- 1Africa Health Research Institute, University of KwaZulu-Natal, Durban, South Africa
- 2Department of Paediatrics, Peter Medawar Building for Pathogen Research, Oxford University, Oxford, United Kingdom
- 3HIV Pathogenesis Programme, Doris Duke Medical Research Institute, University of KwaZulu-Natal, Durban, South Africa
- 4Department of Infectious Diseases, Medizinische Klinik IV, Ludwig-Maximilians-University Munich, Munich, Germany
- 5Ragon Institute of Massachusetts General Hospital, Massachusetts Institute of Technology and Harvard University, Cambridge, MA, United States
- 6Department of Virology, Max von Pettenkofer Institute, Ludwig-Maximilians-University Munich, Munich, Germany
- 7Partner Site Munich, German Center for Infection Research, Munich, Germany
- 8Department of Chemistry and Institute for Medical Engineering and Science, Massachusetts Institute of Technology, Cambridge, MA, United States
- 9Broad Institute of MIT and Harvard, Cambridge, MA, United States
- 10Paediatric Department, Kimberley Hospital, Kimberley, South Africa
- 11Department of Otorhinolaryngology, Stanger Hospital, KwaZulu-Natal, South Africa
- 12Max Planck Institute for Infection Biology, Berlin, Germany
- 13Department of Infection and Immunity, University College London, London, United Kingdom
- 14Centre for HIV and STIs, National Institute for Communicable Diseases of the National Health Laboratory Service, Johannesburg, South Africa
- 15Faculty of Health Sciences, University of the Witwatersrand, Johannesburg, South Africa
- 16Center for the AIDS Programme of Research in South Africa, Durban, South Africa
- 17Department of Immunology and Microbiology, University of Copenhagen, Copenhagen, Denmark
Broadly neutralizing antibodies (bnAbs) against HIV-1 are an effective means of preventing transmission. To better understand the mechanisms by which HIV-specific bnAbs naturally develop, we investigated blood and lymphoid tissue in pediatric infection, since potent bnAbs develop with greater frequency in children than adults. As in adults, the frequency of circulating effector T-follicular helper cells (TFH) in HIV infected, treatment naïve children correlates with neutralization breadth. However, major differences between children and adults were also observed both in circulation, and in a small number of tonsil samples. In children, TFH cells are significantly more abundant, both in blood and in lymphoid tissue germinal centers, than in adults. Second, HIV-specific TFH cells are more frequent in pediatric than in adult lymphoid tissue and secrete the signature cytokine IL-21, which HIV-infected adults do not. Third, the enrichment of IL-21-secreting HIV-specific TFH in pediatric lymphoid tissue is accompanied by increased TFH regulation via more abundant regulatory follicular T-cells and HIV-specific CXCR5+ CD8 T-cells compared to adults. The relationship between regulation and neutralization breadth is also observed in the pediatric PBMC samples and correlates with neutralization breadth. Matching neutralization data from lymphoid tissue samples is not available. However, the distinction between infected children and adults in the magnitude, quality and regulation of HIV-specific TFH responses is consistent with the superior ability of children to develop high-frequency, potent bnAbs. These findings suggest the possibility that the optimal timing for next generation vaccine strategies designed to induce high-frequency, potent bnAbs to prevent HIV infection in adults would be in childhood.
Introduction
A protective HIV-1 vaccine is likely to require the generation of high affinity antibodies recognizing most of the circulating HIV strains worldwide (1–4). Broadly neutralizing antibodies (bnAbs) can prevent SIV/SHIV infection in non-human primates (5–9). However, the mechanism by which a vaccine might elicit bnAbs against HIV remains unclear (10–12).
Broadly neutralizing antibodies are observed in approximately 20% of HIV-infected adults (13–15) and often take years to develop (16). By contrast, bnAbs develop in 75–89% of HIV-infected children, are substantially more potent than those observed in adults (17), and develop as early as the first year of life (18). In a direct comparison between infant and adult responses to the same gp120 vaccine, HIV-uninfected children made higher-magnitude antibody responses (19, 20), further suggesting that children are generally better at generating antibodies than adults. As in adults (21), the generation of bnAbs in infected children is related to viral load (17, 18). However, viral load is only weakly correlated with neutralization breadth (17) indicating that other factors play a more important role.
T-follicular helper (TFH) cells within germinal centers (GC) are CD4 T-cells that provide the B- cell help required to produce increasingly high affinity antibodies over the course of an infection, by the process of somatic hypermutation. Many bnAbs identified to date show a high degree of somatic hypermutation (1, 3), suggesting an efficient GC response mediated by TFH cells (4, 22, 23). However, as B-cell affinity maturation relies on competition between B-cell clones, the help provided by TFH needs to be a limiting resource for effective selection to occur (24, 25). Thus, regulation of TFH in the GC by T-follicular regulatory CD4 cells (TFR) is another critical determinant of antibody breadth and potency in HIV infection. TFR are derived from thymic TREG precursors, express FoxP3, CD25, and low levels of CD127, and subsequently acquire TFH markers (PD1, CXCR5 and Bcl-6) (26–28). These TFR cells contribute to the regulation and proliferation of TFH and GC B-cells (29, 30), and skewing of TFR/TFH ratio leads to unchecked expansion of TFH and an ineffective humoral immune response (31, 32). Growing evidence from murine models show that TFR cells are necessary to ensure the quality of the antibody response (33, 34). CXCR5+ve CD8 T-cells in secondary lymphoid tissue and in circulation can also regulate the TFH response (35–37). These CXCR5+ve CD8 T-cells reduce GC responses through perforin-dependent lysis of GC TFH cells and prevent autoantibody development in murine and SIV models (38–40).
Understanding the immune environment in which high-affinity antibodies develop is a critical step toward new vaccination strategies. We therefore studied the TFH response and its regulatory counterparts in HIV-infected children and adults to understand the immune conditions that do and do not, respectively, support HIV bnAb development.
Materials and Methods
Study Participants
Peripheral blood mononuclear cells (PBMC) of 38 vertically HIV-1 C clade-infected ART-naïve children with matched neutralization data (17) were studied. Additional pediatric and adult samples were obtained from clinics in Durban, South Africa (Ithembalabantu Clinic and Prince Mshiyeni Hospital). Tonsil specimens were obtained from medically indicated tonsillectomies carried out in KwaZulu-Natal (Stanger Hospital in Stanger, Addington Hospital in Durban). All tonsillectomies in this study were conducted for either chronic obstructive symptoms (e.g., snoring) due to tonsillar hypertrophy and/or recurrent tonsillitis. All procedures were elective and, if conducted for recurrent tonsillitis, were carried out after infection and associated inflammation had resolved, as determined by the clinician and typically 6 weeks or more after the last episode. The clinical characteristics of the study cohort are shown in Table S1. Adult participants on whom neutralization assays were undertaken are from the South African CAPRISA 002 cohort, as previously described (41). Viral load measurements were performed as described previously (17). Informed consent was obtained from all adult study participants or from the caregivers of pediatric participants where appropriate. Additionally, assent to participate in the study was given directly by children from the age of six and above. Studies were approved by the University of the Free State Ethics Committee, Bloemfontein; Biomedical Research Ethics Committee, University of KwaZulu-Natal, Durban; and Research Ethics Committee, University of Oxford.
Sample Processing–PBMC and Tonsils
PBMCs were isolated by Ficoll density gradient centrifugation and stored in liquid nitrogen until use. Tonsil mononuclear cells (TMCs) were obtained by mechanical and enzymatic disaggregation using the gentleMACS system (Miltenyi). Mononuclear cells were then isolated by Ficoll density gradient centrifugation and used directly or preserved as above.
Virus Neutralization Assays
The ability of plasma from infected children and adults to neutralize HIV was measured as described before (17).
Flow Cytometry and ICS Assays
PBMCs and TMCs were stained with fluorescent monoclonal antibodies against markers previously associated with TFH cells Table S2. Briefly, cells were thawed and rested in R10 medium for 3 h at 37°C in 5% CO2 and either directly stained with the phenotypic panel as described below or used for Intracellular Cytokine Staining (ICS) by stimulating with SEB at 1 μg/ml or with pools of overlapping 18-mer HIV peptides (Gag and Env at 2 μg/ml for each peptide) in the presence of anti-CD28 and anti-CD49 at 1 μg/ml (BD Biosciences). After 1 h of incubation at 37°C, Brefeldin A and Monensin (5 μg/ml; BD biosciences) were added and the cells were incubated overnight (14 h), washed and stained in the dark for 20 min with antibody cocktail and live dead stain (Fixable Blue, Thermo) and fixed. ICS was performed by standard methods (42), using fix/perm solution (BD), 20% Goat serum for Fc-receptor blockade and antibodies listed as above. Rainbow beads were run at every experiment to ensure interexperimental consistency. Flow cytometry acquisition was performed on a BD LSRFortessa within 5 h of staining and analyzed using FlowJo version 9.9.5.
Multi-Color Immunofluorescence Staining
Tissue samples were fixed in formalin, embedded in paraffin, and sectioned. These specimens were incubated with antibodies: anti-CD4 (clone: CM153A; Biocare), CD8 (clone: ab85792; Abcam), CXCR5 (clone: MAB190; R&D) and Foxp3 (clone: ab22510; Abcam) followed by incubation with secondary antibody using a SuperPicTure™ Polymer Detection Kit (Invitrogen) and an Opal™ 3-Plex Kit (Fluorescein, Cyanine3, and Cyanine5). The samples were mounted with ProLongTM Gold Antifade mountant containing DAPI (Invitrogen).
