- Department of Gastroenterology, Tongji Hospital, Tongji Medical College, Huazhong University of Science and Technology, Wuhan, China
Autoimmune hepatitis (AIH) is a severe and chronic liver disease, and its incidence has increased worldwide in recent years. Research into the pathogenesis of AIH remains limited largely owing to the lack of suitable mouse models. The concanavalin A (ConA) mouse model is a typical and well-established model used to investigate T cell-dependent liver injury. However, ConA-induced hepatitis is acute and usually disappears after 48 h; thus, it does not mimic the pathogenesis of AIH in the human body. Several studies have explored various AIH mouse models, but as yet there is no widely accepted and valid mouse model for AIH. Immunosuppression is the standard clinical therapy for AIH, but patient side effects and recurrence limit its use. Regulatory T cells (Tregs) play critical roles in the maintenance of immune homeostasis and in the prevention of autoimmune diseases, which may provide a potential therapeutic target for AIH therapy. However, the role of Tregs in AIH has not yet been clarified, partly because of difficulties in diagnosing AIH and in collecting patient samples. In this review, we discuss the studies related to Treg in various AIH mouse models and patients with AIH and provide some novel insights for this research area.
Introduction
Autoimmune hepatitis (AIH) is a progressive inflammatory liver disease characterized by chronic inflammation of the liver, circulating autoantibodies, hypergammaglobulinemia, and specific liver biopsy histologic features (interface hepatitis, rosettes, and lymphocyte invasion) (1, 2). AIH occurs in all ethnicities and can affect children and adults of all ages, with a female predominance (3). AIH is a complex multifactorial polygenic disorder that is thought to be caused by the interaction between triggers and environmental factors in genetically susceptible individuals, leading to the loss of tolerance against one's own liver antigens (4, 5). Standard immunosuppressive treatment approaches have remained static for decades; these treatments are effective in most patients, although their mechanisms are unclear (6). Many patients with AIH must receive long-term immunosuppressive treatment to prevent disease relapse (7).
The precise etiology and pathophysiology of AIH remain largely unknown, and related basic research in this field is relatively limited compared with other types of hepatitis, such as viral hepatitis or steatohepatitis. The complexity of the disease, the difficulty of confirming a clinical diagnosis, and the lack of valid animal models for AIH research contribute to this situation. Researchers have been working on the establishment of a mouse model for AIH for a long time, but as yet no valid animal model has been widely accepted (8, 9). In 1992, Tiegs et al. (10) established a T cell-mediated acute hepatitis mouse model using concanavalin A (ConA), which is a typical and well-recognized mouse model used to investigate T cell-mediated liver injury. However, hepatitis in mice livers induced by ConA is acute and often disappears after 48 h; furthermore, there is no autoantibody production or liver fibrosis. In 2008, Christen et al. (11) established a chronic AIH mouse model using adenovirus that expressed human cytochrome P450 2D6 (CYP2D6), which is a well-recognized human autoantigen in type-2 AIH. In 2013, Hardtke-Wolenski et al. (12) established an AIH model against FTCD in NOD with one single adenoviral injection into the tail vein, but in 2016 they did not observe chronic AIH with either soluble liver antigen (SLA) or CYP2D6 in B6, Balb/c and FVB (13). Given that a one-time adenovirus injection may not be sufficient to induce a stable and long-term mouse model, we improved the method to establish this CYP2D6-AIH mouse model through the repeated injection of the CYP2D6 plasmid to achieve multiple gene transection in the liver (14). However, although great efforts have been made, the identification of a valid mouse model for use in the AIH research field remains an urgent, unsolved issue.
Regulatory T cells (Tregs) that express the transcription factor Foxp3 play an important role in the maintenance of immunological self-tolerance and the prevention of autoimmunity (15). Tregs are recognized as the body's main source of tolerance regulation, and their impairment has been associated with the development of various autoimmune diseases (16). Given the ability of Tregs to suppress the destructive pro-inflammatory and cytolytic activities of immune effector cells, the adoptive transfer of Treg has been considered as a potential future treatment for AIH (17). However, the role of Tregs in AIH remains controversial (18, 19), and for nearly 20 years, researchers have been exploring whether or not the Tregs are impaired in AIH. The results of this research are inconclusive, partly because of the different mouse models used in the different studies and patient heterogeneity. Here we review these issues by first summarizing the different mouse models and then focusing on the studies of AIH to understand what roles have been played by Tregs in the pathogenesis of AIH.
AIH: Current Research Status
Autoimmune hepatitis (AIH) is a liver-specific autoimmune disease first described in 1951 (5). AIH manifests in all age groups, and its incidence has shown an increasing trend (20). The disease is now widely known as a consequence of immune tolerance breakdown, leading to an autoimmune response against hepatocytes that induces liver injury (21). AIH is divided into two main types based on the serological profiles of persistent autoantibodies (22). Type 1 AIH is defined by the presence of antinuclear antibodies (ANAs) and/or anti-smooth muscle antibodies (SMAs), while type 2 AIH is defined by the presence of anti-liver-kidney microsomal 1 (LKM-1) antibody and/or anti-liver cytosol type 1 (LC1) antibody (23, 24). The pathogenesis of AIH is complicated and unclear, but it likely involves environmental factors (25), genetic factors, epigenetic factors (26, 27), and a dynamic immunological microenvironment (28). It is widely accepted that both the initiation of the self-attack and the subsequent dysregulation of the immune system in the liver microenvironment contribute to the progressive process of liver damage. During this process, helper T (Th) cells play the most important role in triggering this self-attack process by recognizing the autoantigens (29), while B cells are responsible for the subsequent production of autoantibodies (29). Meanwhile, increasing evidence suggests the emerging role of impaired immunoregulation in this process (30–32). Cells and cytokines involved immunoregulation maintaining the liver immunologic balance to protect the liver from serve damage under inflammatory attack have also been widely studied in recent years (29, 33, 34). However, further studies are required to elucidate their exact roles in the AIH disease process.
Clinically, although a well-established diagnostic scoring system with acceptable specificity and sensitivity is in use (35), AIH diagnosis remains difficult, largely owing to its dependence on liver biopsy. Moreover, due to the insidious and atypical symptoms of patients, AIH is difficult to diagnose in the very early stage, which leads to delays in treatment. Therefore, an increasing number of studies have explored AIH biomarkers that could be applied to obtain an early diagnosis (36, 37). Given the diagnostic difficulties of AIH, the related clinical research is difficult to carry out. Furthermore, there is no effective therapy for patients with AIH except for standard immunosuppressive treatment using corticosteroids with or without azathioprine (2, 38). However, not all patients respond well to this treatment, and most will develop disease relapse after drug withdrawal (39). Immunoregulatory therapy has also been explored as a potential therapy for AIH; however, it has not yet been clinically applied (6, 40, 41). Much research effort has been given in recent decades to comprehensively understand the pathogenesis of AIH and to find potential therapeutic targets (42, 43). However, it is difficult to progress basic research in the area of AIH because of the lack of a suitable and widely accepted mouse model that imitates the AIH disease process in humans.
Different AIH Mouse Models
ConA Mouse Model
There has been a lot of research effort in the last 50 years to establish AIH mouse models (44–46). In 1992, Tiegs et al. (10) were the first to report the use of ConA to establish a T cell-mediated hepatitis mouse model, which is now the most widely used tool to investigate immune-mediated liver injury (47–49). Progressive hepatitis, severe lymphocytes infiltration, and significantly increased transaminase release are observed within 8 h; this condition results from the activation of T lymphocytes by macrophages in the presence of ConA. Subsequently, IFN-γ (interferon-γ) and TNF-α (tumor necrosis factor-α) were proven to be the critical mediators of liver injury in ConA-treated mice (50), which is similar to the situation in patients with AIH (51). Over the past decades, substantial basic research on AIH was carried out using this typical and well-established ConA mouse model due to its convenience and low cost (52–54). However, hepatitis in this mouse model is acute and usually disappears within 48 h (10, 55). Characteristic features of AIH, such as the presence of autoantibodies, typical interface hepatitis, and progressive liver fibrosis, are not observed in this model.
Mouse Model of Type 2 AIH
Autoantibodies against the autoantigens expressed on hepatocytes play crucial roles in the pathogenesis of AIH; therefore, breaking immune tolerance using known autoantigens may provide a pathway for the establishment of a chronic AIH mouse model. In type 2 AIH, CYP2D6 is one of the most thoroughly characterized autoantigens that is recognized by type 1 liver/kidney microsomal autoantibodies (LKM-1) (56), while the target of LC1 antibodies is FTCD (57). In 2004, Lapierre et al. (58) first established a murine model of AIH via DNA immunization against CYP2D6 and FTCD. The authors found cytotoxic-specific T cells and the presence of necroinflammation in the liver, with the alanine aminotransferase (ALT) level peaking at 4 and 7 months post-injection. Anti-LKM1 and anti-LC1 antibodies were also detected in the mice sera, which stay elevated for at least 8 months (59). The genetic background was proven to affect AIH development by the fact that C57Bl/6J mice were more susceptible to the DNA vaccination compared with the 129/Sv or BALB/c strains (60). The age and sex susceptibility bias of AIH was also investigated in this model (61). A few years later, Christen et al. infected mice with adenovirus(Ad) that expressed human CYP2D6 based on an FVB/N or C57Bl/6J background and observed the characteristic pathological features and the presence of anti-LKM1 antibodies in their livers (11, 62–64). In this CYP2D6 model, acute liver damage resulting from the adenovirus infection was first observed, followed by massive infiltration, progressive liver inflammation, and fibrosis at 8 weeks, which is a much shorter timeframe than the model from Lapierre et al. (58).
