Asymptomatic Bacterial Vaginosis Is Associated With Depletion of Mature Superficial Cells Shed From the Vaginal Epithelium
- 1Institute for Genome Sciences, University of Maryland School of Medicine, Baltimore, MD, United States
- 2Department of Microbiology and Immunology, University of Maryland School of Medicine, Baltimore, MD, United States
- 3Department of Epidemiology and Public Health, University of Maryland School of Medicine, Baltimore, MD, United States
Previous studies have described bacterial vaginosis (BV) as associated with increased cell-shedding from the cervicovaginal epithelium. Cell-shedding in excess of cell-proliferation is thought to decrease epithelial barrier function and increase susceptibility to infection. This study evaluated the number of shed cells in mid-vaginal smears from women with a diagnosis of symptomatic BV (sBV, n = 17), asymptomatic BV (aBV, n = 71), or no BV (n = 104) by Amsel criteria. The sBV smears contained significantly more shed cells (median 158/100X field) than no BV smears (median 91/100X field), p = 7.2e−9. However, we observed that aBV smears contained significantly fewer shed cells (median 35/100X field) than no BV smears, p = 22.0e−16. The sizes of cell-aggregates (cells shed in sometimes multilayered sections with intact cell-cell attachments) followed the same pattern. Cell-aggregates in sBV smears were significantly larger (median ~220,000 μm2) than those in no BV smears (median ~50,000 μm2), p = 1.8e−6, but cell-aggregates in aBV smears were significantly smaller (median ~7,000 μm2) than those in no BV smears, p = 0.0028. We also compared the superficial cell index (SCI), a measure of cervicovaginal epithelial cell maturity, in no BV and aBV smears with relatively low numbers of shed cells (≤50/100X field). The SCI of no BV smears was significantly higher (median 0.86) than that of aBV smears (median 0.35), p = 4.3e−98, suggesting a depletion of mature cells with exposure and shedding of underlying immature cells in aBV with low number of shed cells. These results indicate that aBV may contribute disproportionately to the increased susceptibility to reproductive tract infections associated with BV. Our findings remained true when considering only those smears in which the microbiota comprised a diverse set of strict and facultative anaerobic bacteria [Community State Type IV (n = 162)], thus excluding those dominated by Lactobacillus spp. This is consistent with our developing hypothesis that high-shedding sBV and low-shedding aBV could be temporally separated phases of the same condition, rather than two separate forms of BV. These findings might inform future work on clinical management of symptomatic and asymptomatic bacterial vaginosis.
Introduction
The cervicovaginal microbiota is known to contribute to a woman's protection against and susceptibility to reproductive tract infections (RTIs). Women whose cervicovaginal microbiota is composed predominantly of Lactobacillus spp. are at decreased risk of many sexually transmitted infections, including HIV (Taha et al., 1998; Gosmann et al., 2017), HPV (Mitra et al., 2016), HSV-2 (Cherpes et al., 2003), trichomoniasis (Brotman et al., 2012), gonorrhea and chlamydia (Wiesenfeld et al., 2003) compared to women with microbiota comprising strict and facultative anaerobic bacteria. The latter is what broadly characterizes bacterial vaginosis (BV), a condition that is defined differently in clinical and research settings (Mckinnon et al., 2019). Clinically, Amsel-BV (Amsel et al., 1983) is characterized by the presence of certain clinical signs with patients reporting symptoms (symptomatic BV [sBV]) or no symptoms (asymptomatic BV [aBV]) on direct questioning (Fleury, 1983; Eschenbach et al., 1988). In research studies, Nugent-BV is characterized, through microscopic examination of a vaginal smear, by a predominance of Gram-negative Gardnerella and Bacteroides spp. morphotypes rather than Gram-positive Lactobacillus spp. morphotypes (Nugent et al., 1991). Vaginal microbiota assessment using culture-independent approaches based on the quantification and characterization of bacterial 16S rRNA gene sequences defines molecular-BV when a microbiota both lacks high relative abundance of Lactobacillus spp. and is composed predominantly of a wide array of strict and facultative anaerobic bacteria [Community State Type (CST) IV] (Gajer et al., 2012). Neither Nugent-BV nor molecular-BV can distinguish between sBV and aBV in the absence of a clinical examination and patient questioning. Although the concordance among the methods is not complete, it is generally true that Amsel-BV, Nugent-BV, and molecular-BV are progressively more inclusive: in a given population, more women are positive for Nugent-BV than for Amsel-BV, and more are positive for molecular-BV than for Nugent-BV (Mckinnon et al., 2019).
The cervicovaginal epithelium is the site at which multiple defenses against STIs and RTIs are deployed (Hickey et al., 2011) and Lactobacillus spp. contribute to: 1/ physical defenses, including mucus-trapping of pathogens (Nunn et al., 2015), suppression of inflammatory de-keratinization of epithelial cells (Zárate et al., 2009) and control of cell proliferation often necessary for infection (Edwards et al., 2019); 2/ immunological defenses, including suppression of pro-inflammatory signaling through toll-like receptors (Mirmonsef et al., 2011) and suppression of pro-inflammatory cytokine expression (Jespers et al., 2017); and 3/ biochemical defenses, including inducing expression of protective peptides (Yarbrough et al., 2015) and production of lactic acid (Tachedjian et al., 2017).
