- 1Instituto de Investigación en Ganadería y Biotecnología, Facultad de Ingeniería Zootecnista, Agronegocios y Biotecnología, Universidad Nacional Toribio Rodríguez de Mendoza de Amazonas, Chachapoyas, Peru
- 2Laboratorio de Fisiología Molecular, Instituto de Investigación en Ganadería y Biotecnología, Facultad de Ingeniería Zootecnista, Agronegocios y Biotecnología, Universidad Nacional Toribio Rodríguez de Mendoza de Amazonas, Chachapoyas, Peru
- 3Faculdade de Medicina Veterinária e Zootecnia (FMVZ), Universidade Federal de Uberlândia (UFU), Uberlândia, MG, Brazil
- 4Department of Basic Medical Sciences, College of Veterinary Medicine, Purdue University, West Lafayette, IN, United States
- 5Facultad de Medicina, Universidad Nacional Toribio Rodríguez de Mendoza de Amazonas, Chachapoyas, Peru
- 6Facultad de Ingeniería Zootecnista, Agronegocios y Biotecnología, Instituto de Investigación en Ganadería y Biotecnología, Universidad Nacional Toribio Rodríguez de Mendoza de Amazonas, Chachapoyas, Peru
- 7Facultad de Ingeniería Zootecnista, Agronegocios y Biotecnología, Universidad Nacional Toribio Rodríguez de Mendoza de Amazonas, Chachapoyas, Peru
Anaplasmosis remains a significant threat to livestock production in tropical regions, particularly in the Amazon basin, where ecological complexity and limited veterinary infrastructure challenge effective disease management. This review focuses on Anaplasma marginale and Anaplasma phagocytophilum, the primary species associated with bovine and granulocytic anaplasmosis, respectively. We examine the current state of diagnostic tools, highlighting the limited accessibility of molecular techniques in rural settings and the emerging but underutilized potential of technologies. Persistent infection and antigenic variation are explored as major obstacles for disease eradication and vaccine development. Although live attenuated and inactivated vaccines are in use for A. marginale, none provide sterilizing immunity, and no commercial vaccines exist for A. phagocytophilum. The review evaluates recent advances in recombinant antigens, chimeric constructs, and genetically attenuated strains, as well as future directions involving multiepitope design, novel adjuvants, and next-generation vaccine platforms. Additionally, we assess the role of tick control in disease prevention and emphasize the importance of integrated strategies in regions like the Amazon. Together, these findings underscore the need for context-specific solutions that address the ecological and epidemiological complexity of anaplasmosis in the Amazon basin.
1 Introduction
Livestock diseases pose a significant challenge to animal health and threaten the economic stability of agricultural sectors worldwide. Effective management of these diseases is crucial for ensuring the well-being of livestock and maintaining the productivity and profitability of the agricultural industry.
The largest cattle population in South America is found in Brazil (238,626,442 head), followed by Argentina (54,460,799 head), Colombia (27,239,767 head), Paraguay (13,801,993 head), Uruguay (11,400,963 head), and Peru (5,160,000 head). Cattle rearing is a significant economic activity in these countries, which are major players in the agribusiness sector (1). The total bovine population in South America is approximately 384 million, accounting for 25% of the world’s cattle inventory. This makes the region a major contributor to the global beef market, playing a vital role in beef exports (2).
Among the diseases that significantly affect livestock production is anaplasmosis, which disrupts production and reduces yields in milk and meat. Additionally, this disease incurs high costs associated with veterinary care and treatment (3). Anaplasmosis is a vector-borne disease caused by bacteria of the genus Anaplasma, obligate intracellular pathogens belonging to the family Anaplasmataceae. These bacteria infect a wide range of vertebrate hosts, including both wild and domesticated mammals, as well as some birds. Their transmission typically occurs through tick vectors, contributing to the spread and persistence of the disease in diverse ecological settings. Anaplasmosis has been reported in cattle, buffalo, sheep, goats, and some non-domestic ruminants (4–6). It is primarily transmitted by ticks of the genera Ixodes, Rhipicephalus, and Dermacentor (7). Key species include Anaplasma marginale (bovine anaplasmosis), Anaplasma phagocytophilum (granulocytic anaplasmosis in humans and animals), Anaplasma platys (infects platelets in dogs), Anaplasma ovis and Anaplasma capra (affecting small ruminants) (5, 7, 8).
In the Amazonian region, anaplasmosis represents a complex veterinary and zoonotic concern (9), where not only biodiversity and climate play a role, but also cultural practices such as animal trade, uncontrolled livestock movement between regions, and limited implementation of anti-tick control programs contribute to the maintenance of these pathogens in local herds (10).
The Amazon basin covers an area of about 7 million km2, with Amazon forests comprising approximately 5.3 million km2–40% of the world’s tropical forest area (11) (Figure 1). Recent studies suggest unique transmission cycles in wild animals, as evidenced by novel genetic variants of Anaplasma in the Amazon basin. For instance, Candidatus Anaplasma sparouinense, identified in French Guiana, is an emerging species associated with human infections and sloths (12).
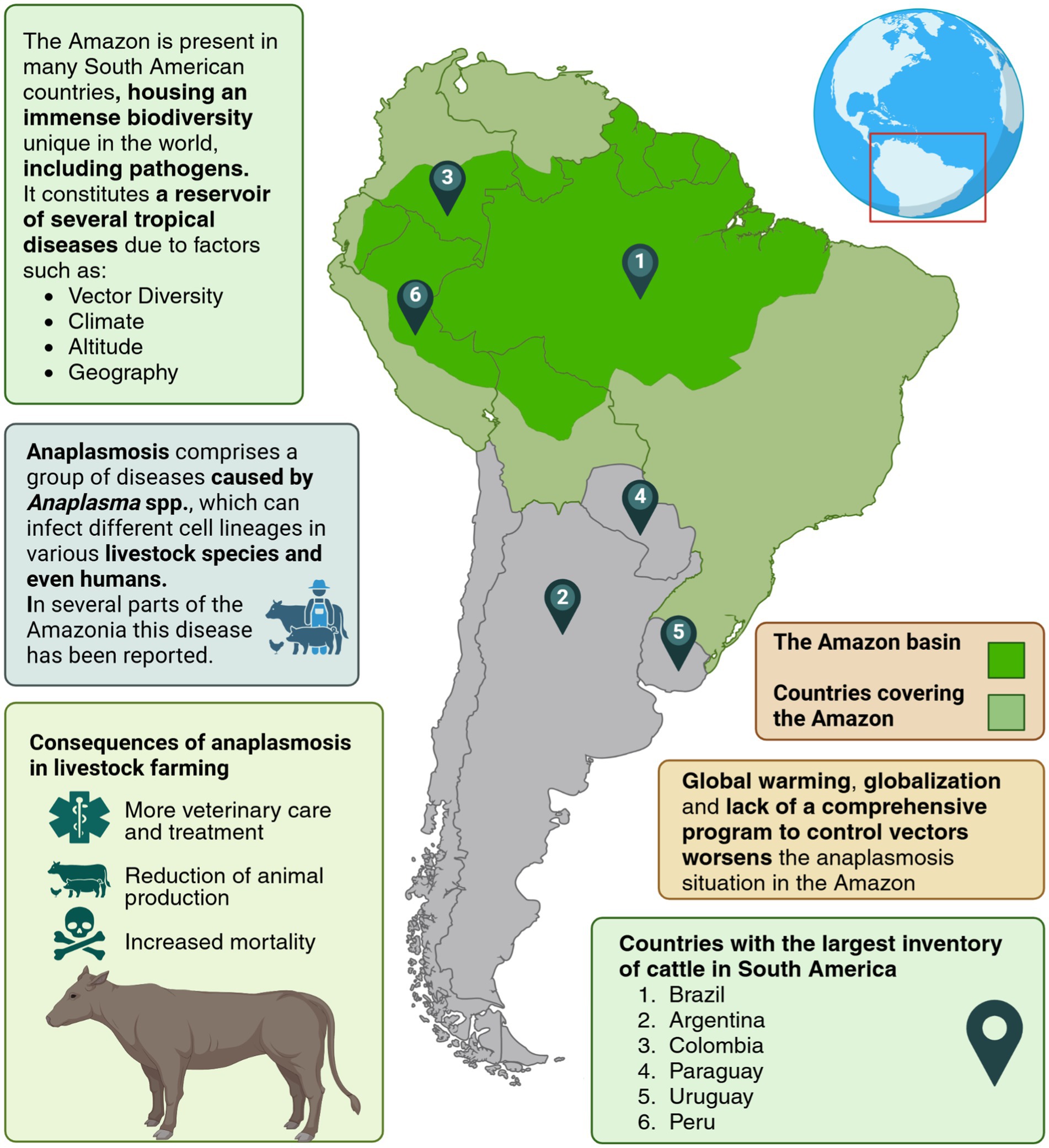
Figure 1. Epidemiology of anaplasmosis in the Amazon: a vector-borne disease affecting livestock. Created in https://BioRender.com.
In the Brazilian Amazon, the presence of A. marginale and A. phagocytophilum has been confirmed in both domestic and wild animals, highlighting the potential risk of zoonotic transmission (12, 13). The region’s high biodiversity of vectors, particularly ticks and tropical climate, facilitates the propagation of these pathogens, making anaplasmosis a disease of significant concern (12). Additionally, other types of transmission have been reported, apart from vectors, such as transplacental in cattle (14) and possibly nosocomial (15, 16).
In Peru, anaplasmosis causes substantial economic losses, particularly in the Peruvian Amazon. A study in the districts of Omia and Molinopampa in Amazonas reported a 67% prevalence of A. marginale in Simmental cattle, emphasizing its negative impact on milk and meat production (17). Small and medium-scale producers are especially vulnerable, as the associated veterinary and treatment costs further strain their resources. These challenges are exacerbated by geographic factors, such as an altitude of 1701 to 2000 meters above sea level, which influences the disease’s prevalence (17).
In South America, the combined economic losses from anaplasmosis and babesiosis are estimated at $875 million annually, with Brazil alone reporting losses of $3.24 billion due to Rhipicephalus microplus and A. marginale. In Mexico, the economic toll is approximately $942.23 million, excluding associated costs such as antibiotics and treatments (18).
2 Anaplasmosis in livestock: clinical symptoms and impacts
A. marginale is the most common pathogen in bovines, primarily infecting erythrocytes and causing severe anemia, fever, jaundice, lethargy, and weight loss (19). These clinical symptoms lead to significant economic losses due to decreased productivity and increased mortality, particularly in regions like the Amazon basin, where small-scale livestock producers face limited access to veterinary care. The transmission of Anaplasma spp. occurs mainly through tick bites, with vectors such as Rhipicephalus microplus, Dermacentor andersoni, Rhipicephalus sanguineus, and Ixodes ricinus. In the Amazon, the high biodiversity of wildlife also serves as reservoirs, complicating disease management in livestock populations (20, 21) (Figure 2A).
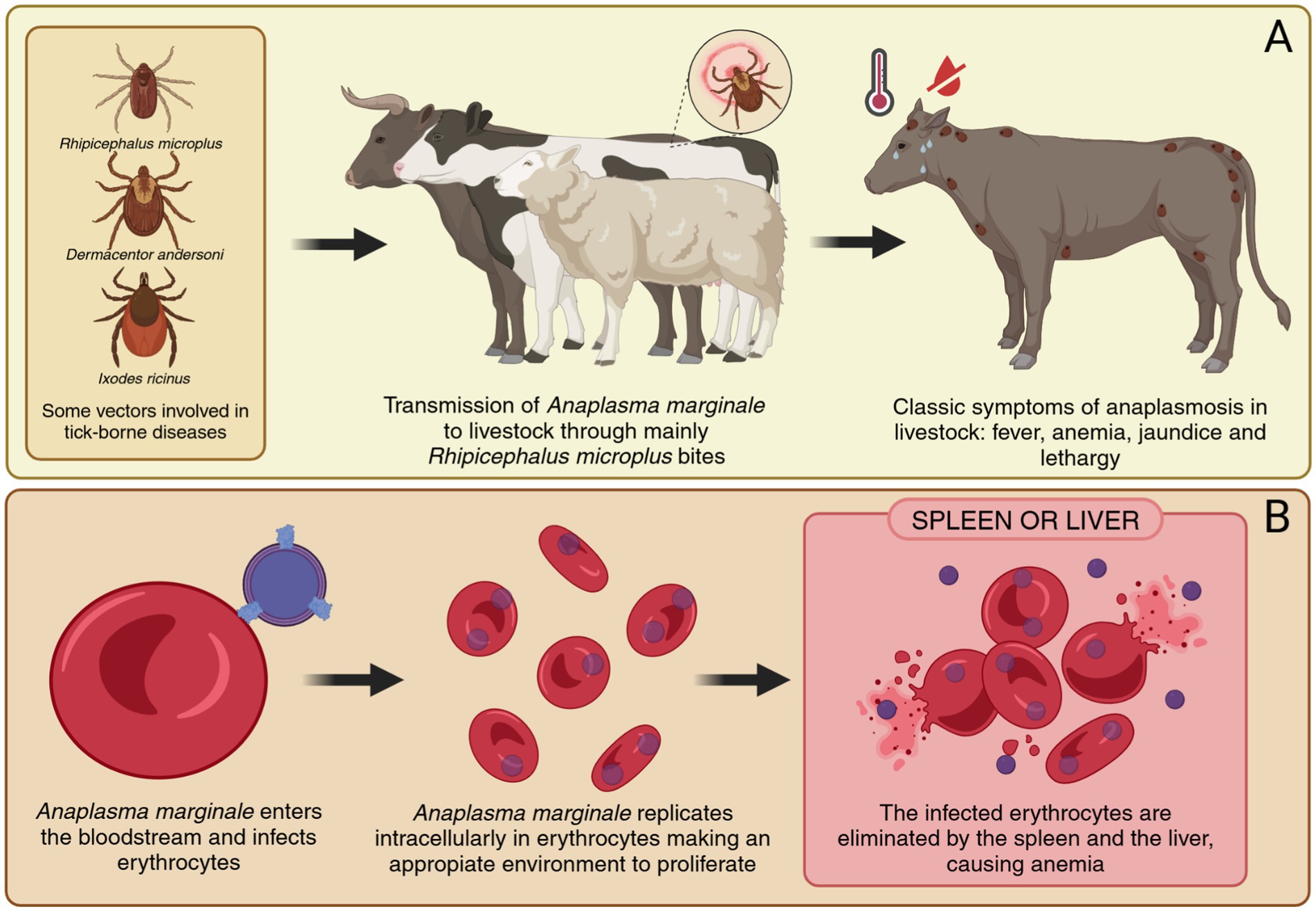
Figure 2. Transmission and pathogenesis of anaplasmosis in livestock caused by A. marginale. (A) Transmission of A. marginale through tick bites, mainly by Rhipicephalus microplus, and onset of clinical symptoms in livestock. (B) Progression of A. marginale infection, including immune evasion, replication in erythrocytes, and destruction of infected erythrocytes. Created in https://BioRender.com.