Statistical Analyses
Statistical analyses were undertaken using Prism GraphPad Software version 7.0; for comparisons between two groups Mann–Whitney–Wilcoxon test was applied and for comparisons >2 groups Kruskal-Wallis test with Benjamini, Krieger and Yekutieli's correction for multiple comparisons. All correlations were performed using the Spearman rank method with exact permutation P-values calculated. All P-values are two-sided with a cut off of p > 0.05. For Figure 5, the Spice (Simplified Presentation of Incredibly Complex Evaluations) package was used to calculate permutation P-values between Spice charts (43).
Results
Circulating Effector Memory TFH Cells Are Abundant in HIV-Infected Children and Correlate With Neutralization Breadth
Neutralizing antibody breadth was previously determined in plasma samples from 85 ART-naïve, HIV-infected children (17). Although 75% of pediatric samples were broadly neutralizing (i.e., neutralized >50% of the virus panel tested), neutralization breadth ranged from 0 to 100%. To investigate the T-cell immunological parameters underlying this variability, we first examined, in the same pediatric cohort (Table S1), the relationship between neutralization breadth and the frequency of circulating TFH cells in ART-naïve children (defined as CD3+CD4+CD45RA−CXCR5+CXCR3−PD1+ lymphocytes) (Figure 1A), cells which have previously been linked with HIV neutralization breadth in adults (44, 45). In these children also, we now observed a clear positive correlation between neutralization breadth and circulating “effector TFH” frequency (CCR7−; r = 0.44, p = 0.007), but no association with “central TFH” frequency (CCR7+; Figure 1B), consistent with previous studies showing that only circulating TFH cells expressing an effector phenotype are functional active (46). Interestingly, there is a significant correlation between the frequency of central TFH and viral load (r = 0.5, p = 0.003), but not with the key effector TFH subset (Figure S1A). Additionally, PD1 expression on total CD4 and CD8 T-cells, which is linked to viral load and immune activation in HIV infection, is inversely correlated with neutralization breadth (Figure S1B). Taken together, these data suggest the observed association between the frequency of circulating effector TFH and the development of broadly neutralizing antibodies is not driven by viremia or immune activation. Finally, consistent with the importance of the effector TFH subset, circulating effector memory TFH but not central memory TFH were substantially more abundant in HIV-infected children compared to HIV-infected adults (effector memory TFH median 4.9 vs. 2.7% of CD4 T-cells, p = 0.004; Figure 1C).
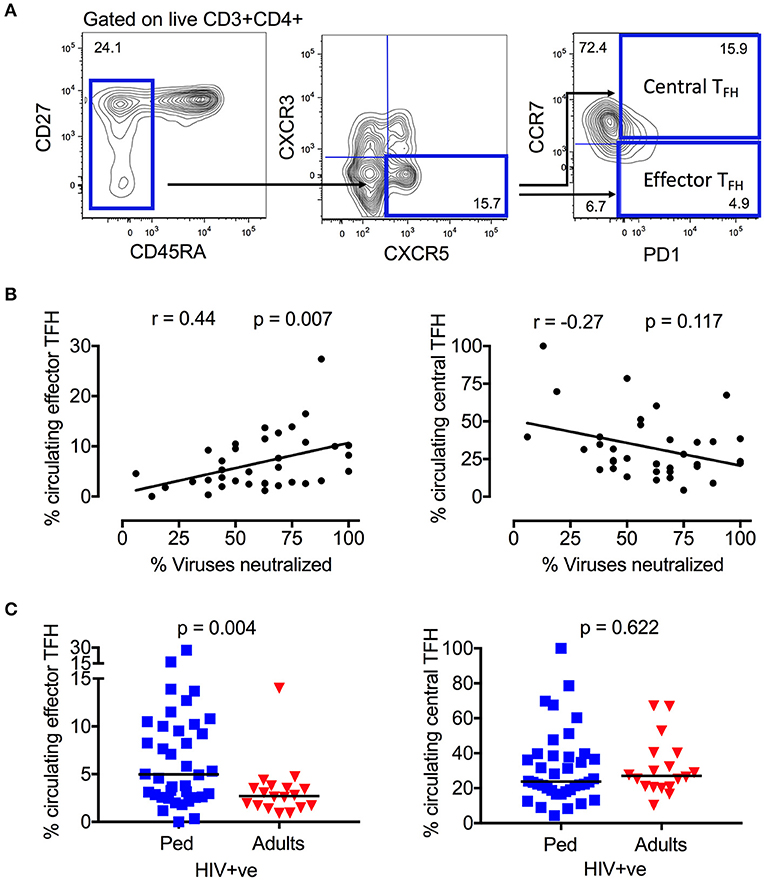
Figure 1. Circulating effector memory TFH cells correlate with neutralization breadth in HIV infected children. (A) Gating strategy of CD4+CD45RA−CXCR5+CXCR3−PD1+ TFH cells in peripheral blood. The majority of circulating TFH cells express a resting central memory phenotype (CCR7+) in contrast to the effector memory (CCR7–) phenotype. (B) Positive correlation between circulating effector TFH cells and neutralization breadth (r = 0.44, p = 0.007; left) and inverse correlation between circulating central TFH cells and neutralization breadth in HIV infected children (n = 36) (r = −0.27, p = 0.117; right). Calculations were made by Spearman's rank correlation test. (C) Increased frequency of circulating effector TFH cells in HIV-infected, ART-naïve children (blue squares; n = 38) compared to infected adults (red triangles; n = 18) (p = 0.004; left). No significant differences in frequency of circulating central TFH between the groups (n.s., right). Comparisons between >2 groups were calculated using Kruskal-Wallis test and corrected for multiple comparisons. In scatter plots medians are shown.
TFH Are More Abundant in Lymphoid Tissue of Children Than Adults
In order to investigate TFH cells within lymphoid tissue, where their function is primarily exerted, we next studied tonsils isolated from HIV infected adults (n = 6) and children (n = 4, age median 11.1, see methods for cohort description). The six adults studied comprised 3 receiving ART and 3 not receiving ART (median viral load 2452 HIV RNA cp/ml), whilst all 4 children were receiving ART (median viral load 54 HIV RNA cp/ml) (Table S1) due to lack of sample availability of an ART-naïve pediatric control group. Plasma neutralization data of these individuals is not available, since the neutralization assays cannot be performed with detectable drug plasma levels. Hence, no direct associations to neutralization breadth can be drawn. In contrast to TFH in circulation, lymphoid TFH express the canonical transcriptional factor Bcl-6, which was used to confirm the identity of the subsets studied (Figure 2A). We observed that tonsil TFH were increased in frequency in HIV infected children compared to adults, although this does not reach statistical significance (15.6 vs. 7.0%, p = 0.17; Figure 2B). TFH cells up-regulate CXCR5 and PD1, and down-regulate CCR7 (47, 48) during migration from the T cell zone into the GC. When looking at “germinal center” TFH (GC TFH; % CCR7− of tonsil TFH) we found that they are enriched in HIV infected children compared to adults (Median 71.2 vs. 41.4%, p = 0.01; Figure 2C). Thus, HIV infected children have significantly higher levels of both circulating effector TFH in the blood and GC TFH from oral mucosal lymphoid tissue compared to infected adults, supporting the hypothesis that these cells contribute to HIV bnAb development.
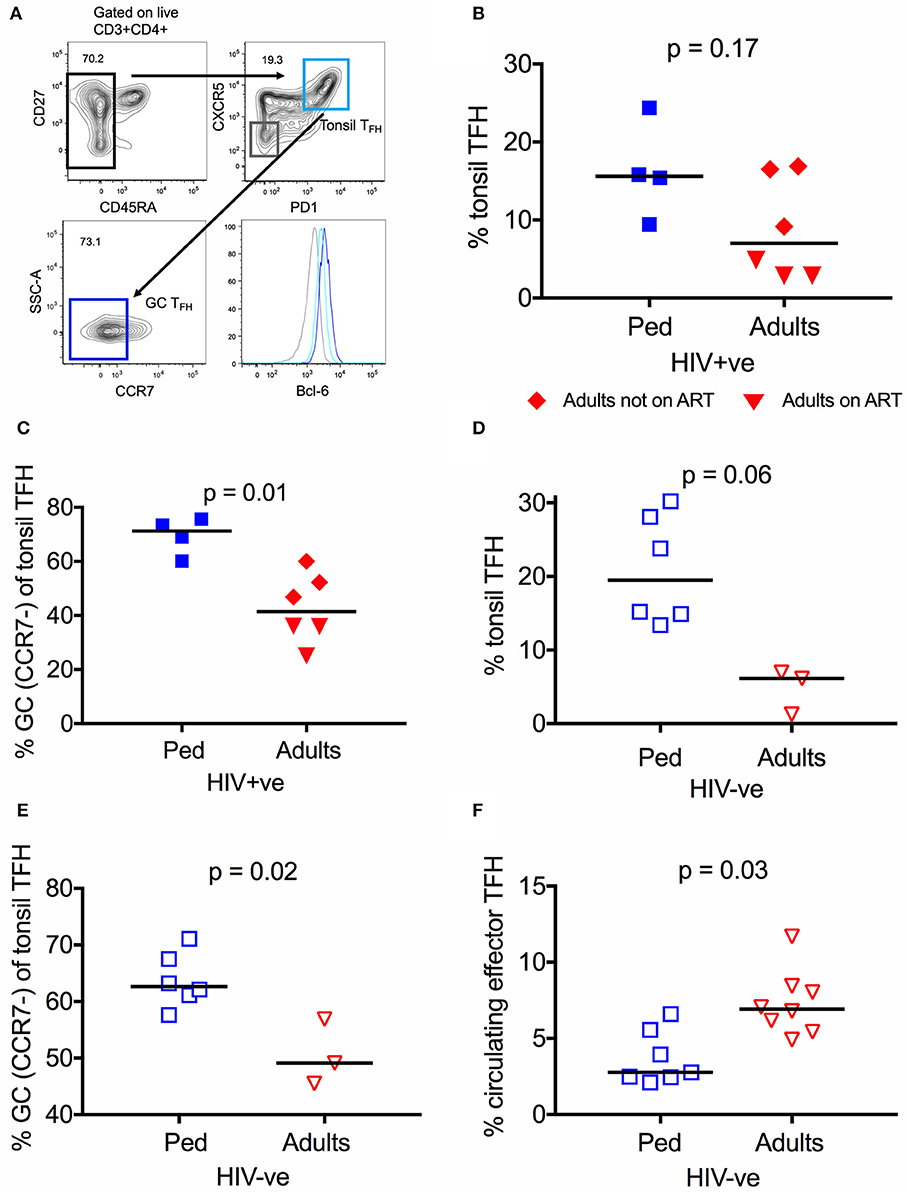
Figure 2. TFH are more abundant in lymphoid tissue of children than adults. (A) Gating strategy of tonsil TFH (CD4+CD45RA−CXCR5+PD1high; light blue) and GC-TFH cells (%CCR7− of total tonsil TFH; dark blue) in secondary lymphoid tissue. Levels of Bcl-6 expression of the different subsets are expressed in MFI (median fluorescent intensity). (B) No significant difference in the frequency of tonsil TFH in secondary lymphoid tissue of infected children (blue squares; n = 4) compared to infected adults (n.s.; Kruskal-Wallis test). Red triangles: Adults on ART (n = 3), red diamonds: Adults not on ART (n = 3). (C) Same as in (B) but showing a significantly increased frequency of GC-TFH cells (% CCR7− of tonsil TFH) (p = 0.01; Kruskal-Wallis test) in infected children. (D) Increased frequency of tonsil TFH (p = 0.06; Kruskal-Wallis test) in secondary lymphoid tissue of uninfected children (open blue squares; n = 6) compared to uninfected adults (open red triangles; n = 3). (E) Same as (D) but showing the increased frequency of GC-TFH cells in uninfected children (p = 0.02; Kruskal-Wallis test) compared to uninfected adults. (F) Increased frequency of circulating effector TFH in HIV uninfected adults (open red triangles; n = 8) compared to uninfected children (open blue squares; n = 7) (p = 0.03; Kruskal-Wallis test). In scatter plots medians are shown.
