- 1Laboratory of Tissue Engineering, College of Life Sciences, Northwest University, Xi'an, China
- 2Laboratory for Functional Glycomics, College of Life Sciences, Northwest University, Xi'an, China
- 3Key Laboratory of Resource Biology and Biotechnology in Western China Ministry of Education, Xi'an, China
- 4Provincial Key Laboratory of Biotechnology of Shaanxi, Xi'an, China
Lung cancer is a disease that influences human health and has become a leading cause of cancer mortality worldwide. However, it is frequently diagnosed at the advanced stage. It is necessary by means of biology to identify specific lung tumor biomarkers with high sensitivity. Glycosylation is one of the most important post-translational modifications and is related to many different diseases. It is involved in numerous essential biological processes, such as cell proliferation, differentiation, migration, cell-cell integrity and recognition, and immune modulation. However, little was known about deregulation of glycosylation in lung cancer and contribution to tumor–microenvironment interactions. Among the numerous glycosylations, fucosylation is the most common modification of glycoproteins and glycosylated oligosaccharides. Increased levels of fucosylation have been detected in various pathological conditions, as well as in lung cancer. In this article, we reviewed the role of fucosylation in lung cancer. We highlighted some of the fucosylation alterations currently being pursued in sera or tissues of lung cancer patients. Moreover, we elaborated on the regulation mechanism of fucosylation in proliferative invasion and metastasis of lung tumor cells. In summary, alterations in fucosylation provide potential biomarkers and therapeutic targets in lung cancer.
Introduction
Lung cancer, a primary cause of death, has increasing incidence and mortality rates worldwide (1–3). According to the World Health Organization (WHO) classification (4–6), lung cancer is categorized into small cell lung cancer (SCLC) and non-SCLC (NSCLC), which can be further subdivided into three major categories: adenocarcinomas (ADCs), squamous cell carcinomas (SQCCs), and large cell carcinomas (LCs). Because of the early stage patients not obvious symptom, most patients with lung cancer are diagnosed in the terminal stage and are estimated to have a survival time lower than 5 years. Now commonly used the methods for early diagnosis of lung cancer are Magnetic Resonance Imaging (MRI), Positron Emission Tomography-Computed Tomography (PET-CT), Endo-Bronchial Ultra-Sound (EBUS), Endocytoscopy (EC), Transbronchial Needle Aspiration (TBNA), and combined Testing of Serum Tumor Markers et al. However, there is a favorable survival rate in patients with earl stage lung cancer. As a result, searching for new biomarkers with high sensitivity and specificity to lung cancer will provide us with critical evidence in early diagnosis, therapy guidance, and prognosis monitoring. It has significance in increasing lung cancer survivability.
Aberrant glycosylation occurs frequently during carcinogenesis. The occurrence and development of malignant disease is accompanied by glycosylation (7). Most of the secreted and cell-surface proteins are glycoproteins which contain different types of oligosaccharide glycans covalently attached to amino acid side-chains are modified by different types of glycan structures. Glycosylation is orchestrated by numerous glycosyltransferases and glycosidases. The N- and O-linked glycan structures of glycoproteins serve different functions related to cell-cell communication. By using systemic glycomics approaches, we can further understand the functional roles of glycansin under physiological and pathological conditions (8). The alteration of the glycan number and structure are tightly related to cancer progression, invasion, metastasis, and epithelial–mesenchymal transition (EMT) in cancer cells (9–11). Studies suggested that factors like sex, age, and blood group are related to glycosylation (12–15). The age- and sex-associated differences in the glycopatterns of human glycoproteins may help us understand some age related to diseases and physiological phenomena specific to women or men. But researches about the glycosylation in different cancer patients with different sex, age, and blood group are seldom. More attention should be paid to this filed. Some glycoproteins have been used as diagnostic indexes of cancer, such as alpha feto-protein (AFP) and carcinoembryonic antigen (16, 17). Extensive progress has been achieved in the research of the glycosylation changes in lung cancer in recent years, which provide references to the diagnosis and treatment of lung cancer. However, due to the pathogenesis underlying lung cancer is complicated, there are almost no corresponding application in clinical diagnosis and treatment. Identifying and characterizing distinctive glycosylation changes in lung cancer by means of proteomic methods is important for its early detection and the target therapy.
Fucosylation, a type of glycosylation, has been studied frequently. It participates in the biosynthesis of blood H antigen and Lewis antigen, leukocyte extravasation mediated by selectin, host-microbe interactions, and modification of signaling (3, 18). Fucosyltransferase mediates the transfer of fucose residues to oligosaccharides and/or proteins. In lung, breast, brain, liver, and thyroid cancer, differential expression of the fucosyltransferase enzyme has been observed (19–23). These aberrant fucosylations of the proteins and glycans are the potential biomarkers of cancers. α-1,6-Fucosyltransferase (core fucosyltransferase, FUT8) is highly expressed in many cancers but is decreased in gastric cancer (24–28). FUT8 is a driver of melanoma metastasis, which when FUT8 silenced, suppresses cell invasion and tumor dissemination were suppressed (28). Therefore, fucosyltransferase is a potential therapeutic target of cancers. In summary, fucosylation plays a pivotal role in proliferation, invasion, metastasis, and immune escape. Currently, many researchers are targeting the abnormality of fucosylation in lung cancer to identify potential biomarkers for diagnosis and treatment. In this article, the function of fucosylation in the progression of lung cancer is reviewed.
Process of Fucosylation
Fucosylation is a typical terminal modification of proteins, and GDP-fucose is the only donor of fucosylation that is synthesized in the cytoplasm. GDP-fucose enters the endoplasmic reticulum or Golgi apparatus through the GDP-L-fucose transporter (GDP L-Fuc Tr) (29, 30). There are two synthesis pathways for the synthesis of GDP-fucose in mammals: de novo synthesis and salvage pathways. In the de novo synthesis pathway, GDP-mannose transforms into GDP-fucose, which is catalyzed by GDP-mannose-4,6-dehydratase (GMDS) and GDP-4-keto-6-deoxymannose-3,5-epimerase-4-reductase (Fx) (31, 32). In the salvage pathway, L-fucose transforms into GDP-fucose, which is catalyzed by L-fucose kinase and GDP-fucose pyrophosphorylase (33, 34) (Figure 1). Fucosylation is categorized according to the location of fucose. There are two kinds of N-fucosylation: core fucosylation (α-1,6-fucosylation) and terminal fucosylation (α-1,2-fucosylation, α-1,3/4-fucosylation). The participation of fucose is necessary for the formation of Lewis antigen. Lewis oligosaccharides have several structure types, such as Lewis a(Lea): Galβ1-3(Fucα-1,4) GlcNAc-R, sLewis a(sLea): NeuAc2-3Galβ1-3(Fucα-1,4) GlcNAc-R, Lewis b(Leb): Fuc-1,2Galβ1-3(Fucα-1,4) GlcNAc-R, Lewis x(Lex): Galβ-1,4(Fucα-1,3) GlcNAc-R, sLweis x(sLex): NeuAc-2,3Galβ-1,4(Fucα-1,3) GlcNAc-R, Lewis y(Ley): Fuc-1,2Galβ-1,4(Fucα-1,3) GlcNAc-R (35). Fucose residues are always involved in the synthesis of the terminal structure of glycans; thus, the transfer of the fucose residue to the glycan marks the end of glycan synthesis.
