- 1Hainan Key Laboratory for Sustainable Utilization of Tropical Bioresources, Haikou, China
- 2Institute of Tropical Agriculture and Forestry, Hainan University, Haikou, China
Bacterial β-galactosidase is involved in lactose metabolism and acts as a prevalent reporter enzyme used in studying the activities of prokaryotic and eukaryotic promoters. Xanthomonas campestris pv. campestris (Xcc) is the pathogen of black rot disease in crucifers. β-Galactosidase activity can be detected in Xcc culture, which makes Escherichia coli LacZ unable to be used as a reporter enzyme in Xcc. To systemically understand the β-galactosidase in Xcc and construct a β-galactosidase -deficient strain for promoter activity analysis using LacZ as a reporter, we here analyzed the putative β-galactosidases in Xcc 8004. As glycosyl hydrolase (GH) family 2 (GH2) and 35 (GH35) family enzymes were reported to have beta-galactosidase activities, we studied all of them encoded by Xcc 8004. When expressed in E. coli, only two of the enzymes, XC1214 and XC2985, were found to have β-galactosidase activity. When deleted from the Xcc 8004 genome, only the XC1214 mutant had no β-galactosidase activity, and other GH2 and GH35 gene deletions resulted in no significant reduction in β-galactosidase activity. Therefore, XC1214 is the main β-galactosidase in Xcc 8004. Notably, we have constructed a β-galactosidase-free strain that can be employed in gene traps using LacZ as a reporter in Xcc. The results reported herein should facilitate the development of high-capacity screening assays that utilize the LacZ reporter system in Xcc.
Introduction
β-Galactosidase is widely distributed across species and is important as a key provider in the production of energy and a source of carbon through the breakdown of lactose to galactose and glucose (Rodríguez et al., 2006; Juers et al., 2012). The enzyme is frequently used in genetics, molecular biology, and other life sciences as it can efficiently hydrolyze X-gal (5-bromo-4-chloro-3-indoyl-β-D-galactopyranoside), a soluble, colorless compound, into galactose and 5-bromo-4-chloro-3-hydroxyindole, which is then oxidized into 5,5'-dibromo-4,4'-dichloro-indigo, an insoluble blue product that makes bacteria encoding β-galactosidase become blue on growth plates containing X-gal (Gary and Kindell, 2005; Smale, 2010; Juers et al., 2012). β-Galactosidase activity can also be easily detected in solution by using ortho-nitrophenyl-β-galactoside (ONPG) as a substrate. As the product ortho-nitrophenol is yellow (λmax = 420 nm), enzyme activity can be measured by the rate of appearance of a yellow color using a spectrophotometer (A420) (Smale, 2010).
Xanthomonas campestris pv. campestris (Xcc) is the pathogen of black rot disease, which is considered the most destructive disease of crucifers, including all cultivated varieties of brassicas worldwide (Vicente and Holub, 2013). Xcc can become blue when growing on a medium containing X-gal and thus expresses β-galactosidase (Fu and Tseng, 1990). There are only three annotated galactosidase genes (designated galA, galB, and galC) in the Xcc genome of 8004 and ATCC 33913 strains (da Silva et al., 2002; Qian et al., 2005). GalA and GalB are similar to glycosyl hydrolase (GH) family 2 enzymes and have no detectable activities in Xcc Xc17 (Yang et al., 2003). GalC is a GH35 enzyme homologous to the X. axonopodis pv. manihotis Bga and has β-galactosidase activity in Xcc Xc17 (Yang et al., 2003). Another galactosidase, GalD, has been reported to be a member of the GH35 family and exhibits a low level of β-galactosidase activity in Xcc Xc17 (Yang et al., 2007). Mutations of galA, galB, and galC did not reduce the ability to grow on lactose or the β-galactosidase activity in Xcc Xc17L, a strain with elevated β-galactosidase activity, indicating that these three genes do not encode the main β-galactosidase in Xc17L (Yang et al., 2003). An Xc17L galD mutant had the same β-galactosidase activity as Xc17, suggesting that GalD may be a β-galactosidase (Yang et al., 2007). However, the identity of the major β-galactosidase in Xcc remains unclear.
In this study, we analyzed the GH2 and GH35 family proteins in Xcc 8004 and compared their β-galactosidase activities in Xcc cells or expression in E. coli. We also constructed a β-galactosidase-free strain that can be employed in gene traps using LacZ as a reporter to detect the regulation of gene expression in Xcc.
Materials and Methods
Bacterial Strains and Growth Conditions
The bacterial strains and plasmids used in this work are listed in Table S1. Xcc strains were grown at 28°C in the rich medium NYG or synthetic medium M4 (Daniels et al., 1984; Tao et al., 2014). E. coli strains were grown in LB medium at 37°C (Tao et al., 2014). Antibiotics were used at the following final concentrations, as required: 100 μg/ml ampicillin (Amp), 50 μg/ml kanamycin (Kan), 100 μg/ml rifampicin (Rif) and 100 μg/ml spectinomycin (Sp).
