- 1Department of Internal Medicine, Reproduction, and Population Medicine, Faculty of Veterinary Medicine, Ghent University, Merelbeke, Belgium
- 2Division of Animal and Human Health Engineering, Department of Biosystems, KU Leuven, Leuven, Belgium
- 3Equine Cardioteam Ghent, Department of Internal Medicine, Reproduction, and Population Medicine, Faculty of Veterinary Medicine, Ghent University, Merelbeke, Belgium
Introduction: The use of transvaginal ultrasound-guided follicle aspiration (TVA) for oocyte collection has become a widely used procedure in horses for in vitro embryo production (IVEP). The TVA procedure is characterized by various manipulations, which are physical restraint, perineal preparation, transrectal palpation, insertion of an intravaginal device, and needle punctures. These repeated transvaginal and transovarian punctures have raised concerns about their potential effects on mare welfare. Our study aimed to investigate the effects of TVA manipulations, and especially puncturing, on pain and stress in mares in a commercial set-up.
Methods: Therefore, eight mares were subjected to three TVA procedures: two with puncturing (P+) and one without (P−, control). Before, during and after all TVA procedures, blood was collected for serum glucose, lactate and cortisol levels, and facial pain scores were assessed. An electrocardiogram (ECG) was recorded for each mare before and during the procedure to measure heart rate (HR) and heart rate variability (HRV). Parameters in response to manipulations and puncturing were analyzed using linear mixed effect models for each outcome variable at different timepoints during the procedure.
Results: Results revealed that puncturing during TVA did not significantly influence serum metabolite levels, facial pain scores, HR, or HRV over the complete procedure (p > 0.05). Notably, HR did not increase at the moment of puncturing (p > 0.05), and no significant changes in HRV parameters between P+ and P− were detected (p > 0.05). Both P+ and P− procedures triggered significant increases in cortisol and lactate levels, facial pain scores, and HR during restraint in stocks and perineal preparation compared to the day before and the day after TVA (p < 0.05). Interestingly, even without puncturing, manipulations in the P− procedure were sufficient to induce significant elevations in metabolite levels and facial pain scores compared to the day before and after (p < 0.05).
Discussion: These results indicate that the TVA procedure induces stress responses in mares, predominantly associated with all manipulations specific to TVA, while the effect of puncturing itself was minimal. All effects were acute, with parameters returning to baseline when measured 24 h later.
1 Introduction
In horses, in vitro embryo production (IVEP) through transvaginal ultrasound-guided follicle aspiration (TVA) followed by intracytoplasmic sperm injection has emerged in the early 1990s as an innovative breeding technique, offering a more advanced alternative to traditional embryo flushing (1–4). TVA involves aspirating follicles directly from the ovaries using an ultrasound-guided needle passed through the vaginal wall, allowing for oocyte retrieval from mares. The commercial demand for these in vitro produced embryos among breeders and owners has grown over the past decade, driven by the improvement in the production of transferable blastocysts and pregnancy success rates (1, 5, 6). According to the International Embryo Transfer Society, in vitro produced embryos have outnumbered those produced by embryo flushing in Europe (1, 7). The efficiency, versatility, and high pregnancy rates achieved with in vitro produced embryos, along with easier embryo cryopreservation and facilitation of international trade, could explain why it now outperforms traditional breeding methods (1, 2, 6, 8, 9).
Despite its benefits, it is crucial to highlight that TVA involves various manipulations, including transrectal palpation, insertion of an intravaginal device, and multiple needle punctures into the vagina wall, peritoneum, and ovaries. Additionally, the same mare often undergoes multiple TVA sessions, sometimes as frequently as every 2 weeks. In women, the TVA procedure is considered painful (10, 11), with the level of pain associated with the diameter of the TVA needle (12, 13), suggesting the need to recognize and minimize discomfort in animals. Limited data exist on the well-being of mares during and after TVA, as most studies focus on health and fertility (8, 14–16). Currently, only a few studies, mainly limited to livestock, have investigated the welfare of animals undergoing reproductive interventions, and specifically TVA. In cattle, TVA induces moderate stress, but repeated procedures do not harm well-being (17–20). In sows, stress is temporary, with feeding drive outweighing discomfort (21). In mares, transrectal ultrasound causes stress (22) and needle puncturing during TVA increases heart rate (23).
Assessing pain and stress in horses is challenging due to the involvement of different physiological systems (e.g., neuroendocrine and cardiovascular) and the stoic nature of prey animals (24, 25). Stress is broadly defined as a disruption of homeostasis or an animal’s inability to cope with its environment (19, 26). As no single parameter is highly specific to pain and stress, an integrated approach that monitors a variety of indicators, including physiological and behavioral parameters, has traditionally been used (24, 25). The behavioral and physiological parameters used in this study were motivated by the two main pathways, the hypothalamic–pituitary–adrenal cortex (HPA) axis and the sympathetic-adrenal medulla (SAM) axis, activated by the equine stress response (27–29). Previous studies in livestock typically evaluated stress during TVA by monitoring behavioral responses, measuring cortisol and ACTH levels, assessing milk production, and tracking HR (18, 19, 21, 23). Other valuable markers to monitor stress and pain in animals are validated pain scales, lactate and glucose levels, and heart rate variability (HRV). Validated pain scales offer a more objective method to monitor pain over time (30), serum lactate and glucose levels are well-established indicators of acute stress (30–33), and HRV reflects the balance between sympathetic and parasympathetic tone and is thus commonly used to assess the autonomic nervous system’s stress response (34). To our knowledge, no previous study in horses investigated the effect of TVA manipulations and puncturing on serum lactate and glucose levels, and HRV.
Since animal welfare is becoming a priority in breeding programs, it is crucial to assess whether the TVA procedure affects the well-being of mares. The main concern with TVA is the repeated transvaginal and transovarian punctures, which are both invasive and specific to this procedure. We hypothesized that all manipulations inherent to the TVA procedure may cause discomfort and stress, with puncturing during TVA being the most sensitive step. Therefore, the aim of the present study was to evaluate the effects of all TVA-related manipulations, and puncturing in particular, on pain and stress in mares in a commercial program, including the standard medication protocol. This was achieved by monitoring HR, HRV, facial expressions, glucose, lactate and cortisol levels during and between procedures.
2 Materials and methods
2.1 Study design
Eight clinically healthy mares of mixed breeds, aged between 5 and 18 years old, were included in this study. All mares were housed in individual boxes with ad libitum access to water and hay. The animals were familiar with the facility, its staff, and the protocols used in the procedure. All experimental protocols were approved by the Ethical Committee of Ghent University (reference number 2023–075).
One month prior to the study, a 24-h electrocardiogram (ECG) was recorded using a Televet100 recording system (Engel Engineering Services GmbH, Germany) to establish the normal cardiovascular parameters, including HR and HRV, for each mare in the stable.
Each mare (Horse A-H) underwent three sessions (Replicate 1–3), with a 14-day interval between each session. The first two TVA sessions involved puncturing (P+), whereas the third session, without puncturing, served as a control (P−). The sessions were conducted between January and March 2024. All experiments were performed between 08 h00 and 12 h00 to account for the circadian rhythm in cortisol release (35). The protocol for each session is outlined below (Figure 1), with detailed methods described further:
• Day before TVA: A facial pain score assessment and blood tests were performed. An intravenous catheter (Mila IV catheter 14G 2.00 × 130 mm; Mila International, Kentucky, United States) was placed in the jugular vein.
• Day of TVA: The ECG recording system was fitted to each mare in the cardiology examination room to continuously monitor HR and HRV. Once the recording system was in place, the mares were moved to the stocks for the TVA procedure. Ten minutes before the start of the TVA procedure, while the mare was in the stocks and after the medication was administered, the first blood sample was collected and the facial pain score was assessed (T−10). The start of the procedure was defined as the moment the intravaginal probe was inserted and the ovary was manipulated (T0). Subsequent blood samplings and facial pain score evaluations were conducted at 10-min intervals throughout the procedure, continuing until 10 min after its completion, while the mare remained in the stocks (T10, T20, T30, T40).
• Post-TVA follow-up: On Day 1 and 3 post-TVA, a comprehensive clinical examination (respiratory rate, HR, and body temperature), facial pain score assessment, and blood sampling were repeated for each mare.