The pathogenesis of anaplasmosis involves sophisticated interactions between Anaplasma pathogens and the host immune system. Several species of the genus Anaplasma are known to infect different hosts and exhibit distinct cellular tropisms. A. marginale primarily affects cattle and invades erythrocytes, while A. phagocytophilum has a broad host range. Including humans, dogs, horses, cattle, sheep, goats, deer, and small mammals, and specifically targets neutrophils and other granulocytes. A. platys infects dogs and parasitizes platelets, whereas A. ovis is found in sheep and goats, also targeting erythrocytes. A. centrale, a naturally less virulent species used as a live vaccine, infects bovine erythrocytes. In contrast, A. bovis has been detected in cattle, buffalo, goats, deer, and dogs, and exhibits a tropism for monocytes (22).
In A. marginale, major surface proteins (MSPs) are located in the outer membrane of the bacterium, allowing direct interaction with host cells. Specifically, MSP1a and MSP1b mediate adhesion to bovine erythrocytes and to the gut epithelial cells of tick vectors, facilitating both infection and transmission (23). Additionally, MSP1a plays a key role in strain characterization and epidemiological studies, as its tandem repeat sequences vary among isolates and reflect geographic and biological diversity (23).
A. marginale evades immune detection through antigenic variation, particularly in MSP2 and MSP3, which are encoded by multigene families subject to recombination and sequential expression. This mechanism enables the pathogen to persist within the host by continuously altering its surface antigens, escaping recognition by the immune system (24). In contrast, MSP4 is a conserved protein encoded by a single gene, serving as a stable molecular marker for species identification but lacking evidence of antigenic variability (25). This intracellular survival, coupled with the ability to downregulate pro-inflammatory cytokines and manipulate immune cell functions, creates a favorable environment for the pathogen’s replication and survival (26, 27). These mechanisms not only facilitate chronic infections but also pose significant challenges for vaccine development and therapeutic strategies (Figure 2B).
In the Amazon region, the warm climate and high biodiversity create ideal conditions for the proliferation of vectors, including ticks of the genus R. microplus (formerly Boophilus microplus) and Dermacentor, which are key vectors for A. marginale. These ticks are also vectors for other bacteria, adding complexity to the control of tick-borne diseases (28).
In the Brazilian Pantanal, part of the Amazon biome, A. marginale is highly prevalent among beef cattle, with studies indicating 72.2% seropositivity using iELISA and 56.7% positivity via qPCR targeting the msp1β gene (29). This prevalence highlights the role of the tropical climate, which favors the proliferation of tick vectors such as R. microplus and Amblyomma sculptum. Cows acted as chronic carriers, perpetuating the pathogen, while male calves sold to other regions posed a risk of disseminating diverse strains of A. marginale. These findings underline the disease’s persistence in tropical regions and its economic implications.
In northeast Brazil, specifically in the region of Médio Mearim, Maranhão state, A. marginale was detected in 95.32% of the dairy cattle using qPCR targeting the gene msp1β, while 81.34% of the samples were seropositive in iELISA assays using the recombinant protein MSP5. The high seropositivity in cows compared to calves suggest an cumulative exposure to the pathogen through time. These findings reflect the high prevalence of A. marginale in Amazon-Cerrado transition zones, where the environmental conditions enhance the proliferation of vectores like R. microplus (30).
3 The role of molecular techniques in advancing anaplasmosis detection and control
Molecular techniques have significantly advanced the detection and control of anaplasmosis by enabling precise identification of Anaplasma species, even in subclinical cases (31, 32). Tools like PCR, qPCR, and LAMP enhance diagnostic accuracy and support surveillance in endemic areas. Their strategic application improves early intervention and disease management. Despite challenges, they bridge research and practical control efforts (33).
The range of diagnostic techniques for Anaplasma detection spans from conventional PCR methods to more advanced genomic tools. While PCR-based assays are commonly used in research and reference laboratories due to their sensitivity and specificity, their routine application in bovine anaplasmosis diagnosis remains limited in many endemic regions because of cost and infrastructure constraints. In field settings, diagnosis still often relies on clinical signs and microscopy. On the other hand, whole-genome sequencing and metagenomic approaches are primarily restricted to experimental or epidemiological studies, where they offer valuable insights into pathogen diversity and host–vector dynamics (34). Nevertheless, the adoption of these molecular tools in routine veterinary practice remains challenging, and their implementation is often restricted to government programs or academic research.
In contrast, serological tests such as ELISA, complement fixation, and card agglutination are currently the most accessible and widely used diagnostic methods for bovine anaplasmosis. These tests provide cost-effective and practical alternatives for detecting exposure to Anaplasma, especially in rural or resource-limited settings, and continue to be essential components of surveillance and herd-level screening.
Moreover, the integration of molecular diagnostics into epidemiological frameworks has enhanced the ability to detect subclinical infections and understand pathogen dynamics within ecosystems (35). These advancements enable early intervention strategies and contribute to the formulation of targeted control measures, ultimately reducing the burden of anaplasmosis on animal health, productivity, and public health (36). The versatility and adaptability of molecular techniques underscore their critical role in bridging scientific research and practical solutions for disease management (Table 1).
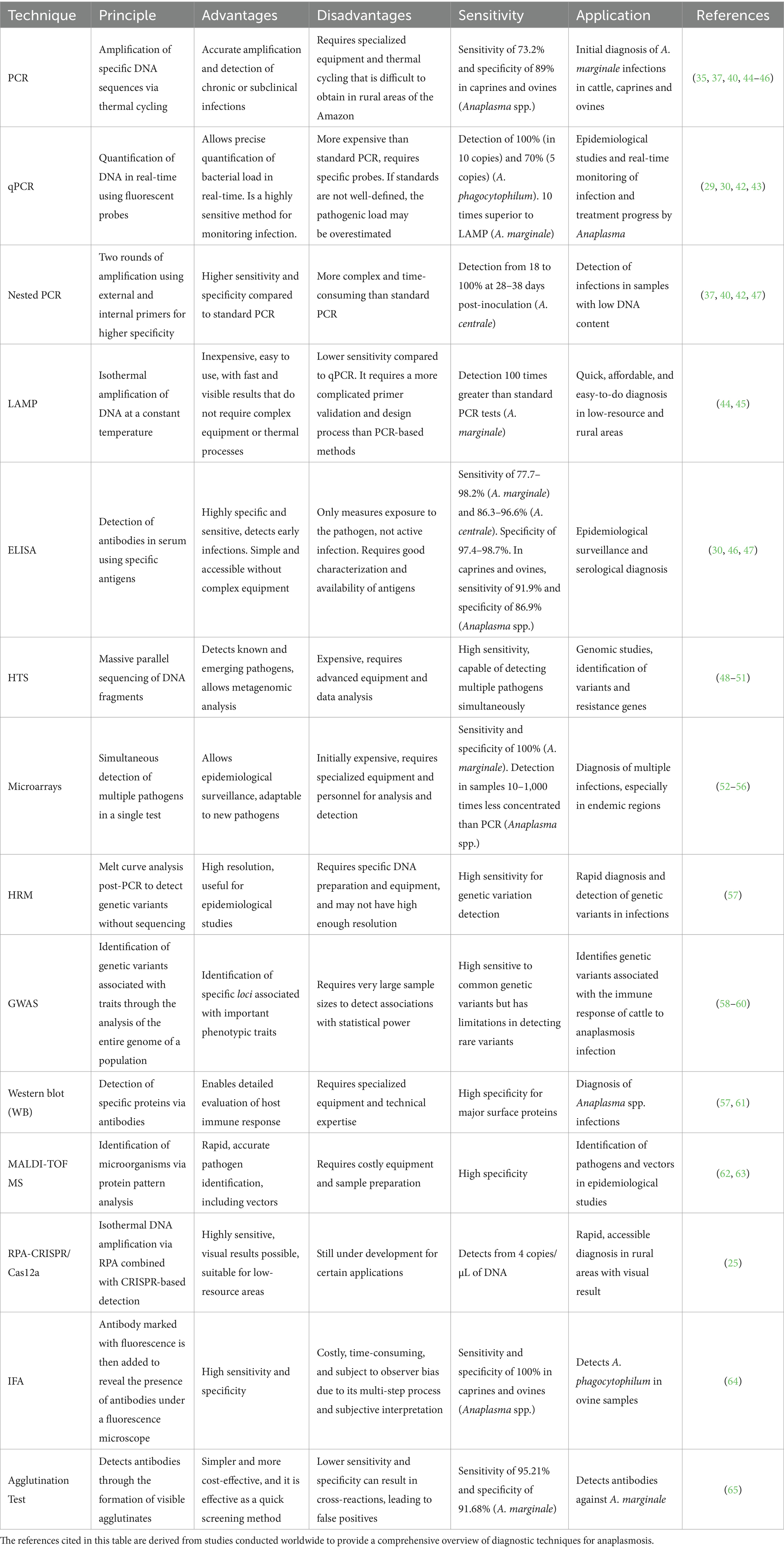
Table 1. Comparative overview of diagnostic techniques for anaplasmosis: global perspectives on principles, applications, and advances.
3.1 PCR, qPCR, and nested PCR
3.1.1 PCR
Polymerase Chain Reaction (PCR) is an essential technique for the molecular identification of A. marginale, because it facilitates the amplification of specific sequences in the DNA which allows the detection of this pathogen in blood samples. This technique is especially useful in chronic or subclinical infections, in which the bacterial load may be low, which makes its detection harder by traditional methods (37). In the case of A. marginale, genes such as msp4 are commonly used as targets in PCR due to their high conservation and usefulness in molecular detection and phylogenetic analysis, rather than a direct role in pathogenesis (37). Other genes like 16S rRNA, msp1α, msp1β, groEL, and gltA have also been employed in PCR and qPCR assays for the detection of anaplasmosis (38–41). In Brazil, combinations of msp1α, msp1β, 16S rRNA, and msp4 have proven effective in characterizing the genetic diversity and phylogeny of A. marginale strains (41).
3.1.2 Quantitative PCR—qPCR
qPCR is an evolution of the conventional PCR because it does not only detect but also quantifies the DNA of Anaplasma in real time, using fluorescent probes which allow it to observe the process in real time. An example of its application is the use of a TaqMan probe in the qPCR to detect the gen msp1b of A. marginale in livestock’s blood sample (30, 42). The qPCR is especially useful in epidemiologic studies and the evaluation of the efficacy of treatments because it allows the detection of sub clinic infections and quantification of the bacterial load, which is essential for the control of anaplasmosis in endemic areas (42).
To identify A. marginale, de Souza Ramos et al. (29) employed a multi-faceted molecular approach. qPCR targeting the msp1β gene achieved high sensitivity, while semi-nested PCR for the msp1α gene revealed 14 distinct strains, including eight novel ones. The use of tools like the RepeatAnalyzer software (43) for analyzing tandem repeats in the msp1α gene further enhanced genetic diversity assessment. Such methodologies are pivotal for surveillance and control, especially in regions like the Amazon, where environmental factors and genetic variability challenge disease management.
3.1.3 Nested PCR
Nested PCR is a variant of the PCR which increases the sensibility and specificity of the conventional technique by two rounds of amplification. In the first round, external primers are used to amplify a specific region of the DNA, and in the second round, internal primers are used to amplify a fragment within the product of the first PCR. This method is particularly useful to detect A. marginale in samples with low load of pathogens or when the DNA quantity is limited (37). Nested PCR has proven to be effective in the identification of infections in animals and ticks, identifying species like A. ovis and A. marginale which is important in epidemiologic and disease control studies in endemic regions (37).
Standard PCR, qPCR and Nested PCR are complementary techniques in the detection of Anaplasma sp. Standard PCR is useful for initial detection and confirmation of infections while qPCR offers advantages in the quantification and detection of sub clinical infections, which is crucial to surveil the progression of the disease and effectiveness of the treatment (40). On the other hand, Nested PCR gives more sensibility, making it ideal for the detection of infections in samples with low load of pathogens (42).