DNA Manipulation and Vector Construction
PCR cloning, restriction enzyme digestion, agarose gel electrophoresis, and SDS-PAGE have been discussed elsewhere (Tao et al., 2014). PCR primers and the application are listed in Table S2. All constructs were verified by sequencing.
Generation of Xcc Deletion Mutants
Primer design, suicide vector construction, and mutant screening were conducted as in a previous report (Tao et al., 2014). Briefly, approximately 500 bp sequences flanking both sides of the region to be deleted were amplified by PCR and cloned into pK18mobSacB. Xcc-competent cells were transformed with suicide plasmids by electroporation and plated on NYG with kanamycin. After two rounds of recombination, the open reading frame was deleted from the genomic DNA. Mutants were confirmed by PCR and DNA sequencing.
β-Galactosidase Activity Assay
β-Galactosidase activity was determined as previously described (Zhang and Bremer, 1995; Cromie et al., 2006; Smale, 2010). Briefly, cells were cultured in LB liquid media (37°C, 200 rpm). When the OD600 reached 0.8, the cells in 1 ml were harvested by centrifugation at 4°C. The supernatant was discarded, and the cells were resuspended in 1 ml of Z buffer (60 mM Na2HPO4, 40 mM NaH2PO4, 10 mM KCl, 1 mM MgSO4, and 50 Mm β-mercaptoethanol). The cells were lysed by sonication. Until the mixture became clear, to ensure complete cell lysis, the reaction was started by adding 0.2 ml of ONPG (4 mg/ml). The reaction was stopped by adding 0.5 ml of Na2CO3 (1 M) stock solution when the sample in the tube developed a pale yellow color. The OD420 of the reactions was measured. The assays were performed in triplicate, and one miller unit (U) was expressed as 1,000 × OD420/(OD600 of assaying culture × volume assayed × time).
In plate assays, bacteria were spotted on LB (for E. coli) or NYG (for Xcc) plates supplemented with 20 mg/l X-gal. For assays for β-galactosidase mutants, each spot was inoculated with 2 μl of a 10-fold dilution series (i.e., 10−2-, 10−3-, 10−4-, and 10−5-fold from left to right) from cells in logarithmic growth phase (OD600 = 0.8).
Extracellular Enzyme Activity Test
Xcc strains were cultivated in NYG medium supplemented with xylan, cellulose, starch, skimmed milk, and β-mannose (Tang et al., 1991; Qian et al., 2008). If a mutant has the ability to secrete the enzyme, a hydrolysis zone will be produced around the colony (Qian et al., 2008). By comparing the size of D/d [diameter of the hydrolysate (D)/colony diameter (d)] to identify strains with enzyme production capacity, the larger the value of D/d, the stronger was the ability of the strain to produce enzymes.
A single colony was picked and cultured in 5 ml of NYG liquid medium; 1 ml of culture (OD600 = 0.8) was centrifuged to collect the cells. Pellets were washed twice with ddH2O and resuspended in 100 μl of NYG. Next, 1 μl of the suspension was spotted onto an NYG solid plate with 0.2% RBB-xylan (Sigma), 0.5% carboxymethyl cellulose (CMC, Sigma), 0.1% soluble starch, 2% skim milk powder or 0.5% locust bean gum for the analysis of xylanase, cellulose, amylase, protease, and mannanase activities, respectively.
Ability of Sugar Utilization
A single colony was picked and cultured in 5 ml of NYG liquid medium, and 1 ml of the culture was centrifuged to collect the cells. Pellets were washed twice with 1 ml ddH2O and resuspended in 100 μl of M4 media. Next, 1 μl of the suspension was spotted onto an M4 solid plate containing 1% glucose, fructose, glycerol, lactose, galactose, sucrose, or maltose. The growth status of the mutants and the ability to utilize sugar were photographed.
Bacterial Disease Assays
The virulence of Xcc for cabbage (Brassica oleracea) was tested as described previously (Qian et al., 2005). Briefly, bacterial cells (OD600 = 0.5) were introduced into the leaves by leaf clipping. Twelve leaves were used, and the lesion length was measured 14 days postinoculation.
Bioinformatics Analysis
Amino acid sequence alignment was conducted with the ClustalX program (Larkin et al., 2007). The putative β-galactosidase sequences in Xcc 8004 were downloaded from GenBank (accession no. CP000050). A bootstrapped neighbor joining tree was constructed with MEGA 6 software based on the sequence alignment by the ClustalX program (Larkin et al., 2007; Tamura et al., 2013). The conserved domains were predicted by the CDD search (Marchler-Bauer et al., 2017).
