- Department of Biomedical and Molecular Sciences, Queen's University, Kingston, ON, Canada
The role of the immune system in anti-tumor immunity cannot be overstated, as it holds the potential to promote tumor eradication or prevent tumor cell escape. Cytokines are critical to influencing the immune responses and interactions with non-immune cells. Recently, the IL-12 and IL-6 family of cytokines have accumulated newly defined members each with specific immune functions related to various cancers and tumorigenesis. There is a need to better understand how cytokines like IL-27, IL-30, and IL-35 interact with one another, and how a developing tumor can exploit these interactions to enhance immune suppression. Current cytokine-based immunotherapies are associated with cytotoxic side effects which limits the success of treatment. In addition to this toxicity, understanding the complex interactions between immune and cancer cells may be one of the greatest challenges to developing a successful immunotherapy. In this review, we bring forth IL-27, IL-30, and IL-35, “sister cytokines,” along with more recent additions to the IL-12 family, which serve distinct purposes despite sharing structural similarities. We highlight how these cytokines function in the tumor microenvironment by examining their direct effects on cancer cells as well their indirect actions via regulatory functions of immune cells that act to either instigate or inhibit tumor progression. Understanding the context dependent immunomodulatory outcomes of these sister cytokines, as well as their regulation within the tumor microenvironment, may shed light onto novel cancer therapeutic treatments or targets.
Introduction
Cytokine production in the tumor microenvironment dictates how innate and adaptive immune cells respond to and influence tumor progression. Irregular cytokine production by tumor cells and immune cells within the tumor microenvironment (TME) can be used as a diagnostic tool to screen patients for cancer (1–3). Cytokines are master regulators of inflammatory cell function and can recruit, activate, or dampen immune responses, in addition to regulating cellular proliferation and differentiation (4, 5).
Due to the complex nature of the TME it is important to understand the role cytokines play in influencing tumor and immune cell interactions (1, 5). Two prominent type I cytokine families, IL-6 and IL-12, have been a recent focus in cancer research. The IL-6 family of cytokines includes IL-6, IL-11, IL-27, IL-31, leukemia-inhibitory factor (LIF), cardiotropin-1 (CT-1), oncostatin M (OSM), ciliary neurotrophic factor (CNTF), and cardiotropin-like cytokine (CLC), which share the glycoprotein 130 (gp130) receptor subunit (6). As prominent factors in several essential cellular processes, IL-6 family members are considered pro-tumorigenic (7, 8), with the exception of IL-27, which has a more complex role in potential anti-tumor therapeutic implications (9). Adding to this complexity, the newly identified IL-30 cytokine, which shares a subunit with IL-27, promotes tumor progression (10). IL-27 also belongs to the IL-12 family, which includes IL-12, IL-23, IL-27, IL-35, and IL-39 (Figure 1A). Although IL-35 is considered to belong to the IL-12 family, due to its usage of the gp130 receptor it is also related to members of the IL-6 family (12). The ability of IL-12 to activate anti-tumor cytotoxic T lymphocytes (CTLs) and natural killer (NK) cells, makes it an attractive cytokine for cancer immunotherapy (13). Despite overwhelming evidence toward the anti-tumor effects of IL-12, its use in the clinic remains problematic due to toxicity concerns (14–16). Cytokines closely related to IL-12 are being investigated for their anti-tumor capacity, and of these cytokines IL-27 has become an attractive alternative based on its potent anti-tumor activity (17). Here, we review the potential interplay of the three sister cytokines; IL-27, IL-30, and IL-35, and their role in tumorigenesis.
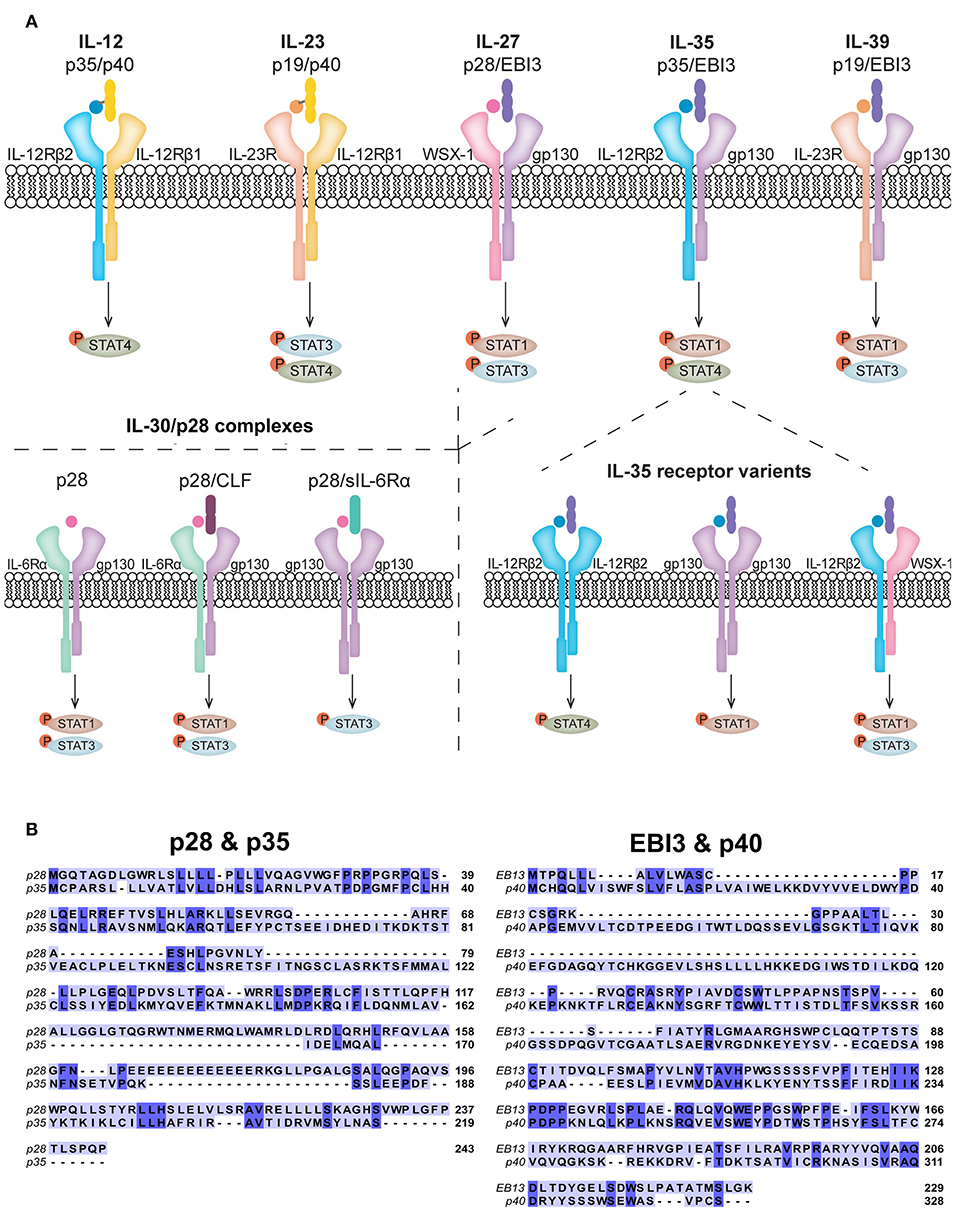
Figure 1. Receptor composition of IL-12 family members and related cytokines. (A) The IL-12 family members are heterodimeric in composition and signal via heterodimeric receptors. IL-27, composed of p28 and EBI3, shares common subunits with IL-35 (p35 and EBI3) and IL-39 (p19 and EBI3). The p28 subunit has also been shown to have biological activity in the absence of EBI3, known as IL-30. In addition to subunit sharing with IL-27 and IL-39, IL-35 shares the p35 subunit with IL-12. p28 is also known to form complexes with CLF and sIL-6Rα. Signaling of all IL-12 family members, IL-30, and other known p28 complexes, predominantly activate the JAK/STAT pathway. Each cytokine preferentially activates specific STATs to achieve its functional role, although other phosphorylated STATs have been observed. (B) Amino acid sequence overlays for human p28 (accession number: NP_663634.2) with p35 (accession number: NP_000873.2) and EBI3 (accession number: NP_005746.2) with p40 (accession number: NP_002178.2) were aligned using the T-COFFEE-espresso program (11). Shared amino acids are indicated by dark blue shading and homology between p28 and p35 was calculated to be 64% (44 of 169) and between EBI3 and p40 was calculated to be 33% (59 of 177).