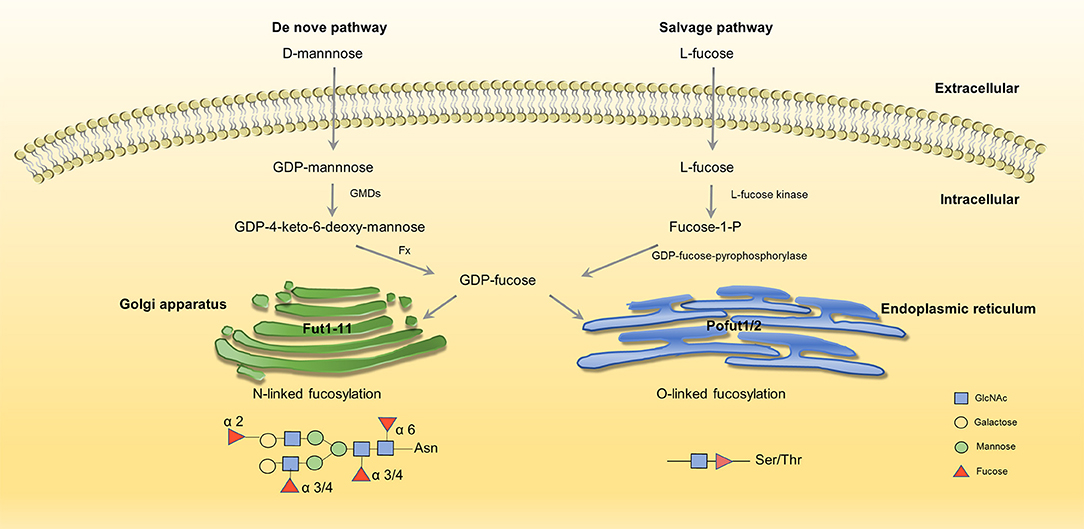
Figure 1. Two synthesis pathways of fucosylation. GDP-fucose is either synthesized from GDP-mannose (de novo pathway, left) or L-fucose (salvage pathway, right) before being transported into Golgi/ER through transporters (35, 36). No permissions were required to use the image.
GDP-fucose serves as an essential substrate, and its synthesis is driven by GMDS and Fx. As a critical enzyme in fucosylation, the expression of GMDS is up-regulated in ADC at both mRNA and protein levels. In ADC, cell proliferation and survival are impaired when knockdown GMDS (37). Moreover, studies show that the abnormal expression of Fx is related to colitis, adenocarcinoma, colorectal cancer, and liver cancer (6, 38, 39)
Fucosyltransferases (FUTs) are enzymes that catalyze fucosylation. There are 13 types of FUTs. FUT1-11 exist in the Golgi apparatus and catalyze N-linked fucosylation, and O-fucosyltransferases (Pofuts) exist in the endoplasmic reticulum and catalyze O-linked fucosylation (35, 36, 40). FUT1 and FUT2 participate in the synthesis of α-1,2-fucosylation, FUT3,4,5,6,7,9 participate in α-1,3/4-fucosylation, FUT8 participates in α-1,6-fucosylation (41). Aberrant expression of FUTs occur in different kinds of cancer. FUT8 promotes breast cancer cell invasiveness by remodeling TGF-β receptor core fucosylation (21) and FUT4 is an effective biomarker for the diagnosis of breast cancer (42). We will explain in detail about the function and aberrant expression of FUTs below.
Aberrant Fucosylation in Lung Cancer
Aberrant Core Fucosylation in Lung Cancer
In mammals, core fucosylation is the primary type of N-fucosylation. The formation of α-1,6-fucosylation is in charge of Fut8, which is the only enzyme responsible for core fucosylation (43). Studies have confirmed that the activity and expression levels of Fut8 are significantly up-regulated in many malignant diseases, which reveal the involvement of Fut8 in the pathological process of these diseases (19, 44). Many studies have concentrated on the aberrant expression of core fucosylation in the sera and tissues of patients with lung cancer. Moreover, identifying the core fucosylated glycoproteins would contribute to confirming biomarkers of lung cancer. Lectin capture is the approach to acquire the core fucosylation glycoproteins, such as aleuria aurantia lectin (AAL), lens culinaris agglutinin (LCA), and pisum sativum agglutinin (PSA), which can recognize the core fucosylation structure specifically.
Aberrant Fucosyltransferase 8
Fut8 transfers a fucose moiety from GDP-β-L-fucose to the innermost GlcNAc residue in an N-glycan, and the resulting α1,6-fucose residue is designated as the core fucose (45). It has been implicated in several physiological and pathological processes. The function of the glycoproteins change due to altered fucosylation as a result of up-regulation of the α(1, 6)fucosyltransferase activity (21). Fut8 expression increased in the tissue of patients with stage I NSCLC, suggesting that Fut8 could be a prognostic factor. Moreover, silencing Fut8 could inhibit tumor growth and metastasis without affecting the proliferation of normal lung epithelial cells (19), indicating the association of high Fut8 expression with deterioration and poor survival. In NSCLC patients, Fut8 could be regarded as an unfavorable prognostic factor of potentially curatively resection, but it can be a potential therapeutic target for NSCLC (46) (Table 1).
Aberrant Fucosylated Glycans and Proteins
E-cadherin was core fucosylated in highly metastatic lung cancer cells which is absent in lowly metastatic lung cancer cells (59). Ruhaak et al. used chip-based nano HPLC coupled with time-of flight mass spectrometry (nLC-chip-TOF-MS) to identify different glycan structures in ADC tissue. They described a detailed analysis of the differential N-glycosylation profile of the early stage lung adenocarcinoma tissue. The level of core fucosylated glycans increased in ADC compared with nonmalignant tissue (51), which may due to the increased expression of FUT8. Narayanasamy et al. found a type of upregulated protein complement component 9 (C9) in the sera of patients with SQCC using label-free liquid chromatography-electrospray ionization-tandem mass spectrometry (LC-ESI-MS/MS) analysis. The C9 protein level was 6.4-fold higher in SQCC patients compared to health control and C9 fucosylation levels were significantly higher (p < 0.05) in patients with SQCC (54), indicating that C9 and its core fucosylated form could serve as a useful marker for SQCC. Haptoglobin (HP) is a protein that is normally present in the blood that imparts antioxidant activity, promoting angiogenesis and other immune functions. HP is closely related to tumors, and the core fucosylation alteration of HP is also related to many diseases (60, 61). The level of HP fucosylation level was 2.55-fold higher in NSCLC patients compared to healthy controls, but no difference was detected in SCLC patients (55). Ahn et al. enriched the core fucosylated glycoproteins by AAL from patient sera. They identified four types of fucosylated proteins. Among them, the serum levels of paraoxonase 1 (PON1) were significantly reduced (< 0.77-fold) in patients with SCLC; nevertheless, the expression level of core fucose on PON1 was significantly increased (53). Studies have demonstrated that PON1 is related to many malignant diseases (62, 63). The core fucosylation level of PON1 and the specific glycan characteristics may serve as diagnostic and prognostic serological markers for SCLC.
Ahn et al. analyzed the core fucosylated proteins alpha-1-acid glycoprotein (AGP) and ceruloplasmin (CP) from ADC patients and healthy individuals using multiple reaction monitoring-mass spectrometry (MRM-MS). The core fucosylation level of AGP and CP were significantly higher in ADC patients compared to controls, although the levels of total plasma protein were comparable between the ADC and control groups (57). It is suggested that the aberrantly fucosylated target glycoproteins AGP and CP could be quantitatively identified as potential biomarkers of ADC. These results indicated that the level of core fucosylation, FUT8, and core-fucosylated products may provide new insights into the therapeutic targets of NSCLC (Table 1).
