- 1College of Animal Science, South China Agricultural University, Guangzhou, China
- 2College of Veterinary Medicine, South China Agricultural University, Guangzhou, China
- 3Wens Food Group Co., Ltd., Yunfu, China
Infectious Bursal Disease (IBD) is an acute, highly infectious, immunosuppressive disease caused by the infectious bursal disease virus (IBDV). To elucidate the prevalence of IBDV in southern China, a total of 60 tissues (including spleen and bursa) suspected of IBDV infection were collected from broiler chickens in 2023. In this study, a total of 31 IBDV strains were successfully isolated. The vp2 gene sequences of these isolates were sequenced and analysed. The results showed that 8 of the isolates were identified as very virulent strains, 11 as classical strains and 12 as novel variants. The nucleotide sequence identity among the isolates ranged from 90.7 to 100%, as determined by MegAlign. Further analysis revealed that the novel mutant strains exhibited characteristic amino acid sites are 252I, 254 N, 262Y, 299S and 318D. Phylogenetic analysis of the IBDV isolates and reference strains from South China demonstrated that the novel mutant strain has diverged from previously prevalent mutant strains, such as Variant E and GLS, forming a distinct lineage. This finding implies that the high mutation rate of IBDV may compromise vaccine efficacy and pose new challenges for the prevention and control of IBDV in poultry production.
1 Introduction
IBD is an acute and highly contagious immunosuppressive disease in chickens caused by the IBDV (1). This virus significantly impairs the host immune system, leading to reduced immune competence and increased susceptibility to other pathogens (2–5). IBDV is classified into two serotypes: Serotype I and Serotype II. Serotype I is pathogenic to chickens and is the primary cause of infections in poultry flocks (6). Notably, there are antigenic differences among the various subtypes of Serotype I, which can lead to variations in the efficacy of vaccine-induced immune protection (7–9). In the past 30 years, under favorable feeding and management conditions and the widespread use of effective vaccines, the virulent strain has been well controlled in China. However, the widespread use of vaccines has triggered high immune selection pressure, promoting the development of genetic diversity in circulating viruses (10). In the past five years, a new mutant strain has emerged in China and has rapidly spread throughout the country, becoming the predominant circulating strain (11, 12). The clinical signs of the new variant strain are not always apparent, but it can cause bursal atrophy, leading to severe immunosuppression and a decline in production performance, posing a new threat to the healthy development of China’s poultry industry (13).
IBDV is a non-enveloped virus with a single capsid exhibiting icosahedral symmetry (14). It belongs to the double-stranded RNA virus family, specifically the genus Avibirnavirus (1). The IBDV genome consists of two double-stranded RNA fragments, A and B. Fragment A is approximately 3.2 kb in length and contains two open reading frames (ORFs). ORF1 encodes the non-structural protein VP5, while ORF2 encodes a polyprotein precursor. This precursor is cleaved by the viral serine protease VP4 into mature viral proteins, including the structural proteins VP2 and VP3, as well as the non-structural protein VP4. Fragment B is approximately 2.8 kb in length and contains a single ORF, which encodes the structural protein VP1 (15). This bipartite structure of IBDV fragments A and B significantly increases the possibility of genome recombination (16, 17). As a result, new variants of IBDV emerge continuously, presenting substantial challenges for the control and prevention of IBD (10, 18).
The VP2 accounts for 51% of the total virion protein and is the sole component of the IBDV outer shell. The VP2 forms three major domains by folding, namely, the basement domain formed by conserved amino acids, the coat domain, and the protrusion domain (19–21). The protrusion domain is formed by the hypervariable region (HVR) of VP2, covering the amino acid 206 to 350 positions, and it is widely believed that the differences between IBDV strains are mainly concentrated in the VP2 HVR (22, 23). In addition, there are 2 hydrophilic regions (aa 210 ~ 225 in the first hydrophilic region and aa 314 ~ 324 in the second hydrophilic region) and 1 heptad region (aa 326 ~ 332) (24). The first hydrophilic region that stabilizes the IBDV conformation, the structure formed by the second hydrophilic region can specifically bind to the neutralizing antibodies, and mutations in the site in the second hydrophilic region may induce antigenic and virulence variation. The heptapeptide region is correlated with the virulence of the virion (25, 26). The two small hydrophilic regions (aa 247 ~ 254 and aa 281 ~ 292) in the VP2 HVR were associated with the virulence and surface antigen of the IBDV strain (17).
The tip of the VP2 HVR is located in the most lateral part of the virion and contains four Loop ring structures, all of which play important roles. PBC (aa 204 ~ 236) covers the first hydrophilic region, and the amino acid at position 222 is related to the replication efficiency of IBDV. The amino acid mutation in this position can change the antigenicity of the strain and lead to immune escape (27). PHI covers the second hydrophilic region with cellular receptor binding sites. Both ring structures contain neutralizing antigen epitopes and, because of their high degree of serum specificity, can be used to distinguish classical, variant, and serotype II strains (28). PDE (aa 240 ~ 265) and PFG (aa 270 ~ 293) were associated with antigenic drift, cytotropism, and virulence of the strain (29). It have found that the two-site mutation Q253H / A284T can adapt the vvIBDV strain not adapted to in vitro cell culture and significantly reduce its lethality, and the mortality rate can be reduced from 60% to 0 (30). Therefore, mutations in this regional locus may alter tissue tropism as well as affect virulence, providing a reference for vaccine development.
Molecular epidemiological studies of IBDV have primarily focused on two aspects: molecular characteristics and genetic evolution. Molecular characteristics can serve as a reference for pathogen typing, while genetic evolution studies are of great significance for understanding the evolutionary trends of strains. Currently, research on molecular characteristics primarily focuses on the hypervariable region of vp2 gene. It’s the protective antigen gene of IBDV and is relatively conserved among very-virulent, classical, and variant strains. Amino acid mutations within the hypervariable region are associated with antigenic differences between strains, with unique amino acid sites in this region playing a critical role (31, 32). These epitopes play a critical role in the host’s antiviral immune response (33, 34). Therefore, genetic and evolutionary analyses of the vp2 gene can provide valuable insights into the epidemiological trends of IBD. This information is essential for guiding the development of effective IBD vaccines in clinical settings.
