (2025) Zearalenone causes ovarian damage and abnormal estradiol secretion in meat rabbits by inducing oxidative stress and inflammatory responses. Front. Vet. Sci. 12:1566284. doi: 10.3389/fvets.2025.1566284
Edited by:
Jie Yu, Sichuan Agricultural University, ChinaReviewed by:
Neha Sharma, Lovely Professional University, IndiaPrecious Oyedokun, Ladoke Akintola University of Technology, Nigeria
Copyright © 2025 Wu, Wang, Tang, Yang, Liu, Zhao, Liu, Han and Chen. This is an open-access article distributed under the terms of the Creative Commons Attribution License (CC BY). The use, distribution or reproduction in other forums is permitted, provided the original author(s) and the copyright owner(s) are credited and that the original publication in this journal is cited, in accordance with accepted academic practice. No use, distribution or reproduction is permitted which does not comply with these terms.
*Correspondence: Baojiang Chen, Y2hlbmJhb2ppYW5nQHZpcC5zaW5hLmNvbQ==
†These authors have contributed equally to this work