The homeostatic balance between the proliferation/maturation and shedding/loss of cells on the vaginal epithelium is likely critical in maintaining these defenses. Balanced proliferation/maturation and shedding/loss means the luminal surface consists of dead detaching cells and the adherence of pathogens to these sloughing cells has been hypothesized as a mechanism to provide some protection to the underlying living cells, which are vulnerable to productive infection (Anderson et al., 2014). Accelerated shedding/loss of cells from the vaginal epithelium, therefore, could be expected to decrease this protective function and thus increase susceptibility to STIs and RTIs. The composition of the cervicovaginal microbiota may affect the proliferation/maturation and shedding/loss balance: approximately twice as many shed epithelial cells were found in a study of vaginal smears from women with Nugent-BV, vs. women with predominantly Lactobacillus spp. microbiota (Amegashie et al., 2017). Additionally, the composition of the cervicovaginal microbiota has been shown to control the expression of the host microRNA miR-193b which reduces cell proliferation (Edwards et al., 2019). Cell proliferation was lower in women with a BV-associated microbiota (Edwards et al., 2019), as were host proteins associated with epithelial maturation (Zevin et al., 2016). Further supporting this finding are observations of fewer mature and more immature epithelial cells in cervicovaginal samples from women lacking lactobacilli (Fowler, 2012). At the same time, increased cell-shedding could eliminate non-Lactobacillus spp. bacteria attaching to the epithelium, such as G. vaginalis or Atopobium vaginae, which can form resilient biofilms (Hardy et al., 2016). Only symptomatic Amsel-BV is typically treated (Workowski and Bolan, 2015); however, inflammatory changes on the cervicovaginal epithelium are found in women with Nugent-BV (Thurman et al., 2015; Jespers et al., 2017) and molecular-BV (Anahtar et al., 2015), and so it is not surprising that the increased susceptibility to infections associated with BV also accrues to women with Nugent-BV (Cherpes et al., 2003; Wiesenfeld et al., 2003) and molecular-BV (Brotman et al., 2012, 2014; Gosmann et al., 2017).
The objective of the current study was to determine whether the number and maturity of shed epithelial cells observed in sBV and aBV differ, utilizing a collection of cervicovaginal smears for which microbiota composition and clinical data were available.
Materials and Methods
Clinical Samples
The current study utilized samples and data from the UMB-HMP study (Ravel et al., 2013). A total of 135 non-pregnant, reproductive-aged women took part in a 10-weeks observational longitudinal study during which participants self-collected daily vaginal samples, under a protocol approved by the Institutional Review Boards of the University of Alabama at Birmingham and of the University of Maryland School of Medicine. Written informed consent was obtained from all participants.
The study included examination by a clinician at enrollment, week 5 and 10, or at interim times if vaginal symptoms were reported. None of the samples used in this study were positive for STI or yeast infection. The clinician's record for each examination included a diagnostic of BV performed according to the Amsel criteria (Amsel et al., 1983). A diagnosis of symptomatic BV (sBV) was established when the participant reported symptoms on direct questioning and fulfilled at least three of the four Amsel's criteria; a diagnosis of asymptomatic BV (aBV) was established when the participant did not report symptoms but fulfilled at least three of the Amsel's criteria. Each day, participants self-collected a vaginal swab, smeared it on a microscope slide and placed the slide in a protective sleeve. Participants dropped off the slides to the clinic every week. The slides were Gram-stained and scored for Nugent-BV (Nugent et al., 1991) by three independent readers (Ravel et al., 2013). Another daily swab stored in Amies transport medium and immediately frozen at −20°C was used for extraction and purification of genomic DNA using a QIAsymphony robotic platform and QIAGEN CellFree 500 kits (QIAGEN, Valencia CA); Metataxonomic analysis (Marchesi and Ravel, 2015): the V3-V4 hypervariable regions of bacterial 16S rRNA genes were amplified and sequenced on an Illumina MiSeq Instrument to obtain the bacterial composition and abundance of each sample as described previously (Fadrosh et al., 2014). Sequencing was performed at the Institute for Genome Sciences' Genomic Resource Center (GRC) at the University of Maryland School of Medicine (marylandgenomics.com). CST (Gajer et al., 2012) were assigned using VALENCIA, a novel nearest centroid classification algorithm based on the classification of over 13,000 vaginal microbiota dataset. This approach to CST assignment is more robust than standard within-study hierarchical clustering and allows for between-studies comparisons (Ravel, 2018).
Microscopy
Vaginal smears collected on the day of clinical examination (study entry, week 5 and 10 for each participants) were evaluated as described below and the findings evaluated with respect to associated clinical and microbiota data.
A total of 192 smears from 126 women met the criteria above and were available to be examined. Following the method previously described to evaluate cervicovaginal epithelial cell-shedding (Amegashie et al., 2017), slides were visualized using a Zeiss Plan-ACHROMAT 10x objective on a Zeiss Primo Star microscope (Carl Zeiss Microscopy LLC, Thornwood NY) for a total magnification of 100x, and images of three “representative” fields were captured from each slide using a Zeiss Axiocam ICc3 camera and software. “Representative” fields were located within the main body of the smear, not near the start, end, or margins; “representative” fields were chosen to exclude aggregations of epithelial cells too dense to distinguish cells. All complete epithelial cells in the three images were counted (i.e., cells falling partly outside the image were not counted) and the mean count calculated. Images of three “representative” cell-aggregations were captured, also from locations within the main body of the smear, away from the start, end, or margins. Cell-aggregation size was measured using the “measure > outline” tool of the imaging software (unit μm2) (AxioVision v 4.8.2.0); the mean cell-aggregation size was calculated. When the mean epithelial cell count was ≤50, the three captured images were further analyzed, counting the number of superficial cells (i.e., cells with a condensed nucleus, large cytoplasmic space, and polygonal shape with thin, angular margins; Anderson et al., 2014). From this, the superficial cell index (SCI = number of superficial cells/number of all epithelial cells) was calculated (range 0.0–1.0; Stupnicki and Teter, 1970). A SCI of 1.0 corresponds to high numbers of mature cells and low or no immature cells, while a low SCI corresponds to low numbers of mature epithelial cells and high numbers of immature epithelial cells. Example of different types of smears are shown on Figure 1. All measurements are listed in Supplementary Table 1.