Therefore, in this study, an epidemiological investigation was conducted in South China in 2023 to isolate and analyze the genetic evolution of IBDV strains. This investigation aimed to elucidate the molecular epidemiological characteristics of IBDV and comprehensively evaluate the epidemic features of circulating strains. The findings provide valuable insights for the effective prevention and control of infectious bursal disease, as well as for the research and development of vaccines.
2 Materials and methods
2.1 Ethics statement
This study was approved by the Animal Care Committee of South China Agricultural University (approval ID: SYXK-2019-0136). All study procedures and animal care activities were conducted per the recommendations in the Guide for the Care and Use of Laboratory Animals of the Ministry of Science and Technology of the People’s Republic of China.
2.2 Animals
For the experimental procedures, 10- to 12-day-old SPF (Specific Pathogen-Free) embryoated chicken eggs were procured from the SPF Experimental Animal Center of Guangdong Xinxing Dahuanong Poultry Egg Co., Ltd.
2.3 Clinical samples
During 2023, outbreaks characterized by atrophy of the bursa of Fabricius occurred in commercial poultry farms in Guangxi, Guangdong, and Fujian, China. A total of 60 clinical samples (including spleens and bursae) were collected from diseased chickens across six commercial farms (includeing two farms in Guangdong, two farms in Guangxi and two farms in Fujian). The date of age of sick chickens is 31 to 63 days. All of the chickens were vaccinated with a live-attenuated W2512 vaccine. All samples, labeled with collection dates and regional locations, were transported to the laboratory on frozen carbon dioxide for processing and analysis.
2.4 Virus identification and isolation
The virus isolation procedure was refer to a previously published method (35). Briefly, sixty diseased tissues (including spleen and bursa) were minced and homogenized in a solution containing 5,000 U/mL of penicillin and streptomycin. The homogenate was subjected to three cycles of freeze-thawing at −80°C to disrupt the tissue and release the virus. Subsequently, the mixture was centrifuged at 10,000 rpm for 5 min at 4°C to separate the supernatant, which was then filtered through a 0.22 μm filter to remove any remaining cellular debris. The filtered supernatant was inoculated onto the chorioallantoic membrane of 10- to 12-day-old SPF chicken embryos. Two PBS samples were used as negative controls. Each inoculation consisted of 0.2 mL, sealed with paraffin, and incubated in a 37°C incubator. The embryoated chicken eggs that died within 24 h were discarded. Starting from the first day post-inoculation, dead chicken embryos were collected daily and stored at 4°C. After 5 days of inoculation, the allantoic membranes and a portion of the allantoic fluid were collected from all chicken embryos. The collected materials were mixed with a double antibiotic solution at a ratio of 1:3, homogenized by grinding, and then frozen. The homogenate was subsequently used to inoculate SPF chicken embryos. This process was repeated for three generations (F3), and the final generation’s chorioallantoic membranes and allantoic fluid were homogenized to prepare the viral stock. The embryoated chicken eggs were harvested, and both the chorioallantoic membrane and a portion of the allantoic fluid were collected for RNA extraction and virus detection.
2.5 Sequencing and analysis
RNA extraction was performed according to the instructions of the Magen viral DNA/RNA extraction kit. The extracted RNA was tested using the already published universal detection primers for IBDV (IBDV-F: 5’-GCCGATGATTACCAATTCTCATC-3′, IBDV-R: 5’-CCGGATTATGTCTTTGAAGC-3′) (35). And synthesized by Shanghai Biotech Co., Ltd. The 31 isolates were subjected to reverse-transcription polymerase chain reaction (Reverse Transcription-Polymerase Chain Reaction, RT–PCR) with a one-step RT–PCR kit, and the PCR reaction procedure was: 50°C 30 min; 94°C 4 min; 94°C 30s, 55°C 45 s, 72°C 40s, 32 cycles; 72°C 10 min; 4°C terminated. The 7 μL PCR amplification products were detected using 1% agarose gel electrophoresis, and then the remaining PCR products were sent to Guangzhou BGI Technology Corporation for Sanger sequencing.
The measured sequences of the hypervariable region of the vp2 gene were assembled using SeqMan in DNASTAR. The sequences of the vp2 gene were analyzed using the ClustalW method in MEGA 7.0, and then the neighbor-joining method was employed to conduct phylogenetic analysis of the vp2 gene and construct the evolutionary tree. Subsequently, the nucleotide sequence identity of the vp2 gene was analyzed using MegAlign (Table 1).
3 Results
3.1 Clinical features of the diseased chickens
In 2023, our laboratory received a total of 60 suspected IBDV-infected samples from three provinces in China. The primary clinical symptoms observed in the affected chickens included depression, drooping heads, and sparse feathers. Upon necropsy, the findings included leg hemorrhages, bursal enlargement, and splenic enlargement (Figures 1A,B). The diseased material was tested using the VP2 universal primer with a band size of 714 bp, meeting the expected band size (Figure 1C). A total of 31 positive samples were detected, resulting in a positivity rate of 51.67%.