Aberrant Terminal Fucosylation in Lung Cancer
FUT1-7 and FUT9-11 are responsible for the terminal fucosylation synthesis process. They are involved in the synthesis of Lewis antigen. α-1,2-Fucosylation is catalyzed by FUT1 and FUT2, α-1,3-fucosylation is catalyzed by FUT3–7 and FUT9, and α-1,4-fucosylation is catalyzed by FUT3 (64). The expression levels of sialyl Lewis x (sLex), Lewis y (Ley), and sialyl Lewis a (sLea) have significant variations in ADC, SQCC, and LC. In ADC, the expression levels of these Lewis antigens are the highest. Higher expression levels of these Lewis antigens decrease the survivability of the patients (65). Researches about terminal fucosylation in lung cancer mostly focus on α-1,2-fucosylation and α-1,3-fucosylation.
Aberrant Fucosyltransferase 1–7, 9–11
Studies have reported that miR-339-5p functions as a tumor suppressor in NSCLC (66). Because miR-339-5p can target FUT1 and regulate the downstream protein Ley, this could promote colony formation and attenuate apoptosis of lung carcinoma cell lines (67). The expression of FUT2 is higher in ADC than in the adjacent non-cancerous tissues. Knockdown of FUT2 in A549 and H1299 cells decreased the abilities of cell proliferation, migration, and invasion, but cell apoptosis increased compared with corresponding control cells. Moreover, FUT2 could enhance the cell migration and invasion of ADC cell lines and might promote ADC metastasis through the EMT initiated by TGF-β/Smad signaling. The results indicated that FUT2 is associated with ADC development and it would be a potential biomarker and therapeutic target of ADC (47, 48).
Many researchers have examined the role of α-1,3-fucosyltransferase (FUT3) in the synthesis of sLex and prognosis of its physiological function in lung cancer. The accumulation of tumor-associated antigen sLex was mostly studied in the sera of patients with lung cancer (68). sLex can serve as a ligand for the E- or P-selectin expressed on the cell surface, and its aberrant expression of sLex leads to cancer metastasis and angiogenesis. FUT3 activity was significantly elevated in the sera of patients with lung cancer compared to patients with benign diseases and healthy controls (49, 50). Ogawa et al. found that the sLex expression level was consistent with FUT4 and FUT7 expression. The survival period of the patients whose tumors showed a strong expression of FUT4 or FUT7 was significantly shorter than that of the patients whose tumors did not express either FUT4 or FUT7 (49). Overexpression of FUT7 promotes tumorigenesis in NSCLC by increasing the synthesis of sLex. It activates the EGFR/AKT/mTOR signaling pathway which trigger cell proliferation (69). This may be the reason why patients who have a strong expression of FUT7 exhibit a shorter survival period. (Table 1).
Aberrant Fucosylated Glycans and Proteins
The tyrosine phosphorylation of epidermal growth factor receptor (EGFR) was inhibited upon Ley was downregulated, which leads to the reduction of cell proliferation and tumor growth (52). Tsai et al. provided evidence of an increase in HP terminal fucosylation in NSCLC and SCLC through MS (70). Vasseur et al. found that levels of fucosylated tetra-antennary structures with varying degrees of sialylation are increased in the lung cancer patients who were former smokers. And the level of outer-arm fucosylation was elevated in the lung cancer samples provided by the former smokers (71).
Monosialotetrahexosylganglioside (GM1) is a type of ganglioside. Fucosyl GM1 has a unique structure of α-1,2-fucosylated galactose, which is expressed in both SCLC cell lines and tumor tissues (58). Fucosyl-GM1 has been considered a potential biomarker for SCLC. Tokuda et al. found increased expression of FUT1 and FUT2 in fucosyl GM1-positive SCLC cell lines, and FUT1 seemed to play a leading role in the fucosylation of GM1 in SCLC (56). The study of FUT1 and FUT2 provides novel insights into the role of fucosyl GM1 in novel therapies for SCLC (Table 1).
Fucosylation and Proliferation, Invasion, and Metastasis
The proliferation, invasion, and metastasis of tumor cells are complex, and the cascade reactions mediated by alterations in the fucosylation patterns of the cell surface proteins (72–76). EMT is an important initiation reaction of the dissemination and metastasis of cancer cells (77). The process of EMT is associated with the alteration in some molecular markers, such as E-cadherin, plakoglobin, and cytokeratins. E-cadherin is a type of epithelial markers that shows lower expression in EMT, leading to impairment of cell-cell adhesion, which allows detachment of cells (78). Tumor cells acquire the ability to invade and migrate when E-cadherin is suppressed, which could lead to tumor progression and metastasis (79, 80). The glycosylation of E-cadherin plays a critical role in the regulation of tumor metastasis (81). The fucosylation level of E-cadherin and its effect on lung cancer were studied.
Wen et al. showed that in ADC progression, the core fucosylation of E-cadherin is increased (82). Geng et al. observed that core fucosylated E-cadherin was prominently expressed in highly metastatic lung cancer cells, while there was almost no expression in cells with low levels of metastasis (59). They observed that when E-cadherin was combined with reduction of core fucosylation, cell–cell adhesion was strengthened. However, cell–cell adhesion weakened when the elevated core fucosylated on E-cadherin. Hu et al. then found that core fucosylated E-cadherin is related to the accumulation of nuclear β-catenin in lung cancer cells. If E-cadherin was core-fucosylated, the nuclear β-catenin was reduced; the opposite occurs upon reduced core fucosylation on E-cadherin (83). Shao et al. explained the mechanism of the results above. They revealed the regulation mechanism of core fucosylated E-cadherin in lung cancer cells. They observed a decreased expression of E-cadherin and its core fucosylation using immunohistochemistry (IHC) in lung tumor tissues. Since the core fucosylated E-cadherin regulated the activity of Src and β-catenin, enhanced levels of phosphorylated β-catenin and Src were observed upon lower core fucosylation on E-cadherin. The Src kinase then induces AKT phosphorylation and the activated AKT in turn phosphorylated GSK-3β. GSK-3β is considered to form a β-catenin-degradation complex, which bind to β-catenin, axin, and APC (adenomatous polyposis coli) (84). The activity of GSK-3β was inhibited after phosphorylation, and the inactivated GSK-3β was then unable to phosphorylate β-catenin. Ubiquitylation and degradation of the β-catenin-degradation complex is inhibited. A high expression of phosphorylated GSK-3β then led to increasing nuclear β-catenin accumulation. This is the reason why the accumulated nuclear β-catenin was observed to increase if the core fucosylation of E-cadherin reduced (85). Core fucosylation could not only affects the dimerization of E-cadherin, but also promotes intracellular combination with catenins; core fucosylation on E-cadherin could then regulate the molecular function and its downstream signaling (83). During the process of EMT in lung cancer cells, the downregulation of E-cadherin leads to an increased accumulation of nuclear β-catenin. The nuclear β-catenin could then specifically bind to lymphoid enhancer factor-1 (LEF-1) transcription factors to activate FUT8 transcription. The increase in core fucose synthesis, for example in EGFR, occurs due to FUT8 upregulation. Increasing sialylation and α-1,3-linked fucosylation suppresses EGFR dimerization and phosphorylation, whereas increasing α-1,6-linked fucosylation promotes dimerization and phosphorylation of EGFR (86). Dimerized and phosphorylated EGFR then promotes its downstream signaling pathway, which promotes tumor cell proliferation, invasion, and metastasis by activating the expression of genes involved in cell adhesion, motility, growth, and angiogenesis (19) (Figure 2). In addition, terminal fucosylation plays a critical role in lung cancer cell proliferation, invasion, and metastasis. Liang et al. studied the molecular and biological mechanisms of FUT7 elevation in lung cancer (69). Farhan et al. showed that EGFR signaling was inhibited by anti-Lewisy antibodies in A431 cells, which could lead to the inhibition of cell proliferation (87).