3.2 LAMP (Loop-Mediated Isothermal Amplification)
Loop-Mediated Isothermal Amplification (LAMP) has demonstrated promising potential for the detection of A. marginale, particularly in low-resource settings. Unlike conventional PCR, which requires repetitive thermal cycling, LAMP operates at a constant temperature, reducing the need for costly equipment such as thermocyclers (44). However, its application to bovine anaplasmosis remains limited, with only a few published studies to date. Further validation and broader adoption are needed before it can be considered a routine diagnostic tool.
The principle of LAMP involves the use of 4–6 primers that recognize distinct sequences within the target DNA. This not only enhances specificity but also accelerates the amplification process, enabling DNA detection in less than an hour. For A. marginale, LAMP assays have been developed using genes such as msp5 and msp1b, which are highly specific to this species (45). Compared to conventional PCR, LAMP offers the additional advantage of allowing visual detection of amplified products, thereby eliminating the need for supplementary techniques like gel electrophoresis (45).
Recent studies have demonstrated that LAMP can be as or even more sensible than conventional PCR for the detection of infections by A. marginale. Giglioti et al. (44) discovered that LAMP can detect from 211 copies/μL of A. marginale DNA, while PCR can detect from 21 copies/μL, which means that although LAMP is highly specific, it has less sensibility than qPCR (44). However, LAMP is still extremely useful in low-income areas due to its simplicity and quick detection without specialized machines.
Another study by Ganguly et al. (45) compared the efficacy of LAMP and conventional PCR in infected livestock samples in Gujarat, India. This study demonstrated that LAMP detected A. marginale in 22.14% of the samples, in comparison with 15.36% detected by PCR, which suggests a higher sensibility in LAMP technique (45). Also, LAMP has demonstrated a high specificity, given that no cross-amplification was observed with other hemiparasites like Babesia bigemina or Theileria annulate.
3.3 ELISA (enzyme-linked immunosorbent assay)
ELISA is a serologic technique widely used for the detections of antibodies against A. marginale in livestock, and it’s one of the most common tools for the surveillance and diagnostics of anaplasmosis. This technique detects specific antibodies in the serum of animals, giving an indirect measure of the exposition to the pathogen (46).
There are different variants of ELISA, including competitive ELISA (cELISA) and indirect ELISA (iELISA). cELISA is one of the most used for antibodies detection against A. marginale due to its high sensibility and specificity. In this assay, monoclonal antibodies directed against Major Surface Proteins like MSP5, which is highly conserved in A. marginale, are used (30, 46). The antibodies that are in the serum of the animals compete with a reference antibody that is marked to bind the antigen, which allows the detection of infections even in the early stages (46).
A more recent advancement is the Double Antibody Sandwich ELISA (dasELISA), which has proven effective in differentiating between animals infected with A. marginale and those vaccinated with A. centrale, a related but less virulent species commonly used in vaccines worldwide (47). This method employs two variants of the MSP5 protein, one specific to A. marginale and another to A. centrale, to capture and detect specific antibodies in the serum dasELISA has demonstrated not only high sensitivity and specificity but also earlier detection compared to conventional ELISA methods (47).
In comparative studies, cELISA has demonstrated a sensibility of 91.9% and a specificity of 86.9% in the diagnosis of Anaplasma sp. in sheep and goats, which reaffirms its use in the diagnosis of this infection in different animals (46). However, the ability of dasELISA to differentiate between species of Anaplasma sp. and detect infections in vaccinated animals, turns this into a useful tool in specific epidemiologic contexts.
3.4 High-throughput sequencing
High-Throughput Sequencing (HTS) has revolutionized the identification and characterization of pathogens, including A. marginale. Unlike traditional techniques, HTS allows massive and parallel DNA fragments sequencing, giving an integral vision of the microbiome and allowing an easier detection of well-known and emerging pathogens in complex samples (48, 49).
High-throughput sequencing (HTS) offers significant advantages for the metagenomic detection of tick-borne pathogens, particularly in complex infections where multiple agents may be present. Unlike conventional PCR, which targets specific sequences, HTS enables the comprehensive sequencing of all genetic material in a sample, eliminating the need for prior pathogen isolation or identification. This approach is especially useful in cases of low bacterial load or when the etiological agent is unknown. For example, in equine samples with suspected tick-borne diseases, HTS successfully identified A. phagocytophilum in cases that were previously PCR-negative, demonstrating its superior sensitivity and diagnostic utility (48).
Another significant advantage of HTS in metagenomic shotgun analysis is its potential to identify antimicrobial resistance genes and virulence factors, offering critical insights for the clinical management of diseases. Although the study by Subbiah et al. (48) did not detect such elements in their analysis, it emphasized that metagenomic approaches could eventually facilitate the detection of resistance determinants and virulence markers in tick-borne pathogens. This capability underscores the broader role of HTS, not only in diagnosis but also in supporting evidence-based treatment and control strategies.
Also, its high sensitivity allows the identification of strains and genetic variation of Anaplasma sp., which is crucial for the epidemiologic surveillance and comprehension of disease evolution. For instance, the sequencing of the whole genome of A. marginale has allowed the identification of variations in the MSPs, which are associated with the virulence and ability of the pathogen to evade the host’s immune system (50).
However, HTS also faces challenges, such as high costs, the need for specialized equipment and software for data analysis, and the requirement for skilled personnel to handle and interpret the data. Also, the interpretation of the results can be complex due to the high amount of generated data and the presence of environmental or background DNA in the samples (51). Despite this limitation, HTS is still a powerful tool for the identification and characterization of A. marginale and its use in the investigation and diagnosis of anaplasmosis is developing.
3.5 Microarray genotyping
The technology of microarrays has proven to be a powerful tool for detecting multiple pathogens in complex samples, including A. marginale. This technology allows for the simultaneous detection of different species and strains of pathogens in a single test, which is particularly useful in areas where multiple infections are common, such as endemic regions for tick-borne diseases (52).
For instance, low-density microarrays have been developed to detect bacterial pathogens and piroplasmids transmitted by ticks in African livestock. Designed to identify species within the Anaplasma genus alongside other pathogens, these microarrays have shown greater sensitivity than conventional techniques like PCR or Sanger sequencing, successfully detecting infections that those methods failed to identify (53).
Furthermore, the use of microarrays in diagnosing anaplasmosis not only enables the identification of active infections but also facilitates epidemiological surveillance by providing data on the prevalence and distribution of various Anaplasma species. Additionally, microarrays can be adapted to incorporate new and emerging pathogens, making this technology a flexible and valuable tool for safeguarding animal health in endemic regions.
While HTS and digital PCR are more sensitive and comprehensive, offering greater resolution and the ability to detect low-abundance pathogens with higher precision (54), microarrays still hold significant utility in certain contexts. Specifically, microarrays remain advantageous when the simultaneous detection of multiple pathogens is required, particularly in resource-limited settings where cost efficiency is essential (55). Their ability to screen for a wide range of pathogens in a single test, combined with relatively lower operational costs (56), makes them a valuable option for diagnostic applications in endemic regions or for large-scale epidemiological surveillance.
3.6 HRM (high-resolution melting) in the detection of Anaplasma sp.
The HMR technique has turned into a valuable technique for the detection and characterization of genetic variations in pathogens like A. phagocytophilum. This technique is based on the amplification of specific sequences of the DNA followed by an analysis of the fusion curves of the DNA, which allows the differentiation of different genetic variations based on differences of the sequence of nucleotide bases (57).
This technique, HRM, has been used to analyze blood samples and ticks infected with A. phagocytophilum in Laikipia, Kenia. This study demonstrated the efficacy of HRM to detect not only A. phagocytophilum but also to distinguish between different haplotypes of the pathogens in mammals and associated ticks (57). The ability of HRM to identify genetic variants in the AND sequence allows a detailed analysis of the epidemiology and transmission dynamics of Anaplasma species in environments where multiple hosts and vectors coexist.
3.7 Genome-wide association studies
The use of GWAS has opened new possibilities to understand the genetic basis of the susceptibility and resistance to diseases like anaplasmosis in livestock. GWAS allow the identification of specific loci associated with important phenotypic traits, like the resistance to tick-borne pathogens, which include Anaplasma sp. (58).
GWAS have demonstrated to be effective in discovering genetic variants that can influence the presence or absence of pathogens like A. marginale in livestock. Recent studies have identified a locus in the bovine genome that is associated with the resistance to tick-borne diseases. In a meta-analysis of GWAS done in African livestock, significant peaks were found in the chromosomes 8 and 24, which are related to the resistance to A. marginale and other hemiparasites transmitted by ticks (58).
These discoveries are important to develop strategies for genetic Markers Assisted Selection (MAS), which could enhance the livestock’s natural resistance to anaplasmosis. More importantly, GWAS allow the researchers to identify genetic variants that noy only are responsible for the disease resistance, but also the livestock’s ability to tolerate infections without showing severe symptoms (59).
GWAS has revealed that certain genetic variants are associated with livestock’s immune response to A. marginale infection. These findings could be utilized to develop genetic improvement programs, selecting animals with greater resistance to anaplasmosis, which would reduce dependence on chemical treatments and enhance the sustainability of livestock production (60). Integrating GWAS into the study of anaplasmosis provides a deeper genetic understanding of disease resistance, which is essential for developing more effective and sustainable control strategies in the livestock industry.
3.8 Immunoblotting, also known as Western blot
WB technique has been used for the specific detection of MSP in infections caused by Anaplasma spp. This technique is useful for the diagnosis of infections caused by A. platys and A. phagocytophilum. Immunoblotting allows the accurate identification of antibodies in the serum of infected animals, providing a detailed evaluation of the host’s immune response.
Mosha et al. (57) used WB along with molecular techniques to confirm the presence of A. phagocytophilum in small mammals and ticks, pointing out the versatility of WB in the detection of infection in multiple species.
Also, Lai et al. (61) developed a specific WB test for A. platys, using MSP antigens. This study highlights the importance of immunoblotting in the differentiation of Anaplasma species, which is crucial for the accurate diagnosis and disease control in production animals.
3.9 Matrix-assisted laser desorption/ionization time-of-flight mass spectrometry
MALDI-TOF MS has gained popularity for its rapid and accurate pathogen identification. It quickly compares protein mass spectra to reference databases, allowing precise identification of bacteria, fungi, and other microbes. This method is highly valuable in clinical microbiology due to its speed, precision, and cost-effectiveness in diagnosing infections, including those causing anaplasmosis. MALDI-TOF MS enables the identification of microorganisms by analyzing specific protein patterns present in samples. Recent studies have demonstrated its high specificity and sensitivity, such as in the identification of A. phagocytophilum in blood and tissue samples (62).
Additionally, MALDI-TOF MS has been successfully used in identifying tick species that serve as vectors for Anaplasma spp., which is crucial for understanding the epidemiology of tick-borne diseases. Hamlili et al. (63) demonstrated its use in identifying ticks from the genus Hyalomma and detecting A. platys in dromedary camels in Algeria. This ability to identify both the vector and the associated pathogen highlights the method’s versatility in epidemiological studies and diagnostics.
The high-resolution and quick analysis of MALDI-TOF MS turns this technique into a promising tool not only for the identification of pathogens but also for the disease’s surveillance and the investigation of new infection biomarkers.
3.10 Recombinase polymerase amplification—clustered regularly interspaced short palindromic repeats associated protein 12a
Recent advances in the detection of A. marginale include the use of RPA-CRISPR/Cas12a, which offers a highly sensitive and specific alternative to conventional methods like PCR. This technology combines isothermal DNA amplification via Recombinase Polymerase Amplification (RPA) with CRISPR/Cas12a-based detection, targeting the msp4 gene of A. marginale. Recent studies have shown that this technique can detect quantities as low as 4 copies/μl of the pathogen’s DNA, demonstrating sensitivity comparable to conventional PCR (25). A significant advantage of this technique is that its lyophilized components can be stored at room temperature, making it easier to use in rural areas and offers a good cost benefit. Additionally, it can be adapted to provide visual results through fluorescence, making it a valuable tool for the quick and affordable diagnosis of bovine anaplasmosis in rural settings (25).
3.11 Indirect Immunofluorescence Assay
Indirect Immunofluorescence Assay (IFA) is an important method for detecting Anaplasma spp. in animals, with its own advantages and disadvantages. IFA uses antigens fixed on slides, and if specific antibodies are present in the patient’s serum, they bind to the antigens. A secondary antibody marked with fluorescence is then added to reveal the presence of antibodies under a fluorescence microscope. This technique offers high sensitivity and specificity, making it very effective for detecting chronic infections like anaplasmosis. However, it is an expensive technique, requiring specialized equipment and training, and it is time-consuming due to the various steps involved. A recent study highlighted the efficacy of IFA in detecting A. phagocytophilum in ovine samples, with a sensitivity of 85.4% and a specificity of 100%, confirming its diagnostic accuracy (64).
3.12 The Agglutination Test
The Agglutination Test, on the other hand, is simpler and more cost-effective, making it widely used in field settings and in laboratories with limited resources. It detects antibodies through the formation of visible agglutinates when the patient’s serum reacts with antigens fixed on particles. While it is less sensitive than IFA, it is effective as a quick screening method. A successful example of this test is the development of a latex agglutination test for detecting antibodies against A. marginale, which showed good results in cattle (65).
