- Root Biology Center, College of Resources and Environment, Fujian Agriculture and Forestry University, Fuzhou, China
Rhizosphere microorganisms play important roles in plant health and nutrition, and interactions among plants and microorganisms are important for establishment of root microbiomes. As yet, plant-microbe and microbe-microbe interactions in the rhizosphere remain largely mysterious. In this study, rhizosphere fungal community structure was first studied in a field experiment with two soybean cultivars contrasting in nodulation grown in two rhizobium inoculation treatments. Following this, recombinant inbred lines (RILs) contrasting in markers across three QTLs for biological nitrogen fixation (BNF) were evaluated for effects of genotype and rhizobium inoculation to the rhizosphere fungal community as assessed using ITS1 amplicon sequencing. The soybean plants tested herein not only hosted rhizosphere fungal communities that were distinct from bulk soils, but also specifically recruited and enriched Cladosporium from bulk soils. The resulting rhizosphere fungal communities varied among soybean genotypes, as well as, between rhizobium inoculation treatments. Besides, Cladosporium were mostly enriched in the rhizospheres of soybean genotypes carrying two or three favorable BNF QTLs, suggesting a close association between soybean traits associated with nodulation and those affecting the rhizosphere fungal community. This inference was bolstered by the observation that introduction of exogenous rhizobia significantly altered rhizosphere fungal communities to the point that these communities could be distinguished based on the combination of soybean genotype and whether exogenous rhizobia was applied. Interestingly, grouping of host plants by BNF QTLs also distinguished fungal community responses to rhizobium inoculation. Taken together, these results reveal that complex cross-kingdom interactions exist among host plants, symbiotic N2 fixing bacteria and fungal communities in the soybean rhizosphere.
Introduction
Since colonizing land 450 million years ago, plants have co-evolved with associated microbes (Rosenberg and Zilber-Rosenberg, 2016). Roots of soil-grown plants not only function in mineral nutrient uptake but also provide sites for association with soil-inhabiting microbes in the rhizosphere (Hacquard et al., 2015). Microbes also interact with each other while establishing distinct plant microbiomes that play important roles in both the phyllosphere and rhizosphere (Agler et al., 2016). Plant microbiota include archaea, fungi, bacteria, and protists, which, on the whole, critically influence plant nutrition, health and productivity (Lindow and Brandl, 2003; Buée et al., 2009; Berendsen et al., 2012; Vorholt, 2012). Currently available research suggests that the establishment of plant root microbiomes largely depends on root exudates, and is strongly influenced by preferences for specific sets of microbial carbohydrates (Hu et al., 2018; Stringlis et al., 2018; Zhalnina et al., 2018; Huang et al., 2019). Beyond this, there is little information available on specific interactions between prokaryotic and eukaryotic microorganisms responsible for the establishment of plant-associated microbiomes (Hassani et al., 2018).
Although less abundant in soils than bacteria, fungi are nonetheless important for soil health through numerous interactions with plants and other microbes, most notably in the current context, as participants in symbiotic relationships. At present, the most documented soil fungi are arbuscular mycorrhiza fungi (AMF), which form symbiotic partnerships with over 80% of land plants (Field et al., 2015; Brundrett and Tedersoo, 2018). Current estimates associate 320,000–340,000 species of plants with more than 50,000 fungal species (van der Heijden et al., 2015). A benefit of joining mycorrhizal associations for plants is that the host plant receives mineral nutrients, especially phosphorus (P) (Bucher, 2007). Almost 70% of the total P acquired by rice enters symbiotically through AMF (Yang et al., 2012). These soil fungal networks are also more resilient than bacterial networks under drought conditions, and some might tolerate disturbances (de Vries et al., 2018; Igiehon and Babalola, 2018). Fungal and bacterial interactions affect plant participation in symbiotic relationships with mycorrhizal fungi, which further reinforces that multiple positive interkingdom interactions exist in plant-associated microbiota (Garbaye, 1994).
Soil fungal communities may also play crucial roles as biological control agents for associated plant species (Katoch and Pull, 2017; Singh et al., 2017). In agriculture, fungi are often used as biological control agents active against pests or pathogens through competition for nutrients and space, or as parasites of pests and pathogens. Entomopathogenic fungi can be used as biopesticides, as they actively kill insects. Globally, Beauveria bassiana, Metarhizium spp., Hirsutella spp., Paecilomyces (Isaria) spp., and Lecanicillium lecanii are all used as biological insecticides (Deshpande, 1999; Thomas and Read, 2007). In short, soil fungal communities acting as symbiotic partners or in tripartite interactions play a variety of crucial roles that benefit plants.
Beyond AMF contributions to plant associated communities, soil microbes also participate in many other interactions that affect plant health. For example, non-mycorrhizal species, such as Arabidopsis thaliana, may interact with endophytic fungi, such as Colletotrichum tofieldiae and Serendipita indica, with the result of increasing host plant acquisition of P, and thus promoting plant growth under low P conditions (Bakshi et al., 2015; Hiruma et al., 2016). Despite the ubiquity of fungal impacts on plants, some fungi are beneficial for plant and most plant-associated fungi exhibit neutral or detrimental effects on the growth of host plants (Durán et al., 2018).
Meanwhile, bacteria also participate in establishment of soil microbial communities. Recently, it has been reported that bacterial microbiota colonizing the roots of Arabidopsis promote plant survival by antagonizing filamentous fungal and oomycete eukaryotes (Durán et al., 2018). In the same work, they also demonstrated that the introduction of bacteria may shift fungal and oomycete communities both around roots and in the absence of host plants (Durán et al., 2018). Overall, a large variety of reports suggest that microbe-microbe interactions are essential components of plant associated communities, yet most of the complex interactions occurring within plant microbiomes remain uncharted.
As the third largest family of angiosperms, legumes not only form nitrogen (N) fixing nodules in symbiosis with rhizobia, but also establish associations with AMF to facilitate P acquisition from soils to host plant tissues (Doyle and Luckow, 2003; Herridge et al., 2008; Wang et al., 2010; Qin et al., 2011; Pandey et al., 2016). Soybean, as the most important leguminous crop in the world, provides protein and oil for growing human and livestock populations. Among the benefits resulting from associations between soybean and soil microbiota, rhizobia are known to fix large amounts of N2 that becomes available for soybeans and future crops, and is not replaceable in modern agricultural systems (Arslanoglu et al., 2011; Gerland et al., 2014).
Rhizobium inoculation is widely applied to promote soybean productivity and reduce N fertilization (Pandey et al., 2016). As documented in a recent report, rhizobium inoculation might also significantly influence the bacterial community in the rhizosphere of soybean plants (Zhong et al., 2019). To date, the extent to which rhizobium introduction influences fungal communities is unclear, with few interactions between rhizobium and fungal community members of soybean rhizospheres revealed. As hosts, this study included two parental soybean cultivars contrasting in nodulation (P1: low nodulation, P2: high nodulation), along with their low nodulation (LN1-4) and high nodulation (HN1-4) recombinant inbred lines (RILs) that also varied across markers for BNF (Biological Nitrogen Fixation), as determined by qBNF QTL (Yang et al., 2017). ITS1 region amplicon sequencing was used to investigate the structure of fungal communities in bulk soils and in rhizospheres of the two parental soybean lines and their RILs grown under rhizobium inoculation and non-inoculation conditions, in order to reveal the potential complex interactions among rhizobia, soybean plants and fungal community.
Materials and Methods
Plant Materials and Field Trials
Two soybean cultivars contrasting in nodulation (P1, Low nodulation; P2, high nodulation), together with eight of their F9:11 recombinant inbred lines (RILs; HN1-4, four lines with high nodulation; LN1-4, four lines with low nodulation) were tested. The RIL lines were selected based on nodule number and the presence (HN) or absence (LN) of at least two QTLs responsible for biological nitrogen fixation (BNF) (Supplementary Figure S1 and Supplementary Table S8; Yang et al., 2017). Field trials were conducted from June to October in 2016 and 2017 on the experimental farm (E114.48°, N38.03°) of the Institute of Cereal and Oil crops, Shijiazhuang City, Heibei Province, China. The basic soil properties are listed in Supplementary Table S1. The rhizobia (Bradyrhizobium sp. Accession No.: EU825988) for the inoculation experiment were previously isolated, identified and studied in our lab, which could form symbiosis with all the tested soybean plants and showed higher nitrogenase activity than USDA110 (Chen et al., 2008). Besides, the Bradyrhizobium spp. strains have been used to identified the QTLs responsible for BNF (Yang et al., 2017).
All soybean accessions were cultivated in split plots containing complete blocks inoculated (R) or not inoculated (N) with rhizobia as previously described (Zhong et al., 2019). The schemes of two years’ field experimental design were shown in the Supplementary Figures S1B,C. In brief, in 2016 there are two blocks for two different rhizobium treatments. In each block, soybean parental genotypes were planted in a split plot with randomized complete blocks, in each block each parental soybean were cultivated in three plots. Totally, there were two big blocks, and 12 plots in 2016 field trial. Thirty seeds were sown in each plot of three 1.5 m rows spaced × 0.5 m apart. In the R treatment, soybean seeds were inoculated with rhizobia as previously described (Qin et al., 2011; Zhong et al., 2019). The field had been used for a wheat and soybean rotation for more than 10 years, with the most recent crop being wheat. No fertilizers were applied during soybean growth, and other field management procedures (e.g., irrigation, pest control, etc.) followed local practices. In 2017, another field trial using eight RILs was carried out in the same field, with a similar experimental design as in 2016. Totally, there were two blocks for two rhizobium treatments and two plots for each RIL in each block with randomized arrange. Hence, there were 32 plots in total in 2017.
Sample Collection
Rhizosphere and bulk soil samples were separately collected when soybeans were at the R5 stage (Reproductive stage 5) of development according to Inceoglu et al. (2010), in which the biomass of soybean plants reach the highest. Soybean plants were harvested as whole plants by first digging out the root system and removing loose soils by shaking the roots. Rhizosphere soils were considered as that which adhered firmly to the roots and were collected using a sterile brush. Then the soil was stored in the liquid nitrogen and transported to the lab and stored at −80°C fridge till the total DNA extraction.
The 2016 parental genotype experiment included three biological replicates for each parent and each treatment, and the 2017 experiment with RILs included two biological replicates for each RIL and each treatment. Since RILs were divided into two groups and subsequently analyzed as two groups (HN or LN) according the QTLs and nodule number, hence, for each group under each treatment condition there were eight biological replicates. For each biological replicate, soils were collected from three independent soybean plants and mixed together. In total, 44 rhizosphere soil samples (12 in 2016 and 32 in 2017) and 6 bulk soil samples (3 each in 2016 and 2017) samples were collected as previously described (Zhong et al., 2019). Bulk soil samples were taken from 0 to 10 cm soils after carefully removing the surface soil from 3 sites near to soybean cultivation field.
ITS1 Amplicon Library Preparation and Sequencing
Total soil DNA was extracted from 500 mg of soil using a PowerSoil DNA Isolation Kit (Mobio Laboratories, Carlsbad, CA, United States) according to the manufacturer’s protocol. DNA concentration and quality were determined using a NanoDrop 1000 spectrophotometer (Thermo Scientific, Waltham, United States). The ITS1 region from 1737 to 2043, which is about 300 bp length, was selected as the target sequencing region. The whole sequence of the Internal Transcribed Spacer 1 region (ITS1) amplicon sequencing libraries were generated using the TruSeq® DNA PCR-Free Sample Preparation Kit (Illumina, United States) following the manufacturer’s recommendations, with index codes added using the following primers: ITS5-1737-F (5’-GGAAGTAAAAGTCGTAACAAGG-3’) and ITS2-2043-R (5’-GCTGCGTTCTTCATCGATGC-3’) (Findley et al., 2013). Library quality was monitored on the Qubit@ 2.0 Fluorometer (Thermo Scientific) and Agilent Bioanalyzer 2100 system. PCR reactions spanning the ITS1 region were carried out using the Phusion® High-Fidelity PCR Master Mix with GC Buffer (New England Biolabs: NEB, United States). PCR products were purified using the QIAquick Gel Extraction Kit (Qiagen, Germany). Purified PCR products were subjected to ITS1 amplicon sequencing at the Novogene Institute using the Illumina Hiseq 2500 platform (Beijing, China).