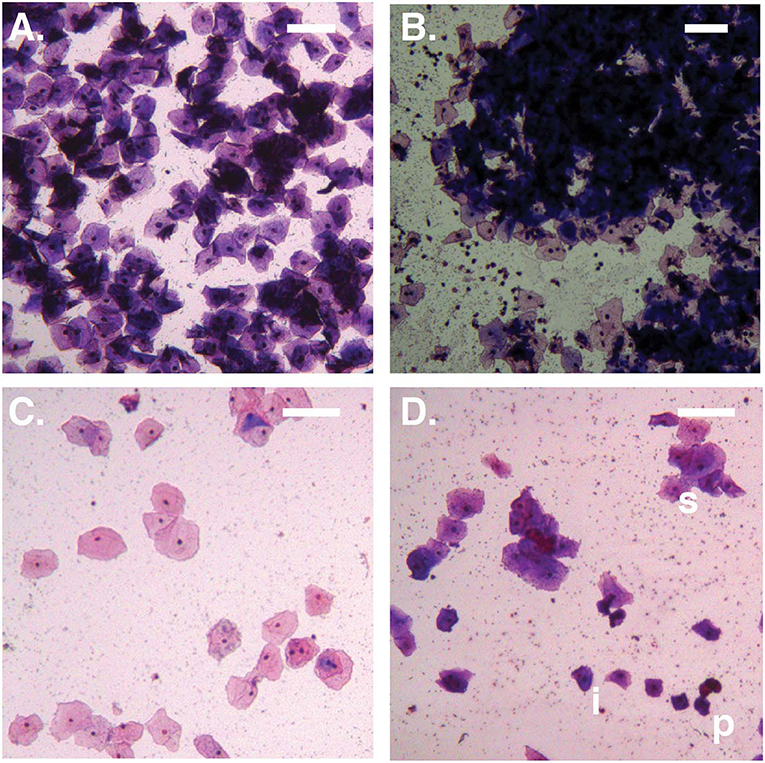
Figure 1. Bright-field micrographs (100X total magnification). (A) No Amsel-BV sample with cell count >100/100X field, showing predominantly superficial cells shed as singletons. (B) Symptomatic Amsel-BV sample with cell count >100/100X field, showing predominantly superficial cells shed in large aggregates. (C) No Amsel-BV sample with cell count <50/100X field, showing predominantly superficial cells. (D) Asymptomatic Amsel-BV sample with cell count <50/100X, showing superficial cells (example marked s) shed in combination with intermediate (example marked i) and parabasal (example marked p) cells. Scale bars represent 100 μm.
Statistical Analysis
Cell counts, cell-aggregate sizes, and SCI values are reported as median and interquartile range. Comparisons of cell counts were performed by fitting a Bayesian Laplace subject-wise random effects model to the log10 transformed data. Comparisons of cell-aggregate sizes were made in the same way. SCI values were computed within a Bayesian binomial mixed effects model with subject-wise random intercept. Adjustment for multiple testing was performed using false discovery rate (FDR). For all comparisons, exact p-values are reported. Within woman pairwise comparison of log10 cell counts and aggregation sizes was made using a Bayesian Poisson within subject two group comparison model. All scripts used in this study are available on GitHub at https://github.com/ravel-lab/BV_CELL_SHEDDING.
Results
Comparison of Total Epithelial Cell Counts Between Asymptomatic, Symptomatic and No BV Smears
Cell counts were first considered based on diagnostic group only, without reference to microbiota CST information. The median cell count of samples with no diagnosis of Amsel-BV (noBV, n = 104) was 91/100X field (interquartile range 58–126). This was significantly lower than the median cell count for samples with a diagnosis of symptomatic Amsel-BV (sBV, n = 17), which had median 158/100X field (IQR 124–179), p = 7.2e−09. Additionally, the median cell count of noBV samples was significantly higher than that of samples with a diagnosis of asymptomatic Amsel-BV (aBV, n = 71), which had a median 35/100X field (IQR 19–50), p = 2.4e−16 (Figure 2A). In addition, median cell counts of sBV and aBV samples were significantly different, p < 10e−12.
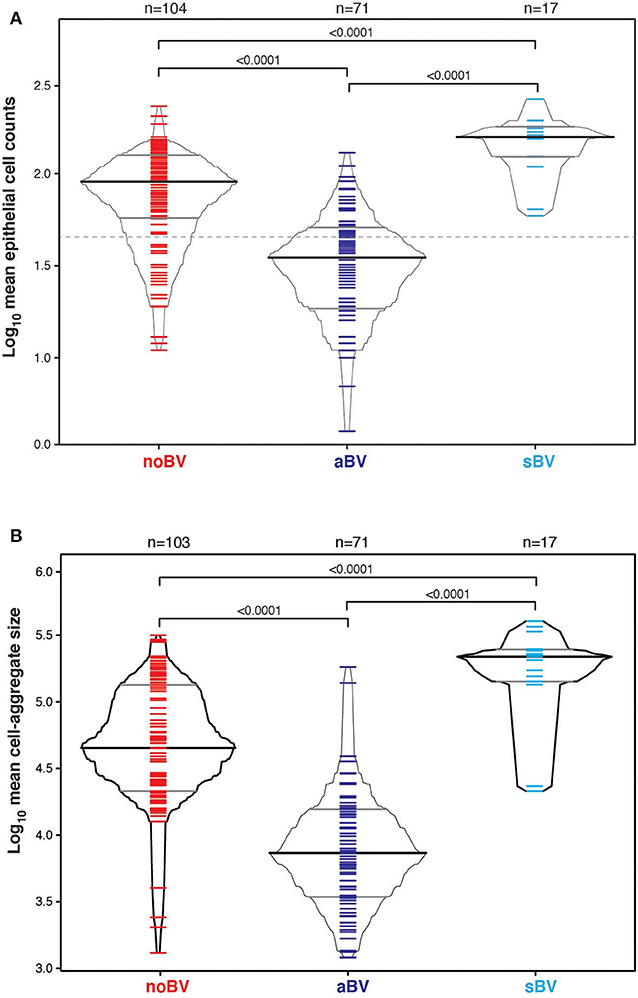
Figure 2. (A) Box-percentile plot of log10 mean epithelial cell counts in different Amsel diagnostic groups. Dash line indicates 50 mean epithelial cells. (B) Box-percentile plot of log10 mean cell-aggregate sizes in different diagnostic groups. The median, 25 and 75th percentiles are marked with line segments across each box. Numbers at the top axis indicate the number of samples in each group. Ticks within percentile-boxes show individual sample values.
Comparison of Cell-Aggregates Size Between Asymptomatic, Symptomatic and No BV Smears
Variability of cell-aggregates on the slides was apparent. Some slides had evenly dispersed cells with aggregates of only a few cells each (Figure 3A), while other slides had multiple aggregations so extensive and dense that they could be distinguished without magnification (Figure 3B). Cell-aggregate size measurements indicated that in noBV samples the median cell-aggregate size (50,000 μm2, IQR 20,000–140,000 μm2) was significantly smaller than that for sBV samples, which had a median cell-aggregate size of 220,000 μm2 (IQR ~140,000–240,000 μm2), p = 1.6e−14. Additionally, the median cell-aggregate size of noBV samples was significantly larger than that of aBV samples, which had a median cell-aggregate size of ~7,000 μm2 (IQR 3,000–14,000 μm2), p < 2.22e−16 (Figure 2B). Lastly, the median cell-aggregate sizes of sBV and aBV samples were significantly different, p = 2.2e−55.