4 Therapeutic and preventive strategies and recent advances
4.1 Antibiotics
The use of tetracyclines such as oxytetracycline and chlortetracycline remains essential in the treatment of bovine anaplasmosis. These antibiotics inhibit bacterial protein synthesis by targeting the 30S ribosomal subunit and are effective in reducing clinical signs during the acute phase. However, they do not eliminate A. marginale completely. In a study performed by Curtis et al. (66), cattle treated for 60 days remained asymptomatic carriers, highlighting the pathogen’s ability to persist despite prolonged treatment.
This persistence is not solely due to antimicrobial resistance. A. marginale exhibits several mechanisms that favor chronic infection: low bacterial loads below therapeutic thresholds, antigenic variation in msp2 and msp3 genes, and intracellular localization in erythrocytes that hinders antibiotic penetration (67). Additionally, incomplete immune responses and the risk of reinfection by vectors in endemic areas make eradication even more difficult. Importantly, while tetracyclines such as oxytetracycline and chlortetracycline remain effective for treating acute episodes, they are insufficient to eliminate carrier status, allowing the pathogen to persist within herds (66, 67).
Although no conclusive phenotypic resistance to tetracyclines has been documented in A. marginale, a recent study by Shahbazi et al. (68) identified the resistance-associated genes otrA and otrB in A. marginale, A. ovis, and A. centrale isolated from cattle blood samples. These genes are linked to ribosomal protection and efflux mechanisms, respectively, and their presence suggests a potential for the development of resistance under sustained antimicrobial pressure. To date, no treatment failures attributable to these genes have been reported, but their detection highlights the importance of continuous surveillance.
Alternative treatments include imidocarb dipropionate, which has been used with variable success, particularly in cases of co-infection. However, studies show that it does not consistently eliminate A. marginale, and oxytetracycline remains more effective in achieving chemosterilization in some cases (69). Therefore, imidocarb can be used as an alternative, specially when there are co infections but it does not replace the use of tetracyclines as base therapy.
In turn, doxycycline is the treatment of choice for A. phagocytophilum in humans and companion animals, due to its superior intracellular penetration. Although its use in ruminants is off-label, it has shown efficacy comparable to oxytetracycline in A. ovis infections (70).
4.2 Vaccines and innovative approaches
4.2.1 Curent commercial vaccines against Anaplasma marginale and Anaplasma phagocytophilum
In cattle, the only commercially available vaccine is heterologous live attenuated strain of A. centrale, included in trivalent vaccines with Babesia in endemic countries (South Africa, Argentina, Brasil) (71). A. centrale is less virulent and offers some cross-protection against A. marginale, although it does not fully prevent the infection. However, its efficacy is limited: vaccine-related outbreaks caused by A. centrale have been reported, as well as failures to protect against virulent A. marginale (71). In the United States, an inactivated vaccine based on the A. marginale St. Maries strain has been used in the field for decades. Although this killed vaccine does not prevent infection by virulent strains, it confers sufficient immunity to protect against acute clinical disease (71). No current vaccine guarantees sterilizing immunity or universal protection across the many A. marginale strains, antigenic diversity among geographic isolates limits cross-protection (72).
Vaccines against A. phagocytophilum: Currently, there is no commercial vaccine available to prevent human granulocytic anaplasmosis or anaplasmosis in domestic animals (71). Prevention relies on tick control, as A. phagocytophilum is an intracellular pathogen for which no licensed vaccines exist (73). However, experimental vaccine candidates have been explored in recent years. For instance, immunizations in murine models with A. phagocytophilum adhesion proteins have shown partial efficacy: vaccines based on adhesin peptides (Asp14, AipA) achieved a 4–5-fold reduction in bacterial load in mice after challenge (74). Similarly, a DNA prime–protein boost strategy using conserved antigens from the type IV secretion system (VirB9-1, VirB9-2, VirB10) elicited immune responses and partial protection in mice; notably, VirB10 induced CD4+ IFN-γ+ T cells and reduced infection following challenge (73). In sheep, trials with the surface protein MSP4 of A. phagocytophilum showed limited protection, leading to “vaccinomic” approaches focused on designing more effective multiepitope chimeric antigens. By mapping protective epitopes of MSP4 and other proteins, a fusion antigen was developed that was able to block in vitro infection of host cells, offering a superior alternative to the use of full-length individual proteins (71).
4.2.2 Local and regional strategies in Brazil
In a study conducted in Brazil, low-virulence isolates of A. marginale (UFMG1 and UFMG3) were evaluated as potential vaccine candidates. Nested PCR targeting the msp4 gene confirmed infection by these isolates, with the animals displaying reduced clinical signs of anaplasmosis, including lower hematocrit drops and milder anemia compared to non-vaccinated animals. These findings highlight the potential of using local low-virulence strains to enhance vaccine strategies in regions with high genetic diversity of A. marginale (75). The study also underscores the significance of adapting vaccine protocols to the local epidemiological context. In tropical regions like the Amazon, where the biodiversity of vectors and circulating strains is high, incorporating local isolates into vaccination programs could reduce disease persistence and improve livestock productivity. These findings align with broader efforts to develop recombinant vaccines targeting highly conserved proteins like MSP1a and MSP2. Combining live attenuated vaccines with subunit vaccines may provide a synergistic approach to tackling A. marginale in the Amazon and beyond.
4.2.3 Challenges in vaccine development
Some limitations of available vaccines include immune evasion via antigenic variation. A. marginale and A. phagocytophilum evade the immune response through sequential variation of their major surface proteins. During chronic infection, A. marginale expresses antigenic variants of MSP2 and MSP3, escaping recognition by IgG2 antibodies and T cells previously primed against earlier variants (76). Similarly, A. phagocitphylum expresses antigenic variants of MSP2/P44 to evade immune response (76). This antigenic diversity, both among strains and within the course of a single infection, makes it difficult for a vaccine based on one or a few antigens to provide broad and lasting protection (72). The lack of cross-protection against heterologous strains has been one of the main causes of failure in previous vaccine efforts.
There are also immunogenicity limitations. Many candidate antigens do not elicit sufficiently protective immune responses on their own. For example, although MSP4 and MSP5 of A. marginale are relatively conserved surface proteins, they have not proven to be strong immunogens: MSP4 often fails to induce high antibody levels, while MSP5 elicits abundant antibody responses that are nonetheless non-protective (72). This underscores that immunogenicity does not necessarily equate to protection. Likewise, native membrane-associated bacterial antigens can induce protective immunity in animals, but their purified recombinant counterparts may not mimic the native conformation or context, resulting in suboptimal immune responses (72). In other words, recombinant antigens may lack key conformational epitopes or cofactors necessary to trigger adequate immunity (72). Additionally, subunit vaccines tend to focus on immunodominant but variable proteins (e.g., MSP2), which the pathogen can easily alter; therefore, researchers are exploring subdominant yet conserved antigens (such as VirB9/10 from the T4SS) as potentially more stable targets (73).
Another limitation is incomplete or skewed immune response. If the vaccine fails to trigger the appropriate cellular immune response, protection is likely to be insufficient. Anaplasmosis requires primarily a Th1-type cellular immune response (CD4+ T cells producing IFN-γ and IgG2 in cattle) to control intracellular infection (72). It has been shown that vaccination with A. marginale antigens combined with an appropriate adjuvant (e.g., Quil-A saponin, which promotes Th1 responses) results in high titers of IgG2, CD4+ T cells, and IFN-γ—correlating with clinical protection (72). In contrast, animals with an IgG1-skewed response (Th2-type humoral immunity) have developed acute disease following challenge (72). This reveals that another obstacle in vaccine development is achieving the correct immune orientation through proper formulation; an inadequate formulation (e.g., weak adjuvant) may induce insufficient or incorrect immune responses, allowing infection to persist.
One of the new approaches is using improved subunit vaccines (multiepitope recombinant antigens). Instead of single antigens, current efforts aim to design vaccines using mosaics of conserved epitopes from multiple proteins. For instance, critical peptides from adhesion proteins (Asp14, AipA, OmpA) of A. phagocytophilum have been assembled into a single chimeric protein (referred to as a chimeritope), allowing the inclusion of multiple protective epitopes while excluding variable or immunodistracting regions (74). This multiepitope strategy could elicit broader and more effective immune responses compared to individual antigens. In fact, a similar approach led to the development of a successful canine Lyme disease vaccine through the fusion of epitopes from various Borrelia OspC variants (74).
4.3 Immunotherapies and novel strategies
The study done by Noh et al. (77) highlighted the use of adhesion proteins like Msp1b and OmpA, which allows the binding of A. marginale to livestock’s erythrocytes, as targets for new sub unitary vaccines and immune therapies. These adhesins play a vital role in the infection process by mediating the adherence of the pathogen to the host’s cells, and eliminating this interaction could significantly reduce the infection rate. The identification of these proteins could also pave the way to the development of monoclonal antibodies that can neutralize their function, offering a new strategy to prevent the infection (77).
Another promising strategy is the use of direct mutagenesis in A. marginale to develop attenuated strains as a basis for vaccines. In the study of Ferm et al. (78), the gene phtcp, responsible for the pathogen-s virulence, was eliminated making a mutant strain that does not produce the disease but generates a protective immune response in bovines. This attenuated live vaccine demonstrated a high effectiveness in the protection against wild strains of A. marginale without causing the clinical symptoms of the disease. This technology could revolutionize the way in which vaccines against anaplasmosis are developed by reducing the severe infection risks (79).
Lastly, immune stimulation through the use of Toll Like Receptor 7 (TLR7) agonists has demonstrated to be an effective tool to activate the innate immune response in bovine livestock, providing early protection against infection by A. marginale. In another study by Futse et al. (80), an adjuvant along with TLR7 and saponin, which was administered to calves in an endemic region in Occidental Africa. This treatment resulted in a significant decrease in mortality and morbidity rates, also reducing the need of treatment with antibiotics. This approach offers a practical solution to protect livestock in areas where regular vaccination may not be viable, and could complement the current vaccination strategies (80).
4.4 Chemical, biological, and physical control strategies for vector management
Effective control of tick populations is a fundamental strategy in reducing the transmission of Anaplasma species, particularly A. marginale and A. phagocytophilum. Since these pathogens rely heavily on tick vectors such as Rhipicephalus microplus and Ixodes ricinus, any successful approach to control anaplasmosis must incorporate measures that directly target the vectors’ ecology and population dynamics.
In addition to R. microplus, multiple tick species have been implicated in the transmission of A. marginale. These include the three-host ticks Dermacentor andersoni and D. variabilis in the United States, Rhipicephalus sanguineus in Israel, R. simus in South Africa, and the one-host tick R. annulatus in Israel, Central and South America, and Mexico. However, vector competence may vary significantly not only between species but also among different geographic populations of the same species (81).
Beyond vector control, an integrated approach is recommended, combining chemotherapy, immunoprophylaxis, and environmental management. Although bovine anaplasmosis causes important production losses, it has not received sufficient global attention in terms of coordinated control strategies (82). Managing grazing practices (e.g., rotational grazing), controlling arthropod vectors, and reducing contact with wildlife reservoirs through fencing are essential components (83–85). Environmental measures such as cleaning stables and proper manure disposal can further reduce vector presence (86).
Acaricides remain an important tool for tick control, but their use presents some challenges. Synthetic acaricides belong to various chemical classes, such as pyrethroids, organophosphates, amidines, macrocyclic lactones, phenylpyrazoles, and growth inhibitors (87). A study by Tosato et al. (88) demonstrated that the use of acaricides and repellents can inadvertently increase the prevalence of tick-borne diseases through a phenomenon known as overcompensation. When the tick population declines due to acaricides, the juvenile population can surge, acting as a reservoir for pathogens and facilitating disease persistence. Additionally, the use of repellents can limit the number of available hosts, increasing the likelihood of transmission between ticks through simultaneous feeding on the same host (88).
Tick control is crucial in managing anaplasmosis, as ticks serve as the primary vectors for A. marginale. Species such as R. microplus are the main transmitters, and controlling them often involves the use of tick repellents. Traditionally, these products are applied via immersion baths, spraying, or dorsal pour-on methods, along with acaricides from chemical groups like organophosphates, pyrethroids, and amidines. However, prolonged use of these products has led to the development of resistance, particularly to pyrethroids and amitraz, complicating their long-term effectiveness (89).
Given the rising resistance to chemical treatments, alternatives based on natural biocides have gained interest. A study in Ecuador evaluated the use of extracts from Ambrosia peruviana and Azadirachta indica for tick control in cattle. The results showed that a 25% solution of A. peruviana extract achieved a tick mortality rate of 88.33%, suggesting that natural biocides can be a sustainable and effective alternative for tick control, reducing dependence on conventional chemical products (90).
The use of entomopathogenic fungi (EPF), like Metarhizium anisopliae and Beauveria bassiana, has gained attention as an alternative for the chemical acaricides. This fungi attacks ticks by infecting their cuticula, which causes their death without the risk of producing resistance unlike the conventional chemical products. In Mexico, it has been demonstrated that EPF are effective to control resistant ticks like R. microplus, in laboratory and in experimental fields. This strategy is not only secure for the environment but can also be integrated with other control methods in an integrated handling of plagues (91).
One effective approach for tick control is habitat modification, which can involve methods such as controlled burns of areas where ticks are commonly found. This technique can hinder tick reproduction and survival by altering their environment. Allan (92) observed that controlled burns significantly reduced the abundance of Amblyomma americanum in Missouri. This method can potentially be extrapolated to other tick species that are responsible for transmitting anaplasmosis, offering a broader application for tick population management.