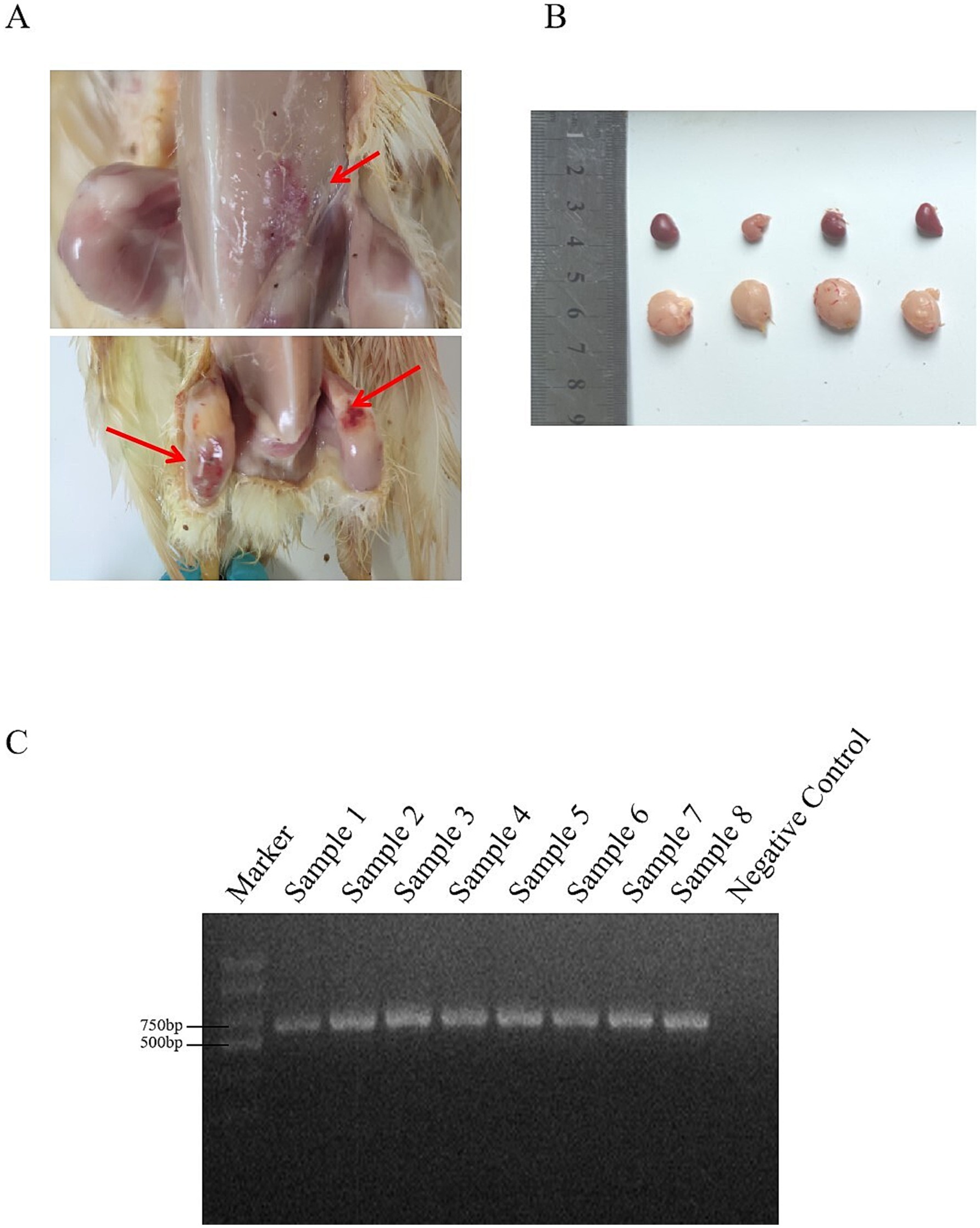
Figure 1. (A) The lesions were haemorrhage of leg muscle and pectoral muscle of IBDV-infection chickens. (B) The lesions of the bursa of Fabricius and spleen. (C) PCR amplification of IBDV.
3.2 Phylogenetic tree analysis of vp2 gene of IBDV isolates
The nucleotide sequences of the vp2 gene of IBDV isolates have been deposited in the GenBank database (Table 2). Phylogenetic analysis at the nucleotide level of vp2 gene of the IBDV isolates, together with reference sequences was conducted. The results showed that the isolates belong to Serotype I and can be classified into three genotypes: very virulent, classical, and novel variants. 12 isolates, including FJZZ10, were identified as novel variants, accounting for 38.7% of the total isolates. Phylogenetic analysis revealed that these novel variants have diverged from the earlier circulating variants, such as Variant E and GLS, and have independently formed a distinct new clade. 11 isolates were identified as classical strains, representing 35.4% of the total isolates. These isolates exhibited a high degree of genetic identity to the reference strain 2512. 8 isolates, including FJZZ05, were identified as very virulent strains, constituting 25.8% of the total isolates. Based on these results, the predominant circulating strains in the sampled regions are the classical strains (Figure 2).