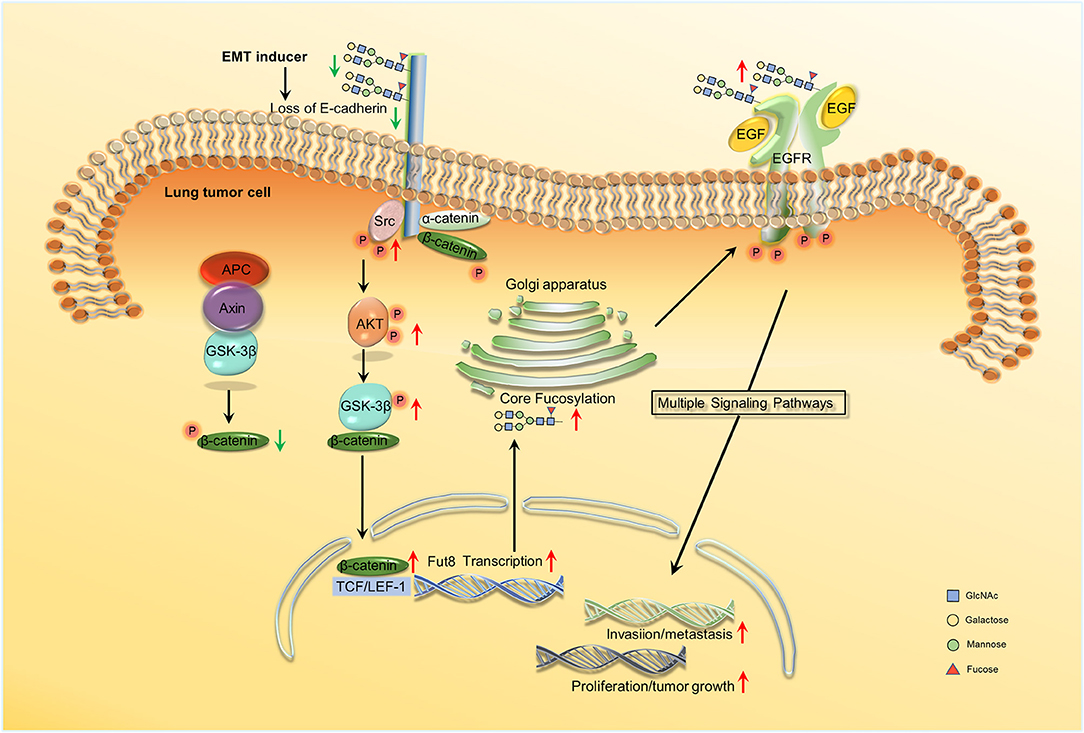
Figure 2. Schema of core fucosylation regulation of lung cancer progression. A decreased level of core fucosylation of E-cadherin activates Src, which leads to the accumulation of nuclear β-catenin (83, 85). β-catenin can further combine with LEF1 and promote the transcription of Fut8. Besides, the upregulation of core fucosylation will result in the dimerization and phosphorylation of EGFR and ultimately activate the downstream signaling and the cascade reactions (86, 19). No permissions were required to use the image.
Discussion
The aberrant fucosylation of tumor is always involved in the activation of EGFR or TGF-βR, and affect the function of integrins, selectins, and apoptotic signaling pathways; ultimately influencing tumor proliferation, invasion, apoptosis, and metastasis. The fucosylation is different in different tumors, different pathological types, and different developmental stages of a tumor. Abnormal expression of fucosylated glycans and proteins were observed in the sera or tissues of patients with lung cancer. In summary, we found that FUTs play an important role in the regulation of fucosylation. Thus, the aberrant expression of FUTs can be considered as clinical biomarkers and therapy target improve the survival rate. FUT8 has received considerable attention because of its absolute specificity. That is why studies about aberrant terminal fucosylated proteins in lung cancer are less frequent, and the terminal structures are characterized by complexity and diversity. Although less is known about the fucosylation in SCLC, it is characterized by more higher malignancy, more often remote metastasis and poor prognosis. The current information might provide us research direction for SCLC. It is significant to pay more attention to SCLC and glycosylation (Table 1).
In the past decades, numerous alterations in fucosylation have been considered as potential biomarkers of lung cancer. The deregulation of fucosylation in cancer and its contribution to tumor–microenvironment interactions remain poorly understood. Moreover, studies have mainly focus on N-fucosylation with little concern about O-fucosylation. Currently, most studies have concentrated on the relationship between POFUT and the Notch pathway (88, 89). The deletion of the O-fucosylation-related Pofut1 gene leads to the failure of Notch binding to the Notch ligands, and thus, blocks signal transmission (90). As the Notch signal is responsible for cell proliferation and apoptosis, POFUT1 may participate in cancer progression.
In contrast, fucosidase, a hydrolase of fucose plays an important role in some malignant diseases. α-L-fucosidase 1 (FUCA1) is a lysosomal enzyme, which reverses the role of FUTs. α-L-fucosidase 1 is responsible for catalyzing the hydrolysis of the terminal fucose residue and plays a crucial role in the breakdown of fucose-containing glycoproteins and glycolipids (91). Cheng et al. reported that the expression of FUCA1 mRNA was increased in breast cancer samples when compared with normal tissue samples. In addition, FUCA1 levels are shown to be higher in advanced-stage tumors. However, the levels of fucosylated Lewis-x antigen are shown to be lower in the advanced-stage patients. Cheng et al. proposed that FUCA1 may be used as a potential prognostic molecular target for breast cancer in clinical investigations (92). In contrast, other researchers have confirmed that FUCA1 pretreatment significantly reduces the invasive capacity of MDA-MB-231 breast cancer cells (93). Vecchio et al. reported that the decreased expression of FUCA1 is related to the augmentation of thyroid cancer, which indicated that FUCA1 levels could be considered reliable evidence for medical diagnoses for aggressive thyroid cancer (94). There is little knowledge on the relationship between FUCA1 and lung cancer. Therefore, we hypothesized that the upregulation of FUCA1 promotes fucose-containing molecules to downregulate their expression on the surface of cancer cells, which can significantly inhibit tumor cell invasion.
Besides fucosylation, other glycosylation types are also closely connected with lung cancer. O-GlcNAcylation and O-GlcNAc transferase (OGT) expression was significantly elevated in lung cancer tissues. O-GlcNAcylation could also enhance lung cancer invasion (95). That the expression of sialyltransferase was up-regulated in lung cancer cell and inhibited the phosphorylation and the dimerization of EGFR (86). Mucins are high molecular weight, heavily glycosylated proteins expressed in the epithelial cells of various organs, are characterized by extensive O-glycosylation. Several reports suggest that mucins are overexpressed in NSCLC and can be used as suitable biomarkers for identifying and monitoring the progression of lung cancer (96, 97).