Moreover, integrated vector management (IVM) strategies, combining chemical, biological, and environmental interventions, are increasingly recommended, particularly in endemic areas like the Amazon where biodiversity and wildlife reservoirs complicate tick control. These strategies not only reduce vector populations but also limit the opportunities for transmission of multiple Anaplasma species simultaneously (Figure 3).
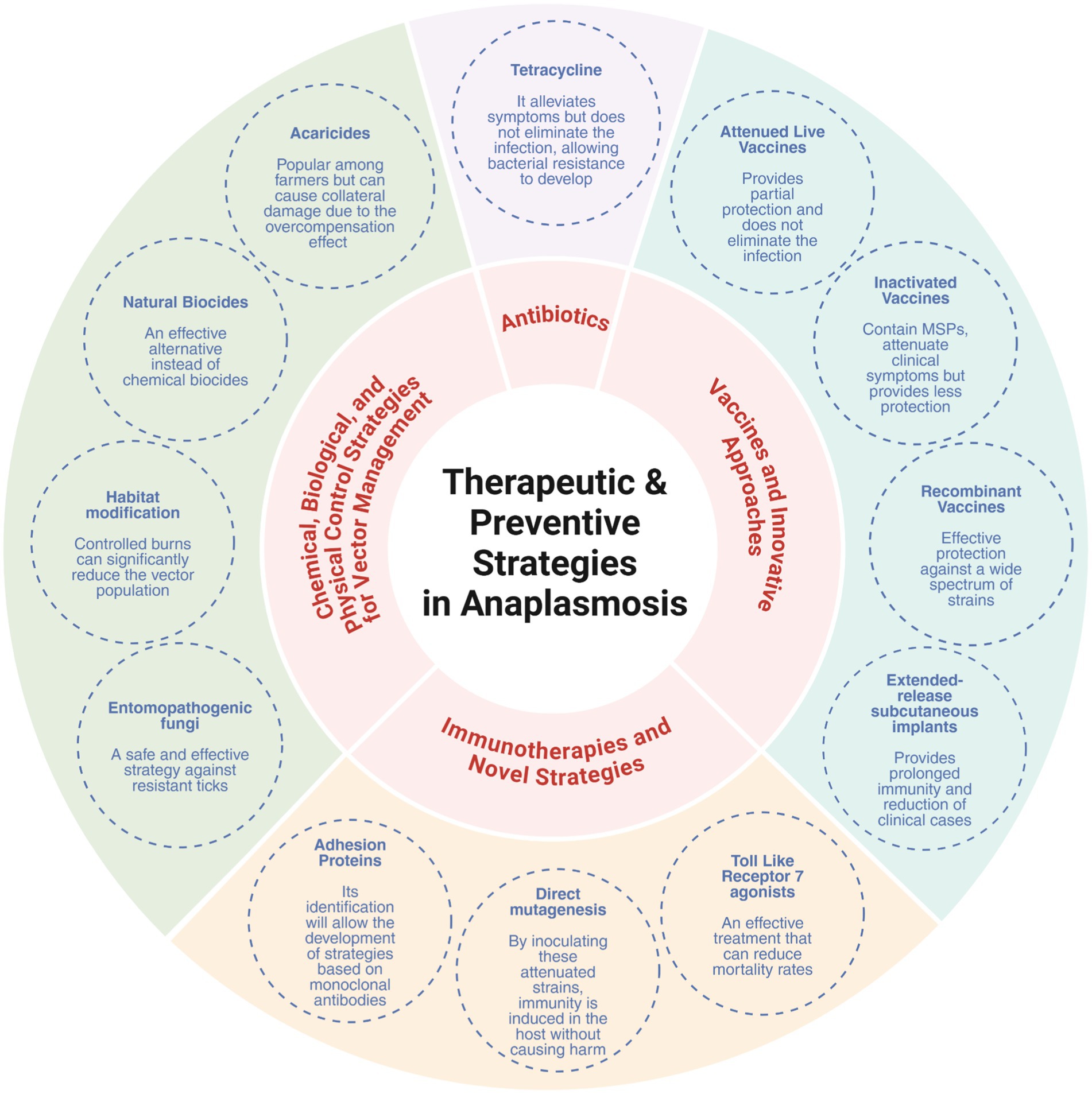
Figure 3. Integrated control strategies for anaplasmosis: therapeutic and preventive approaches. Created in https://BioRender.com.
4.5 Recent advances
Recent advances in molecular technologies and genetic tools have highlighted new strategies for controlling A. marginale infections, particularly in regions like the Amazon, where environmental factors and diverse vector-host interactions pose significant challenges.
One promising approach involves genetic therapy using CRISPR-Cas9. Although this technology has not yet been directly applied to A. marginale, recent studies demonstrate its potential in targeting bacterial pathogens. For instance, CRISPR-Cas phages have been used to reduce Escherichia coli loads in mice, showcasing the feasibility of precision bactericidal interventions (93). This strategy could potentially be adapted to specifically target A. marginale, offering a precise, antibiotic-free method to eliminate the pathogen and mitigate the risks associated with antimicrobial resistance.
Metataxonomic studies using 16S rRNA sequencing have also contributed to understanding the genetic diversity and dynamics of A. marginale. A study conducted by Makgabo et al. (94) in South Africa identified over 50 distinct genotypes of A. marginale across different habitats, including previously undescribed variants. These findings underscore the importance of genetic surveillance in endemic regions. Applying similar approaches in the Amazon could provide insights into the unique genetic variants circulating in the region, which may influence transmission dynamics and persistence.
Recent technological advances have opened new possibilities for the management of bovine anaplasmosis, specifically in the development of vaccine strategies and diagnosis. An example is the use of nanotechnology in vaccine design. A study in Brazil developed a nano vaccine based on Multiwalled Carbon Nanotubes (MWCNT) combined with a synthetic peptide derived from MSP1a. This platform showed promising results in murine models, inducing a balanced Th1/Th2 immune response and significantly reducing the bacteremia in immunized animals. This new approach highlights the potential of nanotechnology to overcome the limitations of traditional vaccines and offers a promising perspective for future applications in livestock, specifically in endemic regions like the Amazon (95).
Multi-omics approaches are also being explored to enhance the diagnosis of A. marginale. By combining genomic and transcriptomic data, researchers can identify novel biomarkers for improved diagnostic accuracy and monitor the pathogen’s genetic evolution in response to environmental and host factors. These methods hold particular promise in the Amazon, where the high biodiversity of vectors and wildlife reservoirs complicates traditional diagnostic strategies.
5 Future directions and challenges
5.1 Impact of climate change and globalization
The study by Tiffin et al. (96) highlights how climate change and globalization are affecting the distribution of ticks. Global warming has expanded the range of species like Ixodes scapularis in Canada, and R. microplus has extended its presence to new areas, making tick control more difficult. Additionally, globalization has increased the introduction of invasive species, such as Haemaphysalis longicornis, which poses new challenges for tick control programs. Global changes, such as climate shifts and environmental degradation, are altering ecological niches, favoring the emergence and reemergence of vector-borne diseases (97), including in forested regions like the Amazon (98).
In this environment, a vast diversity of biological species is found, many of which are arthropod vectors of pathogens or microbial species and parasites associated with diseases in humans (99). For example, rising temperatures and changing rainfall patterns may create new habitats for ticks, increasing their population and the risk of transmitting diseases such as anaplasmosis. These environmental changes not only facilitate the spread of existing diseases but may also contribute to the emergence of new pathogens. International collaboration is crucial in addressing these challenges. This underscores the need for a One Health approach, which integrates human, animal, and environmental health to effectively manage these complex and evolving threats.
5.2 Challenges in surveillance and control
Surveillance and control of R. microplus remain critical to managing anaplasmosis in the Amazon. A study by Mader et al. (100) demonstrated that underfunded and fragmented tick control programs reduce the effectiveness of disease management. Similarly, in Latin America, tropical climates support rapid tick reproduction, increasing the incidence of anaplasmosis. Also, less than 13% of sampled areas directly finance the control programs, which demonstrates the need for more coordinated and sustainable strategies (100).
In Latin America, tick control and surveillance are challenging and the main factor is the tropical weather condition inherent to this area. This condition enhances the expansion of the tick population, thus increasing the incidence of anaplasmosis. Although the use of acaricides and antibiotics help reduce anaplasmosis, tick-resistance to these strategies complicates the efforts to control this disease. Also, the implementation of control programs is difficult and unsustainable long-term.
In Peru, the government through institutions like National Service of Agricultural Sanity (SENASA) and Ministry of Agricultural Development and Irrigation (MIDAGRI) performs tick surveillance in some cities like Huancavelica, Ancash, Tumbes, Lambayeque and Puno. While these efforts help mitigate an outbreak of anaplasmosis, they are still not enough because these campaigns are performed by the government once or twice a year (101–106).
In other countries like Mexico, the approach is similar, surveillance programs once or twice a year in some regions like Jalisco, Morelos, Nayarit and Veracruz, where anaplasmosis had been previously reported and there is a risk of an outbreak of this disease (107). Similarly, the Colombian government through the Colombian Agriculture and Livestock Institute (ICA) performs tick surveillance in regions where this disease could have an outbreak (108).
In Brazil, the primary strategy for tick control heavily relies on the use of synthetic acaricides. Currently, around 250 products are marketed for tick control, and decisions regarding cattle tick management are generally made by farmers, with occasional input from veterinarians. Rhipicephalus (Boophilus) microplus, a highly invasive tick species, is prevalent in tropical and subtropical cattle-raising regions of Asia, Africa, and the Americas. In Brazil, this tick is the main vector for A. marginale and represents the most significant ectoparasite affecting cattle. It caused estimated losses exceeding three billion US dollars in 2014. The country’s tropical climate further complicates efforts, as it favors the proliferation of tick populations, making the control and surveillance of ticks and tick-borne diseases such as anaplasmosis particularly challenging (109).
Despite official governmental programs in other countries like Australia, Argentina, Uruguay, Mexico, and the USA, Brazil does not have an official program specifically aimed at controlling or eradicating R. (B.) microplus. However, the Ministry of Agriculture and Livestock (MAPA) has recently published a manual on the Integrated Parasite Control System (SICOPA) for managing and controlling cattle ticks. The publication, titled Avaliação Seletiva de Bovinos para o Controle do Carrapato – Rhipicephalus microplus (110), provides detailed guidance on selective cattle tick control.
The use of molecular techniques have been critical to improve the surveillance and management of Anaplasma spp., specifically in tropical regions like the Amazon, where the genetic diversity of these pathogens represents an important challenge. A recent study in small ruminants in the northeast of Brazil highlighted the high prevalence of A. marginale, A. platys and “Candidatus A. boleense” through analysis based on genes like msp4, msp1α, y 16S rRNA. These results show the complexity of the epidemiologic systems in tropical regions and the need to use multiple molecular markers for an accurate characterization of strains (111). Also, the study highlighted that the lack of systematic investigations in non-bovine species, like small ruminants, limits the comprehension of the dynamic of inter-specific transmission in environments where multiple species coexist. In the Amazon, where the environmental conditions and biodiversity favors the coexistence of multiple hosts and vetores, this lack of knowledge could compromise the implementation of effective management strategies.
The integration of advanced molecular techniques, along with active surveillance programs in small ruminants and bovines, is critical to address these challenges. This could not only improve the early detection and surveillance of Anaplasma spp., but also could allow the identification of new strains with zoonotic potential and adapt the interventions to local conditions of the Amazon.
5.3 Other emerging technologies: TickBot, gene editing, and phage therapy
Among the emerging technologies for tick control, one of the most innovative is the use of tick-catching robots, such as TickBot. This device is designed to attract and eliminate ticks from the environment, significantly reducing tick populations without relying on chemicals, making it a more sustainable strategy in the long term (112).
Gene editing using technologies like CRISPR-Cas9 has revolutionized research on various disease vectors. However, genetic editing of ticks, such as I. scapularis—a vector for diseases like anaplasmosis, has historically been challenging due to the unique biology of ticks, including their long-life cycle and the technical difficulties of embryonic microinjection (113). A new protocol for embryonic microinjection in I. scapularis has been successfully developed, and manipulation of the genome has been achieved, though the efficiency of genome editing remains low. The REMOT Control technology, which allows the injection of Cas9 complexes directly into adult ticks, has proven more effective for mutagenesis than embryonic injection. However, this technique is currently limited to genetic knockout research and does not yet allow gene insertion (113). Additionally, complementary technologies like transcriptomics and metagenomics are essential for studying how genetic manipulation affects the tick microbiome and its ability to transmit pathogens such as A. marginale, potentially creating new control strategies (113).
As genetic editing technologies continue to evolve, it is expected that techniques like CRISPR and other emerging approaches will offer new alternatives for controlling disease vectors. However, challenges remain regarding accessibility and practical application in the field. The use of omics tools, along with genetic manipulation, could be crucial for developing more accurate intervention strategies, especially in monitoring resistance to pathogens within the tick microbiome (114). The viability of these technologies will depend on overcoming current technical challenges and fostering more international collaboration in research and control efforts for tick-borne diseases (114).
