- 1Department of Zoology and Entomology, Faculty of Science, Helwan University, Cairo, Egypt
- 2Department of Biology, College of Science, Princess Nourah Bint Abdulrahman University, Riyadh, Saudi Arabia
- 3Al-Ayen Scientific Research Center, Al-Ayen Iraqi University, AUIQ, Nasiriyah, Iraq
Introduction: Monosodium glutamate (MG) is a substance often used to enhance food flavor, but its effect on the reproductive system is known to have a negative impact. This study assessed the protective effects of rutin (RUT) and Moringa oleifera leaf extract (MOLE) on testicular toxicity induced by MG in rats.
Methods: There were six groups: Control, RUT, MOLE, MG, RUT + MG, and MOLE + MG. The critical parameters measured were testicular index, hormone levels, antioxidants, oxidative stress markers, inflammation, apoptosis and histopathological changes.
Results: Following MG exposure (60 mg/kg/day for 30 days), the testicular index and serum testosterone, LH, and FSH levels were significantly reduced. The markers of oxidative stress increased, whereas the antioxidants decreased. The levels of inflammatory and apoptotic markers increased. The increased expression of inflammatory and apoptotic markers and significant testicular tissue damage, including degenerative changes in the seminiferous tubules, infiltration of inflammatory cells, and deposition of collagen fibers were investigated in addition to an increase in inflammatory and apoptotic markers.
Discussion: The present study showed that pre-administration of RUT or MOLE ameliorated the deleterious effects of MG, possibly due to antioxidant and anti-inflammatory properties, indicating a protective effect of RUT and MOLE on MG-induced testicular toxicity.
1 Introduction
Monosodium glutamate (MG), often known as sodium glutamate, is the sodium salt of L-glutamic acid, an amino acid (1, 2). MG comprises 78% glutamic acid, 22% sodium, and water (1). MG is a common culinary flavoring agent (3). It is often used to increase the umami flavor in the food business, particularly in the creation of processed and packaged goods as well as in restaurant meals and snacks. MG increases the overall flavor profile of foods by stimulating taste receptors on the tongue (4). The MG market was valued at USD 4.95 billion in 2022 and is projected to see over 5.7% Compound Annual Growth Rate (CAGR) between 2023 and 2032. Driven by the expanding customer preferences for flavor enhancers in the food and beverage industries (5).
The recommended quantity of added glutamate to increase food flavor should be between 0.1 and 0.8% of the product’s weight, akin to the natural L-glutamate concentration in tomatoes or Parmesan cheese. Excessive glutamate levels might severely impact flavor (6). Given the food industry’s high need for this additive, MG has been related to many health problems because its administration might raise the frequency of pachytene stage cells in primary spermatocytes (4), produce oxidative stress (OS) (7), and create free radicals (8). Additionally, MG use has been connected to obesity (9), central nervous system problems (10), reproductive abnormalities (11), hepatic damage (12), and hypertension (13). The testis is the primary organ of reproduction and endocrinal activities in males. It is very susceptible to injury due to its highly proliferating nature (14). The mechanism of action of MG-induced damage to diverse organs such as the liver, brain, testis, and kidney is connected to the production of oxidative stress (15) and inflammatory response (16).
Rutin (RUT), or rutinoside or quercetin-3-O-rutin, is a prevalent flavonoid occurring extensively in many vegetables and fruits (17, 18). This glycoside flavonoid is prevalent in orange peels and tomatoes and is particularly prominent in sophora rice and buckwheat flowers (19, 20). Rutin has shown the power to scavenge free radicals and alter critical signaling pathways such as Nrf2, MAPK, and NF-κB, which are implicated in treating certain disorders (21). Studies have indicated that RUT exhibits diverse pharmacological properties, such as antibacterial, hepatoprotective, immunomodulatory, antioxidant, and estrogenic actions (22). Moreover, investigations have demonstrated that RUT mitigates cisplatin-induced kidney damage and cell death in Wistar rats by reducing tumor necrosis factor-alpha (TNF-α), nuclear factor-kappa B (NF-κB), and caspase-3 mRNA expression levels (23). Kandemir et al. (24) discovered that RUT alleviates apoptosis and oxidative stress, lowering gentamicin-induced nephrotoxicity. This protective effect was coupled with activating antioxidant enzymes, mainly superoxide dismutase and glutathione peroxidase, and decreased lipid peroxidation levels in the testicular tissues (25).
Moringa oleifera (MO) is a fast-growing and drought-tolerant tropical plant. It is a member of the Moringaceae family. It has many names, including miracle, horseradish, benzalum, and drumstick trees (26). It grows in all tropical and subtropical areas such as Pakistan, Arabia, the sub-Himalayan regions of India, Central America, and the northern and southern Philippines (27). It is famous for increasing its biologically active compounds, principally in its leaves, which are broadly important for their nutritional and medicinal advantages (28, 29). The leaves contain vitamins, polyphenols, phenolic acids, flavonoids, alkaloids, carotenoids, glucosinolates, isothiocyanates, saponins, and tannins (30). These combinations have proved effective as antioxidants, anti-carcinogenic, and antimicrobial agents (19). MO leaves additionally provide a safeguard against oxidative stress (20), inflammation (31), bacterial action (32), fibrosis of the liver (33), damage of the liver (34), and hypercholesterolemia (35).
In vivo and in vitro research has been done to study the effects of MO leaves on the male reproductive system (36, 37). These findings emphasize that MO can help decrease numerous risk factors connected with male infertility. MO leaves, abundant in antioxidants and cytoprotective natural compounds, present it as a viable future technique in alleviating anomalies connected to cellular peroxidative damage and apoptosis (38). The amelioration found in testicular abnormalities, poor spermatogenesis, disturbances in the reproductive system, redox imbalances, and increased cell death (39, 40) post-supplementation with MO extract emphasizes their potential in addressing reproductive problems.
This study aimed to investigate the potential protective effects of RUT and MO leaf extract on the gonads of male rats exposed to MG and to explore the mechanisms underlying these effects.
2 Materials and methods
2.1 Chemicals
Monosodium glutamate with 99% purity was acquired online from Morgan Chemical Industry Company in Egypt, while rutin powder (non-citrus source; Sophora japonica; Flower Bud) was obtained from Sigma Company in Egypt.
2.2 Collection and extraction of plant
Fresh leaves of Moringa oleifera were collected from Helwan, Cairo, Egypt, during the summer, then identified and authenticated at the National Research Center in Giza. After cleaning, the leaves were air-dried and ground into powder. An aqueous extract was prepared by mixing 40 g of the dry powder with 100 milliliters (mL) of heated water for 1 h, stirring frequently. The mixture was then filtered using Whatman No. 1 paper at 55°C, and the filtrate was concentrated to 8% of its original volume using an RE-2010 rotary evaporator (BIOBASE, China) (41).
2.3 Phytochemical analysis of MOLE
Using an Interspec 200-X FTIR spectrophotometer (Spectronic Camspec Ltd., United Kingdom), MOLE was subjected to Fourier transform infrared (FTIR) spectroscopic analysis. The transmittance was scanned in the mid-IR region, ranging from 4,000 to 400 cm−1.
2.4 Animals and experimental protocol
Mature male Wistar albino rats (120–150 g, 8 weeks old) were obtained from the Medical Ain-Shams Research Institute in Cairo, Egypt. The experiment adhered to the Ain-Shams Research Institute Animal Facility guidelines under veterinary supervision. The protocol was conducted following the ethical research guidelines of Ain-Shams University, with the Experimental Animal Research Unit code number [RE (189)22]. The rats were housed under standard conditions, with a 12-h light/dark cycle, a temperature of 24 ± 2°C, and humidity of 50 ± 10%. They were allowed to acclimate for 1 week before the experiment began.
To investigate the protective effects of rutin and Moringa oleifera leaf extract on monosodium glutamate-induced reproductive toxicity, 60 rats were divided into six equal groups (n = 10 each) as follows:
Group 1—Control (CONT) group: Rats received no treatment.
Group 2—Rutin (RUT) group: Rats were given a daily dose of 150 mg/kg of rutin for 30 days (23).
Group 3—Moringa oleifera leaf extract (MOLE) group: Rats received a daily dose of 500 mg/kg of Moringa oleifera leaf extract for 30 days (42).
Group 4—Monosodium glutamate (MG) group: Rats were administered an oral daily dose of 60 mg/kg of monosodium glutamate for 30 days (43). The lethal dose 50 (LD50) of MG in Wistar rats has been stated to be 500 mg/kg body weight, according to the study described by Airaodion et al. (44). The selected dose of our study (60 mg/kg body weight) is significantly lower than the reported LD50, representing only 12% of the lethal dose. This indicates that the chosen dose is within a non-lethal range.
Group 5 and 6—RUT + MG and MOLE + MG groups: Rats received rutin and Moringa oleifera leaf extract, respectively, at the same doses as their groups, followed by MG at the same dose 1 h later for 30 days.
2.5 Sample collection
At the end of the experiment, the rats were anesthetized for euthanasia by 1.9% inhaled diethyl ether (0.08 mL/liter of container volume) (45). They were exposed to ether for approximately 2 min in a transparent acrylic jar. After anesthesia, blood samples were collected from the retro-orbital sinus using a microhematocrit capillary tube, draining 2 mL of blood. The collected blood was placed in sterile labeled tubes (Serum Separator Clot Activator with Gel) and centrifuged (3,000 rpm/15 min.) to obtain serum, which was then stored at −20°C for the determination of testosterone, LH, and FSH levels. Testes from each group were carefully dissected, weighed, and divided into two sections. One section was fixed for 24 h. at room temperature in 10% neutral buffered formalin for histological, histochemical, and immunohistochemical investigations. The other section was stored at −80°C until ground and homogenized with cold 50 mM Tris–HCl buffer (pH 7.4). The homogenate (10% w/v) was then centrifuged at 3,000 × g for 10 min at 4°C to obtain the supernatant. This supernatant was kept at −20°C for the biochemical evaluation of oxidative stress, antioxidant, inflammatory, and apoptotic biomarkers.
2.6 Testicular index
The final body and testicular weights were measured with a precise weighing balance (Radwag, Model AS220/C/2, Clarkson Laboratory and Supply Inc., Chula Vista, CA, United States). The relative testicular weight was then calculated using the following formula:
2.7 Hormonal analyses
The levels of serum testosterone, luteinizing hormone (LH), and follicle-stimulating hormone (FSH) were quantitatively measured using the ELISA technique. Rat-specific kits purchased from BioVendor (Gunma, Japan) were used following the protocol provided with each kit.
2.8 Oxidative stress markers in the testicular tissue
Using testicular homogenate, the lipid peroxide (LPO) level was estimated by measuring the concentration of malondialdehyde (MDA), an end product of lipid peroxidation, according to the method described by Ohkawa et al. (46). The nitric oxide (NO) level was detected using the Griess reagent, following the method outlined by Green et al. (47). The reduced glutathione (GSH) concentration was determined using the method developed by Ellman (48).
2.9 Antioxidant status in the testicular tissue
The activity of testicular superoxide dismutase (SOD) was assessed using the standard method described by Nishikimi et al. (49). Testicular catalase (CAT) activity was measured following the technique outlined by Aebi (50). The activities of glutathione peroxidase (GPx) and glutathione reductase (GR) in testicular tissue were determined according to the methods described by Paglia and Valentine (51) and De Vega et al. (52), respectively.
2.10 Inflammatory marker assays
Testicular levels of the pro-inflammatory cytokines tumor necrosis factor-alpha (TNF-α) and interleukin-1 beta (IL-1β) were measured using ELISA kits obtained from R&D Systems and Thermo Fisher Scientific, respectively, following the manufacturer’s protocols.
2.11 Apoptotic marker assays
The levels of Bcl-2, Bax, and caspase-3 proteins in testicular tissues were assessed using commercial ELISA kits obtained from BioVision, Inc. (Bcl-2: Cusabio, Catalog # CSB-E08854r; Bax: BioVision, Inc., Catalog # E4513; caspase-3: Sigma-Aldrich, Catalog # CASP3C-1KT), following the manufacturer’s instructions.
2.12 Histopathological examinations
The fixed testes were prepared for paraffin sectioning, which included dehydration, clearing, infiltration, and embedding in paraffin wax. Sections with a thickness of 5 microns were cut using a rotary microtome, stained for histological, histochemical, and immunohistochemical investigations, examined under a light microscope (LEICA DM4P, Wetzlar, Germany), and photographed using a Zeiss camera.
2.13 Histological and histochemical results
For the general histopathological study of the tissue, we used Hematoxylin and Eosin (H&E) stain (53), and Masson’s Trichrome stain for demonstration of the tissue collagen fibers (54). Periodic acid-Schiff (PAS) histochemical technique was utilized to detect polysaccharides in tissue (55).
2.14 Immunohistochemical results
The proliferating cell nuclear antigen (PCNA) acts as a marker for cell proliferation (56), while caspase-3 indicates apoptosis (57), and cyclooxygenase-2 (COX-2) is primarily linked with inflammation (58). Sections of testicular tissue from each experimental group were subjected to staining using anti-PCNA, anti-Caspase-3, and anti-COX-2 antibodies. The avidin-biotin-peroxidase technique detected PCNA, caspase-3, and COX-2 immunoreactivities in the testis (59).
2.15 Morphometric analysis
Histomorphometric analysis determined the mean area percentage of collagen fibers, polysaccharides, PCNA, Caspase-3, and COX-2 positive immunoreactions. This measurement was taken in 10 non-overlapping high-power fields (40×) of paraffin sections of the testis for each group, utilizing ImageJ software (version 1.46, NIH, United States) and subsequently analyzed statistically.
2.16 Statistical analysis
Data from various evaluations were analyzed using one-way analysis of variance (ANOVA) and Tukey’s multiple comparisons test, utilizing GraphPad Prism software (version 8.00). A p-value of <0.05 was considered statistically significant. The analyzed data are presented as the mean ± SD.
3 Results
3.1 Phytochemical analysis of MOLE
The Fourier Transform Infrared (FTIR) spectrum of the crude aqueous MOLE represented in Figure 1 provided information on the functional groups present in the sample. A detailed analysis of the peaks in the spectrum:
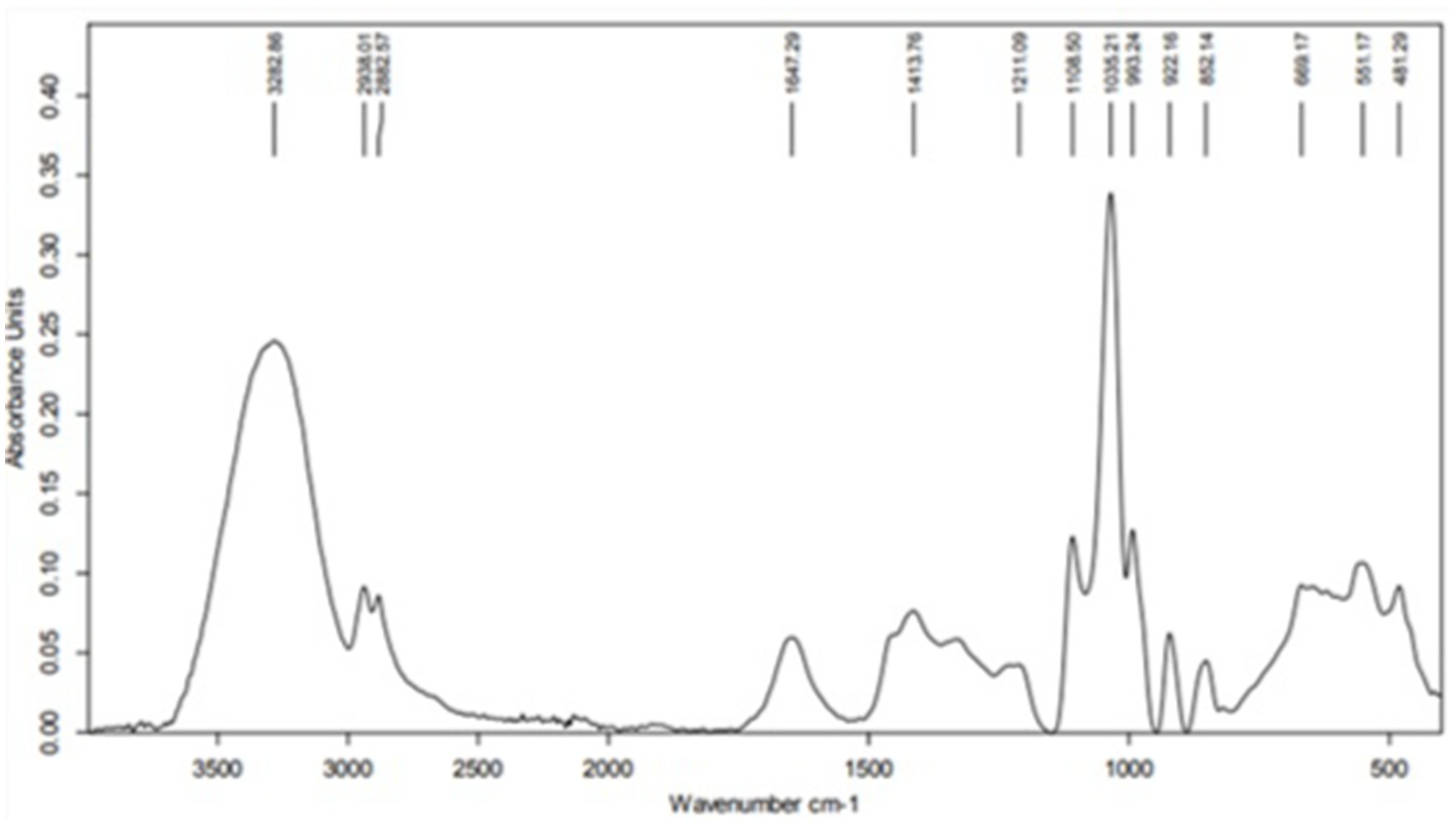
Figure 1. Fourier-transform infrared spectroscopy spectrum of the crude aqueous Moringa oleifera leaf extract.