To investigate whether these differences are observed also in HIV uninfected individuals, we next examined tonsillar lymphoid tissue in uninfected adults (n = 3) and children (n = 6). As in the HIV infected counterparts, the frequency of TFH is higher in uninfected children compared to adults (Figures 2D,E), particularly for GC TFH (62.7 vs. 49.1%, p = 0.02; Figure 2E). Unexpectedly, however, circulating effector TFH cells were found to be significantly less frequent in uninfected children (n = 7) vs. adults (n = 8; p = 0.03; Figure 2F). Taken together, these data indicate that children possess more abundant GC TFH to support the generation of neutralization breadth.
High-Frequency IL-21 Production by HIV-Specific GC-TFH Cells in Children but Not Adults
Germinal center (GC) TFH cells promote B-cell proliferation, somatic hypermutation, and affinity maturation through IL-21 production, and this function is therefore critical for the generation of bnAbs (49–51). In response to stimulation with peptide pools spanning Gag and Env, “Th2”-GC-TFH cells (by far the dominant TFH subset present in tonsil homogenate Figure S2A) from HIV infected children made strong IL-21 responses (median 6.3%), whereas “Th2”-GC-TFH cells from HIV-infected adults produced little or no IL-21 (Figures 3A,B). By contrast, the reverse was the case with respect to IFN-γ production, which was detectable in adults (median 0.3%) but absent in children (Figure 3C). These differences between children and adults were both statistically significant (p = 0.02 and p = 0.04, respectively) despite the small sample size, and the same trend was observed for total cytokine producing CD4 cells (Figures S2B,C). In response to stimulation by SEB, however, there was no difference in the responses made by “Th2”-GC-TFH cells from children or adults, irrespective of HIV infection (Figures 3D,E), indicating that the differences observed between children and adults were among HIV-specific GC-TFH cells. Importantly, the bias in IL-21 and INF-γ production between adults and children observed in HIV-specific GC-TFH was not seen in circulating “Th2”-TFH cells (Figure 3F, Figure S2D). Consistent with this, we found no correlation between HIV-specific IL-21 production by circulating “Th2”-TFH in ART-naïve children and neutralization breadth (Figure 3G). Thus, specifically in the germinal centers of lymphoid tissue, both a greater abundance of TFH and a higher frequency of HIV-specific TFH that secrete the key functional cytokine IL-21 were observed in HIV-infected children compared to adults.
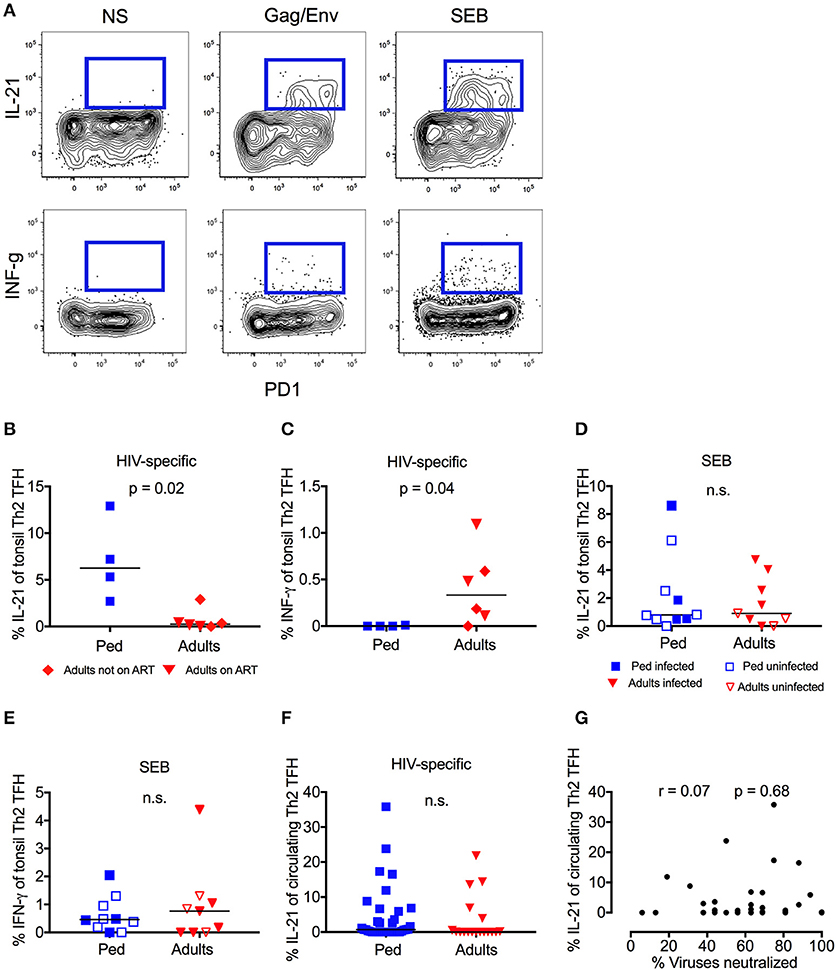
Figure 3. High-frequency IL-21 production in HIV-specific GC-TFH Cells in children but not adults. (A) Representative flow cytometry plot of one pediatric HIV-infected subject. Gated on CD4+CCR6−CXCR3−CXCR5+ “Th2” TFH cells. IL-21 (top row) and INF-γ (bottom row) secretion of PD1+ve cells is shown in response to HIV-peptide pools (middle) or SEB (right). On the left unstimulated control. (B) Gated on tonsil “Th2” GC-TFH cells as follows: CD4+CCR6−CXCR3−CXCR5+PD1+. Increased IL-21 secretion (p = 0.02) and (C) decreased INF-γ secretion (p = 0.04) in HIV infected children (blue squares; n = 4) compared to infected adults in response to Gag/Env. Red triangles: Adults on ART (n = 3), red diamonds: Adults not on ART (n = 3). Mann-Whitneys test was used for comparisons between 2 groups. (D) No significant differences of IL-21 or INF-γ (E) production in response to SEB in children (blue; n = 10) compared to adults (red; n = 9) irrespective of HIV infection and ART status (n.s.; Mann-Whitneys-test). Closed symbols: HIV infected; open symbols: HIV uninfected. (F) No significant differences in Gag/Env specific IL-21 secretion of circulating “Th2”-TFH cells between ART-naïve infected children (blue squares; n = 38) and ART-naïve infected adults (red triangles; n = 18) (n.s.; Kruskal-Wallis test). (G) Lack of correlation between HIV-specific (Gag/Env pool) IL-21 production of circulating “Th2”-TFH and neutralization breadth in HIV-infected, ART-naïve children (n = 36). Calculations were made by Spearman's rank correlation test. In scatter plots medians are shown.
Tonsil Follicular Regulatory T Cells (TFR) Are Increased in HIV-Infected Children
TFH cells are needed to mediate an optimal antibody response, but excessive TFH activity leads to the expansion of low-affinity and auto reactive B cells (24, 25, 52). T-follicular regulatory cells (TFR), a CD4 T cell subset that regulates germinal center TFH responses, are thus essential for the generation of high affinity antibodies (26, 27, 33). Therefore, we next examined the relationship between TFH and TFR within HIV infected tonsils, defining TFR as CD4+CXCR5+CD25+CD127low cells that are enriched for the TREG transcriptional factor FoxP3 (30) (Figure 4A), and having found that CD25 expression is not affect by HIV infection (Figure S3A). In HIV infected children, the TFR frequency was 2.7-fold higher than that observed in HIV-infected adults (median 5.1 vs. 1.9%; p = 0.001), particularly those adults on ART (Figure 4B). This profound enrichment of TFR results in a significantly higher TFR/TFH ratio in HIV infected children, despite the increased frequency of TFH in this group (p = 0.01; Figure 4C). Immunofluorescent staining of a tonsil sample from which histological sections were available confirms the existence of CXCR5+ FoxP3+ CD4 T-cells in proximity to the GC (Figure 4D). In contrast to TFH, however, this trend is not observed in HIV uninfected counterparts, in whom TFR frequency and TFR/TFH ratio are not significantly different (Figures S3B,C). Overall, these data suggest that the enriched and functionally superior GC TFH in HIV-infected children are also better regulated than in HIV-infected adults, which is likely to support affinity maturation and the development of bnAbs.