Phage therapy, involving the use of CRISPR-Cas9 in antibacterial treatments, has shown great potential as an alternative to conventional antibiotics, particularly for controlling resistant bacteria. One of the main challenges, however, lies in the efficiency of delivering CRISPR-Cas9 to pathogenic bacteria. Although phages have been effective as vectors for delivering genetic material, the effectiveness of CRISPR systems across different bacterial species is still limited due to the variability in bacterial susceptibility to the phages used (115). Moreover, genetic editing in bacteria may lead to unintended consequences, such as the selection of more resistant strains or alterations in microbial communities, raising concerns about its long-term use in both clinical and agricultural settings.
As research progresses, it is essential to improve delivery and specificity systems in CRISPR-based therapies to reduce the risk of bacterial resistance and enhance efficacy across different pathogenic strains. Additionally, it is crucial to assess the ecological impact of these interventions, ensuring that genetic modifications in bacteria do not disrupt the microbiome associated with livestock, which could affect both animal and human health (115). Future research should focus on optimizing delivery vectors and discovering new bacterial targets for broader therapeutic applications.
6 Final considerations
Molecular techniques for the surveillance, diagnosis, and treatment of anaplasmosis have proven to be indispensable tools in the Amazon region and neighboring areas. Despite significant advances achieved over the years, the control, treatment, and eradication of the disease still face major challenges. These obstacles are amplified by factors such as the high diversity of vectors present in the region, the immunological complexity of the pathogen, the difficulties in implementing control strategies in extensive production systems, and the impacts of climate change, which favor vector expansion.
Although molecular techniques have enabled faster and more accurate diagnoses, the treatment of anaplasmosis remains limited by bacterial resistance and the lack of new drugs on the market. These tools could be even more effective if integrated into prevention programs that include continuous herd monitoring and proactive vector control measures. However, the economic and social realities of the region demand more accessible and sustainable solutions.
To advance in tackling anaplasmosis, it is essential to prioritize the development of new vaccines that are effective against local pathogen variants and to expand access to molecular techniques for small and medium-scale producers. The integration of control strategies based on epidemiological data, combined with public policies that encourage the adoption of good management practices, will be fundamental in reducing the impact of the disease and promoting healthier and more resilient production systems. Finally, addressing anaplasmosis in the Amazon region is not just a technical issue but also a social and economic one, requiring joint efforts from researchers, veterinarians, producers, and governments to build innovative and inclusive solutions.
Author contributions
JM: Formal analysis, Investigation, Writing - original draft. JD: Methodology, Project administration, Supervision, Visualization, Writing - review & editing. EH: Data curation, Methodology, Visualization, Writing - review & editing. RP: Conceptualization, Data curation, Validation, Writing - review & editing. DV: Conceptualization, Data curation, Formal analysis, Writing - review & editing. ER: Funding acquisition, Supervision, Investigation, Writing – review & editing. WB: Funding acquisition, Resources, Writing – review & editing. JM: Funding acquisition, Visualization, Writing – review & editing. RL: Conceptualization, Resources, Visualization, Writing - review & editing.
Funding
The author(s) declare that financial support was received for the research and/or publication of this article. This work was supported by CONTRATO: PE501087383-2024-PROCIENCIA, project CUI N° 2254946 and vice-rectorate for research from the National University Toribio Rodríguez de Mendoza, Amazonas, Peru.
Conflict of interest
The authors declare that the research was conducted in the absence of any commercial or financial relationships that could be construed as a potential conflict of interest.
Generative AI statement
The author(s) declare that no Gen AI was used in the creation of this manuscript.
Publisher’s note
All claims expressed in this article are solely those of the authors and do not necessarily represent those of their affiliated organizations, or those of the publisher, the editors and the reviewers. Any product that may be evaluated in this article, or claim that may be made by its manufacturer, is not guaranteed or endorsed by the publisher.
References
1. Ferreira, GCM, Canozzi, MEA, Peripolli, V, Moura, GDP, Sánchez, J, and Martins, CEN. Prevalence of bovine Babesia spp., Anaplasma marginale, and their co-infections in Latin America: systematic review-meta-analysis. Ticks Tick Borne Dis. (2022) 13:101967. doi: 10.1016/j.ttbdis.2022.101967
2. Greenwood, PL. Review: an overview of beef production from pasture and feedlot globally, as demand for beef and the need for sustainable practices increase. Animal. (2021) 15:100295. doi: 10.1016/j.animal.2021.100295
3. Teixeira, VA, Lana, AMQ, Bresolin, T, Tomich, TR, Souza, GM, Furlong, J, et al. Using rumination and activity data for early detection of anaplasmosis disease in dairy heifer calves. J Dairy Sci. (2022) 105:4421–33. doi: 10.3168/jds.2021-20952
4. Cao, X-Q, Gu, X-L, Zhang, L, Xu, J, Han, H, and Yu, X. Molecular detection of Rickettsia, Anaplasma, and Bartonella in ticks from free-ranging sheep in Gansu Province, China. Ticks Tick Borne Dis. (2023) 14:102137. doi: 10.1016/j.ttbdis.2023.102137
5. Lin, Z-T, Du, L-F, Zhang, M-Z, Han, X-Y, Wang, B-H, Meng, J, et al. Genomic characteristics of emerging Intraerythrocytic Anaplasma capra and high prevalence in goats, China. Emerg Infect Dis. (2023) 29:1780–8. doi: 10.3201/eid2909.230131
6. Santodomingo, A, Thomas, R, Robbiano, S, Uribe, JE, Parragué-Migone, C, Cabello-Stom, J, et al. Wild deer (Pudu puda) from Chile harbor a novel ecotype of Anaplasma phagocytophilum. Parasit Vectors. (2023) 16:38. doi: 10.1186/s13071-023-05657-9
7. Matei, IA, Estrada-Peña, A, Cutler, SJ, Vayssier-Taussat, M, Varela-Castro, L, Potkonjak, A, et al. A review on the eco-epidemiology and clinical management of human granulocytic anaplasmosis and its agent in Europe. Parasit Vectors. (2019) 12:599. doi: 10.1186/s13071-019-3852-6
8. Parodi, P, Armúa-Fernández, MT, Schanzembach, M, Mir, D, Benítez-Galeano, MJ, Rodríguez-Osorio, N, et al. Characterization of strains of Anaplasma marginale from clinical cases in bovine using major surface protein 1a in Uruguay. Front Vet Sci. (2022) 9:990228. doi: 10.3389/fvets.2022.990228
9. André, MR. Diversity of Anaplasma and Ehrlichia/Neoehrlichia agents in terrestrial wild carnivores worldwide: implications for human and domestic animal health and wildlife conservation. Front Vet Sci. (2018) 5:293. doi: 10.3389/fvets.2018.00293
10. Custódio, WP, Chaves, JVRL, Pantoja, PT, Cárdenas, AMCD, Andrade, RFD, Dapureza, DY, et al. Proceso salud-enfermedad en la región amazónica: factores ambientales y la aparición de enfermedades. Rev Científica Multidiscip Núcleo Conhecimento. (2021) 3:05–21. doi: 10.32749/nucleodoconhecimento.com.br/salud/aparicion-de-enfermedades
11. Marengo, JA, Souza, CM, Thonicke, K, Burton, C, Halladay, K, Betts, RA, et al. Changes in climate and land use over the Amazon region: current and future variability and trends. Front Earth Sci. (2018) 6:228. doi: 10.3389/feart.2018.00228
12. Buysse, M, Koual, R, Binetruy, F, De Thoisy, B, Baudrimont, X, Garnier, S, et al. Detection of Anaplasma and Ehrlichia bacteria in humans, wildlife, and ticks in the Amazon rainforest. Nat Commun. (2024) 15:3988. doi: 10.1038/s41467-024-48459-y
13. Calchi, AC, Vultão, JG, Alves, MH, Yogui, DR, Desbiez, ALJ, De Santi, M, et al. Ehrlichia spp. and Anaplasma spp. in Xenarthra mammals from Brazil, with evidence of novel “Candidatus Anaplasma spp.”. Sci Rep. (2020) 10:12615. doi: 10.1038/s41598-020-69263-w
14. Grau, HEG, Cunha Filho, NAD, Pappen, FG, and Farias, NADR. Transplacental transmission of Anaplasma marginale in beef cattle chronically infected in southern Brazil. Rev Bras Parasitol Vet. (2013) 22:189–93. doi: 10.1590/S1984-29612013000200038
15. Zhang, L. Nosocomial transmission of human granulocytic Anaplasmosis in China. JAMA. (2008) 300:2263. doi: 10.1001/jama.2008.626
16. Krause, PJ. Nosocomial transmission of human granulocytic Anaplasmosis? JAMA. (2008) 300:2308–9. doi: 10.1001/jama.2008.665
17. Frias, H, Murga, L, Bardales, W, Frias, V, Portocarrero-Villegas, SM, Segura Portocarrero, T, et al. Prevalence and risk factors of Anaplasmosis in Simmental cattle in the Peruvian Amazon. Vet Med Int. (2024) 2024:4634440. doi: 10.1155/2024/4634440
18. Rodriguez-Vivas, RI, Jonsson, NN, and Bhushan, C. Strategies for the control of Rhipicephalus microplus ticks in a world of conventional acaricide and macrocyclic lactone resistance. Parasitol Res. (2018) 117:3–29. doi: 10.1007/s00436-017-5677-6
19. Dantas-Torres, F, and Otranto, D. Anaplasmosis. In: CB Marcondes, editor. Arthropod borne diseases. Cham: Springer International Publishing (2017). 215–22.
20. De La Fuente, J, Torina, A, Caracappa, S, Tumino, G, Furlá, R, Almazán, C, et al. Serologic and molecular characterization of Anaplasma species infection in farm animals and ticks from Sicily. Vet Parasitol. (2005) 133:357–62. doi: 10.1016/j.vetpar.2005.05.063
21. Kocan, KM, De La Fuente, J, Guglielmone, AA, and Meléndez, RD. Antigens and alternatives for control of Anaplasma marginale infection in cattle. Clin Microbiol Rev. (2003) 16:698–712. doi: 10.1128/CMR.16.4.698-712.2003
22. Rymaszewska, A, and Grenda, S. Bacteria of the genus Anaplasma – characteristics of Anaplasma and their vectors: a review. Vet Med. (2008) 53:573–84. doi: 10.17221/1861-VETMED
23. Junsiri, W, Watthanadirek, A, Poolsawat, N, Minsakorn, S, Nooroong, P, Jittapalapong, S, et al. Molecular characterization of Anaplasma marginale based on the msp1a and msp1b genes. Vet Microbiol. (2021) 262:109236. doi: 10.1016/j.vetmic.2021.109236
24. Brayton, KA, Palmer, GH, Lundgren, A, Yi, J, and Barbet, AF. Antigenic variation of Anaplasma marginale msp 2 occurs by combinatorial gene conversion. Mol Microbiol. (2002) 43:1151–9. doi: 10.1046/j.1365-2958.2002.02792.x
25. Sutipatanasomboon, A, Wongsantichon, J, Sakdee, S, Naksith, P, Watthanadirek, A, Anuracpreeda, P, et al. RPA-CRISPR/Cas12a assay for the diagnosis of bovine Anaplasma marginale infection. Sci Rep. (2024) 14:7820. doi: 10.1038/s41598-024-58169-6
26. Rikihisa, Y. Mechanisms to create a safe haven by members of the family Anaplasmataceae. Ann N Y Acad Sci. (2003) 990:548–55. doi: 10.1111/j.1749-6632.2003.tb07425.x
27. De La Fuente, J, Garcia-Garcia, JC, Blouin, EF, and Kocan, KM. Characterization of the functional domain of major surface protein 1a involved in adhesion of the rickettsia Anaplasma marginale to host cells. Vet Microbiol. (2003) 91:265–83. doi: 10.1016/S0378-1135(02)00309-7
28. Roczeń-Karczmarz, M, Dudko, P, Demkowska-Kutrzepa, M, Meisner, M, Studzińska, M, Junkuszew, A, et al. Comparison of the occurrence of tick-borne diseases in ticks collected from vegetation and animals in the same area. Med Weter. (2018) 74:484–8. doi: 10.21521/mw.6107