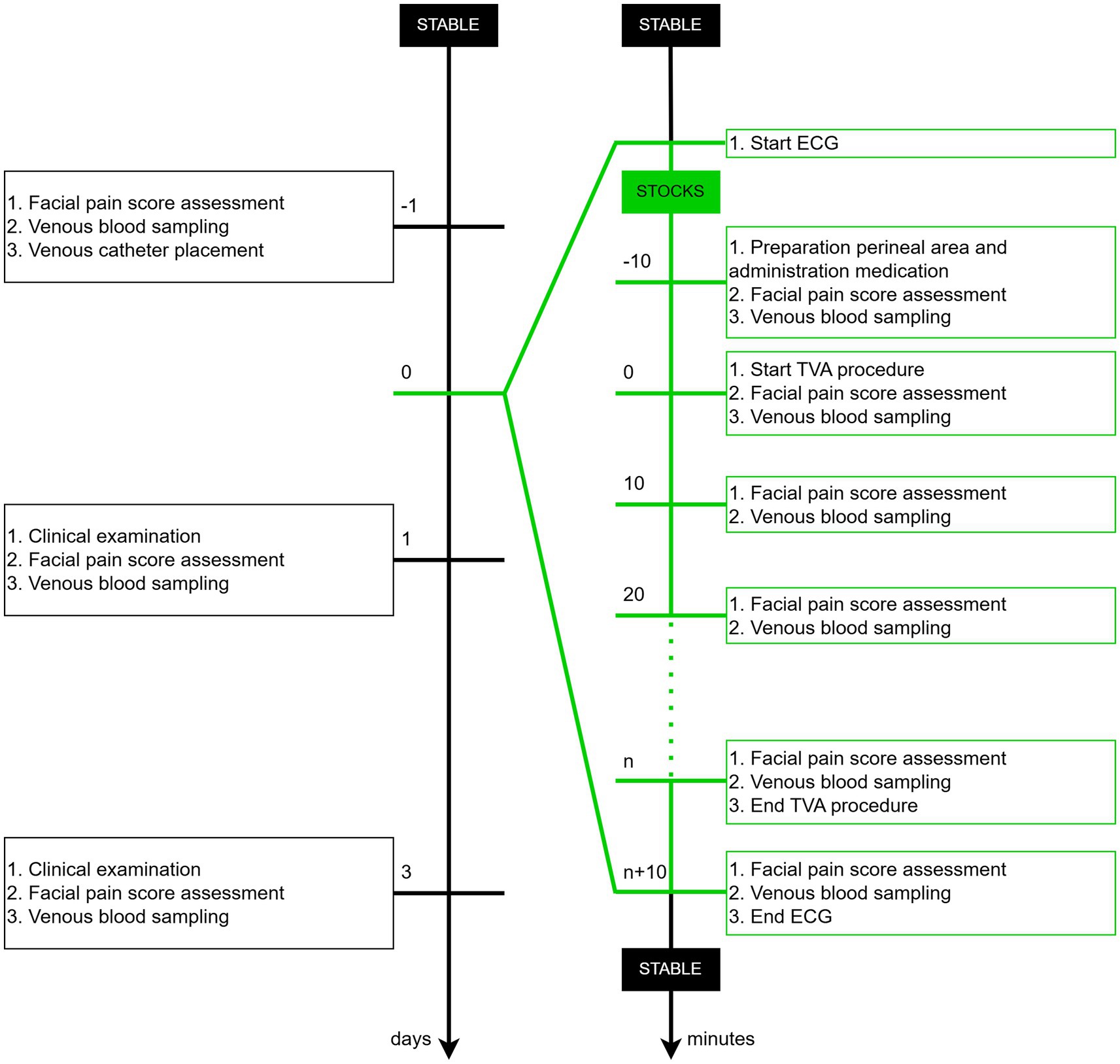
Figure 1. Timeline of the experimental protocol depicting key procedures in chronological order across days and minutes.
2.2 Description of critical methods
2.2.1 Transvaginal ultrasound-guided follicular aspiration
The mares were restrained in the stocks within the examination room for the TVA procedure. The tail was wrapped and secured, the rectum was emptied, and the perineal region was cleaned thoroughly at least three times using 7.5% polyvidone iodine antiseptic soap (Iso-Betadine Germicidal Soap 7.5%; Viatris Healthcare, Pennsylvania, United States). Each mare was administered following medication prior to the TVA procedures:
• 15 μg/kg detomidine IV (Domidine, 10 mg/mL detomidinehydrochloride; Dechra, The Netherlands),
• 10 mg butorphanol IV (Dolorex, 10 mg/mL butorfanol (tartraat); MSD Animal Health, Belgium),
• 1 mg/kg flunixine meglumine IV (Emdofluxin, 50 mg/mL flunixine meglumine; Emdoka, Belgium),
• 21,000 IE/kg procaïne benzylpenicilline IM (Peni-Kel, 300,000 IE/mL procaïne benzylpenicilline; Kela, Belgium),
• 0.2 mg/kg butylhyoscine bromide IV (Buscopan Compositum ad us. Vet., 500 mg/mL natriummetamizol +4 mg/mL butylhyoscine bromide; Boehringer Ingelheim, Germany).
A constant rate infusion of 2 mL/100 kg was administered, consisting of 19 mL isotonic sodium chloride solution and 1 mL Domidine (10 mg/mL detomidinehydrochloride; Dechra, The Netherlands). Additional doses of detomidine [5 μg/kg detomidine IV (Domidine, 10 mg/mL detomidinehydrochloride; Dechra, The Netherlands)] were administered if excessive movement interfered with the operability of the manipulator during the procedure, ensuring the safety of the animal and personnel, and facilitating the completion of the TVA.
After aseptic preparation of the perineal area, a transvaginal ultrasound probe (Esaote EC123 Intra CavityA; Esaote, Italy), with a frequency range of 5.0–7.5 MHz, was fitted with a custom needle guide and inserted into the vagina. The ovary was then manipulated and fixed by rectal palpation against the vaginal wall by an experienced veterinarian. During the P+ procedure, a 12 G x 25 inch double-lumen oocyte aspiration needle (Minitube, Germany) was inserted through the transducer needle guide and once a follicle was visualized, the needle was introduced into the ovary through the vaginal wall, and the follicle was aspirated. All visible follicles were punctured, aspirated, and flushed 10 times with 1–3 mL of prewarmed flushing medium (Equiplus; Minitube, Germany) using a vacuum pump (Minitube, Germany). During aspiration, the follicle walls were scraped by rotating the needle. The P+ procedure ended when all follicles were aspirated.
During the P− procedure, the mares underwent the same procedure with identical manipulations (including preparation of the perineum, insertion of the intravaginal ultrasound probe, and rectal manipulation in search of follicles and thus with traction on the broad ligament), but no needle was introduced. The mares received the same medications but no antibiotics; instead 7 mL/100 kg physiological saline IM was administered as a placebo (NaCl 0.9% Ecoflac Plus; B. Braun, Germany). The P− procedure ended after 17 min of rectal manipulation on each ovary, corresponding to the expected duration of a TVA.
2.2.2 Catheter placement
Handling and restraining animals during blood collection can be stressful and can lead to increased cortisol levels; therefore, an intravenous catheter (Mila IV catheter 14G 2.00 × 130 mm; Mila International, Kentucky, United States) was placed the day before to avoid this potential confounding factor. To place the intravenous catheter into the jugular vein, the jugular groove was located and clipped at the midpoint of the middle third of the jugular vein. The clipped area was aseptically prepared using alternating scrubs of chlorhexidine and alcohol. A volume of 1–2 mL of 2% lidocaine was administered subcutaneously at the planned insertion site to provide local anesthesia and desensitize the area. The jugular vein was occluded manually at the thoracic inlet and the catheter was introduced at a 30–45° angle, oriented caudally in alignment with the direction of venous blood flow. Upon observing a flash of blood in the catheter hub, the stylet was removed, and the catheter was advanced fully into the vein. A sterile perfusion line with a three-way stopcock was attached to the catheter to allow for fluid administration and secure port access. The catheter was then sutured to the skin to ensure stability and prevent dislodgement.
2.2.3 Blood sampling and serum metabolite measurements
Blood was collected aseptically into serum and fluoride/oxalate tubes from the jugular vein before and after the procedure, and from the catheter during the procedure. Samples were stored at 4°C until analysis. Lactate and glucose levels were measured in-house using a RAPIDPoint 405 Blood Gas Analyzer (Siemens Healthcare Diagnostics, Germany). Cortisol levels were sent the same day to an external laboratory for analysis using electrochemiluminescence immunoassays with the Elecsys Cortisol Assay Kit (Roche Diagnostics, Germany; catalog number 11875116). The assay has a detection range of 0.5–1750 nmol/L, with intra-assay and inter-assay coefficients of variation below 5%.
2.2.4 Facial pain score
The Equine Utrecht University Scale for Facial Assessment of Pain (EQUUS-FAP), as described by van Loon and Van Dierendonck (30), was used to assess facial pain scores. This was consistently performed by the same veterinarian. This multifactorial descriptive scale is specifically designed to monitor acute visceral pain in horses and is based on 9 parameters, describing different elements of facial expression (head, eyelids, focus, nostrils, corners mouth/lips, muscle tone head, flehming and/or yawning, teeth grinding and/or moaning, and ears). Each of the nine parameters can be scored from 0 to 2, leading to a total pain score ranging from 0 (no signs of pain) to 18 (maximal pain score) (Table 1).