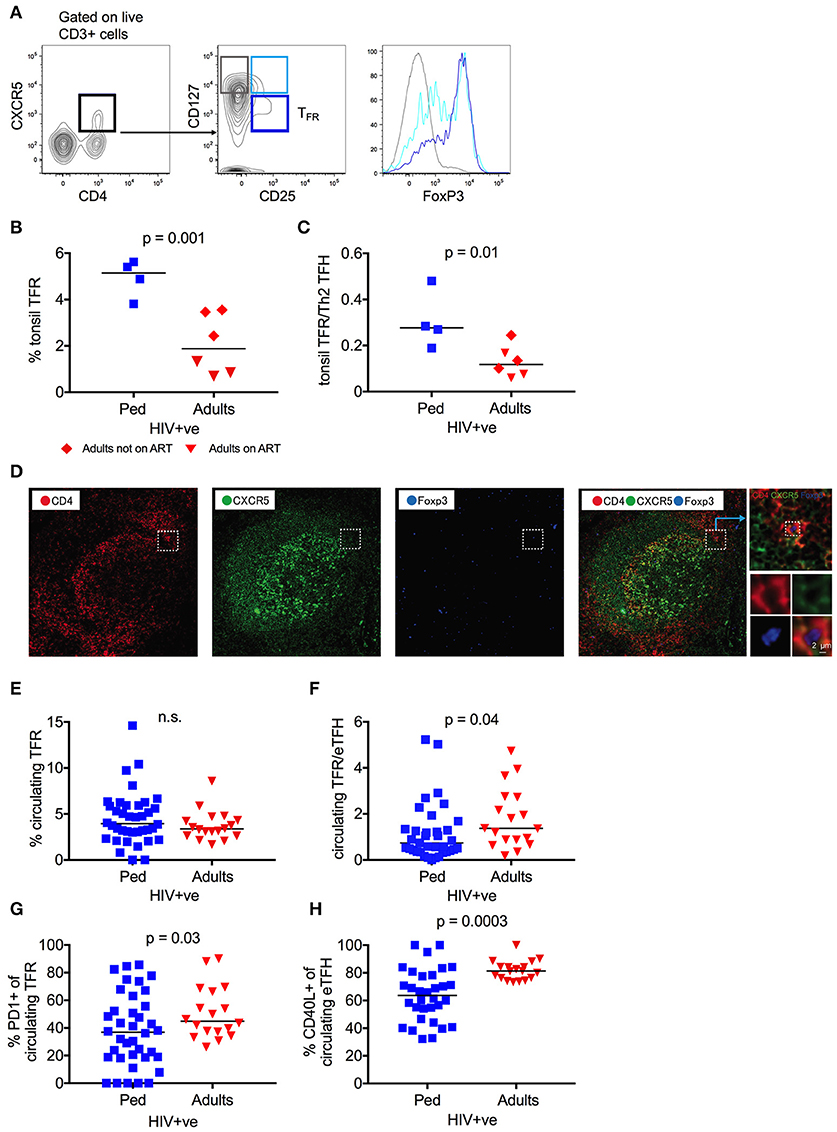
Figure 4. Tonsil follicular regulatory T cells (TFR) are increased in HIV infected children. (A) CD4+CXCR5+CD25+CD127low follicular regulatory T cells (TFR) (dark blue) show the highest FoxP3 expression as expressed in MFI (median fluorescent intensity) when compared to CD4+CXCR5+CD25+CD127high (light blue) and CD4+CXCR5+CD25−CD127high (gray) non-regulatory follicular T cells. (B) Increased frequency of tonsil TFR in HIV infected children (blue squares; n = 4) compared to infected adults (red triangles: Adults on ART, n = 3; red diamonds: Adults not on ART, n = 3) (p = 0.001; Kruskal-Wallis test). (C) Same as B but showing the increased ratio of tonsil TFR to tonsil “Th2” TFH cells (gated on CD4+CCR6−CXCR3−CXCR5+PD1+) in HIV infected children compared to infected adults (p = 0.01; Kruskal-Wallis test). (D). Immunofluorescent staining of a tonsil sample from which histological sections were available confirms the existence of CXCR5+ FoxP3+ CD4 T-cells in proximity to the GC. CD4: red; CXCR5: green; FoxP3: blue. (E) No differences in frequency of circulating TFR between infected, ART-naïve children (blue squares; n = 38) and ART-naïve adults (red triangles; n = 18) (n.s.; Kruskal-Wallis test). (F) Same as F but showing an increased ratio of circulating TFR to circulating effector TFH (gated on CD4+CD45RA−CXCR5+CXCR3−CCR7−PD1+) in HIV infected adults compared to infected children (p = 0.04; Kruskal-Wallis test). (G) Increased expression of PD1 on circulating TFR of HIV infected adults compared to HIV infected children (p = 0.03; Kruskal-Wallis test). (H) Decreased expression of CD40L on circulating effector TFH cells in children when compared to adults (p = 0.0003; Kruskal-Wallis test). In scatterplots, medians are shown.
Interestingly, no differences between HIV-infected children and adults were observed in circulating TFR frequency, and the ratio of circulating TFR to effector TFH cells was significantly lower in HIV-infected, ART-naïve children compared to adults (p = 0.04; Figures 4E,F and Figures S3D,E). However, circulating TFR from HIV-infected adults express significantly higher levels of the exhaustion marker PD1, known to impair TFR function (p = 0.03; Figure 4G) (53). Furthermore, circulating pediatric TFH expressed lower levels of the surface marker CD40L (p = 0.0003; Figure 4H), which implies tighter regulation through this key TFH functional molecule. Thus, although the frequency of circulating TFR is lower in HIV-infected children, in contrast to the frequency of GC TFR, the phenotypic differences observed between children and adults are consistent with increased regulation of the TFH response in HIV infected children in circulation as well as in lymphoid tissue. This is corroborated by a positive trend between the frequency of circulating TFR and neutralization breadth in HIV infected children and negative trend in the same group between PD1 expression and breadth (Figures S3F,G). Again, these data support the importance of TFH regulation in the preferential development of bnAbs in HIV-infected children.
Follicular CD8 T-Cells Correlate With Neutralization Breadth in Children
To further explore the contribution of TFH regulation to bnAb development, we next studied CXCR5+ve CD8 T-cells, a subset also known to limit GC TFH cell activity (35, 36, 38, 40, 54). The overall frequency of CXCR5+ve CD8 T-cells in tonsils did not differ between children and adults, in either HIV infected or uninfected individuals (Figure 5A). However, HIV-specific CXCR5+ve CD8 T-cells that produced IFN-γ in response to stimulation with Gag/ Env peptide pools were of considerably higher magnitude (median 3.7 vs. 0.3%) in infected children compared to adults, although this did not reach statistical significance (p = 0.17; Figure 5B). Immunofluorescent staining of available histological sections confirmed the presence of CXCR5+ve CD8 T-cells in association with CD4 T-cells within the GC (Figure 5C). In circulation, we observed a significant positive correlation between the frequency of CXCR5+ve CD8 T-cells and neutralization breadth in HIV infected, ART-naïve children (r = 0.47, p = 0.008; Figure 5D). As with circulating TFR, the frequency of total CXCR5+ve CD8 T-cells was similar in infected adults and children, as was the frequency of HIV-specific CXCR5+ve CD8 T-cells (Figures 5E,F). However, CXCR5+ve CD8 T-cells were significantly more polyfunctional in children with high neutralization breadth compared to those with low neutralization breadth (p = 0.04; Figure 5G) (functions tested being IL-2, IL-4, IL-17 and IFN-γ production in response to HIV Gag/ Env). These differences in functionality were not observed in CXCR5-ve CD8+ T-cells and no significant differences were seen between adults and children as a whole. Together, these data support a role for CXCR5+ve CD8 T-cells, in addition to TFR in the regulation of TFH in HIV-infected children.
