- 1Department of Animal and Veterinary Sciences, College of Agricultural and Marine Sciences, Sultan Qaboos University, Muscat, Oman
- 2Department of Food Science and Nutrition, College of Agricultural and Marine Sciences, Sultan Qaboos University, Muscat, Oman
Introduction: Phytogenic feed additives have gained increasing attention in ruminant nutrition due to their capacity to modulate ruminal fermentation and reduce methane (CH4) emissions. This study evaluated the effects of three plant-based additives.
Methods: Neem leaf (Azadirachta indica; NL), Indigofera leaf (Indigofera oblongifolia; IL), and Pumpkin peel (Cucurbita pepo; PP) included at four levels (0, 10, 20, and 30 g/kg DM) on in vitro ruminal fermentation, feed degradability, and CH4 emissions. A total mixed ration (TMR) was incubated with each additive and buffered rumen fluid using the in vitro gas production technique for 24 hours.
Results and discussion: NL and IL supplementation significantly (p < 0.05) increased gas and carbon dioxide (CO2) production, organic matter degradability, and total volatile fatty acid concentrations, particularly propionate and butyrate. Simultaneously, acetate concentration, CH4 emissions, NH3-N levels, and protozoa abundance were reduced (p < 0.05). However, PP had limited effects on these parameters. The phenolic profiles of NL and IL, notably rich in 2-hydroxycinnamic acid and p-coumaric acid, likely contributed to these outcomes. In conclusion, these findings support the use of NL and IL as effective phytogenic additives for improving rumen fermentation and mitigating CH4 production. Further in vivo trials are recommended to validate these in vitro results.
Introduction
The use of locally available phytogenic feed additives is increasingly recognized as a sustainable approach to improve ruminant nutrition and reduce environmental impacts. Plant-derived bioactive compounds, particularly phenolics and flavonoids, have been widely documented for their capacity to modulate ruminal fermentation, enhance nutrient utilization, and decrease methane (CH₄) emissions (1–3).
Among these, Indigofera oblongifolia and Azadirachta indica (neem) are multipurpose plants that thrive under arid and semi-arid conditions. Their leaves are rich in phenolic acids and flavonoids substances, which have shown antimicrobial and anti-inflammatory properties with potential effects on rumen microbial ecology (4–6). Indigofera is a wild multi-use leguminous shrub in the Fabaceae family that is widely distributed in Africa, South Asia and Southeast Asia (6). Most of the species of Indigofera generally grow in different areas as well as withstand extremes of drought and temperature environmental conditions. However, neem plant belongs to the family Meliceae and has been used to modulate rumen ecology due to the modulatory potentials of its secondary bioactive (7). Besides, Pumpkin (Cucurbita pepo) belongs to the family Cucurbitaceae and is used as remedies, pharmaceutics, and functional foods sectors for their extreme chemical properties and biological activities (8). Pumpkin peel, an agro-industrial by-product, is also rich in antioxidant and bioactive phytonutrients including carotenoids, tocopherols, and polyphenols (8, 9). Our previous study has demonstrated that dietary inclusion with 40 g of neem leaf powder can modulate rumen fermentation, enhance fiber digestibility, and improve growth performance in small ruminants (10).
However, comprehensive comparative studies evaluating these phytogenic sources at graded inclusion levels under in vitro conditions on rumen fermentative activity and CH4 emissions are still lacking. Therefore, the objective of this study was to assess the effects of incremental inclusion levels (0, 10, 20, and 30 g/kg DM) of neem leaf, indigofera leaf, and pumpkin peel powders on in vitro rumen fermentation characteristics, feed degradability, and CH4 emissions. We hypothesized that phytogenic additives, particularly those with higher phenolic acid content, would improve fermentation efficiency and mitigate CH₄ production.
Materials and methods
Study location and ethics
This study was conducted at the Feed Analysis and Food Science Laboratories, College of Agricultural and Marine Sciences (CAMS), Sultan Qaboos University (SQU), Muscat, Oman.
Phytogenic plants plant material collection and processing
Neem, Indigofera, and pumpkin plants were harvested from the university’s experimental field station (23°59′89.8″ N, 58°16′25.6″ E). Leaves of neem and Indigofera were air-dried and manually chopped. However, pumpkin peels were washed, separated, air-dried, and ground into a fine powder using a laboratory blender.
Phenolic compound analysis
For phenolic acid analysis, 1.0 g of each powdered plant material was extracted with 10 ml of analytical-grade methanol in a centrifuge tube. Samples were vortexed, sonicated at 25°C for 30 min, and filtered through a nylon syringe filter. Phenolic acids were quantified using ultra-performance liquid chromatography (UPLC) equipped with a Shimadzu SIL-30A autosampler (Tokyo, Japan) according to the method descripted by El Hilaly et al. (11) with some modifications.
Experimental diet and treatments
The total mixed ration (TMR; 50:50 forage to concentrate) consisted of Rhodes grass (Chloris gayana) hay (50%), ground yellow corn grain (27%), soybean meal (11.50%), wheat bran (10.30%), calcium carbonate (0.70%), sodium chloride (0.25%) and mineral and vitamin mixture (0.25% containing: 1.00% Ca; 0.60% P; 0.3 mg/kg Se; 8,800 IU/kg of vitamin A,; 2,200 IU/kg of vitamin D, and 33 IU/kg of vitamin E). The chemical composition of the TMR was determined according to AOAC (12) procedure for dry matter (DM) by drying in air-forced oven at 65°C to a constant weight. Ash content was determined by ashing the sample at 600°C in Muffle furnace for 2 h. Organic matter (OM) was calculated as DM—ash content. The ether extract (EE) was analyzed by Soxhlet extraction procedure. Total nitrogen (TN) was measured using a nitrogen Analyzer (BUCHI KjelMaster K-375, Flawil, Switzerland), and crude protein (CP) content was calculated as 6.25 × TN according to Kjeldahl procedure. Fiber fractions (NDF and ADF) were determined (13, 14). The content of non-fiber carbohydrates (NFC, g/kg) were calculated. The TMR chemical composition on DM basis was: 89.92% DM, 9.31% Ash, 12.86% CP, 2.28% EE, 46.74% NDF, 26.27% ADF, and 28.83% NFC.