Sequence Analysis of the ITS1 Amplicons
Raw reads were assigned to the samples according to unique barcodes located on the primers, and both primers and barcodes were removed from the paired-end reads using FLASH (Magoč and Steven, 2011) and merged using Pandaseq (Masella et al., 2012). Low quality and chimeric reads were filtered using QIIME (Quantitative Insights Into Microbial Ecology) to obtain the effective reads, which were used for the subsequent analysis (Caporaso et al., 2010; Bokulich et al., 2013). Effective sequence were then clustered with UPARSE application (version 7.0.1001)1 and sequences with = 97% identity were assigned to the same OTUs (Edgar, 2013). Representative sequences for each OTU were screened for further annotation. For each representative sequence, the Unite Database2 (Kõljalg et al., 2013) was used to annotate taxonomic information (Caporaso et al., 2010) based on the Blast algorithm as calculated in QIIME (version = 1.7.0)3 (Altschul et al., 1990). After that the data for each sample was normalized to the sample with least effective reads and the afterword analysis were all based on this normalized data. A phylogenetic tree was constructed based on multiple sequence alignment conducted in MUSCLE (version 3.8.31)4 (Edgar, 2004). Rarefaction curves of observed species and Chao1 index, which is usually used for the estimation the richness of species in an ecosystem, were analyzed in QIIME. Normalized data were subjected to beta diversity analysis. Non-metric Multidimensional scaling (NMDS) and analysis of similarities (ANOSIM) were carried out using the Vegan package (version 2.3.0, anova.cca) in R (version 3.4.3). The Vegan package in R was also employed for constrained PCoA analysis, along with permutational ANOVA for calculating P values, with the number of permutations set at 999 (Oksanen et al., 2015; R Core Team, 2015). STAMP (version 2.1.3) was used to analyze significant differences in fungal taxa observed between genotypes and rhizobia inoculation treatments in Benjamini-Hochberg FDR corrected two-sided Welch’s t-tests (Parks et al., 2014). Online linear discriminant analysis effect size (LEfSe) analysis was performed to reveal biomarkers for the different sample groups based on a normalized OTU table5. For LEfSe analysis, the Kruskal–Wallis rank sum test was employed to distinguish significantly different species within groups at an alpha value of 0.05 and a threshold of 3.5.
Fungal co-occurrence networks in the soybean rhizosphere were constructed based on Spearman correlation matrices using the R package psych (version: 1.8.4) (Oksanen et al., 2015). Node connectivity, cumulative degree distribution and average path length were analyzed with psych (version: 1.8.4). To filter data, Spearman correlations were required to be statistically significant (P < 0.05) with correlation coefficients exceeding 0.7 (r > 0.7). Nodes of the network represented OTUs and edges connecting these nodes indicated high and significant correlations between the OTUs. Network images were generated using the interactive Gephi platform (Version, 9.2)6.
Results
Fungal Richness Was Similar Between Soybean Rhizospheres and Bulk Soils
To investigate the structure and composition of fungal communities in soybean rhizospheres, amplicon sequencing was employed, with targets selected based on the sequence of the ITS1 region. Sequencing from two years of field trials yielded 3,674,452 raw reads, of which, 3,519,397 could be combined. The average percentage of combined reads was 95.79%, with the average length being 222 bp (Supplementary Tables S2, S3). A total 3,378,293 high quality reads were obtained after removing low quality reads and chimeric reads. The average Q20 and Q30 of reads were 99.10 and 98.29%, respectively (Supplementary Tables S4, S5). Among high quality reads, 2,138,425 could be annotated. After performing OTU cluster analysis with a 97% similarity threshold for grouping sequences, the number of OTUs obtained for each sample ranged from 589 to 1374 (Supplementary Tables S6, S7).
Rarefaction curves suggested that the sequencing depth for most of the samples was enough to discover most of the detectable fungi present in the soil over the 2 year course of these field trials (Supplementary Figure S2). The species observed and Chao1 index values indicated fungal richness was similar between bulk soil and rhizosphere samples (Figures 1A,B and Supplementary Figure S3). In contrast to this similarity between rhizospheres and bulk soil, rhizobium inoculation led to slight but not significant alterations in the richness of fungal communities (Supplementary Figure S3).
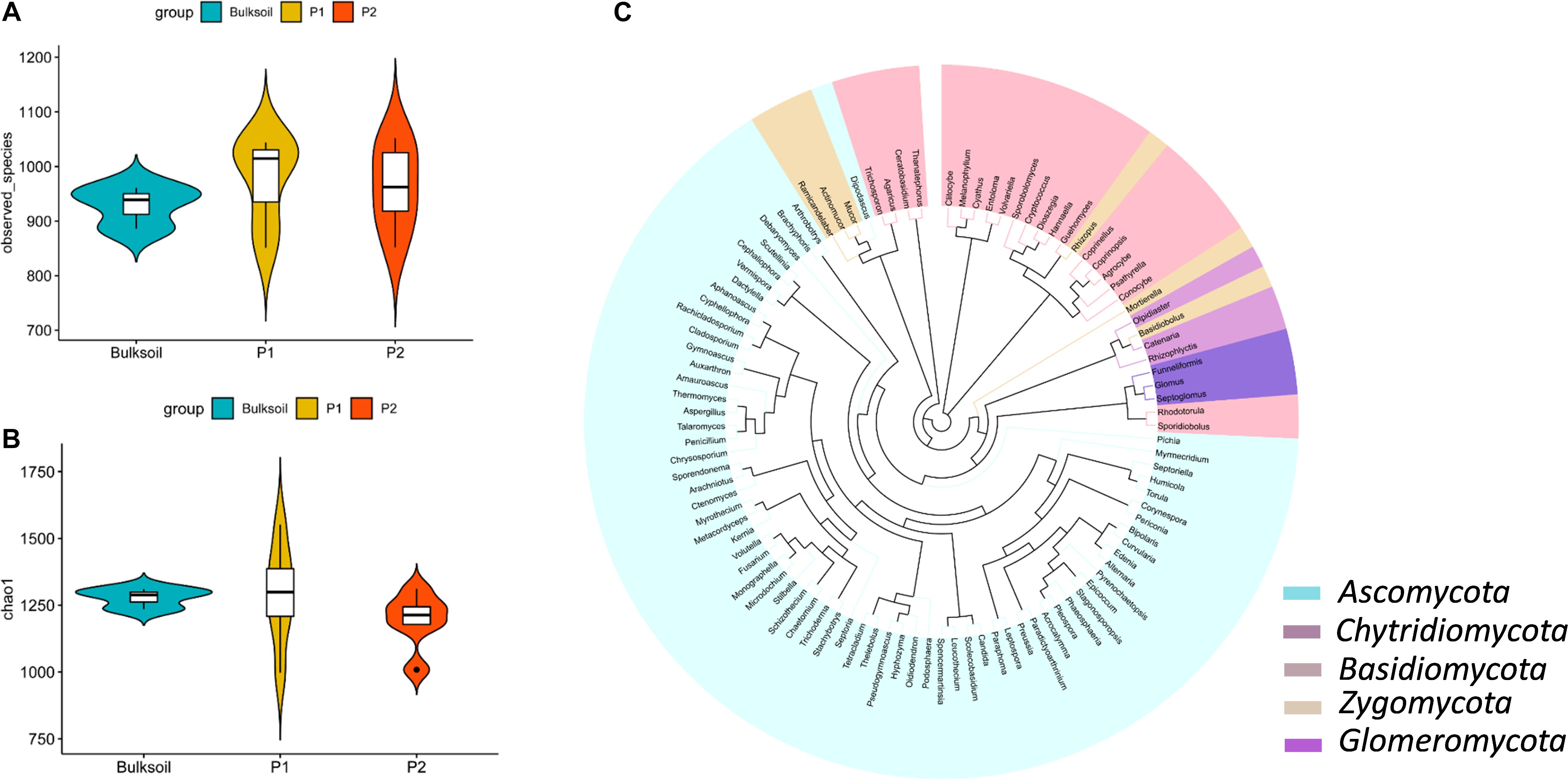
Figure 1. Richness and structure of soybean rhizosphere fungal communities. (A,B) Fungal richness was analyzed by calculating the number of observed fungal species (A) and Chao1 index (B) between bulk soil and rhizosphere soil samples collected from roots of P1 and P2 soybean plants. (C) Phylogenetic analysis of the top 100 soybean rhizosphere fungi in relative abundance identified at the genus level.
There were 831 OTUs common to both bulk soil and the rhizospheres of the two parental cultivars (Supplementary Figure S4A). For RILs, there were 880 and 906 OTUs in both bulk soil and rhizospheres of R and N soybeans, respectively (Supplementary Figures S4B,C). Other fungal OTUs specifically existed in soybean rhizospheres (Supplementary Figure S4).
Phylogenetic analysis showed that most of the high abundance fungi belonged to Ascomycota, Basidiomycota, Chytridiomycota, Zygomycota, and Glomeromycota at the phylum level (Figure 1C). Of these, Ascomycota, Zygomycota, and Basidiomycota were the dominant fungi in soybean rhizospheres, with relative abundances ranging between 45 and 65% of the total fungi counted (Supplementary Figure S5). At the family level, Davidiellaceae, Cucurbitariaceae, Mortierellaceae, Rhizopodaceae, and Mucoraceae were more abundant than other families of fungi (Supplementary Figures S6A,C). Cladosporium, Pyrenochaetopsis, Rhizopus, Mortierella, and Actinomucor were dominant at the genus taxonomic level (Supplementary Figure S6). Taken together, these results suggest that the richness of fungi in soybean rhizosphere and bulk soils mostly overlap.
Structure and Composition Analysis of Soybean Rhizosphere Fungal Communities
While overall richness of fungi did not significantly vary between rhizosphere and bulk soils, interactions within each community of microbes might still vary between soil sources. It was further analyzed at the community level. Rhizosphere soil samples were obviously shifted from bulk soil samples (Figure 2A). In addition, ANOSIM analysis suggested that the fungal communities varied significantly between bulk soil and rhizosphere samples (R = 0.849, P = 0.002) (Figure 2A). Further, similar patterns were observed with NMDS analysis, with the result that most bulk soil samples could be separated from rhizosphere samples (Stress value = 0.063) (Figure 2B). Collectively, these results demonstrate that the structure of soybean rhizosphere fungal communities is distinct from that in bulk soils, leaving open the possibility that soybean plants might drive fungal community differentiation from bulk soils to the rhizospheres.