In 2013, Hardtke-Wolenski et al. (12) developed a model of type 2 AIH by inducing a self-limited adenoviral infection with FTCD. The authors also demonstrated that the development of AIH in autoantibody-positive animals was determined by the genetic background (13). However, they did not observe the development of AIH when using Christen's protocol and a one-time Ad-CYP2D6 injection on the FVB/N background. These differences may have been due to their different injection methods or the different time points at which the mice were sacrificed. Recently, we also introduced an improved method for establishing a CYP2D6-induced AIH mouse model using an initial one-time adenovirus infection and repeated injections of human CYP2D6 plasmid based on the hydrodynamic-based liver-targeted gene delivery technique (14). This achieves the initial transient hepatitis using pure adenovirus and the multiple continuous expression of the naked CYP2D6 plasmid. Autoantibodies and interface hepatitis can be observed at 4 weeks after the first injection, with the appearance of progressive liver fibrosis at 5 weeks. This provides another technical approach to establishing a CYP2D6-induced type 2 AIH mouse model (Figure 1).

Figure 1. Characteristic features of type 2 AIH in an improved mouse model. (A) Chronic liver inflammation (interface hepatitis) and characteristic pathological features (rosettes and lymphocytes invasion) are shown. The red arrow indicates the hepatocyte. (B) Sirius red staining showing the fibrosis in a mouse liver. The red color indicates collagenous fibers. (C) Stained autoantibodies from the plasma of AIH mice.
Other Mouse Models for AIH
In addition to the acute ConA mouse model and the chronic type 2 AIH mouse models, other studies have reported using different methods to mimic AIH in the human body; these are presented in Figure 2. First, liver antigens have been used to initiate AIH by injection of syngeneic liver homogenate (S-100) in complete Freund's adjuvant (46) alone or with thymectomy (65), which can be used to induced AIH in mice. Second, exogenous cytokines such as IL (interleukin)-12 may also be used as triggers to induce AIH (66–68). Third, several transgenic mice that underwent specific T cells adoptive transfer were used to break the peripheral or central tolerance to induce AIH (69–71). The human leukocyte antigen (HLA)-DR3 and DR4 allele related transgenic AIH mouse models have also been established due to their strong genetic association with both type 1 and type 2 AIH (42, 72). Some studies have even reported spontaneous AIH in specific transgenic mouse models (73–75), the mechanism of which largely depends on the breakdown of normal immune tolerance. Furthermore, given that Tregs are the most important regulatory cells maintaining immune tolerance, scientists have also tried to induce AIH in mice by depleting Tregs using PD-1 (programmed cell death protein 1)–/– mice with neonatal thymectomy (76–78).
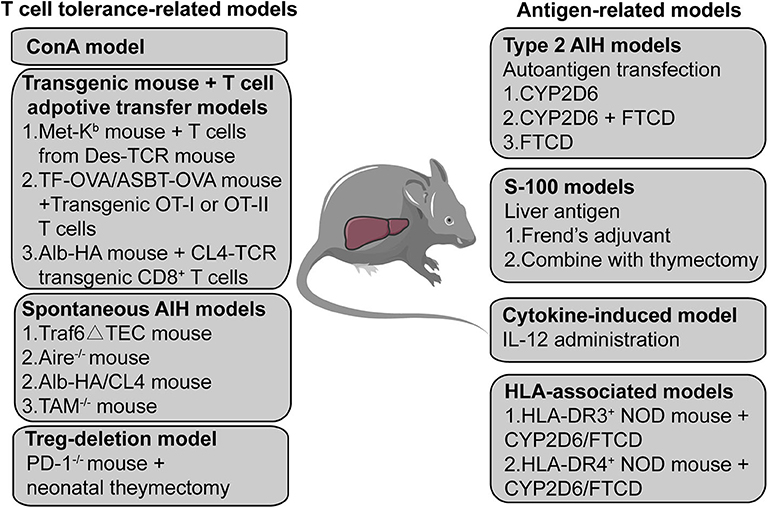
Figure 2. A summary of AIH mouse models. The mouse models are divided into two categories; one is based on a T cell-related mechanism and the other is based on an autoantigen- or liver antigen-related mechanism. The ConA mouse model is the most widely used mouse model to investigate acute T cell-mediated liver injury. Transgenic mice combined with T cell adoptive transfer also provides a method to establish an AIH mouse model. Some transgenic or gene knockout mice can develop spontaneous AIH-like disease. Treg depletion may also function as a potential method to induce AIH in mice. Transfecting the human autoantigen CYP2D6 or FTCD from type 2 AIH into mice may simulate the initiation process of type 2 AIH in humans to establish a chronic type 2 AIH mouse model. S-100, a supernatant of syngeneic liver homogenate, has also been used to induce AIH in mice. The expression of transgenic IL-2 in hepatocytes causes loss of tolerance of hepatocellular antigens, leading to chronic type 1 AIH-like disease in mice. Transfection HLA-DR3 or HLA-DR4 transgenic mice with the non-obese -diabetic background with a plasmid containing CYP2D6 and FTCD can also induce AIH.
Although there appear to be many options for AIH mouse models, different models should be carefully selected based on different research aims. To be more specific, the ConA model is more suitable for the investigation of drug-induced liver injury or the therapeutic effect of certain reagents in controlling the acute immune-mediated liver injury. The models based on transgenic/knockout mice (plus T cell transfer/deletion or not) may not suitable for studying particular immune cells due to their deficient immune system even before AIH initiation. Therefore, spontaneous type 2 AIH in mice that triggered by natural disease antigens can better simulate the natural process of AIH in the human body. It will be more consistent with its clinical characteristic as well, which is more appropriate for investigating the roles of immune cells in the disease process of AIH.
The Role of Tregs in AIH
Tregs in AIH Mouse Models
Tregs are important regulatory cells that maintain immune tolerance and have shown considerable potential in treating multiple autoimmune diseases (79–81). The depletion of Tregs in mice has been reported as a method to establish an AIH mouse model (76). However, the exact role of Tregs in AIH remains unclear. Scientists have explored the function and number of Tregs in different AIH mouse models, which are summarized in Table 1. The conclusions of these studies are conflicting, probably because of the different mechanisms used to induce AIH in the different mouse models and the different markers and methods used to detect Tregs.
For instance, Tregs behave completely differently in acute and chronic mouse models. In the ConA mouse model, the function and frequency of Tregs are increased both in the liver and in the peripheral environment (85–88), but decreased in the type 2 AIH mouse model (82). The increased Tregs in the liver of the ConA mouse model are mainly responding to the severe liver inflammation induced by ConA, while their deficiency results in long-term impaired immune homeostasis in the chronic type 2 mouse model. Moreover, two different studies reported contrary results regarding Tregs in the liver of the S100 liver homogenate mouse model (83, 89). This discrepancy likely arose because of the different sexes of the experimental mice, as discussed in a previous study (61). However, using a drug-induced AIH mouse model, one study showed that IL-33-induced Tregs confer protection against liver damage in both female and male mice (90). Furthermore, several studies have indicated that Tregs behave differently inside and outside of the hepatic microenvironment. Alexandropoulos et al. reported evidence of Tregs-mechanisms in the liver based on the Traf6ΔTEC mouse model, but the peripheral tolerance in the mice was normal (74). Schott et al. found that Tregs accumulated in the liver of the TF-OVA mouse model but remained unchanged in the spleen (84). The different prevalence of Tregs in the mouse liver and peripheral microenvironment (spleen or blood) may reflect the unique and organ-specific pathogenesis of AIH.
Although Tregs show different changes among the different models, some studies have focused on efforts to improve Tregs immunoregulation in mice. In 2015, Hardtke-Wolenski et al. (75) reported hyperproliferative intrahepatic Tregs in a spontaneous transgenic AIH mouse model with ongoing severe AIH, and they also found the AIH in those mice could be treated by adoptive Tregs transfer. Those findings suggest that the intrahepatic Tregs that increase during the process of AIH are dysfunctional or not sufficient to control the severe liver inflammation. Some other studies have also provided evidence for the curative effect of Tregs adoptive transfer in different AIH mouse models (82, 86, 91). Several animal studies have investigated improving the Tregs frequency (88) or the impaired Treg/Th17 balance (83, 85, 92) in the liver to reduce immune-mediated liver damage in mice. In general, owing to the different mechanisms in the different mouse models to trigger AIH, it is reasonable to have incoherent observation results from various studies. However, the various Treg-related studies in different mouse models can still provide us with this consistent enlightenment—improving the Tregs immunoregulation (function and/or number) in AIH can relieve the hepatitis and liver damage in mice.