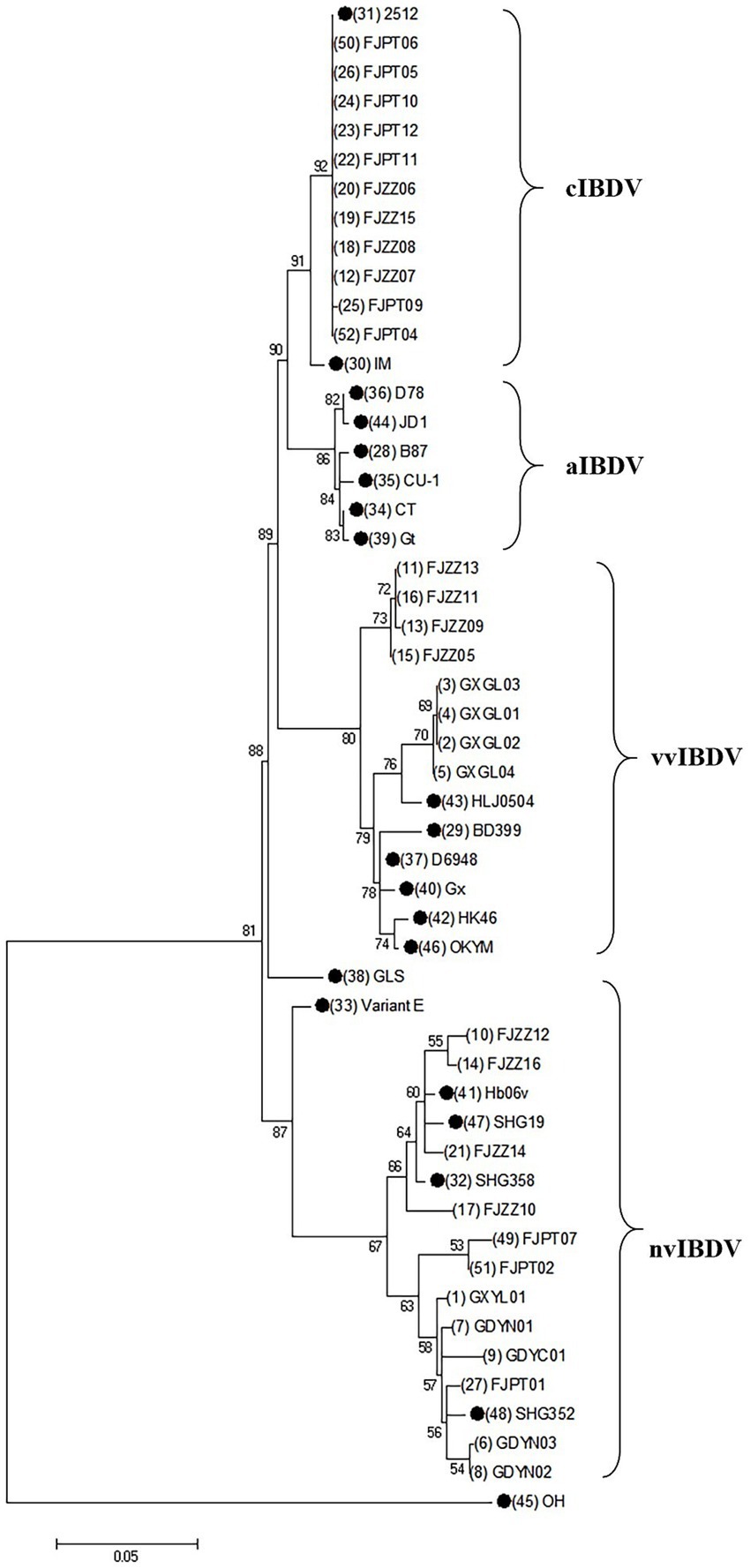
Figure 2. Phylogenic analysis based on the nucleotide sequences of vp2 gene. A phylogenic tree was constructed using the neighbor-joining method from phylogenetic distances calculated using MEGA 7.0. Bootstrap values obtained from 1,000 replicates are shown at the major nodes. The reference strains in this study are indicated by solid dots.
3.3 Identity analysis of the vp2 gene in IBDV isolates
Among the 31 IBDV isolates obtained in this study, the nucleotide sequence fragment length was 714 base pairs (bp) (spanning positions 654 bp to 1,368 bp), and encode approximately 238 amino acids. The nucleotide sequences of the hypervariable region of the vp2 gene were aligned with each other and to the reference sequence. The results revealed that the nucleotide sequence identity of the vp2 gene ranged from 90.7 to 100% among the 31 isolates. The identity between the isolated classical strains and the reference sequence 2512 was 99.8 to 100%. The identity between the isolated novel variant strains and the reference strains SHG352 and SHG19 was 94.5 to 99.7%, and the identity between the very virulent strains and the reference sequence HLJ0504 was 95.6 to 100% (Supplementary Figure S1). The alignment of the amino acid sequence in the hypervariable region of VP2 shows that 11 classical strains showed 100% identity with reference strain 2512, 12 novel variants strains showed 98.1 to 100% identity with reference strains SHG352 and SHG19, and 8 very virulent strains showed 98.1 to 100% identity with reference strain HLJ0504 (Supplementary Figure S2).
3.4 Molecular characteristic of the vp2 gene of IBDV isolates
The characteristic amino acid alignment based on the vp2 hypervariable region showed that the 12 novel variants had different amino acid residues, including 252I, 254 N, 262Y and 299S. And, amino acid site mutations such as 270A and 317S. Furthermore, alignment analysis of the amino acid sequence with virulent strain HLJ0504 revealed a difference of 12 amino acid sites between the isolates and the reference sequence, which may be associated to strain variation. Notably, the 12 isolates had the characteristic amino acid sites of the virulent strains: 299S. And compared with the current novel variant SHG352, isolate FJZZ14 mutation at site 242 (V to I), FJZZ10 mutated at 317 (S to N) and 269 (T to A). In conclusion, the amino acid sequence alignment of the vp2 hypervariable region showed that the characteristic amino acid sites are 252I, 254 N, 262Y, 299S and 318D, indicating that the 12 novel variants have new characteristics and may have the characteristics of virulent strains (Figure 3).