3.1.1 High wavenumber region (4,000–2,500 cm−1)
1. 3282.86 cm−1: This broad peak is characteristic of O-H stretching vibrations, commonly associated with hydroxyl groups in alcohols, phenols, and water. The broad nature of the peak indicates hydrogen bonding, typical of water or phenolic compounds.
2. 2923.01 cm−1 and 2852.57 cm−1: These peaks correspond to the asymmetric and symmetric stretching vibrations of C-H bonds in alkanes, indicating the presence of methylene (-CH₂) groups, which are typical in fatty acids and lipids.
3.1.2 Mid wavenumber region (2,500–1,500 cm−1)
1. 31647.29 cm−1: This peak likely represents the C=O stretching vibration, typically found in carbonyl groups such as ketones, aldehydes, carboxylic acids, or esters. It could also indicate the presence of conjugated alkenes or amides.
2. 41413.76 cm−1: This peak is indicative of C-H bending (scissoring) vibrations, which are characteristic of alkanes.
3. 51211.09 cm−1: This peak could be associated with C-O stretching vibrations, often seen in ethers, alcohols, and esters.
3.1.3 Low wavenumber region (1,500–500 cm−1)
1. 1108.50 cm−1 and 1033.21 cm−1: These peaks are associated with C-O stretching vibrations, typical of alcohols, esters, or carboxylic acids. They may also indicate the presence of polysaccharides or glycosidic linkages.
2. 922.16 cm−1, 852.14 cm−1: These peaks likely represent out-of-plane bending vibrations of = C-H bonds, indicating the presence of alkenes or aromatic compounds.
3. 668.17 cm−1 and 555.17 cm−1: These peaks correspond to bending vibrations of functional groups, which could be associated with alkyl halides or other substituents.
4. 481.29 cm−1: This low-frequency peak might correspond to skeletal vibrations or bending vibrations in larger molecular structures, possibly indicating inorganic compounds or metal-oxygen bonds.
3.2 Testicular index
The effect of MG on final body weights absolute and relative testicular weights was represented in Figure 2. Compared with the standard control group, no marked differences were found between the final body weights of all groups. The administration of MG to rats caused a slight decrease in absolute testicular weight and a significant decrease in relative testicular weight compared to the CONT group. Pre-administration of RUT or MOLE to MG did not change the absolute and relative testes weight compared to the control group.
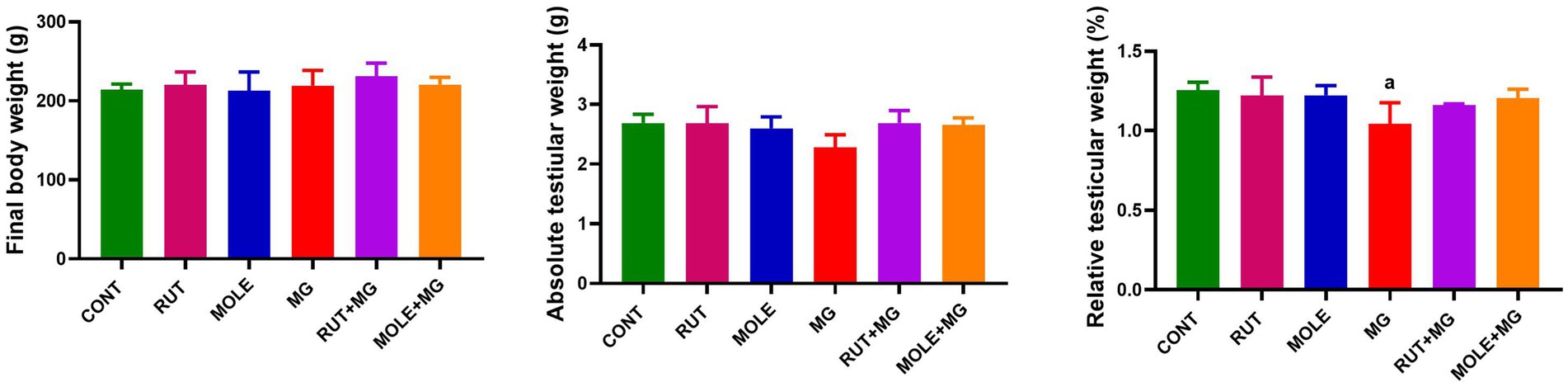
Figure 2. Effects of rutin (RUT) and Moringa oleifera leaf extract (MOLE) pre-administration on final body weight and absolute and relative testicular weight in rats treated with monosodium glutamate (MG). All values are stated as mean ± SD (n = 10). aPoints to a significant change vs. the control (CONT) group at p < 0.05, using Tukey’s multiple comparisons test.
3.3 Hormonal analyses
The administration of MG reduced serum testosterone, LH, and FSH levels compared to the CONT group. The pre-administration of RUT or MOLE to MG increased testosterone, LH, and FSH levels compared to MG, indicating a protective effect of RUT and MOLE against MG-induced reduction in testosterone, LH, and FSH (Figure 3).
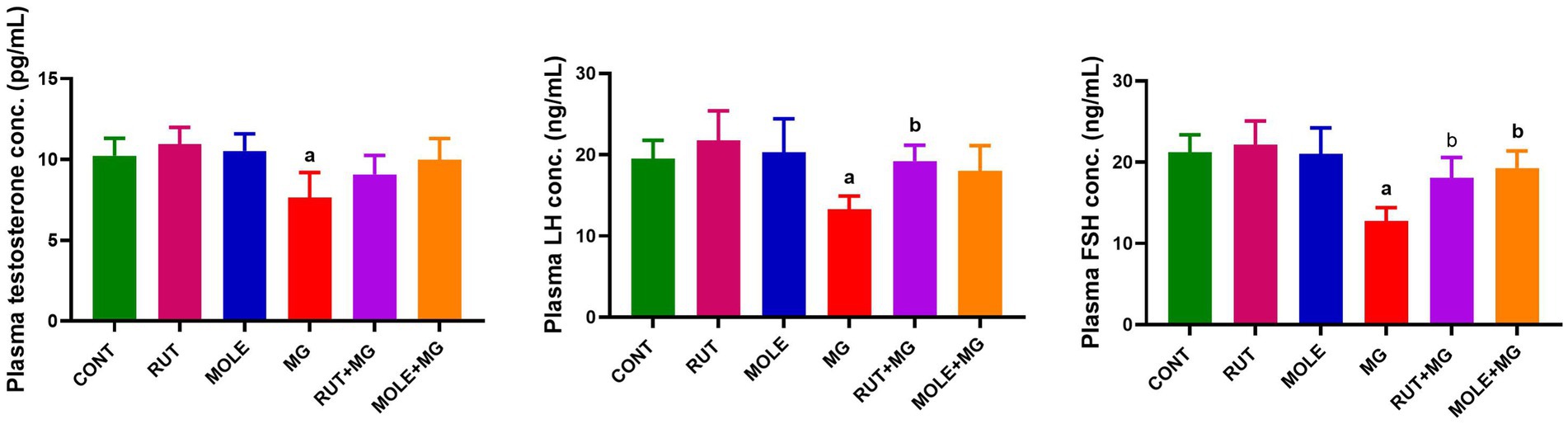
Figure 3. Effects of RUT and MOLE pre-administration on serological testosterone, luteinizing hormone (LH), and follicle-stimulating hormone (FSH) levels in rats exposed to MG. All values are stated as mean ± SD (n = 10). aPoints to a significant change vs. the CONT group at p < 0.05; bpoints to a significant change vs. the MG group at p < 0.05, using Tukey’s multiple comparisons test.
3.4 Oxidative stress markers in the testicular tissue
Administration of MG led to a significant increase in lipid peroxidation, as evidenced by elevated MDA levels and higher NO levels while causing a decrease in GSH levels in the testicular tissues compared to the CONT group. The pre-administration of RUT or MOLE significantly mitigated the oxidative stress markers’ levels in the testes of rats treated with MG. Both RUT and MOLE reduced MDA and nitric oxide levels while increasing GSH levels compared to the MG group, indicating their protective effects against oxidative stress induced by MG treatment. However, the levels of these markers ultimately did not return to the baseline levels observed in the CONT group (Figure 4).
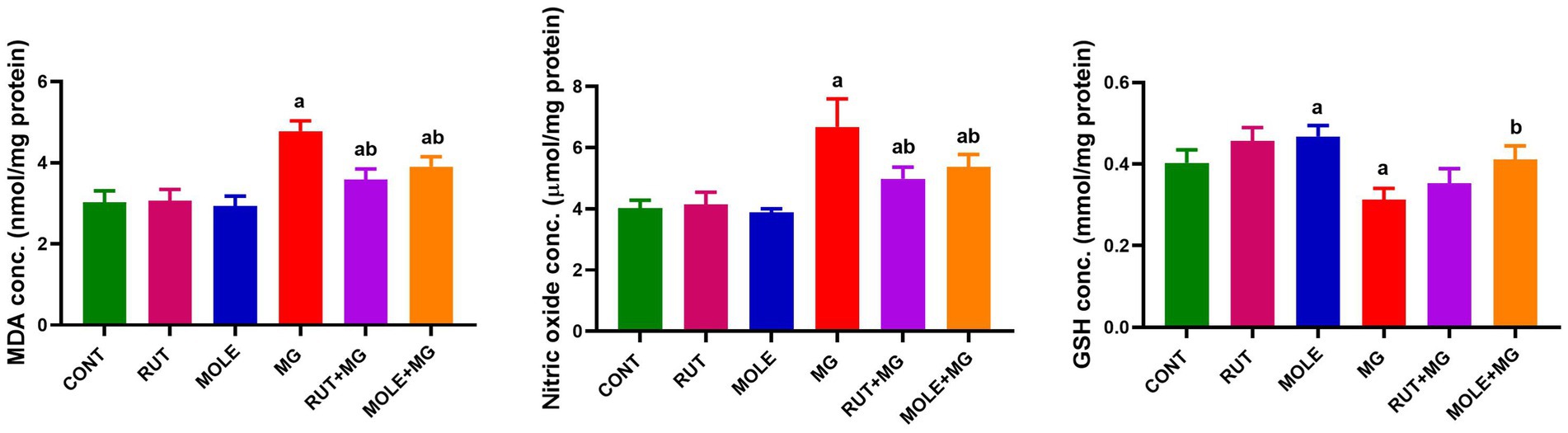
Figure 4. Effects of RUT and MOLE pre-administration on malondialdehyde (MDA), nitric oxide, and reduced glutathione (GSH) levels in the testes of rats treated with MG. Values of oxidative stress markers’ levels are stated as mean ± SD (n = 10). aPoints to a significant change vs. the CONT group at p < 0.05; bpoints to a significant change vs. the MG group at p < 0.05, using Tukey’s multiple comparisons tests.
3.5 Antioxidant status in the testicular tissue
In MG-treated rats, the activity of all antioxidant enzymes (SOD, CAT, GPx, and GR) was significantly reduced when compared to the control group at p < 0.05. However, the SOD activity remains slightly decreased in the RUT + MG group compared to the control group; the pre-administration of RUT or MOLE to MG significantly improved the activities of SOD, CAT, GPx, and GR in the testes of rats treated with MG, counteracting the adverse effects of MG consumption (Figure 5).
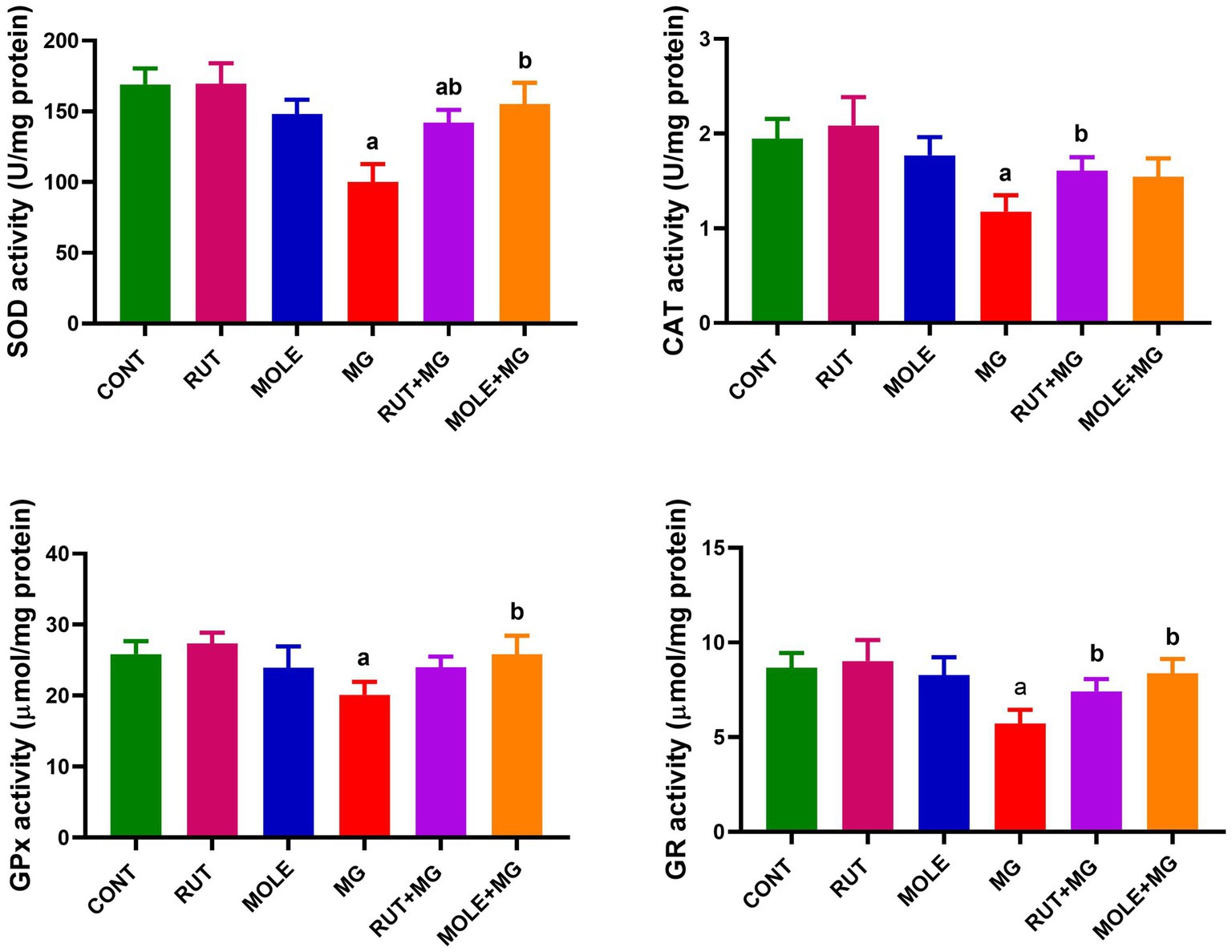
Figure 5. Effects of RUT and MOLE pre-administration on the levels of superoxide dismutase (SOD), catalase (CAT), glutathione peroxidase (GPx), glutathione reductase (GR) in the testes of rats treated with MG. Values of antioxidant enzyme activities are expressed as the mean ± SD (n = 10). aPoints to a significant change vs. the CONT group at p < 0.05; bpoints to a significant change vs. the MG group at p < 0.05, using Tukey’s multiple comparisons test.