Tregs in Patients With AIH
Over the past 20 years, Tregs in patients with AIH have been investigated, but the function and number of Tregs in these patients remains controversial (Table 2). The conflicting results may be due to patient heterogeneity, the use of different markers, and differences in the methodologies applied in various studies. It was long believed that in AIH, Tregs were functionally and/or numerically impaired (18, 32, 95, 97–99). Several studies suggested that decreases of suppressive molecules or genes in patients with AIH may lead to Tregs deficiency (96, 99, 100). In contrast, recent studies have shown that Tregs in patients with AIH are fully functional and are not reduced in frequency (19, 31). Moreover, some studies have even shown aggregated Tregs in patients with AIH (19, 89, 94). A study reported the isolated Tregs from the peripheral blood of patients with AIH are suppressive, possess the functional markers CD39 and CTLA-4, and express the C-X-C chemokine receptor (CXCR3) (101). As mentioned above for the mouse model, altered levels of Tregs inside and outside of the liver have also been found in patients with AIH (19).
Meanwhile, Tregs seem to behave differently between adult and pediatric patients with AIH. Unlike adult patients, a numerical Tregs defect has long been considered in pediatric patients. However, recent studies showed enriched intrahepatic Tregs in pediatric AIH (93, 94). Moreover, the increase of Treg/total T cells was reported to be even more pronounced in pediatric AIH than in adult AIH due to fewer infiltrating T and B cells (93). Overall, given the severe inflammatory microenvironment in the liver of patients with AIH, the enrichment of Tregs is probably because of their recruitment from the periphery via various chemokine pathways. However, whether the Tregs can maintain their full function in the special hepatic microenvironment in AIH remains unknown. Although the Tregs isolated from the peripheral blood of patients with AIH can suppress the proliferation of effector T cells in vitro, the intrahepatic Tregs are the ones that modulate the immune microenvironment in the liver. Although isolating Tregs from the liver of patients is challenging, we can still speculate that the Tregs in the liver in AIH are impaired or not sufficient to control the inflammation.
As mentioned, the current management of AIH involves administering corticosteroids alone or in combination with azathioprine (102). Most patients achieve remission (103), but up to 90% develop a disease relapse after therapy withdrawal (38, 104, 105). Notably, the Tregs frequency has been reported as being significantly higher in patients with active AIH than in those who are in a state of remission (19). Moreover, patients with AIH who are untreated appeared to have a higher frequency of Tregs in the blood compared to patients under treatment (19, 93), which may be caused by a decrease of IL-2 levels (32). The disproportional decrease of intrahepatic Tregs during therapy might explain the high relapse rates after discontinuation of immunosuppressants (106), which suggests that increasing intrahepatic immunoregulation may be a better treatment strategy for the long-term control of AIH (97). Low-dose IL-2 has been suggested as a treatment for type 2 AIH and it can result in the expansion of Tregs (41, 107). Blocking Th17 is also a potential avenue for therapy development because it can converts Tregs into a more suppressive phenotype (108). Moreover, growing evidence supports Tregs adoptive transfer as a novel and effective mode of treatment for autoimmune diseases (80, 109, 110). Functionally enhanced Tregs can be expanded and generated de novo in patients with AIH (111). The generation of antigen-specific Tregs may represent a superior therapy option due to its more specific and potent suppressive function for effector T cells (112, 113). Based on the above discussion, enhanced Tregs adoptive transfer may open an avenue for long-term AIH treatment.
Interaction of Treg With Other Cells in AIH
AIH can be described as a clinical syndrome of the immune-mediated destruction of hepatocytes. The processes of various immune and non-immune cells in the hepatic microenvironment affect each other, forming an interacting network. Tregs, the main regulators of the immune system, can work with other cells in the liver, contributing to the disease process of immune-mediated liver injury (Figure 3). It is well-documented that an imbalance between Tregs and effector T cells is related to AIH pathogenesis (114, 115). The Treg/Th17 ratio has been regarded as a predictor of the degree of liver inflammation, as well as a therapeutic target in AIH (30, 83, 85, 89, 116). The inability of Tregs to efficiently suppress IL-17 production by Th17 cells may be crucial to the pathogenesis of AIH (100). In turn, inhibiting IL-17 has been proven to increase the expression of Foxp3 by CD25− cells (ngTregs), which allows ngTregs to differentiate into functionally stable immune inhibitory cells (108). Additionally, Tregs in patients with AIH are reportedly unable to regulate CD8+ T cell proliferation and cytokine production, which may contribute to the initiation of AIH damage.
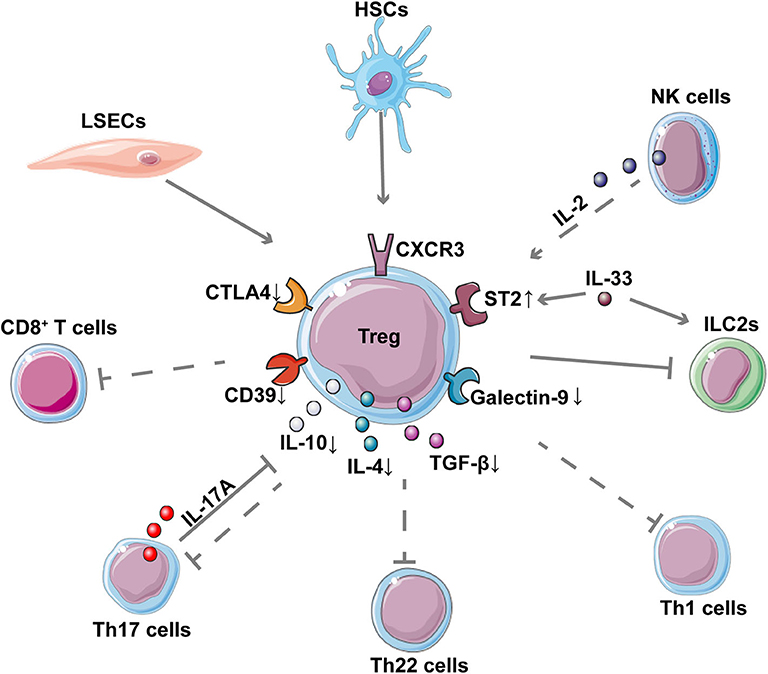
Figure 3. Interaction between Tregs and other cells in AIH. The network shows the interaction of Tregs with other cells as well as the down-regulation of important inhibitory molecules and cytokines in Tregs of patients with AIH. The dotted lines represent the reported decreased regulation in AIH. CD8+ T cells, Th17 cells, Th22 cells, and Th1 cells contribute to inflammatory liver injury in AIH; these cells, are suppressed by Tregs. LSECs and NK cells contribute to the expansion of Tregs while HSCs can enhance the suppressive function of Tregs in AIH. Th17 is reported to inhibit Treg through IL-17A. IL-33 can enhance the expression of ST2 on the surface of Treg, thereby regulating the pro-inflammatory ILC2s in immune-mediated hepatitis.
The crosstalk of innate immune cells and Tregs has also been reported recently. A clinical study of type 2 AIH showed that natural killer (NK) cells display an altered cytokine pattern characterized by increased IFN-γ and reduced IL-2 production, which can contribute to impaired Tregs function (107). Using a Con A mouse model, a study indicated that the IL-33-elicited hepatic ST2+ Tregs might counteract the inflammatory activity of type 2 innate lymphoid cells (ILC2s), which participate in the pathogenesis of immune-mediated hepatitis (117).
Some non-immune cells in the liver may also affect the behavior of Tregs in AIH. For example, liver sinusoidal endothelial cells (LSECs) were reported to prime CD4+ T cells into a CD45RBlow memory phenotype, which might belong to the expanding group of Foxp3− Tregs in the TF-OVA mouse model (118). Moreover, Huang et al. (91) also found that hepatic stellate cells (HSCs) can stimulate allogeneic Tregs proliferation and can also enhance the suppressive activity of Tregs to inhibit the proliferation of effector T cells in the ConA mouse model. An altered cytokines profile secreted by Tregs in AIH, such as IL-10, IL-4, and transforming growth factor β (TGF-β), and their decreased expression of inhibitory molecules such as cytotoxic T-lymphocytes-associated protein 4 (CTLA-4) and CD39 has also been reported (96, 100). These changes can influence the direct and indirect interactions of Tregs with other target cells. In addition, one study indicated that the reduced levels of T cell immunoglobulin and mucin domain 3 (Tim-3) on CD4+CD25− effector cells and of galectin-9 in Tregs contributes to the impaired immunoregulation in patients with AIH by enabling effector T cells to evade Tregs (99).
Tregs in Other Autoimmune Liver Diseases
Studies in other autoimmune liver diseases, such as primary biliary cirrhosis (PBC) or primary sclerosing cholangitis (PSC), have also provided evidence that the intrahepatic Tregs may be affected by the unique microenvironment of AIH. Unlike in AIH, peripheral and intrahepatic Tregs have long been well-documented to be numerically and functionally defective in patients with PBC and murine models of PBC (119–121). Gershwin et al. has confirmed the important role of Treg deficiency in the initiation of PBC, which may be due to the loss of the IL-2 receptor alpha (IL2RA) gene (122–124). They also achieve successful immunotherapy of PBC by adoptive transfer of Tregs (125). Moreover, a patient study revealed the level of Tregs was markedly lower in affected PBC portal tracts compared with AIH, while the CD8+T cell/FoxP3+ Treg ratio was significantly higher in the livers of late-stage PBC compared with early-stage AIH (126). Compared to PBC, the number of intrahepatic Tregs was reported to be even lower in PSC, which is also associated with the IL2RA gene (127). One study indicated that Tregs adoptive transfer and neutralization of IL-12 may be a treatment strategy to control the cholangitis in PSC (128). Therefore, a reduced number of Tregs has long been regarded as an initiating and promoting factor of the disease processes of PBC and PSC. Compared to PBC and PSC, the increased intrahepatic Tregs in AIH seem to be a consequence rather than a driver of the disease process. This hypothesis can explain why the increased Tregs cannot control the inflammation while the adoptive transfer of in vitro enhanced Tregs can relieve AIH.