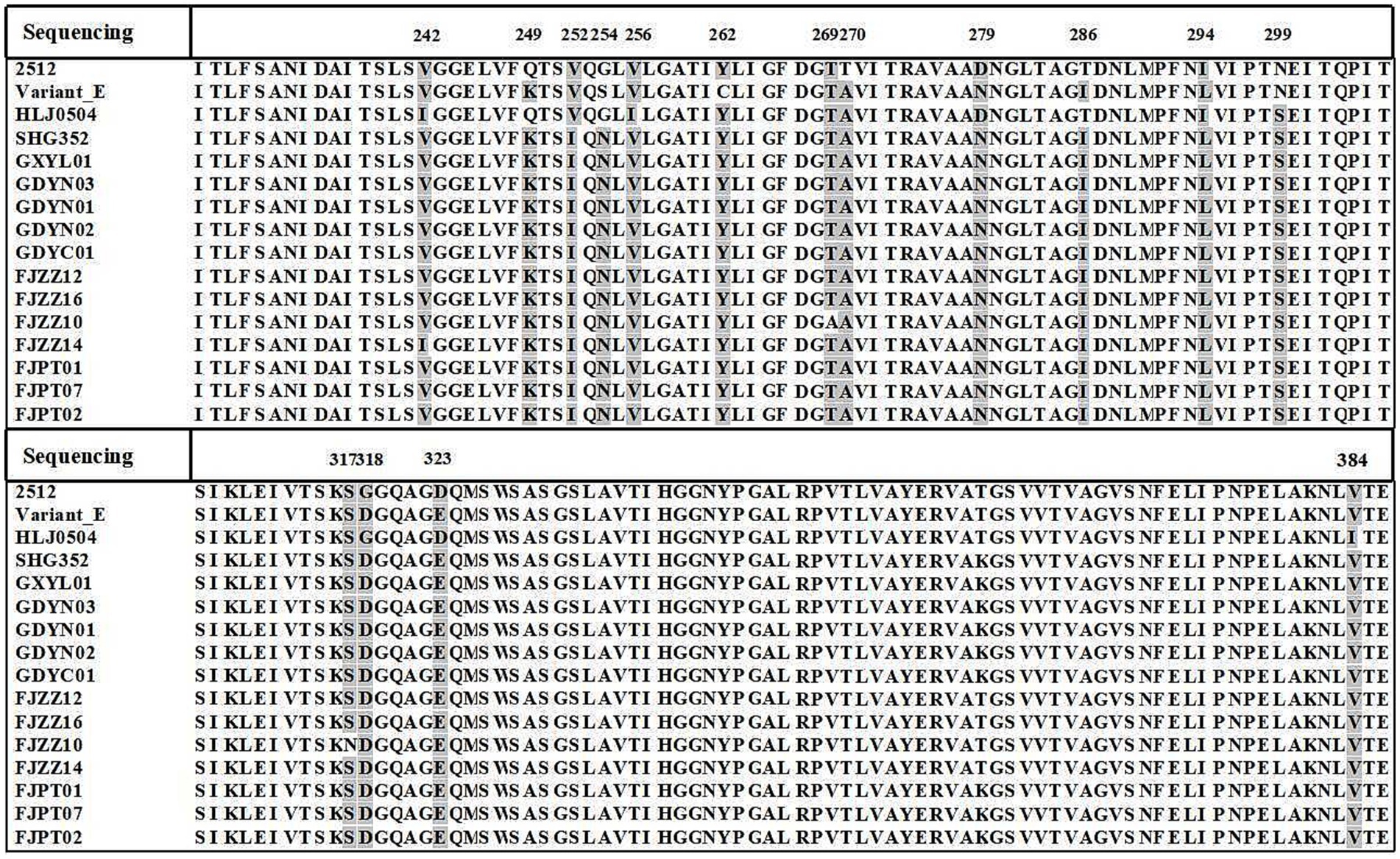
Figure 3. Amino acid sequence alignment of vp2 gene. Amino acid alignment of 12 novel variants with variant E, HLJ0504, 2512, and SHG352 using MegAlign. All variant residues within the sequences are highlighted against a background. The amino acid coordinates are provided at the top.
4 Discussion
Infectious bursal disease (IBD) is one of the diseases that cannot be ignored in poultry. Its prevalence in China can be traced back to 1979 (36). Among them, very virulent strains have attracted significant attention due to their high incidence, high mortality, and ability to cause severe immunosuppression (16, 37). These very virulent strains pose a serious threat to the healthy breeding of China’s poultry industry, causing substantial economic losses. However, with the modernization of China’s poultry farming practices and effective vaccination programs, outbreaks of very virulent strains have been effectively controlled.
The South China region of China is home to several major poultry-raising provinces. In the past few years, the predominant strains of IBDV circulating in South China were highly virulent or very virulent strains (38, 39). However, in recent years, novel variant strains have been successively detected in South China. These novel variant strains do not cause mortality in chicken flocks but can lead to severe immunosuppression (40, 41). Reports have indicated that the currently used commercial vaccines still fail to prevent the damage of these novel variant strains to the bursa of Fabricius (42). This suggests that commercial IBDV vaccines targeting very virulent strains cannot protect chicken flocks from the novel variant strains, resulting in vaccination failure.
To understand the epidemiological trends of IBD in South China, this study selected three regions: Guangdong, Guangxi, and Fujian for epidemiological detection. A total of 31 strains were isolated, including 12 novel variants, 8 very virulent strains, and 11 classical strains. This indicates that multiple types of strains coexist, and mixed infections of novel variant strains, very virulent strains, and classical strains are gradually becoming the main epidemiological trend in South China.
In this study, the clinical samples were collected from diseased chickens aged 31 to 63 days in six poultry farms across three provinces in southern China (including Guangdong, Guangxi and Fujian). All the chickens vaccinated with attenuated live vaccine at 10-days-old. Therefore, we detected the vaccine strain W2512 in the field. The novel variant strains were isolated in two flocks in Guangdong province, suggesting that the attenuated live vaccine provides partial protection against novel variants. Several reports also confirmed the partial protection of commercial vaccines against novel variants (43). Our data further highlight the urgent need to reevaluate vaccine formulations, particularly in regions where novel variants are endemic.