The TMR was ground and supplemented with NL, IL, or PP at 0, 10, 20, or 30 g/kg DM. Each treatment was replicated six times in a completely randomized design.
In vitro gas production
This in vitro study utilized the GP technique using 100-ml glass syringes fitted with plungers in different incubation intervals following the methods described by Menke and Steingass (15). Briefly, a 0.500 g of each basal TMR (DM basis) along with its respective additive level, was placed into 100 ml glass syringes fitted with plungers. Rumen fluid was obtained from a fistulated Holstein cow (450 ± 15 kg BW) before morning feeding. The rumen contents were filtered and maintained at 39°C. The rumen-buffer solution was prepared according to the method described by Menke and Steingass (15). Each syringe was injected with 30 ml of buffered rumen fluid (10 ml rumen fluid: 20 ml buffer). The syringes were purged with CO₂ and incubated at 39°C for 24 h. Each phytogenic additive (n = 4 levels/experiment) was tested in six replicates, along with four blank syringes, incubated in three separate runs repeated over two different weeks. Therefore, the total number of syringes each tested additive was: 84 syringes (4 treatment levels × 6 replicates +4 blanks) × 3 runs.
Total gas, CH4 and CO2 production measurements
Net gas production (GP) was recorded at 2, 4, 8, 12, and 24 h using the syringe scale by subtracting the blank syringe (containing only rumen fluid and buffer solution) values. At each time point, 1 ml gas samples were collected from the empty area of the syringe for CH4 and CO2 concentrations analysis via gas chromatography (Agilent, model GC, 6890 N, California, US) a TCD-back detector according to Budiman and Zuas (16). After each sampling time point, the cumulative gas was vented after each reading to maintain microbial activity (17).
Feed degradability and rumen fermentation attributes measurement
Immediately after 24 h of incubation, the undigested residue in each syringe was transferred into pre-weighed porcelain crucible and dried in an oven at 65°C for 72 h, then assayed for DM and OM contents. The in vitro degradability of DM and OM was calculated based on the weight lost after 24 h following the protocol described by Al-Hasani et al. (18).
At 24 h of incubation, the buffered-rumen fluids pH was measured using a digital pH meter (HANNA Instruments, HI Microcomputer 9,025, Smithfield, VA, USA) and rumen fluid was centrifuged. The supernatant was assayed for the concentrations of ammonia nitrogen (NH3-N) using the method described by Konitzer and Voigt (19) and volatile fatty acid (VFA) following the procedure described by Al-Hasani et al. (18) and El-Zaiat et al. (20) analyses. The VFA were analyzed using a gas chromatograph (Agilent, model GC, 6890 N, California, US). The individual VFAs were identified using a polyethylene glycol TPA capillary column (HP-FFAP, Agilent 19091F-115) and a flame ionization detector (FID) set at a temperature of 260°C. The hydrogen was used as the carrier gas at a flow rate of 40 ml/min. Total protozoa count was performed according to the procedures of Dehority et al. (21).
Statistical analysis
All data were analyzed using the GLM procedure of SAS 9.1 software (SAS Inst. Inc., Cary, NC, USA). The linear and quadratic contrasts were applied to evaluate the effect of additive incremental level responses. Duncan’s test was used to assess the significant differences at p ≤ 0.05.
Results
Phenolic composition of plant additives
Table 1 presents the phenolic acid composition of the three plant-based additives. Indigofera leaf (IL) exhibited the highest total phenolic content (412.56 mg/g DM), followed by neem leaf (NL; 291.68 mg/g DM), and Pumpkin peel (PP; 63.68 mg/g DM). Furthermore, p-Coumaric (145.05 mg/g DM) and ferulic acids (104.65 mg/g DM) were the predominant phenolics in IL, while 2-hydroxycinnamic acid was the major compound in NL. The PP additive exhibited only low concentrations of caffeic acid (17.00 mg/g DM), syringic acid (14.95 mg/g DM), p-coumaric acid (8.75 mg/g DM), and ferulic acid (6.24 mg/g DM).