Statistical Methods
Data were analyzed by ANOVA using the PROC GLM (P ≤ 0.05) procedure in SAS software.
Results
In Silico Analysis of the GH2 and GH35 Family Proteins in Xcc
In the Xcc 8004 genome (Qian et al., 2005), there are five putative genes encoding GH2 proteins and three putative genes for GH35 proteins, according to the CDD-BLAST (Figure 1; Marchler-Bauer et al., 2017). All these proteins have predicated GH2 or GH35 domains in the N-terminus, and most of them have no other known domains (Figure 1A). GH2 proteins have low similarity, but GH35 proteins have high similarity with each other in Xcc (Figures 1B and Figure S1). The GH2 and GH35 domains in these proteins are not conserved (Figure S1). Moreover, none of the GH2 proteins in Xcc is highly similar (>75%, amino acid) to the best known β-galactosidase, LacZ, in E. coli. The GH35 proteins in Xcc are homologous to the Bga of X. axonopodis pv. manihotis (Figures 1B and Figure S1), which is a known β-galactosidase(Yang et al., 2003). These data suggest that GH2 proteins have diverse functions but that GH35 proteins might have β-galactosidase activities in Xcc.
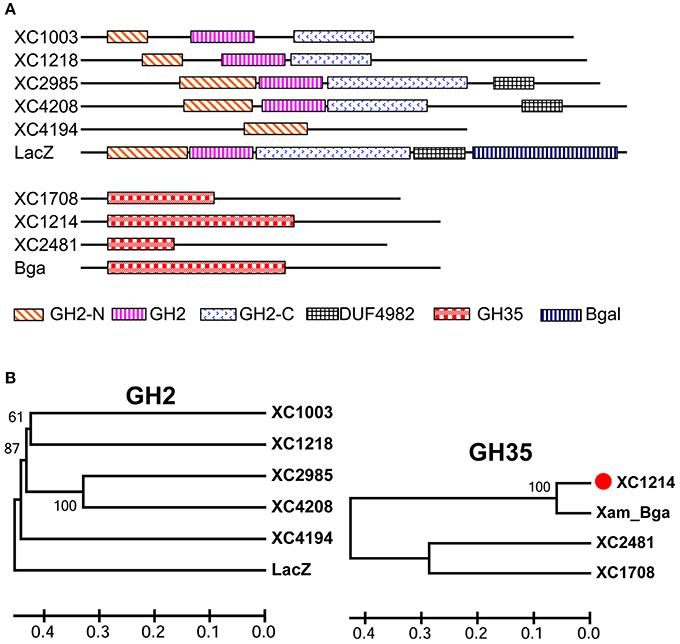
Figure 1. The putative β-galactosidases in Xcc 8004. (A) The domains in the eight putative β-galactosidases of Xcc 8004, the LacZ of E. coli and Bga of X. axonopodis pv. manihotis predicted by CDD-BLAST. (B) The evolutionary relationship of the two protein (GH2 and GH35) families of Xcc putative β-galactosidases computed with the UPGMA rule in MEGA6 software and full-length proteins. The horizontal axis shows the Nei distance.
Expression and β-Galactosidase Activity Analysis of the GH2 and GH35 Family Proteins in E. coli
To analyze the enzyme activities of GH2 and GH35 proteins, we cloned the individual corresponding genes into the expression vector and expressed them in E. coli DH5α, which has no β-galactosidase activity. When these strains grew on LB media supplemented with X-gal, only the XC1214-expressing strain became blue (Figure 2A), indicating that the other seven proteins might not be β-galactosidases. The β-galactosidase activities of the lysates of the eight gene-expressing cells were also analyzed. The results showed that XC1214 and XC2985 had detectable β-galactosidase activities but that the others did not. The activity of XC2985 was ~137 U, but that of XC1214 was ~896 U (Figure 2B). All of the genes were sufficiently expressed in E. coli (Figure S2), indicating that the activity difference of these strains is not due to the fact that these GH2 and GH35 genes cannot be expressed in E. coli. Taken together, our data imply that XC1214 and XC2985, not the other six GH2 and GH35 proteins, might be the β-galactosidase in Xcc.