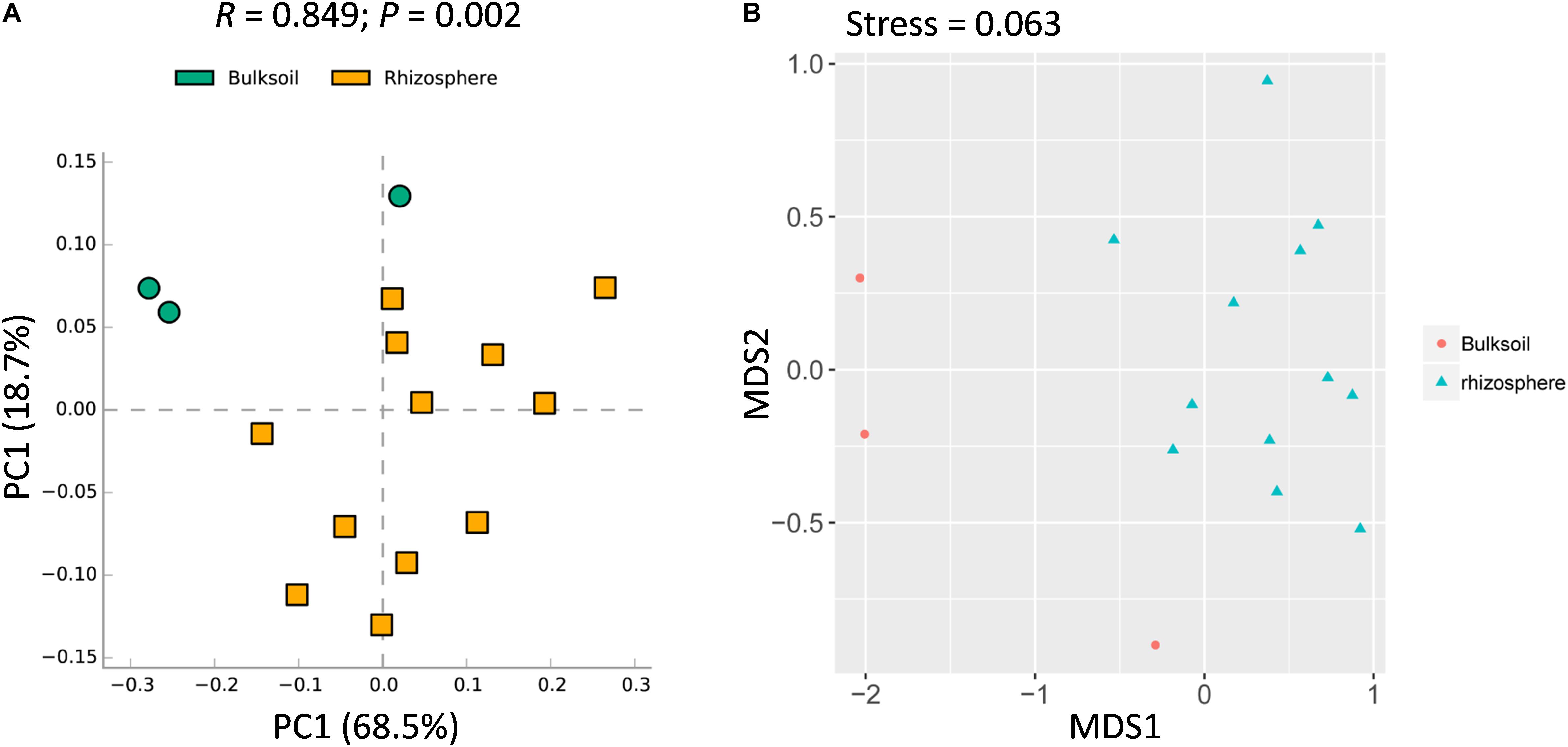
Figure 2. Rhizosphere fungal communities of soybean plants are distinct compared to bulk soil. (A) PCA analysis of bacterial communities performed using a normalized OTU and the STAMP software application (version: 2.1.3). (B) NMDS analysis based on Brad_Curtis distance matrix. The R and P value were calculated in the Vegan R package (version: 2.3.0) running ANOSIM analysis based on a Bray_Curtis distance matrix of bulk soil and rhizosphere samples. In the permutation test, the number of permutations tested was 999.
To analyze which fungi were significantly selected by soybean plants, LEfSe was employed to identify biomarker taxa. In these field samples, Capnodiales, Davidellaceae, Cladosporium, and Mycosphaerellaceae were identified as biomarkers for rhizosphere samples, while Pleosporales, Cucurbitariaceae, Pyrenochaetopsis, Rhizopodaceae, and Rhizopus were biomarkers for bulk soil samples (LDA score > 3.5; Kruskal–Wallis rank sum test, P < 0.05). These results suggest that soybean plants might provide resources most favorable for the proliferation of Capnodiales, Davidellaceae, and Cladosporium (Figures 3A,B). Further investigation of fungal families and genera favored in soybean rhizospheres as determined in the STAMP application was consistent with the results from LEfSe. Cladosporium were significantly enriched in soybean rhizospheres at the genus taxonomic level (adjusted P value < 0.001) (Figure 3C). In addition, Septoria and Phaeosphaeria were also significantly enriched by soybean plants (adjusted P value = 1.52e-3; P = 0.017). At the family level, Davidiellaceae (adjusted P = 2.81e-4), Mycosphaerellaceae (adjusted P = 1.40e-4), and Phaeosphaeriaceae (adjusted P = 0.018) were all significantly recruited by soybean plants (Supplementary Figure S7); and Davidiellaceae was also a biomarker in soybean rhizosphere communities (Figure 3B).
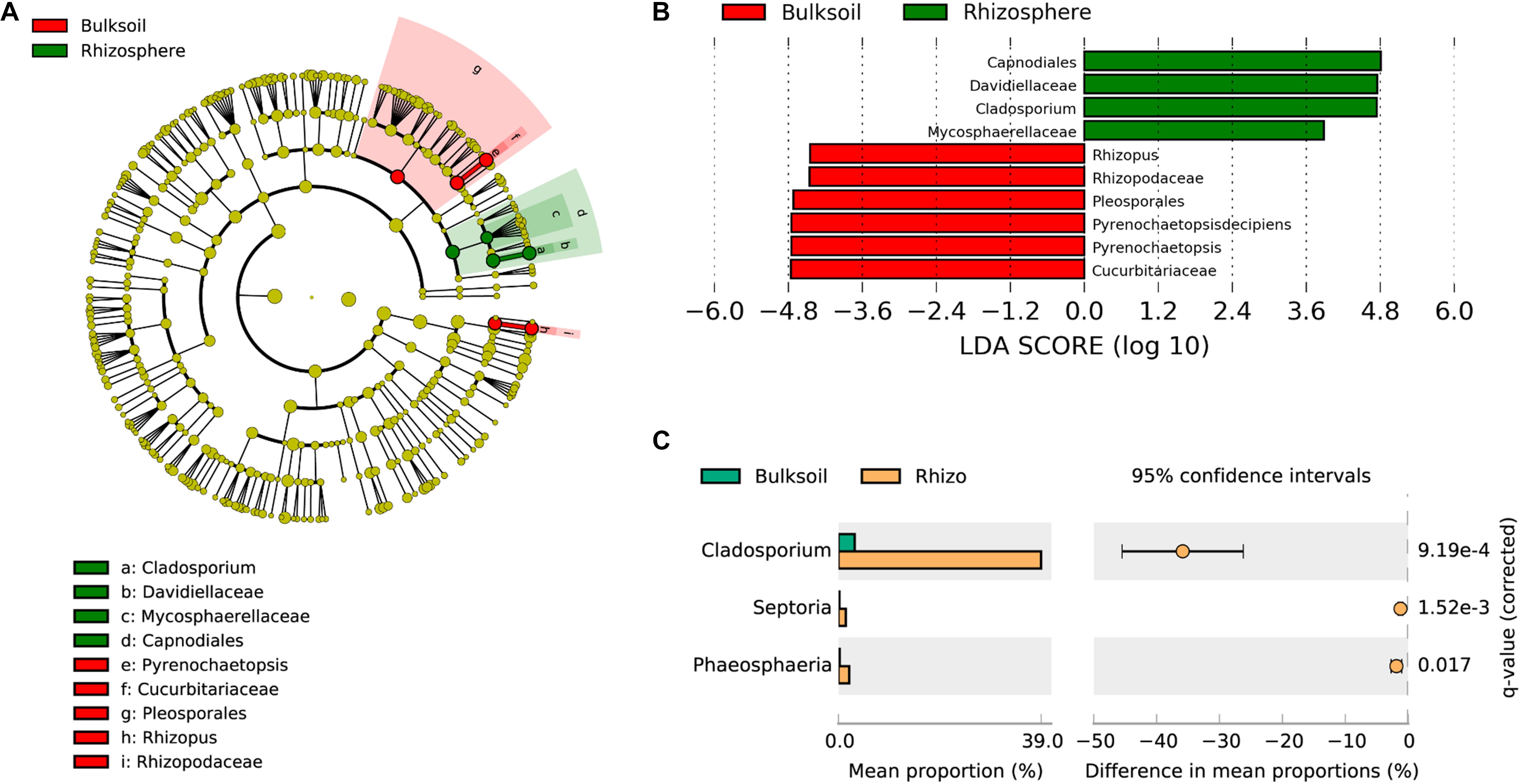
Figure 3. Soybean plants specifically select fungi from bulk soils. (A) Phylogenetic dendrogram of biomarkers identified in bulk soil and soybean rhizospheres. The circles from inside to outside indicate bacterial taxonomic levels from phylum to genus. Yellow dots represent fungi not varying significantly in relative abundance. Biomarker fungi are colored according to the corresponding class colors on the right. (B) LDA scores of biomarker fungi for bulk soil and rhizosphere samples. (C) Fungi strongly selected by soybean plants were analyzed in the STAMP software application (version: 2.1.3). Corrected P-values were calculated using the two-sided Welch’s t-test with the Benjamini–Hochberg FDR correction applied.
Soybean Genotype Influences Rhizosphere Fungal Community
We previously demonstrated that host plant genotype can significantly influence the soybean rhizosphere bacterial community (Zhong et al., 2019). ANOSIM analysis indicated that the rhizosphere fungal community varied significantly between P1 and P2 samples (R = 0.456; P = 0.004), which strongly suggests that traits that vary among soybean genotypes significantly influence the rhizosphere fungal community (Figure 4A). Besides, the PCA suggested that P1 and P2 could be clearly separated by the first principal component, which explained 84.3% of variance (Figure 4A). The result of NMDS was similar with that from the PCA analysis (Stress value = 0.089) (Supplementary Figure S8). Results from LEfSe clustering of rhizosphere biomarkers at the phylum taxonomic level returned a preponderance of Basidiomycetes in P1 samples and Ascomycota in P2 samples, with P1 and P2 being clearly separated in a cladogram (Figure 4B). The identified biomarkers based on LDA scores were Dothidemycetes, Capnodiales, Davidiellaceae, Cladosporium, and Ascomynota for P2, along with Mucorales, Basidiomycota, Rhizopus, and Pyrenochaetopsis for P1 (Figure 4C). Taken together, these results suggest that the two parental soybean genotypes studied herein significantly influence the structure and composition of rhizosphere fungal communities in the rhizosphere.