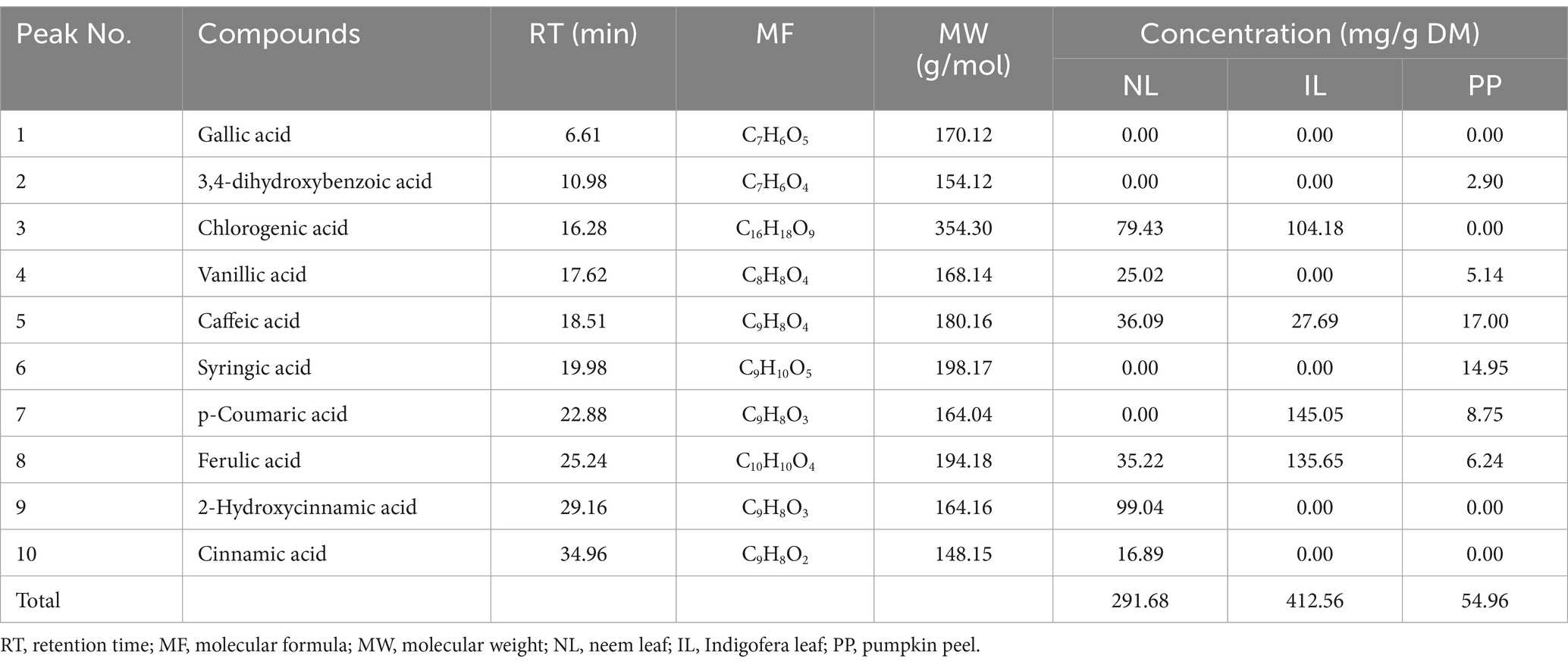
Table 1. Principal identified phenolic acids compounds of the evaluated phytogenic supplements using ultra-performance liquid chromatography.
Total GP, CH4 and CO2 emission, and feed degradability
As shown in Table 2, net GP after 24 h increased linearly (p < 0.05) with rising inclusion levels of NL and IL but not with PP. All NL, IP, and PP levels linearly increased (p < 0.05) CO2 production. Conversely, CH4 production declined significantly (p < 0.05) in response to increasing inclusion levels of NL, IL, and PP. However, OM degradability increased linearly with NL and IL (p < 0.05), but no significant effect was observed with PP.
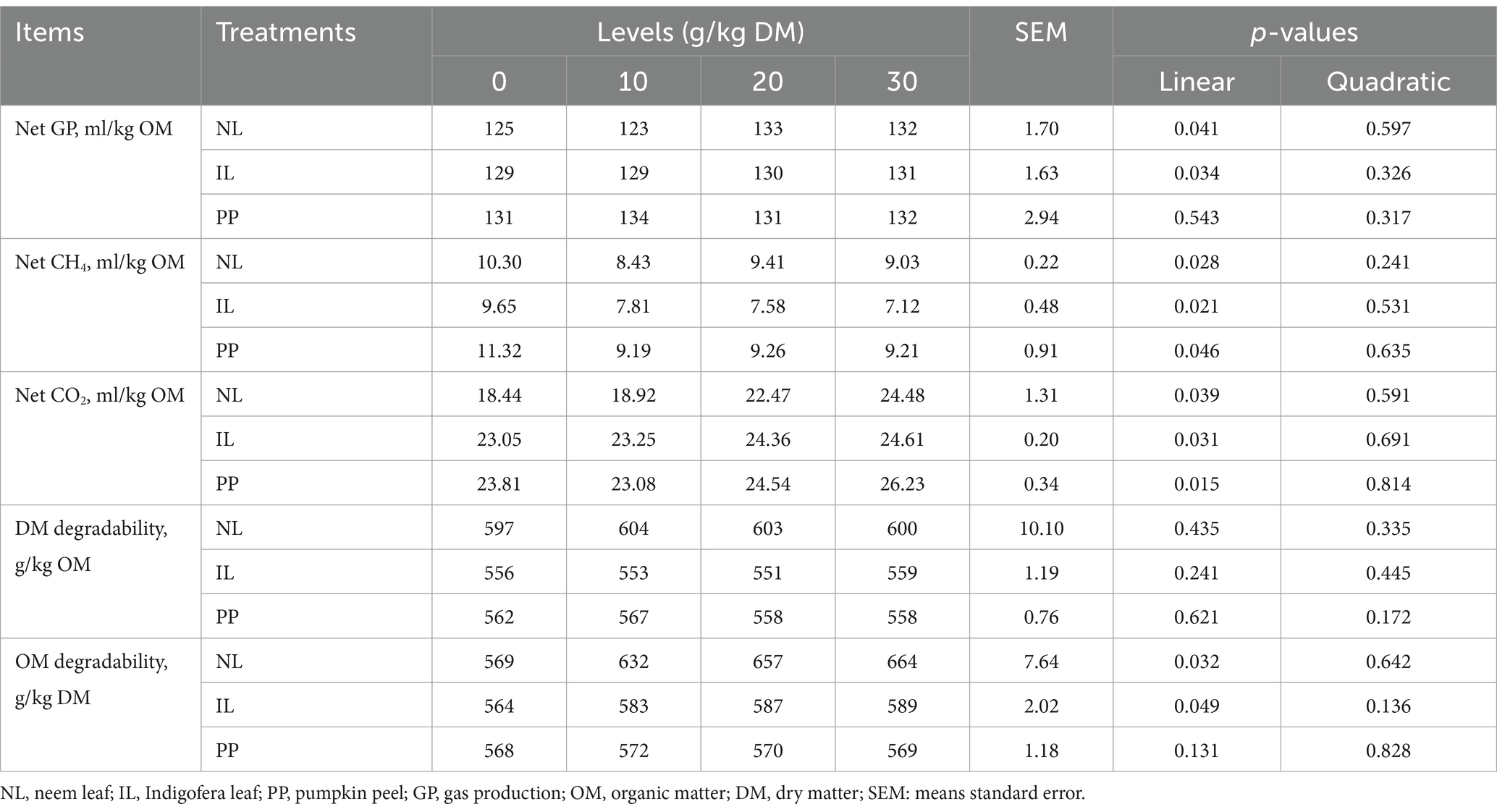
Table 2. Effect of different phytogenic supplements on in vitro gas production (GP), methane production (CH4) and substrate degradation within 24 h of incubation.