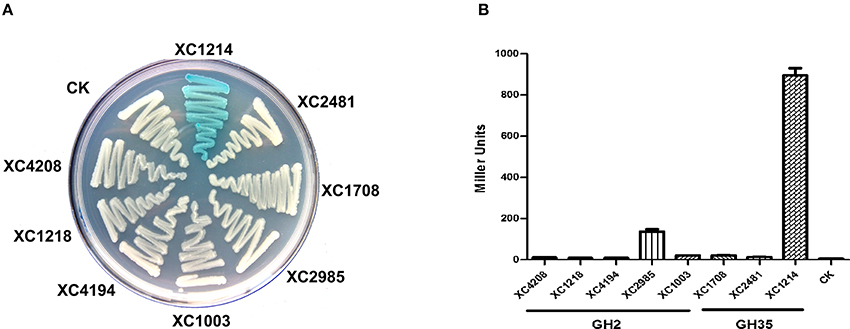
Figure 2. β-galactosidase activities of Xcc GH2 and GH35 proteins expressed in E. coli. (A) Morphology of GH2- and GH35-expressing E. coli cultured on X-gal containing media. (B) The β-galactosidase activities of GH2- and GH35-expressing E. coli. CK: E. coli containing the empty vector. Triplicate experiments were performed, and the means ± standard deviations (SDs) were calculated.
Mutagenesis Analysis of the GH2 and GH35 Genes in Xcc
To systemically analyze the functions of the GH2 and GH35 proteins in Xcc, we deleted all eight genes from the Xcc 8004 genome. ΔXC1214 and the mutant (Δlac8) that had neither GH2 nor GH35 genes were not able to turn blue when grown on NYG medium containing X-gal (Figure 3A and Figure S3); the mutant (Δlac7) that was deficient in all these genes except XC1214 showed a stronger blue color than that of wild-type Xcc 8004. The β-galactosidase activity of Δlac7 was also higher than that of Xcc 8004 (Figure 3B). These data indicate that some of the other GH2 and GH35 genes may negatively regulate XC1214 expression or XC1214 activity. However, we did not detect a difference of XC1214 expression in Xcc 8004 and Δlac7 (data not shown), suggesting that the other GH2 and GH35 proteins may regulate XC1214 activity.
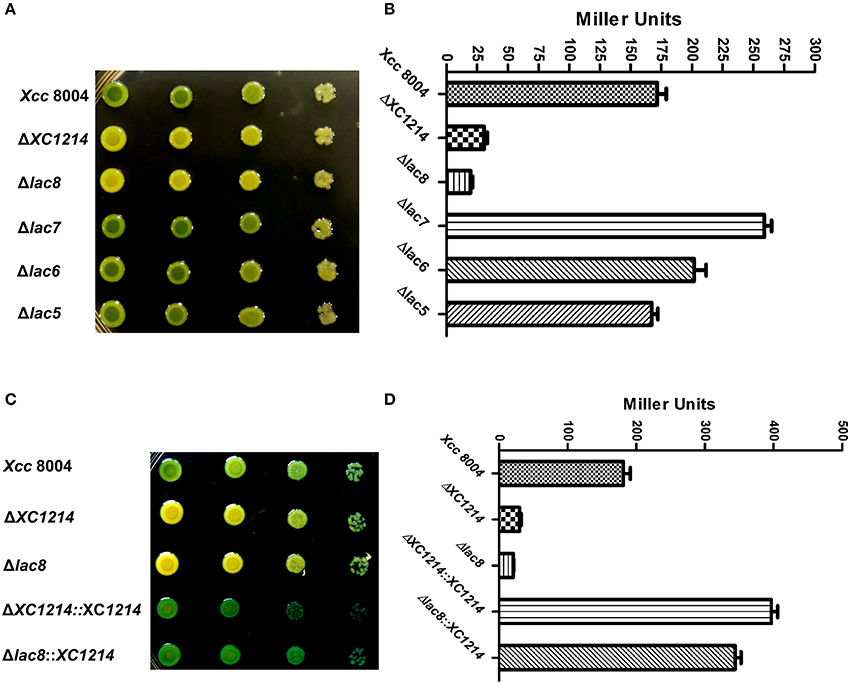
Figure 3. The enzyme activities of GH2 and GH35 proteins in Xcc 8004. Morphology of the GH2 and GH35 deletion mutants (A) on X-gal containing plates and the β-galactosidase activities of these strains (B). The morphology (C) and β-galactosidase activity (D) of the XC1214 mutant and its complementary strain. Each spot was inoculated with 2 μl of a 10-fold dilution series (i.e., 10−2-, 10−3-, 10−4-, and 10−5-fold from left to right) from cells in the logarithmic growth phase (OD600 = 0.8). Triplicate experiments were performed, and the means ± SDs were calculated.
Because XC2985 expressed in E. coli had β-galactosidase activity (Figure 2B), it may also have had activity in Xcc. However, the mutant (Δlac5) in which XC1003/XC2985/XC4208/XC2481/XC1708 was deleted had the same phenotypes as Xcc 8004 (Figure 3B). Thus, XC2985 was not the β-galactosidase or was not expressed in Xcc under our experimental conditions. We also transformed the individual GH2 and GH35 genes controlled by a strong promoter into Δlac8 and detected the β-galactosidase activities of these transformed strains. Only XC1214 introduction resulted in restored Δlac8 β-galactosidase activity (Figures 3C,D). Furthermore, we substituted the alanine for the conserved active amino acid (Glu186) of XC1214. This mutation resulted in loss of β-galactosidase activity of XC1214 (Figures 4A,B). Taken together, these data confirmed that XC1214 was the major β-galactosidase in Xcc 8004.