Notably, alignment analysis of the vp2 HVR in 12 novel variants revealed conserved residues 286I and 318D, consistent with previously reported variant-specific signatures. In particular, not only distinguishes these novel variants from historical lineages (e.g., early Variant E) but also emerges as a potential key site driving viral evolution. Phylogenetic reconstruction based on vp2 HVR sequences demonstrated that the 12 novel variants diverged significantly from earlier strains (e.g., Variant E and GLS) circulating in the United States, forming a distinct clade with multiple sublineages. Furthermore, among eight newly isolated strains (GXGL01–04 and FJZZ09–13), four clustered with the reference virulent strain HLJ0504, while the remaining four (FJZZ09, FJZZ11, and FJZZ13) constituted an independent subclade. This genetic diversification underscores the ongoing evolution of IBDV vp2 in southern China, posing challenges for regional disease control strategies. These findings highlight two critical implications: The coexistence of variant-specific (286I/318D) and virulence-associated (299S) residues in novel variants suggests a convergent evolutionary trajectory, potentially enhancing both immune evasion and pathogenicity. The emergence of multiple subclades within the novel variant group reflects accelerated genomic diversification, likely driven by selective pressures in immunized flocks. Such evolutionary trends necessitate vigilant surveillance of vp2 polymorphisms to update vaccine formulations and refine diagnostic tools. Future studies should explore the structural and functional consequences of 299S and other critical residues using reverse genetics, as well as assess cross-protective efficacy of current vaccines against these evolving lineages.
In conclusion, this study isolated and identified 31 IBDV isolates, including 8 very virulent strains, 11 classical strains, and 12 novel variants. Further analysis revealed that the novel variant strains exhibited characteristic amino acid sites are 252I, 254 N, 262Y, 299S and 318D, suggesting that these isolates possess traits of both mutant and virulent phenotypes. Phylogenetic analysis of IBDV isolates and reference strains from South China demonstrated that the novel variant strains were distinct from previously reported strains, such as variant E and GLS, and formed a unique evolutionary lineage. These findings indicate that the high mutation rate of IBDV may compromise vaccine efficacy and present new challenges for the prevention and control of IBDV in poultry production.
Data availability statement
The datasets presented in this study can be found in online repositories. The names of the repository/repositories and accession number(s) can be found in the article/Supplementary material.
Ethics statement
The animal study was approved by Animal Care Committee of South China Agricultural University (approval ID: SYXK-2019-0136). The study was conducted in accordance with the local legislation and institutional requirements.
Author contributions
KZ: Data curation, Validation, Writing – original draft, Writing – review & editing. QW: Investigation, Writing – original draft, Writing – review & editing. ML: Formal analysis, Investigation, Writing – original draft, Writing – review & editing. ZW: Data curation, Writing – review & editing. WL: Funding acquisition, Project administration, Resources, Supervision, Writing – review & editing.
Funding
The author(s) declare that financial support was received for the research and/or publication of this article. This work was supported by grants from the National Key Research and Development Program Projects (2022YFD1801300), the Yunfu City Science and Technology Plan Project (2024090301).
Conflict of interest
ZW was employed by Wens Food Group Co., Ltd.
The remaining authors declare that the research was conducted in the absence of any commercial or financial relationships that could be construed as a potential conflict of interest.
Generative AI statement
The author(s) declare that no Gen AI was used in the creation of this manuscript.
Publisher’s note
All claims expressed in this article are solely those of the authors and do not necessarily represent those of their affiliated organizations, or those of the publisher, the editors and the reviewers. Any product that may be evaluated in this article, or claim that may be made by its manufacturer, is not guaranteed or endorsed by the publisher.
Supplementary material
The Supplementary material for this article can be found online at: https://www.frontiersin.org/articles/10.3389/fvets.2025.1575407/full#supplementary-material
SUPPLEMENTARY FIGURE S1 | Alignment of nucleotide sequence identity in the hypervariable region of the vp 2 gene.
SUPPLEMENTARY FIGURE S2 | Alignment of amino acid sequence identity in the hypervariable region of the vp 2 gene.
References
1. Mahgoub, HA, Bailey, M, and Kaiser, P. An overview of infectious bursal disease. Arch Virol. (2012) 157:2047–57. doi: 10.1007/s00705-012-1377-9
2. Zhang, TWS, Liu, Y, Qi, X, and Gao, Y. Advances on adaptive immune responses affected by infectious bursal disease virus in chicken. Front Immunol. (2024) 14:1330576. doi: 10.3389/fimmu.2023.1330576
3. Faragher, JT, Allan, WH, and Cullen, GA. Immunosuppressive effect of the infectious bursal agent in the chicken. Nature New Biol. (1972) 237:118–9. doi: 10.1038/newbio237118a0