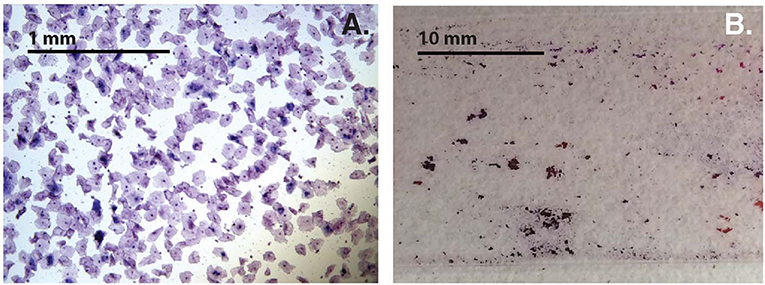
Figure 3. (A) Bright-field micrograph (100X total magnification) showing sample with single cells and small cell-aggregates. (B) Photograph without magnification showing samples with cell-aggregates large enough to see with the naked eye.
Comparison of the Superficial Cell Index Between Asymptomatic, Symptomatic and No BV Smears
The superficial cell index (SCI) was calculated for samples with a mean cell count of ≤50/100X field, corresponding to a total of 78 samples from 54 women. Mean cell count of ≤50/100X field was chosen since it included almost all aBV samples and was well-represented among noBV samples. The median SCI of noBV samples (n = 23) was 0.86 (IQR 0.81–0.94), significantly higher than that of aBV samples (n = 53), which had a median SCI of 0.35 (IQR 0.22–0.51), p = 4.3e−98 (Figure 4). No sBV samples had mean cell count ≤50/100X field.
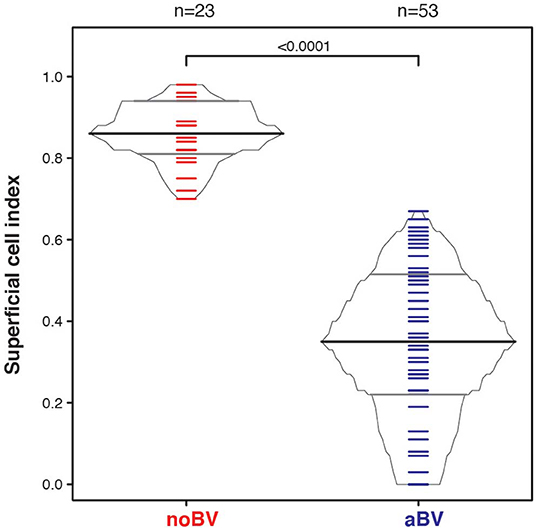
Figure 4. Box-percentile plot of superficial cell index in no Amsel-BV and asymptomatic Amsel-BV samples with a mean epithelial cell counts of <50.
Comparison of Total Epithelial Cell Counts, Cell-Aggregate Sizes and SCI Stratified by Amsel Diagnostic Groups and CSTs
Interestingly, differences between noBV, sBV, and aBV remained when samples were considered based on Amsel diagnostic groups and CSTs. Among samples assigned to CST IV (microbiota comprising a wide range of facultative and strict anaerobic bacteria and a lack of Lactobacillus spp.), the median cell count of noBV samples (64/100X field, IQR 38–112), n = 31, Amsel-BV negative, but molecular-BV positive was significantly lower than that of sBV samples (157/100X field, IQR 157–171, n = 13), p = 9.5e−7, and significantly higher than aBV samples (33/100X field, IQR 18–48), n = 62), p = 0.00011 (Figure 5A). In addition, median cell counts of sBV and aBV samples assigned to CST IV were significantly different, p = 1.3e−24. Similarly, the median cell-aggregate size of CST IV noBV samples (20,000 μm2, IQR 15,000–25,000 μm2) was significantly smaller than that of CST IV sBV samples (25,000 μm2, IQR 15,000–36,000 μm2), p = 5.8e−16, and significantly larger than that of aBV samples (7,000 μm2, IQR 3,600–13,000 μm2), p = 1.06e−9 (Figure 5B). Further, the median cell-aggregate sizes of sBV and aBV samples assigned to CST IV were significantly different, p = 3.15e−45. Further, Lastly, among the 61 samples with mean cell count of ≤50/100X field and that were CST IV, the median SCI of noBV samples (0.94 IQR 0.84–0.95), n = 11) was significantly higher than that of aBV samples (0.34, IQR 0.22–0.51, n = 48), p = 5.3e−73 (Figure 6). There were too few samples with a microbiota dominated by Lactobacillus spp. that were categorized as sBV (n = 4, all CST III) or aBV (n = 4, one CST I and three CST III) to permit any analysis for CSTs other than CST IV.
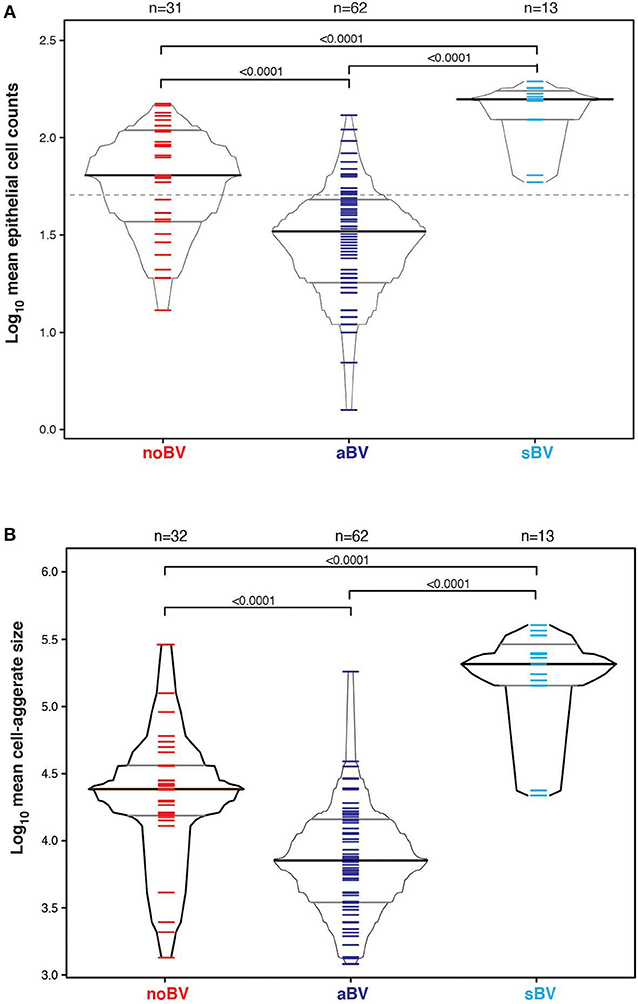
Figure 5. (A) Box-percentile plot of log10 mean epithelial cell counts in different diagnostic groups for only CST IV samples. Dash line indicates 50 mean epithelial cells. (B) Box-percentile plot of log10 mean cell-aggregate sizes in different diagnostic groups for only CST IV samples. The median, 25 and 75th percentiles are marked with line segments across each box. Numbers at the top axis indicate the number of samples in each group. Ticks within percentile-boxes show individual sample values.