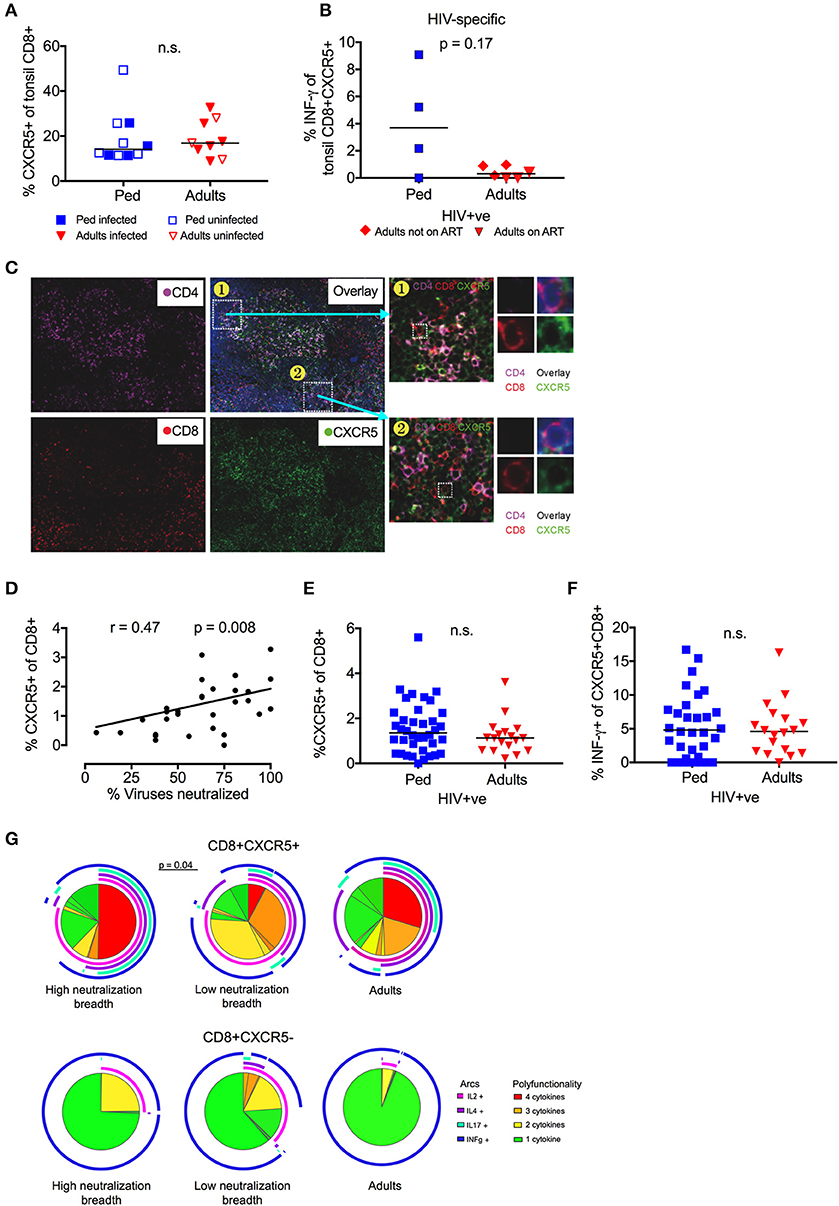
Figure 5. Follicular CD8 T-cells correlate with neutralization breadth in HIV infected children. (A) No significant difference in frequency of tonsil CXCR5+ve CD8 T-cells between children (blue squares; n = 10) and adults (red triangles; n = 9) irrespective of HIV infection (closed symbols: HIV infected; open symbols: HIV uninfected) (n.s; Mann-Whitney test). (B) Increased secretion of INF-γ of tonsil CXCR5+ve CD8 T-cells in response to Gag/Env pool in HIV-infected pediatric study participants (n = 4) compared to infected adults (red triangles: Adults on ART, n = 3; red diamonds: Adults not on ART, n = 3) (p = 0.17; Mann-Whitneys test). (C) Immunofluorescent staining of available histological sections shows the presence of CXCR5+ve CD8 T-cells in association with the GC. CD4: violet; CD8: red; CXCR5: green. (D) Frequency of circulating CXCR5+ve CD8 T cells correlates with neutralization breadth within the cohort of HIV infected children (p = 0.008, r = 0.47; Spearman's rank test) (n = 36). (E) No significant differences in the frequency of circulating CXCR5+ve CD8 T-cells or HIV-specific (Gag/Env pool) INF-γ production (F) between HIV-infected, ART-naïve children (blue squares; n = 38) and adults (red triangles; n = 18). Comparisons between 2 groups were performed using Mann-Whitneys test and between >2 groups using Kruskal-Wallis-test and corrected for multiple comparisons. (G) Circulating CXCR5+ve CD8 T-cells (left) show a more polyfunctional cytokine response to HIV Gag/Env compared to CXCR5–ve CD8 T-cells (right). Circulating CXCR5+ve CD8 T-cells of children with high neutralization breadth show a stronger polyfunctional profile than those of children with low neutralization breadth (p = 0.04) (Monte Carlo simulation partial permutation test). Cytokine-negative cells are excluded from the pie chart. (color coding pie chart: red: 4 cytokines, orange: 3 cytokines, yellow: 2 cytokines, green: 1 cytokine; color coding arcs: pink: IL-2, violet: IL-4, turquoise: IL-17, blue: INF-γ).
Discussion
Understanding the immunological conditions in which bnAbs against HIV-1 are generated will help to optimize and target future vaccine strategies. Although most HIV-infected children produce broad and potent neutralizing antibodies against HIV-1 (17, 18), the relationship between the TFH response, which is critical for affinity maturation, and neutralization breadth has not been investigated to date in children. The need to examine lymphoid tissue in particular was made apparent by the current study, as many of the key differences in TFH activity between children and adults were only evident within the tonsil. Circulating effector TFH were more frequent in infected, ART-naïve children than adults and correlate well with neutralization breadth; and GC TFH in tonsil were more than double the frequency in children. However, differences between children and adults in antigen specific TFH were only observed in lymphoid tissue. HIV-specific “Th2”-GC TFH were more abundant in children and produced IL-21 and not IFN-y, whereas adult HIV-GC TFH cells secreted IFN-y not IL-21. Additionally, these studies of lymphoid tissue underline the key role of TFH regulation in the development of HIV bnAbs in children, as TFR cells were present at substantially higher frequencies than in infected adults, giving rise to significantly higher TFR/TFH ratios in HIV-infected but not in uninfected children. Furthermore, HIV-specific CXCR5+ CD8 T-cells, described to have regulatory function in the GC (36, 39, 54), were more frequent in pediatric lymphoid tissue; and total circulating CXCR5+ve “follicular” CD8 T-cells correlated with neutralization breadth and were more polyfunctional in children with high neutralization breadth. Together these data are consistent with the notion that increased HIV-specific TFH activity and increased regulation within GC both contribute to the high frequency of potent, bnAbs observed in HIV-infected children.
In response to stimulation by HIV peptides, peripheral blood TFH cells produced very low levels of the canonical TFH cytokine, IL-21 (55), and no correlation with antibody breadth or differences between children and adults were observed. These children were not on ART at the time of sampling, which reduces circulating HIV-specific T-cells (56). Thus, in children at least, limited information on HIV-specific TFH can be obtained from the blood. This contrasted with the striking HIV-specific IL-21 Th2 TFH responses in HIV infected pediatric tonsils and their almost complete absence in the tonsils of the adults tested. TFH provide help to B-cells in an antigen-specific manner (57), and hence the presence of HIV-specific TFH secreting the appropriate TFH cytokines is likely to be critical to effective affinity maturation of HIV antibodies. The GC HIV-specific TFH responses in adults were both less abundant and functionally distinct in secreting IFN-γ rather than IL-21. The Th2 TFH bias observed here in HIV-infected children, compared with adults, and the more frequent development of bnAbs in children is consistent with other recent findings. In HIV-infected adults, IFN-γ secreting TFH did not support antibody class switching as effectively as Th2 biased TFH secreting Th2 cytokines (58). Furthermore, in the SIV model, Th2-skewed TFH correlated with the development of broadly neutralizing antibodies, whereas IFN-γ producing Th1 TFH did not (59). Other studies in both humans and non-human primates point to the overall Th1 polarization of the GC TFH response in chronic infection that impairs optimal B-cell activity (60, 61). Together, these data support the hypothesis that the HIV-specific IL-21-producing TFH within the lymphoid tissue of HIV-infected children play a critical role in the generation of the high-frequency, potent bnAbs that are characteristically observed in children. The mechanism by which these differences arise remains unclear, especially since responses to SEB did not differ between adults and children. It is possible that the increased frequency of TFH observed in children reflects recent exposure to other common childhood infections. Indeed, this is consistent with the increased frequency of GC TFH observed in the tonsils of HIV uninfected children compared to adults. However, both the correlation between TFH frequency and neutralization breadth, and the existence of high frequency HIV-specific GC TFH, demonstrates a significant HIV-specific component to this trend.
The other key difference between HIV infected children and adults highlighted by this study is the evidence of greater regulation of the TFH response in children. Again, this is most apparent in the lymphoid tissue, where regulation of TFH activity is most relevant (25). This makes biological sense, as the absence of TFR has been shown to cause an outgrowth of non-antigen specific or low-affinity B cells in germinal centers and leads to fewer antigen-specific cells (23, 27). Other work has suggested that expansion of regulatory cells in the GC in adult HIV and SIV infection may inhibit the generation of bnAbs (62). In addition, several studies have linked HIV bnAb development to a loss of immune tolerance and to autoimmunity (63–66), and reduced circulating TREG (66). This has led to the hypothesis that bnAb development is constrained by host tolerance controls and that lower regulation would promote the development of breadth. However, this concept has been based exclusively on work in adult infection, and this present study highlights the immune differences between HIV infected adults and children (4). For example, although we observed a similar increase in circulating TFH in children and adults, pediatric TFH expressed significantly lower levels of CD40L, which is crucial for providing B-cell help. Neonatal T cells are known to express lower levels of CD40L than adult counterparts (67), and these levels appear to increase with age (68). Overexpression of CD40L can lead to hypergammaglobulinemia in a lymphopenic mouse model (52), a characteristic of untreated HIV infection in adults that is associated with TFH activity (48). Therefore, lower expression of CD40L on pediatric TFH may help to regulate these cells as the developing immune system is exposed to new antigens and facilitate the development of bnAbs. Whether children make superior antibodies to adults per se is unclear and is complicated both by exposure that, for most common infections, occurs in childhood, and the influence of maternal antibodies. Infants infected with Rota virus, display superior neutralizing antibody responses greater than their mothers (69), and potent cross-clade neutralizing antibody responses against Enterovirus exist in children (70). However, children do not appear to make neutralizing antibodies to Influenza A H5/N1 infection, whilst adults do (71). Further study is required to better understand the process of antibody development in children, which may vary depending on pathogen, route and timing of infection.