Current Treg-Based Therapy in Autoimmune Diseases
Growing evidence shows the excellent safety and therapeutic effect of Treg-based therapy. There have been 51 clinical trials related to Treg-based therapy, with 12 of these trials related to autoimmune diseases (129). Clinical trials performed in patients with type 1 diabetes (T1D) (109, 110) demonstrated the efficacy of Treg therapy in these patients, with no infusion reactions or cell therapy-related high-grade adverse events. A case report described a patient with systemic lupus erythematosus (SLE) treated with autologous adoptive Treg cell therapy (80). The patient initially developed a transient but then a sustained increase in the percentage of highly activated Tregs. A recent study also reported that three patients with amyotrophic lateral sclerosis (ALS) benefited from expanded autologous Treg infusions (130). Several clinical trials have been initiated to assess the safety and efficacy of Treg therapy in other autoimmune diseases such as inflammatory bowel disease, Guillain–Barré syndrome, pemphigus vulgaris, and Alzheimer's disease (129). Given the important role of IL-2 in Treg survival and expansion and the better availability of IL-2 in vitro, clinical trials of low-dose IL-2 in various autoimmune diseases have been undertaken (41, 131–134). A phase I clinical trial investigating Treg-based therapy has also been initiated in AIH (NCT02704338) (129). However, much more research effort is needed before adoptive Treg therapy can be clinically translated to patients with AIH.
Conclusions and Future Perspectives
AIH is a chronic and progressive immune-mediated liver disease that can lead to cirrhosis, hepatocellular carcinoma, liver transplantation, and death (135–137). The pathogenesis of AIH largely remains unclear, while the main clinical treatment consists of immunosuppressive therapy. Although most patients respond well to immunosuppressants, the disease relapses after withdrawal. Animal models are crucial tools to better understand the pathogenetic mechanisms of AIH and to identify potential therapeutic targets. As yet, there is no widely accepted AIH mouse model for this research field. The chronic type 2 AIH mouse model might be a better option to simulate the natural disease process of AIH. Tregs therapy is now emerging as a potential treatment route for a wide variety of autoimmune diseases, while the efficacy of this therapy in AIH remains unclear. Although there is an increased understanding of the roles of Tregs in animal models and patients with AIH, whether their intrahepatic Tregs are impaired or not is not clear. The fact that the increased number of Tregs in the liver of patients with AIH are unable to control the hepatitis indicates the impaired function of Tregs in the special hepatic microenvironment of AIH. However, even though AIH is not driven by the impairment of Tregs, Treg-based therapies for AIH might be effective, providing a potential avenue for the long-term control of AIH.
Author Contributions
DT designed the topic. HW wrote the manuscript. XF generated figures and tables. WY helped to review and edited the manuscript. All authors contributed to the article and approved the submitted version.
Funding
This work was supported by National Natural Science Foundation of China, Nos. 81974071 and 81772610 to DT and Nos. 81974383 and 81772607 to WY.
Conflict of Interest
The authors declare that the research was conducted in the absence of any commercial or financial relationships that could be construed as a potential conflict of interest.
Abbreviations
AIH, autoimmune hepatitis; ConA, concanavalin A; CYP2D6, cytochrome P450 2D6; FTCD, formiminotransferase cyclodeaminase; Treg, regulatory T cell; ANA, antinuclear antibodies; SMA, anti-smooth muscle antibodies; LKM-1, anti-liver-kidney microsomal 1; LC1, anti-liver cytosol type 1; Th cells, T-helper cells; ALT, alanine aminotransferase; Ad, adenovirus; S-100, syngeneic liver homogenate; HLA, human leukocyte antigen; PD-1, programmed cell death protein 1; Ntx, neonatal theymectomy; Met-Kb mouse, mouse express class I gene (H-2Kb) under the control of metallothionein promoter; Des-TCR mouse, mouse expresses on 98% of their CD8+ T cells a TCR recognizing a complex formed by the class I gene (H-2Kb) molecule and self-peptides; TF-OVA mouse, the model antigen ovalbumin is expressed in hepatocytes of the mouse; ASBT-OVA mice, the model antigen ovalbumin is expressed in cholangiocytes of the mouse; OT-I T cell, CD8+ T cell; OT-II T cell, CD4+ T cell; Alb-HA mouse, mouse expresses the influenza virus hemagglutinin (HA) autoantigen under control of mouse albumin regulatory elements and alpha-fetoprotein enhancers (Alb) specifically in the liver; CL4-TCR mouse, mouse expresses the clone 4/T-cell receptor; Traf6ΔTEC, conditional deletion of tumor necrosis factor receptor-associated factor 6 expression in the thymic epithelial cells; TAM–/– mouse, mouse that is deficient in Tyro3, Axl, and Mer receptor tyrosine kinases; IL, interleukin; IFN-γ, interferon-γ; TFN-α, tumor necrosis factor-α; TGF-β, transforming growth factor β; CXCR3, C-X-C chemokine receptor; NK cell, natural killer cell; ILC2, type 2 innate lymphoid cell; LSEC, liver sinusoidal endothelial cell; HSC, hepatic stellate cell; Teff, effector T cell; ASC, autoimmune hepatitis-sclerosing cholangitis; HC, healthy control; CTLA-4, T-lymphocytes-associated protein 4; Tim-3, T cell immunoglobulin and mucin domain 3; PBC, primary biliary cirrhosis; PSC, primary sclerosing cholangitis; T1D, type 1 diabetes; SLE, systemic lupus erythematosus; ALS, amyotrophic lateral sclerosis; FC, flow cytometry; WB, western blotting; PCR, polymerase chain reaction; IHC, immunohistochemistry.
References
1. Schmeltzer PA, Russo MW. Clinical narrative: autoimmune hepatitis. Am J Gastroenterol. (2018) 113:951–8. doi: 10.1038/s41395-018-0058-z
2. Manns MP, Czaja AJ, Gorham JD, Krawitt EL, Mieli-Vergani G, Vergani D, et al. Diagnosis and management of autoimmune hepatitis. Hepatology. (2010) 51:2193–213. doi: 10.1002/hep.23584
3. Mieli-Vergani G, Vergani D, Czaja AJ, Manns MP, Krawitt EL, Vierling JM, et al. Autoimmune hepatitis. Nat Rev Dis Primers. (2018) 4:18017. doi: 10.1038/nrdp.2018.17
4. Heneghan MA, Yeoman AD, Verma S, Smith AD, Longhi MS. Autoimmune hepatitis. Lancet. (2013) 382:1433–44. doi: 10.1016/S0140-6736(12)62163-1
5. Manns MP, Lohse AW, Vergani D. Autoimmune hepatitis–update 2015. J Hepatol. (2015) 62:100–11. doi: 10.1016/j.jhep.2015.03.005
6. Doycheva I, Watt KD, Gulamhusein AF. Autoimmune hepatitis: current and future therapeutic options. Liver Int. (2019) 39:1002–13. doi: 10.1111/liv.14062
7. Harrison L, Gleeson D. Stopping immunosuppressive treatment in autoimmune hepatitis (AIH): is it justified (and in whom and when)? Liver Int. (2019) 39:610–20. doi: 10.1111/liv.14051
8. Christen U, Hintermann E. Immunopathogenic mechanisms of autoimmune hepatitis: how much do we know from animal models? Int J Mol Sci. (2016) 17:2007. doi: 10.3390/ijms17122007
9. Christen U. Animal models of autoimmune hepatitis. Biochim Biophys Acta Mol Basis Dis. (2019) 1865:970–81. doi: 10.1016/j.bbadis.2018.05.017
10. Tiegs G, Hentschel J, Wendel A. A T cell-dependent experimental liver injury in mice inducible by concanavalin A. J Clin Invest. (1992) 90:196–203. doi: 10.1172/JCI115836
11. Holdener M, Hintermann E, Bayer M, Rhode A, Rodrigo E, Hintereder G, et al. Breaking tolerance to the natural human liver autoantigen cytochrome P450 2D6 by virus infection. J Exp Med. (2008) 205:1409–22. doi: 10.1084/jem.20071859