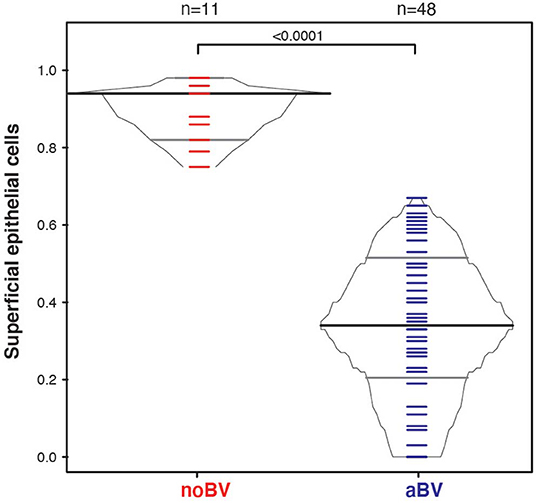
Figure 6. Box-percentile plot of superficial cell index in no Amsel-BV and asymptomatic Amsel-BV samples for only CST IV samples with a mean epithelial cell counts of <50.
Discussion
The equilibrium among the continuous processes of cell proliferation, maturation and shedding on the vaginal epithelium is probably essential to maintaining an effective barrier against pathogenic and non-pathogenic microbes alike. Here, we report an association of symptomatic Amsel-BV (sBV) with evidence of increased cell-shedding, and of asymptomatic Amsel-BV (aBV) with both decreased cell-shedding and increased presence of immature epithelial cells.
These findings raise two intriguing possibilities. Firstly, rather than sBV and aBV being two forms of the condition, we hypothesize that they are sometimes two phases of a single form. Under this model (Figure 7), sBV may be rapidly followed by aBV. Though the dataset presented here is effectively cross-sectional and cannot directly address this possibility (there were only 7 women who provided both sBV and aBV samples, collected several weeks apart). Under this hypothesis, the increased cell-shedding events associated with sBV exhaust the superficial and intermediate epithelial cell layers resulting in reduced cell-shedding, and exposure and loss of immature epithelial cells associated with aBV. This hypothesis is supported by the finding that there are no significant differences in microbiota structure between aBV and sBV [high frequency of CST IV (93.94 and 76.47%, respectively, p = 0.205) and CST III (4.55 and 23.53%, respectively, p = 0.096)] (Supplementary Figure 1), although absolute abundances of bacteria have not been evaluated and could be different between sBV and aBV. We are currently undertaking larger, longitudinal studies to address these possibilities.
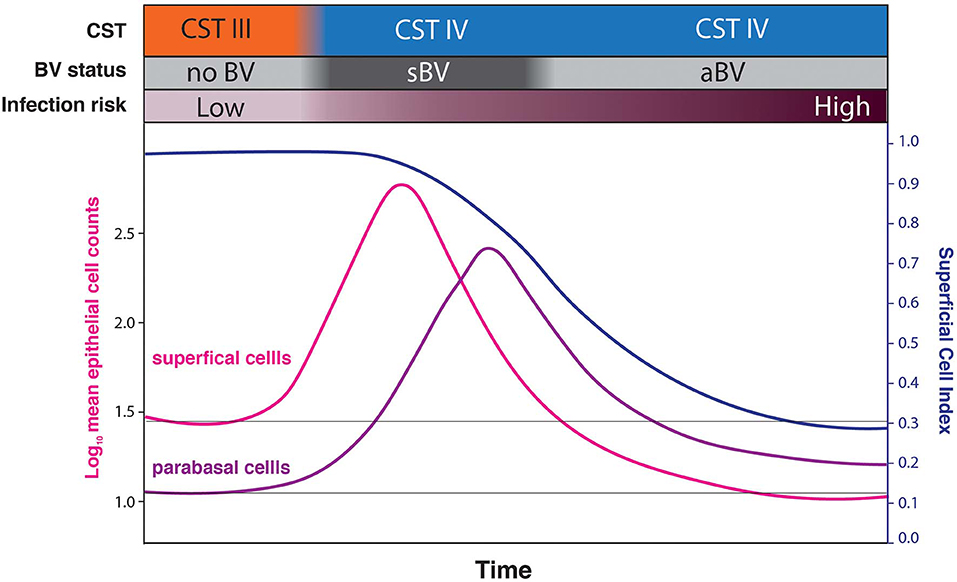
Figure 7. Conceptual model hypothesizing that sBV may be rapidly followed by aBV. Under this hypothesis, the increased cell-shedding events associated with sBV exhaust the superficial and intermediate epithelial cell layers resulting in reduced cell-shedding, and exposure and loss of immature epithelial cells (low SCI) associated with aBV. This progression is hypothesized to be associated with increased risk of infections.