29. De Souza Ramos, IA, Herrera, HM, Fernandes, SDJ, Do Amaral, RB, Zanatto, DCDS, Da Silva, TMV, et al. Genetic diversity of Anaplasma marginale in beef cattle in the Brazilian Pantanal. Ticks Tick Borne Dis. (2019) 10:805–14. doi: 10.1016/j.ttbdis.2019.03.015
30. Pereira, JG, Garcia, AB, Gonçalves, LR, Ramos, IADS, Braga, MDSCO, Santos, LSD, et al. High genetic diversity of Anaplasma marginale infecting dairy cattle in northeastern Brazil. Rev bras Parasitol. Veterinaria. (2021) 30:e014321. doi: 10.1590/s1984-29612021097
31. Alhassan, A, Hove, P, Sharma, B, Matthew-Belmar, V, Karasek, I, Lanza-Perea, M, et al. Molecular detection and characterization of Anaplasma platys and Ehrlichia canis in dogs from the Caribbean. Ticks Tick Borne Dis. (2021) 12:101727. doi: 10.1016/j.ttbdis.2021.101727
32. Salvioni Recalde, OD, Rolón, MS, Velázquez, MC, Kowalewski, MM, Alfonso Ruiz Diaz, JJ, Rojas De Arias, A, et al. Diversity of Anaplasmataceae transmitted by ticks (Ixodidae) and the first molecular evidence of Anaplasma phagocytophilum and Candidatus Anaplasma boleense in Paraguay. Microorganisms. (2024) 12:1893. doi: 10.3390/microorganisms12091893
33. Springer, A, Glass, A, Probst, J, and Strube, C. Tick-borne zoonoses and commonly used diagnostic methods in human and veterinary medicine. Parasitol Res. (2021) 120:4075–90. doi: 10.1007/s00436-020-07033-3
34. Suminda, GGD, Bhandari, S, Won, Y, Goutam, U, Kanth Pulicherla, K, Son, Y-O, et al. High-throughput sequencing technologies in the detection of livestock pathogens, diagnosis, and zoonotic surveillance. Comput Struct Biotechnol J. (2022) 20:5378–92. doi: 10.1016/j.csbj.2022.09.028
35. Muellner, P, Zadoks, RN, Perez, AM, Spencer, SEF, Schukken, YH, and French, NP. The integration of molecular tools into veterinary and spatial epidemiology. Spat Spatiotemporal Epidemiol. (2011) 2:159–71. doi: 10.1016/j.sste.2011.07.005
36. Sacarrão-Birrento, L, Harrison, LJS, Pienaar, R, Toka, FN, Torres-Acosta, JFJ, Vilela, VLR, et al. Challenges for animal health and production in the tropics and Mediterranean for the next 55 years. Trop Anim Health Prod. (2024) 56:381. doi: 10.1007/s11250-024-04212-7
37. Torina, A, Agnone, A, Blanda, V, Alongi, A, D’Agostino, R, Caracappa, S, et al. Development and validation of two PCR tests for the detection of and differentiation between Anaplasma ovis and Anaplasma marginale. Ticks Tick Borne Dis. (2012) 3:283–7. doi: 10.1016/j.ttbdis.2012.10.033
38. Sahin, OF, Erol, U, and Altay, K. Buffaloes as new hosts for Anaplasma capra: molecular prevalence and phylogeny based on gtlA, groEL, and 16S rRNA genes. Res Vet Sci. (2022) 152:458–64. doi: 10.1016/j.rvsc.2022.09.008
39. Stańczak, J, Cieniuch, S, Lass, A, Biernat, B, and Racewicz, M. Detection and quantification of Anaplasma phagocytophilum and Babesia spp. in Ixodes ricinus ticks from urban and rural environment, northern Poland, by real-time polymerase chain reaction. Exp Appl Acarol. (2015) 66:63–81. doi: 10.1007/s10493-015-9887-2
40. Shen, Z, Zhang, MZ, Stich, RW, Mitchell, WJ, and Zhang, S. Development of a tick-borne pathogen QPCR panel for detection of Anaplasma, Ehrlichia, Rickettsia, and Lyme disease Borrelia in animals. J Microbiol Methods. (2018) 151:83–9. doi: 10.1016/j.mimet.2018.05.019
41. Silva, CBD, Santos, HA, Navarrete, MG, Ribeiro, CCDU, Gonzalez, BC, Zaldivar, MF, et al. Molecular detection and characterization of Anaplasma platys in dogs and ticks in Cuba. Ticks Tick Borne Dis. (2016) 7:938–44. doi: 10.1016/j.ttbdis.2016.04.012
42. Elelu, N, Ferrolho, J, Couto, J, Domingos, A, and Eisler, MC. Molecular diagnosis of the tick-borne pathogen Anaplasma marginale in cattle blood samples from Nigeria using qPCR. Exp Appl Acarol. (2016) 70:501–10. doi: 10.1007/s10493-016-0081-y
43. Catanese, HN, Brayton, KA, and Gebremedhin, AH. RepeatAnalyzer: a tool for analysing and managing short-sequence repeat data. BMC Genomics. (2016) 17:422. doi: 10.1186/s12864-016-2686-2
44. Giglioti, R, Bassetto, CC, Okino, CH, De Oliveira, HN, and De Sena Oliveira, MC. Development of a loop-mediated isothermal amplification (LAMP) assay for the detection of Anaplasma marginale. Exp Appl Acarol. (2019) 77:65–72. doi: 10.1007/s10493-018-0327-y
45. Ganguly, N, Kumar, N, Solanki, J, Kalyani, I, Thakuria, N, and Patel, D. Loop-mediated isothermal amplification assay for the rapid detection of Anaplasma marginale in cattle based on major surface protein 5 gene. J Vector Borne Dis. (2023) 60:49–56. doi: 10.4103/0972-9062.353249
46. Shabana, II, Alhadlag, NM, and Zaraket, H. Diagnostic tools of caprine and ovine anaplasmosis: a direct comparative study. BMC Vet Res. (2018) 14:165. doi: 10.1186/s12917-018-1489-x
47. Sarli, M, Thompson, CS, Novoa, MB, Valentini, BS, Mastropaolo, M, Echaide, IE, et al. Development and evaluation of a double-antigen sandwich ELISA to identify Anaplasma marginale– infected and A. centrale– vaccinated cattle. J Vet Diagn Invest. (2020) 32:70–6. doi: 10.1177/1040638719892953
48. Subbiah, M, Thirumalapura, N, Thompson, D, Kuchipudi, SV, Jayarao, B, and Tewari, D. Detection of Anaplasma Phagocytophilum in horses with suspected tick-borne disease in northeastern United States by metagenomic sequencing. Front Vet Sci. (2021) 8:673193. doi: 10.3389/fvets.2021.673193
49. Regier, Y, Komma, K, Weigel, M, Kraiczy, P, Laisi, A, Pulliainen, AT, et al. Combination of microbiome analysis and serodiagnostics to assess the risk of pathogen transmission by ticks to humans and animals in Central Germany. Parasit Vectors. (2019) 12:11. doi: 10.1186/s13071-018-3240-7
50. Dzięgiel, B, Adaszek, Ł, Kalinowski, M, and Winiarczyk, S. Equine granulocytic anaplasmosis. Res Vet Sci. (2013) 95:316–20. doi: 10.1016/j.rvsc.2013.05.010
51. Huggins, LG, Koehler, AV, Ng-Nguyen, D, Wilcox, S, Schunack, B, Inpankaew, T, et al. A novel metabarcoding diagnostic tool to explore protozoan haemoparasite diversity in mammals: a proof-of-concept study using canines from the tropics. Sci Rep. (2019) 9:12644. doi: 10.1038/s41598-019-49118-9
52. El-Ashker, M, Hotzel, H, Gwida, M, El-Beskawy, M, Silaghi, C, and Tomaso, H. Molecular biological identification of Babesia, Theileria, and Anaplasma species in cattle in Egypt using PCR assays, gene sequence analysis and a novel DNA microarray. Vet Parasitol. (2015) 207:329–34. doi: 10.1016/j.vetpar.2014.12.025
53. Abanda, B, Paguem, A, Achukwi, MD, Renz, A, and Eisenbarth, A. Development of a low-density DNA microarray for detecting tick-borne bacterial and Piroplasmid pathogens in African cattle. Trop Med Infect Dis. (2019) 4:64. doi: 10.3390/tropicalmed4020064
54. Mirabile, A, Sangiorgio, G, Bonacci, PG, Bivona, D, Nicitra, E, Bonomo, C, et al. Advancing pathogen identification: the role of digital PCR in enhancing diagnostic power in different settings. Diagnostics. (2024) 14:1598. doi: 10.3390/diagnostics14151598
55. Melničáková, J, Derdáková, M, and Barák, I. A system to simultaneously detect tick-borne pathogens based on the variability of the 16S ribosomal genes. Parasit Vectors. (2013) 6:269. doi: 10.1186/1756-3305-6-269
56. Uttamchandani, M, Neo, JL, Ong, BNZ, and Moochhala, S. Applications of microarrays in pathogen detection and biodefence. Trends Biotechnol. (2009) 27:53–61. doi: 10.1016/j.tibtech.2008.09.004
57. Mosha, ET, Kuria, JKN, Otiende, M, and Lekolool, I. Molecular detection of Anaplasma phagocytophilum in small mammals and infesting ticks in Laikipia County, Kenya. Vet Med Int. (2024) 2024:5575162. doi: 10.1155/2024/5575162
58. Riggio, V, Madder, M, Labuschagne, M, Callaby, R, Zhao, R, Djikeng, A, et al. Meta-analysis of heritability estimates and genome-wide association for tick-borne haemoparasites in African cattle. Front Genet. (2023) 14:1197160. doi: 10.3389/fgene.2023.1197160
59. Cavani, L, Braz, CU, Giglioti, R, Okino, CH, Gulias-Gomes, CC, Caetano, AR, et al. Genomic study of Babesia bovis infection level and its association with tick count in Hereford and Braford cattle. Front Immunol. (2020) 11:1905. doi: 10.3389/fimmu.2020.01905
60. Valente, D, Serra, O, Carolino, N, Gomes, J, Coelho, AC, Espadinha, P, et al. A genome-wide association study for resistance to tropical theileriosis in two bovine Portuguese autochthonous breeds. Pathogens. (2024) 13:71. doi: 10.3390/pathogens13010071
61. Lai, T-H, Parraga, ME, Alvarez, E, and Rikihisa, Y. Anaplasma platys immunoblot test using major surface antigens. Vector Borne Zoonotic Dis. (2016) 16:581–7. doi: 10.1089/vbz.2016.1964
62. Dzięgiel, B, Adaszek, Ł, Banach, T, and Winiarczyk, S. Specificity of mass spectrometry (MALDI-TOF) in the diagnosis of Babesia canis regarding to other canine vector-borne diseases. Ann Parasitol. (2016) 62:101–5. doi: 10.17420/ap6202.39
63. Hamlili, FZ, Maureen, L, Diarra, AZ, Lafri, I, Gassen, B, Boutefna, B, et al. MALDI-TOF MS identification of dromedary camel ticks and detection of associated microorganisms, Southern Algeria. Microorganisms. (2022) 10:2178. doi: 10.3390/microorganisms10112178
64. Athanasiou, LV, Tsokana, CN, Katsogiannou, EG, Boutsini, S, and Katsoulos, PD. Evaluation of an indirect immunofluorescence assay for the detection of Anaplasma phagocytophilum antigen in ovine Buffy coat smears. Microorganisms. (2022) 10:276. doi: 10.3390/microorganisms10020276
65. Ramos, CAN, Araújo, FR, Santos, RC, Melo, ESP, Sousa, LC, Vidal, CES, et al. Development and assessment of a latex agglutination test based on recombinant MSP5 to detect antibodies against Anaplasma marginale in cattle. Braz J Microbiol. (2014) 45:199–204. doi: 10.1590/S1517-83822014005000039
66. Curtis, AK, Kleinhenz, MD, Anantatat, T, Martin, MS, Magnin, GC, Coetzee, JF, et al. Failure to eliminate persistent Anaplasma marginale infection from cattle using labeled doses of chlortetracycline and Oxytetracycline antimicrobials. Vet Sci. (2021) 8:283. doi: 10.3390/vetsci8110283
67. Reinbold, JB, Coetzee, JF, Hollis, LC, Nickell, JS, Riegel, C, Olson, KC, et al. The efficacy of three chlortetracycline regimens in the treatment of persistent Anaplasma marginale infection. Vet Microbiol. (2010) 145:69–75. doi: 10.1016/j.vetmic.2010.02.031
68. Shahbazi, P, Nouri Gharajalar, S, Mohebbi, K, Taeb, J, Hashemzadeh Farhang, H, Nikvand, AA, et al. First survey on the presence and distribution of Oxytetracycline-resistance genes in Anaplasma species. Acta Parasitol. (2021) 66:501–7. doi: 10.1007/s11686-020-00306-y
69. Sarli, M, Novoa, MB, Mazzucco, MN, Morel, N, Primo, ME, De Echaide, ST, et al. Efficacy of long-acting oxytetracycline and imidocarb dipropionate for the chemosterilization of Anaplasma marginale in experimentally infected carrier cattle in Argentina. Vet Parasitol Reg Stud Reports. (2021) 23:100513. doi: 10.1016/j.vprsr.2020.100513
70. Lacasta, D, Ruiz, H, Ortín, A, Villanueva-Saz, S, Estrada-Peña, A, González, JM, et al. Comparative study of the use of doxycycline and Oxytetracycline to treat Anaplasmosis in fattening lambs. Animals. (2022) 12:2279. doi: 10.3390/ani12172279
71. Alzan, HF, Mahmoud, MS, and Suarez, CE. Current vaccines, experimental immunization trials, and new perspectives to control selected vector borne blood parasites of veterinary importance. Front Vet Sci. (2024) 11:1484787. doi: 10.3389/fvets.2024.1484787
72. Salinas-Estrella, E, Amaro-Estrada, I, Cobaxin-Cárdenas, ME, Preciado De La Torre, JF, and Rodríguez, SD. Bovine Anaplasmosis: will there ever be an almighty effective vaccine? Front Vet Sci. (2022) 9:946545. doi: 10.3389/fvets.2022.946545