12. Hardtke-Wolenski M, Fischer K, Noyan F, Schlue J, Falk CS, Stahlhut M, et al. Genetic predisposition and environmental danger signals initiate chronic autoimmune hepatitis driven by CD4+ T cells. Hepatology. (2013) 58:718–28. doi: 10.1002/hep.26380
13. Hardtke-Wolenski M, Dywicki J, Fischer K, Hapke M, Sievers M, Schlue J, et al. The influence of genetic predisposition and autoimmune hepatitis inducing antigens in disease development. J Autoimmun. (2017) 78:39–45. doi: 10.1016/j.jaut.2016.12.001
14. Wang H, Yan W, Feng Z, Gao Y, Zhang L, Feng X, et al. Plasma proteomic analysis of autoimmune hepatitis in an improved AIH mouse model. J Transl Med. (2020) 18:3. doi: 10.1186/s12967-019-02180-3
15. Wing JB, Tanaka A, Sakaguchi S. Human FOXP3(+) regulatory T cell heterogeneity and function in autoimmunity and cancer. Immunity. (2019) 50:302–16. doi: 10.1016/j.immuni.2019.01.020
16. Kleinewietfeld M, Hafler DA. The plasticity of human Treg and Th17 cells and its role in autoimmunity. Semin Immunol. (2013) 25:305–12. doi: 10.1016/j.smim.2013.10.009
17. Than NN, Jeffery HC, Oo YH. Autoimmune hepatitis: progress from global immunosuppression to personalised regulatory T cell therapy. Can J Gastroenterol Hepatol. (2016) 2016:7181685. doi: 10.1155/2016/7181685
18. Longhi MS, Hussain MJ, Mitry RR, Arora SK, Mieli-Vergani G, Vergani D, et al. Functional study of CD4+CD25+ regulatory T cells in health and autoimmune hepatitis. J Immunol. (2006) 176:4484–91. doi: 10.4049/jimmunol.176.7.4484
19. Peiseler M, Sebode M, Franke B, Wortmann F, Schwinge D, Quaas A, et al. FOXP3+ regulatory T cells in autoimmune hepatitis are fully functional and not reduced in frequency. J Hepatol. (2012) 57:125–32. doi: 10.1016/j.jhep.2012.02.029
20. Gronbaek L, Otete H, Ban L, Crooks C, Card T, Jepsen P, et al. Incidence, prevalence, and mortality of autoimmune hepatitis in England 1997–2015. A population-based cohort study. Liver Int. (2020) 40:1634–44. doi: 10.1111/liv.14480
22. Sebode M, Weiler-Normann C, Liwinski T, Schramm C. Autoantibodies in autoimmune liver disease-clinical and diagnostic relevance. Front Immunol. (2018) 9:609. doi: 10.3389/fimmu.2018.00609
23. Muratori P, Lalanne C, Fabbri A, Cassani F, Lenzi M, Muratori L. Type 1 and type 2 autoimmune hepatitis in adults share the same clinical phenotype. Aliment Pharmacol Ther. (2015) 41:1281–7. doi: 10.1111/apt.13210
24. Couto CA, Bittencourt PL, Porta G, Abrantes-Lemos CP, Carrilho FJ, Guardia BD, et al. Antismooth muscle and antiactin antibodies are indirect markers of histological and biochemical activity of autoimmune hepatitis. Hepatology. (2014) 59:592–600. doi: 10.1002/hep.26666
25. Ngu JH, Gearry RB, Frampton CM, Stedman CA. Autoimmune hepatitis: the role of environmental risk factors: a population-based study. Hepatol Int. (2013) 7:869–75. doi: 10.1007/s12072-013-9448-x
26. Wen JW, Kohn MA, Wong R, Somsouk M, Khalili M, Maher J, et al. Hospitalizations for autoimmune hepatitis disproportionately affect Black and Latino Americans. Am J Gastroenterol. (2018) 113:243–53. doi: 10.1038/ajg.2017.456
27. de Boer YS, Gerussi A, van den Brand FF, Wong GW, Halliday N, Liberal R, et al. Association between black race and presentation and liver-related outcomes of patients with autoimmune hepatitis. Clin Gastroenterol Hepatol. (2019) 17:1616–24. doi: 10.1016/j.cgh.2018.11.028
28. Than NN, Ching DK, Hodson J, McDowell P, Mann J, Gupta R, et al. Difference in clinical presentation, immunology profile and treatment response of type 1 autoimmune hepatitis between United Kingdom and Singapore patients. Hepatol Int. (2016) 10:673–9. doi: 10.1007/s12072-016-9727-4
29. Beland K, Marceau G, Labardy A, Bourbonnais S, Alvarez F. Depletion of B cells induces remission of autoimmune hepatitis in mice through reduced antigen presentation and help to T cells. Hepatology. (2015) 62:1511–23. doi: 10.1002/hep.27991
30. Liberal R, Grant CR, Yuksel M, Graham J, Kalbasi A, Ma Y, et al. Regulatory T-cell conditioning endows activated effector T cells with suppressor function in autoimmune hepatitis/autoimmune sclerosing cholangitis. Hepatology. (2017) 66:1570–84. doi: 10.1002/hep.29307
31. John K, Hardtke-Wolenski M, Jaeckel E, Manns MP, Schulze-Osthoff K, Bantel H. Increased apoptosis of regulatory T cells in patients with active autoimmune hepatitis. Cell Death Dis. (2017) 8:3219. doi: 10.1038/s41419-017-0010-y
32. Liberal R, Grant CR, Holder BS, Cardone J, Martinez-Llordella M, Ma Y, et al. In autoimmune hepatitis type 1 or the autoimmune hepatitis-sclerosing cholangitis variant defective regulatory T-cell responsiveness to IL-2 results in low IL-10 production and impaired suppression. Hepatology. (2015) 62:863–75. doi: 10.1002/hep.27884
33. Zhang H, Lian M, Zhang J, Bian Z, Tang R, Miao Q, et al. A functional characteristic of cysteine-rich protein 61: modulation of myeloid-derived suppressor cells in liver inflammation. Hepatology. (2018) 67:232–46. doi: 10.1002/hep.29418
34. Umeshappa CS, Singha S, Blanco J, Shao K, Nanjundappa RH, Yamanouchi J, et al. Suppression of a broad spectrum of liver autoimmune pathologies by single peptide-MHC-based nanomedicines. Nat Commun. (2019) 10:2150. doi: 10.1038/s41467-019-09893-5
35. Yeoman AD, Westbrook RH, Al-Chalabi T, Carey I, Heaton ND, Portmann BC, et al. Diagnostic value and utility of the simplified International Autoimmune Hepatitis Group (IAIHG) criteria in acute and chronic liver disease. Hepatology. (2009) 50:538–45. doi: 10.1002/hep.23042
36. Ebadi M, Bhanji RA, Mazurak VC, Lytvyak E, Mason A, Czaja AJ, et al. Severe vitamin D deficiency is a prognostic biomarker in autoimmune hepatitis. Aliment Pharmacol Ther. (2019) 49:173–82. doi: 10.1111/apt.15029
37. Abe K, Takahashi A, Fujita M, Imaizumi H, Hayashi M, Okai K, et al. Dysbiosis of oral microbiota and its association with salivary immunological biomarkers in autoimmune liver disease. PLoS ONE. (2018) 13:e0198757. doi: 10.1371/journal.pone.0198757
38. European Association for the Study of the Liver. EASL clinical practice guidelines: autoimmune hepatitis. J Hepatol. (2015) 63:971–1004. doi: 10.1016/j.jhep.2015.06.030
39. Zachou K, Gatselis N, Papadamou G, Rigopoulou EI, Dalekos GN. Mycophenolate for the treatment of autoimmune hepatitis: prospective assessment of its efficacy and safety for induction and maintenance of remission in a large cohort of treatment-naive patients. J Hepatol. (2011) 55:636–46. doi: 10.1016/j.jhep.2010.12.032
40. Mei Y, Wang Y, Xu L. Suppression of immune-mediated liver injury after vaccination with attenuated pathogenic cells. Immunol Lett. (2007) 110:29–35. doi: 10.1016/j.imlet.2007.02.008
41. Lim TY, Martinez-Llordella M, Kodela E, Gray E, Heneghan MA, Sanchez-Fueyo A. Low-dose interleukin-2 for refractory autoimmune hepatitis. Hepatology. (2018) 68:1649–52. doi: 10.1002/hep.30059
42. Yuksel M, Wang Y, Tai N, Peng J, Guo J, Beland K, et al. A novel “humanized mouse” model for autoimmune hepatitis and the association of gut microbiota with liver inflammation. Hepatology. (2015) 62:1536–50. doi: 10.1002/hep.27998
43. Alexandropoulos K, Bonito AJ, Weinstein EG, Herbin O. Medullary thymic epithelial cells and central tolerance in autoimmune hepatitis development: novel perspective from a new mouse model. Int J Mol Sci. (2015) 16:1980–2000. doi: 10.3390/ijms16011980
44. Buschenfelde KH, Kossling FK, Miescher PA. Experimental chronic active hepatitis in rabbits following immunization with human liver proteins. Clin Exp Immunol. (1972) 11:99–108.
45. Mihas AA, Subramony C, Achord JL. Experimental autoimmune hepatitis in mice following immunization with syngeneic liver proteins. J Med. (1995) 26:309–22.