Overview of IL-27, IL-30, and IL-35 Signaling
IL-27
Discovered in 2002, IL-27 is composed of two non-covalently linked subunits, IL-27p28 (p28) and Epstein-Barr-virus-induced molecule 3 (EBI3) (18) (Figure 1A). These subunits exhibit structural and sequence homology to IL-12 subunits and IL-6 (19). The p28 subunit shares ~64% amino acid homology with the p35 subunit of IL-12, based on their sequence alignment (Figure 1B). p28 also shares ~27% homology with IL-6 (20, 21). EBI3 shares ~33% amino acid homology with IL-12p40 based on their sequence alignment (Figure 1B) and in agreement with Devergne et al. (22) who calculated 27% homology. IL-27 binds a heterodimeric receptor comprised of gp130 and WSX-1 (23). WSX-1 is highly expressed on lymphocytes and at lower levels on myeloid cells (23, 24). Gp130 is ubiquitously expressed, therefore the specificity of IL-27 activity is determined by co-expression of WSX-1 (25). IL-27 binds WSX-1 with low affinity in the absence of gp130 (18, 23), however, both receptor subunits are required for efficient signaling (23). In addition, WSX-1 and gp130 exist in soluble forms (sWSX-1 and sgp130) and binding of sWSX-1 to IL-27 acts as a signaling antagonist (26). Interestingly, sgp130 does not inhibit IL-27 signaling (27), although it does block IL-11 and IL-6 trans-signaling (28). This indicates the possibility that sgp130 exclusively inhibits gp130 homodimer signaling. Upon IL-27 binding to its receptor, phosphorylation of signal transducer and activator of transcription (STAT) 1 and 3 occurs, which is negatively regulated by suppressor of cytokine signaling 3 (SOCS3) (23, 29). The cell type and activation state may influence IL-27 signaling to modify comparative levels of STAT activation (30, 31).
IL-27 is produced by antigen presenting cells (APCs) following Toll-like receptor (TLR) activation (18, 32, 33). Initially, IL-27 was characterized as a positive mediator of Th1 development (18, 34). Alternatively, IL-27 also demonstrates anti-inflammatory and inhibitory properties, particularly with regards to inhibition of Th2, Th17 and Treg differentiation (35, 36). However, more recent studies have contrasted these results, indicating that IL-27 can promote the growth and survival of Treg cells (37, 38).
IL-27 produced in response to TLR activation also modulates myeloid cell function and results in upregulation of TLR expression on these cells (21, 39–42). This indicates that IL-27 may participate in a positive feedback loop, whereby activated cells express IL-27 which then acts to further sensitize cells to TLR responsiveness. IL-27-treated myeloid cells and epithelial cells also exhibit enhanced antigen presentation by upregulation of major histocompatibility complex (MHC) I and II as well as co-stimulatory molecules (43–45). Due to its ability to affect antigen presentation and modulate helper T cell differentiation and activation, IL-27 holds the potential to be a master regulator in the TME.
IL-30
Originally identified as a subunit of IL-27, p28 alone, known as IL-30, can exert biological activities independently from EBI3 (21, 46–49). Sharing homology with IL-6 family members: IL-6, IL-11, and CLC (18), p28 can function as an antagonist of gp130 signaling, blocking IL-6, IL-11, and IL-27 functions (50). Various p28 binding partners have been identified to induce signaling, these include CLF, p40, and sIL-6Rα (51). Recent reports showed that IL-30 signals similarly to IL-6, by interacting with CLF or soluble IL-6Rα and membrane-bound gp130 homodimers, resulting in phosphorylation of STAT1 and STAT3 (21, 51, 52) (Figure 1A). Interestingly, IL-30 at high concentrations can also signal through gp130 homodimers in the absence of soluble IL-6Rα (52). Furthermore, in human monocytes, recombinant IL-30 treatment resulted in delayed STAT1 and STAT3 phosphorylation compared to that induced by IL-27; the delay in IL-30-mediated STAT phosphorylation correlated with levels of sIL-6Rα expression, further supporting a role for IL-6Rα in IL-30 signaling (21).
IL-30 production measured by detection of p28 mRNA or secreted protein levels can be detected in activated macrophages and splenocytes (53). Furthermore, recombinant IL-30 acts on monocytes to induce IFN-γ-inducible protein 10 (IP-10; CXCL10) and TLR4 expression (21). Recent evidence supports a protective role of IL-30 against liver injury and fibrosis (46). It is important to consider which cells specifically express and/or respond to p28 and its potential binding partners which can exert supplementary functions compared to IL-30 (p28 alone). For example, the p28/CLF complex is produced by activated DCs and modulates NK cell and T cell function (51) and enhances proliferation and differentiation of plasma cells (54). The existence of different p28 binding partners implies that IL-30, either as p28 alone or in combination with one or more partners, may result in differential functions depending on cell type and activation state.
IL-35
IL-35 was discovered in 2007 and, similar to other IL-12 family members, is a heterodimeric cytokine comprised of the IL-12p35 (p35) and EBI3 (55, 56). The IL-35 receptor components vary by cell type; in T cells, IL-35 binds gp130 and IL-12Rβ2 to signal through either gp130/IL-12Rβ2 heterodimers or homodimers of each subunit (57, 58). Alternatively, in B cells, IL-35 signals through IL-12Rβ2 and WSX-1 (59). Since IL-35 can signal through gp130 and/or WSX-1, this indicates the potential for competition for receptor usage between IL-27, IL-30 and IL-35. Depending on the receptor chains that are engaged, IL-35 elicits activation of various STATs (Figure 1A). For example, IL-35 binding to IL-12Rβ2:WSX-1 results in STAT1 and STAT3 phosphorylation (59), while binding to IL-12Rβ2:gp130 induces formation of a STAT1:STAT4 heterodimer. IL-35 signaling via IL-12Rβ2 or gp130 homodimers results in STAT4 or STAT1 phosphorylation, respectively (58).