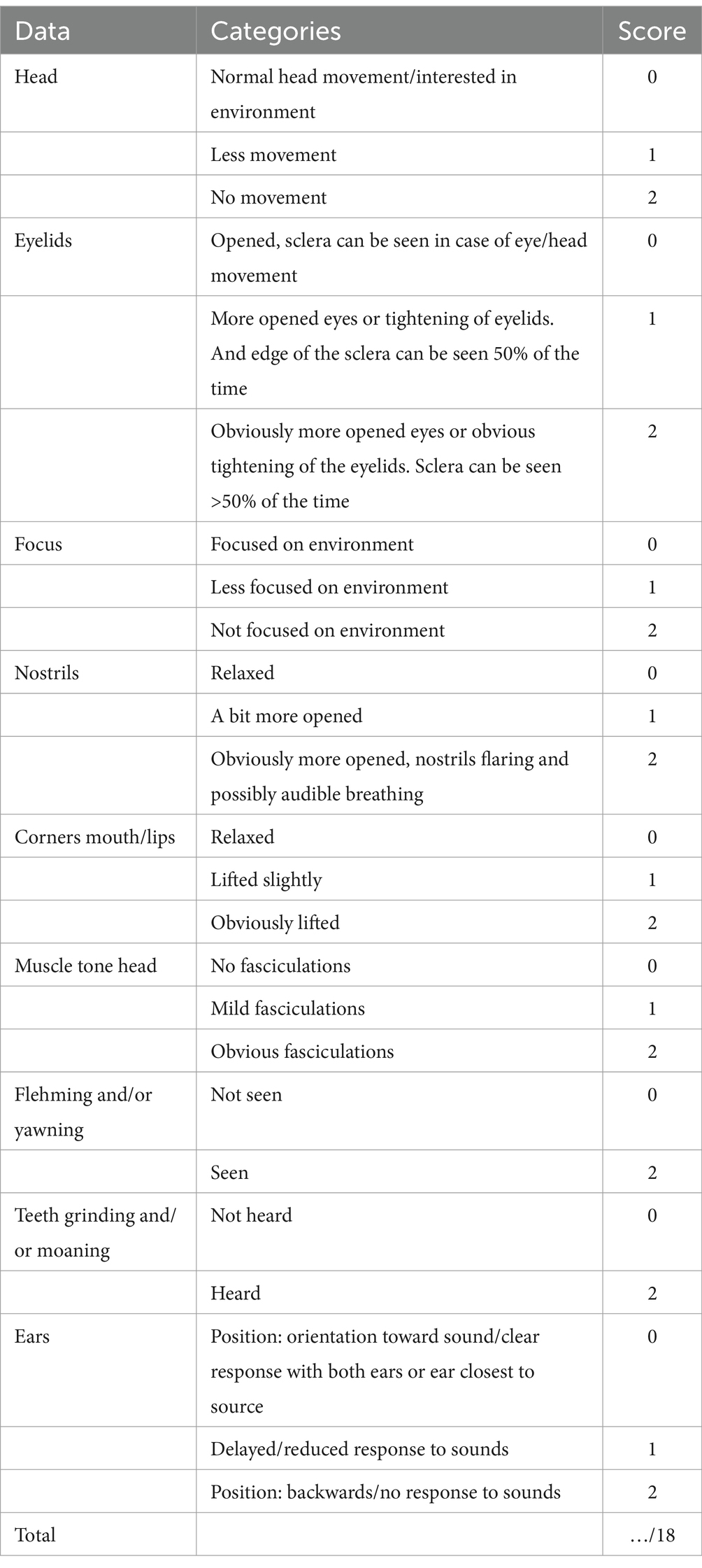
Table 1. Score sheet of the Equine Utrecht University Scale for Facial Assessment of Pain (EQUUS-FAP) (29).
2.2.5 Heart rate and heart rate variability
In all mares, ECG recordings were obtained using a Televet100 recording system as described by Broux et al. (36). Four self-adhesive electrodes were placed underneath a girth (Mainat Vet, Spain). The right arm electrode was positioned 15 cm right of the withers, the left leg electrode caudal to the left elbow on the thorax, and the left arm electrode 10 cm above the left leg electrode resulting in a modified base-apex configuration. A reference electrode was placed on the left side of the withers. All electrodes were connected to the recording device, placed in the girth.
ECG data were processed with custom Matlab (Mathworks, MA, United States) scripts for filtering, normalization and QRS complex detection (37). Automatic QRS markers were manually corrected. Bifid P waves were identified within specific windows set by Q onset and T wave offset, considering amplitude, slope, morphology, and prominence to detect P onset (Pon), P1 peak and P2 peak. Ten HRV parameters were calculated from the different P wave indices and RR intervals over 2 min time windows. Considering the acceptable standards for ECG recordings, the usage of 2-min intervals for determining the 10 HRV parameters, specifically the SDNN parameter, is shorter than the 5-min standard. However, the choice of shorter segments was made in the context of our brief intervention.
Four time domain parameters were determined: SDNN (standard deviation of the RR intervals), RMSSD (root mean squared successive differences in RR intervals), pNN50 (proportion of intervals above 50 ms) and TRI (triangular index; integral of RR interval histogram divided by height of the histogram); four frequency domain parameters were calculated: very low- (VLF, 0.0033–0.04 Hz), low- (LF, from 0.04–0.15 Hz), and high-frequency (HF, 0.15–0.4 Hz) components, and LF/HF ratio (the ratio of the low frequency band over the high frequency band); two nonlinear parameters were determined: SD1 and SD2 (standard deviation of the Poincaré plot perpendicular to and along the line of identity, respectively). For a comprehensive overview of HRV variables, including their baseline values and variation ranges, please refer to Supplementary Table 1.
2.3 Statistical analysis of data
All statistical analyses were performed using R (38), version 4.4.0 including packages “tidyverse” (39), “skimr” (40), “lme4” (41), “lmerTest” (42), “performance” (43), “sjPlot” (44), “ggforce” (45), “emmeans” (46), “ggpubr” (47), “simr” (48). All statistical analyses including code scripts can be consulted at https://doi.org/10.5281/zenodo.14245595.
Serum metabolite levels, facial pain scores, HR, HRV and sedation levels in response to puncturing (P+/P−) were analyzed using linear mixed effect models for each outcome variable. For serum metabolite levels and facial pain scores, puncturing and time were included as fixed effects, horse as a random intercept, and replicate as a random slope. For HR, two models were made. In the first HR model, housing environment (stable or stocks) and sedation were included as fixed effects, and horse as a random intercept. In the second HR model, sedation, intravaginal device insertion, rectal manipulation, and puncturing were included as fixed effects, horse as a random effect, and replicate as a random slope. HRV parameters were analyzed in a specific time window between the insertion and removal of the intravaginal device. To normalize these parameters, we applied a natural logarithm transformation to SDNN, RMSSD, TRI, SD1, and SD2, and a square root transformation to pNN50, VLF, LF, HF, and the LF/HF ratio. For HRV analysis, puncturing was included as a fixed effect, horse as a random intercept, and replicate as a random slope. For sedation levels, the total dosage of detomidine administered during the procedure was calculated per kilogram body weight per minute. Next, a natural logarithm transformation was applied. Puncturing was included as a fixed effect and Horse as a random effect. Statistical significance was defined at p-value ≤0.05.
3 Results
3.1 Descriptive analysis
Baseline measurements taken 24 h before all TVA procedures showed average cortisol levels of 5.3 μg/dL [normal range: 1.5–7.0 μg/dL (49–51)], lactate levels of 0.7 mmol/L [normal range: 0.0–1.5 mmol/L (52)], glucose levels of 90 mg/dL (normal range: 75–115 mg/dL (53)), and facial pain scores averaging 1.25 [normal range: 0–3 (30)]. Each mare underwent a median of six transovarian punctures (range: 5–8) per P+ procedure, with a median of 11 follicles aspirated (range: 7–32), and an oocyte recovery rate of 56% ± 18%. The average time per TVA procedure, counted from the insertion to the removal of the intravaginal device, was 35 min. The longest TVA procedure lasted 56 min; however, since it involved only one procedure and given the study’s model design, times over 50 min were excluded from statistical analysis. All clinical examinations performed on Day 1 and 3 after TVA procedure were within normal physiological levels.
3.2 Model performances
3.2.1 Metabolites
No significant effect on cortisol levels was found for P+ when compared to P− in the main effect, but a significant interaction effect was found between puncturing and time. At individual timepoints, no differences were observed between P+ and P−, as shown in Figure 2A. For both P+ and P−, cortisol levels on Day 1 and 3 were significantly lower than during the procedure (T−10, T0, T10, T20, T30, and T40). Similarly, P+ showed significantly lower cortisol levels on Day-1 compared to procedural levels. Within the P+ procedure, cortisol levels at T20 and T30 were higher than at other timepoints. Additional significant interaction effects were found at T10, T20, and T30, and are shown in Figure 2B.
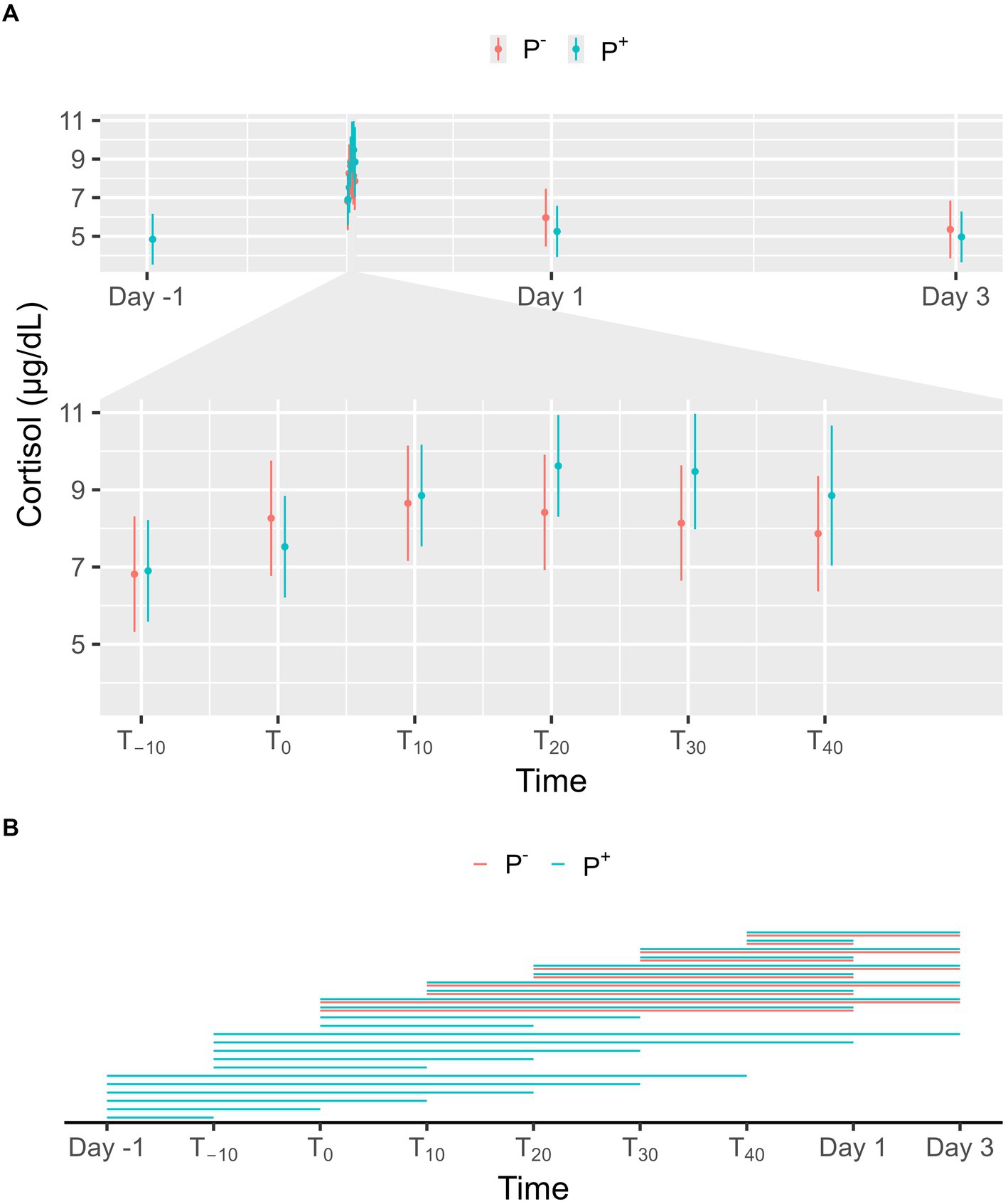
Figure 2. (A) The interaction between time and puncturing in the linear regression model with cortisol (μg/dL) as the dependent variable is shown. The least-squares means (LSM) of puncturing are plotted in 2 categories: no puncturing (P−) during transvaginal ultrasound-guided follicle aspiration (TVA) (red) and puncturing (P+) during TVA (blue). The bars represent the 95% CI of these effects. Significant differences (p < 0.05) found in a pairwise comparison between the LSM within each timepoint are indicated with “*.” (B) Pairwise comparison within the P+ (blue) and P− (red) procedure over the different timepoints are plotted in this panel. A line between two timepoints indicates a significant difference (p < 0.05).