46. Lohse AW, Manns M, Dienes HP, Meyer zum Buschenfelde KH, Cohen IR. Experimental autoimmune hepatitis: disease induction, time course and T-cell reactivity. Hepatology. (1990) 11:24–30. doi: 10.1002/hep.1840110106
47. Xu C, Zhang C, Ji J, Wang C, Yang J, Geng B, et al. CD36 deficiency attenuates immune-mediated hepatitis in mice by modulating the proapoptotic effects of CXC chemokine ligand 10. Hepatology. (2018) 67:1943–55. doi: 10.1002/hep.29716
48. Bartneck M, Schlosser CT, Barz M, Zentel R, Trautwein C, Lammers T, et al. Immunomodulatory therapy of inflammatory liver disease using selectin-binding glycopolymers. ACS Nano. (2017) 11:9689–700. doi: 10.1021/acsnano.7b04630
49. Fujita T, Soontrapa K, Ito Y, Iwaisako K, Moniaga CS, Asagiri M, et al. Hepatic stellate cells relay inflammation signaling from sinusoids to parenchyma in mouse models of immune-mediated hepatitis. Hepatology. (2016) 63:1325–39. doi: 10.1002/hep.28112
50. Küsters S, Gantner F, Künstle G, Tiegs G. Interferon gamma plays a critical role in T cell–dependent liver injury in mice initiated by concanavalin A. Gastroenterology. (1996) 111:462–71. doi: 10.1053/gast.1996.v111.pm8690213
51. Bovensiepen CS, Schakat M, Sebode M, Zenouzi R, Hartl J, Peiseler M, et al. TNF-producing Th1 cells are selectively expanded in liver infiltrates of patients with autoimmune hepatitis. J Immunol. (2019) 203:3148–56. doi: 10.4049/jimmunol.1900124
52. Salas JR, Chen BY, Wong A, Cheng D, Van Arnam JS, Witte ON, et al. (18)F-FAC PET selectively images liver-infiltrating CD4 and CD8 T cells in a mouse model of autoimmune hepatitis. J Nucl Med. (2018) 59:1616–23. doi: 10.2967/jnumed.118.210328
53. Li H, Li G, Zhao X, Wu Y, Ma W, Liu Y, et al. Complementary serum proteomic analysis of autoimmune hepatitis in mice and patients. J Transl Med. (2013) 11:146. doi: 10.1186/1479-5876-11-146
54. Zheng C, Yin S, Yang Y, Yu Y, Xie X. CD24 aggravates acute liver injury in autoimmune hepatitis by promoting IFN-gamma production by CD4(+) T cells. Cell Mol Immunol. (2018) 15:260–71. doi: 10.1038/cmi.2016.57
55. Deng G, Li Y, Ma S, Gao Z, Zeng T, Chen L, et al. Caveolin-1 dictates ferroptosis in the execution of acute immune-mediated hepatic damage by attenuating nitrogen stress. Free Radic Biol Med. (2020) 148:151–61. doi: 10.1016/j.freeradbiomed.2019.12.026
56. Manns MP, Johnson EF, Griffin KJ, Tan EM, Sullivan KF. Major antigen of liver kidney microsomal autoantibodies in idiopathic autoimmune hepatitis is cytochrome P450db1. J Clin Invest. (1989) 83:1066–72. doi: 10.1172/JCI113949
57. Lapierre P, Hajoui O, Homberg JC, Alvarez F. Formiminotransferase cyclodeaminase is an organ-specific autoantigen recognized by sera of patients with autoimmune hepatitis. Gastroenterology. (1999) 116:643–9. doi: 10.1016/S0016-5085(99)70186-1
58. Lapierre P, Djilali-Saiah I, Vitozzi S, Alvarez F. A murine model of type 2 autoimmune hepatitis: xenoimmunization with human antigens. Hepatology. (2004) 39:1066–74. doi: 10.1002/hep.20109
59. Piché C, Béland K, Lapierre P, Massie B, Alvarez F. Different sites of xenoantigen delivery lead to a virally induced late-onset hepatitis in mice through molecular mimicry. Liver International. (2011) 31:1306–14. doi: 10.1111/j.1478-3231.2011.02600.x
60. Lapierre P, Beland K, Djilali-Saiah I, Alvarez F. Type 2 autoimmune hepatitis murine model: the influence of genetic background in disease development. J Autoimmun. (2006) 26:82–9. doi: 10.1016/j.jaut.2005.11.001
61. Lapierre P, Beland K, Martin C, Alvarez F Jr, Alvarez F. Forkhead box p3+ regulatory T cell underlies male resistance to experimental type 2 autoimmune hepatitis. Hepatology. (2010) 51:1789–98. doi: 10.1002/hep.23536
62. Christen U, Holdener M, Hintermann E. Cytochrome P450 2D6 as a model antigen. Dig Dis. (2010) 28:80–5. doi: 10.1159/000282068
63. Ehser J, Holdener M, Christen S, Bayer M, Pfeilschifter JM, Hintermann E, et al. Molecular mimicry rather than identity breaks T-cell tolerance in the CYP2D6 mouse model for human autoimmune hepatitis. J Autoimmun. (2013) 42:39–49. doi: 10.1016/j.jaut.2012.11.001
64. Muller P, Messmer M, Bayer M, Pfeilschifter JM, Hintermann E, Christen U. Non-alcoholic fatty liver disease (NAFLD) potentiates autoimmune hepatitis in the CYP2D6 mouse model. J Autoimmun. (2016) 69:51–8. doi: 10.1016/j.jaut.2016.02.007
65. Watanabe Y, Kawakami H, Kawamoto H, Ikemoto Y, Masuda K, Takezaki E, et al. Effect of neonatal thymectomy on experimental autoimmune hepatitis in mice. Clin Exp Immunol. (1987) 67:105–13.
66. Djilali-Saiah I, Lapierre P, Vittozi S, Alvarez F. DNA vaccination breaks tolerance for a neo-self antigen in liver: a transgenic murine model of autoimmune hepatitis. J Immunol. (2002) 169:4889–96. doi: 10.4049/jimmunol.169.9.4889
67. Tamaki S, Homma S, Enomoto Y, Komita H, Zeniya M, Ohno T, et al. Autoimmune hepatic inflammation by vaccination of mice with dendritic cells loaded with well-differentiated hepatocellular carcinoma cells and administration of interleukin-12. Clin Immunol. (2005) 117:280–93. doi: 10.1016/j.clim.2005.08.010
68. Gil-Farina I, Di Scala M, Salido E, Lopez-Franco E, Rodriguez-Garcia E, Blasi M, et al. Transient expression of transgenic IL-12 in mouse liver triggers unremitting inflammation mimicking human autoimmune hepatitis. J Immunol. (2016) 197:2145–56. doi: 10.4049/jimmunol.1600228
69. Warren A, Bertolino P, Benseler V, Fraser R, McCaughan GW, Le Couteur DG. Marked changes of the hepatic sinusoid in a transgenic mouse model of acute immune-mediated hepatitis. J Hepatol. (2007) 46:239–46. doi: 10.1016/j.jhep.2006.08.022
70. Derkow K, Loddenkemper C, Mintern J, Kruse N, Klugewitz K, Berg T, et al. Differential priming of CD8 and CD4 T-cells in animal models of autoimmune hepatitis and cholangitis. Hepatology. (2007) 46:1155–65. doi: 10.1002/hep.21796
71. Zierden M, Kuhnen E, Odenthal M, Dienes HP. Effects and regulation of autoreactive CD8+ T cells in a transgenic mouse model of autoimmune hepatitis. Gastroenterology. (2010) 139:975–86. doi: 10.1053/j.gastro.2010.05.075
72. Yuksel M, Xiao X, Tai N, Vijay M, Gulden E, Beland K, et al. The induction of autoimmune hepatitis in the human leucocyte antigen-DR4 non-obese diabetic mice autoimmune hepatitis mouse model. Clin Exp Immunol. (2016) 186:164–76. doi: 10.1111/cei.12843
73. Qi N, Liu P, Zhang Y, Wu H, Chen Y, Han D. Development of a spontaneous liver disease resembling autoimmune hepatitis in mice lacking tyro3, axl and mer receptor tyrosine kinases. PLoS ONE. (2013) 8:e66604. doi: 10.1371/journal.pone.0066604
74. Bonito AJ, Aloman C, Fiel MI, Danzl NM, Cha S, Weinstein EG, et al. Medullary thymic epithelial cell depletion leads to autoimmune hepatitis. J Clin Invest. (2013) 123:3510–24. doi: 10.1172/JCI65414
75. Hardtke-Wolenski M, Taubert R, Noyan F, Sievers M, Dywicki J, Schlue J, et al. Autoimmune hepatitis in a murine autoimmune polyendocrine syndrome type 1 model is directed against multiple autoantigens. Hepatology. (2015) 61:1295–305. doi: 10.1002/hep.27639
76. Kido M, Watanabe N, Okazaki T, Akamatsu T, Tanaka J, Saga K, et al. Fatal autoimmune hepatitis induced by concurrent loss of naturally arising regulatory T cells and PD-1-mediated signaling. Gastroenterology. (2008) 135:1333–43. doi: 10.1053/j.gastro.2008.06.042
77. Maruoka R, Aoki N, Kido M, Iwamoto S, Nishiura H, Ikeda A, et al. Splenectomy prolongs the effects of corticosteroids in mouse models of autoimmune hepatitis. Gastroenterology. (2013) 145:209–20. doi: 10.1053/j.gastro.2013.03.011
78. Ikeda A, Aoki N, Kido M, Iwamoto S, Nishiura H, Maruoka R, et al. Progression of autoimmune hepatitis is mediated by IL-18-producing dendritic cells and hepatic CXCL9 expression in mice. Hepatology. (2014) 60:224–36. doi: 10.1002/hep.27087