Secreted primarily by Treg cells in response to either IFN-γ or TLR stimulation, IL-35 opposes pro-inflammatory functions of other IL-12 family members (55). Cell types such as B cells, endothelial cells, smooth muscle cells, DCs, and monocytes also produce IL-35, but to lesser extent than Tregs (59–65). IL-35 converts T cells and B cells into regulatory populations that produce IL-35, referred to as inducible Treg-IL-35 (iTr35) and IL-35+ Breg cells (60, 64). IL-35 inhibits differentiation and function of Th1 and Th17 cells by promoting the expansion of Tregs and their production of IL-10 (55, 60). IL-35 is an important cytokine with immunosuppressive functions, which play a role in promoting a pro-tumor phenotype within the TME. Competition between IL-27, IL-30, and IL-35 for receptor subunits may affect the overall balance of pro- vs. anti-tumor effects of these cytokines. The differential roles of each cytokine in cancer is discussed below.
IL-27, IL-30, and IL-35: Influencing Cancer Development
IL-27: The Cytokine That Swings Both Ways
Recently, both anti-tumor and pro-tumor roles have been attributed to IL-27 (Figure 2). This dichotomy may be explained, in part, by the differential roles played by IL-27 in the immune response. The roles for IL-27 in cancer have been studied in both in vitro and in vivo models and it is important to consider how IL-27 is introduced to the model system given that this cytokine is heterodimeric and the subunits are non-covalently associated in nature. Commercially available recombinant IL-27 and IL-27 expression vectors may contain an engineered flexible amino acid linker sequence between EBI3 and p28 subunits, potentially preventing subunit dissociation and thus formation of IL-30 or IL-35 (Figure 3A). While several studies examine both recombinant and transduced IL-27, caution should be considered when interpreting data from studies where the linker in synthetic IL-27 is used because its presence or absence has yet to be directly compared and assessed. By treating cells in vitro with recombinant cytokine, the dose, cell number, and length of exposure to a specific cell type can be defined, where these parameters are more difficult to control in an in vivo model. In vivo studies using cancer cells transduced with an IL-27 expression vector permits continual IL-27 production and ensures that IL-27 is present within the TME; however, the dose and length of exposure becomes more challenging to control in the studied model. When taking into account the use of knockout animals, it is important to acknowledge that deficiency in cytokine or receptor subunits may impact more than one particular cytokine as outlined in Figure 3B.
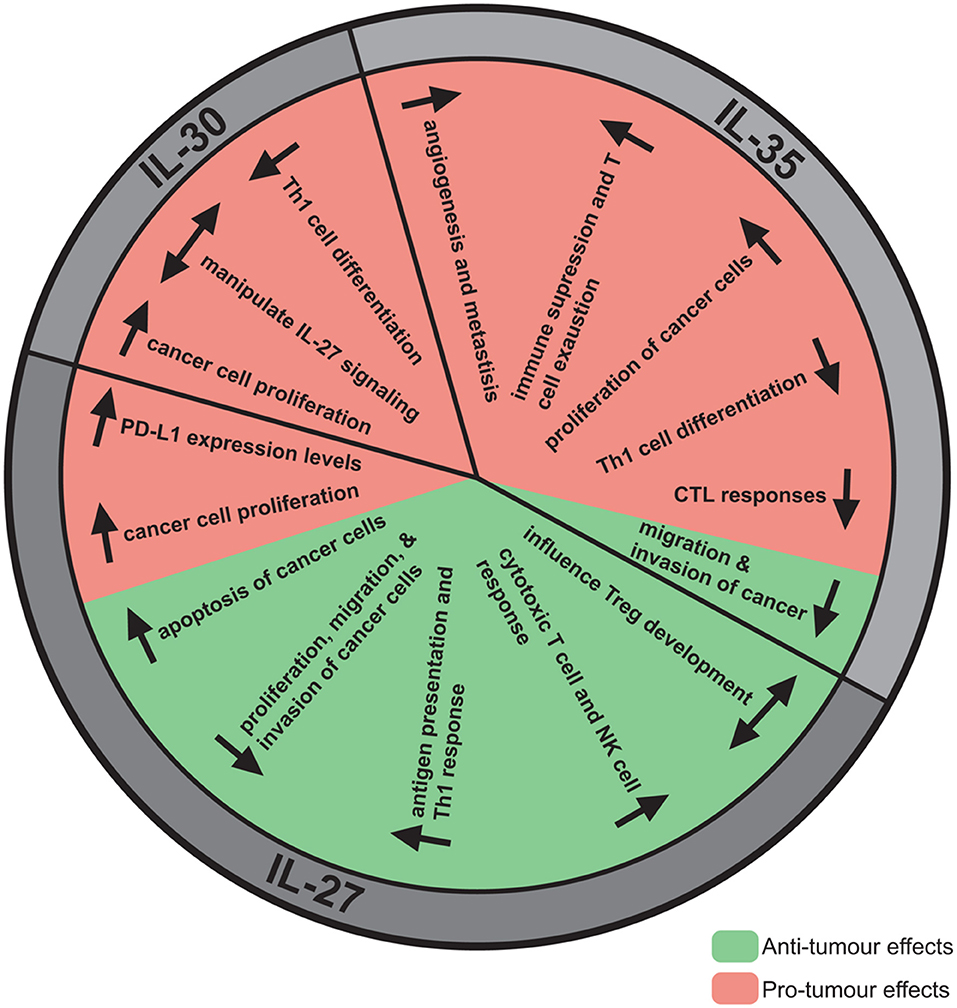
Figure 2. The anti- and pro-tumor effects of IL-27, IL-30, and IL-35. Although IL-27, IL-30, and IL-35 share subunits, these cytokines have direct and indirect effects on the tumor resulting in either tumor progression or elimination. IL-27 has mainly been demonstrated to have anti-tumor effects, most notably decreasing proliferation, migration, and invasion, enhancing apoptosis, and promoting cytotoxic immune responses. Pro-tumor effects have also been observed for IL-27, such as upregulation of PD-L1. Alternatively, IL-30 has not been studied extensively but pro-tumor effects have been identified, such as promoting cancer cell proliferation, and decreasing Th1 differentiation. IL-35 has been implicated in promoting tumor advancement by increasing cancer cell proliferation, angiogenesis, metastasis, immune suppression, and T cell exhaustion. Contrastingly, IL-35 may have anti-tumor effects attributed to its potential role in decreasing cancer cell migration and invasion.