No significant effect on lactate levels was found for P+ when compared to P− in the main effect, but a significant interaction effect was found between puncturing and time. No difference between P+ and P− was found at any specific timepoint, as shown in Figure 3A. For P−, lactate levels on Day 1 and 3 were significantly lower than during the procedure (T−10, T0, T10, T20, T30, and T40). For P+, lactate levels were significantly lower on Day-1 (except at T40), on Day 1 (except at T30 and T40), and on Day 3 compared to procedural levels. Additionally, lactate levels for P+ were significantly higher on Day 1 compared to Day 3. During the procedure, lactate levels at T−10 and T0 were significantly higher than at other timepoints for both P+ and P−. Other significant interaction effects were found at T−10, T0, T30, and T40 and are shown in Figure 3B.
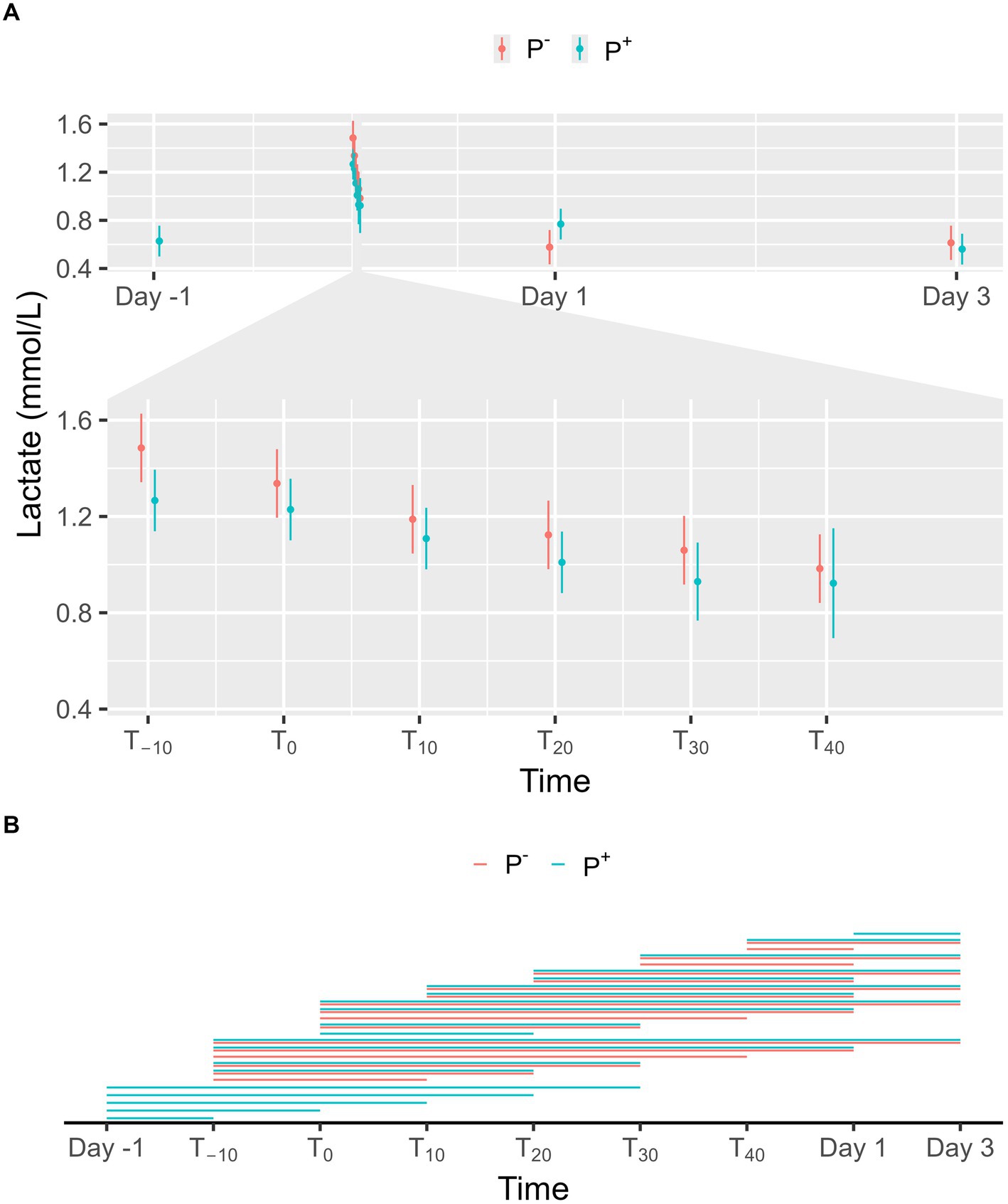
Figure 3. (A) The interaction between time and puncturing in the linear regression model with lactate (mmol/L) as the dependent variable is shown. The least-squares means (LSM) of puncturing are plotted in 2 categories: no puncturing (P−) during transvaginal ultrasound-guided follicle aspiration (TVA) (red) and puncturing (P+) during TVA (blue). The bars represent the 95% CI of these effects. Significant differences (p < 0.05) found in a pairwise comparison between the LSM within each timepoint are indicated with “*.” (B) Pairwise comparison within the P+ (blue) and P− (red) procedure over the different timepoints are plotted in this panel. A line between two timepoints indicates a significant difference (p < 0.05).
No significant effect on glucose levels was found for P+ when compared to P− in the main effect, but a significant interaction effect was found between puncturing and time. At T10, T20 and T40, glucose levels were significantly higher in P+ compared to P−, as shown in Figure 4A. For P−, glucose levels on Day 1 and 3 were significantly lower than at T20, T30, and T40. For P+, glucose levels on Day-1, Day 1, and Day 3 were significantly lower than at T10, T20, T30, and T40. During the procedure, glucose levels at T−10, T0, and T10 were significantly lower than at later timepoints for both P+ and P−. Additional significant interaction effects were found for T−10, T0, and T10 and are shown in Figure 4B.