3.6 Inflammatory marker assays
In MG-treated rats, the levels of inflammatory markers (TNF-α and IL-1β) were significantly increased compared to the control group at p < 0.05. The pre-administration of RUT or MOLE significantly reduced TNF-α and IL-1β levels in the testes of rats treated with MG. These findings indicated that both RUT and MOLE had protective effects against inflammation induced by MG treatment. Pre-administration of these compounds to MG mitigated the elevation of TNF-α and IL-1β caused by MG, but the levels remained slightly elevated compared to the control group (Figure 6).
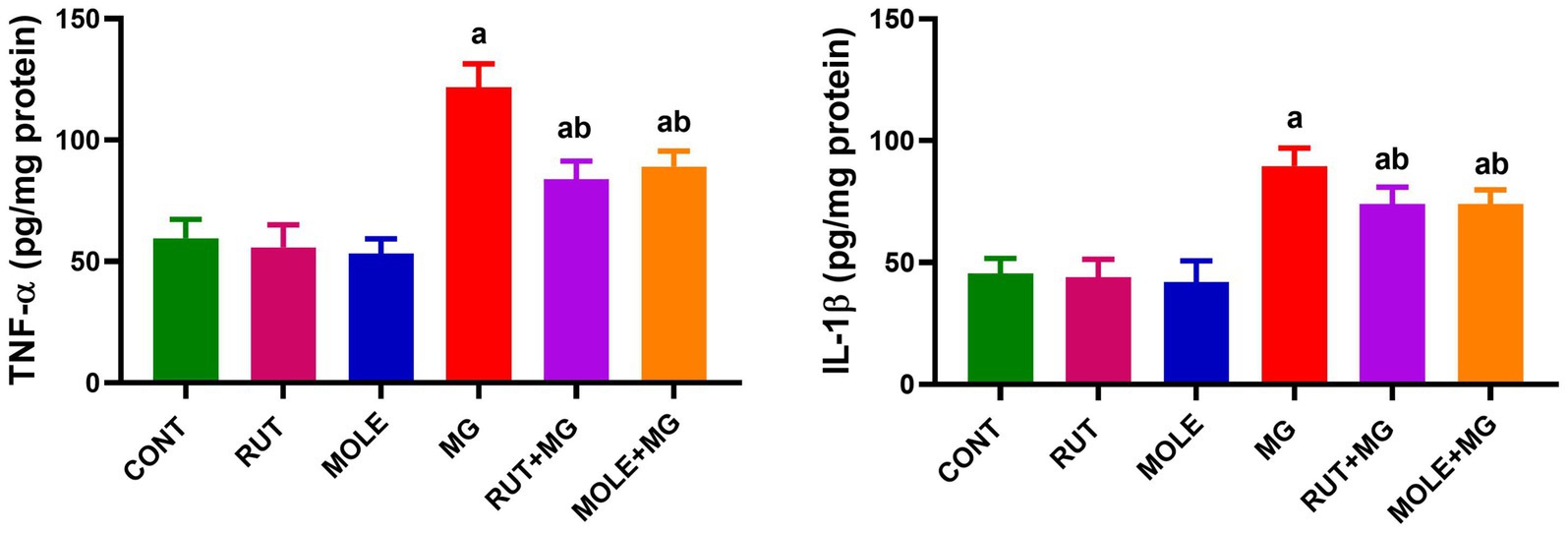
Figure 6. Effects of RUT and MOLE pre-administration on the levels of tumor necrosis factor-α (TNF-α) and interleukin-1β (IL-1β) in the testes of rats treated with MG. All values are stated as mean ± SD (n = 10). aPoints to a significant change vs. the CONT group at p < 0.05; bpoints to a significant change vs. the MG group at p < 0.05, using Tukey’s multiple comparisons test.
3.7 Apoptotic marker assays
MG treatment increased apoptotic protein levels (Bax and caspase-3) and decreased anti-apoptotic protein levels (Bcl-2) in the testes of rats. However, the caspase-3 level remained slightly elevated in the RUT + MG group compared to the control group; the pre-administration of RUT or MOLE to MG counteracted the effects of MG, resulting in increased levels of Bcl-2 and decreased levels of Bax and caspase-3, suggesting a protective effect against MG-induced apoptosis (Figure 7).
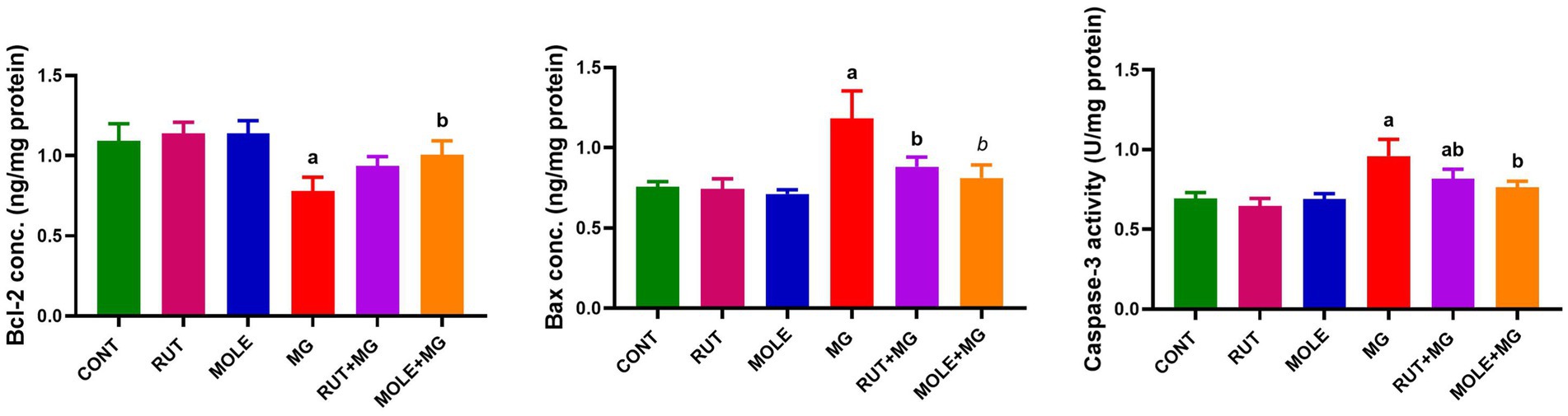
Figure 7. Effects of RUT and MOLE pre-administration on the levels of apoptotic proteins (Bcl-2, Bax and caspases-3) in the testes of rats treated with MG. All values are stated as mean ± SD (n = 10). aPoints to a significant change vs. the CONT group at p < 0.05; bpoints to a significant change vs. the MG group at p < 0.05, using Tukey’s multiple comparisons test.
3.8 Histological and histochemical results
3.8.1 Hematoxylin and eosin staining
Light microscopy examination of the H&E-stained sections of testes from the CONT group (Figure 8a) revealed the typical histo-architecture of the testis. The seminiferous tubules were separated by interstitial tissue containing blood vessels and clusters of Leydig cells, which exhibited pale acidophilic cytoplasm. A basement membrane and flat peritubular myoid cells bounded each tubule. These myoid cells resemble smooth muscle cells and are classified as myoepithelial cells. The seminiferous tubules were lined by stratified germinal epithelium consisting of spermatogenic cells and supportive Sertoli cells. Both cell types rested on a prominent basal lamina.
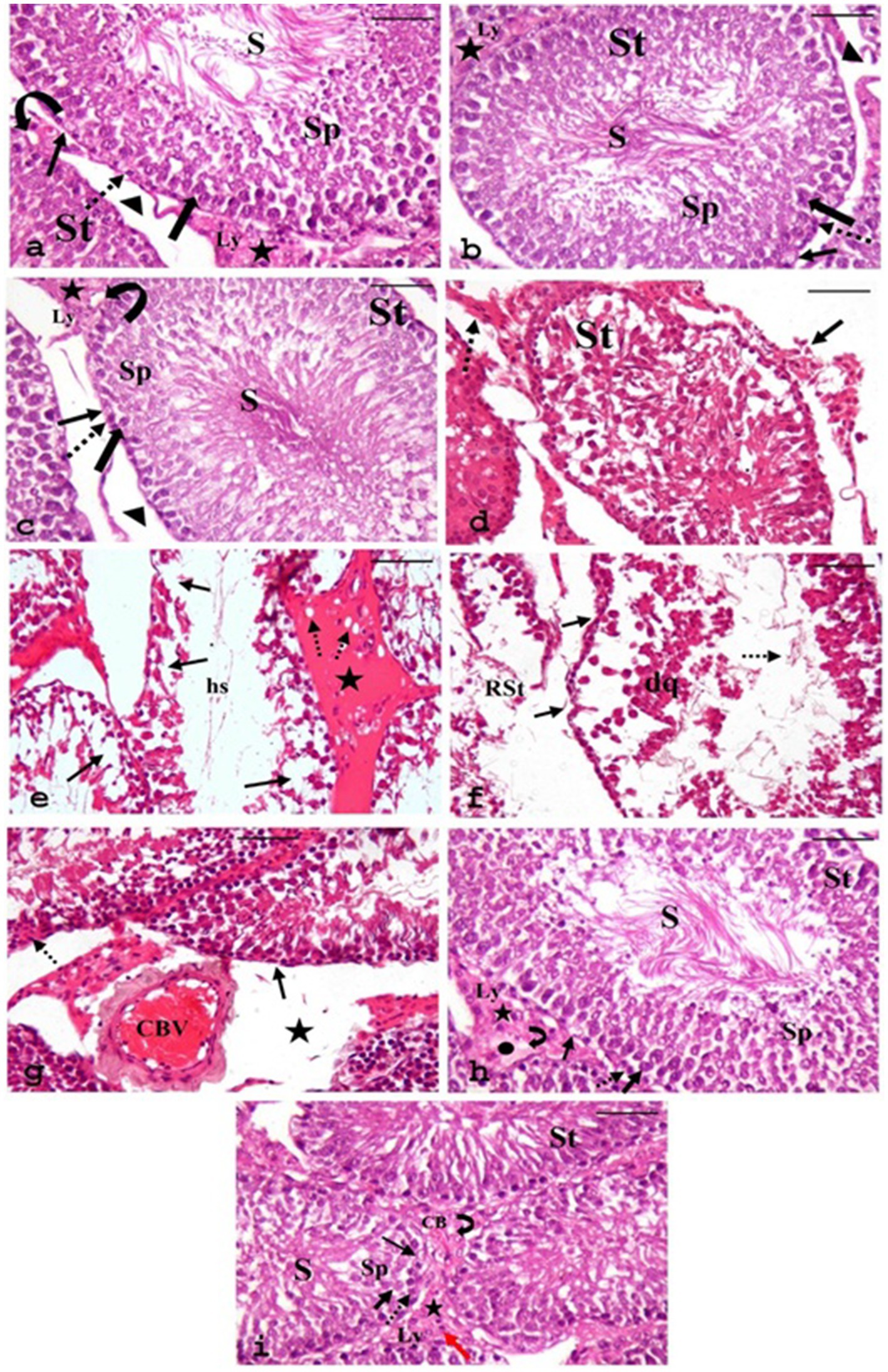
Figure 8. Photomicrographs of H&E-stained sections of testes from various groups. (a) CONT, (b) RUT, and (c) MOLE groups display seminiferous tubules (ST) surrounded by a basement membrane and myoid cells (arrowhead); interstitial tissue (asterisks) with blood vessels (curved arrow) and in between, clusters of Leydig cells (Ly) are seen. Each tubule is lined with Sertoli cells (thin arrow) and spermatogenic cells: spermatogonia (dashed arrow), primary spermatocytes (thick arrow), spermatids (SP), and numerous spermatozoa (S) filling the lumen. Panels (d–g) depict the MG group: panel (d) shows irregular organization of germinal epithelium in the seminiferous tubule (St) with discrete interstitial tissue (arrow) and inflammatory infiltration in the interstitium (dashed arrow). Panel (e) reveals distorted seminiferous tubules with focal areas of spermatogenic cell loss (arrow), wide lumina without sperm, filled with acidophilic hyaline streaks (hs), and widened, vacuolated interstitium (dashed arrow) containing acidophilic hyaline material (star). Panel (f) demonstrates a ruptured seminiferous tubule (RST). Another tubule shows irregular basement membranes (arrow), detachment of spermatogenic cells from the basement membrane with desquamated cells (dq), and a few sperms (dashed arrow) within the tubular lumen. Panel (g) indicates congested blood vessels (CBV) in the interstitial tissue and the widening of interstitial spaces (star). Pyknosis (dashed arrow) and reduction in the height of the germinal epithelium (arrow) are evident. (h,i) RUT + MG and MOLE + MG groups show seminiferous tubules with active spermatogenesis and numerous sperm (S) in the lumen. The interstitial tissue (asterisks) appears nearly normal, with vacuolation (curved arrow) and edema (circle) in the RUT + MG group, while vacuolation (curved arrow), a few inflammatory cells (red arrow), and congestion of blood vessels (CB) are noted in the MOLE + MG group. Dashed arrow, Spermatogonia; thick arrow, Primary spermatocytes; SP, Spermatids; thin arrow, Sertoli cells; Ly, Leydig cells (scale bar = 50 μm).
Sertoli cells were elongated, with large, basal, rounded, and pale nuclei. The spermatogenic cells were arranged in several layers. Spermatogonia, the small, rounded cells, rested on the basal lamina. Primary spermatocytes, the most significant and numerous rounded cells, were located above the spermatogonia. Spermatids, the smallest spermatogenic cells, were arranged in several rows near the tubular lumen. Spermatozoa were observed in the lumen of the seminiferous tubules.
The RUT and MOLE groups also displayed normal testicular histo-architecture (Figures 8b,c, respectively). In contrast, the sections from the MG group (Figures 8d–g) displayed numerous histological changes compared to the CONT group. The germinal epithelium of the seminiferous tubules exhibited irregular organization, with noticeable distortion of the seminiferous tubules and focal areas of spermatogenic cell loss. The lumina were expanded, devoid of sperm, and filled with acidophilic hyaline streaks. Some tubules exhibited irregular basement membranes and detachment of spermatogenic cells from the basement membrane, with desquamated cells and few sperm within the tubular lumen. Rupture of some seminiferous tubules was also observed. Pyknosis and a reduction in the height of the germinal epithelium of the seminiferous tubules were noted. The interstitium appeared widened, vacuolated, and filled with acidophilic hyaline material; leukocytic infiltration and congested blood vessels were also seen.
Compared to the MG group, sections from the RUT + MG group (Figure 8h) and the MOLE + MG group (Figure 8i) displayed fewer pathological changes. Most tubules exhibited typical structures with active spermatogenesis, and the lumens were filled with mature spermatozoa. However, the interstitial tissue between seminiferous tubules showed vacuolation and edema in the RUT + MG group, while the MOLE + MG group exhibited vacuolation, a few inflammatory cells and congestion of blood vessels.
3.8.2 Masson’s trichrome staining
Masson’s trichrome stained sections of testes demonstrated that the collagen fibers appeared blue, the CONT group (Figure 9a), RUT group (Figure 9b), and MOLE group (Figure 9c) showed that the tunica albuginea capsule was formed of collagen fibers and exhibited no deposition of collagen fibers in the interstitial tissues or the basement membranes of the tubules. In the MG group (Figures 9d,e), collagen fibers were corrugated in the thickened capsule, along with evident deposition of collagen fibers in the interstitial tissues and the basal lamina of the seminiferous tubules. Sections from the RUT+MG group (Figure 9f) and MOLE+MG group (Figure 9g) showed a capsule appearance similar to the control group. The tubules’ interstitial tissue and basal lamina did not display collagenous fibers.