Secondly and more importantly, our findings suggest that aBV might be associated with more severe disruption of the vaginal epithelium than sBV, and hence lead to a greater increase in susceptibility to infections. By analogy, the spermicide nonoxynol-9 (Hillier et al., 2005) is known to cause accelerated cell-shedding (Niruthisard et al., 1991), thinning (Vincent et al., 2011), disruption (Hoffman et al., 2004) and pro-inflammatory changes (Smith-Mccune et al., 2015) on the vaginal epithelium, and was found to increase women's susceptibility to HIV (Wilkinson et al., 2002), as well as susceptibility to HSV-2 (Cone et al., 2006) and HPV (Roberts et al., 2007) in a mouse model. Since aBV is associated with similar physiological effects on the epithelium, we hypothesize it would be associated with an increased risk of infections.
If validated, the hypothesis that aBV does have a particularly disruptive effect on the cervicovaginal epithelium would make it necessary to reevaluate the relative importance of sBV and aBV. While vaginal symptoms have been linked in part to a patient's self-report (Klebanoff et al., 2004) thus symptoms perception, six women in this study presented with sBV and aBV at separate clinical visits over the course of the study (Supplementary Table 1), indicating that women who have had aBV also recognize sBV. However, we hypothesize that based on our findings, there may be relevant biological differences between aBV and sBV. Current CDC guidelines recommend antibiotic treatment only for sBV, not aBV (Workowski and Bolan, 2015). Future work may lead clinicians to reconsider the merits of treating aBV and amending counseling messages for women with aBV to manage their increased susceptibility to infection, for example by avoiding unprotected sexual intercourse. However, expanded screening based on high-resolution molecular assays of both the microbiota and the state of the epithelium would need to be implemented at routine gynecological visits, and more importantly, the development of specific therapies to address the epithelial disruption associated with aBV would be needed.
In our study, the median cell count of noBV samples assigned to CST IV (70/100X field, n = 32) was significantly lower than that of other noBV samples (93/100X field, n = 69), p = 0.0384 (Supplementary Figure 2). This is likely because molecular-BV broadly defines BV and is not limited to Amsel-sBV and Amsel-aBV (as evidenced by our data set, in which 31/104 noBV samples were CST IV). Interestingly, it has been noted that the BV-associated increased risk of HIV acquisition is higher in studies using molecular-BV (defined as all forms of CST IV) as the exposure variable, compared to studies using Amsel-BV or Nugent-BV (Mckinnon et al., 2019). A recent meta-analysis showed that Amsel-BV was associated with a 2-fold increase in risk for HIV acquisition (OR: 1.93, 95% CI: 1.45–2.57; Atashili et al., 2008). A subsequent study suggested that molecular-BV was associated with a 4-fold hazard ratios compared to L. crispatus-dominated samples (HR:4.41, 95% CI: 1.17–16.61, p = 0.028; Gosmann et al., 2017). Molecular-BV is associated with proinflammatory cytokines (Gosmann et al., 2017) and activated HIV-target cells (Anahtar et al., 2015) in the cervicovaginal epithelium and thus with increased risk of HIV acquisition. These findings support a higher risk associated with asymptomatic molecular-BV, and suggest mechanisms involving impaired physiological state of the epithelium and increased pro-inflammatory markers. The above epidemiologic studies of BV and risk for HIV suggest that molecular-BV might trend toward higher point estimates for HIV risk than Amsel-BV because molecular-BV includes more women with at-risk aBV states. To our knowledge, no studies have directly assessed the risk of HIV or other STI acquisition in women with molecular-BV vs. sBV; further studies are needed to test our hypothesis.
Data Availability Statement
Raw counts and metadata used in this study are available in Supplementary Table 1.
Ethics Statement
Samples used in this study were archived vaginal smears obtained in accordance with protocols approved by the University of Maryland Baltimore Institutional Review Board.
Author Contributions
DO'H, RB, and JR designed the study. DO'H executed the laboratory part of the study. PG performed the statistical analyses. DO'H, PG, RB, and JR wrote/edited the manuscript.
Funding
This research reported in this publication was supported in part by the National Institute of Allergy and Infectious Diseases of the National Institutes of Health under award numbers U19AI084044, UH2AI083264, R01AI119012, and R01NR015495.
Conflict of Interest
JR is co-founder of LUCA Biologics, a biotechnology company focusing on translating microbiome research into live biotherapeutics drugs for women's health.
The remaining authors declare that the research was conducted in the absence of any commercial or financial relationships that could be construed as a potential conflict of interest.
Acknowledgments
The authors are grateful to the participants and the clinical staff (Dr. Jane Schwebke laboratory) in Birmingham, AL, who contributed and supported the parent study. We acknowledge the support of the Genomic Resource Center (GRC) at the Institute for Genome Sciences at the University of Maryland School of Medicine for their help with the metataxonomic sequencing.