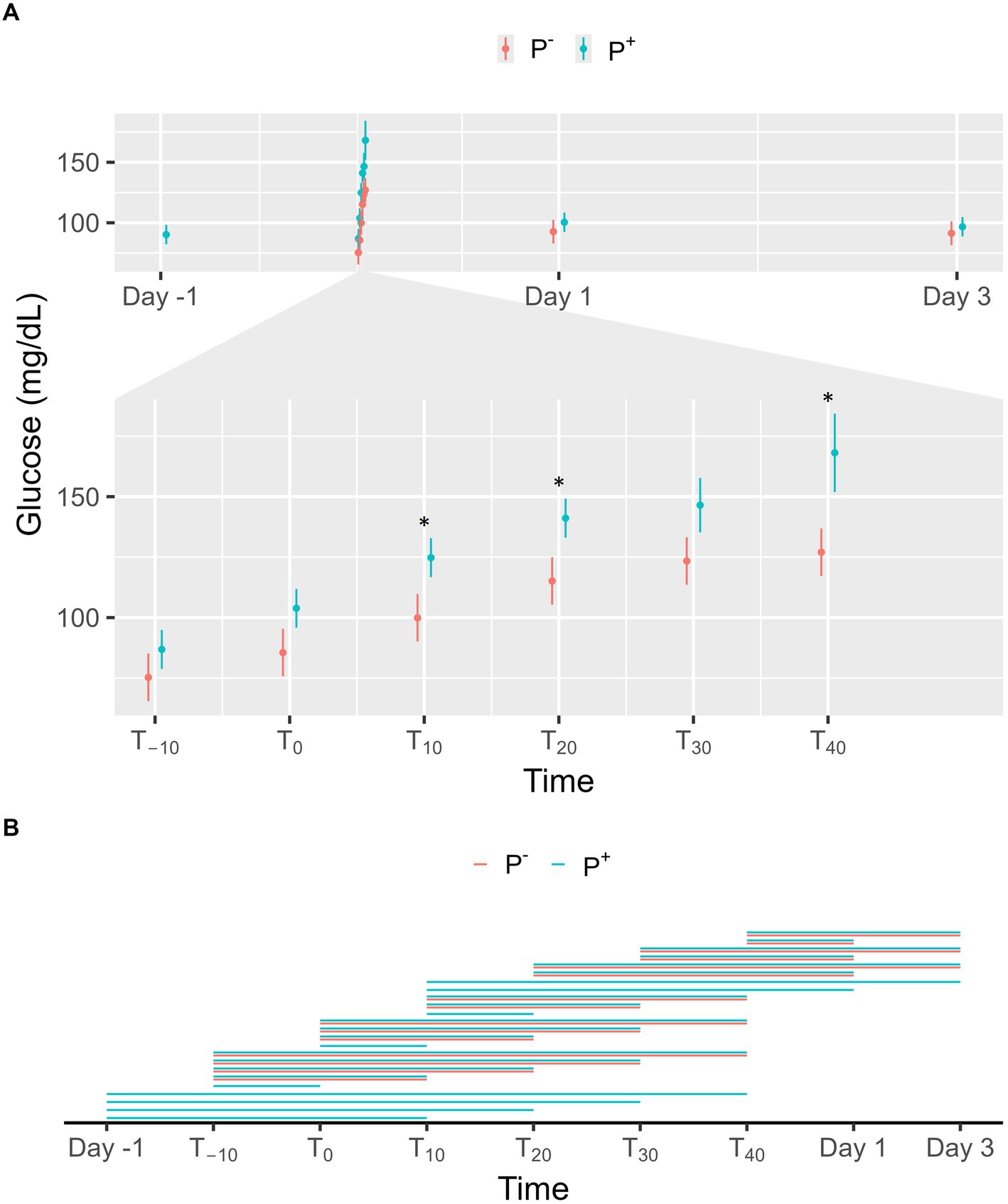
Figure 4. (A) The interaction between time and puncturing in the linear regression model with glucose (mg/dL) as the dependent variable is shown. The least-squares means (LSM) of puncturing are plotted in 2 categories: no puncturing (P−) during transvaginal ultrasound-guided follicle aspiration (TVA) (red) and puncturing (P+) during TVA (blue). The bars represent the 95% CI of these effects. Significant differences (p < 0.05) found in a pairwise comparison between the LSM within each timepoint are indicated with “*.” (B) Pairwise comparison within the P+ (blue) and P− (red) procedure over the different timepoints are plotted in this panel. A line between two timepoints indicates a significant difference (p < 0.05).
3.2.2 Facial pain score
No significant effect on facial pain scores was found for P+ when compared to P− in the main effect, but a significant interaction effect was found between puncturing and time. As shown in Figures 5A, a significant difference was found at T30 with facial pain scores being higher in P+ than in P−. For P−, facial pain scores on Day 1 and 3 were significantly lower than during the procedure (T−10, T0, T10, T20, T30, and T40). For P+, facial pain scores were significantly lower on Day-1, on Day 1, and on Day 3 compared to procedural levels. During the procedure, facial pain scores at T−10 and T0 were significantly lower than at later timepoints for P+. Additional significant interaction effects were found for T−10 and T0 and are shown in Figure 5B.
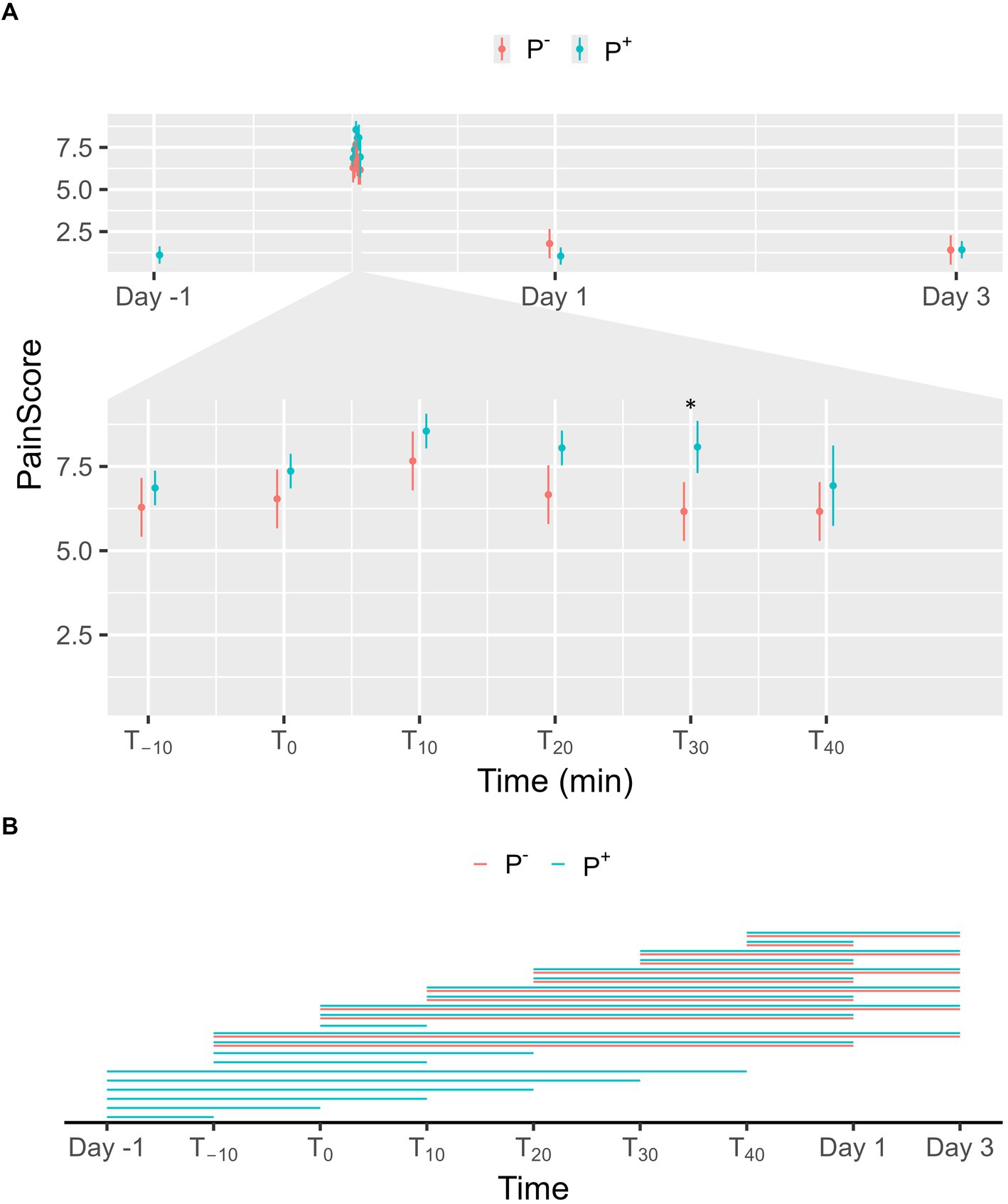
Figure 5. (A) The interaction between time and puncturing in the linear regression model with facial pain score [according to Equine Utrecht University Scale for Facial Assessment of Pain (EQUUS-FAP)] as the dependent variable is shown. The least-squares means (LSM) of puncturing are plotted in 2 categories: no puncturing (P−) during transvaginal ultrasound-guided follicle aspiration (TVA) (red) and puncturing (P+) during TVA (blue). The bars represent the 95% CI of these effects. Significant differences (p < 0.05) found in a pairwise comparison between the LSM within each timepoint are indicated with “*.” (B) Pairwise comparison within the P+ (blue) and P− (red) procedure over the different timepoints are plotted in this panel. A line between two timepoints indicates a significant difference (p < 0.05).
3.2.3 Heart rate and heart rate variability
An estimated increase of 22.6 bpm in HR was found when the mares were placed in the stocks. Sedation significantly decreased HR, with an estimated decrease of 8.5 bpm. No effect was observed on HR for intravaginal device insertion, rectal manipulation, and puncturing. No effect on SDNN, RMSSD, TRI, VLF, LF, HF, LF/HF, SD1, and SD2 was found between the P+ and P− procedure.
3.2.4 Sedation
Not more than one additional dose of detomidine was given to a mare. An additional dose was administered to four mares in replicate 1, to one mare in replicate 2, and to one mare in replicate 3. The median time an additional dose was given was at 24 min after T0 (range: 14–41 min). There was a significant effect on sedation found between P+ en P− procedures with detomidine doses being significantly higher during P+ procedures. The estimated effect of LSM for P+ was 55 μg detomidine/100 kg/min ± 0.052 and for P− was 39 μg detomidine/100 kg/min ± 0.077.
4 Discussion
The objective of this study was to evaluate the impact of the manipulations characteristic to the TVA procedure, as applied in clinical practice, on pain and stress in mares. We aimed to replicate the conditions of a commercial TVA program by ensuring a sufficient number of follicles for aspiration and scheduling procedures at two-week intervals. The number of follicles aspirated and the oocyte recovery rate aligned with clinical studies reporting an average of 14–26 aspirated follicles per procedure and oocyte recovery rates of 50–70% (2, 54–57). The sample size of 8 mares and the age range (5–18 years) may introduce variability in the results. Despite the age differences, it is important to note that each mare served as their own control. To address this, we have considered individual variances in our analysis by using mixed linear models. This method allows us to build a separate model for each horse, ensuring the consideration of individual variations. Nevertheless, future research with larger sample sizes could further enhance the robustness of these findings.