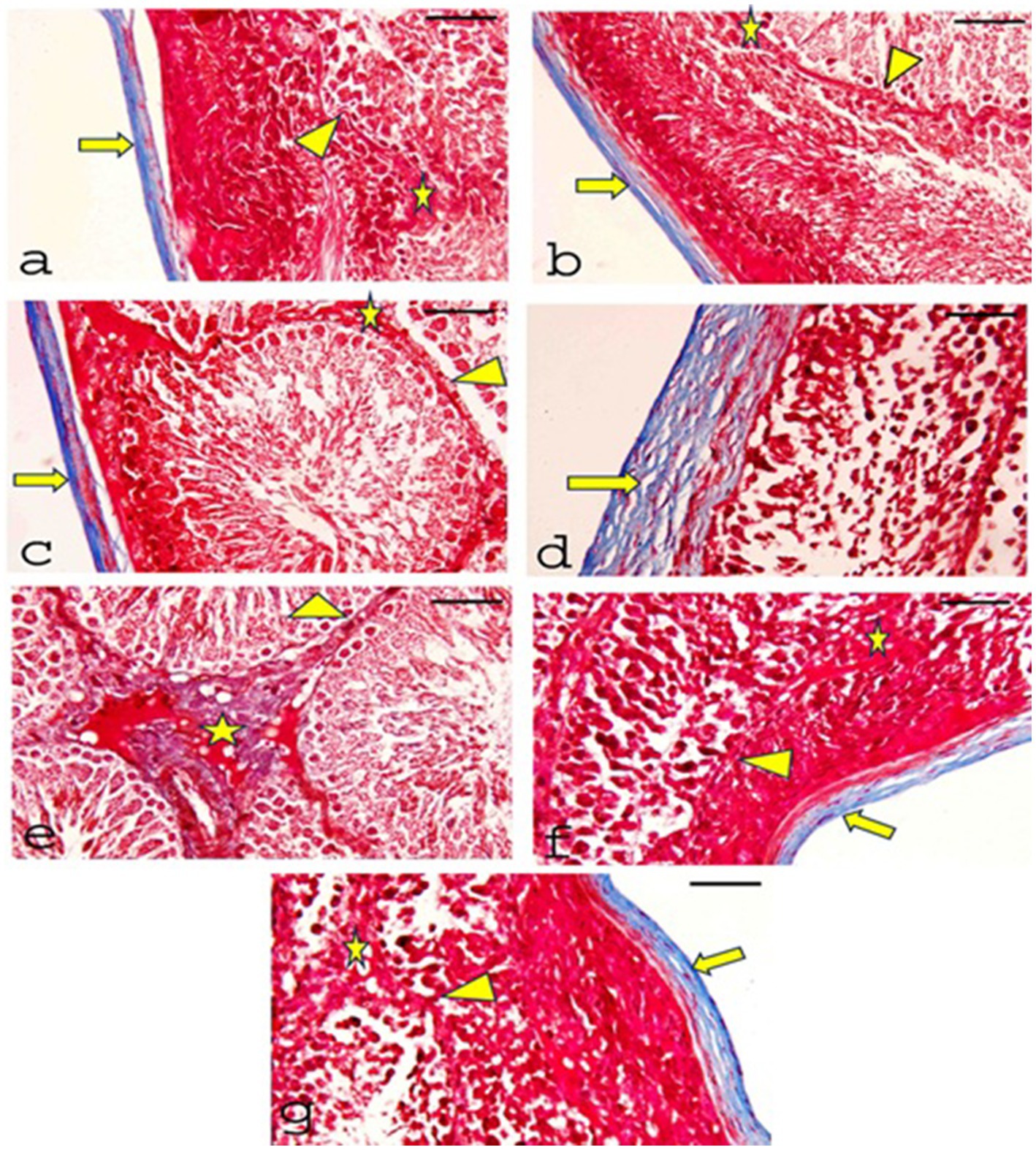
Figure 9. Photomicrographs of Masson’s Trichrome-stained sections of testes from all groups. (a) CONT, (b) RUT, and (c) MOLE groups show the tunica albuginea capsule formed of collagen fibers (blue) (arrow). There is no collagen fiber deposition in the interstitial tissues (asterisks) or the basement membranes of the tubules (arrowhead). (d) Moreover, (e) the MG group displays significant collagen fiber deposition in the capsule, which appears thickened and shows corrugation (arrow). Notable collagen fiber deposition is also observed in the interstitial tissue (asterisks) and basal lamina (arrowhead). (f) RUT + MG and (g) MOLE + MG groups exhibit a small number of collagen fibers in the capsule (arrow) but no collagen fibers in the interstitial tissue (asterisks) or the basal lamina of the seminiferous tubules (arrowhead) (scale bar = 50 μm).
3.8.3 Periodic acid Schiff reaction “PAS”
Sections of testes stained with PAS from the CONT group (Figure 10a), RUT group (Figure 10b), and MOLE group (Figure 10c) showed a strong PAS reaction in the basal lamina of tubules, interstitial tissues, and spermatogenic cells. The MG group (Figure 10d) exhibited a strong PAS reaction in the basal lamina, interstitial tissue, and spermatogenic cells. In contrast, sections from the RUT+MG (Figure 10e) and MOLE+MG (Figure 10f) groups displayed a reaction closely similar to that of the CONT group.
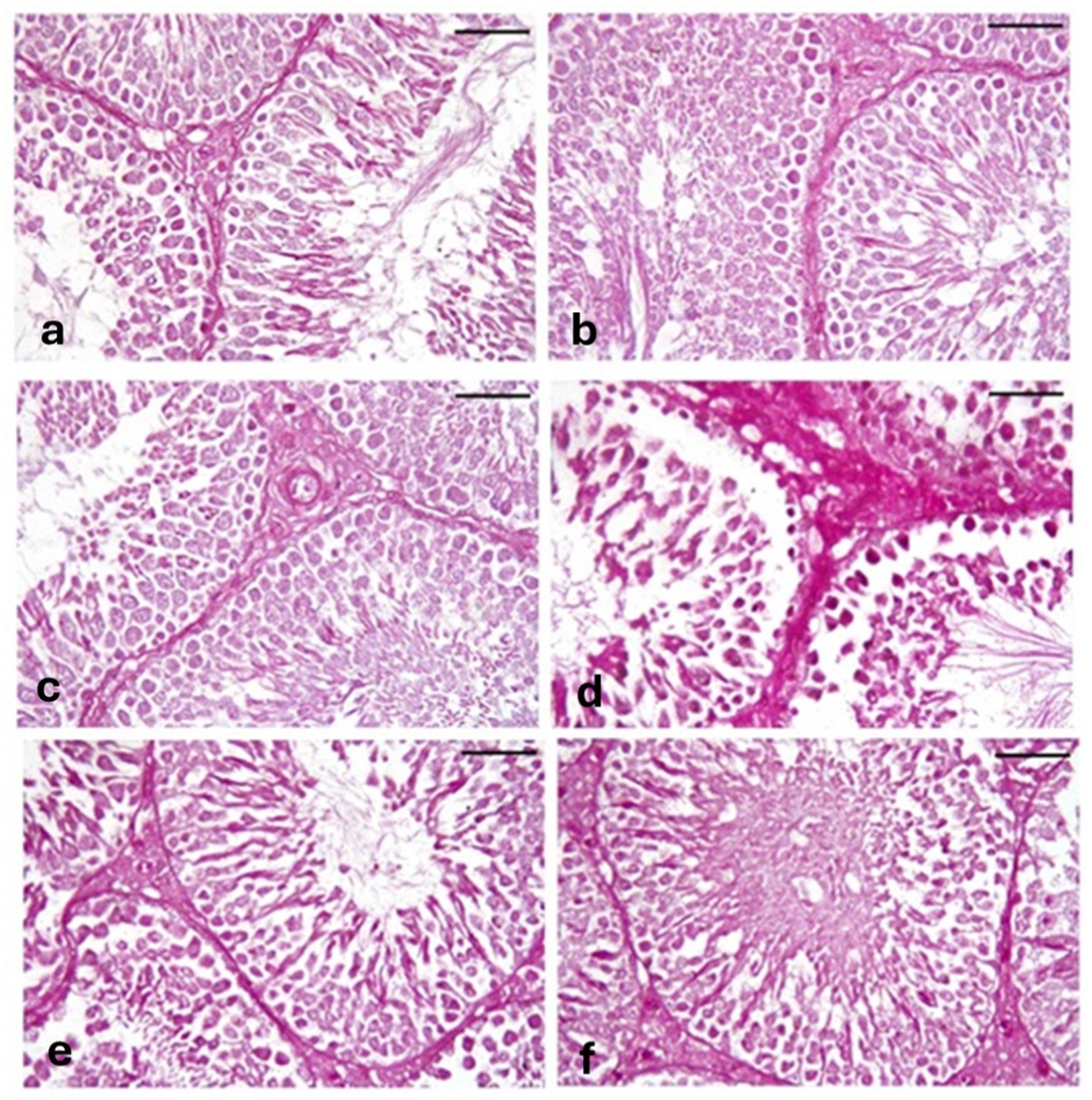
Figure 10. Photomicrographs of PAS-stained sections of testes across all groups. (a) CONT, (b) RUT, and (c) MOLE groups display a strong PAS reaction in the basal lamina of tubules, interstitial tissues, and spermatogenic cells. (d) The MG group exhibits an extreme PAS reaction in the basal lamina, interstitial tissues, and spermatogenic cells. (e) RUT + MG and (f) MOLE + MG groups show a reaction nearly similar to that of the control group (scale bar = 50 μm).
3.9 Immunohistochemical results
3.9.1 Immunohistochemical staining for proliferating cell nuclear antigen
A positive reaction for proliferating cell nuclear antigen (PCNA) is indicated by brown nuclear staining. The CONT (Figure 11a), RUT (Figure 11b), and MOLE (Figure 11c) groups exhibited positive PCNA immunoreactivity in all nuclei of the germinal cells. In contrast, sections from the MG group (Figure 11d) showed weak PCNA reactivity in most of the nuclei of the germinal cells, with only a few spermatogenic cells displaying positive PCNA immunoreactivity. Sections from the RUT+MG (Figure 11e) and MOLE+MG (Figure 11f) groups showed positive PCNA immunoreactivity in most nuclei of the germ cells, although a few nuclei displayed a weak reaction.
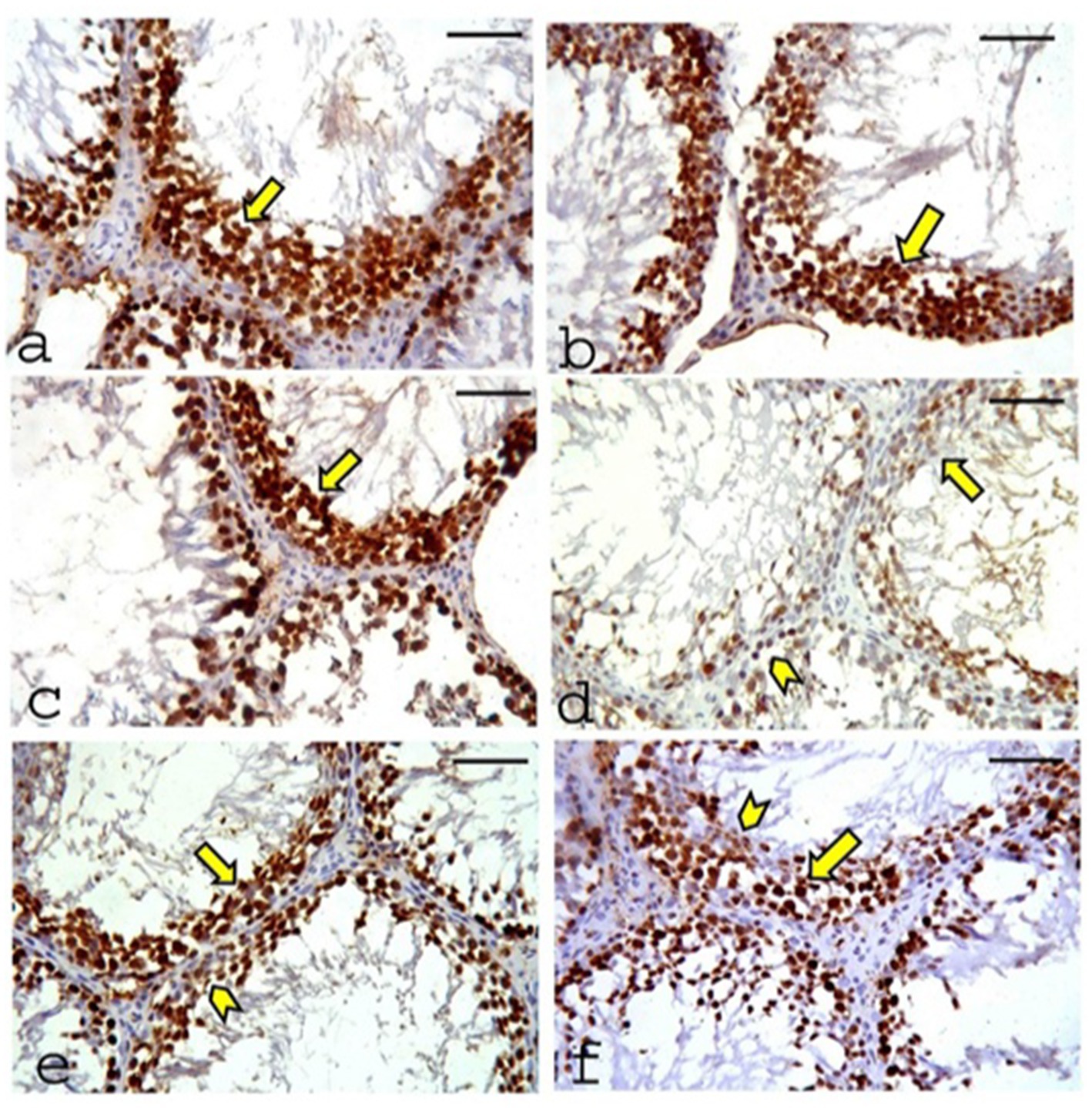
Figure 11. Photomicrographs of proliferating cell nuclear antigen (PCNA) expression in the testes of the studied groups. (a) CONT group, (b) RUT group, and (c) MOLE group show positive PCNA immunoreactivity (indicated by a deep brown reaction) in the nuclei of all germinal cells (arrow). (d) The MG group exhibits weak PCNA reactivity in most germinal cell nuclei (arrow), with only a few spermatogenic cells showing positive PCNA immunoreactivity (arrowhead). (e) RUT+MG and (f) MOLE+MG groups demonstrate positive PCNA immunoreactivity in most germ cell nuclei (arrow), although a few nuclei show a weak reaction (arrowhead) (scale bar = 50 μm).
3.9.2 Immunohistochemical staining for Cyclooxygenase-2
A positive reaction for Cyclooxygenase-2 (COX-2) is indicated by brown cytoplasmic or nuclear staining. Negative to weak cytoplasmic or nuclear COX-2 immunoreaction was observed in the germinal epithelia and interstitial Leydig cells of the CONT (Figure 12a), RUT (Figure 12b) and MOLE (Figure 12c) groups. In contrast, the MG group (Figure 12d) exhibited strong COX-2 immunoreactivity in the germinal epithelium and Leydig cells. The RUT + MG (Figure 12e) and MOLE + MG (Figure 12f) groups showed weak-to-moderate COX-2 immunoreaction in some seminiferous epithelia and Leydig cells.
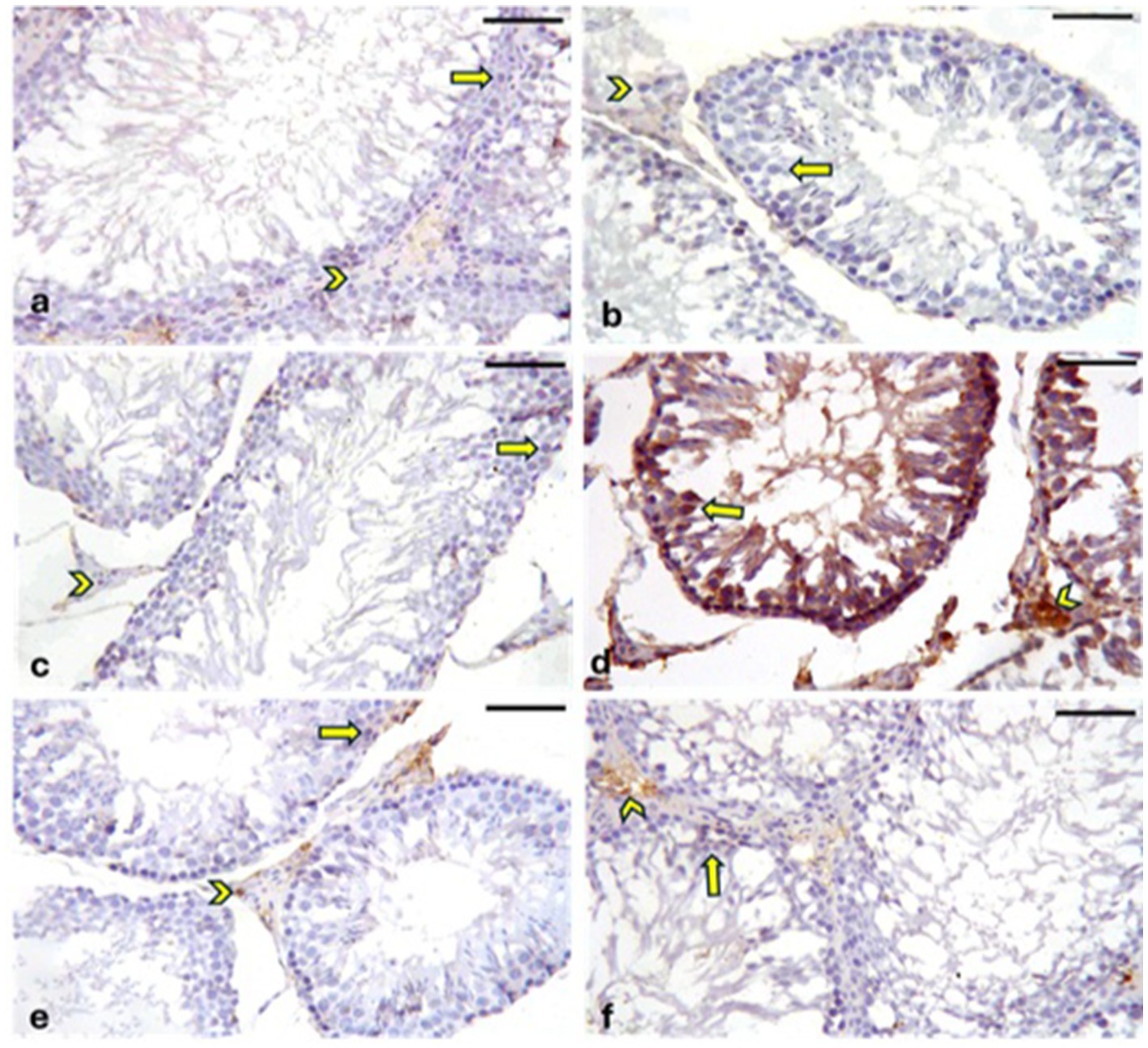
Figure 12. Photomicrographs showing Cyclooxygenase-2 (COX-2) expression in the testes across different groups. The CONT (a), RUT (b), and MOLE (c) groups exhibit negative to weak COX-2 immunoreaction in the cytoplasm or nucleus of the germinal epithelium (arrows) and interstitial Leydig cells (arrowheads). In contrast, the MG (d) group displays positive COX-2 immunoreactivity, indicated by a deep brown reaction, in the seminiferous epithelium (arrows) and interstitial Leydig cells (arrowheads). The RUT + MG (e) and MOLE + MG (f) groups show weak-to-moderate COX-2 immunoreaction in some seminiferous epithelia (arrows) and Leydig cells (arrowheads) (scale bar = 50 μm).