We found that these aberrant glycosylations lead to disorder of the proliferation, apoptosis, invasion, and metastasis in lung tumor cells, which in common with fucosylation. The difference between these patterns is that the glycosylation modification is different in different proteins, which is involved in the process of lung cancer. These proteins function in tumor cells metabolism. Therefore, there is plenty of scope for further exploration in this field.
Conclusion
This review aimed to improve the overall understanding of the relationship between lung cancer and fucosylation. Lung cancer is a common problem and a major cause of morbidity and mortality. Using molecular biology and glycomics approaches, we will have a better understanding in the pathophysiological features of lung cancer. They provide us new ideas about the development of novel biomarkers for early diagnosis and targeted therapy. We hope that increased awareness of up-to-date evidence-based knowledge and targeting the abnormality of fucosylation in lung cancer will become a new strategy.
Author Contributions
All authors participated in literature research and data classification. LJ, TM, and YG wrote the manuscript. JZ, YY, and JC reviewed and edited the manuscript before submission.
Conflict of Interest Statement
The authors declare that the research was conducted in the absence of any commercial or financial relationships that could be construed as a potential conflict of interest.
Acknowledgments
The Project Supported by Natural Science Basic Research Plan in Shaanxi Province of China (Program No.2017JQ3021).
Abbreviations
NSCLC, non-small cell carcinoma; SCLC, small cell carcinoma; ADC, adenocarcinomas, SQCC, squamous cell carcinomas; LC, large cell carcinomas; PTM, post translationally modified; FUCA-1, α-L-fucosidase-1; PTC, papillary thyroid carcinomas; ATC, anaplastic thyroid carcinomas; EMT, epithelial–mesenchymal transition; sLex, sialyl Lewis x; Ley, Lewis y; sLea, sialyl Lewis a; EGFR, epidermal growth factor receptor; FUCA1, α-L-fucosidase 1.
References
1. American Cancer Society. Cancer Facts and Figures 2018. New York, NY: American Cancer Society (2018).
2. Bray F, Ferlay J, Soerjomataram I, Siegel RL, Torre LA, Jemal A. Global cancer statistics 2018: GLOBOCAN estimates of incidence and mortality worldwide for 36 cancers in 185 countries. CA Cancer J Clin. (2018). 68:394–424. doi: 10.3322/caac.21492
3. Carrascal MA, Silva M, Ramalho JS, Pen C, Martins M, Pascoal C, et al. Inhibition of fucosylation in human invasive ductal carcinoma reduces E-selectin ligand expression, cell proliferation, and ERK1/2 and p38 MAPK activation. Mol Oncol. (2018) 12:579–93. doi: 10.1002/1878-0261.12163
4. Lantuejoul S, Rouquette I, Brambilla E, Travis WD. New WHO classification of lung adenocarcinoma and preneoplasia. Ann Pathol. (2016) 36:5–14. doi: 10.1016/j.annpat.2015.11.010
5. Micke P, Mattsson JS, Djureinovic D, Nodin B, Jirstrom K, Tran L, et al. The impact of the fourth edition of the WHO classification of lung tumours on histological classification of resected pulmonary NSCCs. J Thorac Oncol. (2016) 11:862–72. doi: 10.1016/j.jtho.2016.01.020
6. World Health Organization. Global Health Observatory. Geneva: World Health Organization. (2018). Available online at: who.int/gho/database/en/ (Accessed June 21, 2018).
7. Vajaria B, Patel P. Glycosylation: a hallmark of cancer? Glycoconj J. (2017) 34:147–56. doi: 10.1007/s10719-016-9755-2
8. Cummings RD, Pierce JM. The challenge and promise of glycomics. Chem Biol. (2014) 21:1–15. doi: 10.1016/j.chembiol.2013.12.010
9. Li N, Xu H, Fan K, Liu X, Qi J, Zhao C, et al. Altered beta1,6-GlcNAc branched N-glycans impair TGF-beta-mediated epithelial-to-mesenchymal transition through Smad signalling pathway in human lung cancer. J Cell Mol Med. (2014) 18:1975–91. doi: 10.1111/jcmm.12331
10. Tan Z, Lu W, Li X, Yang G, Guo J, Yu H, et al. Altered N-Glycan expression profile in epithelial-to-mesenchymal transition of NMuMG cells revealed by an integrated strategy using mass spectrometry and glycogene and lectin microarray analysis. J Proteome Res. (2014) 13:2783–95. doi: 10.1021/pr401185z
11. Oliveira-Ferrer L, Legler K, Milde-Langosch K. Role of protein glycosylation in cancer metastasis. Semin Cancer Biol. (2017) 44:141–52. doi: 10.1016/j.semcancer.2017.03.002
12. Qin Y, Zhong Y, Zhu M, Dang L, Yu H, Chen Z, et al. Age- and sex-associated differences in the glycopatterns of human salivary glycoproteins and their roles against influenza A virus. J Proteome Res. (2013) 12:2742–54. doi: 10.1021/pr400096w
13. Sun S, Zhao F, Wang Q, Zhong Y, Cai T, Wu P, et al. Analysis of age and gender associated N-glycoproteome in human whole saliva. Clin Proteomics (2014) 11:25. doi: 10.1186/1559-0275-11-25
14. Zhong Y, Qin Y, Yu H, Yu J, Wu H, Chen L, et al. Avian influenza virus infection risk in humans with chronic diseases. Sci Rep. (2015) 5:8971. doi: 10.1038/srep08971
15. Ryzhov IM, Korchagina EY, Popova IS, Tyrtysh TV, Paramonov AS, Bovin NV. Block synthesis of A (type 2) and B (type 2) tetrasaccharides related to the human ABO blood group system. Carbohydr Res. (2016) 430:59–71. doi: 10.1016/j.carres.2016.04.029
16. Bei R, Mizejewski GJ. Alpha fetoprotein is more than a hepatocellular cancer biomarker: from spontaneous immune response in cancer patients to the development of an AFP-based cancer vaccine. Curr Mol Med. (2011) 11:564–81. doi: 10.2174/156652411800615162
17. Tang S, Zhou F, Sun Y, Wei L, Zhu S, Yang R, et al. CEA in breast ductal secretions as a promising biomarker for the diagnosis of breast cancer: a systematic review and meta-analysis. Breast Cancer (2016) 23:813–9. doi: 10.1007/s12282-016-0680-9
18. Miyoshi E, Moriwaki K, Terao N, Tan CC, Terao M, Nakagawa T, et al. Fucosylation is a promising target for cancer diagnosis and therapy. Biomolecules (2012) 2:34–45. doi: 10.3390/biom2010034
19. Chen CY, Jan YH, Juan YH, Yang CJ, Huang MS, Yu CJ, et al. Fucosyltransferase 8 as a functional regulator of nonsmall cell lung cancer. Proc Natl Acad Sci USA. (2013) 110:630–5. doi: 10.1073/pnas.1220425110