Rumen fluid fermentation end-products characteristics
Table 3 displays the effects of the additives on ruminal pH, NH3-N, and protozoa abundance. After 24 h of incubation, ruminal pH was unaffected by additive inclusion (p > 0.05). However, ruminal NH3-N concentration and protozoal counts decreased linearly (p < 0.05) with increasing levels of NL and IL. According to Table 4, total VFA concentration increased linearly with all levels of NL (p = 0.047) and IL (p = 0.031), while no change was observed with PP. Acetate proportion decreased linearly and propionate and butyrate proportions increased with all three additives (p < 0.05), leading to a linear reduction (p < 0.05) in acetate-to-propionate ratio.
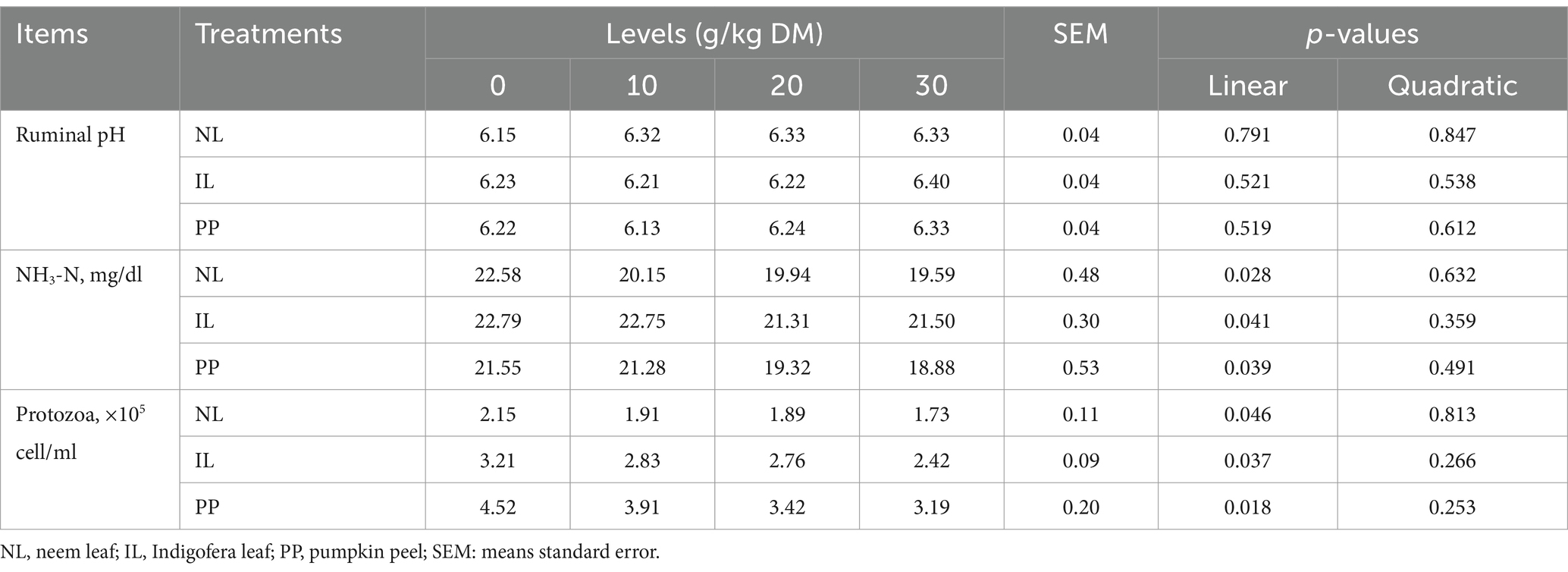
Table 3. Effect of different phytogenic supplements on in vitro ruminal pH, ammonia-nitrogen (NH3-N) concentration and protozoa abundance within 24 h of incubation.