3.9.3 Immunohistochemical staining for caspase-3
A positive reaction for caspase-3 is indicated by brown cytoplasmic or nuclear staining. In the CONT (Figure 13a), RUT (Figure 13b), and MOLE (Figure 13c) groups, the immunoreaction of caspase-3 was either negative or weak in the cytoplasmic or nuclear regions of both the germinal epithelia and interstitial Leydig cells. Conversely, the MG group (Figure 13d) exhibited strong caspase-3 immunoreactivity in germinal epithelia and Leydig cells. The RUT + MG (Figure 13e) and MOLE + MG (Figure 13f) groups showed moderate caspase-3 immunoreaction in some germinal epithelia and Leydig cells.
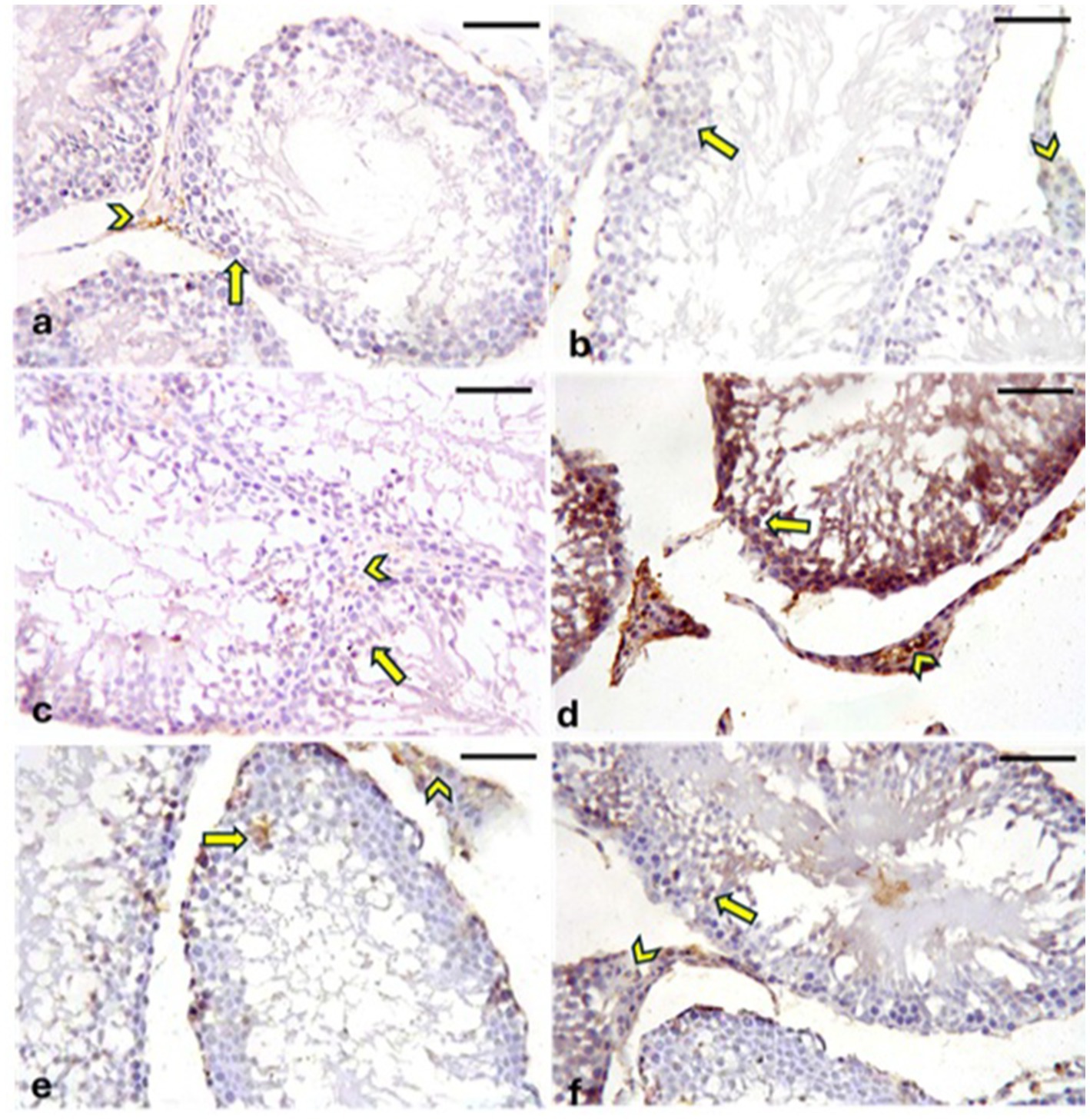
Figure 13. Photomicrographs showing caspase-3 expression in the testes across all groups. The CONT (a), RUT (b), and MOLE (c) groups display negative to weak caspase-3 immunoreactions in the germinal epithelia (arrows) and interstitial Leydig cells (arrowheads). In the MG group (d), strong caspase-3 immunoreactivity (deep brown staining) is observed in both the seminiferous epithelia (arrows) and Leydig cells (arrowheads). The RUT + MG (e) and MOLE + MG (f) groups exhibit moderate caspase-3 immunoreactions in some seminiferous epithelia (arrows) and Leydig cells (arrowheads) (scale bar = 50 μm).
3.10 Morphometric analysis
The influence of RUT and MOLE on the mean area percentage values in the testicular tissue of rats intoxicated with MG was examined in Figure 14. The MG group showed a significant increase in the mean area percentage of collagen contents, PAS +ve reaction, COX2 and caspase-3 +ve immunoreaction, and a significant decrease in PCNA +ve immunoreaction compared to the CONT group at p < 0.05. pre-administration of rutin and MOLE to MG significantly increased the mean area percentage of collagen contents, PAS +ve reaction, COX2 and caspase-3 +ve immunoreaction and significantly decreased in PCNA +ve immunoreaction in testicular tissues compared to the MG group at p < 0.05. This impact indicated their protective effects against the damage induced by MG treatment, although the mean area percentage of COX2 and caspase-3 +ve immunoreaction levels did not return ultimately to the baseline levels observed in the CONT group.
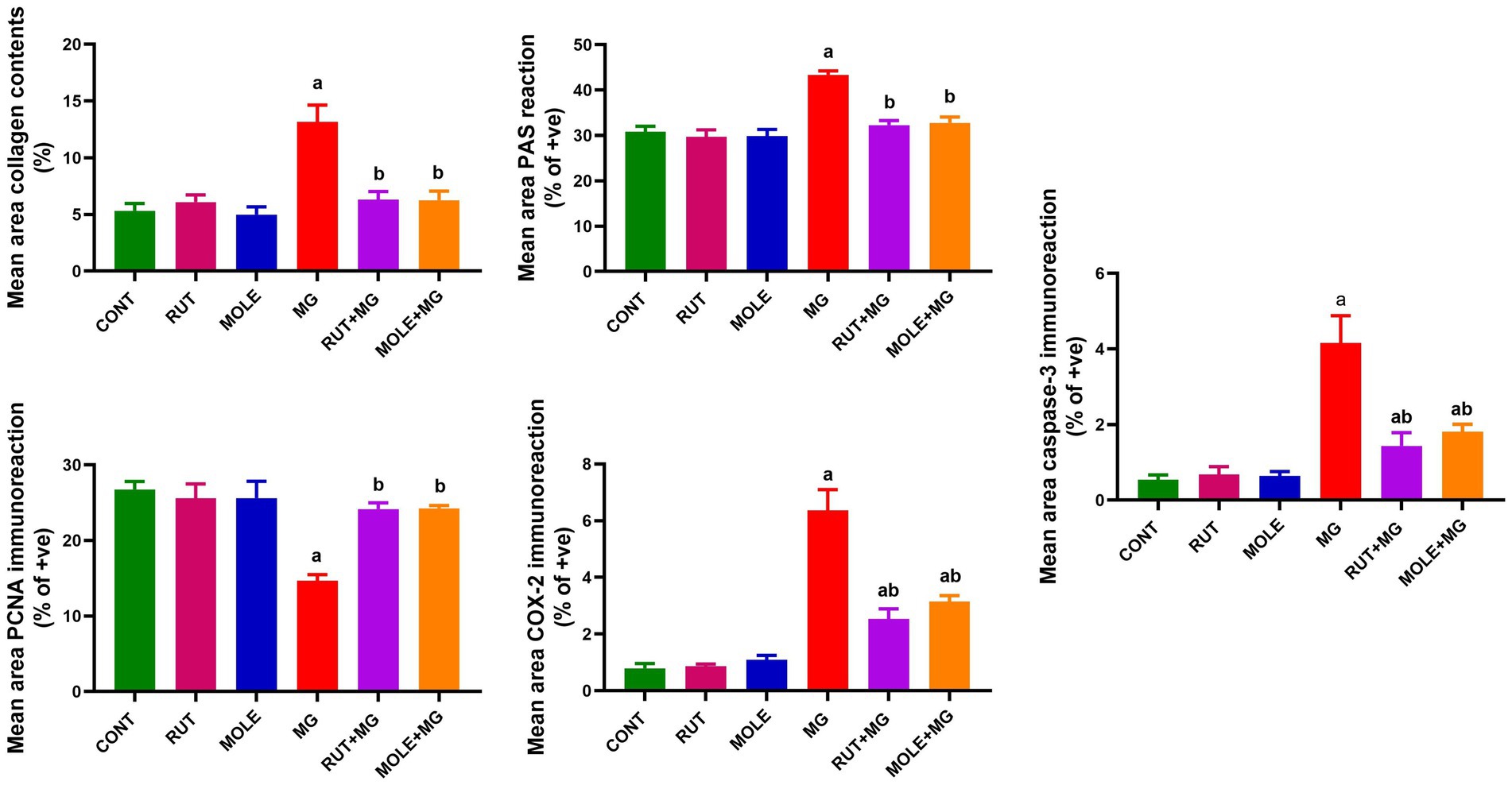
Figure 14. Quantitative analysis of mean area percentage of Masson’s trichrome-stained collagen contents, positive PAS reaction, and positive PCNA, COX2 and caspase-3 immunoreactions in the testicular tissues of different studied groups. Results are presented as mean ± SD. aIndicates significant change vs. the control group at p < 0.05; bindicates significant changes vs. the MG-treated group at p < 0.05.
4 Discussion
Monosodium glutamate is a prevalent food ingredient in numerous processed and seasoned foods. Although MG has been linked to numerous adverse consequences on biological functions, studies have specifically emphasized its deleterious influence on the male reproductive system. This study examined the possible protective effects of rutin and Moringa oleifera leaf extract against MG-induced testicular damage in male rats.
Our biochemical experiments demonstrated that MG treatment markedly raised oxidative stress, evidenced by higher levels of nitric oxide and MDA, a biomarker of lipid peroxidation. A significant reduction in GSH levels further emphasized compromised antioxidant defenses. Furthermore, diminished activities of critical antioxidant enzymes (SOD, CAT, GPx, and GR) in testicular tissues indicate that MG compromises the enzymatic antioxidant defense mechanism, exacerbating testicular injury.
Oxidative stress appears after an imbalance between the capacity of oxidation and antioxidation in the body that leads to the excessive manufacture of reactive molecules like reactive nitrogen species (RNS) and reactive oxygen species (ROS). These unsteady radicals, involving hydrogen peroxide (H2O2), hydroxyl radicals (OH−), nitric oxide (NO), and superoxide anion (O2−), can produce significant tissue damage (60).
Lipid peroxidation, driven by ROS, results in the generation of lipid hydroperoxides (LOOHs) as primary products and secondary aldehyde products, including MDA, propanal, hexanal, and 4-hydroxynonenal (4-HNE). These compounds contribute to peroxidative damage, notably in reproductive cells, decreasing cell viability (61). Testicular tissues are susceptible to oxidative stress due to their high amount of unsaturated fatty acids, rapid cell division, and high mitochondrial oxygen demand. Environmental contaminants, nutrition, and exposure to X-rays and chemotherapy can further worsen this damage (62).
Monosodium glutamate has been found to generate oxidative stress in experimental rats through chronic administration at doses of 4 mg/kg body weight and sub-chronic administration at dosages of 0.6 to 1.6 mg/kg body weight (63). Once ingested, MG is transformed into sodium ions and L-glutamate (2). The reproductive organs are rich in glutamate receptors, and when these receptors are overstimulated by glutamate or its analogs, it leads to increased ROS generation and lipid peroxidation (64).
Egbuonu et al. (65) stated that an increment in levels of MDA after MG treatment returns to oxidative stress. Ayoola et al. (66) also reported higher nitrite concentrations in experimental animals after MG administration, indicating oxidative stress. A decline in the activities of antioxidant enzymes such as CAT, SOD, GR, and GPx, likely due to the excellent production of ROS, was observed by Wang et al. (67). The inhibition of antioxidant enzymes after MG exposure may result from ROS protein inactivation (68).
This pre-administration of RUT and MOLE attenuated the effects generated by MG. The considerable reduction in oxidative stress markers (MDA, nitric oxide), augmentation of GSH level, and the restoration of antioxidant enzyme (SOD, CAT, GPx, and GR) activities imply that these substances boost the testicular antioxidant defense system.
FTIR spectrum of crude aqueous MOLE in this study showed the presence of various functional groups, including hydroxyl groups (OH), carbonyl groups (CO), alkanes (CH), and others. This outcome proposes that the extract contains water, fatty acids, lipids, proteins, polysaccharides, and maybe phenolic or aromatic compounds. The presence of these functional groups promotes the extract’s potential bioactive qualities, which are commonly connected to its medical and nutritional advantages.
Moringa oleifera leaves are rich in bioactive components, including vital minerals and phytonutrients (69). These necessary nutrients consist of proteins, vitamins (E, C, beta-carotene, and B6), minerals (such as calcium, phosphorus, and magnesium), and fatty acids (70). The bioactive phytonutrients in MO leaves include flavonoids (like quercetin and kaempferol) and phenolic acids such as ferulic, gallic, vanillic, and ellagic acids, with chlorogenic acid being the most abundant, as well as glycosides, saponins, alkaloids, tannins, isothiocyanates, and glucosinolates (71). Rutin (quercetin-3-O-rutin or rutinoside) is an abundant flavonoid component in numerous fruits and vegetables (17).