20. Cao Q, Zhao Q, Qian X, Ying W. Identification of core-fucosylated glycoproteome in human plasma. Methods Mol Biol. (2017) 1619:127–37. doi: 10.1007/978-1-4939-7057-5_10
21. Tu CF, Wu MY, Lin YC, Kannagi R, Yang RB. FUT8 promotes breast cancer cell invasiveness by remodeling TGF-beta receptor core fucosylation. Breast Cancer Res. (2017) 19:111. doi: 10.1186/s13058-017-0904-8
22. Veillon L, Fakih C, Abou-El-Hassan H, Kobeissy F, Mechref Y. Glycosylation changes in brain cancer. ACS Chem Neurosci. (2018) 9:51–72. doi: 10.1021/acschemneuro.7b00271
23. Zabczynska M, Kozlowska K, Pochec E. Glycosylation in the thyroid gland: vital aspects of glycoprotein function in thyrocyte physiology and thyroid disorders. Int J Mol Sci. (2018) 19:E2792. doi: 10.3390/ijms19092792
24. Arnold JN, Saldova R, Hamid UM, Rudd PM. Evaluation of the serum N-linked glycome for the diagnosis of cancer and chronic inflammation. Proteomics (2008) 8:3284–93. doi: 10.1002/pmic.200800163
25. An HJ, Kronewitter SR, De Leoz ML, Lebrilla CB. Glycomics and disease markers. Curr Opin Chem Biol. (2009) 13:601–7. doi: 10.1016/j.cbpa.2009.08.015
26. Christiansen MN, Chik J, Lee L, Anugraham M, Abrahams JL, Packer NH. Cell surface protein glycosylation in cancer. Proteomics (2014) 14:525–46. doi: 10.1002/pmic.201300387
27. Zhao YP, Xu XY, Fang M, Wang H, You Q, Yi CH, et al. Decreased core-fucosylation contributes to malignancy in gastric cancer. PLoS ONE (2014) 9:e94536. doi: 10.1371/journal.pone.0094536
28. Magalhaes A, Duarte HO, Reis CA. Aberrant glycosylation in cancer: a novel molecular mechanism controlling metastasis. Cancer Cell (2017) 31:733–5. doi: 10.1016/j.ccell.2017.05.012
29. Lubke T, Marquardt T, Etzioni A, Hartmann E, Von Figura K. Complementation cloning identifies CDG-IIc, a new type of congenital disorders of glycosylation, as a GDP-fucose transporter deficiency. Nat Genet. (2001) 28:73–6. doi: 10.1038/ng0501-73
30. Luhn K, Wild MK, Eckhardt M, Gerardy-Schahn R. The gene defective in leukocyte adhesion deficiency II encodes a putative GDP-fucose transporter. Nat Genet. (2001) 28:69–72. doi: 10.1038/ng0501-69
31. Sullivan FX, Kumar R, Kriz R, Stahl M, Xu GY, Rouse J, et al. Molecular cloning of human GDP-mannose 4,6-dehydratase and reconstitution of GDP-fucose biosynthesis in vitro. J Biol Chem. (1998) 273:8193–202. doi: 10.1074/jbc.273.14.8193
32. Smith PL, Myers JT, Rogers CE, Zhou L, Petryniak B, Becker DJ, et al. Conditional control of selectin ligand expression and global fucosylation events in mice with a targeted mutation at the FX locus. J Cell Biol. (2002) 158:801–15. doi: 10.1083/jcb.200203125
33. Park SH, Pastuszak I, Drake R, Elbein AD. Purification to apparent homogeneity and properties of pig kidney L-fucose kinase. J Biol Chem. (1998) 273:5685–91. doi: 10.1074/jbc.273.10.5685
34. Pastuszak I, Ketchum C, Hermanson G, Sjoberg EJ, Drake R, Elbein AD. GDP-L-fucose pyrophosphorylase. Purification, cDNA cloning, and properties of the enzyme. J Biol Chem. (1998) 273:30165–74. doi: 10.1074/jbc.273.46.30165
35. Becker DJ, Lowe JB. Fucose: biosynthesis and biological function in mammals. Glycobiology (2003) 13:41R−53R. doi: 10.1093/glycob/cwg054
36. Ma B, Simala-Grant JL, Taylor DE. Fucosylation in prokaryotes and eukaryotes. Glycobiology (2006) 16:158r−84r. doi: 10.1093/glycob/cwl040
37. Wei X, Zhang K, Qin H, Zhu J, Qin Q, Yu Y, et al. GMDS knockdown impairs cell proliferation and survival in human lung adenocarcinoma. BMC Cancer (2018) 18:600. doi: 10.1186/s12885-018-4524-1
38. Noda K, Miyoshi E, Gu J, Gao CX, Nakahara S, Kitada T, et al. Relationship between elevated FX expression and increased production of GDP-L-fucose, a common donor substrate for fucosylation in human hepatocellular carcinoma and hepatoma cell lines. Cancer Res. (2003) 63:6282–9. Available online at: http://cancerres.aacrjournals.org/content/63/19/6282.long
39. Wang Y, Huang D, Chen KY, Cui M, Wang W, Huang X, et al. Fucosylation deficiency in mice leads to colitis and adenocarcinoma. Gastroenterology (2017) 152:193-205.e110. doi: 10.1053/j.gastro.2016.09.004
40. Okajima T, Xu A, Lei L, Irvine KD. Chaperone activity of protein O-fucosyltransferase 1 promotes notch receptor folding. Science (2005) 307:1599–603. doi: 10.1126/science.1108995
41. Miyoshi E, Moriwaki K. Biological function of fucosylation in cancer biology. J Biochem. (2008) 143:725–9. doi: 10.1093/jb/mvn011
42. Yan X, Lin Y, Liu S, Aziz F. Fucosyltransferase IV (FUT4) as an effective biomarker for the diagnosis of breast cancer. Biomed Pharmacother. (2015) 70:299–304. doi: 10.1016/j.biopha.2014.12.048
43. Miyoshi E, Noda K, Yamaguchi Y, Inoue S, Ikeda Y, Wang W, et al. The alpha1-6-fucosyltransferase gene and its biological significance. Biochim Biophys Acta (1999) 1473:9–20. doi: 10.1016/S0304-4165(99)00166-X
44. Comunale MA, Lowman M, Long RE, Krakover J, Philip R, Seeholzer S, et al. Proteomic analysis of serum associated fucosylated glycoproteins in the development of primary hepatocellular carcinoma. J Proteome Res. (2006) 5:308–15. doi: 10.1021/pr050328x
45. Taniguchi N, Kizuka Y. Glycans and cancer: role of N-glycans in cancer biomarker, progression and metastasis, and therapeutics. Adv Cancer Res. (2015) 126:11–51. doi: 10.1016/bs.acr.2014.11.001
46. Honma R, Kinoshita I, Miyoshi E, Tomaru U, Matsuno Y, Shimizu Y, et al. Expression of fucosyltransferase 8 is associated with an unfavorable clinical outcome in non-small cell lung cancers. Oncology (2015) 88:298–308. doi: 10.1159/000369495
47. Zhou W, Ma H, Deng G, Tang L, Lu J, Chen X. Clinical significance and biological function of fucosyltransferase 2 in lung adenocarcinoma. Oncotarget (2017) 8:97246–59. doi: 10.18632/oncotarget.21896
48. Deng G, Chen L, Zhang Y, Fan S, Li W, Lu J, et al. Fucosyltransferase 2 induced epithelial-mesenchymal transition via TGF-β/Smad signaling pathway in lung adenocarcinaoma. Exp Cell Res. (2018) 370:613–22. doi: 10.1016/j.yexcr.2018.07.026
49. Ogawa J, Inoue H, Koide S. Expression of alpha-1,3-fucosyltransferase type IV and VII genes is related to poor prognosis in lung cancer. Cancer Res. (1996) 56:325–9.