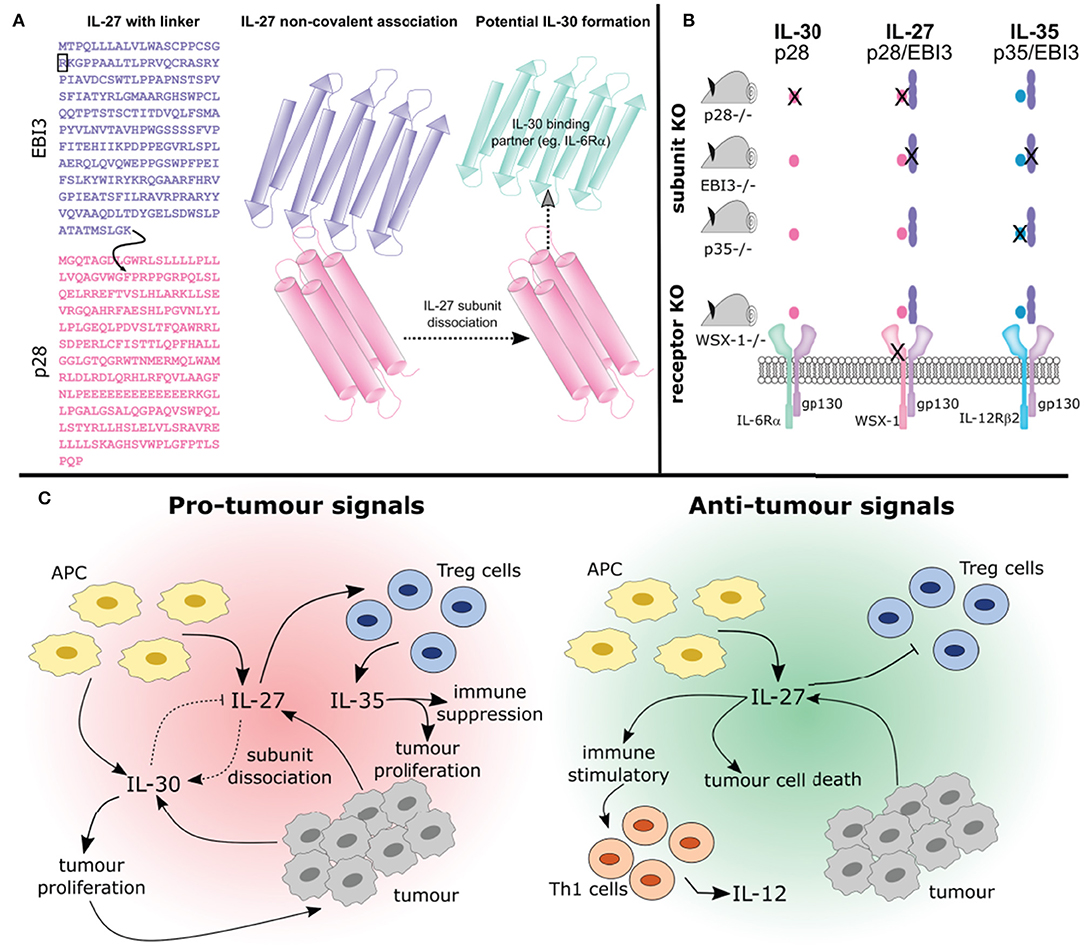
Figure 3. Studying the interplay between IL-27, IL-30, and IL-35. (A) The synthesis of IL-27 as a purified recombinant protein or transduced expression vector varies. Both of these forms of IL-27 are available in two formats: (1) containing a flexible amino acid linker sequence (indicated by the curved black arrow), that joins the EBI3 subunit lacking its signal sequence (indicated by the black box) to phenylalanine 29, after the signal sequence of p28 (A; left) or (2) the two subunits co-expressed which associate non-covalently (A; middle). Thus, engineered IL-27 may differ from its endogenously expressed counterpart whereby the flexible amino acid linker prevents the possibility of subunit dissociation. Furthermore, whether non-covalently associated IL-27 subunits can dissociate to form IL-30 (i.e., the p28 subunit) or if they associate with another binding partner is not known (A; right). (B) Studying the functions of cytokines using knockout mice is complex and the outcomes should be carefully considered. Using p28 knockout mice will result in IL-30 and IL-27 elimination, whereas knockout of p35 eliminates IL-35 and IL-12 (not depicted). Knockout of EBI3 removes both IL-27 and IL-35 (IL-39 is also removed, not shown). Using a WSX-1 receptor chain knockout will prevent IL-27 signaling and may prevent signaling of IL-30. Additionally, IL-35 signaling on B cells will be inhibited (not shown). (C) How these cytokines interact will influence tumor development. The pleiotropic effects of IL-27 produced by APCs can be seen here. IL-27 can promote differentiation of Treg cells which secrete IL-35 resulting in immune suppression and tumor development. Alternatively IL-27 can prevent Treg development and promote anti-cancer Th1 cell development. Importantly, IL-27 may undergo subunit dissociation giving rise to the pro-tumor cytokine IL-30, or can directly act on cancer cells resulting in apoptosis. Overall, the complex relationships between IL-27, IL-30, and IL-35 need to be considered when discussing their potential role in cancer immunity.
Anti-tumor Impact of IL-27
IL-27 can directly suppress tumorigenesis by interacting with cancer cells, or indirectly by stimulating different subsets of immune cells resulting in their activation against a developing tumor. The direct anti-tumor effects of IL-27 results from its ability to suppress cancer cell proliferation, migration, and invasion, and enhance cancer cell death (66–70). For example, IL-27 has direct anti-proliferative effects on both mouse and human melanoma cells in a STAT1 dependent manner (71). IL-27 also directly induces apoptosis in prostate cancer cells, multiple myeloma, non-small cell lung cancer, and ovarian cancer cells (72–75). Furthermore, IL-27 has the potential to interact with novel therapeutics; it synergizes with poly(I:C), a TLR3 agonist, to reduce proliferation of melanoma and prostate cancer cells via TRAIL or TLR3, respectively (76, 77). IL-27 upregulates TLRs in cancer cells and immune cells, resulting in enhanced cellular responsiveness to TLR agonists, supporting the combination of IL-27 and TLR agonists as a potential cancer therapy (39–42, 76–79).
Additionally, IL-27 can promote anti-tumor immune responses by altering development and activation of various T cell subsets and NK cells. CTLs specifically target and eliminate cancer cells expressing a tumor associated antigen (80–84). IL-27 enhances the development, proliferation, and cytotoxic activity of CD8+ T cells, thereby indirectly promoting anti-tumor immunity (85). In in vitro and in vivo models, the use of either recombinant IL-27 or IL-27-transduced cancer cell lines has characterized the role of IL-27 in regulation of the CTL response. Using a colon cancer cell line (C26) transduced with IL-27, Hisada et al. demonstrated that IL-27 promoted C26-specific CD8+ T cell activity resulting in a robust anti-tumor immune response (17). Furthermore, IL-27 enhances anti-tumor CD8+ T cell survival (86). Schreider et al. observed a similar role for IL-27 acting directly on CD8+ human T cells and driving cytotoxic activity (87). The use of mice deficient in WSX-1, EBI3, or p28 also suggest a role for IL-27 in promoting anti-tumor CD8+ T cell responses (88–91). IL-27 is also involved in the development of memory CD8+ T cell responses (89). Taken together, these studies present IL-27 as an anti-tumor cytokine capable of promoting tumor antigen specific CTL development, enhancing their survival, and differentiation into memory effector T cells.