79. Brunstein CG, Miller JS, McKenna DH, Hippen KL, DeFor TE, Sumstad D, et al. Umbilical cord blood-derived T regulatory cells to prevent GVHD. kinetics, toxicity profile, and clinical effect. Blood. (2016) 127:1044–51. doi: 10.1182/blood-2015-06-653667
80. Dall'Era M, Pauli ML, Remedios K, Taravati K, Sandova PM, Putnam AL, et al. Autoimmunity E centers of: adoptive Treg cell therapy in a patient with systemic lupus erythematosus. Arthritis Rheumatol. (2019) 71:431–40. doi: 10.1002/art.40737
81. Esensten JH, Muller YD, Bluestone JA, Tang Q. Regulatory T-cell therapy for autoimmune and autoinflammatory diseases: the next frontier. J Allergy Clin Immunol. (2018) 142:1710–8. doi: 10.1016/j.jaci.2018.10.015
82. Lapierre P, Beland K, Yang R, Alvarez F. Adoptive transfer of ex vivo expanded regulatory T cells in an autoimmune hepatitis murine model restores peripheral tolerance. Hepatology. (2013) 57:217–27. doi: 10.1002/hep.26023
83. Hu ED, Chen DZ, Wu JL, Lu FB, Chen L, Zheng MH, et al. High fiber dietary and sodium butyrate attenuate experimental autoimmune hepatitis through regulation of immune regulatory cells and intestinal barrier. Cell Immunol. (2018) 328:24–32. doi: 10.1016/j.cellimm.2018.03.003
84. An Haack I, Derkow K, Riehn M, Rentinck MN, Kuhl AA, Lehnardt S, et al. The role of regulatory CD4 T cells in maintaining tolerance in a mouse model of autoimmune hepatitis. PLoS ONE. (2015) 10:e0143715. doi: 10.1371/journal.pone.0143715
85. Xia G, Wu S, Wang X, Fu M. Inhibition of microRNA-155 attenuates concanavalin-A-induced autoimmune hepatitis by regulating Treg/Th17 cell differentiation. Can J Physiol Pharmacol. (2018) 96:1293–300. doi: 10.1139/cjpp-2018-0467
86. Erhardt A, Biburger M, Papadopoulos T, Tiegs G. IL-10, regulatory T cells, and Kupffer cells mediate tolerance in concanavalin A-induced liver injury in mice. Hepatology. (2007) 45:475–85. doi: 10.1002/hep.21498
87. Ju Y, Shang X, Liu Z, Zhang J, Li Y, Shen Y, et al. The Tim-3/galectin-9 pathway involves in the homeostasis of hepatic Tregs in a mouse model of concanavalin A-induced hepatitis. Mol Immunol. (2014) 58:85–91. doi: 10.1016/j.molimm.2013.11.001
88. Hegde VL, Hegde S, Cravatt BF, Hofseth LJ, Nagarkatti M, Nagarkatti PS. Attenuation of experimental autoimmune hepatitis by exogenous and endogenous cannabinoids: involvement of regulatory T cells. Mol Pharmacol. (2008) 74:20–33. doi: 10.1124/mol.108.047035
89. Liang M, Liwen Z, Yun Z, Yanbo D, Jianping C. The imbalance between Foxp3(+)Tregs and Th1/Th17/Th22 cells in patients with newly diagnosed autoimmune hepatitis. J Immunol Res. (2018) 2018:3753081. doi: 10.1155/2018/3753081
90. Thomas D, Wu TY, Cottagiri M, Nyandjo M, Njoku DB. Induction of drug-induced, autoimmune hepatitis in BALB/c mice for the study of its pathogenic mechanisms. J Vis Exp. (2020) e59174. doi: 10.3791/59174
91. Huang H, Deng Z. Adoptive transfer of regulatory T cells stimulated by Allogeneic Hepatic Stellate Cells mitigates liver injury in mice with concanavalin A-induced autoimmune hepatitis. Biochem Biophys Res Commun. (2019) 512:14–21. doi: 10.1016/j.bbrc.2019.02.147
92. Wang H, Feng X, Han P, Lei Y, Xia Y, Tian D, et al. The JAK inhibitor tofacitinib ameliorates immunemediated liver injury in mice. Mol Med Rep. (2019) 20:4883–92. doi: 10.3892/mmr.2019.10750
93. Diestelhorst J, Junge N, Schlue J, Falk CS, Manns MP, Baumann U, et al. Pediatric autoimmune hepatitis shows a disproportionate decline of regulatory T cells in the liver and of IL-2 in the blood of patients undergoing therapy. PLoS ONE. (2017) 12:e0181107. doi: 10.1371/journal.pone.0181107
94. Behairy BE, El-Araby HA, Abd El Kader HH, Ehsan NA, Salem ME, Zakaria HM, et al. Assessment of intrahepatic regulatory T cells in children with autoimmune hepatitis. Ann Hepatol. (2016) 15:682–90. doi: 10.5604/16652681.1212319
95. Ferri S, Longhi MS, De Molo C, Lalanne C, Muratori P, Granito A, et al. A multifaceted imbalance of T cells with regulatory function characterizes type 1 autoimmune hepatitis. Hepatology. (2010) 52:999–1007. doi: 10.1002/hep.23792
96. Okumura A, Ishikawa T, Sato S, Yamauchi T, Oshima H, Ohashi T, et al. Deficiency of forkhead box P3 and cytotoxic T-lymphocyte-associated antigen-4 gene expressions and impaired suppressor function of CD4(+)CD25(+) T cells in patients with autoimmune hepatitis. Hepatol Res. (2008) 38:896–903. doi: 10.1111/j.1872-034X.2008.00349.x
97. Longhi MS, Ma Y, Mitry RR, Bogdanos DP, Heneghan M, Cheeseman P, et al. Effect of CD4+ CD25+ regulatory T-cells on CD8 T-cell function in patients with autoimmune hepatitis. J Autoimmun. (2005) 25:63–71. doi: 10.1016/j.jaut.2005.05.001
98. Longhi MS, Ma Y, Bogdanos DP, Cheeseman P, Mieli-Vergani G, Vergani D. Impairment of CD4(+)CD25(+) regulatory T-cells in autoimmune liver disease. J Hepatol. (2004) 41:31–7. doi: 10.1016/j.jhep.2004.03.008
99. Liberal R, Grant CR, Holder BS, Ma Y, Mieli-Vergani G, Vergani D, et al. The impaired immune regulation of autoimmune hepatitis is linked to a defective galectin-9/tim-3 pathway. Hepatology. (2012) 56:677–86. doi: 10.1002/hep.25682
100. Grant CR, Liberal R, Holder BS, Cardone J, Ma Y, Robson SC, et al. Dysfunctional CD39(POS) regulatory T cells and aberrant control of T-helper type 17 cells in autoimmune hepatitis. Hepatology. (2014) 59:1007–15. doi: 10.1002/hep.26583
101. Oo YH, Ackrill S, Cole R, Jenkins L, Anderson P, Jeffery HC, et al. Liver homing of clinical grade Tregs after therapeutic infusion in patients with autoimmune hepatitis. JHEP Reports. (2019) 1:286–96. doi: 10.1016/j.jhepr.2019.08.001
102. Wong LL, Fisher HF, Stocken DD, Rice S, Khanna A, Heneghan MA, et al. The impact of autoimmune hepatitis and its treatment on health utility. Hepatology. (2018) 68:1487–97. doi: 10.1002/hep.30031
103. Feld JJ, Dinh H, Arenovich T, Marcus VA, Wanless IR, Heathcote EJ. Autoimmune hepatitis: effect of symptoms and cirrhosis on natural history and outcome. Hepatology. (2005) 42:53–62. doi: 10.1002/hep.20732
104. Hoeroldt B, McFarlane E, Dube A, Basumani P, Karajeh M, Campbell MJ, et al. Long-term outcomes of patients with autoimmune hepatitis managed at a nontransplant center. Gastroenterology. (2011) 140:1980–9. doi: 10.1053/j.gastro.2011.02.065
105. Hartl J, Ehlken H, Weiler-Normann C, Sebode M, Kreuels B, Pannicke N, et al. Patient selection based on treatment duration and liver biochemistry increases success rates after treatment withdrawal in autoimmune hepatitis. J Hepatol. (2015) 62:642–6. doi: 10.1016/j.jhep.2014.10.018
106. Taubert R, Hardtke-Wolenski M, Noyan F, Wilms A, Baumann AK, Schlue J, et al. Intrahepatic regulatory T cells in autoimmune hepatitis are associated with treatment response and depleted with current therapies. J Hepatol. (2014) 61:1106–14. doi: 10.1016/j.jhep.2014.05.034
107. Mele D, Bossi G, Maggiore G, Oliviero B, Mantovani S, Bonelli B, et al. Altered natural killer cell cytokine profile in type 2 autoimmune hepatitis. Clin Immunol. (2018) 188:31–7. doi: 10.1016/j.clim.2017.12.004
108. Longhi MS, Liberal R, Holder B, Robson SC, Ma Y, Mieli-Vergani G, et al. Inhibition of interleukin-17 promotes differentiation of CD25(-) cells into stable T regulatory cells in patients with autoimmune hepatitis. Gastroenterology. (2012) 142:1526–35. doi: 10.1053/j.gastro.2012.02.041
109. Marek-Trzonkowska N, Mysliwiec M, Dobyszuk A, Grabowska M, Techmanska I, Juscinska J, et al. Administration of CD4+CD25highCD127- regulatory T cells preserves β-cell function in type 1 diabetes in children. Diabetes Care. (2012) 35:1817–20. doi: 10.2337/dc12-0038
110. Bluestone JA, Buckner JH, Fitch M, Gitelman SE, Gupta S, Hellerstein MK, et al. Type 1 diabetes immunotherapy using polyclonal regulatory T cells. Sci Transl Med. (2015) 7:315ra189. doi: 10.1126/scitranslmed.aad4134