50. Asao T, Yazawa S, Nagamachi Y, Abbas SA, Matta KL. Serum alpha(1–−3)-L-fucosyltransferase, carcinoembryonic antigen, and sialyl Lewis X-i antigen levels in lung cancer. Cancer (1989) 64:2541–5. doi: 10.1002/1097-0142(19891215)64:12<2541::AID-CNCR2820641222>3.0.CO;2-7
51. Ruhaak LR, Taylor SL, Stroble C, Nguyen UT, Parker EA, Song T, et al. Differential N-glycosylation patterns in lung adenocarcinoma tissue. J Proteome Res. (2015) 14:4538–49. doi: 10.1021/acs.jproteome.5b00255
52. Zhang Z, Sun P, Liu J, Fu L, Yan J, Liu Y, et al. Suppression of FUT1/FUT4 expression by siRNA inhibits tumor growth. Biochim Biophys Acta (2008) 1783:287–96. doi: 10.1016/j.bbamcr.2007.10.007
53. Ahn JM, Sung HJ, Yoon YH, Kim BG, Yang WS, Lee C, et al. Integrated glycoproteomics demonstrates fucosylated serum paraoxonase 1 alterations in small cell lung cancer. Mol Cell Proteomics (2014) 13:30–48. doi: 10.1074/mcp.M113.028621
54. Narayanasamy A, Ahn JM, Sung HJ, Kong DH, Ha KS, Lee SY, et al. Fucosylated glycoproteomic approach to identify a complement component 9 associated with squamous cell lung cancer (SQLC). J Proteomics (2011) 74:2948–58. doi: 10.1016/j.jprot.2011.07.019
55. Ferens-Sieczkowska M, Kratz EM, Kossowska B, Passowicz-Muszynska E, Jankowska R. Comparison of haptoglobin and alpha(1)-acid glycoprotein glycosylation in the sera of small cell and non-small cell lung cancer patients. Postepy Higieny I Medycyny Doswiadczalnej (2013) 67:828–36. doi: 10.5604/17322693.1061788
56. Tokuda N, Zhang Q, Yoshida S, Kusunoki S, Urano T, Furukawa K, et al. Genetic mechanisms for the synthesis of fucosyl GM1 in small cell lung cancer cell lines. Glycobiology (2006) 16:916–25. doi: 10.1093/glycob/cwl022
57. Ahn YH, Ji ES, Oh NR, Kim YS, Ko JH, Yoo JS. Differential proteomic approach for identification and verification of aberrantly glycosylated proteins in adenocarcinoma lung cancer (ADLC) plasmas by lectin-capturing and targeted mass spectrometry. J Proteomics (2014) 106:221–9. doi: 10.1016/j.jprot.2014.04.031
58. Zhang S, Cordon-Cardo C, Zhang HS, Reuter VE, Adluri S, Hamilton WB, et al. Selection of tumor antigens as targets for immune attack using immunohistochemistry: I. Focus on gangliosides. Int J Cancer (1997) 73:42–9. doi: 10.1002/(SICI)1097-0215(19970926)73:1<42::AID-IJC8>3.0.CO;2-1
59. Geng F, Shi BZ, Yuan YF, Wu XZ. The expression of core fucosylated E-cadherin in cancer cells and lung cancer patients: prognostic implications. Cell Res. (2004) 14:423–33. doi: 10.1038/sj.cr.7290243
60. Okuyama N, Ide Y, Nakano M, Nakagawa T, Yamanaka K, Moriwaki K, et al. Fucosylated haptoglobin is a novel marker for pancreatic cancer: a detailed analysis of the oligosaccharide structure and a possible mechanism for fucosylation. Int J Cancer. (2006) 118:2803–8. doi: 10.1002/ijc.21728
61. Kim JH, Lee SH, Choi S, Kim U, Yeo IS, Kim SH, et al. Direct analysis of aberrant glycosylation on haptoglobin in patients with gastric cancer. Oncotarget (2017) 8:11094–104. doi: 10.18632/oncotarget.14362
62. Akcay MN, Polat MF, Yilmaz I. Serum paraoxonase levels in pancreatic cancer. Hepatogastroenterology (2003) 50(Suppl. 2):ccxxv–vii. Available online at: http://europepmc.org/abstract/MED/15244186
63. Akcay MN, Yilmaz I, Polat MF. Serum paraoxonase levels in gastric cancer. Hepatogastroenterology (2003) 50(Suppl. 2):cclxxiii–xxv. Available online at: http://europepmc.org/abstract/med/15244199
64. Potapenko IO, Haakensen VD, Luders T, Helland A, Bukholm I, Sorlie T, et al. Glycan gene expression signatures in normal and malignant breast tissue; possible role in diagnosis and progression. Mol Oncol. (2010) 4:98–118. doi: 10.1016/j.molonc.2009.12.001
65. Ogawa J, Sano A, Inoue H. Expression of Lewis-related antigen and prognosis in stage I non-small cell lung cancer. Ann Thorac Surg. (1995) 59:412–5. doi: 10.1016/0003-4975(94)00866-6
66. Li Y, Zhao W, Bao P, Li C, Ma XQ, Li Y, et al. miR-339-5p inhibits cell migration and invasion in vitro and may be associated with the tumor-node-metastasis staging and lymph node metastasis of non-small cell lung cancer. Oncol Lett. (2014) 8:719–25. doi: 10.3892/ol.2014.2165
67. Gan CZ, Li G, Luo QS, Li HM. miR-339-5p downregulation contributes to Taxol resistance in small-cell lung cancer by targeting alpha1,2-fucosyltransferase 1. IUBMB Life (2017) 69:841–9. doi: 10.1002/iub.1679
68. Kannagi R, Fukushi Y, Tachikawa T, Noda A, Shin S, Shigeta K, et al. Quantitative and qualitative characterization of human cancer-associated serum glycoprotein antigens expressing fucosyl or sialyl-fucosyl type 2 chain polylactosamine. Cancer Res. (1986) 46:2619–26.