It is important to highlight that tonsil samples studied here are not from the same subjects studied previously and from which the neutralization data derive (17). Moreover, it was not possible to obtain neutralization data from these children, due to ongoing ART (72). However, given that the ability of typical, progressing HIV infected children to neutralize a broad range of viruses is so much greater than that of HIV infected adults [a median of 63% of viruses neutralized vs. 25% of the same test set of viruses, respectively, p < 0.0001, (17)], it is clear that children are fundamentally different from adults in this respect. There is no reason to suppose that the children and adults studied here are not representative of those in whom neutralizing antibody responses have been previously mapped. The impact of ART on tonsil TFH and regulatory T-cell frequency and function within infected children is unclear. Limited studies indicate that ART treatment of chronically infected adults can reduce the frequency of TFH within lymph nodes, though it remains higher than uninfected adults (73). This is consistent with our own data in which the 3 ART treated adults have fewer tonsil TFH and TFR than the 3 untreated individuals. Indeed, if one considers only ART treated individuals, the difference in frequency of both cell types is even greater between children and adults.
Taken together, these data suggest, in children, the immune system is well adapted to the production of broadly HIV neutralizing antibodies, likely as a result of the immunotolerant immune environment in early life (74–76), that includes a relatively high degree of regulation of a high-frequency TFH response. In adults, where TFH cells appear to be substantially less abundant, better generation of bnAbs may depend on the strategy of decreasing TFH regulation (66). However, given the inherent aggressive nature of the adult immune response, decreasing T-cell regulation to increase bnAb generation may simultaneously bring the unwanted consequences of increased autoimmunity. Children, by contrast, may represent a much more attractive group in which long lasting broadly neutralizing antibodies against HIV infection are more easily generated, thereby providing protection against HIV infection when they become adults.
Author Contributions
JR designed the study, conducted experimental work within the study, analyzed the data, and wrote the paper. TM conducted experimental work within the study and analyzed the data. AN, DR, MM, EA, TA, and SK conducted experimental work within the study. WK, TN, FK, and PJ designed the study, recruited the subjects, and analyzed the data. AS, LM, PM, and SP conducted/supervised experimental work within the study. HK, PG, and AL supervised experimental work within the study, analyzed data, and wrote the paper. PG and AL established research cohorts.
Funding
AL: WT and HHMI; PG: WT (Grant 104748MA); HK: WT (Grant 202485/Z/16/Z).
Conflict of Interest Statement
The authors declare that the research was conducted in the absence of any commercial or financial relationships that could be construed as a potential conflict of interest.
Supplementary Material
The Supplementary Material for this article can be found online at: https://www.frontiersin.org/articles/10.3389/fimmu.2018.01975/full#supplementary-material
Figure S1. No correlation between viral load and effector TFH frequency. (A) Frequency of circulating central TFH correlates significantly with viral load within the pediatric cohort (r = 0.5, p = 0.0003; left) while there is no correlation with circulating effector TFH frequency (right). (B) Inverse correlation between PD1 expression on bulk CD4 (left) and CD8 (right) T-cells and viral load within the pediatric cohort. All correlations are performed by Spearman's rank test (n = 36).
Figure S2. High-frequency IL-21 production in HIV-specific GC-TFH cells in children but not adults. (A) The majority of tonsil TFH cells are “Th2”-TFH cells (CD4+CCR6−CXCR3−CXCR5+PD1+). See gating strategy on the left and distribution of Th2 (CCR6−CXCR3−), Th17 (CCR6+CXCR3−) and Th1 (CCR6−CXCR3+) of tonsil TFH on the right. (B) Tonsil bulk CD4 T-cells of infected children (blue squares; n = 4) secrete more IL-21 and less INF-g (C) in response to HIV peptide than CD4 T cells of infected adults (red triangles: Adults on ART, n = 3; red diamonds: Adults not on ART, n = 3) (n.s., Mann–Whitneys test). (D) No significant differences in Gag/Env specific IL-21 secretion of circulating “Th2”-TFH cells between ART-naïve infected children (blue squares; n = 38) and ART-naïve infected adults (red triangles; n = 18) (n.s., Kruskal–Wallis test). In scatterplots, medians are shown.
Figure S3. Tonsil follicular regulatory T cells (TFR) are increased in HIV-infected children. (A) Similar expression of CD25 in HIV infected and uninfected children (left: exemplary FACS plot; right: summary data of all pediatric samples). Closed blue squares: HIV infected (n = 38), open blue squares: HIV uninfected (n = 7). (B) No significant differences in the frequency of tonsil TFR or ratio of tonsil TFR to tonsil “Th2” TFH cells (gated on CD4+CCR6−CXCR3−CXCR5+PD1+) (C) in uninfected children (open blue squares; n = 6) compared to uninfected adults (open red triangles; n = 3) (n.s., Kruskal–Wallis test). (D) No significant differences in the frequency of circulating TFR in uninfected children (open blue squares; n = 7) compared to adults (open red triangles; n = 8) (n.s., Kruskal–Wallis test). (E) Uninfected children shown an increased ratio of circulating TFR to circulating effector TFH (gated on CD4+CD45RA−CXCR5+CXCR3−CCR7−PD1+) compared to uninfected adults (p = 0.02; Kruskal–Wallis test). (F) Positive non-significant correlation of frequency of circulating TFR and neutralization breadth in HIV-infected children (r = 0.29, p = 0.09; Spearman's rank test) (n = 36). (G) Inverse non-significant correlation of PD1 expression on circulating TFR and neutralization breadth within the pediatric cohort (r = −0.3, p = 0.08; Spearman's rank test) (n = 36). Medians are shown in scatter plots.
Table S1. Clinical characteristics of study cohort.
Table S2. List of antibodies for flow cytometry.
References
1. Mascola JR, Haynes BF. HIV-1 neutralizing antibodies: understanding nature's pathways. Immunol Rev. (2013) 254:225–44. doi: 10.1111/imr.12075
2. Streeck H, D'Souza MP, Littman DR, Crotty S. Harnessing CD4(+) T cell responses in HIV vaccine development. Nat Med. (2013) 19:143–9. doi: 10.1038/nm.3054
3. West APJr, Scharf L, Scheid JF, Klein F, Bjorkman PJ, Nussenzweig MC. Structural insights on the role of antibodies in HIV-1 vaccine and therapy. Cell (2014) 156:633–48. doi: 10.1016/j.cell.2014.01.052
4. Borrow P, Moody MA. Immunologic characteristics of HIV-infected individuals who make broadly neutralizing antibodies. Immunol Rev. (2017) 275:62–78. doi: 10.1111/imr.12504
5. Baba TW, Liska V, Hofmann-Lehmann R, Vlasak J, Xu W, Ayehunie S, et al. Human neutralizing monoclonal antibodies of the IgG1 subtype protect against mucosal simian-human immunodeficiency virus infection. Nat Med. (2000) 6:200–6. doi: 10.1038/72309
6. Mascola JR, Stiegler G, VanCott TC, Katinger H, Carpenter CB, Hanson CE, et al. Protection of macaques against vaginal transmission of a pathogenic HIV-1/SIV chimeric virus by passive infusion of neutralizing antibodies. Nat Med. (2000) 6:207–10. doi: 10.1038/72318
7. Parren PW, Marx PA, Hessell AJ, Luckay A, Harouse J, Cheng-Mayer C, et al. Antibody protects macaques against vaginal challenge with a pathogenic R5 simian/human immunodeficiency virus at serum levels giving complete neutralization in vitro. J Virol. (2001) 75:8340–7. doi: 10.1128/JVI.75.17.8340-8347.2001