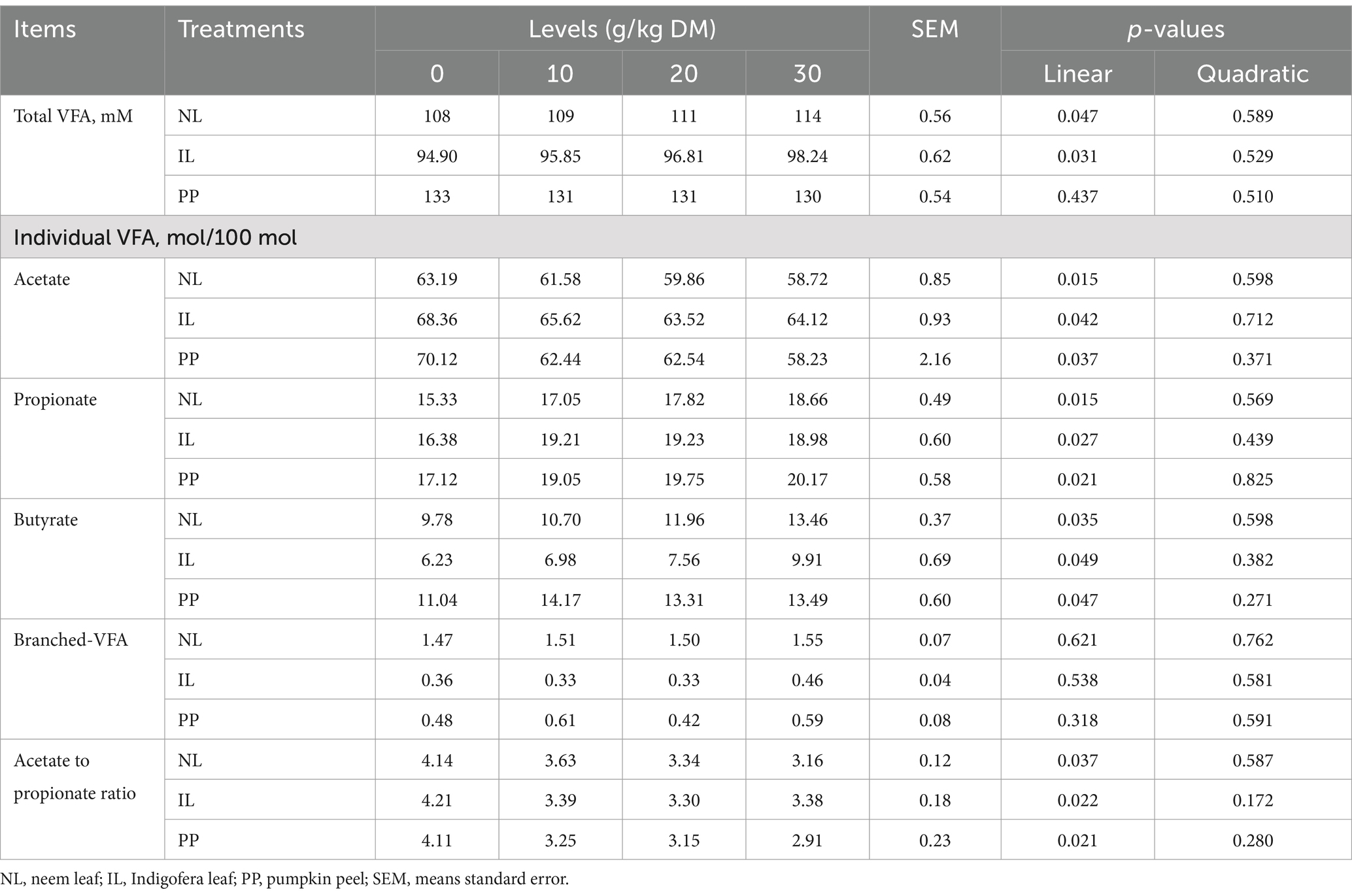
Table 4. Effect of different phytogenic supplements on in vitro ruminal volatile fatty acids (VFA) profile within 24 h of incubation.
Discussion
The in vitro short-term fermentation techniques have been thoroughly applied as a rapid and cost-effective assessment approach to investigate the potential of natural-based additives on rumen fermentation patterns and CH4 emission (18, 22). This study evaluated the effects of three phytogenic feed additives on in vitro rumen fermentation, gas emissions, and nutrient degradability. The results demonstrated that Neem leaf (NL) and Indigofera leaf (IL) significantly influenced fermentation parameters, whereas Pumpkin peel (PP) exhibited limited effects. The current in vitro study is the first to evaluate whether NL, IL and PP derived additives can modify the rumen fermentation profile, including methanogenesis suppression. All phytogenic-based feed ingredients used had effects on ruminal fermentation parameters that were measured. Consistent with previous findings (23, 24), our current results showed that p-coumaric acid and ferulic acid present in IL additive effectively modified ruminal fermentation characteristics by affecting both rumen microbiota and/or their enzyme activity. Additionally, 2-Hydroxycinnamic acid, one of major phenolic acid metabolites represents 33.90% of NL (99.04 mg/g DM) compounds. This compound may potentially affect the enzyme activity of rumen microbes due to their antimicrobial and anti-inflammatory properties (5). It has been well documented that greater substrate degradability demonstrated higher GP (25). In this context, increased net GP in this study coupled with greater substrate degradation rate (22, 26). The predominance of phenolic acids profile in the tested additives substantially affected TMR digestion and fermentability, resulted in increased OM degradability through maintaining the ruminal pH in normal ranges after 24 h of incubation. The increased gas and CO₂ production observed with NL and IL inclusion are indicative of enhanced microbial fermentation, likely driven by the abundance of phenolic compounds such as p-coumaric and 2-hydroxycinnamic acids. These compounds have been shown to modulate microbial populations and enzymatic activity in the rumen (5, 23). The improvement in organic matter degradability with NL and IL further supports the hypothesis that these bioactives enhance ruminal fermentability. A notable finding was the consistent reduction in CH₄ production across all additive levels for NL and IL (14 and 35%, respectively). This supports earlier evidence that the phenolic compounds can disrupt methanogenesis by altering microbial hydrogen use, favoring propionate formation over acetate (27, 28). These mode of action against the rumen microorganisms involves damaging the cell membranes and structures and also by the inactivation of cell enzymes (29). Comparable findings were reported by Haisan et al. (30), greater propionate production at the expense of acetate could be responsible for the greater CO2 production in the rumen.