Supplementary Material
The Supplementary Material for this article can be found online at: https://www.frontiersin.org/articles/10.3389/fcimb.2020.00106/full#supplementary-material
References
Amegashie, C. P., Gilbert, N. M., Peipert, J. F., Allsworth, J. E., Lewis, W. G., and Lewis, A. L. (2017). Relationship between nugent score and vaginal epithelial exfoliation. PLoS ONE 12:e0177797. doi: 10.1371/journal.pone.0177797
Amsel, R., Totten, P. A., Spiegel, C. A., Chen, K. C., Eschenbach, D., and Holmes, K. K. (1983). Non-specific vaginitis. Diagnostic criteria and microbial and epidemiologic associations. Am. J. Med. 74, 14–22. doi: 10.1016/0002-9343(83)91112-9
Anahtar, M. N., Byrne, E. H., Doherty, K. E., Bowman, B. A., Yamamoto, H. S., Soumillon, M., et al. (2015). Cervicovaginal bacteria are a major modulator of host inflammatory responses in the female genital tract. Immunity 42, 965–976. doi: 10.1016/j.immuni.2015.04.019
Anderson, D. J., Marathe, J., and Pudney, J. (2014). The structure of the human vaginal stratum corneum and its role in immune defense. Am. J. Reprod. Immunol. 71, 618–623. doi: 10.1111/aji.12230
Atashili, J., Poole, C., Ndumbe, P. M., Adimora, A. A., and Smith, J. S. (2008). Bacterial vaginosis and HIV acquisition: a meta-analysis of published studies. AIDS 22, 1493–1501. doi: 10.1097/QAD.0b013e3283021a37
Brotman, R. M., Bradford, L. L., Conrad, M., Gajer, P., Ault, K., Peralta, L., et al. (2012). Association between Trichomonas vaginalis and vaginal bacterial community composition among reproductive-age women. Sex Transm. Dis. 39, 807–812. doi: 10.1097/OLQ.0b013e3182631c79
Brotman, R. M., Shardell, M. D., Gajer, P., Tracy, J. K., Zenilman, J. M., Ravel, J., et al. (2014). Interplay between the temporal dynamics of the vaginal microbiota and human papillomavirus detection. J. Infect. Dis. 210, 1723–1733. doi: 10.1093/infdis/jiu330
Cherpes, T. L., Meyn, L. A., Krohn, M. A., Lurie, J. G., and Hillier, S. L. (2003). Association between acquisition of herpes simplex virus type 2 in women and bacterial vaginosis. Clin. Infect. Dis. 37, 319–325. doi: 10.1086/375819
Cone, R. A., Hoen, T., Wong, X., Abusuwwa, R., Anderson, D. J., and Moench, T. R. (2006). Vaginal microbicides: detecting toxicities in vivo that paradoxically increase pathogen transmission. BMC Infect. Dis. 6:90. doi: 10.1186/1471-2334-6-90
Edwards, V. L., Smith, S. B., Mccomb, E. J., Tamarelle, J., Ma, B., Humphrys, M. S., et al. (2019). The cervicovaginal microbiota-host interaction modulates Chlamydia trachomatis infection. MBio 10, e01548–e01519. doi: 10.1128/mBio.01548-19
Eschenbach, D. A., Hillier, S., Critchlow, C., Stevens, C., Derouen, T., and Holmes, K. K. (1988). Diagnosis and clinical manifestations of bacterial vaginosis. Am. J. Obstet. Gynecol. 158, 819–828. doi: 10.1016/0002-9378(88)90078-6
Fadrosh, D. W., Ma, B., Gajer, P., Sengamalay, N., Ott, S., Brotman, R. M., et al. (2014). An improved dual-indexing approach for multiplexed 16S rRNA gene sequencing on the Illumina MiSeq platform. Microbiome 2:6. doi: 10.1186/2049-2618-2-6
Fleury, F. J. (1983). The clinical signs and symptoms of Gardnerella-associated vaginosis. Scand J. Infect. Dis. Suppl. 40, 71–72.
Fowler, R. S. (2012). Quantification of normal vaginal constituents using a new wet preparation technique. J. Low Genit. Tract. Dis. 16, 437–441. doi: 10.1097/LGT.0b013e31825a8b08
Gajer, P., Brotman, R. M., Bai, G., Sakamoto, J., Schütte, U. M., Zhong, X., et al. (2012). Temporal dynamics of the human vaginal microbiota. Sci. Transl. Med. 4:132ra152. doi: 10.1126/scitranslmed.3003605
Gosmann, C., Anahtar, M. N., Handley, S. A., Farcasanu, M., Abu-Ali, G., Bowman, B. A., et al. (2017). Lactobacillus-deficient cervicovaginal bacterial communities are associated with increased HIV acquisition in young South African women. Immunity 46, 29–37. doi: 10.1016/j.immuni.2016.12.013
Hardy, L., Jespers, V., Abdellati, S., De Baetselier, I., Mwambarangwe, L., Musengamana, V., et al. (2016). A fruitful alliance: the synergy between Atopobium vaginae and Gardnerella vaginalis in bacterial vaginosis-associated biofilm. Sex Transm. Dis. 92, 487–491. doi: 10.1136/sextrans-2015-052475
Hickey, D. K., Patel, M. V., Fahey, J. V., and Wira, C. R. (2011). Innate and adaptive immunity at mucosal surfaces of the female reproductive tract: stratification and integration of immune protection against the transmission of sexually transmitted infections. J. Reprod. Immunol. 88, 185–194. doi: 10.1016/j.jri.2011.01.005
Hillier, S. L., Moench, T., Shattock, R., Black, R., Reichelderfer, P., and Veronese, F. (2005). In vitro and in vivo: the story of nonoxynol 9. J. Acquir. Immune Defic. Syndr. 39, 1–8. doi: 10.1097/01.qai.0000159671.25950.74
Hoffman, I. F., Taha, T. E., Padian, N. S., Kelly, C. W., Welch, J. D., Martinson, F. E., et al. (2004). Nonoxynol-9 100 mg gel: multi-site safety study from sub-Saharan Africa. AIDS 18, 2191–2195. doi: 10.1097/00002030-200411050-00012
Jespers, V., Kyongo, J., Joseph, S., Hardy, L., Cools, P., Crucitti, T., et al. (2017). A longitudinal analysis of the vaginal microbiota and vaginal immune mediators in women from sub-Saharan Africa. Sci. Rep. 7:11974. doi: 10.1038/s41598-017-12198-6
Klebanoff, M. A., Schwebke, J. R., Zhang, J., Nansel, T. R., Yu, K. F., and Andrews, W. W. (2004). Vulvovaginal symptoms in women with bacterial vaginosis. Obstet. Gynecol. 104, 267–272. doi: 10.1097/01.AOG.0000134783.98382.b0
Marchesi, J. R., and Ravel, J. (2015). The vocabulary of microbiome research: a proposal. Microbiome 3:31. doi: 10.1186/s40168-015-0094-5
Mckinnon, L. R., Achilles, S. L., Bradshaw, C. S., Burgener, A., Crucitti, T., Fredricks, D. N., et al. (2019). The evolving facets of bacterial vaginosis: implications for HIV transmission. AIDS Res. Hum. Retroviruses 35, 219–228. doi: 10.1089/aid.2018.0304
Mirmonsef, P., Gilbert, D., Zariffard, M. R., Hamaker, B. R., Kaur, A., Landay, A. L., et al. (2011). The effects of commensal bacteria on innate immune responses in the female genital tract. Am. J. Reprod. Immunol. 65, 190–195. doi: 10.1111/j.1600-0897.2010.00943.x
Mitra, A., Macintyre, D. A., Marchesi, J. R., Lee, Y. S., Bennett, P. R., and Kyrgiou, M. (2016). The vaginal microbiota, human papillomavirus infection and cervical intraepithelial neoplasia: what do we know and where are we going next? Microbiome 4:58. doi: 10.1186/s40168-016-0203-0
Niruthisard, S., Roddy, R. E., and Chutivongse, S. (1991). The effects of frequent nonoxynol-9 use on the vaginal and cervical mucosa. Sex Transm. Dis. 18, 176–179. doi: 10.1097/00007435-199107000-00010
Nugent, R. P., Krohn, M. A., and Hillier, S. L. (1991). Reliability of diagnosing bacterial vaginosis is improved by a standardized method of gram stain interpretation. J. Clin. Microbiol. 29, 297–301. doi: 10.1128/JCM.29.2.297-301.1991
Nunn, K. L., Wang, Y. Y., Harit, D., Humphrys, M. S., Ma, B., Cone, R., et al. (2015). Enhanced trapping of HIV-1 by human cervicovaginal mucus is associated with Lactobacillus crispatus-dominant microbiota. MBio 6, e01084–e01015. doi: 10.1128/mBio.01084-15
Ravel, J. (2018). “Progress toward an in-depth understanding of the vaginal microbiome,” Keystone Symposium, Role of the Genital Tract Microbiome in Sexual and Reproductive Health Southern Sun Cape Sun. Cape Town, Western Cape South Africa.