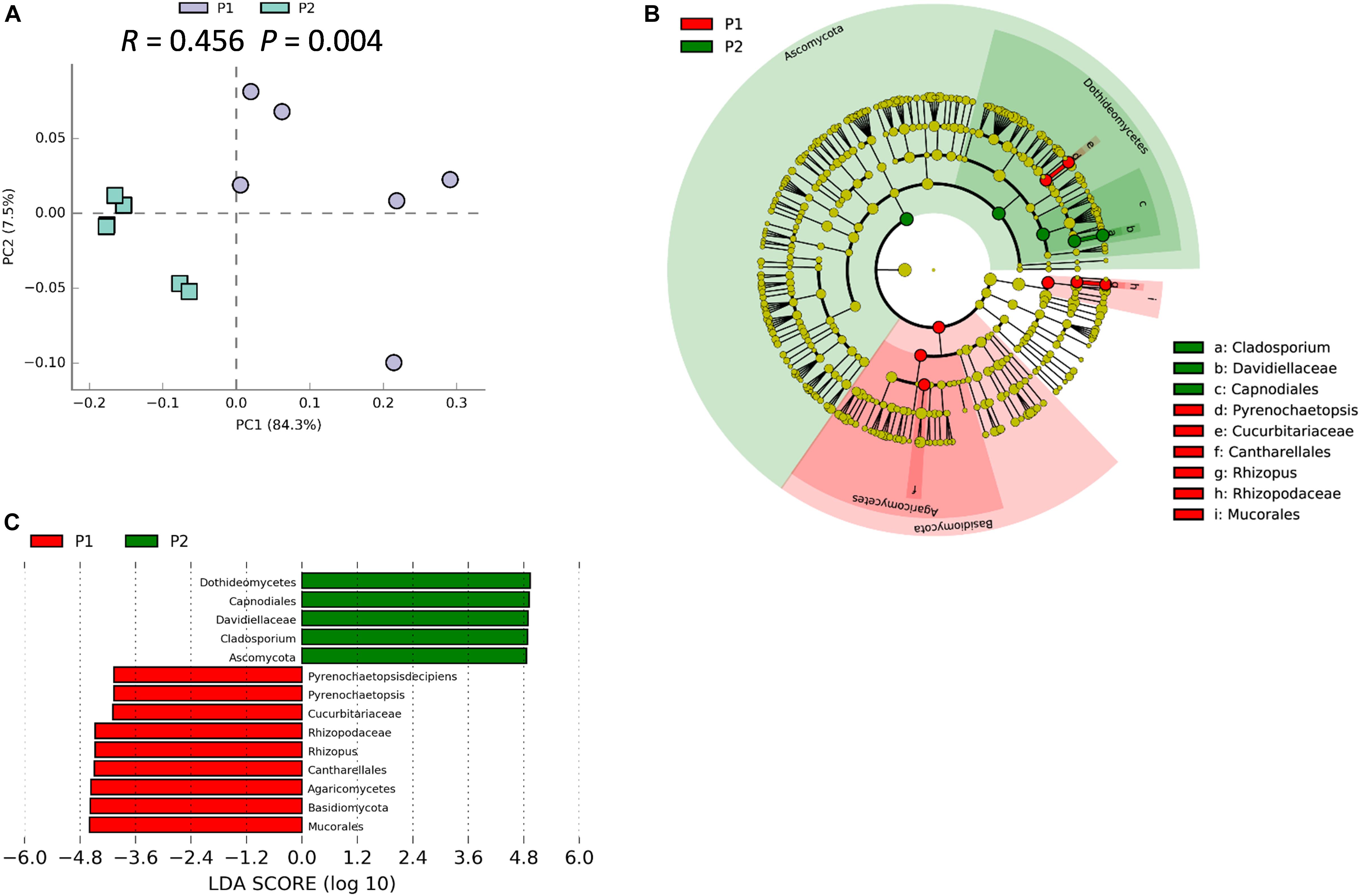
Figure 4. Soybean genotype has influence on rhizosphere fungal communities. (A) PCA of rhizosphere fungal communities associated with two parental soybean genotypes, P1 and P2, as performed based on a normalized OTU table using STAMP software. ANOSIM analysis of soybean rhizosphere fungal communities was used to calculate the difference between the two parental soybean genotypes, P1 and P2, based on the Bray_Curtis distance matrix. In the permutation test, the number of permutations was 999; R = 0.456, P = 0.004. (B) Biomarker fungi with LDA scores > 3.5 identified in rhizospheres surrounding roots of each parental soybean genotype as determined in the Kruskal–Wallis rank sum test, P < 0.05. (C) Phylogenetic dendrogram of biomarkers in the rhizospheres of two parental soybean genotypes. Biomarker fungi are marked according to the corresponding class colors on the right.
Rhizobium Inoculation Shifts Soybean Rhizosphere Fungal Communities
To study the impacts of rhizobium inoculation on soybean rhizosphere fungal communities, rhizosphere samples were grouped into N_Parent (Parental lines without rhizobium inoculation) and R_Parent (Parental lines with rhizobium inoculation). Analysis with ANOSIM suggested that rhizobium inoculation significantly impacted rhizosphere fungal communities (R = 0.24; P = 0.044) (Figure 5A). Further, NMDS showed that N_Parent and R_Parent rhizospheres could not be clearly separated (Figure 5B). Moreover, samples could be grouped into N_P1, N_P2, R_P1, or R_P2 categories according to the rhizobium treatment and genotype. These groups were clearly separated in CPCoA analysis, with treatment and genotype explaining 39% of the variation and significantly influencing fungal communities in the rhizosphere (P = 0.003) (Figure 5C). These results suggest that both soybean genotype and rhizobium inoculation exert considerable influence on the soybean rhizosphere fungal community.
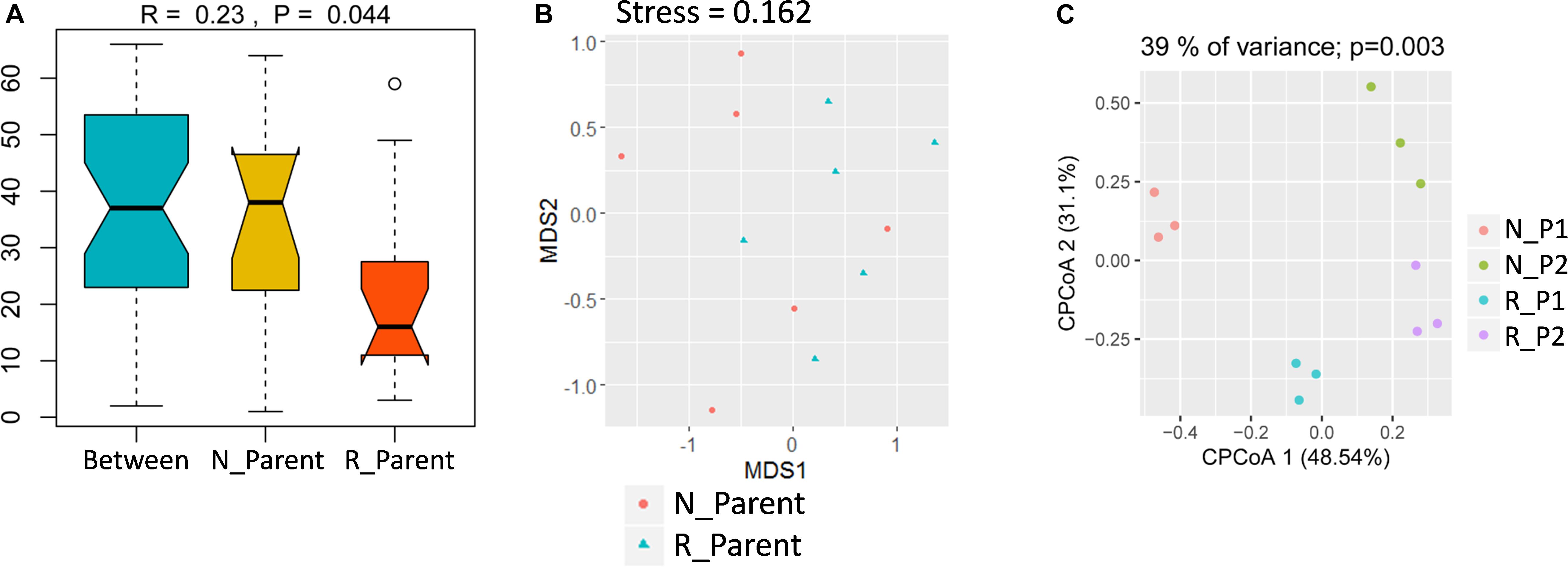
Figure 5. Influence of rhizobium inoculation on rhizosphere fungal communities and AMF infection rate. (A) Dissimilarity of fungal communities determined by ANOSIM. The P value was calculated by permutation testing with the number of permutation set to 999. (B) Differences in fungal communities of parental line rhizospheres either inoculated (R_Parent) or not inoculated (N_Parent) with rhizobium as calculated by NMDS. (C) Constrained principal component analysis (CPCoA) was carried out to asses clustering of rhizosphere fungal communities among N_P1, N_P2, R_P1, and R_P2 plots using a normalized OTU table and acc.anova in the Vegan R package (version: 2.3.0). The P value was calculated by permutation testing with the number of permutations set to 999. N_P1, Parental line1 without rhizobium inoculation; R_P1, Parental line1 with rhizobium inoculation; N_P2, Parental line 2 without rhizobium inoculation; R_P2, Parental line2 with rhizobium inoculation.
Linear discriminant analysis effect size was further employed to evaluate specific fungi significantly influenced by rhizobium inoculation. In this analysis, the biomarkers for N_Parent and R_Parent were clearly separated in a cladogram (Figure 6A). Eurotiales, Trichocomaceae, Septoria, Myrmecridium, Penicilliumoxalicum, and Mycosphaerella were the biomarkers associated with R_Parent rhizospheres, while Mortierellaalpina, Gymnoascus, Leucothecium, and Zygomycota were associated with N_Parent rhizospheres (Figure 6B).
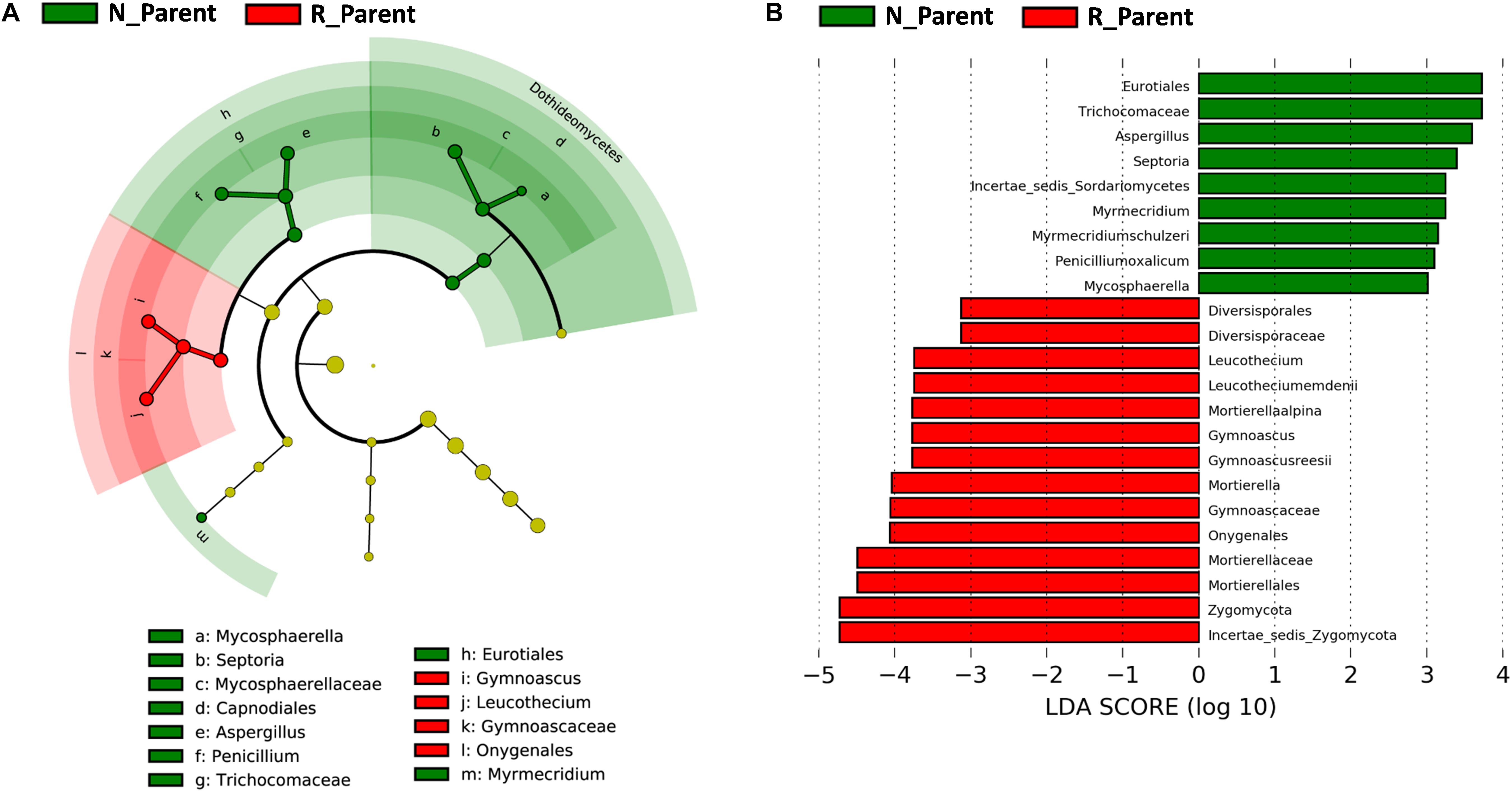
Figure 6. Influence of rhizobium inoculation on rhizosphere fungal communities. LEfSE analysis revealed soybean biomarkers for the rhizobium non-inoculation and inoculation treatments. (A) Biomarkers for the N_Parent and R_Parent rhizosphere fungi are indicated in the phylogenetic dendrogram by different colors. Red: N_Parent, Green: R_Parent. (B) LDA scores > 3 for biomarker fungi for N_Parent and R_Parent rhizospsheres as calculated in the Kruskal–Wallis rank sum test, P < 0.05. N_Parent: Parental lines grown in plots not inoculated with rhizobium; R_Parent: Parental lines grown in rhizobium inoculated plots.