Moringa oleifera has continuously shown antioxidative properties through much research. It has been shown to increase glutathione concentrations in cells exposed to 250 μg/mL of the extract (36), reduce lipid peroxidation, and enhance antioxidant defenses by elevating activities of SOD, CAT, GSH, and ascorbic acid in the testes of diabetes mellitus-induced rats, suggesting a protective role in male fertility (72). Additionally, MO has been demonstrated to raise SOD and CAT activities while lowering MDA in animal models exposed to environmental toxicants (73). It also boosted testicular levels of GPx (74) and lowered glutathione S-transferase (GST) activity (75). In oxidative stress models, Moringa extract dramatically lowered MDA levels in acetaminophen-induced oxidative stress (76) and reduced MDA concentrations in obese rats while enhancing antioxidant enzymes like SOD, CAT, and GSH (40). Fakurazi et al. (76) further confirmed a substantial increase in catalase activity with Moringa extract treatment (p < 0.05).
Our results with RUT and MOLE coincide with recent findings revealing that some flavonoids from plants and fruits are potent O2•− scavengers (77). Moreover, various investigations have revealed that the pharmacological effects of flavonoids are connected to their antioxidant activity, which may entail scavenging OH• and O2•−, chelating metal ions, and exerting synergistic effects with other antioxidant metabolites (78). Quercetin is a dietary oxidant that boosts the antioxidant defense system and removes oxygen radicals created during cellular metabolism. The antioxidant activity of quercetin comprises various processes. On the one hand, as a polyphenol, the polyphenol hydroxyl group in its molecular structure can act as a hydrogen donor and combine with free radicals to directly scavenge ROS (60). Together with these findings, our results suggest that flavonoids could be major active components of RUT and MOLE in addition to phenolic chemicals in MOLE.
Organ weight analysis is a crucial marker in toxicological research for determining the detrimental effects of substances (79). Studies have revealed that changes in reproductive organ weight significantly indicate changes in reproductive hormone levels (80). Consistent with these findings, prior studies have demonstrated that the testicular weight of rats treated with MG considerably decreased (80, 81). Our findings supported this data since MG treatment resulted in a reduction in relative testicular weight. Additionally, we detected a significant drop in serum testosterone, LH, and FSH levels, which accords with findings from multiple other studies (80, 82). These results may be related to the reproductive system’s susceptibility to glutamate-induced damage due to many glutamate receptors in reproductive organs and sperm. Excitative toxicity damage to these cells was caused by excessive glutamate. Besides, glutamate-induced toxicity and elevated levels of ROS may interrupt hormonal balance by impairing the hypothalamic–pituitary-gonadal (HPG) axis, a critical system for controlling reproductive function (83). The disruption is linked to downregulating genes’ encoded enzymes participating in steroidogenesis, LH receptor activity, and signal transduction (84). Consequently, there is a reduction in the release of LH and FSH from the anterior pituitary gland (85). Decreased LH secretion hinders the stimulation of Leydig cells, leading to insufficient testosterone production (86), while lower FSH levels adversely influence the release of androgen-binding protein (ABP), which is important for concentrating testosterone (87). These hormonal disruptions are correlated to reduce spermatogenesis (82), which can negatively influence the development and preservation of testicular tissue, potentially reducing testis’ weight (43, 88). Since testis weight is correlated with the mass of differentiated spermatogenic cells, the detected decrease may be ascribed to a lower density of germ cells and mature spermatids (89).
Rutin’s structural closeness to estradiol significantly decreases toxicity induced by Zearalenone (ZEN). RUT has the ability to competitively bind to estrogen receptors, hence decreasing the deleterious effects of ZEN on these receptors. This competitive binding may help manage the hormonal abnormalities generated by ZEN exposure, notably throughout the reproductive system (90). Elsawy et al. (25) established RUT’s potential to restore lowered serum levels of testosterone (T), LH, and FSH in male mice exposed to CCl4, attributing this impact to its antioxidant characteristics. Consistent with these observations, Sayed et al. (90) found that RUT successfully ameliorated endocrine abnormalities generated by ZEN. MOLE at 300 mg/kg has been proven to raise serum testosterone, FSH, LH, sperm count, and sperm motility. The androgenic effects of MOLE on the male reproductive system have been demonstrated in vitro (36). Studies on male rats and rabbit bucks have indicated that MOLE dramatically enhances serum testosterone levels and the gene expression of LH and FSH (75, 91).
In this work, MG treatment promoted apoptosis in testicular germ cells, as demonstrated by increased expression of pro-apoptotic markers Bax and caspase-3, alongside decreased expression of the anti-apoptotic protein Bcl-2. A positive immunoreactivity of caspase-3 in the germinal epithelium and Leydig cells indicated the elevation of apoptosis. These results are reliable with those of Anbarkeh et al. (88), who noted that exposure to MG-activated caspase-3, a key enzyme in the apoptosis pathway, guides to increased germ cell apoptosis and subsequent testicular atrophy in mice. Similarly, Sarhan (92) found that MG promoted apoptosis in the testicular cells of rats.
In this investigation, rutin (a flavonoid constituent) and Moringa oleifera leaf extract and their flavonoid and polyphenolic fractions revealed strong anti-apoptotic protective effects against MG-induced apoptosis. This safeguard was proved by a decline in pro-apoptotic markers Bax and caspase-3 levels and increased anti-apoptotic protein Bcl-2 in testicular tissues. These results were also confirmed by a negative to moderate immunoreactivity of caspase-3 in the reproductive epithelium and Leydig cells.
NF-kB is known to stimulate the transcription of genes implicated in the apoptosis of male germ cells, often through the stimulation of Bax/Bcl-2 and the start of caspases (93). Furthermore, it has been shown that methods lowering ROS production ensure that the Bcl-2 gene prevents apoptosis, safeguarding the cells (94). Moringa oleifera extract downregulates caspase-3 and inhibits the activation of pathways such as NF-kB and phosphatidylinositide 3-kinase/protein kinase B (P13K/PKB), consequently inhibiting testicular apoptosis by reducing Bax expression (95). This action helps avoid male infertility. Sayed et al. (90) observed that RUT suppresses the apoptotic signaling cascade by enhancing the structural integrity of the spermatogenic tubules in the testes of mice exposed to Zearalenone (ZEN).
Our study indicated that rats exposed to MG displayed higher testicular levels of inflammatory markers, such as TNF-α and IL-1β. High COX-2 antibody seen in both the germinal epithelium and Leydig cells, further corroborating these effects’ heightened inflammation. These findings are comparable with those of Kumar et al. (96), who reported that the inflammatory responses initiated by MG would contribute to dysfunction and damage of the testis. The exposure to MG will induce oxidative stress, a precursor to inflammation (97). Oxidative stress may activate a transcription factor, “NF-κB” (98), which transfers to the nucleus, leading to the production of inflammatory mediators like IL-1β, IL-6 and TNF-α (99, 100).
When RUT and MOLE were given before the study, the levels of TNF-α and IL-1β in the testicles went down, which showed an anti-inflammatory response. This impact is further substantiated by the negative to moderate COX-2 antibody detected in the germinal epithelium and Leydig cells. MOLE has shown the capacity to lower the production of pro-inflammatory cytokines, including TNF-α and IL-6, and suppress the expression of RelA, a gene implicated in NF-κB p65 activation during inflammation. This outcome correlates with recent findings on the anti-inflammatory properties of Moringa oleifera (101, 102). Moreover, RUT from Marrubium alysson L. has shown potent anti-inflammatory efficacy in protecting testicular functioning in methotrexate-injected mice (103).
Our study suggests that MG treatment generates considerable oxidative stress, hormonal abnormalities, apoptosis, and inflammation, resulting in widespread cellular damage and testicular toxicity. The histological, histochemical, and immunohistochemical examinations of the testes confirmed these pathological effects, which appeared as pyknosis and reduced the heights of the germinal epithelium. Degenerated seminiferous tubules with few or no sperms and detached spermatogenic cells from the basement membranes with desquamated cells appeared. Interstitial edema, vacuolation, inflammatory infiltration and congested blood vessels were shown. Significant depositions of collagen fibers in capsules, interstitial and basal lamina of seminiferous tubules exhibited severity of damage. Strong PAS reaction shown in the basal lamina of tubules, spermatogenic cells and interstitial tissues detects structural and functional disorders of testes. The decline in cell proliferation appeared as most seminiferous tubules containing few or no sperm confirmed through a weak PCNA immunoreaction in germinal epithelium.
Due to its propensity to influence reproductive cells, MG can cause sperm modifications, histological changes, hormonal imbalances, and oxidative damage, finally leading to problems in reproductive function (104). Histological changes in the reproductive organs have been documented in rats treated with MG, including detachment and vacuolation of the seminal epithelium, degeneration of spermatogenesis, and edema inside the testicular lumen (80). Exfoliation of spermatocytes and spermatids within the lumens of seminiferous tubules was shown after MG treatment (105). Many spermatogenic cells appeared necrotic, with pyknotic nuclei and dilated, congested blood vessels shown under MG’s effects on the testes (106).
Severe histological changes such as degeneration of seminiferous tubules and reduction of sperm production observed by Acikel-Elmas et al. (107) resulted in oxidative stress and apoptosis produced after high doses of exposure MG. Abdelhameed et al. (103) stressed that oxidative stress and inflammation are significant factors in testicular injury, with elevated ROS levels destroying cellular components such as lipids, proteins, and DNA, ultimately compromising testicular function (108). Moreover, degenerative alterations in the seminiferous tubules produced by MG lead to decreased spermatogenesis and lower overall testis weight (83, 109).
The ameliorative effects of rutin and Moringa oleifera leaf extract in this study were demonstrated by noticeable improvements of histopathological changes in testicular sections returned to their constituents. Flavonoids have antioxidant and anti-inflammatory characteristics and can potentially improve the toxicity of testes induced by Zearalenone (110). The antioxidant property of rutin in reducing reproductive toxicity of germinal epithelium, cellular configuration and structural integrity in mice induced by cadmium was emphasized by Abarikwu et al. (111). Similar protective effects of RUT against testicular toxicity generated by several substances have been demonstrated in male mice (90, 111).
Moringa oleifera extract contains antioxidative, anti-inflammatory, anti-diabetic, anti-obesity, and anti-apoptotic activities (40, 101). Studies have demonstrated that Moringa treatment improves testicular shape, boosts spermatogenic cell activity, and raises sperm density in numerous animal models. In rabbit bucks, large dosages of Moringa leaves led to increased germinal cell activity, improved spermatozoa presence in the lumen of seminiferous tubules, and more significant interstitial regions with normal Leydig cells compared to non-supplemented bucks (39, 112). Additionally, treatment with Moringa in male rats dramatically enhanced seminiferous tubule width, epithelial height, spermatogenic efficiency, and the number of Sertoli and spermatogenic cells (37). MO also demonstrated ameliorative effects on the testicular histology of diabetic rats, expanding the number of spermatogonia and spermatids, increasing seminiferous tubule diameter and Leydig cell nuclear diameter, and boosting epididymal weight (72, 113).
Study limitation includes no direct clinical trials on humans in this concern, hence further clinical studies are needed.
5 Conclusion
The ameliorative effects of rutin and Moringa oleifera leaf extract against monosodium glutamate-induced testicular toxicity in rats were emphasized in this study and can be attributed to their potent antioxidant, anti-inflammatory, and anti-apoptotic characteristics. So, they may serve as a protective agent to alleviate reproductive toxicity caused by dietary and environmental toxins. Additional studies are required to explore the long-term effects, optimal dosage for therapeutic applications, and a more detailed exploration of their mechanism of action at the molecular level of these compounds.
Data availability statement
The original contributions presented in the study are included in the article/supplementary material, further inquiries can be directed to the corresponding author.
Ethics statement
The animal study was approved by the experiment adhered to the Ain-Shams Research Institute Animal Facility guidelines under veterinary supervision. The protocol was conducted following the ethical research guidelines of Ain-Shams University, with the Experimental Animal Research Unit code number [RE (189)22]. The study was conducted in accordance with the local legislation and institutional requirements.
Author contributions
DA-A: Data curation, Formal analysis, Writing – review & editing. MI: Conceptualization, Investigation, Supervision, Validation, Visualization, Writing – review & editing. SM: Data curation, Formal analysis, Investigation, Writing – review & editing. ME-K: Conceptualization, Funding acquisition, Project administration, Resources, Writing – review & editing. AA: Conceptualization, Data curation, Visualization, Writing – review & editing. AF: Conceptualization, Data curation, Formal analysis, Writing – review & editing. DS: Conceptualization, Data curation, Formal analysis, Writing – original draft, Writing – review & editing.
Funding
The author(s) declare that financial support was received for the research and/or publication of this article. Princess Nourah bint Abdulrahman University researchers supporting the project number (PNURSP2025R23), Princess Nourah bint Abdulrahman University, Riyadh, Saudi Arabia.
Conflict of interest
The authors declare that the research was conducted in the absence of any commercial or financial relationships that could be construed as a potential conflict of interest.
The author(s) declared that they were an editorial board member of Frontiers, at the time of submission. This had no impact on the peer review process and the final decision.
Generative AI statement
The author(s) declare that no Gen AI was used in the creation of this manuscript.
Publisher’s note
All claims expressed in this article are solely those of the authors and do not necessarily represent those of their affiliated organizations, or those of the publisher, the editors and the reviewers. Any product that may be evaluated in this article, or claim that may be made by its manufacturer, is not guaranteed or endorsed by the publisher.
References
1. Hernandez Bautista, RJ, Mahmoud, AM, Konigsberg, M, and Lopez Diaz Guerrero, NE. Obesity: pathophysiology, monosodium glutamate-induced model and anti-obesity medicinal plants. Biomed Pharmacother. (2019) 111:503–16. doi: 10.1016/j.biopha.2018.12.108
2. Kayode, OT, Bello, JA, Oguntola, JA, Kayode, AAA, and Olukoya, DK. The interplay between monosodium glutamate (MSG) consumption and metabolic disorders. Heliyon. (2023) 9:e19675. doi: 10.1016/j.heliyon.2023.e19675
3. Niaz, K, Zaplatic, E, and Spoor, J. Guest editorial: extensive use of monosodium glutamate: a threat to public health? EXCLI J. (2018) 17:964. doi: 10.17179/excli2018-1092a
4. Zanfirescu, A, Ungurianu, A, Tsatsakis, AM, Nitulescu, GM, Kouretas, D, Veskoukis, A, et al. A review of the alleged health hazards of monosodium glutamate. Compr Rev Food Sci Food Saf. (2019) 18:1111–34. doi: 10.1111/1541-4337.12448
5. Insights, GM (2025) Monosodium glutamate market size & Share Analysis-Growth Trends & Forecasts (2025–2030). Mordor intelligence. Available online at: https://www.mordorintelligence.com/industry-reports/monosodium-glutamate-msg-market. (Accessed January 9, 2025).
6. Katranci, Y, Aydemir, A, Kizilkaya, B, Bastemur, GY, and Ozkorucuklu, SP. Investigation of monosodium glutamate content in flavors, seasonings, and sauces from local markets in Turkey. Food Sci Nutr. (2024) 12:7806–13. doi: 10.1002/fsn3.4406
7. Kassab, RB, Theyab, A, Al-Ghamdy, AO, Algahtani, M, Mufti, AH, Alsharif, KF, et al. Protocatechuic acid abrogates oxidative insults, inflammation, and apoptosis in liver and kidney associated with monosodium glutamate intoxication in rats. Environ Sci Pollut Res Int. (2022) 29:12208–21. doi: 10.1007/s11356-021-16578-4
8. Mondal, M, Sarkar, K, Nath, PP, and Paul, G. Monosodium glutamate suppresses the female reproductive function by impairing the functions of ovary and uterus in rat. Environ Toxicol. (2018) 33:198–208. doi: 10.1002/tox.22508
9. Hernandez-Bautista, RJ, Alarcon-Aguilar, FJ, Del, CE-VM, Almanza-Perez, JC, Merino-Aguilar, H, Fainstein, MK, et al. Biochemical alterations during the obese-aging process in female and male monosodium glutamate (MSG)-treated mice. Int J Mol Sci. (2014) 15:11473–94. doi: 10.3390/ijms150711473
10. Datta, A, Hossain, A, and Roy, S. An overview on monosodium glutamate: its direct and indirect effects. Res J Pharmacy Technol. (2019) 12:6187–92. doi: 10.5958/0974-360X.2019.01074.6
11. Oladipo, I, Adebayo, EA, and Kuye, OM. Effects of monosodium glutamate in ovaries of female Sprague-Dawley rats. Int J Curr Microbiol Appl Sci. (2015) 4:737–45.