111. Longhi MS, Meda F, Wang P, Samyn M, Mieli-Vergani G, Vergani D, et al. Expansion and de novo generation of potentially therapeutic regulatory T cells in patients with autoimmune hepatitis. Hepatology. (2008) 47:581–91. doi: 10.1002/hep.22071
112. Holder BS, Grant CR, Liberal R, Ma Y, Heneghan MA, Mieli-Vergani G, et al. Retinoic acid stabilizes antigen-specific regulatory T-cell function in autoimmune hepatitis type 2. J Autoimmun. (2014) 53:26–32. doi: 10.1016/j.jaut.2014.02.001
113. Longhi MS, Hussain MJ, Kwok WW, Mieli-Vergani G, Ma Y, Vergani D. Autoantigen-specific regulatory T cells, a potential tool for immune-tolerance reconstitution in type-2 autoimmune hepatitis. Hepatology. (2011) 53:536–47. doi: 10.1002/hep.24039
114. Webb GJ, Hirschfield GM, Krawitt EL, Gershwin ME. Cellular and molecular mechanisms of autoimmune hepatitis. Annu Rev Pathol. (2018)13:247–92. doi: 10.1146/annurev-pathol-020117-043534
115. Liang M, Liwen Z, Juan D, Yun Z, Yanbo D, Jianping C. Dysregulated TFR and TFH cells correlate with B-cell differentiation and antibody production in autoimmune hepatitis. J Cell Mol Med. (2020) 24:3948–57. doi: 10.1111/jcmm.14997
116. Ji W, Peng X, Lou T, Wang J, Qiu W. Total flavonoids from Tetrastigma hemsleyanum ameliorates inflammatory stress in concanavalin A-induced autoimmune hepatitis mice by regulating Treg/Th17 immune homeostasis. Inflammopharmacology. (2019) 27:1297–307. doi: 10.1007/s10787-019-00599-0
117. Neumann K, Karimi K, Meiners J, Voetlause R, Steinmann S, Dammermann W, et al. A proinflammatory role of type 2 innate lymphoid cells in murine immune-mediated hepatitis. J Immunol. (2017) 198:128–37. doi: 10.4049/jimmunol.1600418
118. Kruse N, Neumann K, Schrage A, Derkow K, Schott E, Erben U, et al. Priming of CD4+ T cells by liver sinusoidal endothelial cells induces CD25low forkhead box protein 3- regulatory T cells suppressing autoimmune hepatitis. Hepatology. (2009) 50:1904–13. doi: 10.1002/hep.23191
119. Wang YH, Yang W, Yang JB, Jia YJ, Tang W, Gershwin ME, et al. Systems biologic analysis of T regulatory cells genetic pathways in murine primary biliary cirrhosis. J Autoimmun. (2015) 59:26–37. doi: 10.1016/j.jaut.2015.01.011
120. Rong G, Zhou Y, Xiong Y, Zhou L, Geng H, Jiang T, et al. Imbalance between T helper type 17 and T regulatory cells in patients with primary biliary cirrhosis: the serum cytokine profile and peripheral cell population. Clin Exp Immunol. (2009) 156:217–25. doi: 10.1111/j.1365-2249.2009.03898.x
121. Cichoz-Lach H, Grywalska E, Michalak A, Kowalik A, Mielnik M, Rolinski J. Deviations in peripheral blood cell populations are associated with the stage of primary biliary cholangitis and presence of itching. Arch Immunol Ther Exp. (2018) 66:443–52. doi: 10.1007/s00005-018-0515-9
122. Aoki CA, Roifman CM, Lian ZX, Bowlus CL, Norman GL, Shoenfeld Y, et al. IL-2 receptor alpha deficiency and features of primary biliary cirrhosis. J Autoimmun. (2006) 27:50–3. doi: 10.1016/j.jaut.2006.04.005
123. Wakabayashi K, Lian ZX, Moritoki Y, Lan RY, Tsuneyama K, Chuang YH, et al. IL-2 receptor alpha(-/-) mice and the development of primary biliary cirrhosis. Hepatology. (2006) 44:1240–9. doi: 10.1002/hep.21385
124. Hsu W, Zhang W, Tsuneyama K, Moritoki Y, Ridgway WM, Ansari AA, et al. Differential mechanisms in the pathogenesis of autoimmune cholangitis versus inflammatory bowel disease in interleukin-2Ralpha(-/-) mice. Hepatology. (2009) 49:133–40. doi: 10.1002/hep.22591
125. Tanaka H, Zhang W, Yang GX, Ando Y, Tomiyama T, Tsuneyama K, et al. Successful immunotherapy of autoimmune cholangitis by adoptive transfer of forkhead box protein 3(+) regulatory T cells. Clin Exp Immunol. (2014) 178:253–61. doi: 10.1111/cei.12415
126. Lan RY, Cheng C, Lian ZX, Tsuneyama K, Yang GX, Moritoki Y, et al. Liver-targeted and peripheral blood alterations of regulatory T cells in primary biliary cirrhosis. Hepatology. (2006) 43:729–37. doi: 10.1002/hep.21123
127. Sebode M, Peiseler M, Franke B, Schwinge D, Schoknecht T, Wortmann F, et al. Reduced FOXP3(+) regulatory T cells in patients with primary sclerosing cholangitis are associated with IL2RA gene polymorphisms. J Hepatol. (2014) 60:1010–6. doi: 10.1016/j.jhep.2013.12.027
128. Schwinge D, von Haxthausen F, Quaas A, Carambia A, Otto B, Glaser F, et al. Dysfunction of hepatic regulatory T cells in experimental sclerosing cholangitis is related to IL-12 signaling. J Hepatol. (2017) 66:798–805. doi: 10.1016/j.jhep.2016.12.001
129. Raffin C, Vo LT, Bluestone JA. Treg cell-based therapies: challenges and perspectives. Nat Rev Immunol. (2020) 20:158–72. doi: 10.1038/s41577-019-0232-6
130. Thonhoff JR, Beers DR, Zhao W, Pleitez M, Simpson EP, Berry JD, et al. Expanded autologous regulatory T-lymphocyte infusions in ALS. A phase I, first-in-human study. Neurol Neuroimmunol Neuroinflamm. (2018) 5:e465. doi: 10.1212/NXI.0000000000000465
131. He J, Zhang R, Shao M, Zhao X, Miao M, Chen J, et al. Efficacy and safety of low-dose IL-2 in the treatment of systemic lupus erythematosus: a randomised, double-blind, placebo-controlled trial. Ann Rheum Dis. (2020) 79:141–9. doi: 10.1136/annrheumdis-2019-215396
132. Rosenzwajg M, Lorenzon R, Cacoub P, Pham HP, Pitoiset F, El Soufi K, et al. Immunological and clinical effects of low-dose interleukin-2 across 11 autoimmune diseases in a single, open clinical trial. Ann Rheum Dis. (2019) 78:209–17. doi: 10.1136/annrheumdis-2018-214229
133. Seelig E, Howlett J, Porter L, Truman L, Heywood J, Kennet J, et al. The DILfrequency study is an adaptive trial to identify optimal IL-2 dosing in patients with type 1 diabetes. JCI Insight. (2018) 3:e99306. doi: 10.1172/jci.insight.99306
134. Todd JA, Evangelou M, Cutler AJ, Pekalski ML, Walker NM, Stevens HE, et al. Regulatory T cell responses in participants with type 1 diabetes after a single dose of interleukin-2: a non-randomised, open label, adaptive dose-finding trial. PLoS Med. (2016) 13:e1002139. doi: 10.1371/journal.pmed.1002139
135. Kirstein MM, Metzler F, Geiger E, Heinrich E, Hallensleben M, Manns MP, et al. Prediction of short- and long-term outcome in patients with autoimmune hepatitis. Hepatology. (2015) 62:1524–35. doi: 10.1002/hep.27983
136. Gronbaek L, Vilstrup H, Jepsen P. Autoimmune hepatitis in Denmark: incidence, prevalence, prognosis, and causes of death. A nationwide registry-based cohort study. J Hepatol. (2014) 60:612–7. doi: 10.1016/j.jhep.2013.10.020
Keywords: autoimmune hepatitis, regulatory T cell, mouse model, cytochrome P450 2D6, treatment
Citation: Wang H, Feng X, Yan W and Tian D (2020) Regulatory T Cells in Autoimmune Hepatitis: Unveiling Their Roles in Mouse Models and Patients. Front. Immunol. 11:575572. doi: 10.3389/fimmu.2020.575572
Received: 23 June 2020; Accepted: 07 September 2020;
Published: 07 October 2020.
Edited by:
Urs Christen, Goethe University Frankfurt, GermanyReviewed by:
Matthias Hardtke-Wolenski, Essen University Hospital, GermanyPascal Lapierre, University of Montreal Hospital Centre (CRCHUM), Canada
Yun Ma, King's College London, United Kingdom
Copyright © 2020 Wang, Feng, Yan and Tian. This is an open-access article distributed under the terms of the Creative Commons Attribution License (CC BY). The use, distribution or reproduction in other forums is permitted, provided the original author(s) and the copyright owner(s) are credited and that the original publication in this journal is cited, in accordance with accepted academic practice. No use, distribution or reproduction is permitted which does not comply with these terms.
*Correspondence: Wei Yan, eWFud2VpQHRqaC50am11LmVkdS5jbg==; Dean Tian, ZGF0aWFuQHRqaC50am11LmVkdS5jbg==