Soybean Genotype and Rhizobium Inoculation Coordinately Influence Soybean Rhizosphere Fungal Communities
To investigate the extent of soybean genotypic effects on the response of fungal communities to rhizobium inoculation, we tested eight RILs selected from F9:11 recombinant inbred lines (HN1-4, four lines with high nodulation; LN1-4, four lines with low nodulation) varying in nodulation number and BNF genetic markers (Yang et al., 2017). The RILs were cultivated with (R) and without (N) rhizobium inoculation, and their rhizosphere samples were categorized into N_HN, N_LN, R_HN and R_LN groups. In CPCoA results, soybean genotype and rhizobium inoculation significantly influenced the structure of rhizosphere fungal communities (11.6% of variance; P = 0.049) (Figure 7A). Rhizobium inoculation significantly impacted rhizosphere fungal populations around both LN and HN roots (Figure 7B). Interestingly, R_HN rhizosphere fungal communities were not distinct from those in N_LN plots (Figure 7A). In other words, the fungal community of HN under R conditions was similar in comparison to LN under N conditions. These results suggest that rhizobium inoculation induces different responses in HN and LN soybeans.
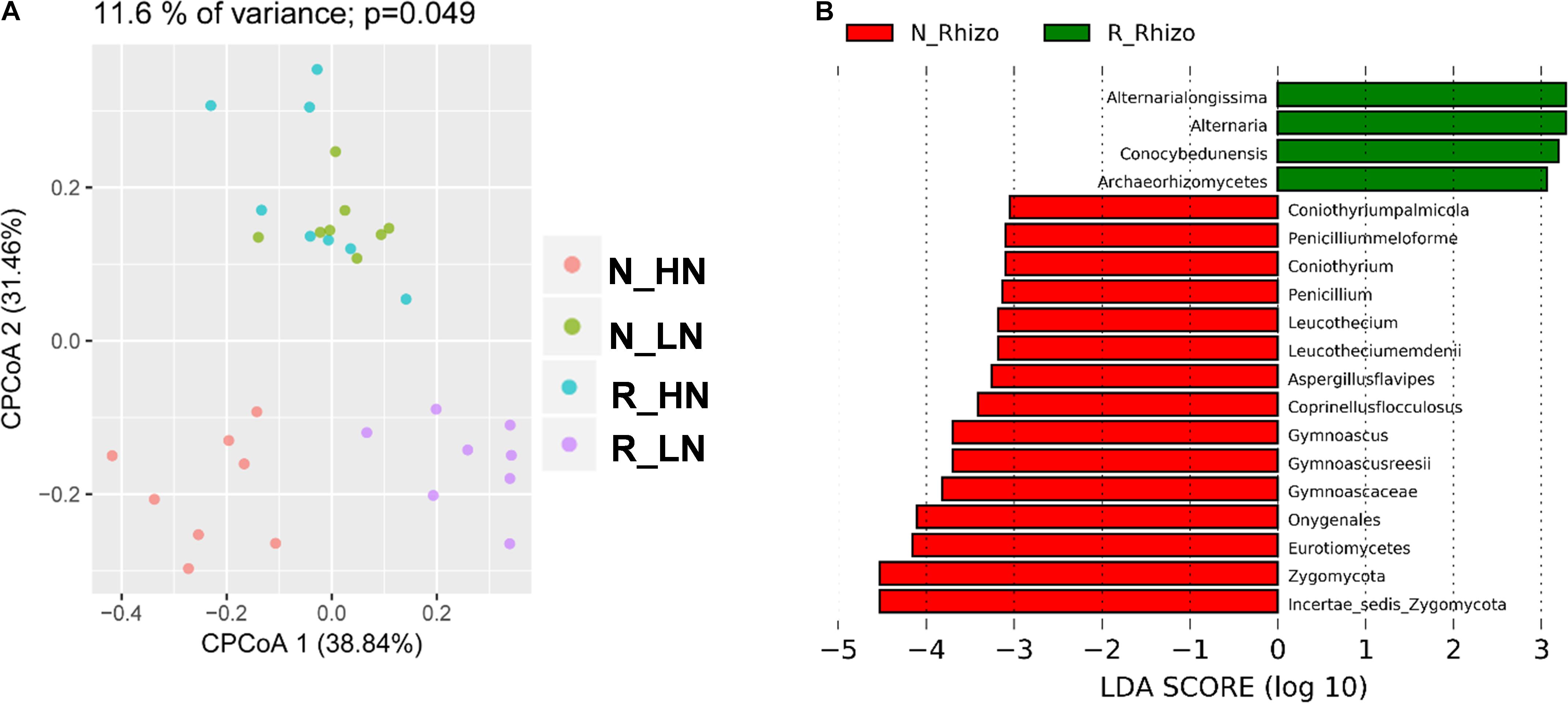
Figure 7. Differential responses among fungal community members to rhizobium inoculation. Data were grouped into N_HN, R_HN, N_LN, and R_LN categories to investigate the influence of nodulation traits on soybean rhizosphere fungal community responses to rhizobium inoculation. Samples were also grouped into N_Rhizo and R_Rhizo categories according to the rhizobium treatment. (A) CPCoA was carried out based on the Bray_Curtis distance matrix, with the P value calculated through ANOVA-like permutation analysis. (B) LDA scores > 3.0 for biomarkers of N_Rhizo and R_Rhizo grouped treatments. as calculated using the Kruskal–Wallis rank sum test, P < 0.05.
In more granular analysis, biomarkers were compared of P1, P2, HN, and LN between different rhizobium treatment conditions, respectively. These biomarkers ended up being entirely different between tested pairs of rhizospheres, suggesting that soybean responses to rhizobium inoculation may vary among soybean genotypes (Supplementary Figures S9, S10), which was further confirmed in group comparisons of N_HN with R_HN and N_LN with R_LN (Supplementary Figures S11A,B). Taken together, our results demonstrate that soybean rhizosphere fungal community responses to rhizobium inoculation are strongly influenced by genotypic differences at a few key markers associated with N2 fixation.
In a final analysis of impacts of rhizobium inoculation on soybean rhizosphere fungal communities, fungal co-occurrence networks were constructed for N and R communities based on the normalized OTU data. There were 100 nodes for both non-inoculated and inoculated rhizospheres. However, the number of connections varied between networks, with 78 found in the N network and 130 found in the R network (Figure 8). Plus, the hub fungi also changed with rhizobium inoculation. In non-inoculated plots, the hub rhizosphere fungi were Cladosporium, Pyrenochaetopsis, Sporobolomyces, Hannaella, and Phaeosphaeria (Figure 8A), while, with inoculation, the hub fungi shifted to Phaeosphaeria, Sporobolomyces, Septoria, Edenia, and Leptospora (Figure 8B). These results suggest that rhizobium inoculation leads to an increase in the number of connections between fungi, as well as, changes in which species act as hubs in the soybean rhizosphere fungal co-occurrence network.
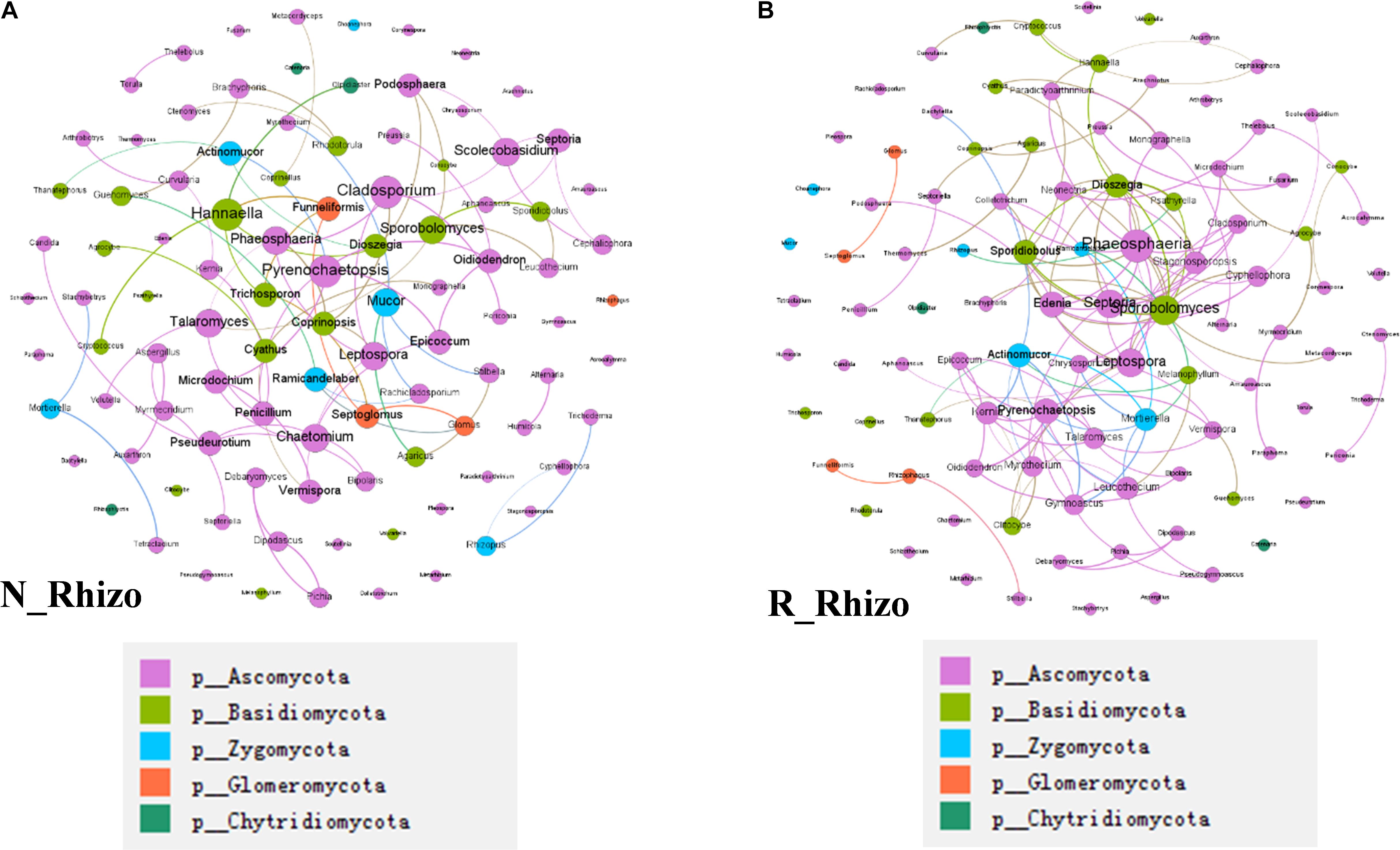
Figure 8. Rhizobium inoculation shifts rhizosphere fungal communities. Co-occurrence networks were constructed based on correlation analysis of the top 100 fungi in relative abundance as assessed at the genus taxonomic level. (A) Non-inoculated, node size is proportional to node degree, node color matches the taxa level identified for the fungus. Linkers drawn between nodes indicate significant (P < 0.05; Spearman’s rank correlation test) and high (Spearman’s r > 0.70) correlations. The number of nodes is 100 for (A,B). There are 91 connections among nodes in (A) and 81 node connections in (B). (A) The hub fungi for rhizospheres in non-inoculated plots were Cladosporium, Pyrenochaetopsis, Hannaella, Phaeosphaeria, and Sporobolomyces. (B) The hub fungi for LN soybean plants were Phaeosphaeria, Septeria, Sporobolomyces, Leptospora, and Spordidobolus. N_Rhizo: rhizosphere samples from non-inoculated plots. R_Rhizo: rhizosphere samples from rhizobium inoculated plots.