Ravel, J., Brotman, R. M., Gajer, P., Ma, B., Nandy, M., Fadrosh, D. W., et al. (2013). Daily temporal dynamics of vaginal microbiota before, during and after episodes of bacterial vaginosis. Microbiome 1:29. doi: 10.1186/2049-2618-1-29
Roberts, J. N., Buck, C. B., Thompson, C. D., Kines, R., Bernardo, M., Choyke, P. L., et al. (2007). Genital transmission of HPV in a mouse model is potentiated by nonoxynol-9 and inhibited by carrageenan. Nat. Med. 13, 857–861. doi: 10.1038/nm1598
Smith-Mccune, K., Chen, J. C., Greenblatt, R. M., Shanmugasundaram, U., Shacklett, B. L., Hilton, J. F., et al. (2015). Unexpected inflammatory effects of intravaginal gels (universal placebo gel and nonoxynol-9) on the upper female reproductive tract: a randomized crossover study. PLoS ONE 10:e0129769. doi: 10.1371/journal.pone.0129769
Stupnicki, R., and Teter, J. (1970). A dynamic approach to the evaluation of oestrogenic effects on the vaginal epithelium in women. J. Reprod. Fertil. 22, 563–567. doi: 10.1530/jrf.0.0220563
Tachedjian, G., Aldunate, M., Bradshaw, C. S., and Cone, R. A. (2017). The role of lactic acid production by probiotic Lactobacillus species in vaginal health. Res. Microbiol. 168, 782–792. doi: 10.1016/j.resmic.2017.04.001
Taha, T. E., Hoover, D. R., Dallabetta, G. A., Kumwenda, N. I., Mtimavalye, L. A., Yang, L. P., et al. (1998). Bacterial vaginosis and disturbances of vaginal flora: association with increased acquisition of HIV. AIDS 12, 1699–1706. doi: 10.1097/00002030-199813000-00019
Thurman, A. R., Kimble, T., Herold, B., Mesquita, P. M., Fichorova, R. N., Dawood, H. Y., et al. (2015). Bacterial vaginosis and subclinical markers of genital tract inflammation and mucosal immunity. AIDS Res. Hum. Retroviruses 31, 1139–1152. doi: 10.1089/aid.2015.0006
Vincent, K. L., Stanberry, L. R., Moench, T. R., Breitkopf, C. R., Loza, M. L., Wei, J., et al. (2011). Optical coherence tomography compared with colposcopy for assessment of vaginal epithelial damage: a randomized controlled trial. Obstet. Gynecol. 118, 1354–1361. doi: 10.1097/AOG.0b013e318238f563
Wiesenfeld, H. C., Hillier, S. L., Krohn, M. A., Landers, D. V., and Sweet, R. L. (2003). Bacterial vaginosis is a strong predictor of Neisseria gonorrhoeae and Chlamydia trachomatis infection. Clin. Infect. Dis. 36, 663–668. doi: 10.1086/367658
Wilkinson, D., Ramjee, G., Tholandi, M., and Rutherford, G. (2002). Nonoxynol-9 for preventing vaginal acquisition of HIV infection by women from men. Cochrane Database Syst. Rev. 2002:CD003936. doi: 10.1002/14651858.CD003936
Workowski, K. A., and Bolan, G. A. (2015). Sexually transmitted diseases treatment guidelines, 2015. MMWR Recomm Rep 64, 1–137.
Yarbrough, V. L., Winkle, S., and Herbst-Kralovetz, M. M. (2015). Antimicrobial peptides in the female reproductive tract: a critical component of the mucosal immune barrier with physiological and clinical implications. Hum. Reprod. Update 21, 353–377. doi: 10.1093/humupd/dmu065
Zárate, G., Santos, V., and Nader-Macias, M. E. (2009). Protective effect of vaginal Lactobacillus paracasei CRL 1289 against urogenital infection produced by Staphylococcus aureus in a mouse animal model. Infect. Dis. Obstet. Gynecol. 2009:48358. doi: 10.1155/2007/48358
Keywords: women's health, gynecology, BV, vaginal microbiome, vaginal microbiota
Citation: O'Hanlon DE, Gajer P, Brotman RM and Ravel J (2020) Asymptomatic Bacterial Vaginosis Is Associated With Depletion of Mature Superficial Cells Shed From the Vaginal Epithelium. Front. Cell. Infect. Microbiol. 10:106. doi: 10.3389/fcimb.2020.00106
Received: 15 October 2019; Accepted: 27 February 2020;
Published: 10 March 2020.
Edited by:
Alexander Swidsinski, Charité Medical University of Berlin, GermanyReviewed by:
Daniel Champlin Propheter, University of Texas Southwestern Medical Center, United StatesWerner Mendling, Deutsches Zentrum Für Infektionen in Gynäkologie und Geburtshilfe, Germany
Copyright © 2020 O'Hanlon, Gajer, Brotman and Ravel. This is an open-access article distributed under the terms of the Creative Commons Attribution License (CC BY). The use, distribution or reproduction in other forums is permitted, provided the original author(s) and the copyright owner(s) are credited and that the original publication in this journal is cited, in accordance with accepted academic practice. No use, distribution or reproduction is permitted which does not comply with these terms.
*Correspondence: Jacques Ravel, jravel@som.umaryland.edu