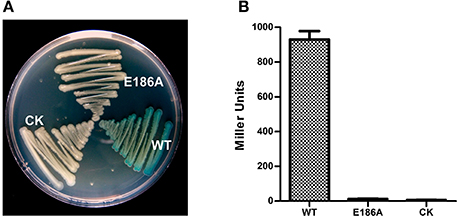
Figure 4. The active site for enzyme activity of the XC1214 protein. Mutation of the active site (E186) of XC1214 resulted in loss of β-galactosidase activity both on plates (A) and in liquid media (B). Triplicate experiments were performed, and the means ± SDs were calculated.
Phenotypic Analysis of the GH2 and GH35 Mutants
As discussed above, except for XC1214, the functions of other GH2 and GH35 proteins are unclear. Because β-galactosidase is the essential component for lactose utilization, we wanted to know whether these GH2 and GH35 proteins are also involved in sugar utilization. Therefore, the ability of the bacteria to utilize sugar was studied. We determined whether a given bacterium had the ability to disintegrate a certain saccharide by using glucose, lactose, maltose, β-galactose, sucrose, or glycerol as the sole carbon source. It was found that the mutant strains grew as well as Xcc 8004 on the glucose-, sucrose-, galactose-, maltose-, and glycerol-containing plates (Figure 5). When lactose was the sole carbon source, ΔXC1214 and Δlac8 were found to grow poorly compared with Xcc 8004 and the other mutants (Figure 5). Therefore, XC1214 was the major enzyme involved in lactose metabolism.
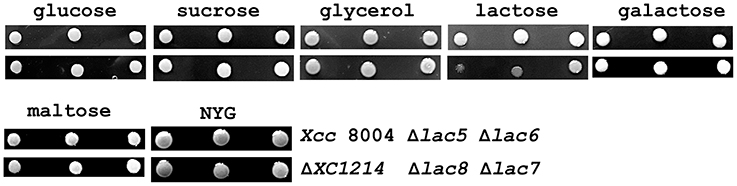
Figure 5. The sugar usage of the GH2 and GH35 mutants in Xcc 8004. The growth of the indicated strains (right end panel) on solid plates when the individual sugars were used as the sole carbon source. Δlac5: ΔXC1003/ΔXC2985/ΔXC4208/ΔXC2481/ΔXC1708; Δlac6: Δlac5/ΔXC4194; Δlac7: Δlac6/ΔXC1218; Δlac8: Δlac7/ΔXC1214.
Many enzymes are secreted into the extracellular compartment to hydrolyze their respective substrates. Thus, we compared the activities of extracellular enzymes such as pectinase, cellulase, protease, amylase, and xylanase in Xcc 8004 and in the GH2 and GH35 mutants. The results showed no obvious difference between Xcc 8004 and the GH2 and GH35 mutants (Figures 6A,B), indicating that GH2 and GH35 proteins should not have or regulate these extracellular enzyme activities.
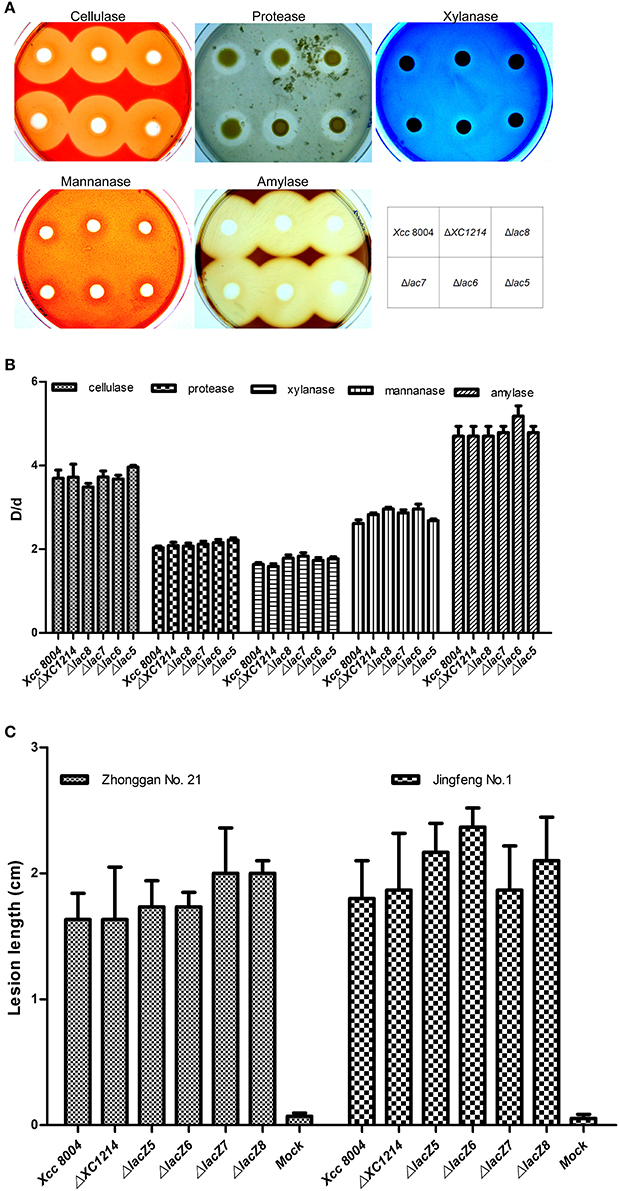
Figure 6. Virulence-related analysis of the GH2 and GH35 mutants. (A,B) The extracellular enzyme activities of the GH2 and GH35 mutants. (C) The virulence of the GH2 and GH35 mutants on cabbage 9 days after inoculation. Jingfeng No. 1 and Zhonggan No. 21 are two species of Brassica oleracea. Virulence assays were performed on 12 leaves, and the means ± SDs were calculated.