Discussion
Plant-associated microbial communities are essential for plant health and nutrient acquisition (Falkowski et al., 2008; Hacquard et al., 2015). Although microbiota have been reported in associations with many plant species (Edwards et al., 2015; Haney et al., 2015; Durán et al., 2018; Zhong et al., 2019), plant-associated fungi have received little attention. And only 5–10% of fungal community members were detectable by culture-dependent techniques (Hawksworth and Rossman, 1997; Hawksworth, 2001). In addition, interkingdom interactions among host plants and associated microbiota or among microbiota remain rarely reported. As rhizobium inoculation is a widely applied strategy in soybean production, this classic agriculture practice presents a practical means to study complex interactions between host plants and associated microbiota, including interkingdom microbial interactions between rhizobium and rhizosphere fungal community members.
Soybean Plants Select and Enrich Some Species of Fungi in the Rhizosphere
In this study, since most OTU overlapped between rhizospheres and bulk soils, only a few specific fungi appeared to be selected in soybean rhizospheres from bulk soils (Supplementary Figure S4). This is consistent with previous reports that soil type is the major factor determining the fungal and bacterial communities, as the soil is the source of microbes available to colonize plant roots (de Ridder-Duine et al., 2005; Singh et al., 2007; Wang et al., 2009). We found no significant differences in overall fungal richness between bulk soils and rhizospheres (Figures 1A,B and Supplementary Figures S2, S3), which is also consistent with a recent report that fungal community richness only declines in the root compartment, and may not significantly vary between rhizospheres and bulk soils (Almario et al., 2017). Interestingly, this pattern stands in contrast to bacterial community differences between bulk soils and rhizospheres, wherein bacterial richness is significantly lower in the rhizosphere than in bulk soils due to selection by host plants (Bulgarelli et al., 2012; Zhong et al., 2019).
Most of soybean rhizosphere fungi detected in this study were categorized as functionally unknown. However, the most studied plant-associated fungi belonged to Glomeromycota and Ectomycorrhizal (Bonito et al., 2014). These functional known fungi might include members that benefit plants lacking associations with mycorrhizal fungi, such as A. thaliana, Arabisalpina, and Microthlaspi (Glynou et al., 2016; Hiruma et al., 2016; Almario et al., 2017; Hassani et al., 2018). We found that the dominant fungi in soybean rhizospheres were Ascomycota, Zygomycota, and Basidiomycota (Figure 1C and Supplementary Figure S5), which is consistent with previous reports that the two major phyla Ascomycota and Basidiomycota are the dominate fungi in the rhizosphere and within soybean plants (Jumpponen and Jones, 2009; Wang et al., 2009; Toju et al., 2013; Hardoim et al., 2015). Not surprisingly, Ascomycetes and Basidiomycetes have also been reported as the dominated fungi in soils (Anderson et al., 2003; Bastias et al., 2006). At the genus taxonomic level, Cladosporium and Pyrenochaetopsis were the most abundant taxa in the soybean rhizospheres observed herein (Supplementary Figure S6).
Although overall fungal community richness was similar between rhizosphere and bulk soil samples (Figure 1A and Supplementary Figure S3), PCA, NMDS, and ANOSIM analysis suggested that the structure and composition of fungal communities varied between soybean rhizospheres and bulk soils (Figure 2). This result is consistent with previous reports, in which soybean plants could recruit some species of fungi from bulk soils (Mougel et al., 2006; Wang et al., 2009; Almario et al., 2017; Zimudzi et al., 2018). In this study we further identified the fungi which were specific enriched by the soybean plant (Figures 3B,C).
Functional characterization of the fungi abundant in soybean rhizospheres is largely unexplored. Among the few known functions, a Cladosporium sp. isolated from soybean plants is known to exhibit GA biosynthesis activity, along with the capacity to promote plant growth (Hamayun et al., 2009). Other fungi reported to be enriched by soybean plants are Septoria spp. (Figure 6), which have been reported to be phytopathgens of wheat, but with unknown functions in soybean rhizospheres (Arraiano and Brown, 2017). A third type of enriched fungus, Phaeosphaeris, has been reported to produce a variety of chemical compounds, such as pyrazine alkaloids, isocoumarins, perylenequinones, and diterpenes (El-Demerdash, 2018). In short, though few specifics are known, soybean plants appear to select some species of fungi from bulk soils, which leads to distinctive rhizosphere fungal communities that likely fulfill specific functions in partnership with the host plants.
Soybean Nodulation Is Associated With Rhizosphere Fungal Community Dynamics
Previous studies reported that the assembly of plant-associated fungal communities is not only dependent on soil type and plant compartments, but also on plant genotype and other environmental factors (Gottel et al., 2011; Cordier et al., 2012; Balint et al., 2013; Shakya et al., 2013). In this study, we demonstrated that soybean genotype significantly influenced the structure of rhizosphere fungal communities (Figure 4A and Supplementary Figure S8). This result stands in contrast to a former report in which no significant differences were detected among fungal communities associating with three soybean cultivars (Wang et al., 2009), possibly due to differences in profile methods, soil types and soybean genotypes used in the studies (Wang et al., 2009; Simon and Daniel, 2011; Zhong et al., 2019). This indicated the genetic traits that control rhizobium symbiosis might be also involved in the regulation of fungal community or rhizosphere fungi (Genre and Russo, 2016). Consistent with our results, a recent paper showed that the key genes in the symbiotic signaling pathway significantly influenced the enrichment of some fungi in both rhizosphere and within the roots (Thiergart et al., 2019).
In this study, Cladosporium sp. was significantly enriched in the rhizosphere of the high nodulation cultivar P2 (Figures 4B,C). This fungus not only was most abundant in the P2 rhizosphere, but was also exhibited higher relative abundance in high nodulation RILs compared with low nodulation RILs (Figure 3 and Supplementary Figure S6). However, Cladosporium was not included in a previous report on fungal communities in soybean rhizospheres (Wang et al., 2009), which might due to differences in soil types and/or soybean genotypes studied (Wieland et al., 2001; Singh et al., 2007; Wang et al., 2009). Other studies have suggested that Cladosporium spp. were the most frequently isolated fungi from eggplant roots (Narisawa et al., 2002) and soybean plants (Pimentel et al., 2006; Pan et al., 2008; Hamayun et al., 2009). However, outlining the exact functions of Cladosporium sp. in soybean microbiomes will require further experimentation. The other fungus significantly enriched in the P2 rhizosphere, Dothideomycetes, has been most commonly isolated from porous Antarctic rocks (Selbmann et al., 2005, 2008; Egidi et al., 2014). Whatever explanations account for selection processes in soybean rhizospheres, the results generated herein suggest that rhizosphere fungal communities are strongly influenced by variation among soybean genotypes (Supplementary Figure S9). This variation might be attributable to differences in root exudates produced by different genotypes of soybean (Ryan et al., 2001; Hu et al., 2018; Stringlis et al., 2018; Zhalnina et al., 2018; Huang et al., 2019).
Rhizobium Inoculation Shifts the Rhizosphere Fungal Community in a Genotype Dependent Manner
Bacterial and fungal communities are the major microbial constituents of plant rhizospheres (Berendsen et al., 2012). In a previous report, we showed that rhizobium inoculation has a significant influence on the structure of rhizosphere bacterial communities in a genotype dependent manner (Zhong et al., 2019). In contrast, other studies have shown that rhizosphere fungal communities are more stable than rhizosphere bacterial communities (de Vries et al., 2018). In this study, introduction of exogenous rhizobia also changed fungal communities in soybean rhizospheres (Figure 5), with relative abundance of some fungi being significantly increased under rhizobium inoculation condition (Supplementary Figure S10A; Hamayun et al., 2009). Intriguingly, rhizobium inoculation produced different responses in the rhizospheres of two parental soybean genotypes contrasting in BNF traits and markers (Supplementary Figure S10). These results indicated that the influence of rhizobium inoculation to the fungal community is mediated by the plant genotypes, since different genotypes of soybean exhibited different responses to rhizobium inoculation. But does rhizobium inoculation influence the nitrogen status in the plant and then influenced the associated fungi needed further investigation.
After crossing the parent lines, further study with RILs varying across QTLs identified as responsible for much of BNF allowed for more in depth analysis of soybean genotypic effects on rhizosphere fungal communities in a second year of field experiments (Yang et al., 2017). The two RIL groups, categorized on the basis of BNF QTLs, varied in their rhizosphere fungal community responses to rhizobium inoculation (Figure 7A). Taken together, results from experiments with parents and RILs reinforce the conclusion that soybean rhizosphere fungal communities are impacted by soybean genotypic variation (Figure 7A and Supplementary Figures S10, S11). More specifically, QTLs identified in BNF studies, and that are known to contribute to the establishment of bacterial communities, were also found in this study to influence rhizosphere fungal communities. On the whole, results presented here suggest that soybean genotypic influences on rhizosphere fungal communities exhibit differential responses to rhizobium inoculation. While soybean rhizosphere fungal communities appear to be more stable than bacterial communities, rhizobium inoculation still influences the rhizosphere fungal community, which suggests that complex direct and indirect interactions exist between host plants and root microbiota from multiple kingdoms of organisms.
Conclusion
In this study, we demonstrated that soybean plants could recruit certain fungi from bulk soils, which led to distinctive fungal communities in soybean rhizospheres. Soybean genotypic variation played an important role in the establishment of the rhizosphere fungal community. In addition, rhizobium inoculation also changed rhizosphere fungal communities, with the relative abundance of certain rhizosphere fungi significantly altered in directions that varied among soybean genotypes. In short, this study contributes to our understanding that complex interkingdom interactions occur in soybean rhizospheres, which might be useful in attempts to develop new strategies to improve crop performance through manipulation of rhizosphere microorganisms.
Data Availability Statement
The raw data supporting the conclusion of this article is deposited in the NCBI: https://www.ncbi.nlm.nih.gov/bioproject/PRJNA597193.
Author Contributions
HL, YZ, and YY designed the research. YY and RX performed the research. YZ, HX, and YT analyzed the data. YZ, HX, and HL wrote the manuscript.
Funding
This work was jointly supported by the Strategic Priority Research Program of the Chinese Academy of Sciences (XDB15030202) and China National Key Program for Research and Development (2016YFD0100700). HX was supported by the K+S Group scholarship from the International Magnesium Institute.
Conflict of Interest
The authors declare that the research was conducted in the absence of any commercial or financial relationships that could be construed as a potential conflict of interest.
Acknowledgments
We thank Dr. Thomas Walk for valuable suggestions and comments.