4. Sharma, J. Infectious bursal disease virus of chickens: pathogenesis and immunosuppression. Dev Compar Immunol. (2000) 24:223–35. doi: 10.1016/s0145-305x(99)00074-9
5. Mashaly, MM, Hendricks, GL, Kalama, MA, Gehad, AE, Abbas, AO, and Patterson, PH. Effect of heat stress on production parameters and immune responses of commercial laying hens. Poult Sci. (2004) 83:889–94. doi: 10.1093/ps/83.6.889
6. Jackwood, DJ, Sommer-Wagner, SE, Crossley, BM, Stoute, ST, Woolcock, PR, and Charlton, BR. Identification and pathogenicity of a natural reassortant between a very virulent serotype 1 infectious bursal disease virus (IBDV) and a serotype 2 IBDV. Virology. (2011) 420:98–105. doi: 10.1016/j.virol.2011.08.023
7. Gao, H, Zhang, S, Chang, H, Guo, Y, Li, Z, Wang, Y, et al. Generation of a novel attenuated IBDV vaccine strain by mutation of critical amino acids in IBDV VP5. Vaccine. (2024) 42:126081. doi: 10.1016/j.vaccine.2024.06.048
8. Wang, G, Jiang, N, Yu, H, Niu, X, Huang, M, Zhang, Y, et al. Loop PDE of viral capsid protein is involved in immune escape of the emerging novel variant infectious bursal disease virus. Vet Microbiol. (2024) 293:110094. doi: 10.1016/j.vetmic.2024.110094
9. Jackwood, DJ, and Sommer-Wagner, SE. Amino acids contributing to antigenic drift in the infectious bursal disease Birnavirus (IBDV). Virology. (2011) 409:33–7. doi: 10.1016/j.virol.2010.09.030
10. Gao, H, Wang, Y, Gao, L, and Zheng, SJ. Genetic insight into the interaction of IBDV with host—a clue to the development of novel IBDV vaccines. Int J Mol Sci. (2023) 24:8255. doi: 10.3390/ijms24098255
11. Fan, L, Wu, T, Hussain, A, Gao, Y, Zeng, X, Wang, Y, et al. Novel variant strains of infectious bursal disease virus isolated in China. Vet Microbiol. (2019) 230:212–20. doi: 10.1016/j.vetmic.2019.01.023
12. Xu, G, Li, J, Shen, Q, Hou, L, Xia, Y, Li, Q, et al. Complete genome characterization of a novel infectious bursal disease virus strain isolated from a chicken farm in China. Microbiol Res Announc. (2019) 8. doi: 10.1128/mra.00632-19
13. Payne, LN, and Nair, V. The long view: 40 years of avian leukosis research. Avian Pathol. (2012) 41:11–9. doi: 10.1080/03079457.2011.646237
14. Luque, D, Rivas, G, Alfonso, C, Carrascosa, JL, Rodríguez, JF, and Castón, JR. Infectious bursal disease virus is an icosahedral polyploid dsRNA virus. Proc Natl Acad Sci. (2009) 106:2148–52. doi: 10.1073/pnas.0808498106
15. Müller, H, Scholtissek, C, and Becht, H. The genome of infectious bursal disease virus consists of two segments of double-stranded RNA. J Virol. (1979) 31:584–9. doi: 10.1128/jvi.31.3.584-589.1979
16. Niu, Y, Sun, Q, Zhang, G, Sun, W, Liu, X, Xiao, Y, et al. Epidemiological investigation of outbreaks of fowl adenovirus infections in commercial chickens in China. Transbound Emerg Dis. (2018) 65:e121–6. doi: 10.1111/tbed.12691
17. Durairaj, V, Sellers, HS, Linnemann, EG, Icard, AH, and Mundt, E. Investigation of the antigenic evolution of field isolates using the reverse genetics system of infectious bursal disease virus (IBDV). Arch Virol. (2011) 156:1717–28. doi: 10.1007/s00705-011-1040-x
18. Azad, AA, Barrett, SA, and Fahey, KJ. The characterization and molecular cloning of the double-stranded RNA genome of an Australian strain of infectious bursal disease virus. Virology. (1985) 143:35–44. doi: 10.1016/0042-6822(85)90094-7
19. Birghan, C. A non-canonical Lon proteinase lacking the ATPase domain employs the Ser-Lys catalytic dyad to exercise broad control over the life cycle of a double-stranded RNA virus. EMBO J. (2000) 19:114–23. doi: 10.1093/emboj/19.1.114
20. Garriga, D, Querol-Audí, J, Abaitua, F, Saugar, I, Pous, J, Verdaguer, N, et al. The 2.6-angstrom structure of infectious bursal disease virus-derived T=1 particles reveals new stabilizing elements of the virus capsid. J Virol. (2006) 80:6895–905. doi: 10.1128/jvi.00368-06
21. Lee, C-C, Ko, T-P, Chou, C-C, Yoshimura, M, Doong, SR, Wang, MY, et al. Crystal structure of infectious bursal disease virus VP2 subviral particle at 2.6 Å resolution: implications in virion assembly and immunogenicity. J Struct Biol. (2006) 155:74–86. doi: 10.1016/j.jsb.2006.02.014
22. Alfonso-Morales, A, Martínez-Pérez, O, Dolz, R, Valle, R, Perera, CL, Bertran, K, et al. Spatiotemporal phylogenetic analysis and molecular characterisation of infectious bursal disease viruses based on the VP2 hyper-variable region. PLoS One. (2013) 8:e65999. doi: 10.1371/journal.pone.0065999
23. Drissi Touzani, C, Fellahi, S, Gaboun, F, Fassi Fihri, O, Baschieri, S, Mentag, R, et al. Molecular characterization and phylogenetic analysis of very virulent infectious bursal disease virus circulating in Morocco during 2016-2017. Arch Virol. (2019) 164:381–90. doi: 10.1007/s00705-018-4076-3
24. Azad, AA, Jagadish, MN, Brown, MA, and Hudson, PJ. Deletion mapping and expression in Escherichia coli of the large genomic segment of a birnavirus. Virology. (1987) 161:145–52. doi: 10.1016/0042-6822(87)90180-2
25. Mohamed, MA, Elzanaty, KES, Bakhit, BM, and Safwat, MM. Genetic characterization of infectious bursal disease viruses associated with Gumboro outbreaks in commercial broilers from Asyut Province. Egypt ISRN Vet Sci. (2014) 2014:1–9. doi: 10.1155/2014/916412
26. Raja, P, Senthilkumar, TMA, Parthiban, M, Thangavelu, A, Gowri, AM, Palanisammi, A, et al. Complete genome sequence analysis of a naturally Reassorted infectious bursal disease virus from India. Genome Announc. (2016) 4:e00709–16. doi: 10.1128/genomea.00709-16