CD4+ T cells play an important role in the TME and can contribute to an anti-tumor immune response. One of the reasons IL-12 demonstrated success as an anti-tumor agent was in part due to its ability to promote a Th1 immune response (92). Similarly, IL-27 can act on naive CD4+ T cells to influence their differentiation into Th1 cells and establish enhanced CD4+ T cell responsiveness to IL-12 (18, 35). On the other hand, IL-27 prevents GATA-3 expression, resulting in reduction of Th2 polarization (35, 93, 94). Since IL-27 regulates the balance of Th1 and Th2 cells, it has the potential to skew the CD4+T cell response toward Th1, which could enhance tumor regression. IL-27 also inhibits the development and function of Treg cells, which suppress T cell activation in the TME permitting tumor cell escape (95–98). Therefore, targeting Tregs and preventing their differentiation, serves as an important consideration in the development of successful cancer immunotherapies. Using a recombinant adenovirus vector expressing IL-27, Zhu et al. demonstrated that IL-27 decreases Treg cell numbers, while increasing IL-10 to prevent excessive inflammation (99). Taken together, IL-27 has important implications in the development of an anti-tumor T cell response by enhancing CTL activation, promoting Th1 mediated anti-tumor immune responses, and inhibiting Th2 and Treg mediated immune suppression.
In addition to having effects on T cells, IL-27 also influences macrophage polarization. Macrophages exist along a spectrum of phenotypes but can be generally classified as M1, predominantly exhibiting anti-tumor effects, or M2, generally displaying pro-tumor functions (100–102). Yao et al. observed that IL-27 was able to skew M2 polarized macrophages toward an M1 phenotype (103). Moreover, the presence of IL-27 reduced proliferation, migration, and invasion of pancreatic cancer cell lines co-cultured with M2 polarized macrophages (103). IL-27 was also able to sensitize the pancreatic cancer cells to gemcitabine, a chemotherapeutic drug, in the co-culture system. This suggests that IL-27 may influence the macrophage phenotype within the TME leading to modulation of tumor growth.
IL-27 also enhances NK cell cytotoxicity toward cancer cells (104–106). The ability of IL-27 to enhance NK cell mediated killing of cancer cells has been demonstrated in a series of murine models including hepatocellular carcinoma, melanoma, head and neck squamous cell carcinoma (107–110). These studies use either recombinant IL-27 or IL-27-transduced cancer cell lines to evaluate how IL-27 modulates NK cell function. In humans, the impact of IL-27 on NK cells in a tumor setting needs to be further investigated, in particular how IL-27 could simultaneously coordinate NK cell activity and tumor cell death.
Pro-Tumor Impact of IL-27
IL-27 has been implicated in promoting cancer progression, as it can promote proliferation of human leukemic cell lines (111). Furthermore, IL-27 can be secreted by tumor cells and high IL-27 levels are associated with advanced cancer (112–114). This association could be attributed to several ways in which IL-27 can influence the immune cells within the TME. As stated earlier, IL-27 directly induces apoptosis of cancer cells resulting in an anti-tumor immune response. However, these apoptotic cancer cells are endocytosed by DCs, which results in IL-27 expression leading to IL-27-dependent Treg activation (115). This concept is further supported by work in EBI3 and IL-27R KO mice demonstrating that IL-27 directly enhances Treg activity by inducing CD39 expression, resulting in tumor growth (116). Additionally, IL-27 stimulation induces PD-L1 expression on several types of cancer cells including: prostate, lung, and hepatocellular carcinoma (117, 118). PD-L1 expression is also induced by IL-27 in immune cell populations such as T cells and myeloid cells (119–121). It is important to consider the impact of IL-27 on PD-L1 expression as increased PD-L1 expression is associated with dampening CTL tumor-specific immune responses. IL-27 can also increase expression of CTLA4, LAG-3 and TIM-3 on T cells (122). There is evidence that combining IL-27 with an immune checkpoint inhibitor may enhance an immune response while simultaneously promoting the anti-tumor effects of IL-27, but further investigation is needed for these combination therapies (99). Taken together, IL-27 serves as an important modulating cytokine in the TME that can be used to either enhance the activation of potent anti-tumor immune cells, directly inhibiting cancer cell proliferation and angiogenesis or promote tumor development and survival (Figure 3C). Understanding how IL-27 is able to provide both immune stimulating and suppressing mechanisms in the TME is an important area of research requiring further investigation.
IL-30: The Loss of Something Gives New Meaning
With the discovery that p28, without EBI3, can function independently as IL-30, new research has emerged to understand how IL-30 modulates immune responses in the TME and its role in tumorigenesis (Figure 2). IL-30 expression has been detected in human breast and prostate cancer tissue samples; administration of IL-30 in murine models enhanced tumor cell proliferation and migration (10, 123, 124). As an independent cytokine, IL-30 can inhibit gp130 signaling (50) in murine cells giving it the potential to negate the anti-tumor effects that IL-27 provides. The mechanisms involved in IL-30 production and its ability to function independently still need to be fully investigated. Understanding these mechanisms will provide insight into the factors involved in EBI3 and IL-27p28 engagement. By promoting this interaction, it may be possible to decrease the amount of independent p28 within the TME resulting in enhanced IL-27 activity, and in turn, a more robust anti-tumor immune response.
In addition to preventing IL-27 signaling, IL-30 can limit IL-12-induced Th1 differentiation (46), thus serving as an additional contributing factor to promoting tumor growth. Indeed, EBI3 KO mice have greater numbers of Treg cells (125). Using a B16-F10 malignant cell-line, Liu et al. demonstrated that s.c injection of B16-F10 cancer cells into EBI3 KO mice resulted in rapid tumor growth when compared to wild type (126). Since these studies use EBI3 KO mice, these mice are lacking IL-27 and IL-35, thus potentiating a role for IL-30 in promoting Treg development and contributing to tumor progression (Figure 3C). It is possible that IL-30 may inhibit the function of IL-27 in the event that both cytokines are co-expressed within the TME. With the limited understanding of IL-30 biology and specifically how it functions within the TME, more research is needed to fully elucidate the interplay between IL-27, IL-30, and IL-35 in cancer progression.
IL-35: Different Partnership, Opposing Effect
It appears that IL-35, consisting of EBI3 associating with p35, rather than p28, brings a plethora of functions and responses that are generally contrasting that of IL-27. Early findings point to a role for IL-35 in promoting cancer development (Figure 2); expression of p35, but not p28, was correlated with EBI3 in Hodgkin lymphoma and nasopharengeal carcinoma (112, 127). In breast cancer, EBI3 levels positively correlate with p35, however no significant correlation between EBI3 and p28 was detected, implicating a role for IL-35 rather than IL-27 (128). The correlation between elevated levels of EBI3 and p35 rather than p28 may hold potential information to how a tumor is able to influence the TME cytokine profile to promote tumorigenesis. Others have shown high levels of EBI3 expression in other cancers, but these studies did not correlate EBI3 expression with p35 or p28 (127, 129, 130). Sauer et al. demonstrated that upon introduction of B16-F10 cells to EBI3 deficient mice, the mice were more resistant to lung metastasis (131); however, this study did not take into account the role of the other cytokine subunits. Currently, the general consensus is that IL-35 is positively correlated with increasing severity and stage of cancer. Using a combination of immunohistochemistry and/or IL-35-specific ELISA, several studies document increased levels of IL-35 in several cancers including: acute myeloid leukemia, colorectal cancer, pancreatic ductal adenocarcinoma (PDAC), clear cell renal carcinoma, breast invasive ductal carcinoma, and prostate carcinoma (132–138).