Supplementary Material
The Supplementary Material for this article can be found online at: https://www.frontiersin.org/articles/10.3389/fmicb.2019.03135/full#supplementary-material
Footnotes
- ^ http://drive5.com/uparse/
- ^ http://unite.ut.ee/
- ^ http://qiime.org/scripts/assign_taxonomy.html
- ^ http://www.drive5.com/muscle/
- ^ http://huttenhower.sph.harvard.edu/galaxy/root/index
- ^ http://gephi.github.io/
References
Agler, M. T., Ruhe, J., Kroll, S., Morhenn, C., Kim, S. T., Weigel, D., et al. (2016). Microbial hub taxa link host and abiotic factors to plant microbiome variation. PLoS Biol. 14:e1002352. doi: 10.1371/journal.pbio.1002352
Almario, J., Jeena, G., Wunder, J., Langen, G., Zuccaro, A., Coupland, G., et al. (2017). Root-associated fungal microbiota of nonmycorrhizal Arabis alpina and its contribution to plant phosphorus nutrition. Proc. Natl. Acad. Sci. U.S.A. 114, 9403–9412. doi: 10.1073/pnas.1710455114
Altschul, S. F., Gish, W., Miller, W., Myers, E. W., and Lipman, D. J. (1990). Basic local alignment search tool. J. Mol. Biol. 215, 403–410. doi: 10.1006/jmbi.1990.9999
Anderson, I. C., Campbell, C. D., and Prosser, J. I. (2003). Diversity of fungi in organic soils under a moorland - Scots pine (Pinus sylvestris L.) gradient. Environ. Microbiol. 5, 1121–1132. doi: 10.1046/j.1462-2920.2003.00522.x
Arraiano, L. S., and Brown, J. K. (2017). Sources of resistance and susceptibility to Septoria tritici blotch of wheat. Mol. Plant Pathol. 18, 276–292. doi: 10.1111/mpp.12482
Arslanoglu, F., Aytac, S., and Oner, E. K. (2011). Effect of genotype and environment interaction on oil and protein content of soybean (Glycine max (L.) Merrill) seed. Afr. J. Biotechnol. 10, 18409–18417.
Bakshi, M., Vahabi, K., Bhattacharya, S., Sherameti, I., Varma, A., Yeh, K. W., et al. (2015). WRKY6 restricts Piriformospora indica-stimulated and phosphate-induced root development in Arabidopsis. BMC Plant Biol. 15:305. doi: 10.1186/s12870-015-0673-4
Balint, M., Tiffin, P., Hallstrom, B., O’Hara, R. B., Olson, M. S., Fankhauser, J. D., et al. (2013). Host genotype shapes the foliar fungal microbiome of balsam poplar (Populus balsamifera). PLoS One 8:e053987. doi: 10.1371/journal.pone.0053987
Bastias, B. A., Xu, Z., and Cairney, J. W. (2006). Influence of long-term repeated prescribed burning on mycelial communities of ectomycorrhizal fungi. New Phytol. 172, 149–158. doi: 10.1111/j.1469-8137.2006.01793.x
Berendsen, R. L., Pieterse, C. M., and Bakker, P. A. (2012). The rhizosphere microbiome and plant health. Trends Plant Sci. 17, 478–486. doi: 10.1016/j.tplants.2012.04.001
Bokulich, N. A., Subramanian, S., Faith, J. J., Gevers, D., Gordon, J. I., Knight, R., et al. (2013). Quality-filtering vastly improves diversity estimates from Illumina amplicon sequencing. Nat. Methods 10, 57–59. doi: 10.1038/nmeth.2276
Bonito, G., Reynolds, H., Robeson, M. S. II, Nelson, J., Hodkinson, B. P., Tuskan, G., et al. (2014). Plant host and soil origin influence fungal and bacterial assemblages in the roots of woody plants. Mol. Ecol. 23, 3356–3370. doi: 10.1111/mec.12821
Brundrett, M. C., and Tedersoo, L. (2018). Evolutionary history of mycorrhizal symbioses and global host plant diversity. New Phytol. 220, 1108–1115. doi: 10.1111/nph.14976
Bucher, M. (2007). Functional biology of plant phosphate uptake at root and mycorrhiza interfaces. New Phytol. 173, 11–26. doi: 10.1111/j.1469-8137.2006.01935.x
Buée, M., De Boer, W., Martin, F., van Overbeek, L., and Jurkevitch, E. (2009). The rhizosphere zoo: an overview of plant-associated communities of microorganisms, including phages, bacteria, archaea, and fungi, and of some of their structuring factors. Plant Soil 321, 189–212. doi: 10.1007/s11104-009-9991-3
Bulgarelli, D., Rott, M., Schlaeppi, K., Ver Loren van Themaat, E., Ahmadinejad, N., Assenza, F., et al. (2012). Revealing structure and assembly cues for Arabidopsis root-inhabiting bacterial microbiota. Nature 488, 91–95. doi: 10.1038/nature11336
Caporaso, J. G., Kuczynski, J., Stombaugh, J., Bittinger, K., Bushman, F. D., Costello, E. K., et al. (2010). QIIME allows analysis of highthroughput community sequencing data. Nat. Methods 7, 335–336. doi: 10.1038/nmeth.f.303
Chen, F. X., Cao, G. Q., Wang, X. R., Zhao, J., Yan, X. L., and Liao, H. (2008). Isolation and application of effective rhizobium in soybean at acidic and low phosphate soil in South China. Chin. Sci. Bull. 23, 2903–2910.
Cordier, T., Robin, C., Capdevielle, X., Fabreguettes, O., Desprez-Loustau, M. L., and Vacher, C. (2012). The composition of phyllosphere fungal assemblages of European beech (Fagus sylvatica) varies significantly along an elevation gradient. New Phytol. 196, 510–519. doi: 10.1111/j.1469-8137.2012.04284.x
de Ridder-Duine, A. S., Kowalchuk, G. A., Klein Gunnewiek, P. J. A., Smant, W., van Veen, J. A., and de Boer, W. (2005). Rhizosphere bacterial community composition in natural stands of Carex arenaria (sand sedge) is determined by bulk soil community composition. Soil Biol. Biochem. 37, 349–357. doi: 10.1016/j.soilbio.2004.08.005
de Vries, F. T., Griffiths, R. I., Bailey, M., Craig, H., Girlanda, M., Gweon, H. S., et al. (2018). Soil bacterial networks are less stable under drought than fungal networks. Nat. Commun. 9, 1–12. doi: 10.1038/s41467-018-05516-7
Deshpande, M. V. (1999). Mycopesticide production by fermentation: potential and challenges. Crit. Rev. Microbiol. 25, 229–243. doi: 10.1080/10408419991299220
Doyle, J. J., and Luckow, M. A. (2003). The rest of the iceberg. Legume diversity and evolution in a phylogenetic context. Plant Physiol. 131, 900–910. doi: 10.1104/pp.102.018150
Durán, P., Thiergart, T., Garrido-Oter, R., Agler, M., Kemen, E., Schulze-Lefert, P., et al. (2018). Microbial interkingdom interactions in roots promote Arabidopsis survival. Cell 175, 973–983. doi: 10.1016/j.cell.2018.10.020
Edgar, R. C. (2004). MUSCLE: multiple sequence alignment with high accuracy and high throughput. Nucleic Acids Res. 32, 1792–1797. doi: 10.1093/nar/gkh340
Edgar, R. C. (2013). UPARSE: highly accurate OTU sequences from microbial amplicon reads. Nat. Methods 10, 996–998. doi: 10.1038/nmeth.2604
Edwards, J., Johnson, C., Santos-Medellin, C., Lurie, E., Podishetty, N. K., Bhatnagar, S., et al. (2015). Structure, variation, and assembly of the root-associated microbiomes of rice. Proc. Natl. Acad. Sci. U.S.A. 112, 911–920.
Egidi, E., de Hoog, G. S., Isola, D., Onofri, S., Quaedvlieg, W., de Vries, M., et al. (2014). Phylogeny and taxonomy of meristematic rock-inhabiting black fungi in the Dothideomycetes based on multi-locus phylogenies. Fungal Divers. 65, 127–165. doi: 10.1007/s13225-013-0277-y
El-Demerdash, A. (2018). Chemical diversity and biological activities of Phaeosphaeria fungi genus: a systematic review. J. Fungi 5:E130. doi: 10.3390/jof4040130
Falkowski, P. G., Fenchel, T., and Delong, E. F. (2008). The microbial engines that drive Earth’s biogeochemical cycles. Science 320, 1034–1039. doi: 10.1126/science.1153213
Field, K. J., Pressel, S., Duckett, J. G., Rimington, W. R., and Bidartondo, M. I. (2015). Symbiotic options for the conquest of land. Trends Ecol. Evol. 30, 477–486. doi: 10.1016/j.tree.2015.05.007
Findley, K., Oh, J., Yang, J., Conlan, S., Deming, C., Meyer, J. A., et al. (2013). Topographic diversity of fungal and bacterial communities in human skin. Nature 498, 367–370. doi: 10.1038/nature12171
Garbaye, J. (1994). Tansley Review No. 76 Helper bacteria: a new dimension to the mycorrhizal symbiosis. New Phytol. 128, 197–210. doi: 10.1111/j.1469-8137.1994.tb04003.x
Genre, A., and Russo, G. (2016). Does a common pathway transduce symbiotic signals in plant-microbe interactions? Front. Plant Sci. 7:96. doi: 10.3389/fpls.2016.00096
Gerland, P., Raftery, A. E., Sevcikova, H., Li, N., Gu, D., Spoorenberg, T., et al. (2014). World population stabilization unlikely this century. Science 346, 234–237. doi: 10.1126/science.1257469
Glynou, K., Ali, T., Buch, A. K., Haghi Kia, S., Ploch, S., Xia, X., et al. (2016). The local environment determines the assembly of root endophytic fungi at a continental scale. Environ. Microbiol. 18, 2418–2434. doi: 10.1111/1462-2920.13112
Gottel, N. R., Castro, H. F., Kerley, M., Yang, Z., Pelletier, D. A., Podar, M., et al. (2011). Distinct microbial communities within the endosphere and rhizosphere of Populus deltoides roots across contrasting soil types. Appl. Environ. Microbiol. 77, 5934–5944. doi: 10.1128/AEM.05255-11
Hacquard, S., Garrido-Oter, R., Gonzalez, A., Spaepen, S., Ackermann, G., Lebeis, S., et al. (2015). Microbiota and host nutrition across plant and animal kingdoms. Cell Host Microbe 17, 603–616. doi: 10.1016/j.chom.2015.04.009
Hamayun, M., Afzal Khan, S., Ahmad, N., Tang, D.-S., Kang, S.-M., Na, C.-I., et al. (2009). Cladosporium sphaerospermum as a new plant growth-promoting endophyte from the roots of Glycine max (L.) Merr. World J. Microbiol. Biotechnol. 25, 627–632. doi: 10.1007/s11274-009-9982-9
Haney, C. H., Samuel, B. S., Bush, J., and Ausubel, F. M. (2015). Associations with rhizosphere bacteria can confer an adaptive advantage to plants. Nat. Plants 1, 1–9.
Hardoim, P. R., van Overbeek, L. S., Berg, G., Pirttila, A. M., Compant, S., Campisano, A., et al. (2015). The hidden world within plants: ecological and evolutionary considerations for defining functioning of microbial endophytes. Microbiol. Mol. Biol. Rev. 79, 293–320. doi: 10.1128/MMBR.00050-14
Hassani, M. A., Duran, P., and Hacquard, S. (2018). Microbial interactions within the plant holobiont. Microbiome 6, 1–17.