To determine whether GH2 and GH35 proteins are involved in pathogenesis, Xcc 8004 and the mutants were inoculated onto different varieties of cabbage. The disease symptoms on the leaves were observed 9 days after inoculation. The results showed that there were no significant differences between Xcc 8004 and the mutants (Figure 6C), indicating that GH2 and GH35 proteins were not the main virulence factors of Xcc 8004.
Discussion
The E. coli lacZ gene encoding β-galactosidase (β-gal) can be used as a standard reporter to monitor promoters or enhancers in transient or stable transfection experiments (Evans et al., 2013; Aviv and Gal-Mor, 2018). Xcc 8004 has the property of degrading lactose and forms blue spots on X-GAL plates (Figures 3, 6). Therefore, LacZ cannot be used as a tool for promoter activity analysis in Xcc 8004. Therefore, the current reporter system widely used in Xcc is based on β-glucuronidase, but this system is time consuming and costly and cannot be directly detected on plates. As the LacZ reporter system is an easy, low-cost, visible and stable detectable method (Smale, 2010; Juers et al., 2012; Aviv and Gal-Mor, 2018), we wanted to construct a β-galactosidase-free strain that could be employed in gene traps using LacZ as a reporter for high-throughput detection of the regulation of gene expression in Xcc. To this end, we analyzed the activity of the putative β-galactosidase in E. coli and Xcc. We found the major β-galactosidase in Xcc and constructed a β-galactosidase-deficient strain (Δlac8) that had no difference in virulence, growth, or extracellular enzyme activity compared with the wild-type strain. Therefore, LacZ can be used as a reporter gene for high-throughput screening of promoter activity in the Δlac8 strain.
Although previous studies reported several putative β-galactosidase in Xcc Xc17 (Fu and Tseng, 1990; Yang et al., 2003, 2007), the genomic analysis was not performed. In this study, we systemically analyzed the putative β-galactosidases (GH2 and GH35 family proteins) in Xcc 8004. By analyzing the carbohydrate utilization capacity and β-galactosidase activities of the eight genes, Only XC1214 was found to have detectable enzyme activity in Xcc.8004. XC2481 (GalD) has been reported as the β-galactosidase that contributes to the elevated β-galactosidase activity in XC17L (Yang et al., 2007). However, our results showed that XC2481 had no β-galactosidase activity in E. coli and Xcc 8004. In addition, deletion of galC (XC1214) did not change the β-galactosidase activity in XC17L (Yang et al., 2003), which is opposite to our results in Xcc 8004. The difference may be due to the different Xcc strains, but the mechanism should be mined in detail.
What functions of the other seven GH2 and GH35 proteins were not found in this study. One possibility is that these proteins also have β-galactosidase activity in growth environments differing from our test conditions. The other possibility is that these proteins have a novel activity to cope with environmental change during the Xcc-environment interaction. In addition to β-galactosidase, GH2 were described to function as β-mannosidase, β-glucuronidase, endo-β-mannosidase, β-glucosidase, or exo-β-glucosaminidase (Schröder et al., 2015). For example, the GH2 protein, NixH, was found to have, β-mannosidase activity (Dupoiron et al., 2015). As low similarity between Xcc GH2 proteins and E. coli LacZ, XC1003, XC1218, XC4194, and XC4208 in Xcc 8004 might act as the enzymes as aforementioned. The GH35 comprises enzymes with the activities of β-galactosidase, galacto-β-D-galactanase, and exo-β-glucosaminidases (Kotake et al., 2005; Gamauf et al., 2007). Thus, XC1708 and XC2481 that had no β-galactosidase activity (Figures 2, 3) might have one of the other activities. To uncover the functions of these GH2 and GH35 proteins in Xcc 8004, further chemical and genetic analyses should be addressed in the future work.