73. Crosby, FL, Lundgren, AM, Hoffman, C, Pascual, DW, and Barbet, AF. VirB10 vaccination for protection against Anaplasma phagocytophilum. BMC Microbiol. (2018) 18:217. doi: 10.1186/s12866-018-1346-x
74. Naimi, WA, Gumpf, JJ, Green, RS, Izac, JR, Zellner, MP, Conrad, DH, et al. Immunization against Anaplasma phagocytophilum Adhesin binding domains confers protection against infection in the mouse model. Infect Immun. (2020) 88:e00106–20. doi: 10.1128/IAI.00106-20
75. Mendonça, FLDM, Coelho, MF, Bastos, CV, Da Silveira, JAG, Nicolino, RR, Uribe, JAZ, et al. Calf immunization protocols with low-virulence isolates of Anaplasma marginale: analysis of post-inoculation effects and protection against natural challenge. Ticks Tick Borne Dis. (2025) 16:102394. doi: 10.1016/j.ttbdis.2024.102394
76. Brown, WC. Unraveling the immune regulatory mechanisms imposed by Anaplasma. Vet J. (2008) 175:10–1. doi: 10.1016/j.tvjl.2007.02.026
77. Noh, SM, Ujczo, J, and Alperin, DC. Identification of Anaplasma marginale adhesins for bovine erythrocytes using phage display. Front Trop Dis. (2024) 5:1422860. doi: 10.3389/fitd.2024.1422860
78. Ferm, J, Jaworski, DC, Stoll, I, Kleinhenz, MD, Kocan, KM, Madesh, S, et al. Genetically modified live vaccine offers protective immunity against wild-type Anaplasma marginale tick-transmission challenge. Vaccine. (2024) 42:126069. doi: 10.1016/j.vaccine.2024.06.036
79. Hove, P, Madesh, S, Nair, A, Jaworski, D, Liu, H, Ferm, J, et al. Targeted mutagenesis in Anaplasma marginale to define virulence and vaccine development against bovine anaplasmosis. PLoS Pathog. (2022) 18:e1010540. doi: 10.1371/journal.ppat.1010540
80. Futse, JE, Zumor-Baligi, S, Ashiagbor, CNK, Noh, SM, Fox, CB, and Palmer, GH. An adjuvant formulation containing toll-like receptor 7 agonist stimulates protection against morbidity and mortality due to Anaplasma marginale in a highly endemic region of West Africa. PLoS One. (2024) 19:e0306092. doi: 10.1371/journal.pone.0306092
81. Kocan, KM, De La Fuente, J, Blouin, EF, Coetzee, JF, and Ewing, SA. The natural history of Anaplasma marginale. Vet Parasitol. (2010) 167:95–107. doi: 10.1016/j.vetpar.2009.09.012
82. Aubry, P, and Geale, DW. A review of bovine Anaplasmosis: review of bovine Anaplasmosis. Transbound Emerg Dis. (2011) 58:1–30. doi: 10.1111/j.1865-1682.2010.01173.x
83. Tucker, TR, Aly, SS, Maas, J, Davy, JS, and Foley, JE. Investigation of Anaplasma marginale Seroprevalence in a traditionally managed large California beef herd. Vet Med Int. (2016) 2016:1–7. doi: 10.1155/2016/6186078
84. Zabel, TA, and Agusto, FB. Transmission dynamics of bovine Anaplasmosis in a cattle herd. Interdiscip Perspect Infect Dis. (2018) 2018:1–16. doi: 10.1155/2018/4373981
85. Miller, E, Warburg, A, Novikov, I, Hailu, A, Volf, P, Seblova, V, et al. Quantifying the contribution of hosts with different parasite concentrations to the transmission of visceral Leishmaniasis in Ethiopia. PLoS Negl Trop Dis. (2014) 8:e3288. doi: 10.1371/journal.pntd.0003288
86. Paramanandham, K, Mohankumar, A, Puttahonnappa Suresh, K, Susan Jacob, S, and Roy, P. Prevalence of Anaplasma species in India and the world in dairy animals: a systematic review and meta-analysis. Res Vet Sci. (2019) 123:159–70. doi: 10.1016/j.rvsc.2019.01.013
87. Moraes, N, Nicaretta, JE, Rodrigues, DDC, Gonzaga, BCF, Barrozo, MM, Vale, FL, et al. Comparison of the efficacy of different methods to apply acaricides for control of Rhipicephalus (Boophilus) microplus. Ticks Tick Borne Dis. (2023) 14:102190. doi: 10.1016/j.ttbdis.2023.102190
88. Tosato, M, Nah, K, and Wu, J. Are host control strategies effective to eradicate tick-borne diseases (TBD)? J Theor Biol. (2021) 508:110483. doi: 10.1016/j.jtbi.2020.110483
89. Cabrera, A. Evaluación de eficacia de formulaciones garrapaticidas en terneros estabulados. Argentina: Universidad Nacional del Nordeste (2022). 37 p.
90. Chávez García, DS, Andrade Yucailla, V, Acosta Lozano, NV, and Universidad Estatal Península de Santa ElenaTumbaco González, YUniversidad Estatal Península de Santa Elena. Efecto de biocida natural a base de (ambrosia peruviana, azadirachta indica) para el control de garrapatas en bovinos. Rev Investig Talent. (2022) 9:60–8. doi: 10.33789/talentos.9.1.161
91. Alonso-Díaz, MA, and Fernández-Salas, A. Entomopathogenic Fungi for tick control in cattle livestock from Mexico. Front Fungal Biol. (2021) 2:657694. doi: 10.3389/ffunb.2021.657694
92. Allan, BF. Influence of prescribed burns on the abundance of Amblyomma americanum (Acari: Ixodidae) in the Missouri Ozarks. J Med Entomol. (2009) 46:1030–6. doi: 10.1603/033.046.0509
93. Gencay, YE, Jasinskytė, D, Robert, C, Semsey, S, Martínez, V, Petersen, AØ, et al. Engineered phage with antibacterial CRISPR–Cas selectively reduce E. coli burden in mice. Nat Biotechnol. (2024) 42:265–74. doi: 10.1038/s41587-023-01759-y
94. Makgabo, SM, Brayton, KA, Oosthuizen, MC, and Collins, NE. Unravelling the diversity of Anaplasma species circulating in selected African wildlife hosts by targeted 16S microbiome analysis. Curr Res Microb Sci. (2023) 5:100198. doi: 10.1016/j.crmicr.2023.100198
95. Pimentel, LS, Turini, CA, Santos, PS, Morais, MAD, Souza, AG, Barbosa, MB, et al. Balanced Th1/Th2 immune response induced by MSP1a functional motif coupled to multiwalled carbon nanotubes as anti-anaplasmosis vaccine in murine model. Nanomedicine. (2020) 24:102137. doi: 10.1016/j.nano.2019.102137
96. Tiffin, HS, Rajotte, EG, Sakamoto, JM, and Machtinger, ET. Tick control in a connected world: challenges, solutions, and public policy from a United States border perspective. Trop Med Infect Dis. (2022) 7:388. doi: 10.3390/tropicalmed7110388
97. Chala, B, and Hamde, F. Emerging and re-emerging vector-borne infectious diseases and the challenges for control: a review. Front Public Health. (2021) 9:715759. doi: 10.3389/fpubh.2021.715759
98. Guégan, J-F, De Thoisy, B, Gomez-Gallego, M, and Jactel, H. World forests, global change, and emerging pests and pathogens. Curr Opin Environ Sustain. (2023) 61:101266. doi: 10.1016/j.cosust.2023.101266
99. Confalonieri, UEC, Margonari, C, and Quintão, AF. Environmental change and the dynamics of parasitic diseases in the Amazon. Acta Trop. (2014) 129:33–41. doi: 10.1016/j.actatropica.2013.09.013
100. Mader, EM, Ganser, C, Geiger, A, Harrington, LC, Foley, J, Smith, RL, et al. A survey of tick surveillance and control practices in the United States. J Med Entomol. (2021) 58:1503–12. doi: 10.1093/jme/tjaa094
101. SENASA. Ayacucho: Vigilancia epidemiológica pasiva de enfermedades en bovinos. SENASA Al Día (2017). Available online at: https://www.senasa.gob.pe/senasacontigo/ayacucho-vigilancia-epidemiologica-pasiva-de-enfermedades-en-bovinos/ (accessed January 8, 2025).
102. SENASA. Tumbes: Identificación de garrapatas causantes de enfermedades en bovinos. SENASA Al Día (2017). Available online at: https://www.senasa.gob.pe/senasacontigo/tumbes-identificacion-de-garrapatas-causantes-de-enfermedades-en-bovinos/ (accessed January 8, 2025).
103. SENASA. Cusco: Acciones sanitarias del Senasa benefician a 480 productores de Espinar. SENASA Al Día (2017). Available online at: https://www.senasa.gob.pe/senasacontigo/cusco-acciones-sanitarias-del-senasa-benefician-480-productores-de-espinar/ (accessed January 8, 2025).
104. SENASA. Lambayeque: Senasa busca identificar especies de garrapatas que afectan la ganadería regional. SENASA Al Día (2017). Available online at: https://www.senasa.gob.pe/senasacontigo/lambayeque-senasa-busca-identificar-especies-de-garrapatas-que-afectan-la-ganaderia-regional/ (accessed January 8, 2025).
105. SENASA. Puno: Garrapatas en proceso de identificación para aplicar mejoras en la producción pecuaria. SENASA Al Día (2018). Available online at: https://www.senasa.gob.pe/senasacontigo/puno-garrapatas-en-proceso-de-identificacion-para-aplicar-mejoras-en-la-produccion-pecuaria/ (accessed January 8, 2025).
106. SENASA. Piura: Atención a ganadería familiar se mantiene vigente. SENASA Al Día (2020). Available online at: https://www.senasa.gob.pe/senasacontigo/piura-atencion-a-ganaderia-familiar-se-mantiene-vigente/ (accessed January 8, 2025).
107. INIFAP. Diagnóstico de la anaplasmosis bovina. gobmx (2023). Available online at: http://www.gob.mx/inifap/articulos/diagnostico-de-la-anaplasmosis-bovina (accessed January 8, 2025).
108. ICA. Toma de muestras a bovinos en La Guajira por notificaciones de cuadros compatibles con enfermedades hemoparasitarias | ICA – Instituto Colombiano Agropecuario. Portal Corp ICA (2023). Available online at: https://www.ica.gov.co/noticias/muestra-guajira-enfermedades-hemoparasitarias (accessed January 8, 2025).
109. Klafke, GM, Golo, PS, Monteiro, CMO, Costa-Júnior, LM, and Reck, J. Brazil’s battle against Rhipicephalus (Boophilus) microplus ticks: current strategies and future directions. Rev Bras Parasitol Vet. (2024) 33:e001423. doi: 10.1590/s1984-29612024026
110. Ministério da Agricultura, Pecuária e Abastecimento. Avaliação seletiva de bovinos para o controle do carrapato Rhipicephalus microplus. Brasilia, Brasil: Ministério da Agricultura, Pecuária e Abastecimento (MAPA). (2020). Available online at: https://www.gov.br/agricultura/pt-br/assuntos/producao-animal/arquivos-publicacoes-bem-estar-animal/CARRAPATOS2.pdf (Accessed January 13th, 2025).
111. Silva, EMC, Marques, ICL, De Mello, VVC, Amaral, RBD, Gonçalves, LR, MDSCO, B, et al. Neta AVDC. Molecular and serological detection of Anaplasma spp. in small ruminants in an area of Cerrado biome in northeastern Brazil. Ticks Tick Borne Dis. (2024) 15:102254. doi: 10.1016/j.ttbdis.2023.102254
112. Gaff, HD, White, A, Leas, K, Kelman, P, Squire, JC, Livingston, DL, et al. TickBot: a novel robotic device for controlling tick populations in the natural environment. Ticks Tick Borne Dis. (2015) 6:146–51. doi: 10.1016/j.ttbdis.2014.11.004
113. Sharma, A, Pham, MN, Reyes, JB, Chana, R, Yim, WC, Heu, CC, et al. Cas9-mediated gene editing in the black-legged tick, Ixodes scapularis, by embryo injection and ReMOT control. iScience. (2022) 25:103781. doi: 10.1016/j.isci.2022.103781
114. Gulia-Nuss, M, Nuss, AB, Meyer, JM, Sonenshine, DE, Roe, RM, Waterhouse, RM, et al. Genomic insights into the Ixodes scapularis tick vector of Lyme disease. Nat Commun. (2016) 7:10507. doi: 10.1038/ncomms10507
Keywords: anaplasmosis, Anaplasma marginale , Anaplasma phagocytophilum , Amazon basin, tick-borne pathogens
Citation: Mauri Pablo JD, Del Solar JJC, Hinojosa Enciso ET, Polveiro RC, Vieira DdS, Ramos Sanchez EM, Bardales Escalante W, Maicelo Quintana JL and Lopez Lapa RM (2025) Anaplasmosis in the Amazon: diagnostic challenges, persistence, and control of Anaplasma marginale and Anaplasma phagocytophilum. Front. Vet. Sci. 12:1571694. doi: 10.3389/fvets.2025.1571694
Edited by:
Yasser Mahmmod, Long Island University, United StatesReviewed by:
Sergio D. Rodríguez Camarillo, INIFAP, MexicoJoanna Hildebrand, University of Wrocław, Poland
Copyright © 2025 Mauri Pablo, Del Solar, Hinojosa Enciso, Polveiro, Vieira, Ramos Sanchez, Bardales Escalante, Maicelo Quintana and Lopez Lapa. This is an open-access article distributed under the terms of the Creative Commons Attribution License (CC BY). The use, distribution or reproduction in other forums is permitted, provided the original author(s) and the copyright owner(s) are credited and that the original publication in this journal is cited, in accordance with accepted academic practice. No use, distribution or reproduction is permitted which does not comply with these terms.
*Correspondence: Rainer Marco Lopez Lapa, cmFpbmVyLmxvcGV6QHVudHJtLmVkdS5wZQ==