Like IL-27, expression of IL-35 can directly impact cancer cell survival by acting on tumor cells and due to its non-covalent heterodimeric nature, it is important to consider how IL-35 is delivered in the model system. Nicholl et al. found that recombinant IL-35 enhanced proliferation and survival of the pancreas adenocarcinoma cell line, MiaPaCA-2, in a dose-dependent manner (139). Furthermore, like IL-27, IL-35 influences immune responses in the TME. In both immune competent and Rag1/2-deficient mice, tumor cells engineered to express IL-35 exhibited increased myeloid cell accumulation in the TME resulting in increased tumor angiogenesis (140). This indicates that tumor-produced IL-35 is involved in promoting tumor survival. As well, immune competent mice lacked spontaneous CTL responses to tumors (140), thereby favoring tumor promoting conditions. This study showed that using monoclonal antibodies to neutralize IL-35, tumor development was abrogated. In addition to Tregs, Wang et al. demonstrated that IL-35 can also be produced by human cancer tissues (140). For instance, in breast and lung cancer models, TME macrophages secrete IL-35, which ultimately facilitated metastatic colonization of the cancer (141). Neutralization or knockout of IL-35 in the tumor associated macrophages (TAMs) reduced metastatic colonization (141). Similarly, the continual presence of IL-35 using an expression vector system in vivo can permit neutrophil infiltration into the TME and promote neutrophil polarization toward a pro-tumor phenotype (N2) (142). Together, these studies suggest that cancer cells as well as TAMs can be a source of IL-35, which function alongside neutrophils to contribute to immune suppression and enhanced tumorigenesis.
In human non-small-cell lung carcinoma (NSCLC) patients, IL-35 expression was increased in bronchoalveolar lavage fluid (BALF) from tumor sites. Upon exposure of purified CD4+ or CD8+ T cell populations to recombinant IL-35, both populations exhibited reduced cytotoxicity (143). Additionally, CD8+ T cells from patients with non-viral hepatitis-related hepatocellular carcinoma (HCC) showed a decrease in cytotoxic function when treated with recombinant IL-35 (144). Similarly, human breast cancer cells produce IL-35 which functions to convert conventional T cell populations to iTr35 cells (145). Together, this work supports a role for IL-35 in shifting the TME toward an immunosuppressive state (Figure 3C). Indeed, in a murine model of melanoma, IL-35 promotes T cell exhaustion within the TME by increasing expression of inhibitory receptors PD1, TIM3, and LAG3 (146). When IL-35 was neutralized within the tumor, T cell proliferation and activation were enhanced (146). Interestingly, IL-35 treatment of human pancreatic ductal adenocarcinoma (PDAC) cell lines induced ICAM1 expression indicating a role for IL-35 in tumor metastasis (147). In an orthotopic xenograft mouse model, overexpression of IL-35 contributed significantly to tumor establishment in an ICAM1-dependent manner (147).
The primary function of IL-35 within the TME appears to be in favor of a pro-tumor immune environment. In contrast to the work discussed above, Sun et al. outlined a potential anti-tumor role for IL-35 in NSCLC (148). In human patient samples, decreased IL-35 expression was observed in cancer sites compared to non-cancer sites. Through the use of NSCLC cell lines, this study demonstrated that IL-35 treatment resulted in suppressed beta-catenin expression and decreased migration, invasion, and proliferation of the cancer cells (148). Therefore IL-35 may behave similarly to IL-27 to have divergent roles in cancer progression that may be dependent on cancer type, stage, and TME landscape.
Therapeutic Potential of IL-27, IL-30, and IL-35 Within the TME
The TME is home to many different immune cells that play critical roles in regulating tumor development, notably: myeloid derived suppressor cells (MDSCs), tumor associated macrophages (TAMs), cancer associated fibroblasts (CAFs), tumor associated neutrophils (TANs), T cells, and NK cells. To date, the role of this triumvirate of cytokines in the TME is not well understood, with IL-30 being the most understudied. Furthermore, IL-27 is the only cytokine of the three that is well-characterized and exhibits anti-tumoral properties. Below, we focus on the role of IL-27 and how its anti-tumor potential can be harnessed, as well as how neutralizing the apparent pro-tumor cytokines, IL-30 and IL-35, can further enhance an anti-tumor response in the TME.
MDSCs have immunosuppressive functions within the TME that make these cells a major obstacle for the success of cancer immunotherapies, therefore it is important to consider decreasing their activity in the TME. IL-27 can increase IRF8 expression, which negatively regulates MDSC development, by promoting apoptosis of myeloid cells and decreasing GM-CSF production, a critical cytokine for myeloid cell development (149). Furthermore, immunotherapies focused on inhibiting STAT3 have demonstrated success in preventing MDSC development, which may be attributed to the ability of STAT3 to downregulate IRF8 expression (150–152). Having greater STAT1 than STAT3 signaling can promote anti-tumor immune responses (68, 153). Therefore, it is possible that the addition of IL-27 alongside STAT3 inhibitors may serve to further enhance their efficacy, as inhibition of STAT3 could favor STAT1-dominant signaling, promoting the anti-tumor capacity of IL-27. The addition of IL-27 can promote CXCL10 production by MDSCs, resulting in the recruitment of anti-tumor NK and NKT cells reducing tumor size (154). In contrast, IL-35 can enhance MDSC accumulation resulting in suppression of anti-tumor immune responses (140). Therefore, it is possible that neutralizing IL-35 in the TME can reduce MDSC accumulation and favor an anti-tumor immune response.
Monocytic-MDSCs can differentiate into TAMs, which can be further classified as being M1 (classically activated; anti-tumor) or M2 (alternatively activated; pro-tumor) (100, 155). The majority of TAMs with an M2 phenotype are also PD-L1+ and exhibit an immunosuppressive capacity (156, 157). IL-27 has overlapping functions with IFN-γ (158) and may be able to promote M1 macrophage polarization while inhibiting M2 polarization (103). Furthermore, IL-27 is able to drive Th1 polarization and reduce Th2 polarization, which may also indirectly skew macrophage population to an M1 dominant subset within the TME. Whether IL-27 is able to activate tumoricidal macrophage functions while suppressing MDSC activity within the TME requires future investigation. TAMs can also secrete IL-35 resulting in tumorigenesis (141). This further highlights the importance of neutralizing IL-35 in the TME to favor tumor eradication.