Author Contributions
HW, CS, and QX did most of the preparation and characterizations; YW and SL contributed to bacterial growth and enzyme activity assays; CL helped with the experimental data; CH and JT designed the experimental scheme and wrote the manuscript. All authors reviewed the manuscript.
Conflict of Interest Statement
The authors declare that the research was conducted in the absence of any commercial or financial relationships that could be construed as a potential conflict of interest.
Acknowledgments
We thank Dr. Lingling Zhen for the discussion of the manuscript. This work was supported by the Natural Science Foundation of Hainan Province (20163049 to CL and 20163050 to JT), the Scientific Research Foundation of Hainan University (kyqd1546 and kyqd1547), and the Scientific Basic Research (Youth Foundation) of Hainan University (hdkyxj201701 to JT). The funders had no role in the study design, data collection and analysis, decision to publish, or preparation of the manuscript.
Supplementary Material
The Supplementary Material for this article can be found online at: https://www.frontiersin.org/article/10.3389/fmicb.2018.00957/full#supplementary-material
References
Aviv, G., and Gal-Mor, O. (2018). lacZ reporter system as a tool to study virulence gene regulation in bacterial pathogens. Methods Mol. Biol. 1734, 39–45. doi: 10.1007/978-1-4939-7604-1_5
Cromie, M. J., Shi, Y., Latifi, T., and Groisman, E. A. (2006). An RNA sensor for intracellular Mg2+. Cell 125, 71–84. doi: 10.1016/j.cell.2006.01.043
Daniels, M. J., Barber, C. E., Turner, P. C., Cleary, W. G., and Sawczyc, M. K. (1984). Isolation of mutants of Xanthomonas campestris pathovarcampestris showing altered pathogenicity. J. Gen. Microbiol. 130, 2447–2455. doi: 10.1099/00221287-130-9-2447
da Silva, A. C., Ferro, J. A., Reinach, F. C, Farah, C. S., Furlan, L. R., Quaggio, R. B., et al. (2002). Comparison of the genomes of two Xanthomonas pathogens with differing host specificities. Nature 417, 459–463. doi: 10.1038/417459a
Dupoiron, S., Zischek, C., Ligat, L., Carbonne, J., Boulanger, A., Duge de Bernonville, T., et al. (2015). The N-Glycan cluster from Xanthomonas campestris pv campestris: a toolbox for sequential plant N-glycan processing. J. Biol. Chem. 290, 6022–6036. doi: 10.1074/jbc.M114.624593
Evans, A. S., Pybus, C., and Hansen, E. J. (2013). Development of a LacZ-based transcriptional reporter system for use with Moraxella catarrhalis. Plasmid 69, 180–185. doi: 10.1016/j.plasmid.2012.11.003
Fu, J. F., and Tseng, Y. H. (1990). Construction of lactose-utilizing Xanthomonas campestris and production of xanthan gum from whey. Appl. Environ. Microbiol. 56, 919–923.
Gamauf, C., Marchetti, M., Kallio, J., Puranen, T., Vehmaanperä, J., Allmaier, G., et al. (2007). Characterization of the bga1-encoded glycoside hydrolase family 35 beta-galactosidase of Hypocrea jecorina with galacto-beta-D-galactanase activity. FEBS J. 274, 1691–1700. doi: 10.1111/j.1742-4658.2007.05714.x
Gary, R. K., and Kindell, S. M. (2005). Quantitative assay of senescence-associated beta-galactosidase activity in mammalian cell extracts. Anal. Biochem. 343, 329–334. doi: 10.1016/j.ab.2005.06.003
Juers, D. H., Matthews, B. W., and Huber, R. E. (2012). LacZ beta-galactosidase: structure and function of an enzyme of historical and molecular biological importance. Protein Sci. 21, 1792–1807. doi: 10.1002/pro.2165
Kotake, T., Dina, S., Konishi, T., Kaneko, S., Igarashi, K., Samejima, M., et al. (2005). Molecular cloning of a {beta}-galactosidase from radish that specifically hydrolyzes {beta}-(1->3)- and {beta}-(1->6)-galactosyl residues of Arabinogalactan protein. Plant Physiol. 138, 1563–1576. doi: 10.1104/pp.105.062562
Larkin, M. A., Blackshields, G., Brown, N. P., Chenna, R., McGettigan, P. A., McWilliam, H., et al., (2007). Clustal W and Clustal X version 2.0. Bioinformatics 23, 2947–2948. doi: 10.1093/bioinformatics/btm404