The reduced acetate-to-propionate ratio observed aligns with this mechanism, indicating a shift towards glucogenic fermentation pathways that are energetically more favorable and environmentally beneficial. Therefore, the reduced fiber digestion further reveals the antimicrobial effects of the phenolic metabolites of the additives tested on the rumen microbiome as evidenced by reduced acetate production. These findings suggest that differences in the potentials of these phytonutrients (phenolic compounds) could be the result of differences in the bacterial (Gram-negative and Gram-positive) species cell wall structures (29). These findings have suggested that feed ingredients used could be considered feed additive candidates for use in ruminants. The ruminal VFAs produced are considered the primary source of metabolizable energy supply for ruminants (31). Although PP supplementation decreased acetate and increased propionate concentrations, it did not significantly affect gas production or total VFA concentration. This could be attributed to its relatively low phenolic content, as confirmed by UPLC analysis. These findings suggest that the effectiveness of phytogenic additives depends not only on their botanical source but also on their phytochemical composition and concentration. The decline in NH₃-N concentrations and protozoa abundance with NL and IL addition points to improved nitrogen utilization and possible anti-protozoal effects of their bioactives. Such effects may contribute to reduced protein degradation in the rumen, aligning with the observed decrease in ammonia levels. This also has implications for reducing nitrogen excretion and its associated environmental impact (32).
Increased propionate production is closely associated with reduced methanogenesis in the rumen (28). Additionally, propionate formation mostly competes with the methanogenesis for the hydrogen available in the rumen (33). A consistent finding when P-Coumaric acid was included in the in vitro fermentation is the inhibition of Ruminococcus albus and Ruminococcus flavefaciens in the rumen, leading to reduce fiber degradation (24). Thus, altered ruminal VFA profile might be related to the presence of phytonutrient phenolic used on ruminal microflora, shifting the microbiota activities away from acetate through diverted hydrogen to more propionate, and subsequently hence greater CH4 suppression (37). While promising, the current findings are limited by the in vitro nature of the study, which does not capture the full complexity of rumen dynamics in vivo. Moreover, microbial profiling was not conducted, which would have strengthened the understanding of the mechanisms underpinning the observed responses. Future studies should explore microbial population shifts and validate these results under practical feeding conditions. Additional influences from the presence of phenolic compounds in tested additives that may protect the dietary protein from ruminal degradation (34), resulting in large NH3-N concentration reduction reported herein. Consequently, reduced N losses may be expected through feces and urine excretion, taking into account mitigating the environmental impact.
Interestingly, the ruminal methanogenesis is strongly influenced without adversely affecting the other ruminal fermentation parameters, such as the VFA profile. The reason for the small reduction in CH4 production might be that ruminal microbes susceptibility could be adapted against the presence of flavonoids and phenolic metabolites (35). As a result, the effects on ruminal acetogens and methanogens could be expected, which may explain the pronounced reduction in acetate and CH4 production as a result of reduced fiber degradation. However, the phenolic compounds exhibit antimicrobial properties by reducing ruminal protozoal abundance (36), which may be another reason for the decreased CH4 formation (35). Thus, the linear reduction in CH4 formation is likely not to be solely ascribed to the changes in the VFAs profile. The present study highlights the potential of NL and IL as effective phytogenic additives to enhance rumen fermentation and mitigate methane and nitrogen emissions. Their rich phenolic content appears to be central to these effects. Although PP contains some bioactive compounds, it demonstrated only modest benefits in this context.
Conclusion
This in vitro study demonstrated that neem and indigofera leaf powders, when included at increasing dietary levels, can positively influence ruminal fermentation dynamics by enhancing gas production, organic matter degradability, and volatile fatty acid profiles, while concurrently reducing methane and ammonia nitrogen concentrations. These effects are likely attributable to their high phenolic acid content. In contrast, pumpkin peel powder exhibited minimal influence on fermentation parameters, potentially due to its lower phytochemical content. These findings support the potential use of neem and indigofera leaf powders as natural phytogenic additives to promote environmentally sustainable ruminant feeding strategies. However, further in vivo research is necessary to confirm their efficacy under practical animal production conditions.
Data availability statement
The raw data supporting the conclusions of this article will be made available by the authors, without undue reservation.
Ethics statement
The animal studies were reviewed and approved by Sultan Qaboos University, Ethics Committee for Animal Use in Research (Approval No.: SQU/EC-AUR/2024-2025/28).
Author contributions
HE-Z: Conceptualization, Funding acquisition, Investigation, Project administration, Software, Writing – original draft, Writing – review & editing. HM: Methodology, Writing – original draft. SA-H: Formal analysis, Methodology, Writing – original draft. RA-M: Formal analysis, Methodology, Writing – original draft. SAR: Data curation, Formal analysis, Writing – original draft. KA-K: Formal analysis, Methodology, Resources, Writing – original draft. AA-S: Formal analysis, Methodology, Resources, Writing – original draft. NA-H: Investigation, Validation, Writing – original draft.
Funding
The author(s) declare that financial support was received for the research and/or publication of this article. All authors are deeply grateful for the financial support by the SQU (Project No.: IG/AGR/ANVS/21/01).
Acknowledgments
We gratefully acknowledge the farm staff at the Agricultural Experimental Station (AES/SQU) for their cooperation, time, and support. This study is part of the undergraduate research projects carried out by Hussein A. Masood, Samira S. Al Hinai, and Reem H. Al Maamari in at CAMS/SQU.
Conflict of interest
The authors declare that the research was conducted in the absence of any commercial or financial relationships that could be construed as a potential conflict of interest.
Generative AI statement
The authors declare that no Generative AI was used in the creation of this manuscript.
Publisher’s note
All claims expressed in this article are solely those of the authors and do not necessarily represent those of their affiliated organizations, or those of the publisher, the editors and the reviewers. Any product that may be evaluated in this article, or claim that may be made by its manufacturer, is not guaranteed or endorsed by the publisher.