CAFs secrete soluble factors in the TME generating cross-talk with immune cells and cancer cells, resulting in the simultaneous suppression of anti-tumor immune cells and activation of immune suppressive cells (159). Decreasing the proliferation of CAFs or decreasing their immunosuppressive secretory profile may prove to be an effective cancer therapy. In fact, IL-27 can suppress lung fibroblast proliferation by inhibiting TGF-β1 (160). The presence of IL-27 can also promote human lung fibroblast secretion of CXCL10 (161). It is possible that IL-27 may induce production of CXCL10 by CAFs, which may help with immune cell recruitment to the TME. Thus, IL-27 may serve to decrease fibroblast proliferation, while re-educating these cells to produce cytokines/chemokines and trigger an unfavorable environment for tumor cells.
TANs are generally recognized as being pro-tumor in the TME; however, because of their functional plasticity, TANs can take on pro- or anti-tumor states depending on the cytokine environment (162, 163). By inhibiting TGF-β, it may be possible to skew TANs to a more anti-tumor-like (N1) cell, compared to their pro-tumor counterpart (N2), which requires TGF-β (164). The ability of IL-27 to limit TGF-β may serve to promote N1 polarization, while IL-35 has demonstrated the capacity to promote N2 polarization favoring tumor progression (142, 160). Therefore, promoting IL-27 and reducing IL-35 functions on TANs needs further investigation for use as a potential immunotherapy.
To further promote tumorigenesis, the TME can prevent cytotoxic T cell and NK cell activation, while enhancing Treg activity. Using an adenovirus-vector secreting IL-27, Zhu et al. found that the presence of IL-27 in the TME enhanced T cell and NK cell recruitment, while subsequently depleting Tregs (99). This is further supported by research that outlines the role of gp130 signaling in reducing Helios, a transcription factor critical in Treg function (165). These studies outline how IL-27 can reduce Tregs, which may result in the loss of a cellular source of IL-35 leading to a potential anti-tumor immune response. Moreover, IL-27 can enhance cytotoxic anti-tumor responses of CD8+ T cells and NK cells, and therefore may be used to advance current T cell and NK cell therapies (86, 104–106, 166). The ability of IL-27 to increase CTL cytotoxicity while decreasing Treg activity, outlines the potential use of IL-27 in gene-therapy, cancer vaccines, T cell adoptive transfer, or CAR T cell therapies. In addition, use of IL-27 may enhance cytokine-mediated toxicity of NK cells. For example, the use of IL-15 can enhance NK cell cytotoxic function against ovarian cancer (167, 168) and IL-27 has been shown to enhance IL-15 mediated NK cell activation (169). This suggests that IL-27 should be further explored for use in combination with other cytokines to improve cytotoxic immune cell therapies.
As discussed above, IL-27 is endogenously produced as two separate subunits; however, IL-27p28 (IL-30) can signal alone resulting in tumor progression. Using a murine model, IL-30 conditional knockdown in prostate cancer stem cell-like cells resulted in decreased vascularization, and MDSC and Treg numbers, while increasing CTL presence in the tumor (170). Therefore, using therapies that inhibit the presence and/or activity of IL-30 within the TME may aid in tumor reduction. Alternatively, enhancing the probability of IL-27p28 binding with EBI3 to yield IL-27 would increase the abundance of IL-27 in the TME, while decreasing the availability of IL-30, further promoting IL-27 mediated anti-tumor immunity. Moreover, IL-27 can influence the availability of IL-35 and thus may affect the outcome of tumor survival and metastasis. Therefore, targeting different subsets of tumor associated cells by inhibiting IL-30 and IL-35, while promoting IL-27 signaling and production highlights the impact this cytokine triumvirate has on the fate of the TME.
New IL-12 Family Members: IL-39 and IL-Y
IL-39, a complex of IL-23p19 and EBI3 subunits is thought to bind a receptor comprised of IL-23R and gp130 (171). IL-39 is expressed by activated B cells and promotes inflammation in a murine model of lupus (172). Recently, antibodies to IL-39 were shown to be effective in treatment of lupus (173). Interestingly, IL-39 has pro-tumorigenic effects in pancreatic cancer; in a human pancreatic cancer cell line, recombinant IL-39 treatment resulted in decreased p21 mRNA expression, enhancement of proliferation, and decreased apoptosis (174). However, little is known about the biology of this cytokine and its role in other cancers.
IL-Y is a synthetic complex of p28 and p40 subunits, and is thought to bind to a receptor comprised of WSX-1 and IL-12β1 (171). In experimental settings, IL-Y is generally synthesized with the two subunits connected with a linker sequence, and it is commercially available as such. So far, IL-Y has been examined in therapeutic models. In a model of experimental autoimmune uvetis (EAU), injection of IL-Y, created as a fusion protein, inhibited Th1 and Th17 differentiation and function, thereby suppressing EAU (175). Interestingly, p40 alone inhibited Th1 differentiation and function, but p28 alone inhibited Th17 differentiation and function. Injection of the p40 subunit did impact EAU, however injection of p28 alone also suppressed EAU. Together, this indicates the individual functions of p28 and p40 with respect to Th differentiation are not compromised upon the formation of IL-Y. Moreover, the p28 component of IL-Y may be responsible for IL-Y-mediated EAU inhibition. In a diabetic murine model, use of an adenovirus vector expressing IL-Y decreased expression of regulators of inflammation, such as IFN-γ, which resulted in prevention of onset of hyperglycemia (176). Moreover, IL-Y also prevented anti-tumor responses in a murine model of fibrosarcoma and resulted in increased tumor growth in vivo (176). Given the novel uses of IL-Y, it is possible that synthetic combinations of the IL-27-IL-30-IL-35 cytokine subunits may be utilized to direct immune cell activities in the TME in novel immunotherapeutic cancer treatments targeted toward enhancing anti-tumor immune responses, while directly targeting the tumors themselves.
Interplay Between IL-27, IL-30, and IL-35 Subunits
As discussed above, commercially available recombinant IL-27, IL-35, IL-39, and IL-Y may be produced with a peptide linker chain between the two subunits to prevent dissociation, allowing for the study of the specific heterodimeric cytokines (Figure 3A). However, it should be noted that results from subunit deficient mice should be considered carefully (Figure 3B). For example, deficiency in EBI3 will impact IL-27 and IL-35 expression as well as the newly defined IL-39. Similarly, use of p28 deficient mice not only knocks out IL-27, but also IL-30. Several studies using p28 knockout animals perform control experiments whereby recombinant IL-27, containing a linker between the EBI3 and p28 subunits to prevent dissociation, is injected into p28 deficient mice to establish specificity of IL-27 vs. IL-30. Similar experiments should be considered in the use of EBI3 deficient animals as well. Furthermore, it is not known if, upon secretion, the IL-27 or IL-35 subunits could dissociate, leading to the formation of IL-30, IL-39, or other as of yet unidentified cytokines. The somewhat promiscuous use of receptor chains by IL-27-IL-30-IL-35 also adds complexity in teasing out the functional requirements of each cytokine. Studies in models deficient in WSX-1 or gp130 would indicate that one or more of the three cytokines is unable to function, thus obfuscating the interpretations of such models. Furthering our understanding in this regard is critical to defining the functions of these cytokines in the TME.