Hawksworth, D. L. (2001). The magnitude of fungal diversity: the 1.5 million species estimate revisited. Mycol. Res. 105, 1422–1432. doi: 10.1128/microbiolspec.FUNK-0052-2016
Hawksworth, D. L., and Rossman, A. Y. (1997). Where are all the undescribed fungi? Phytopathology 87, 888–891. doi: 10.1094/PHYTO.1997.87.9.888
Herridge, D. F., Peoples, M. B., and Boddey, R. M. (2008). Global inputs of biological nitrogen fixation in agricultural systems. Plant Soil 311, 1–18. doi: 10.1007/s11104-008-9668-3
Hiruma, K., Gerlach, N., Sacristan, S., Nakano, R. T., Hacquard, S., Kracher, B., et al. (2016). Root endophyte Colletotrichum tofieldiae confers plant fitness benefits that are phosphate status dependent. Cell 165, 464–474. doi: 10.1016/j.cell.2016.02.028
Hu, L., Robert, C. A. M., Cadot, S., Zhang, X., Ye, M., Li, B., et al. (2018). Root exudate metabolites drive plant-soil feedbacks on growth and defense by shaping the rhizosphere microbiota. Nat. Commun. 9:2738. doi: 10.1038/s41467-018-05122-7
Huang, A. C., Jiang, T., Liu, Y. X., Bai, Y. C., Reed, J., Qu, B., et al. (2019). A specialized metabolic network selectively modulates Arabidopsis root microbiota. Science 364:6440. doi: 10.1126/science.aau6389
Igiehon, N. O., and Babalola, O. O. (2018). Below-ground-above-ground plant-microbial interactions: focusing on soybean, rhizobacteria and mycorrhizal fungi. Open Microbiol. J. 12, 261–279. doi: 10.2174/1874285801812010261
Inceoglu, O., Hoogwout, E. F., Hill, P., and van Elsas, J. D. (2010). Effect of DNA extraction method on the apparent microbial diversity of soil. Appl. Environ. Microbiol. 76, 3378–3382. doi: 10.1128/AEM.02715-09
Jumpponen, A., and Jones, K. L. (2009). Massively parallel 454 sequencing indicates hyperdiverse fungal communities in temperate Quercus macrocarpa phyllosphere. New Phytol. 184, 438–448. doi: 10.1111/j.1469-8137.2009.02990.x
Katoch, M., and Pull, S. (2017). Endophytic associated with Monarda citriodora, an aromatic and medicinal plant and their biocontrol potential. Pharm. Biol. 1, 1528–1535. doi: 10.1080/13880209.2017.1309054
Kõljalg, U., Nilsson, R. H., Abarenkov, K., Tedersoo, L., Taylor, A. F., Bahram, M., et al. (2013). Towards a unified paradigm for sequence-based identification of fungi. Mol. Ecol. 22, 5271–5277. doi: 10.1111/mec.12481
Lindow, S. E., and Brandl, M. T. (2003). Microbiology of the phyllosphere. Appl. Environ. Microbiol. 69, 1875–1883. doi: 10.1128/aem.69.4.1875-1883.2003
Magoč, T., and Steven, L. S. (2011). FLASH: fast length adjustment of short reads to improve genome assemblies. Bioinformatics 27, 2957–2963. doi: 10.1093/bioinformatics/btr507
Masella, A. P., Bartram, A. K., Truszkowski, J. M., Brown, D. G., and Neufeld, J. D. (2012). PANDAseq: paired-end assembler for illumina sequences. BMC Bioinformatics 14:13–31. doi: 10.1186/1471-2105-13-31
Mougel, C., Offre, P., Ranjard, L., Corberand, T., Gamalero, E., Robin, C., et al. (2006). Dynamic of the genetic structure of bacterial and fungal communities at different developmental stages of Medicago truncatula Gaertn. cv. Jemalong line J5. New Phytol. 170, 165–175. doi: 10.1111/j.1469-8137.2006.01650.x
Narisawa, K., Kawamata, H., Currah, R. S., and Hashiba, T. (2002). Suppression of Verticillium wilt in eggplant by some fungal root endophytes. Eur. J. Plant Pathol. 108, 103–109.
Oksanen, J., Blanchet, F. G., and Kindt, R. (2015). Vegan: Community Ecology Package. R Package Version 2.3-0.
Pan, J. J., Baumgarten, A. M., and May, G. (2008). Effects of host plant environment and Ustilago maydis infection on the fungal endophyte community of maize (Zea mays). New Phytol. 178, 147–156. doi: 10.1111/j.1469-8137.2007.02350.x
Pandey, M. K., Roorkiwal, M., Singh, V. K., Ramalingam, A., Kudapa, H., Thudi, M., et al. (2016). Emerging genomic tools for legume breeding: current status and future prospects. Front. Plant Sci. 7:455. doi: 10.3389/fpls.2016.00455
Parks, D. H., Tyson, G. W., Hugenholtz, P., and Beiko, R. G. (2014). STAMP: statistical analysis of taxonomic and functional profiles. Bioinformatics 30, 3123–3124. doi: 10.1093/bioinformatics/btu494
Pimentel, I. C., Glienke-Blanco, C., Gabardo, J., Stuart, R. M., and Azevedo, J. L. (2006). Identification and colonization of endophytic fungi from soybean (Glycine max (L.) Merril) under different environmental conditions. Br. Arch. Biol. Technol. 49, 705–711. doi: 10.1590/s1516-89132006000600003
Qin, L., Jiang, H., Tian, J., Zhao, J., and Liao, H. (2011). Rhizobia enhance acquisition of phosphorus from different sources by soybean plants. Plant Soil 349, 25–36. doi: 10.1007/s11104-011-0947-z
R Core Team (2015). R: A Language and Environment for Statistical Computing. Vienna: R Foundation for Statistical Computing.
Rosenberg, E., and Zilber-Rosenberg, I. (2016). Microbes drive evolution of animals and plants: the hologenome concept. mBio 7, 1–8. doi: 10.1128/mBio.01395-15
Ryan, P., Delhaize, E., and Jones, D. (2001). Function and mechanism of organic anion exudation from plant roots. Annu. Rev. Plant Physiol. Plant Mol. Biol. 52, 527–560.
Selbmann, L., de Hoog, G. S., Mazzaglia, A., Friedmann, E. I., and Onofri, S. (2005). Fungi at the edge of life: cryptoendolithic black fungi from Antarctic desert. Stud. Mycol. 51, 1–32.
Selbmann, L., de Hoog, G. S., Zucconi, L., Isola, D., Ruisi, S., van den Ende, A. G., et al. (2008). Drought meets acid: three new genera in a dothidealean clade of extremotolerant fungi. Stud. Mycol. 61, 1–20. doi: 10.3114/sim.2008.61.01
Shakya, M., Gottel, N., Castro, H., Yang, Z. K., Gunter, L., Labbe, J., et al. (2013). A multifactor analysis of fungal and bacterial community structure in the root microbiome of mature Populus deltoides trees. PLoS One 8:e076382. doi: 10.1371/journal.pone.0076382
Simon, C., and Daniel, R. (2011). Metagenomic analyses: past and future trends. Appl. Environ. Microbiol. 77, 1153–1161. doi: 10.1128/AEM.02345-10
Singh, B. K., Munro, S., Potts, J. M., and Millard, P. (2007). Influence of grass species and soil type on rhizosphere microbial community structure in grassland soils. Appl. Soil Ecol. 36, 147–155. doi: 10.1016/j.apsoil.2007.01.004
Singh, G., Katoch, A., Razak, M., Kitchlu, S., Goswami, A., and Katoch, M. (2017). Bioactive and biocontrol potential of endophytic fungi associated with Brugmansia aurea Lagerh. FEMS Microbiol. Lett. 15:364. doi: 10.1093/femsle/fnx194
Stringlis, I. A., Yu, K., Feussner, K., de Jonge, R., Van Bentum, S., Van Verk, M. C., et al. (2018). MYB72-dependent coumarin exudation shapes root microbiome assembly to promote plant health. Proc. Natl. Acad. Sci. U.S.A. 22, 5213–5222. doi: 10.1073/pnas.1722335115
Thiergart, T., Zgadzaj, R., Bozsóki, Z., Garrido-Oter, R., Radutoiu, S., and Schulze-Lefert, P. (2019). Lotus japonicus symbiosis genes impact microbial interactions between symbionts and multikingdom commensal communities. mBio 5:e1833-19. doi: 10.1128/mBio.01833-19
Thomas, M. B., and Read, A. F. (2007). Can fungal biopesticides control malaria? Nat. Rev. Microbiol. 5, 377–383. doi: 10.1038/nrmicro1638
Toju, H., Yamamoto, S., Sato, H., and Tanabe, A. S. (2013). Sharing of diverse mycorrhizal and root-endophytic fungi among plant species in an oak-dominated cool-temperate forest. PLoS One 8:e078248. doi: 10.1371/journal.pone.0078248
van der Heijden, M. G., Martin, F. M., Selosse, M. A., and Sanders, I. R. (2015). Mycorrhizal ecology and evolution: the past, the present, and the future. New Phytol. 205, 1406–1423. doi: 10.1111/nph.13288
Vorholt, J. A. (2012). Microbial life in the phyllosphere. Nat. Rev. Microbiol. 10, 828–840. doi: 10.1038/nrmicro2910
Wang, G., Xu, Y., Jin, J., Liu, J., Zhang, Q., and Liu, X. (2009). Effect of soil type and soybean genotype on fungal community in soybean rhizosphere during reproductive growth stages. Plant Soil 317, 135–144. doi: 10.1007/s11104-008-9794-y
Wang, X., Yan, X., and Liao, H. (2010). Genetic improvement for phosphorus efficiency in soybean: a radical approach. Ann. Bot. 106, 215–222. doi: 10.1093/aob/mcq029
Wieland, G., Neumann, R., and Backhaus, H. (2001). Variation of microbial communities in soil, rhizosphere, and rhizoplane in response to crop species, soil type, and crop development. Appl. Environ. Microbiol. 67, 5849–5854. doi: 10.1128/aem.67.12.5849-5854.2001
Yang, S. Y., Grønlund, M., Jakobsen, I., Grotemeyer, M. S., Rentsch, D., Miyao, A., et al. (2012). Nonredundant regulation of rice arbuscular mycorrhizal symbiosis by two members of the phosphate transporter1 gene family. Plant Cell 24, 4236–4251. doi: 10.1105/tpc.112.104901
Yang, Y., Zhao, Q., Li, X., Ai, W., Liu, D., Qi, W., et al. (2017). Characterization of genetic basis on synergistic interactions between root architecture and biological nitrogen fixation in soybean. Front. Plant Sci. 8:1466. doi: 10.3389/fpls.2017.01466
Zhalnina, K., Louie, K. B., Hao, Z., Mansoori, N., da Rocha, U. N., Shi, S., et al. (2018). Dynamic root exudate chemistry and microbial substrate preferences drive patterns in rhizosphere microbial community assembly. Nat. Microbiol. 3, 470–480. doi: 10.1038/s41564-018-0129-3
Zhong, Y., Yang, Y., Liu, P., Xu, R., Rensing, C., Fu, X., et al. (2019). Genotype and rhizobium inoculation modulate the assembly of soybean rhizobacterial communities. Plant Cell Environ. 42, 2028–2044. doi: 10.1111/pce.13519
Keywords: rhizobium inoculation, soybean, fungal community, rhizosphere, microbiome
Citation: Xu H, Yang Y, Tian Y, Xu R, Zhong Y and Liao H (2020) Rhizobium Inoculation Drives the Shifting of Rhizosphere Fungal Community in a Host Genotype Dependent Manner. Front. Microbiol. 10:3135. doi: 10.3389/fmicb.2019.03135
Received: 30 October 2019; Accepted: 26 December 2019;
Published: 21 January 2020.
Edited by:
Ying Ma, University of Coimbra, PortugalReviewed by:
Birinchi Kumar Sarma, Banaras Hindu University, IndiaIoannis Stringlis, Utrecht University, Netherlands
Copyright © 2020 Xu, Yang, Tian, Xu, Zhong and Liao. This is an open-access article distributed under the terms of the Creative Commons Attribution License (CC BY). The use, distribution or reproduction in other forums is permitted, provided the original author(s) and the copyright owner(s) are credited and that the original publication in this journal is cited, in accordance with accepted academic practice. No use, distribution or reproduction is permitted which does not comply with these terms.
*Correspondence: Yongjia Zhong, WW9uZ2ppYVpob25nQGZhZnUuZWR1LmNvbQ==