References
1. El-Zaiat, HM, Ku-Vera, JC, and Soltan, YA. Natural phytochemicals to enhance animal productivity and health status with low greenhouse gas emissions. Front Vet Sci. (2023) 10:1280611. doi: 10.3389/fvets.2023.1280611
2. Michalak, M, Wojnarowski, K, Cholewińska, P, Szeligowska, N, Bawej, M, and Pacoń, J. Selected alternative feed additives used to manipulate the rumen microbiome. Animals. (2021) 11:1542. doi: 10.3390/ani11061542
3. Wanapat, M, Viennasay, B, Matra, M, Totakul, P, Phesatcha, B, Ampapon, T, et al. Supplementation of fruit peel pellet containing phytonutrients to manipulate rumen pH, fermentation efficiency, nutrient digestibility and microbial protein synthesis. J Sci Food Agric. (2021) 101:4543–50. doi: 10.1002/jsfa.11096
4. Niroula, A, Khatri, S, Khadka, D, and Timilsina, R. Total phenolic contents and antioxidant activity profile of selected cereal sprouts and grasses. Int J Food Prop. (2019) 22:427–37. doi: 10.1080/10942912.2019.1588297
5. Peña-Torres, EF, González-Ríos, H, Avendaño-Reyes, L, Valenzuela-Grijalva, NV, Pinelli-Saavedra, A, Muhlia-Almazán, A, et al. Hydroxycinnamic acids in animal production: pharmacokinetics, pharmacodynamics and growth promoting effects. Review. Rev Mex Cienc Pecu. (2019) 10:391–415. doi: 10.22319/rmcp.v10i2.4526
6. Singh, JP, Patidar, A, Venkatesan, K, Kumar, M, Swami, S, Saranya, R, et al. Collection, distribution, characterization and utilization of Indigofera oblongifolia Forssk.: an important underutilized multi-use leguminous shrub of Indian hot arid region. Plant Genet Res. (2023) 21:369–76. doi: 10.1017/S1479262123000837
7. Taethaisong, N, Paengkoum, S, Kaewwongsa, W, Onjai-Uea, N, Thongpea, S, and Paengkoum, P. The effect of neem leaf supplementation on growth performance, rumen fermentation, and ruminal microbial population in goats. Animals. (2023) 13:890. doi: 10.3390/ani13050890
8. Gavril, RN, Stoica, F, Lipsa, FD, Constantin, OE, Stănciuc, N, Aprodu, I, et al. Pumpkin and pumpkin by-products: a comprehensive overview of phytochemicals, extraction, health benefits, and food applications. Food Secur. (2024) 13:2694. doi: 10.3390/foods13172694
9. Ibrahim, RE, Elshopakey, GE, Younis, EM, Abdelwarith, AA, Yaseen, A, Khamis, T, et al. Peel of pumpkin (Cucurbita pepo) diets boost growth, digestive-absorptive functions, immune-antioxidant indices, and regulate immunomodulatory genes and mTOR/MAPK-P38/apoptosis signaling pathway in Oreochromis niloticus. Aquacult Rep. (2025) 40:102548. doi: 10.1016/j.aqrep.2024.102548
10. El-Zaiat, HM, Elshafie, EI, Al-Marzooqi, W, and Dughaishi, KA. Effects of neem (Azadirachta indica) leaf powder supplementation on rumen fermentation, feed intake, apparent digestibility and performance in Omani sheep. Animals. (2022) 12:3146. doi: 10.3390/ani12223146
11. El Hilaly, J, Ennassir, J, Benlyas, M, Alem, C, Amarouch, MY, and Filali-Zegzouti, Y. Anti-inflammatory properties and phenolic profile of six Moroccan date fruit (Phoenix dactylifera L.) varieties. J King Saud Univ Sci. (2018) 30:519–26. doi: 10.1016/j.jksus.2017.08.011
12. AOAC. Official Methods of Analysis, 17th ed.; Association of Official Analytical Chemists. MD, USA: Gaithersburg, AOAC (2000).
13. Roberston, JB, and Van Soest, PJ. Chapter 9: the detergent system of analysis In: WPT James and O Theander, editors. The analysis of dietary Fiber in food. New York, NY, USA: Marcel Dekker (1981). 123–58.
14. Van Soest, PJ, Robertson, JB, and Lewis, BA. Methods for dietary fibre, neutral detergent fibre, and nonstarch polysaccharides in relation to animal nutrition. J Dairy Sci. (1991) 74:3583–97. doi: 10.3168/jds.S0022-0302(91)78551-2
15. Menke, KH, and Steingass, H. Estimation of the energetic feed value obtained from chemical analysis and in vitro gas production using rumen fluid. Anim Res Dev. (1988) 28:7–55.