Our findings revealed that puncturing during TVA (P+) did not significantly affect serum metabolite levels nor facial pain scores over the complete procedure. Additionally, HR did not increase at the time of puncturing, and no changes in HRV parameters were observed, when assessed from the insertion up to the removal of the intravaginal device to isolate the effect of puncturing. However, for serum metabolite levels and facial pain scores, differences were observed between specific timepoints and could indicate an effect of puncturing on the course. Despite this, no significant differences were observed between P+ and P− at individual timepoints, except for serum glucose levels (T10, T20, T40) and facial pain scores (T30).
During restraint in stocks and perineal preparation, serum cortisol and lactate levels and facial pain scores significantly increased in both P+ and P−. Restraint and preparation were also associated with an estimated increase in HR of 22.6 bpm. These responses may reflect an anticipatory stress response, because the mares were already familiar with the procedure and the associated manipulations. In this sense, it would be valuable to study naïve mares to determine whether similar responses occur without prior experience. However, during the manipulations in the P− procedure (from T0 onwards), we observed significant increases in serum cortisol and glucose levels and facial pain scores compared to T−10. This aligns with findings from Schönbom et al. (22), who observed a rise in salivary cortisol following transrectal examination. These results suggest that restraint in stocks, preparation of the perineum, insertion of an intravaginal device and rectal manipulation cause an activation of the HPA and SAM axis, indicating not only puncturing itself but all manipulations induce discomfort in mares. Importantly, facial pain scores, HR, and serum metabolite levels returned to baseline after the procedure as measured on Day 1 and 3, suggesting the observed stress response is acute.
The anticipatory stress response, observed by increased serum cortisol and lactate levels, elevated facial pain scores and higher HR at T−10 compared to stable measurements, may arise from the mares associating the preparation routine with the TVA procedure itself. This is in accordance with the stress responses documented in horses during activities like loading onto a transport vehicle or being groomed and saddled before riding (58, 59). However, the supposed anticipatory stress response is not reflected in glucose levels in the P+ and P− procedure. While the control procedure was conducted last, potentially affecting the anticipatory response, this is unlikely as the mares in this study had undergone multiple TVA procedures prior to the study. This suggests they were already familiar with the procedure, reducing potential confounding effects related to procedural novelty. However, future studies should investigate the cumulative impact of repeated TVA procedures over time.
In contrast to our findings, Diego et al. (23) reported that pain induced by grabbing the ovary and pulling the ligament appeared to be controlled under their experimental conditions, despite using similar medication but without flunixin meglumine. They observed no significant differences in salivary cortisol levels, respiratory rate, or facial expressions between the TVA and control groups, and neither during the TVA procedure overall. Their control group was prepared for the TVA procedure (emptying of the rectum, cleaning of the perineum and placement of a urinary probe) but no intravaginal device was inserted and no rectal manipulations were performed. It is worth noting that they did not measure basal salivary cortisol levels, so it is possible that the mares in their study experienced an anticipatory stress response, as their salivary cortisol levels measured before entry were already elevated above typical resting levels of 0.06–0.11 μg/dL (35). They also suggested that the lack of difference in cortisol between the groups could be attributed to a delay in the detection of salivary cortisol concentrations.
Our findings contribute valuable insights, advancing the current knowledge in this area. However, the monitored parameters may be influenced by some of the administered medications, but administration of medication is intrinsic to this procedure and cannot be left out due to ethical reasons. For instance, butylscopolamine is known to induce tachycardia, while butorphanol and detomidine are both associated with bradycardia, with detomidine additionally causing hyperglycemia (60–62). In horses, constant rate infusion of butorphanol has also been reported to decrease cortisol concentrations (63), and a more recent study in calves found that flunixin meglumine reduces plasma cortisol levels (64). However, the same dosages, adjusted per body weight, were administered to all mares in each procedure, except for detomidine. Therefore, any potential effects on the results between P+ and P− due to the administration of butylscopolamine, butorphanol, and flunixin meglumine were likely minimal. A control group undergoing TVA without medication would reflect the ideal positive control to determine the effect of the different aspects of the procedure on pain and stress from a scientifical point-of-view. However, this is ethically not feasible and it was also not the scope of our study.
The detomidine dosage used in P+ procedures was significantly higher compared to P− procedures. While the initial dosage of detomidine (15 μg/kg) was standardized for all mares, additional doses (5 μg/kg) were administered as needed to minimize excessive movement that interfered with manipulator operability. Most physiological and behavioral parameters showed no significant differences between P+ and P− procedures at any specific timepoint. Importantly, no additional doses of detomidine were administered between T−10 and T10, suggesting that any difference in parameters observed between P+ and P- during these timepoints cannot be ascribed to variations in detomidine dosage. At these specific timepoints (T−10, T0, T10), no significant differences were observed between P+ and P− for most parameters, except for glucose levels, which were elevated at T10. Additionally, significantly higher glucose levels in P+ at T20 and T40 observed may be attributed to the higher dosage of detomidine administered after T10. Importantly, despite sedation, all procedures showed clear indicators of pain and stress. This implies that, while sedation may effectively provide chemical restraint and suppress expressions of stress during TVA, it may not fully alleviate discomfort from manipulations inherent to the procedure. These findings align with Wilson et al. (65), who observed that detomidine infusion provides prolonged chemical restraint in standing horses but insufficient analgesia for surgical interventions.
The EQUUS-FAP scoring system evaluates pain in horses using 9 facial expression parameters, each scored from 0 to 2 points. A score of 4 points or higher indicates the presence of pain, while a score of 6 points or higher signifies severe pain (30). During the TVA procedure, mares’ scores averaged 7, ranging from 0 to 12, indicating severe pain during the procedure. However, a key limitation is the lack of a validated facial pain scoring system for sedated horses. This makes it challenging to determine whether observed facial expressions reflect pain or sedation. The FaceSed score (66) has shown that sedation significantly affects facial features, such as ear position, orbital opening, and lip relaxation, potentially confounding the assessment of pain with the EQUUS-FAP scoring system. Therefore, these findings should be interpreted with caution. In addition, further studies should aim to develop a pain scoring scale for urogenital pain implementing a checklist to guide sedation and analgesia based on real-time pain evaluations. Importantly, all mares scored a maximum of 3 on Day 1 and 3 after the TVA procedure, showing that the mares experience no pain after the procedure.
Future research could explore alternative methods of pain relief, such as epidural anesthesia or topical gels to relieve the pain experienced when inserting the vaginal probe and to alleviate the tension on the rectal sphincter and hindgut during rectal manipulation. Epidural anesthesia is already standard practice in some equine reproductive clinics, while others use it only selectively. However, recommended dosage of epidural anesthesia desensitizes the anus, rectum, perineum, vulva, vagina, urethra, and bladder, but not the ovaries and ligaments (67). Administration of larger dilutional volumes of drugs into the epidural space may facilitate cranial migration of the drugs but would also cause ataxia or general discomfort in the horse (68). A comparative study examining the effects of TVA with and without epidural anesthesia on pain and stress in mares would be valuable. Although Petyim et al. (18) found that in cattle epidural anesthesia itself caused more stress than the TVA procedure, this area still warrants further investigation. Similarly, the effect of topical gels, like those used in human medicine to reduce pain during intrauterine device insertion, could also be studied. While McNicholas et al. (69) observed no significant reduction in pain with lidocaine gel during intra-uterine device insertion in women, testing various topical gels in mares may yield different results.
In conclusion, the present study investigated the physiological and behavioral responses of mares to the TVA procedure, by evaluating HR, HRV, facial expression changes, glucose, lactate, and cortisol levels before, during, and after the procedure. Restraint in stocks and preparation for the procedure were associated with significant stress, indicating an anticipatory stress response. Signs of discomfort, evidenced by elevated physiological and behavioral parameters, were observed during rectal and vaginal manipulations, while the effect of puncturing itself was minimal. These effects were acute, with all parameters returning to baseline the following day. Although this study established a solid foundation for quantifying pain and stress in mares during the TVA procedure, further research is needed. Efforts should focus on refining the TVA procedure, developing improved pain management protocols, and exploring innovative technologies for more accurate pain and stress assessment to enhance equine welfare.
Data availability statement
The raw data supporting the conclusions of this article will be made available by the authors, without undue reservation.
Ethics statement
The animal study was approved by Ethics Committee of the Faculty of Veterinary Medicine, Ghent University. The study was conducted in accordance with the local legislation and institutional requirements.
Author contributions
EB: Conceptualization, Investigation, Methodology, Project administration, Validation, Visualization, Writing – original draft, Writing – review & editing. MS: Data curation, Formal Analysis, Validation, Visualization, Writing – review & editing. KB: Investigation, Writing – review & editing. SP: Investigation, Writing – review & editing. EP: Investigation, Writing – review & editing. MD: Investigation, Writing – review & editing. GS: Methodology, Resources, Software, Writing – review & editing. GL: Methodology, Resources, Writing – review & editing. KS: Funding acquisition, Resources, Supervision, Writing – review & editing. JG: Conceptualization, Funding acquisition, Investigation, Methodology, Project administration, Resources, Supervision, Validation, Writing – review & editing.
Funding
The author(s) declare that no financial support was received for the research and/or publication of this article.
Acknowledgments
The authors would like to thank the staff at the Clinic of Large Animal Reproduction, Faculty of Veterinary Medicine, Merelbeke, for their support during the experiment and their care of the mares.
Conflict of interest
The authors declare that the research was conducted in the absence of any commercial or financial relationships that could be construed as a potential conflict of interest.
Generative AI statement
The authors declare that no Gen AI was used in the creation of this manuscript.
Publisher’s note
All claims expressed in this article are solely those of the authors and do not necessarily represent those of their affiliated organizations, or those of the publisher, the editors and the reviewers. Any product that may be evaluated in this article, or claim that may be made by its manufacturer, is not guaranteed or endorsed by the publisher.