8. Moldt B, Rakasz EG, Schultz N, Chan-Hui PY, Swiderek K, Weisgrau KL, et al. Highly potent HIV-specific antibody neutralization in vitro translates into effective protection against mucosal SHIV challenge in vivo. Proc Natl Acad Sci USA. (2012) 109:18921–5. doi: 10.1073/pnas.1214785109
9. Pegu A, Yang ZY, Boyington JC, Wu L, Ko SY, Schmidt SD, et al. Neutralizing antibodies to HIV-1 envelope protect more effectively in vivo than those to the CD4 receptor. Sci Transl Med. (2014) 6:ra288. doi: 10.1126/scitranslmed.3008992
10. Rerks-Ngarm S, Pitisuttithum P, Nitayaphan S, Kaewkungwal J, Chiu J, Paris R, et al. Vaccination with ALVAC and AIDSVAX to prevent HIV-1 infection in Thailand. N Engl J Med. (2009) 361:2209–20. doi: 10.1056/NEJMoa0908492
11. Montefiori DC, Karnasuta C, Huang Y, Ahmed H, Gilbert P, de Souza MS, et al. Magnitude and breadth of the neutralizing antibody response in the RV144 and Vax003 HIV-1 vaccine efficacy trials. J Infect Dis. (2012) 206:431–41. doi: 10.1093/infdis/jis367
12. Hammer SM, Sobieszczyk ME, Janes H, Karuna ST, Mulligan MJ, Grove D, et al. Efficacy trial of a DNA/rAd5 HIV-1 preventive vaccine. N Engl J Med. (2013) 369:2083–92. doi: 10.1056/NEJMoa1310566
13. Binley JM, Lybarger EA, Crooks ET, Seaman MS, Gray E, Davis KL, et al. Profiling the specificity of neutralizing antibodies in a large panel of plasmas from patients chronically infected with human immunodeficiency virus type 1 subtypes B and C. J Virol. (2008) 82:11651–68. doi: 10.1128/JVI.01762-08
14. van Gils MJ, Euler Z, Schweighardt B, Wrin T, Schuitemaker H. Prevalence of cross-reactive HIV-1-neutralizing activity in HIV-1-infected patients with rapid or slow disease progression. AIDS (2009) 23:2405–14. doi: 10.1097/QAD.0b013e32833243e7
15. Landais E, Huang X, Havenar-Daughton C, Murrell B, Price MA, Wickramasinghe L, et al. Broadly neutralizing antibody responses in a large longitudinal sub-saharan HIV primary infection cohort. PLoS Pathog. (2016) 12:e1005369. doi: 10.1371/journal.ppat.1005369
16. Sather DN, Armann J, Ching LK, Mavrantoni A, Sellhorn G, Caldwell Z, et al. Factors associated with the development of cross-reactive neutralizing antibodies during human immunodeficiency virus type 1 infection. J Virol. (2009) 83:757–69. doi: 10.1128/JVI.02036-08
17. Muenchhoff M, Adland E, Karimanzira O, Crowther C, Pace M, Csala A, et al. Nonprogressing HIV-infected children share fundamental immunological features of nonpathogenic SIV infection. Sci Transl Med. (2016) 8:358ra125. doi: 10.1126/scitranslmed.aag1048
18. Goo L, Chohan V, Nduati R, Overbaugh J. Early development of broadly neutralizing antibodies in HIV-1-infected infants. Nat Med. (2014) 20:655–8. doi: 10.1038/nm.3565
19. Fouda GG, Cunningham CK, McFarland EJ, Borkowsky W, Muresan P, Pollara J, et al. Infant HIV type 1 gp120 vaccination elicits robust and durable anti-V1V2 immunoglobulin G responses and only rare envelope-specific immunoglobulin A responses. J Infect Dis. (2015) 211:508–17. doi: 10.1093/infdis/jiu444
20. McGuire EP, Fong Y, Toote C, Cunningham CK, McFarland EJ, Borkowsky W, et al. HIV-exposed infants vaccinated with an MF59/recombinant gp120 vaccine have higher-magnitude anti-V1V2 IgG responses than adults immunized with the same vaccine. J Virol. (2018) 92:e01070-17. doi: 10.1128/JVI.01070-17
21. Doria-Rose NA. HIV neutralizing antibodies: clinical correlates and implications for vaccines. J Infect Dis. (2010) 201:981–3. doi: 10.1086/651143
22. Crotty S. A brief history of T cell help to B cells. Nat Rev Immunol. (2015) 15:185–9. doi: 10.1038/nri3803
23. Vinuesa CG, Linterman MA, Yu D, MacLennan IC. Follicular helper T Cells. Annu Rev Immunol. (2016) 34:335–68. doi: 10.1146/annurev-immunol-041015-055605
24. Victora GD, Schwickert TA, Fooksman DR, Kamphorst AO, Meyer-Hermann M, Dustin ML, et al. Germinal center dynamics revealed by multiphoton microscopy with a photoactivatable fluorescent reporter. Cell (2010) 143:592–605. doi: 10.1016/j.cell.2010.10.032
25. Pratama A, Vinuesa CG. Control of TFH cell numbers: why and how? Immunol Cell Biol. (2014) 92:40–8. doi: 10.1038/icb.2013.69
26. Chung Y, Tanaka S, Chu F, Nurieva RI, Martinez GJ, Rawal S, et al. Follicular regulatory T cells expressing Foxp3 and Bcl-6 suppress germinal center reactions. Nat Med. (2011) 17:983–8. doi: 10.1038/nm.2426
27. Linterman MA, Pierson W, Lee SK, Kallies A, Kawamoto S, Rayner TF, et al. Foxp3+ follicular regulatory T cells control the germinal center response. Nat Med. (2011) 17:975–82. doi: 10.1038/nm.2425
28. Wollenberg I, Agua-Doce A, Hernandez A, Almeida C, Oliveira VG, Faro J, et al. Regulation of the germinal center reaction by Foxp3+ follicular regulatory T cells. J Immunol. (2011) 187:4553–60. doi: 10.4049/jimmunol.1101328
29. Ding Y, Li J, Yang P, Luo B, Wu Q, Zajac AJ, et al. Interleukin-21 promotes germinal center reaction by skewing the follicular regulatory T cell to follicular helper T cell balance in autoimmune BXD2 mice. Arthritis Rheumatol. (2014) 66:2601–12. doi: 10.1002/art.38735
30. Sage PT, Alvarez D, Godec J, von Andrian UH, Sharpe AH. Circulating T follicular regulatory and helper cells have memory-like properties. J Clin Invest (2014) 124:5191–204. doi: 10.1172/JCI76861
31. Vinuesa CG. HIV and T follicular helper cells: a dangerous relationship. J Clin Invest (2012) 122:3059–62. doi: 10.1172/JCI65175
32. Chowdhury A, Del Rio Estrada PM, Tharp GK, Trible RP, Amara RR, Chahroudi A, et al. Decreased T follicular regulatory cell/T follicular helper cell (TFH) in simian immunodeficiency virus-infected rhesus macaques may contribute to accumulation of TFH in chronic infection. J Immunol. (2015) 195:3237–47. doi: 10.4049/jimmunol.1402701
33. Kawamoto S, Maruya M, Kato LM, Suda W, Atarashi K, Doi Y, et al. Foxp3+ T cells regulate immunoglobulin a selection and facilitate diversification of bacterial species responsible for immune homeostasis. Immunity (2014) 41:152–65. doi: 10.1016/j.immuni.2014.05.016
34. Leon B, Bradley JE, Lund FE, Randall TD, Ballesteros-Tato A. FoxP3+ regulatory T cells promote influenza-specific Tfh responses by controlling IL-2 availability. Nat Commun. (2014) 5:3495. doi: 10.1038/ncomms4495
35. Quigley MF, Gonzalez VD, Granath A, Andersson J, Sandberg JK. CXCR5+ CCR7-CD8 T cells are early effector memory cells that infiltrate tonsil B cell follicles. Eur J Immunol. (2007) 37:3352–62. doi: 10.1002/eji.200636746
36. He R, Hou S, Liu C, Zhang A, Bai Q, Han M, et al. Follicular CXCR5- expressing CD8+ T cells curtail chronic viral infection. Nature (2016) 537:412–28. doi: 10.1038/nature19317
37. Mylvaganam GH, Rios D, Abdelaal HM, Iyer S, Tharp G, Mavigner M, et al. Dynamics of SIV-specific CXCR5+ CD8 T cells during chronic SIV infection. Proc Natl Acad Sci USA. (2017) 114:1976–81. doi: 10.1073/pnas.1621418114
38. Kim HJ, Verbinnen B, Tang X, Lu L, Cantor H. Inhibition of follicular T-helper cells by CD8+ regulatory T cells is essential for self tolerance. Nature (2010) 467:328–32. doi: 10.1038/nature09370
39. Kim HJ, Wang X, Radfar S, Sproule TJ, Roopenian DC, Cantor H. CD8+ T regulatory cells express the Ly49 Class I MHC receptor and are defective in autoimmune prone B6-Yaa mice. Proc Natl Acad Sci USA. (2011) 108:2010–5. doi: 10.1073/pnas.1018974108
40. Leavenworth JW, Tang X, Kim HJ, Wang X, Cantor H. Amelioration of arthritis through mobilization of peptide-specific CD8+ regulatory T cells. J Clin Invest (2013) 123:1382–9. doi: 10.1172/JCI66938
41. Gray ES, Madiga MC, Hermanus T, Moore PL, Wibmer CK, Tumba NL, et al. The neutralization breadth of HIV-1 develops incrementally over four years and is associated with CD4+ T cell decline and high viral load during acute infection. J Virol. (2011) 85:4828–40. doi: 10.1128/JVI.00198-11
42. Lovelace P, Maecker HT. Multiparameter intracellular cytokine staining. In Hawley T, Hawley R, editors. Flow Cytometry Protocols. Methods in Molecular Biology. Vol. 1678. New York, NY: Humana Press (2018).