16. Budiman, H, and Zuas, O. Comparison between GC-TCD and GC-FID for the determination of propane in gas mixture. Proc Chem. (2015) 16:465–72. doi: 10.1016/j.proche.2015.12.080
17. Theodorou, MK, Williams, BA, Dhanoa, MS, McAllan, AB, and France, J. A simple gas production method using a pressure transducer to determine the fermentation kinetics of ruminant feeds. Anim Feed Sci Technol. (1994) 48:185–97. doi: 10.1016/0377-8401(94)90171-6
18. Al-Hasani, F, El-Zaiat, HM, Al-Kharousi, K, Rahman, MS, Al-Khalili, M, and Al-Habsi, N. Assessing the efficacy of date-pits holocellulose as a novel additive candidate for ruminant feeding. Trop Anim Health Prod. (2024) 56:229. doi: 10.1007/s11250-024-04071-2
19. Konitzer, K, and Voigt, S. Direct determination of ammonium in blood and tissue extracts by means of the phenol by chlorite reaction Clinica chimica acta Int J. Clin Chem. (1963) 8:5–11. doi: 10.1016/0009-8981(63)90192-X
20. El-Zaiat, HM, Al-Marzooqi, W, and Al-Kharousi, K. Exploring rumen fermentation and microbial populations in Dhofari goats fed chitosan-added diet. Anim Biotechnol. (2024) 35:2337748. doi: 10.1080/10495398.2024.2337748
21. Dehority, B, Damron, W, and McLaren, J. Occurrence of the rumen ciliate Oligoisotricha bubali in domestic cattle (Bos taurus). Appl Environ Microbiol. (1983) 45:1394–7. doi: 10.1128/aem.45.4.1394-1397.1983
22. Ronquillo, G, Ghavipanje, M, and Sainz-Ramírez, N. Effects of plant extracts on in-vitro gas production kinetics and ruminal fermentation of four fibrous feeds: towards sustainable animal diets. Chil J Agric Anim Sci. (2023) 39:319–26. doi: 10.29393/CHJAA39-28EPME70028
23. López-Baca, MÁ, Muhlia-Almazán, A, Montalvo-Corral, M, Pinelli-Saavedra, A, Islava-Lagarda, TY, Dávila-Ramírez, JL, et al. Ferulic acid and Clinoptilolite affect in vitro rumen fermentation characteristics and bacterial abundance. Ferment. (2024) 10:549. doi: 10.3390/fermentation10110549
24. Varel, VH, and Jung, HJG. Influence of forage phenolics on ruminal fibrolytic bacteria and in vitro fiber degradation. Appl Environ Microbiol. (1986) 52:275–80. doi: 10.1128/aem.52.2.275-280.1986
25. Dijkstra, J, Kebreab, E, Bannink, A, France, J, and Lopez, S. Application of the gas production technique to feed evaluation systems for ruminants. Anim Feed Sci Technol. (2005) 123-124:561–78. doi: 10.1016/j.anifeedsci.2005.04.048
26. Akanmu, AM, and Hassen, A. The use of certain medicinal plant extracts reduced in vitro methane production while improving in vitro organic matter digestibility. Anim Prod Sci. (2017) 58:900–8. doi: 10.1071/AN16291
27. Kholif, AE, Rahman, MA, Abo El-Nor, SA, Morsy, TA, Gouda, GA, Fahmy, M, et al. Efficacy of Salvia officinalis shrub as a sustainable feed additive for reducing ruminal methane production and enhancing fermentation in ruminants. Animals. (2024) 14:1648. doi: 10.3390/ani14111648
28. Wang, K, Xiong, B, and Zhao, X. Could propionate formation be used to reduce enteric methane emission in ruminants? Sci Total Environ. (2023) 855:158867. doi: 10.1016/j.scitotenv.2022.158867
29. Suriyapha, C, Phupaboon, S, Dagaew, G, Sommai, S, Matra, M, Prachumchai, R, et al. In vitro fermentation end-products and rumen microbiome as influenced by microencapsulated phytonutrient pellets (LEDRAGON) supplementation. Sci Rep. (2024) 14:14425. doi: 10.1038/s41598-024-59697-x
30. Haisan, J, Sun, Y, Guan, LL, Beauchemin, KA, Iwaasa, A, Duval, S, et al. The effects of feeding 3-Nitrooxypropanol on methane emissions and productivity of Holstein cows in mid lactation. J Dairy Sci. (2014) 97:3110–9. doi: 10.3168/jds.2013-7834
31. Van Soest, PJ. Use of detergents in the analysis of fibrous feeds. II. A rapid method for the determination of fiber and lignin. J Assoc Off Anal Chem. (1963) 46:829–35. doi: 10.1093/jaoac/46.5.829
32. Busquet, M, Calsamiglia, S, Ferret, A, and Kamel, C. Plant extracts affect in vitro rumen microbial fermentation. J Dairy Sci. (2006) 89:761–71. doi: 10.3168/jds.S0022-0302(06)72137-3
33. Silva, TH, Amâncio, BR, Magnani, E, Meurer, GW, Reolon, HG, Timm, TG, et al. Evaluation of direct-fed microbials on in vitro ruminal fermentation, gas production kinetic, and greenhouse gas emissions in different ruminants’ diet. Front Anim Sci. (2024) 5:1320075. doi: 10.3389/fanim.2024.1320075
34. Kumar, K, Dey, A, Rose, MK, and Dahiya, SS. Impact of dietary phytogenic composite feed additives on immune response, antioxidant status, methane production, growth performance and nutrient utilization of buffalo (Bubalus bubalis) calves. Antioxidants. (2022) 11:325. doi: 10.3390/antiox11020325
35. Li, M, Hassan, F, Peng, L, Xie, H, Liang, X, Huang, J, et al. Mulberry flavonoids modulate rumen bacteria to alter fermentation kinetics in water buffalo. PeerJ. (2022) 10:e14309. doi: 10.7717/peerj.14309
36. Patra, AK, and Saxena, J. A new perspective on the use of plant secondary metabolites to inhibit methanogenesis in the rumen. Phytochemistry. (2010) 71:1198–222. doi: 10.1016/j.phytochem.2010.05.010
Keywords: feed degradability, methane mitigation, phenolic acids, ruminal characteristics, tropical additives
Citation: El-Zaiat HM, Masood HA, Al Hinai SS, Al Maamari RH, Al Riyami SS, Al-Kharousi K, Al-Salami AH and Al-Habsi N (2025) Assessment of different phytogenic-based additives on in vitro rumen fermentation profile and methane emissions. Front. Vet. Sci. 12:1591700. doi: 10.3389/fvets.2025.1591700
Edited by:
Adham Al-Sagheer, Zagazig University, EgyptReviewed by:
Ahmed E. Kholif, North Carolina Agricultural and Technical State University, United StatesAdibe Luiz Abdalla Filho, University of Lisbon, Portugal
Copyright © 2025 El-Zaiat, Masood, Al Hinai, Al Maamari, Al Riyami, Al-Kharousi, Al-Salami and Al-Habsi. This is an open-access article distributed under the terms of the Creative Commons Attribution License (CC BY). The use, distribution or reproduction in other forums is permitted, provided the original author(s) and the copyright owner(s) are credited and that the original publication in this journal is cited, in accordance with accepted academic practice. No use, distribution or reproduction is permitted which does not comply with these terms.
*Correspondence: Hani M. El-Zaiat, aC5hbHphaWF0QHNxdS5lZHUub20=