Supplementary material
The Supplementary material for this article can be found online at: https://www.frontiersin.org/articles/10.3389/fvets.2025.1574351/full#supplementary-material
References
1. Lazzari, G, Colleoni, S, Crotti, G, Turini, P, Fiorini, G, Barandalla, M, et al. Laboratory production of equine embryos. J Equine Vet Sci. (2020) 89:103097. doi: 10.1016/j.jevs.2020.103097
2. Claes, A, and Stout, TAE. Success rate in a clinical equine in vitro embryo production program. Theriogenology. (2022) 187:215–8. doi: 10.1016/j.theriogenology.2022.04.019
3. Galli, C, Colleoni, S, Duchi, R, Lagutina, I, and Lazzari, G. Equine assisted reproduction and embryo technologies. Anim Reprod. (2013) 10:334–43.
4. Galli, C, Colleoni, S, Duchi, R, Lagutina, I, and Lazzari, G. Developmental competence of equine oocytes and embryos obtained by in vitro procedures ranging from in vitro maturation and ICSI to embryo culture, cryopreservation and somatic cell nuclear transfer. Anim Reprod Sci. (2007) 98:39–55. doi: 10.1016/j.anireprosci.2006.10.011
5. Martin-Pelaez, S, Stout, TAE, Leemans, B, Dini, P, and Claes, AN. Ovum pick up in a clinical program can induce mild transient discomfort in mares. AAEP Proceedings. (2022) 68:280–1.
6. Morris, LHA. The development of in vitro embryo production in the horse. Equine Vet J. (2018) 50:712–20. doi: 10.1111/evj.12839
7. Viana, JHM. Statistics of embryo production and transfer in domestic farm animals. Embryo Technology Newsletter. (2022) 40:22–40.
8. Vanderwall, DK, Hyde, KJ, and Woods, GL. Effect of repeated transvaginal ultrasound-guided follicle aspiration on fertility in mares. J Am Vet Med Assoc. (2006) 228:248–50. doi: 10.2460/javma.228.2.248
9. Stout, TAE. Clinical application of in vitro embryo production in the horse. J Equine Vet Sci. (2020) 89:103011. doi: 10.1016/j.jevs.2020.103011
10. Seyhan, A, Ata, B, Son, WY, Dahan, MH, and Tan, SL. Comparison of complication rates and pain scores after transvaginal ultrasound-guided oocyte pickup procedures for in vitro maturation and in vitro fertilization cycles. Fertil Steril. (2014) 101:705–9. doi: 10.1016/j.fertnstert.2013.12.011
11. Gilboa, D, Seidman, L, Kimiagarov, P, Noni, A, Doron, R, and Seidman, DS. Why do women choose to undergo oocyte aspiration without sedation or analgesia? Reprodu Fertil. (2021) 2:89–94. doi: 10.1530/RAF-20-0064
12. D’Angelo, A, Panayotidis, C, Amso, N, Marci, R, Matorras, R, Onofriescu, M, et al. Recommendations for good practice in ultrasound: oocyte pick up†. Hum Reprod Open. (2019) 2019:hoz025. doi: 10.1093/hropen/hoz025
13. Awonuga, A, Waterstone, J, Oyesanya, O, Curson, R, Nargund, G, and Parsons, J. A prospective randomized study comparing needles of different diameters for transvaginal ultrasound-directed follicle aspiration. Fertil Steril. (1996) 65:109–13. doi: 10.1016/S0015-0282(16)58036-4
14. Velez, IC, Arnold, C, Jacobson, CC, Norris, JD, Choi, YH, Edwards, JF, et al. Effects of repeated transvaginal aspiration of immature follicles on mare health and ovarian status. Equine Vet J. (2012) 44:78–83. doi: 10.1111/j.2042-3306.2012.00606.x
15. Bøgh, IB, Brink, P, Jensen, HE, Lehn-Jensen, H, and Greve, T. Ovarian function and morphology in the mare after multiple follicular punctures. Equine Vet J. (2003) 35:575–9. doi: 10.2746/042516403775467243
16. Fernández-Hernández, P, Valero-González, M, Fuentes-Romero, B, Iglesias-García, M, Ezquerra-Calvo, LJ, Martín-Cuervo, M, et al. Resolution of two cases of ovarian abscesses in mares subjected to ovum pick up. Equine Vet J. (2024) 56:751–8. doi: 10.1111/evj.14031
17. Campbell, MLH, and Sandøe, P. Welfare in horse breeding. Vet Rec. (2015) 176:436–40. doi: 10.1136/vr.102814
18. Petyim, S, Båge, R, Madej, A, and Larsson, B. Ovum pick-up in dairy heifers: does it affect animal well-being? Reprod Domest Anim. (2007) 42:623–32. doi: 10.1111/j.1439-0531.2006.00833.x
19. Chastant-Maillard, S, Quinton, H, Lauffenburger, J, Cordonnier-Lefort, N, Richard, C, Marchal, J, et al. Consequences of transvaginal follicular puncture on well-being in cows. Reproduction. (2003) 125:555–63. doi: 10.1530/rep.0.1250555
20. Alam, M, and Dobson, H. Effect of various veterinary procedures on plasma concentrations of cortisol, luteinising hormone and prostaglandin F2 alpha metabolite in the cow. Vet Rec. (1986) 118:7–10. doi: 10.1136/vr.118.1.7
21. Oltedal, A, Gaustad, AH, Peltoniemi, O, Björkman, S, Skaare, A, and Oropeza-Moe, M. Experiences with transvaginal ovum pick-up (OPU) in sows. Theriogenology. (2024) 214:157–65. doi: 10.1016/j.theriogenology.2023.09.021
22. Schönbom, H, Kassens, A, Hopster-Iversen, C, Klewitz, J, Piechotta, M, Martinsson, G, et al. Influence of transrectal and transabdominal ultrasound examination on salivary cortisol, heart rate, and heart rate variability in mares. Theriogenology. (2015) 83:749–56. doi: 10.1016/j.theriogenology.2014.11.010
23. Diego, R, Douet, C, Reigner, F, Blard, T, Cognié, J, Deleuze, S, et al. Influence of transvaginal ultrasound-guided follicular punctures in the mare on heart rate, respiratory rate, facial expression changes, and salivary cortisol as pain scoring. Theriogenology. (2016) 86:1757–63. doi: 10.1016/j.theriogenology.2016.05.040
24. Hernández-Avalos, I, Mota-Rojas, D, Mendoza-Flores, JE, Casas-Alvarado, A, Flores-Padilla, K, Miranda-Cortes, AE, et al. Nociceptive pain and anxiety in equines: physiological and behavioral alterations. Vet World. (2021) 14:2984–95. doi: 10.14202/vetworld.2021.2984-2995
25. Rietmann, TR, Stuart, AEA, Bernasconi, P, Stauffacher, M, Auer, JA, and Weishaupt, MA. Assessment of mental stress in warmblood horses: heart rate variability in comparison to heart rate and selected behavioural parameters. Appl Anim Behav Sci. (2004) 88:121–36. doi: 10.1016/j.applanim.2004.02.016
26. Ishizaka, S, Aurich, JE, Ille, N, Aurich, C, and Nagel, C. Acute physiological stress response of horses to different potential short-term stressors. J Equine Vet Sci. (2017) 54:81–6. doi: 10.1016/j.jevs.2017.02.013
27. König, V, Borstel, U, Visser, EK, and Hall, C. Indicators of stress in equitation. Appl Anim Behav Sci. (2017) 190:43–56. doi: 10.1016/j.applanim.2017.02.018
29. Malancus, RN, Rusu, RO, and Ailincăi, LI. Stress levels in Hanoverian horses used in competition and other recreational activities. Rev Rom Med Vet. (2022) 32:56–8.
30. van Loon, JPAM, and Van Dierendonck, MC. Monitoring acute equine visceral pain with the equine Utrecht University scale for composite pain assessment (EQUUS-COMPASS) and the equine Utrecht University scale for facial assessment of pain (EQUUS-FAP): a scale-construction study. Vet J. (2015) 206:356–64. doi: 10.1016/j.tvjl.2015.08.023
31. Covalesky, ME, Russoniello, CR, and Malinowski, K. Effects of show-jumping performance stress on plasma cortisol and lactate concentrations and heart rate and behavior in horses. J Equine Vet Sci. (1992) 12:244–51. doi: 10.1016/S0737-0806(06)81454-1
32. Massányi, M, Halo, M, Mlyneková, E, Kováčiková, E, Tokárová, K, Greń, A, et al. The effect of training load stress on salivary cortisol concentrations, health parameters and hematological parameters in horses. Heliyon. (2023) 9:e19037. doi: 10.1016/j.heliyon.2023.e19037
33. Bruschetta, G, Zanghì, G, Giunta, RP, Ferlazzo, AM, Satué, K, D’Ascola, A, et al. Short road transport and slaughter stress affects the expression profile of serotonin receptors, adrenocortical, and Hematochemical responses in horses. Vet Sci. (2024) 11:113. doi: 10.3390/vetsci11030113
34. Aurich, C, and Aurich, J. Effects of stress on reproductive functions in the horse. Pferdeheilkunde. (2008) 24:99–102. doi: 10.21836/PEM20080121
35. Sikorska, U, Maśko, M, Ciesielska, A, Zdrojkowski, Ł, and Domino, M. Role of cortisol in Horse’s welfare and health. Agriculture. (2023) 13:2219. doi: 10.3390/agriculture13122219
36. Broux, B, De Clercq, D, Decloedt, A, Ven, S, Vera, L, van Steenkiste, G, et al. Heart rate variability parameters in horses distinguish atrial fibrillation from sinus rhythm before and after successful electrical cardioversion. Equine Vet J. (2017) 49:723–8. doi: 10.1111/evj.12684
37. Van Steenkiste, G, van Loon, G, and Crevecoeur, G. Transfer learning in ECG classification from human to horse using a novel parallel neural network architecture. Sci Rep. (2020) 10:186. doi: 10.1038/s41598-019-57025-2
38. R Core Team. R: A language and environment for statistical computing. R Foundation for Statistical Computing, Vienna, Austria. (2020). Available at: https://www.R-project.org/ (Accessed April 3, 2025).