69. Liang JX, Gao W, Cai L. Fucosyltransferase VII promotes proliferation via the EGFR/AKT/mTOR pathway in A549 cells. Oncotargets Ther. (2017) 10:3971–8. doi: 10.2147/OTT.S140940
70. Tsai HY, Boonyapranai K, Sriyam S, Yu CJ, Wu SW, Khoo KH, et al. Glycoproteomics analysis to identify a glycoform on haptoglobin associated with lung cancer. Proteomics (2011) 11:2162–70. doi: 10.1002/pmic.201000319
71. Vasseur JA, Goetz JA, Alley WR, Novotny MV. Smoking and lung cancer-induced changes in N-glycosylation of blood serum proteins. Glycobiology (2012) 22:1684–708. doi: 10.1093/glycob/cws108
72. Orntoft TF, Vestergaard EM. Clinical aspects of altered glycosylation of glycoproteins in cancer. Electrophoresis (1999) 20:362–71. doi: 10.1002/(SICI)1522-2683(19990201)20:2<362::AID-ELPS362>3.0.CO;2-V
73. Hakomori S. Glycosylation defining cancer malignancy: new wine in an old bottle. Proc Natl Acad Sci USA. (2002) 99:10231–3. doi: 10.1073/pnas.172380699
74. Dube DH, Bertozzi CR. Glycans in cancer and inflammation–potential for therapeutics and diagnostics. Nat Rev Drug Discov. (2005) 4:477–88. doi: 10.1038/nrd1751
75. Brockhausen I. Mucin-type O-glycans in human colon and breast cancer: glycodynamics and functions. EMBO Rep. (2006) 7:599–604. doi: 10.1038/sj.embor.7400705
76. Pinho SS, Reis CA. Glycosylation in cancer: mechanisms and clinical implications. Nat Rev Cancer (2015) 15:540–55. doi: 10.1038/nrc3982
77. Brabletz T. EMT and MET in metastasis: where are the cancer stem cells? Cancer Cell (2012) 22:699–701. doi: 10.1016/j.ccr.2012.11.009
78. Schmalhofer O, Brabletz S. E-cadherin, beta-catenin, and ZEB1 in malignant progression of cancer. Cancer Metastasis Rev. (2009) 28:151–66. doi: 10.1007/s10555-008-9179-y
79. Yang J, Weinberg RA. Epithelial-mesenchymal transition: at the crossroads of development and tumor metastasis. Dev Cell. (2008) 14:818–29. doi: 10.1016/j.devcel.2008.05.009
80. Kedinger V, Sansregret L, Harada R, Vadnais C, Cadieux C, Fathers K, et al. p110 CUX1 homeodomain protein stimulates cell migration and invasion in part through a regulatory cascade culminating in the repression of E-cadherin and occludin. J Biol Chem. (2009) 284:27701–11. doi: 10.1074/jbc.M109.031849
81. Kitada T, Miyoshi E, Noda K, Higashiyama S, Ihara H, Matsuura N, et al. The addition of bisecting N-acetylglucosamine residues to E-cadherin down-regulates the tyrosine phosphorylation of beta-catenin. J Biol Chem. (2001) 276:475–80. doi: 10.1074/jbc.M006689200
82. Wen CL, Chen KY, Chen CT, Chuang JG, Yang PC, Chow LP. Development of an AlphaLISA assay to quantify serum core-fucosylated E-cadherin as a metastatic lung adenocarcinoma biomarker. J Proteomics (2012) 75:3963–76. doi: 10.1016/j.jprot.2012.05.015
83. Hu P, Shi BZ, Geng F, Zhang CY, Wu W, Wu X. E-cadherin core fucosylation regulates nuclear beta-catenin accumulation in lung cancer cells. Glycoconj J. (2008) 25:843–50. doi: 10.1007/s10719-008-9144-6
84. Ikeda S, Kishida S, Yamamoto H, Murai H, Koyama S. Axin, a negative regulator of the Wnt signaling pathway, forms a complex with GSK-3beta and beta-catenin and promotes GSK-3beta-dependent phosphorylation of beta-catenin. EMBO J. (1998) 17:1371–84. doi: 10.1093/emboj/17.5.1371
85. Shao K, Chen ZY, Gautam S, Deng NH, Zhou Y, Wu XZ. Posttranslational modification of E-cadherin by core fucosylation regulates Src activation and induces epithelial-mesenchymal transition-like process in lung cancer cells. Glycobiology (2016) 26:142–54. doi: 10.1093/glycob/cwv089
86. Liu YC, Yen HY, Chen CY, Chen CH, Cheng PF, Juan YH, et al. Sialylation and fucosylation of epidermal growth factor receptor suppress its dimerization and activation in lung cancer cells. Proc Natl Acad Sci USA. (2011) 108:11332–7. doi: 10.1073/pnas.1107385108
87. Farhan H, Schuster C, Klinger M, Weisz E, Waxenecker G, Schuster M, et al. Inhibition of xenograft tumor growth and down-regulation of ErbB receptors by an antibody directed against Lewis Y antigen. J Pharmacol Exp Ther. (2006) 319:1459–66. doi: 10.1124/jpet.106.107318
88. Al-Shareffi E, Chaubard JL, Leonhard-Melief C, Wang SK, Wong CH, Haltiwanger RS. 6-alkynyl fucose is a bioorthogonal analog for O-fucosylation of epidermal growth factor-like repeats and thrombospondin type-1 repeats by protein O-fucosyltransferases 1 and 2. Glycobiology (2013) 23:188–98. doi: 10.1093/glycob/cws140
89. Muller J, Rana NA, Serth K, Kakuda S, Haltiwanger RS. O-fucosylation of the notch ligand mDLL1 by POFUT1 is dispensable for ligand function. PLoS ONE (2014) 9:e88571. doi: 10.1371/journal.pone.0088571
90. Stanley P. Roles of glycosylation in Notch signaling. Curr Top Dev Biol. (2010) 92:131–64. doi: 10.1016/S0070-2153(10)92004-8
91. Panmontha W, Amarinthnukrowh P, Damrongphol P, Desudchit T, Suphapeetiporn K, Shotelersuk V. Novel mutations in the FUCA1 gene that cause fucosidosis. Genet Mol Res. (2016) 15:1–6. doi: 10.4238/gmr.15038733
92. Cheng TC, Tu SH, Chen LC, Chen MY, Chen WY, Lin YK, et al. Down-regulation of alpha-L-fucosidase 1 expression confers inferior survival for triple-negative breast cancer patients by modulating the glycosylation status of the tumor cell surface. Oncotarget (2015) 6:21283–300. doi: 10.18632/oncotarget.4238
93. Yuan K, Listinsky CM, Singh RK, Listinsky JJ, Siegal GP. Cell surface associated alpha-L-fucose moieties modulate human breast cancer neoplastic progression. Pathol Oncol Res. (2008) 14:145–56. doi: 10.1007/s12253-008-9036-x
94. Vecchio G, Parascandolo A, Allocca C, Ugolini C, Basolo F, Moracci M, et al. Human a-L-fucosidase-1 attenuates the invasive properties of thyroid cancer. Oncotarget (2017) 8:27075–92. doi: 10.18632/oncotarget.15635
95. Mi W, Gu Y, Han C, Liu H, Fan Q, Zhang X, et al. O-GlcNAcylation is a novel regulator of lung and colon cancer malignancy. Biochim Biophys Acta (2011) 1812:514–9. doi: 10.1016/j.bbadis.2011.01.009
96. Duruisseaux M, Antoine M, Rabbe N, Poulot V, Fleury-Feith J, Vieira T, et al. The impact of intracytoplasmic mucin in lung adenocarcinoma with pneumonic radiological presentation. Lung Cancer (2014) 83:334–40. doi: 10.1016/j.lungcan.2013.12.016
Keywords: lung cancer, fucosylation, fucosyltransferase, biomarker, epithelial–mesenchymal transition
Citation: Jia L, Zhang J, Ma T, Guo Y, Yu Y and Cui J (2018) The Function of Fucosylation in Progression of Lung Cancer. Front. Oncol. 8:565. doi: 10.3389/fonc.2018.00565
Received: 26 June 2018; Accepted: 12 November 2018;
Published: 07 December 2018.
Edited by:
Taofeek K. Owonikoko, Emory University, United StatesReviewed by:
Rachel E. Sanborn, Providence Portland Medical Center, United StatesYaogang Zhong, The Ohio State University, United States
Ganglong Yang, Johns Hopkins Medicine, United States
Hongqi LI, University of Texas MD Anderson Cancer Center, United States
Copyright © 2018 Jia, Zhang, Ma, Guo, Yu and Cui. This is an open-access article distributed under the terms of the Creative Commons Attribution License (CC BY). The use, distribution or reproduction in other forums is permitted, provided the original author(s) and the copyright owner(s) are credited and that the original publication in this journal is cited, in accordance with accepted academic practice. No use, distribution or reproduction is permitted which does not comply with these terms.
*Correspondence: Jihong Cui, cjh@nwu.edu.cn
†These authors have contributed equally to this work