27. Qi, X, Gao, X, Lu, Z, Zhang, L, Wang, Y, Gao, L, et al. A single mutation in the PBC loop of VP2 is involved in the in vitro replication of infectious bursal disease virus. Sci China Life Sci. (2016) 59:717–23. doi: 10.1007/s11427-016-5054-1
28. Heine, HG, Haritou, M, Failla, P, Fahey, K, and Azad, A. Sequence analysis and expression of the host-protective immunogen VP2 of a variant strain of infectious bursal disease virus which can circumvent vaccination with standard type I strains. J Gen Virol. (1991) 72:1835–43. doi: 10.1099/0022-1317-72-8-1835
29. Boot, HJ, Ter Huurne, AAHM, Hoekman, AJW, Peeters, BPH, and Gielkens, ALJ. Rescue of very virulent and mosaic infectious bursal disease virus from cloned cDNA: VP2 is not the sole determinant of the very virulent phenotype. J Virol. (2000) 74:6701–11. doi: 10.1128/jvi.74.15.6701-6711.2000
30. Qi, X, Gao, H, Gao, Y, Qin, L, Wang, Y, Gao, L, et al. Naturally occurring mutations at residues 253 and 284 in VP2 contribute to the cell tropism and virulence of very virulent infectious bursal disease virus. Antivir Res. (2009) 84:225–33. doi: 10.1016/j.antiviral.2009.09.006
31. Hoque, MM, Omar, AR, Chong, LK, Hair-Bejo, M, and Aini, I. Pathogenicity of SspI-positive infectious bursal disease virus and molecular characterization of the VP2 hypervariable region. Avian Pathol. (2001) 30:369–80. doi: 10.1080/03079450120066377
32. Parade, LH, Sapats, S, Gould, G, Rudd, M, Lowther, S, and Ignjatovic, J. Characterization of infectious bursal disease virus isolates from Indonesia indicates the existence ofvery virulent strains with unique genetic changes. Avian Pathol. (2003) 32:511–8. doi: 10.1080/0307945031000154116
33. Becht, H, Muller, H, and Muller, HK. Comparative studies on structural and antigenic properties of two serotypes of infectious bursal disease virus. J Gen Virol. (1988) 69:631–40. doi: 10.1099/0022-1317-69-3-631
34. Coulibaly, F, Chevalier, C, Gutsche, I, Pous, J, Navaza, J, Bressanelli, S, et al. The Birnavirus crystal structure reveals structural relationships among icosahedral viruses. Cell. (2005) 120:761–72. doi: 10.1016/j.cell.2005.01.009
35. Wang, Q, Hu, H, Chen, G, Liu, H, Wang, S, Xia, D, et al. Identification and assessment of pathogenicity of a naturally reassorted infectious bursal disease virus from Henan, China. Poult Sci. (2019) 98:6433–44. doi: 10.3382/ps/pez498
36. Ren, G, Wang, H, Yan, Y, Liu, F, Huang, M, and Chen, R. Pathogenicity of a fowl adenovirus serotype 4 isolated from chickens associated with hydropericardium-hepatitis syndrome in China. Poult Sci. (2019) 98:2765–71. doi: 10.3382/ps/pez042
37. Zhang, W, Wang, X, Gao, Y, and Qi, X. The Over-40-years-epidemic of infectious bursal disease virus in China. Viruses. (2022) 14:2253. doi: 10.3390/v14102253
38. He, X, Xiong, Z, Yang, L, Guan, D, Yang, X, and Wei, P. Molecular epidemiology studies on partial sequences of both genome segments reveal that reassortant infectious bursal disease viruses were dominantly prevalent in southern China during 2000-2012. Arch Virol. (2014) 159:3279–92. doi: 10.1007/s00705-014-2195-z
39. Xu, A, Pei, Y, Zhang, K, Xue, J, Ruan, S, and Zhang, G. Phylogenetic analyses and pathogenicity of a variant infectious bursal disease virus strain isolated in China. Virus Res. (2020) 276:197833. doi: 10.1016/j.virusres.2019.197833
40. Wang, W, He, X, Zhang, Y, Qiao, Y, Shi, J, Chen, R, et al. Analysis of the global origin, evolution and transmission dynamics of the emerging novel variant IBDV (A2dB1b): the accumulation of critical aa-residue mutations and commercial trade contributes to the emergence and transmission of novel variants. Transbound Emerg Dis. (2022) 69:e2832–51. doi: 10.1111/tbed.14634
41. Wang, W, Huang, Y, Zhang, Y, Qiao, Y, Deng, Q, Chen, R, et al. The emerging naturally reassortant strain of IBDV (genotype A2dB3) having segment a from Chinese novel variant strain and segment B from HLJ 0504-like very virulent strain showed enhanced pathogenicity to three-yellow chickens. Transbound Emerg Dis. (2022) 69:e566–79. doi: 10.1111/tbed.14336
42. Fan, L, Wu, T, Wang, Y, Hussain, A, Jiang, N, Gao, L, et al. Novel variants of infectious bursal disease virus can severely damage the bursa of fabricius of immunized chickens. Vet Microbiol. (2020) 240:108507. doi: 10.1016/j.vetmic.2019.108507
Keywords: infectious bursal disease virus (IBDV), vp2 gene, phylogenetic analysis, virus isolation, immunosuppression
Citation: Zhu K, Wu Q, Leng M, Wang Z and Lin W (2025) Phylogenetic analysis of vp2 gene of the infectious bursal disease virus in South China during 2023. Front. Vet. Sci. 12:1575407. doi: 10.3389/fvets.2025.1575407
Edited by:
Amro Hashish, Iowa State University, United StatesReviewed by:
Abhijeet Anil Bakre, U.S. National Poultry Research Center, Agricultural Research Service (USDA), United StatesSara Ahmed, Agricultural Research Center, Egypt
Copyright © 2025 Zhu, Wu, Leng, Wang and Lin. This is an open-access article distributed under the terms of the Creative Commons Attribution License (CC BY). The use, distribution or reproduction in other forums is permitted, provided the original author(s) and the copyright owner(s) are credited and that the original publication in this journal is cited, in accordance with accepted academic practice. No use, distribution or reproduction is permitted which does not comply with these terms.
*Correspondence: Wencheng Lin, d2VuY2hlbmdsaW5Ac2NhdS5lZHUuY24=
†These authors share first authorship