39. Wickham, H, Averick, M, Bryan, J, Chang, W, McGowan, LD, François, R, et al. Welcome to the tidyverse. J Open Source Softw. (2019) 4:1686. doi: 10.21105/joss.01686
40. Waring, E, Quinn, M, McNamara, A, Arino de la Rubia, E, Zhu, H, and Ellis, S. skimr: compact and flexible summaries of data. (2022). Available online at: https://CRAN.R-project.org/package=skimr (Accessed April 3, 2025).
41. Bates, D, Mächler, M, Bolker, B, and Walker, S. Fitting linear mixed-effects models using lme4. J Stat Softw. (2015) 67:1–48. doi: 10.18637/jss.v067.i01
42. Kuznetsova, A, Brockhoff, PB, and Christensen, RHB. lmerTest package: tests in linear mixed effects models. J Stat Softw. (2017) 82:1–26. doi: 10.18637/jss.v082.i13
43. Lüdecke, D, Ben-Shachar, MS, Patil, I, Waggoner, P, and Makowski, D. Performance: an R package for assessment, comparison and testing of statistical models. J Open Source Softw. (2021) 6:3139. doi: 10.21105/joss.03139
44. Lüdecke, D. sjPlot: data visualization for statistics in social science. (2024). Available online at: https://CRAN.R-project.org/package=sjPlot (Accessed April 3, 2025).
45. Pedersen, TL. Ggforce: accelerating “ggplot2.” (2024). Available online at: https://CRAN.R-project.org/package=ggforce (Accessed April 3, 2025).
46. Lenth, R V. Emmeans: estimated marginal means, aka least-squares means. (2024). Available online at: https://CRAN.R-project.org/package=emmeans (Accessed April 3, 2025).
47. Kassambara, A. Ggpubr: “ggplot2” based publication ready plots. (2023). Available online at: https://CRAN.R-project.org/package=ggpubr (Accessed April 3, 2025).
48. Green, P, and MacLeod, CJ. Simr: an R package for power analysis of generalised linear mixed models by simulation. Methods Ecol Evol. (2016) 7:493–8. doi: 10.1111/2041-210X.12504
49. Haffner, JC, Fecteau, KA, Eiler, H, Tserendorj, T, Hoffman, RM, and Oliver, JW. Blood steroid concentrations in domestic Mongolian horses. J Vet Diagn Invest. (2010) 22:537–43. doi: 10.1177/104063871002200407
50. Kirchmeier, A, van Herwaarden, AE, van der Kolk, JH, Sauer, FJ, and Gerber, V. Plasma steroid profiles before and after ACTH stimulation test in healthy horses. Domest Anim Endocrinol. (2020) 72:106419–5. doi: 10.1016/j.domaniend.2019.106419
51. Marc, M, Parvizi, N, Ellendorff, F, Kallweit, E, and Elsaesser, F. Plasma cortisol and ACTH concentrations in the warmblood horse in response to a standardized treadmill exercise test as physiological markers for evaluation of training status. J Anim Sci. (2000) 78:1936–46. doi: 10.2527/2000.7871936x
52. Tennent-Brown, B. Blood lactate measurement and interpretation in critically ill equine adults and neonates. Vet Clin North Am Equi Pract. (2014) 30:399–413. doi: 10.1016/j.cveq.2014.04.006
53. Kaneko, JJ, Harvey, JW, and Bruss, ML. Clinical biochemistry of domestic animals. 6th ed. Amsterdam: Elsevier (2008).
54. Papas, M, Govaere, J, Peere, S, Gerits, I, Van de Velde, M, Angel-Velez, D, et al. Anti-müllerian hormone and opu-icsi outcome in the mare. Animals. (2021) 11:2004. doi: 10.3390/ani11072004
55. Le Breton, A, and Lewis, N. Equine ART and antral follicle count: can we deepen our understanding to improve outcomes? Reprod Domest Anim. (2024) 59:e14625. doi: 10.1111/rda.14625
56. Jacobson, CC, Choi, YH, Hayden, SS, and Hinrichs, K. Recovery of mare oocytes on a fixed biweekly schedule, and resulting blastocyst formation after intracytoplasmic sperm injection. Theriogenology. (2010) 73:1116–26. doi: 10.1016/j.theriogenology.2010.01.013
57. Galli, C, Duchi, R, Colleoni, S, Lagutina, I, and Lazzari, G. Ovum pick up, intracytoplasmic sperm injection and somatic cell nuclear transfer in cattle, buffalo and horses: from the research laboratory to clinical practice. Theriogenology. (2014) 81:138–51. doi: 10.1016/j.theriogenology.2013.09.008
58. Schmidt, A, Biau, S, Möstl, E, Becker-Birck, M, Morillon, B, Aurich, J, et al. Changes in cortisol release and heart rate variability in sport horses during long-distance road transport. Domest Anim Endocrinol. (2010) 38:179–89. doi: 10.1016/j.domaniend.2009.10.002
59. Becker-Birck, M, Schmidt, A, Lasarzik, J, Aurich, J, Möstl, E, and Aurich, C. Cortisol release and heart rate variability in sport horses participating in equestrian competitions. J Vet Behav. (2013) 8:87–94. doi: 10.1016/j.jveb.2012.05.002
60. Morton, AJ, Varney, CR, Ekiri, AB, and Grosche, A. Cardiovascular effects of N-butylscopolammonium bromide and xylazine in horses. Equine Vet J. (2011) 43:117–22. doi: 10.1111/j.2042-3306.2011.00400.x
61. Hallman, I, Tapio, H, Raekallio, M, and Karikoski, N. Effect of constant rate infusion of detomidine with and without vatinoxan on blood glucose and insulin concentrations in horses. Vet Anaesth Analg. (2024) 51:144–51. doi: 10.1016/j.vaa.2023.11.005
62. Buhl, R, Ersbøll, AK, Larsen, NH, Eriksen, L, and Koch, J. The effects of detomidine, romifidine or acepromazine on echocardiographic measurements and cardiac function in normal horses. Vet Anaesth Analg. (2007) 34:1–8. doi: 10.1111/j.1467-2995.2005.00269.x
63. Sellon, DC, Roberts, MC, Blikslager, AT, Ulibarri, C, and Papich, MG. Effects of continuous rate intravenous infusion of butorphanol on physiologic and outcome variables in horses after celiotomy. J Vet Intern Med. (2004) 18:555–63. doi: 10.1111/j.1939-1676.2004.tb02585.x
64. Röder, M, Heuwieser, W, Borchardt, S, Plenio, JL, Palme, R, and Sutter, F. The effect of transdermal flunixin meglumine on blood cortisol levels in dairy calves after cautery disbudding with local anesthesia. J Dairy Sci. (2022) 105:3468–76. doi: 10.3168/jds.2021-21257
65. Wilson, D, Bohart, G, Evans, A, Robertson, S, and Rondenay, Y. Retrospective analysis of detomidine infusion for standing chemical restraint in 51 horses. Vet Anaesth Analg. (2002) 29:54–7. doi: 10.1046/j.1467-2987.2001.00047.x
66. de Oliveira, AR, Gozalo-Marcilla, M, Ringer, SK, Schauvliege, S, Fonseca, MW, Trindade, PHE, et al. Development and validation of the facial scale (FaceSed) to evaluate sedation in horses. PLoS One. (2021) 16:e0251909. doi: 10.1371/journal.pone.0251909
67. Robinson, EP, and Natalini, CC. Epidural anesthesia and analgesia in horses. Vet Clin N Am Equine Pract. (2002) 18:61–82. doi: 10.1016/S0749-0739(02)00010-X
68. Michielsen, AJHC, and Schauvliege, S. Epidurale anesthesie en analgesie bij paarden. Vlaams Diergeneeskd Tijdschr. (2019) 88:233–40. doi: 10.21825/vdt.v88i4.16013
Keywords: equine, assisted reproduction, ovum pick-up, animal welfare, stress
Citation: Van den Branden E, Salamone M, Broothaers K, Peere S, Polfliet E, Dewulf M, Van Steenkiste G, van Loon G, Smits K and Govaere J (2025) Physiological and behavioral parameters of pain and stress in mares during and after transvaginal ultrasound-guided follicular aspiration. Front. Vet. Sci. 12:1574351. doi: 10.3389/fvets.2025.1574351
Edited by:
Stefan Gregore Ciornei, Iasi, University of Life Science (IULS), RomaniaReviewed by:
Gian Guido Donato, University of Turin, ItalyRazvan Nicolae Malancus, Ion Ionescu de la Brad University of Agricultural Sciences and Veterinary Medicine of Iași, Romania
Copyright © 2025 Van den Branden, Salamone, Broothaers, Peere, Polfliet, Dewulf, Van Steenkiste, van Loon, Smits and Govaere. This is an open-access article distributed under the terms of the Creative Commons Attribution License (CC BY). The use, distribution or reproduction in other forums is permitted, provided the original author(s) and the copyright owner(s) are credited and that the original publication in this journal is cited, in accordance with accepted academic practice. No use, distribution or reproduction is permitted which does not comply with these terms.
*Correspondence: Emma Van den Branden, ZW1tYS52YW5kZW5icmFuZGVuQHVnZW50LmJl
†These authors have contributed equally to this work and share last authorship