43. Roederer M, Nozzi JL, Nason MC. SPICE: exploration and analysis of post-cytometric complex multivariate datasets. Cytometry A (2011) 79:167–74. doi: 10.1002/cyto.a.21015
44. Locci M, Havenar-Daughton C, Landais E, Wu J, Kroenke MA, Arlehamn CL, et al. Human circulating PD-1+CXCR3-CXCR5+ memory Tfh cells are highly functional and correlate with broadly neutralizing HIV antibody responses. Immunity (2013) 39:758–69. doi: 10.1016/j.immuni.2013.08.031
45. Cohen K, Altfeld M, Alter G, Stamatatos L. Early preservation of CXCR5+ PD-1+ helper T cells and B cell activation predict the breadth of neutralizing antibody responses in chronic HIV-1 infection. J Virol. (2014) 88:13310–21. doi: 10.1128/JVI.02186-14
46. He J, Tsai LM, Leong YA, Hu X, Ma CS, Chevalier N, et al. Circulating precursor CCR7(lo)PD-1(hi) CXCR5+ CD4+ T cells indicate Tfh cell activity and promote antibody responses upon antigen reexposure. Immunity (2013) 39:770–81. doi: 10.1016/j.immuni.2013.09.007
47. Haynes NM, Allen CD, Lesley R, Ansel KM, Killeen N, Cyster JG. Role of CXCR5 and CCR7 in follicular Th cell positioning and appearance of a programmed cell death gene-1high germinal center-associated subpopulation. J Immunol. (2007) 179:5099–108. doi: 10.4049/jimmunol.179.8.5099
48. Lindqvist M, van Lunzen J, Soghoian DZ, Kuhl BD, Ranasinghe S, Kranias G, et al. Expansion of HIV-specific T follicular helper cells in chronic HIV infection. J Clin Invest (2012) 122:3271–80. doi: 10.1172/JCI64314
49. Pene J, Gauchat JF, Lecart S, Drouet E, Guglielmi P, Boulay V, et al. Cutting edge: IL-21 is a switch factor for the production of IgG1 and IgG3 by human B cells. J Immunol. (2004) 172:5154–7. doi: 10.4049/jimmunol.172.9.5154
50. Good KL, Bryant VL, Tangye SG. Kinetics of human B cell behavior and amplification of proliferative responses following stimulation with IL-21. J Immunol. (2006) 177:5236–47. doi: 10.4049/jimmunol.177.8.5236
51. Bryant VL, Ma CS, Avery DT, Li Y, Good KL, Corcoran LM, et al. Cytokine-mediated regulation of human B cell differentiation into Ig-secreting cells: predominant role of IL-21 produced by CXCR5+ T follicular helper cells. J Immunol. (2007) 179:8180–90. doi: 10.4049/jimmunol.179.12.8180
52. Baumjohann D, Preite S, Reboldi A, Ronchi F, Ansel KM, Lanzavecchia A, et al. Persistent antigen and germinal center B cells sustain T follicular helper cell responses and phenotype. Immunity (2013) 38:596–605. doi: 10.1016/j.immuni.2012.11.020
53. Sage PT, Francisco LM, Carman CV, Sharpe AH. The receptor PD-1 controls follicular regulatory T cells in the lymph nodes and blood. Nat Immunol. (2013) 14:152–61. doi: 10.1038/ni.2496
54. Miles B, Miller SM, Folkvord JM, Levy DN, Rakasz EG, Skinner PJ, et al. Follicular Regulatory CD8 T Cells Impair the Germinal Center Response in SIV and Ex Vivo HIV Infection. PLoS Pathog. (2016) 12:e1005924. doi: 10.1371/journal.ppat.1005924
55. Belanger S, Crotty S. Dances with cytokines, featuring TFH cells, IL-21, IL-4 and B cells. Nat Immunol. (2016) 17:1135–6. doi: 10.1038/ni.3561
56. Conrad JA, Ramalingam RK, Duncan CB, Smith RM, Wei J, Barnett L, et al. Antiretroviral therapy reduces the magnitude and T cell receptor repertoire diversity of HIV-specific T cell responses without changing T cell clonotype dominance. J Virol. (2012) 86:4213–21. doi: 10.1128/JVI.06000-11
57. Crotty S. Follicular helper CD4 T cells (TFH). Annu Rev Immunol. (2011) 29:621–63. doi: 10.1146/annurev-immunol-031210-101400
58. Schultz BT, Teigler JE, Pissani F, Oster AF, Kranias G, Alter G, et al. Circulating HIV-specific interleukin-21+CD4+ T cells represent peripheral Tfh cells with antigen-dependent helper functions. Immunity (2016) 44:167–78. doi: 10.1016/j.immuni.2015.12.011
59. Yamamoto T, Lynch RM, Gautam R, Matus-Nicodemos R, Schmidt SD, Boswell KL, et al. Quality and quantity of TFH cells are critical for broad antibody development in SHIVAD8 infection. Sci Transl Med. (2015) 7:298ra120. doi: 10.1126/scitranslmed.aab3964
60. Cubas R, van Grevenynghe J, Wills S, Kardava L, Santich BH, Buckner CM, et al. Reversible reprogramming of circulating memory T follicular helper cell function during chronic HIV infection. J Immunol. (2015) 195:5625–36. doi: 10.4049/jimmunol.1501524
61. Velu V, Mylvaganam GH, Gangadhara S, Hong JJ, Iyer SS, Gumber S, et al. Induction of Th1-biased T follicular helper (Tfh) cells in lymphoid tissues during chronic simian immunodeficiency virus infection defines functionally distinct germinal center Tfh cells. J Immunol. (2016) 197:1832–42. doi: 10.4049/jimmunol.1600143
62. Miles B, Miller SM, Folkvord JM, Kimball A, Chamanian M, Meditz AL, et al. Follicular regulatory T cells impair follicular T helper cells in HIV and SIV infection. Nat Commun. (2015) 6:8608. doi: 10.1038/ncomms9608
63. Doyle-Cooper C, Hudson KE, Cooper AB, Ota T, Skog P, Dawson PE, et al. Immune tolerance negatively regulates B cells in knock-in mice expressing broadly neutralizing HIV antibody 4E10. J Immunol. (2013) 191:3186–91. doi: 10.4049/jimmunol.1301285
64. Liao HX, Lynch R, Zhou T, Gao F, Alam SM, Boyd SD, et al. Co-evolution of a broadly neutralizing HIV-1 antibody and founder virus. Nature (2013) 496:469–76. doi: 10.1038/nature12053
65. Bonsignori M, Wiehe K, Grimm SK, Lynch R, Yang G, Kozink DM, et al. An autoreactive antibody from an SLE/HIV-1 individual broadly neutralizes HIV-1. J Clin Invest (2014) 124:1835–43. doi: 10.1172/JCI73441
66. Moody MA, Pedroza-Pacheco I, Vandergrift NA, Chui C, Lloyd KE, Parks R, et al. Immune perturbations in HIV-1-infected individuals who make broadly neutralizing antibodies. Sci Immunol. (2016) 1:aag0851. doi: 10.1126/sciimmunol.aag0851
67. Nonoyama S, Penix LA, Edwards CP, Lewis DB, Ito S, Aruffo A, et al. Diminished expression of CD40 ligand by activated neonatal T cells. J Clin Invest. (1995) 95:66–75. doi: 10.1172/JCI117677
68. Gilmour KC, Walshe D, Heath S, Monaghan G, Loughlin S, Lester T, et al. Immunological and genetic analysis of 65 patients with a clinical suspicion of X linked hyper-IgM. Mol Pathol. (2003) 56:256–62. doi: 10.1136/mp.56.5.256
69. Ray PG, Kelkar SD. Prevalence of neutralizing antibodies against different rotavirus serotypes in children with severe rotavirus-induced diarrhea and their mothers. Clin Diagn Lab Immunol. (2004) 11:186–94. doi: 10.1128/CDLI.11.1.186-194.2004
70. Arthur Huang KY, Chen MF, Huang YC, Shih SR, Chiu CH, Lin JJ, et al. Epitope-associated and specificity-focused features of EV71-neutralizing antibody repertoires from plasmablasts of infected children. Nat Commun. (2017) 8:762. doi: 10.1038/s41467-017-00736-9
71. Khuntirat B, Love CS, Buddhari D, Heil GL, Gibbons RV, Rothman AL, et al. Absence of neutralizing antibodies against influenza A/H5N1 virus among children in Kamphaeng Phet, Thailand. J Clin Virol. (2015) 69:78–80. doi: 10.1016/j.jcv.2015.05.025
72. Gach JS, Achenbach CJ, Chromikova V, Berzins B, Lambert N, Landucci G, et al. HIV-1 specific antibody titers and neutralization among chronically infected patients on long-term suppressive antiretroviral therapy (ART): a cross-sectional study. PLoS ONE (2014) 9:e85371. doi: 10.1371/journal.pone.0085371
73. Hong JJ, Chang KT, Villinger F. The dynamics of T and B cells in lymph node during chronic HIV infection: TFH and HIV, unhappy dance partners? Front Immunol. (2016) 7:522. doi: 10.3389/fimmu.2016.00522
74. Mold JE, McCune JM. At the crossroads between tolerance and aggression: revisiting the “layered immune system” hypothesis. Chimerism (2011) 2:35–41. doi: 10.4161/chim.2.2.16329
75. Kollmann TR, Levy O, Montgomery RR, Goriely S. Innate immune function by Toll-like receptors: distinct responses in newborns and the elderly. Immunity (2012) 37:771–83. doi: 10.1016/j.immuni.2012.10.014
Keywords: pediatric HIV infection, broadly neutralizing antibodies (bnAb), T-follicular helper cells (Tfh), T-follicular regulatory helper cells (Tfreg), follicular CD8 T-cells, germinal center, vaccination
Citation: Roider J, Maehara T, Ngoepe A, Ramsuran D, Muenchhoff M, Adland E, Aicher T, Kazer SW, Jooste P, Karim F, Kuhn W, Shalek AK, Ndung'u T, Morris L, Moore PL, Pillai S, Kløverpris H, Goulder P and Leslie A (2018) High-Frequency, Functional HIV-Specific T-Follicular Helper and Regulatory Cells Are Present Within Germinal Centers in Children but Not Adults. Front. Immunol. 9:1975. doi: 10.3389/fimmu.2018.01975
Received: 19 June 2018; Accepted: 10 August 2018;
Published: 12 September 2018.
Edited by:
Leonidas Stamatatos, Fred Hutchinson Cancer Research Center, United StatesReviewed by:
Catarina E. Hioe, Icahn School of Medicine at Mount Sinai, United StatesLisa A. Chakrabarti, Institut Pasteur, France
Copyright © 2018 Roider, Maehara, Ngoepe, Ramsuran, Muenchhoff, Adland, Aicher, Kazer, Jooste, Karim, Kuhn, Shalek, Ndung'u, Morris, Moore, Pillai, Kløverpris, Goulder and Leslie. This is an open-access article distributed under the terms of the Creative Commons Attribution License (CC BY). The use, distribution or reproduction in other forums is permitted, provided the original author(s) and the copyright owner(s) are credited and that the original publication in this journal is cited, in accordance with accepted academic practice. No use, distribution or reproduction is permitted which does not comply with these terms.
*Correspondence: Philip Goulder, cGhpbGlwLmdvdWxkZXJAcGFlZGlhdHJpY3Mub3guYWMudWs=
Alasdair Leslie, YWwubGVzbGllQGFocmkub3Jn
†These authors have contributed equally to this work