12. Abd-Elkareem, M, Soliman, M, Abd El-Rahman, MAM, and Abou Khalil, NS. The protective effect of Nigella sativa seeds against monosodium glutamate-induced hepatic dysfunction in rats. Toxicol Rep. (2022) 9:147–53. doi: 10.1016/j.toxrep.2022.01.014
13. Lopes, FNC, da Cunha, NV, de Campos, BH, Fattori, V, Panis, C, Cecchini, R, et al. Antioxidant therapy reverses sympathetic dysfunction, oxidative stress, and hypertension in male hyperadipose rats. Life Sci. (2022) 295:120405. doi: 10.1016/j.lfs.2022.120405
14. El-Khadragy, MF, Al-Megrin, WA, Alomar, S, Alkhuriji, AF, Metwally, DM, Mahgoub, S, et al. Chlorogenic acid abates male reproductive dysfunction in arsenic-exposed mice via attenuation of testicular oxido-inflammatory stress and apoptotic responses. Chem Biol Interact. (2021) 333:109333. doi: 10.1016/j.cbi.2020.109333
15. Omogbiya, AI, Ben-Azu, B, Eduviere, AT, Eneni, AO, Nwokoye, PO, Ajayi, AM, et al. Monosodium glutamate induces memory and hepatic dysfunctions in mice: ameliorative role of Jobelyn((R)) through the augmentation of cellular antioxidant defense machineries. Toxicol Res. (2021) 37:323–35. doi: 10.1007/s43188-020-00068-9
16. Takumi, A, Kawamata, Y, Sakai, R, and Narita, T. In vitro and in vivo genotoxicity studies on monosodium L-glutamate monohydrate. Regul Toxicol Pharmacol. (2019) 107:104399. doi: 10.1016/j.yrtph.2019.05.024
17. Abdelfattah, MS, Badr, SE, Lotfy, SA, Attia, GH, Aref, AM, Abdel Moneim, AE, et al. Rutin and selenium co-administration reverse 3-nitropropionic acid-induced neurochemical and molecular impairments in a mouse model of Huntington’s disease. Neurotox Res. (2020) 37:77–92. doi: 10.1007/s12640-019-00086-y
18. Mohamed, KM, Abdelfattah, MS, El-khadragy, M, Al-Megrin, WA, Fehaid, A, Kassab, RB, et al. Rutin-loaded selenium nanoparticles modulated the redox status, inflammatory, and apoptotic pathways associated with pentylenetetrazole-induced epilepsy in mice. Green Process Synth. (2023) 12:10. doi: 10.1515/gps-2023-0010
19. Davinelli, S, Bertoglio, JC, Zarrelli, A, Pina, R, and Scapagnini, G. A randomized clinical trial evaluating the efficacy of an anthocyanin-Maqui berry extract (Delphinol (R)) on oxidative stress biomarkers. J Am Coll Nutr. (2015) 34:28–33. doi: 10.1080/07315724.2015.1080108
20. Pareek, A, Pant, M, Gupta, MM, Kashania, P, Ratan, Y, Jain, V, et al. Moringa oleifera: An updated comprehensive review of its pharmacological activities, Ethnomedicinal, Phytopharmaceutical formulation, clinical, phytochemical, and toxicological aspects. Int J Mol Sci. (2023) 24:2098. doi: 10.3390/ijms24032098
21. Al-Harbi, NO, Imam, F, Al-Harbi, MM, Al-Shabanah, OA, Alotaibi, MR, As Sobeai, HM, et al. Rutin inhibits carfilzomib-induced oxidative stress and inflammation via the NOS-mediated NF-kappa B signaling pathway. Inflammopharmacology. (2019) 27:817–27. doi: 10.1007/s10787-018-0550-5
22. Farha, AK, Gan, RY, Li, HB, Wu, DT, Atanasov, AG, Gul, K, et al. The anticancer potential of the dietary polyphenol rutin: current status, challenges, and perspectives. Crit Rev Food Sci Nutr. (2022) 62:832–59. doi: 10.1080/10408398.2020.1829541
23. Qu, S, Dai, C, Lang, F, Hu, L, Tang, Q, Wang, H, et al. Rutin attenuates vancomycin-induced nephrotoxicity by ameliorating oxidative stress, apoptosis, and inflammation in rats. Antimicrob Agents Chemother. (2019) 63:18. doi: 10.1128/AAC.01545-18
24. Kandemir, FM, Ozkaraca, M, Yildirim, BA, Hanedan, B, Kirbas, A, Kilic, K, et al. Rutin attenuates gentamicin-induced renal damage by reducing oxidative stress, inflammation, apoptosis, and autophagy in rats. Ren Fail. (2015) 37:518–25. doi: 10.3109/0886022X.2015.1006100
25. Elsawy, H, Badr, GM, Sedky, A, Abdallah, BM, Alzahrani, AM, and Abdel-Moneim, AM. Rutin ameliorates carbon tetrachloride (CCl4)-induced hepatorenal toxicity and hypogonadism in male rats. PeerJ. (2019) 7:e7011. doi: 10.7717/peerj.7011
26. Susanto, H, Taufiq, A, Sunaryono, S, Soontaranon, S, Hariyanto, YA, Mawardi, AI, et al. Moringa oleifera leaf powder Madura variety: characterization and biomaterials property for biomedical and nanotechnology application. J Phys Conf Ser. (2018) 1093:12007. doi: 10.1088/1742-6596/1093/1/012007
27. Baldisserotto, A, Buso, P, Radice, M, Dissette, V, Lampronti, I, Gambari, R, et al. Moringa oleifera leaf extracts as multifunctional ingredients for "natural and organic" sunscreens and Photoprotective preparations. Molecules. (2018) 23:664. doi: 10.3390/molecules23030664
28. El-Khadragy, M, Alolayan, EM, Metwally, DM, El-Din, MFS, Alobud, SS, Alsultan, NI, et al. Clinical efficacy associated with enhanced antioxidant enzyme activities of silver nanoparticles biosynthesized using Moringa oleifera leaf extract, against cutaneous Leishmaniasis in a murine model of Leishmania major. Int J Environ Res Public Health. (2018) 15:1037. doi: 10.3390/ijerph15051037
29. Saini, RK, Sivanesan, I, and Keum, YS. Phytochemicals of Moringa oleifera: a review of their nutritional, therapeutic and industrial significance. 3 Biotech. (2016) 6:203. doi: 10.1007/s13205-016-0526-3
30. Leone, A, Spada, A, Battezzati, A, Schiraldi, A, Aristil, J, and Bertoli, S. Cultivation, genetic, Ethnopharmacology, Phytochemistry and pharmacology of Moringa oleifera leaves: An overview. Int J Mol Sci. (2015) 16:12791–835. doi: 10.3390/ijms160612791
31. Mahajan, SG, Banerjee, A, Chauhan, BF, Padh, H, Nivsarkar, M, and Mehta, AA. Inhibitory effect of n-butanol fraction of Moringa oleifera lam. Seeds on ovalbumin-induced airway inflammation in a guinea pig model of asthma. Int J Toxicol. (2009) 28:519–27. doi: 10.1177/1091581809345165
32. Abdallah, R, Mostafa, NY, Kirrella, GAK, Gaballah, I, Imre, K, Morar, A, et al. Antimicrobial effect of Moringa oleifera leaves extract on foodborne pathogens in ground beef. Food Secur. (2023) 12:766. doi: 10.3390/foods12040766
33. Nurhayati, T, Ridho, MF, Santoso, PTR, Setiawan, S, Goenawan, H, and Tarawan, VM. Effects of Moringa oleifera leaf extract on liver histopathology: a systematic review. J Nutr Metab. (2024) 2024:6815993. doi: 10.1155/2024/6815993
34. Abd-Elnaby, YA, ElSayed, IE, Abd Eldaim, MA, Badr, EA, Abdelhafez, MM, and Elmadbouh, I. Anti-inflammatory and antioxidant effect of Moringa oleifera against bisphenol-A-induced hepatotoxicity. Egypt Liver J. (2022) 12:57. doi: 10.1186/s43066-022-00219-7
35. Okwari, O. Anti-Hypercholesterolemic and Hepatoprotective effect of aqueous leaf extract of Moringa oleifera in rats fed with Thermoxidized palm oil diet. IOSR J Pharmacy Biol Sci. (2013) 8:57–62. doi: 10.9790/3008-0825762
36. Opuwari, CS, Matshipi, MN, Phaahla, MK, Setumo, MA, Moraswi, RT, Zitha, AA, et al. Androgenic effect of aqueous leaf extract of Moringa oleifera on Leydig TM3 cells in vitro. Andrologia. (2020) 52:e13825. doi: 10.1111/and.13825
37. Laoung-On, J, Saenphet, K, Jaikang, C, and Sudwan, P. Effect of Moringa oleifera lam. Leaf Tea on Sexual Behavior and Reproductive Function in Male Rats. Plants. (2021) 10:2019. doi: 10.3390/plants10102019
38. Khalid, S, Arshad, M, Mahmood, S, Siddique, F, Roobab, U, Ranjha, MMAN, et al. Extraction and quantification of Moringa oleifera leaf powder extracts by HPLC and FTIR. Food Anal Methods. (2023) 16:787–97. doi: 10.1007/s12161-023-02470-z
39. Ogunlade, B, Jeje, SO, Adelakun, SA, and Akingbade, GT. Moringa oleifera restored semen quality, hormonal profile, and testicular morphology against highly active antiretroviral therapy-induced toxicity in adult male Wistar rats. JBRA Assist Reprod. (2022) 26:3–12. doi: 10.5935/1518-0557.20210032
40. Alkafafy, ME, Sayed, SM, El-Shehawi, AM, El-Shazly, S, Farouk, S, Alotaibi, SS, et al. Moringa oleifera ethanolic extract ameliorates the testicular dysfunction resulted from HFD-induced obesity rat model. Andrologia. (2021) 53:e14126. doi: 10.1111/and.14126
41. Shah, MA, Bosco, SJD, and Mir, SA. Effect of Moringa oleifera leaf extract on the physicochemical properties of modified atmosphere packaged raw beef. Food Packag Shelf Life. (2015) 3:31–8. doi: 10.1016/j.fpsl.2014.10.001
42. Sadek, KM, Abouzed, TK, Abouelkhair, R, and Nasr, S. The chemo-prophylactic efficacy of an ethanol Moringa oleifera leaf extract against hepatocellular carcinoma in rats. Pharm Biol. (2017) 55:1458–66. doi: 10.1080/13880209.2017.1306713
43. Hamza, RZ, and Al-Harbi, MS. Monosodium glutamate induced testicular toxicity and the possible ameliorative role of vitamin E or selenium in male rats. Toxicol Rep. (2014) 1:1037–45. doi: 10.1016/j.toxrep.2014.10.002
44. Airaodion, AI, Ngwogu, KO, Ngwogu, AC, Megwas, AU, and Ekenjoku, JA. Nephrotoxicity of monosodium glutamate (MSG) in Wistar rats. Int J Adv Nephrol Res. (2020) 3:1–10.
45. Essa, AH, Khudhair, N, and Alrafas, HR. Effect of different methods of anesthesia on physio-biochemical parameters in laboratory male rats. Basrah J Vet Res. (2020) 19:206–14.
46. Ohkawa, H, Ohishi, N, and Yagi, K. Assay for lipid peroxides in animal tissues by thiobarbituric acid reaction. Anal Biochem. (1979) 95:351–8. doi: 10.1016/0003-2697(79)90738-3
47. Green, LC, Wagner, DA, Glogowski, J, Skipper, PL, Wishnok, JS, and Tannenbaum, SR. Analysis of nitrate, nitrite, and [15N] nitrate in biological fluids. Anal Biochem. (1982) 126:131–8. doi: 10.1016/0003-2697(82)90118-X
48. Ellman, GL. Tissue sulfhydryl groups. Arch Biochem Biophys. (1959) 82:70–7. doi: 10.1016/0003-9861(59)90090-6
49. Nishikimi, M, Rao, NA, and Yagi, K. The occurrence of superoxide anion in the reaction of reduced phenazine methosulfate and molecular oxygen. Biochem Biophys Res Commun. (1972) 46:849–54. doi: 10.1016/S0006-291X(72)80218-3
50. Aebi, H. Catalase in vitro. Methods Enzymol. (1984) 105:121–6. doi: 10.1016/S0076-6879(84)05016-3
51. Paglia, DE, and Valentine, WN. Studies on the quantitative and 8thglutathione peroxidase. J Lab Clin Med. (1967) 70:158–69.
52. De Vega, L, Fernandez, RP, Mateo, MC, Bustamante, JB, Herrero, AM, and Munguira, EB. Glutathione determination and a study of the activity of glutathione-peroxidase, glutathione-transferase, and glutathione-reductase in renal transplants. Ren Fail. (2002) 24:421–32. doi: 10.1081/JDI-120006769
53. Bancroft, JD, and Layton, C. 10—The Hematoxylins and Eosin In: SK Suvarna, C Layton, and JD Bancroft, editors. Bancroft's theory and practice of histological techniques. 8th ed. Philadelphia, Ch: Elsevier (2019). 126–38.
54. Bancroft, JD, and Layton, C. 12—Connective and Other Mesenchymal Tissues with their Stains In: SK Suvarna, C Layton, and JD Bancroft, editors. Bancroft's theory and practice of histological techniques. 8th ed. Philadelphia, Ch: Elsevier (2019). 153–75.
55. Abd-Elkareem, M, Abd El-Rahman, MAM, Khalil, NSA, and Amer, AS. Antioxidant and cytoprotective effects of Nigella sativa L. seeds on the testis of monosodium glutamate challenged rats. Sci Rep. (2021) 11:13519. doi: 10.1038/s41598-021-92977-4
56. Naryzhny, SN. Proliferating cell nuclear antigen: a proteomics view. Cell Mol Life Sci. (2008) 65:3789–808. doi: 10.1007/s00018-008-8305-x
57. Elmore, S. Apoptosis: a review of programmed cell death. Toxicol Pathol. (2007) 35:495–516. doi: 10.1080/01926230701320337
58. Tsujii, M, Kawano, S, Tsuji, S, Sawaoka, H, Hori, M, and DuBois, RN. Cyclooxygenase regulates angiogenesis induced by Colon Cancer cells. Cell. (1998) 93:705–16. doi: 10.1016/S0092-8674(00)81433-6
59. Meyerholz, DK, Beck, AP, Goeken, JA, Leidinger, MR, Ofori-Amanfo, GK, Brown, HC, et al. Glycogen depletion can increase the specificity of mucin detection in airway tissues. BMC Res Notes. (2018) 11:763. doi: 10.1186/s13104-018-3855-y
60. Ulusoy, HG, and Sanlier, N. A minireview of quercetin: from its metabolism to possible mechanisms of its biological activities. Crit Rev Food Sci Nutr. (2020) 60:3290–303. doi: 10.1080/10408398.2019.1683810
61. Mohlala, K, Offor, U, Monageng, E, Takalani, NB, and Opuwari, CS. Overview of the effects of Moringa oleifera leaf extract on oxidative stress and male infertility: a review. Appl Sci. (2023) 13:4387. doi: 10.3390/app13074387
62. Asadi, N, Bahmani, M, Kheradmand, A, and Rafieian-Kopaei, M. The impact of oxidative stress on testicular function and the role of antioxidants in improving it: a review. J Clin Diagn Res. (2017) 11:IE01-IE05. doi: 10.7860/JCDR/2017/23927.9886
63. Singh, P, Mann, KA, Mangat, HK, and Kaur, G. Prolonged glutamate excitotoxicity: effects on mitochondrial antioxidants and antioxidant enzymes. Mol Cell Biochem. (2003) 243:139–45. doi: 10.1023/a:1021668314070
64. Mirzakhani, N, Farshid, AA, Tamaddonfard, E, Tehrani, A, and Imani, M. Comparison of the effects of hydroalcoholic extract of Capparis spinosa fruit, quercetin and vitamin E on monosodium glutamate-induced toxicity in rats. Vet Res Forum. (2020) 11:127–34. doi: 10.30466/vrf.2018.83041.2091
65. Egbuonu, ACC, Obidoa, O, Ezeokonkwo, C, and Ejikeme, P. Hepatotoxic effects of low dose oral administration of monosodium glutamate in male albino rats. Afr J Biotech. (2008) 8:3031–5.