Marchler-Bauer, A., Bo, Y., Han, L., He, J., Lanczycki, C. J., Lu, S., et al. (2017). CDD/SPARCLE: functional classification of proteins via subfamily domain architectures. Nucleic Acids Res. 45, D200–D203. doi: 10.1093/nar/gkw1129
Qian, W., Han, Z. J., Tao, J., and He, C. (2008). Genome-scale mutagenesis and phenotypic characterization of two-component signal transduction systems in Xanthomonas campestris pv. campestris ATCC 3. Mol. Plant Microbe. Interact. 21, 1128–1138. doi: 10.1094/MPMI-21-8-1128
Qian, W., Jia, Y., Ren, S. X., He, Y. Q., Feng, J. X., Lu, L. F., et al. (2005). Comparative and functional genomic analyses of the pathogenicity of phytopathogen Xanthomonas campestris pv. campestris. Genome Res. 15, 757–767. doi: 10.1101/gr.3378705
Rodríguez, A. P., Leiro, R. F., Trillo, M. C., Cerdán, M. E., Siso, M. I., and Becerra, M. (2006). Secretion and properties of a hybrid Kluyveromyces lactis-Aspergillus niger beta-galactosidase. Microb. Cell Fact. 5:41. doi: 10.1186/1475-2859-5-41
Schröder, C., Blank, S., and Antranikian, G. (2015). First glycoside hydrolase family 2 enzymes from Thermus antranikianii and Thermus brockianus with beta-glucosidase activity. Front. Bioeng. Biotechnol. 3:76. doi: 10.3389/fbioe.2015.00076
Smale, S. T. (2010). Beta-galactosidase assay. Cold Spring Harb. Protoc., 2010:pdb prot5423. doi: 10.1101/pdb.prot5423
Tamura, K., Stecher, G., Peterson, D., Filipski, A., and Kumar, S. (2013). MEGA6: Molecular Evolutionary Genetics Analysis version 6.0. Mol. Biol. Evol. 30, 2725–2729. doi: 10.1093/molbev/mst197
Tang, J. L., Liu, Y. N., Barber, C. E., Dow, J. M., Wootton, J. C., and Daniels, M. J. (1991). Genetic and molecular analysis of a cluster of rpf genes involved in positive regulation of synthesis of extracellular enzymes and polysaccharide in Xanthomonas campestris pathovar campestris. Mol. Gen. Genet. 226, 409–417. doi: 10.1007/BF00260653
Tao, J., Li, C., Luo, C., and He, C. (2014). RavA/RavR two-component system regulates Xanthomonas campestris pathogenesis and c-di-GMP turnover. FEMS Microbiol. Lett. 358, 81–90. doi: 10.1111/1574-6968.12529
Vicente, J. G., and Holub, E. B. (2013). Xanthomonas campestris pv. campestris (cause of black rot of crucifers) in the genomic era is still a worldwide threat to brassica crops. Mol. Plant Pathol. 14, 2–18. doi: 10.1111/j.1364-3703.2012.00833.x
Yang, T. C., Hu, R. M., Hsiao, Y. M., Weng, S. F., and Tseng, Y. H. (2003). Molecular genetic analyses of potential beta-galactosidase genes in Xanthomonas campestris. J. Mol. Microbiol. Biotechnol. 6, 145–154. doi: 10.1159/000077245
Yang, T. C., Hu, R. M., Weng, S. F., and Tseng, Y. H. (2007). Identification of a hypothetical protein of plant pathogenic Xanthomonas campestris as a novel beta-galactosidase. J. Mol. Microbiol. Biotechnol. 13, 172–180. doi: 10.1159/000103609
Keywords: β-galactosidase, glycosyl hydrolase (GH) family 2 and 35, LacZ, reporter gene, Xanthomonas campestris pv. campestris
Citation: Wang H, Shi C, Xie Q, Wang Y, Liu S, Li C, He C and Tao J (2018) Genome-Wide Analysis of β-Galactosidases in Xanthomonas campestris pv. campestris 8004. Front. Microbiol. 9:957. doi: 10.3389/fmicb.2018.00957
Received: 21 January 2018; Accepted: 24 April 2018;
Published: 11 May 2018.
Edited by:
Michael Benedik, Texas A&M University, United StatesReviewed by:
Samantha J King, The Ohio State University, United StatesEwa Lojkowska, University of Gdansk, Poland
Copyright © 2018 Wang, Shi, Xie, Wang, Liu, Li, He and Tao. This is an open-access article distributed under the terms of the Creative Commons Attribution License (CC BY). The use, distribution or reproduction in other forums is permitted, provided the original author(s) and the copyright owner are credited and that the original publication in this journal is cited, in accordance with accepted academic practice. No use, distribution or reproduction is permitted which does not comply with these terms.
*Correspondence: Jun Tao, dGFvakBoYWludS5lZHUuY24=
†These authors have contributed equally to this work.