66. Ezeagu, I, Ayoola, M, Ejiofor, N, and Achukwu, P. Organo-protective effect of Moringa oleifera (Moringa) and Camellia sinensis (Green tea) against histopathological damage in monosodium glutamate-induced oxidative-stressed rats. Adv Food Technol Nutr Sci. (2019) 5:26–37. doi: 10.17140/AFTNSOJ-5-154
67. Wang, EH, Yu, ZL, Bu, YJ, Xu, PW, Xi, JY, and Liang, HY. Grape seed proanthocyanidin extract alleviates high-fat diet induced testicular toxicity in rats. RSC Adv. (2019) 9:11842–50. doi: 10.1039/c9ra01017c
68. Tabatabaie, T, and Floyd, RA. Susceptibility of glutathione peroxidase and glutathione reductase to oxidative damage and the protective effect of spin trapping agents. Arch Biochem Biophys. (1994) 314:112–9. doi: 10.1006/abbi.1994.1418
69. Vergara-Jimenez, M, Almatrafi, MM, and Fernandez, ML. Bioactive components in Moringa Oleifera leaves protect against chronic disease. Antioxidants. (2017) 6:91. doi: 10.3390/antiox6040091
70. Chan Sun, M, Ruhomally, ZB, Boojhawon, R, and Neergheen-Bhujun, VS. Consumption of Moringa oleifera lam leaves lowers postprandial blood pressure. J Am Coll Nutr. (2020) 39:54–62. doi: 10.1080/07315724.2019.1608602
71. Jamili, MA, Toleng, AL, Yusuf, M, and Sahiruddin, M. The effect of Moringa oleifera leaves on the quality of Bali bulls semen. IOP Conf Series Earth Environ Sci. (2021) 788:012146. doi: 10.1088/1755-1315/788/1/012146
72. Ram Niwas, J, and Gyan Chand, J. Ameliorative effect of Moringa oleifera lam. Leaves extract on the sex hormone profile and testicular dysfunctions in Streptozotocin-induced diabetic Wistar rats. Pharm Res. (2022) 14:225–32. doi: 10.5530/pres.14.2.32
73. Ododo, A, Ojeka, S, and Dapper, D. Ameliorative effect of aqueous leaf extract of Moringa oleifera on reproductive function following cadmium chloride induced oxidative stress in male Wistar rats. Notulae Sci Biol. (2019) 11:352–7. doi: 10.15835/nsb11410485
74. Fouad Mansour, M, Arisha, A, AlGamal, M, Elsayed, A, Saad, S, and Bohi, K. Effect of Moringa oleifera leaves extract-SeNPs conjugate administration on testicular toxicity induced by melamine in rats. Zagazig Vet J. (2020) 48:213–27. doi: 10.21608/zvjz.2020.20447.1089
75. Elblehi, SS, El Euony, OI, and El-Nahas, AF. Partial ameliorative effect of Moringa leaf ethanolic extract on the reproductive toxicity and the expression of steroidogenic genes induced by subchronic cadmium in male rats. Environ Sci Pollut Res Int. (2019) 26:23306–18. doi: 10.1007/s11356-019-05607-y
76. Fakurazi, S, Sharifudin, SA, and Arulselvan, P. Moringa oleifera hydroethanolic extracts effectively alleviate acetaminophen-induced hepatotoxicity in experimental rats through their antioxidant nature. Molecules. (2012) 17:8334–50. doi: 10.3390/molecules17078334
77. Coballase-Urrutia, E, Pedraza-Chaverri, J, Cardenas-Rodriguez, N, Huerta-Gertrudis, B, Garcia-Cruz, ME, Ramirez-Morales, A, et al. Hepatoprotective effect of acetonic and methanolic extracts of Heterotheca inuloides against CCl (4)-induced toxicity in rats. Exp Toxicol Pathol. (2011) 63:363–70. doi: 10.1016/j.etp.2010.02.012
78. Raja, S, Ahamed, KF, Kumar, V, Mukherjee, K, Bandyopadhyay, A, and Mukherjee, PK. Antioxidant effect of Cytisus scoparius against carbon tetrachloride treated liver injury in rats. J Ethnopharmacol. (2007) 109:41–7. doi: 10.1016/j.jep.2006.06.012
79. Walum, E, Clemedson, C, and Ekwall, B. Principles for the validation of in vitro toxicology test methods. Toxicol In Vitro. (1994) 8:807–12. doi: 10.1016/0887-2333(94)90073-6
80. Iamsaard, S, Sukhorum, W, Samrid, R, Yimdee, J, Kanla, P, Chaisiwamongkol, K, et al. The sensitivity of male rat reproductive organs to monosodium glutamate. Acta Med Acad. (2014) 43:3–9. doi: 10.5644/ama2006-124.94
81. Nosseir, N, Ali, MHM, and Ebaid, HM. A histological and morphometric study of monosodium glutamate toxic effect on testicular structure and potentiality of recovery in adult albino rats. Res J Biol. (2012) 2:66–78.
82. Jubaidi, FF, Mathialagan, RD, Noor, MM, Taib, IS, and Budin, SB. Monosodium glutamate daily oral supplementation: study of its effects on male reproductive system on rat model. Syst Biol Reprod Med. (2019) 65:194–204. doi: 10.1080/19396368.2019.1573274
83. Kianifard, D, Shoar, SMM, Karkan, MF, and Aly, A. Effects of monosodium glutamate on testicular structural and functional alterations induced by quinine therapy in rat: An experimental study. Int J Reprod Biomed. (2021) 19:167–80. doi: 10.18502/ijrm.v19i2.8475
84. Liu, Q, Wang, H, Wang, H, Li, N, He, R, and Liu, Z. Per 1/per 2 disruption reduces testosterone synthesis and impairs fertility in elderly male mice. Int J Mol Sci. (2022) 23:37399. doi: 10.3390/ijms23137399
85. Spiers, JG, Chen, HJ, Sernia, C, and Lavidis, NA. Activation of the hypothalamic-pituitary-adrenal stress axis induces cellular oxidative stress. Front Neurosci. (2014) 8:456. doi: 10.3389/fnins.2014.00456
86. Greifova, H, Jambor, T, Tokarova, K, Spevakova, I, Knizatova, N, and Lukac, N. Resveratrol attenuates hydrogen peroxide-induced oxidative stress in TM3 Leydig cells in vitro. J Environ Sci Health A Tox Hazard Subst Environ Eng. (2020) 55:585–95. doi: 10.1080/10934529.2020.1717899
87. Leisegang, K, and Henkel, R. The in vitro modulation of steroidogenesis by inflammatory cytokines and insulin in TM3 Leydig cells. Reproduct Biol Endocrinol. (2018) 16:26. doi: 10.1186/s12958-018-0341-2
88. Rahimi Anbarkeh, F, Baradaran, R, Ghandy, N, Jalali, M, Reza Nikravesh, M, and Soukhtanloo, M. Effects of monosodium glutamate on apoptosis of germ cells in testicular tissue of adult rat: An experimental study. Int J Reprod Biomed. (2019) 17:261–70. doi: 10.18502/ijrm.v17i4.4551
89. Mesbah, SF, Shokri, S, Karbalay-Doust, S, and Mirkhani, H. The effect of Nandrolone Decanoate on the body, testis and epididymis weight and semen parameters in adult male rats. Iran J Med Sci. (2007) 32:93–9.
90. Sayed, H, Zhang, Q, Tang, Y, Wang, Y, Guo, Y, Zhang, J, et al. Alleviative effect of Rutin on Zearalenone-induced reproductive toxicity in male mice by preventing Spermatogenic cell apoptosis and modulating gene expression in the hypothalamic-pituitary-gonadal Axis. Toxins. (2024) 16:121. doi: 10.3390/toxins16030121
91. Nayak, G, Rao, A, Mullick, P, Mutalik, S, Kalthur, SG, Adiga, SK, et al. Ethanolic extract of Moringa oleifera leaves alleviate cyclophosphamide-induced testicular toxicity by improving endocrine function and modulating cell specific gene expression in mouse testis. J Ethnopharmacol. (2020) 259:112922. doi: 10.1016/j.jep.2020.112922
92. Sarhan, NR. The ameliorating effect of sodium selenite on the histological changes and expression of Caspase-3 in the testis of monosodium glutamate-treated rats: light and Electron microscopic study. J Microsc Ultrastruct. (2018) 6:105–15. doi: 10.4103/JMAU.JMAU_2_18
93. Orrenius, S, Nicotera, P, and Zhivotovsky, B. Cell death mechanisms and their implications in toxicology. Toxicol Sci. (2011) 119:3–19. doi: 10.1093/toxsci/kfq268
94. Bisht, S, Faiq, M, Tolahunase, M, and Dada, R. Oxidative stress and male infertility. Nat Rev Urol. (2017) 14:470–85. doi: 10.1038/nrurol.2017.69
95. Kou, X, Li, B, Olayanju, JB, Drake, JM, and Chen, N. Nutraceutical or pharmacological potential of Moringa oleifera lam. Nutrients. (2018) 10:343. doi: 10.3390/nu10030343
96. Kumar, N, Na, V, Gowda, KMD, Ahemed, B, and Ramaswamy, C. Role of ascorbic acid in monosodium glutamate mediated effect on testicular weight, sperm morphology and sperm count, in rat testis. J Chin Clin Med. (2008) 3:1–5.
97. Onyema, OO, Farombi, EO, Emerole, GO, Ukoha, AI, and Onyeze, GO. Effect of vitamin E on monosodium glutamate induced hepatotoxicity and oxidative stress in rats. Indian J Biochem Biophys. (2006) 43:20–4.
98. Biswas, SK. Does the interdependence between oxidative stress and inflammation explain the antioxidant paradox? Oxidative Med Cell Longev. (2016) 2016:5698931. doi: 10.1155/2016/5698931
99. Al-Taher, AY, Morsy, MA, Rifaai, RA, Zenhom, NM, and Abdel-Gaber, SA. Paeonol attenuates methotrexate-induced cardiac toxicity in rats by inhibiting oxidative stress and suppressing TLR4-induced NF-kappa B inflammatory pathway. Mediat Inflamm. (2020) 2020:8641026. doi: 10.1155/2020/8641026
100. Owumi, SE, Ochaoga, SE, Odunola, OA, and Farombi, EO. Protocatechuic acid inhibits testicular and epididymal toxicity associated with methotrexate in rats. Andrologia. (2019) 51:e13350. doi: 10.1111/and.13350
101. Kilany, OE, Abdelrazek, HMA, Aldayel, TS, Abdo, S, and Mahmoud, MMA. Anti-obesity potential of Moringa olifera seed extract and lycopene on high fat diet induced obesity in male Sprauge Dawely rats. Saudi J Biol Sci. (2020) 27:2733–46. doi: 10.1016/j.sjbs.2020.06.026
102. Kooltheat, N, Sranujit, RP, Chumark, P, Potup, P, Laytragoon-Lewin, N, and Usuwanthim, K. An ethyl acetate fraction of Moringa oleifera lam. Inhibits human macrophage cytokine production induced by cigarette smoke. Nutrients. (2014) 6:697–710. doi: 10.3390/nu6020697
103. Abdelhameed, RFA, Ali, AI, Elhady, SS, Abo Mansour, HE, Mehanna, ET, Mosaad, SM, et al. Marrubium alysson L. ameliorated methotrexate-induced testicular damage in mice through regulation of apoptosis and mi RNA-29a expression: LC-MS/MS metabolic profiling. Plants. (2022) 11:2309. doi: 10.3390/plants11172309
104. Kayode, OT, Rotimi, DE, Kayode, AAA, Olaolu, TD, and Adeyemi, OS. Monosodium glutamate (MSG)-induced male reproductive dysfunction: A Mini Review. Toxics. (2020) 8:10007. doi: 10.3390/toxics8010007
105. Nakanishi, Y, Tsuneyama, K, Fujimoto, M, Salunga, TL, Nomoto, K, An, JL, et al. Monosodium glutamate (MSG): a villain and promoter of liver inflammation and dysplasia. J Autoimmun. (2008) 30:42–50. doi: 10.1016/j.jaut.2007.11.016
106. Bailey, SA, Zidell, RH, and Perry, RW. Relationships between organ weight and body/brain weight in the rat: what is the best analytical endpoint? Toxicol Pathol. (2004) 32:448–66. doi: 10.1080/01926230490465874
107. Acikel-Elmas, M, Algilani, SA, Sahin, B, Bingol Ozakpinar, O, Gecim, M, Koroglu, K, et al. Apocynin ameliorates monosodium glutamate induced testis damage by impaired blood-testis barrier and oxidative stress parameters. Life. (2023) 13:822. doi: 10.3390/life13030822
108. Abdel Moneim, AE. Indigofera oblongifolia prevents lead acetate-induced hepatotoxicity, oxidative stress, fibrosis and apoptosis in rats. PLoS One. (2016) 11:e0158965. doi: 10.1371/journal.pone.0158965
109. Alalwani, AD. Monosodium glutamate induced testicular lesions in rats (histological study). Middle East Fertility Soc J. (2014) 19:274–80. doi: 10.1016/j.mefs.2013.09.003
110. Ullah, A, Munir, S, Badshah, SL, Khan, N, Ghani, L, Poulson, BG, et al. Important flavonoids and their role as a therapeutic agent. Molecules. (2020) 25:243. doi: 10.3390/molecules25225243
111. Abarikwu, SO, Njoku, RC, John, IG, Amadi, BA, Mgbudom-Okah, CJ, and Onuah, CL. Antioxidant and anti-inflammatory protective effects of rutin and kolaviron against busulfan-induced testicular injuries in rats. Syst Biol Reprod Med. (2022) 68:151–61. doi: 10.1080/19396368.2021.1989727
112. Khalifa, W, Ibrahim, F, El Makawy, A, Sharaf, H, Khalil, W, and Maghraby, N. Safety and fertility enhancing role of Moringa Oleifera leaves aqueous extract in New Zealand rabbit bucks. Int J Pharm. (2015) 6:156–68.
113. Shokry, DM, Badr, MR, Sakr, A-AM, Elmesiry, AM, Assy, MM, Rawash, Z, et al. Enhancement potential of Moringa oleifera leaves extract on buffalo bull cryopreserved semen quality and fertilization capacity. Anim Reprod Sci. (2024) 262:107414. doi: 10.1016/j.anireprosci.2024.107414
Glossary
4-HNE - 4-hydroxynonenal
ANOVA - Analysis of Variance
Bax - B-cell lymphoma protein 2 (Bcl-2)-associated X
Bcl-2 - B-cell lymphoma protein 2
CAGR - Compound Annual Growth Rate
CAT - Catalase
CONT - Control
COX-2 - Cyclooxygenase-2
ELISA - Enzyme-linked immunosorbent assay
FSH - Follicle-stimulating hormone
FTIR - Fourier transform infrared
GPx - Glutathione peroxidase
GR - Glutathione reductase
GSH - Reduced glutathione
H&E - Hematoxylin and Eosin
HPG - Hypothalamic–pituitary-gonadal
IL-1β - Interleukin-1 beta
LD50 - Lethal dose 50
LH - Luteinizing hormone
LOOHs - lipid hydroperoxides
LPO - Lipid peroxide
MAPK - Mitogen-activated protein kinase
MDA - Malondialdehyde
mL - Milliliter
MG - Monosodium glutamate
MO - Moringa oleifera
MOLE - Moringa oleifera leaf extract
mRNA - Messenger ribonucleic acid
NF-κB - Nuclear factor-kappa B
NO - Nitric oxide
Nrf2 - Nuclear factor erythroid 2–related factor 2
OS - Oxidative stress
P13K/PKB - Phosphatidylinositide 3-kinase/protein kinase B
PAS - Periodic acid-Schiff
PCNA - Proliferating cell nuclear antigen
ROS - Reactive oxygen species
RUT - Rutin
RWT - Relative testicular weight
SOD - Superoxide dismutase
TNF-α - Tumor necrosis factor-alpha
USD - United States dollar
ZEN - Zearalenone
Keywords: antioxidants, male reproductive system, oxidative stress, inflammation, histology, histochemistry, immunohistochemistry
Citation: Abdel-Aty DM, Ibrahim MA, Mohamed SR, El-Khadragy MF, Abdel Moneim AE, Fathalla AS and Soliman D (2025) Rutin and Moringa oleifera leaf extract prevent monosodium glutamate-induced testicular toxicity in adult male albino rats. Front. Vet. Sci. 12:1566471. doi: 10.3389/fvets.2025.1566471
Edited by:
Ramesh Chandra Gupta, Murray State University, United StatesReviewed by:
Sabreen Ezzat Fadl, Matrouh University, EgyptGhadeer Abdelaal, Zagazig University, Egypt
Copyright © 2025 Abdel-Aty, Ibrahim, Mohamed, El-Khadragy, Abdel Moneim, Fathalla and Soliman. This is an open-access article distributed under the terms of the Creative Commons Attribution License (CC BY). The use, distribution or reproduction in other forums is permitted, provided the original author(s) and the copyright owner(s) are credited and that the original publication in this journal is cited, in accordance with accepted academic practice. No use, distribution or reproduction is permitted which does not comply with these terms.
*Correspondence: Ahmed E. Abdel Moneim, YWVzdDE5NzdAaG90bWFpbC5jb20=; YWhtZWRfYWJkZWxtb25laW1Ac2NpZW5jZS5oZWx3YW4uZWR1LmVn