Conclusion
Interactions between IL-27, IL-30, and IL-35 subunits within the TME may influence tumor development and may contribute to immunotherapeutic outcomes. More research is needed to uncover the individual and unique functions of each cytokine within the TME and their potential positive or inhibitory effects on both immune and cancer cells. When using models to investigate the interactions at play within this group of cytokines it is important to consider that neutralization or knockout of cytokine subunits or their receptors may affect more than one cytokine. For example, knockout of p28 has the potential to affect not only IL-30 activity but also p28/CLF, p28/sIL-6Rα, and IL-27 activities. Furthermore, the promiscuous use of receptor chains by this cytokine triumvirate combined with the potential recombination between cytokine subunits adds to the complexity of interpreting data from knockout or neutralization models. The presence of the artificially added linker sequences in recombinant and transduced IL-27 and IL-35 is important to consider when studying the potential interplay between these cytokines as it impedes the potential formation of IL-30 via dissociation, which would also impact formation of p28 complexes. Understanding the mechanisms of these three cytokines and their complex roles within the TME and the immune landscape is critical for developing therapeutics that promote anti-tumor responses by influencing dynamics between IL-27, IL-30, and IL-35.
Author Contributions
OK, KS, NO, SB, and KG contributed to the conceptualization, discussion, and writing of this review. OK, KS, and KG designed and composed the figures.
Funding
This work was supported by the Prostate Cancer Fight Foundation and the TELUS Ride for Dad to KG. OK and KS was supported by an Ontario Graduate Scholarship. NO was supported by a Natural Sciences and Engineering Research Council of Canada (NSERC) scholarship.
Conflict of Interest
The authors declare that the research was conducted in the absence of any commercial or financial relationships that could be construed as a potential conflict of interest.
References
1. Hanahan D, Weinberg RA. Hallmarks of cancer: the next generation. Cell. (2011) 144:646–74. doi: 10.1016/j.cell.2011.02.013
2. Lippitz BE, Harris RA. Cytokine patterns in cancer patients: a review of the correlation between interleukin 6 and prognosis. Oncoimmunology. (2016) 5:e1093722. doi: 10.1080/2162402X.2015.1093722
3. Li J, Xu L, Run ZC, Feng W, Liu W, Zhang PJ, et al. Multiple cytokine profiling in serum for early detection of gastric cancer. World J Gastroenterol. (2018) 24:2269–78. doi: 10.3748/wjg.v24.i21.2269
4. Goldring MB, Goldring SR. Cytokines and cell growth control. Crit Rev Eukaryot Gene Expr. (1991) 1:301–26.
5. Balkwill FR, Capasso M, Hagemann T. The tumor microenvironment at a glance. J Cell Sci. (2012) 125(Pt 23):5591–6. doi: 10.1242/jcs.116392
6. Rose-John S. Interleukin-6 family cytokines. Cold Spring Harb Perspect Biol. (2018) 10:a028415. doi: 10.1101/cshperspect.a028415
7. Hong DS, Angelo LS, Kurzrock R. Interleukin-6 and its receptor in cancer: implications for translational therapeutics. Cancer. (2007) 110:1911–28. doi: 10.1002/cncr.22999
8. Taniguchi K, Karin M. IL-6 and related cytokines as the critical lynchpins between inflammation and cancer. Semin Immunol. (2014) 26:54–74. doi: 10.1016/j.smim.2014.01.001
9. Yoshimoto T, Chiba Y, Furusawa J, Xu M, Tsunoda R, Higuchi K, et al. Potential clinical application of interleukin-27 as an antitumor agent. Cancer Sci. (2015) 106:1103–10. doi: 10.1111/cas.12731
10. Di Carlo E. Interleukin-30: a novel microenvironmental hallmark of prostate cancer progression. Oncoimmunology. (2014) 3:e27618. doi: 10.4161/onci.27618
11. Notredame C, Higgins DG, Heringa J. T-coffee: a novel method for fast and accurate multiple sequence alignment. J Mol Biol. (2000) 302:205–17. doi: 10.1006/jmbi.2000.4042
12. Murakami M, Kamimura D, Hirano T. Pleiotropy and specificity: insights from the interleukin 6 family of cytokines. Immunity. (2019) 50:812–31. doi: 10.1016/j.immuni.2019.03.027
13. Koneru M, O'Cearbhaill R, Pendharkar S, Spriggs DR, Brentjens RJ. A phase I clinical trial of adoptive T cell therapy using IL-12 secreting MUC-16(ecto) directed chimeric antigen receptors for recurrent ovarian cancer. J Transl Med. (2015) 13:102. doi: 10.1186/s12967-015-0460-x
14. Car BD, Eng VM, Schnyder B, LeHir M, Shakhov AN, Woerly G, et al. Role of interferon-gamma in interleukin 12-induced pathology in mice. Am J Pathol. (1995) 147:1693–707.
15. Leonard JP, Sherman ML, Fisher GL, Buchanan LJ, Larsen G, Atkins MB, et al. Effects of single-dose interleukin-12 exposure on interleukin-12-associated toxicity and interferon-gamma production. Blood. (1997) 90:2541–8.
16. Car BD, Eng VM, Lipman JM, Anderson TD. The toxicology of interleukin-12: a review. Toxicol Pathol. (1999) 27:58–63. doi: 10.1177/019262339902700112
17. Hisada M, Kamiya S, Fujita K, Belladonna ML, Aoki T, Koyanagi Y, et al. Potent antitumor activity of interleukin-27. Cancer Res. (2004) 64:1152–6. doi: 10.1158/0008-5472.CAN-03-2084
18. Pflanz S, Timans JC, Cheung J, Rosales R, Kanzler H, Gilbert J, et al. IL-27, a heterodimeric cytokine composed of EBI3 and p28 protein, induces proliferation of naive CD4+ T cells. Immunity. (2002) 16:779–90. doi: 10.1016/S1074-7613(02)00324-2
19. Rousseau F, Basset L, Froger J, Dinguirard N, Chevalier S, Gascan H. IL-27 structural analysis demonstrates similarities with ciliary neurotrophic factor (CNTF) and leads to the identification of antagonistic variants. Proc Natl Acad Sci USA. (2010) 107:19420–5. doi: 10.1073/pnas.1005793107
20. Gorshkova EA, Nedospasov SA, Shilov ES. [Evolutionary plasticity of IL-6 cytokine family]. Mol Biol. (2016) 50:1039–48. doi: 10.1134/S0026893316060066
21. Petes C, Mariani MK, Yang Y, Grandvaux N, Gee K. Interleukin (IL)-6 Inhibits IL-27- and IL-30-Mediated Inflammatory Responses in Human Monocytes. Front Immunol. (2018) 9:256. doi: 10.3389/fimmu.2018.00256
22. Devergne O, Hummel M, Koeppen H, Le Beau MM, Nathanson EC, Kieff E, et al. A novel interleukin-12 p40-related protein induced by latent Epstein-Barr virus infection in B lymphocytes. J Virol. (1996) 70:1143–53.
23. Pflanz S, Hibbert L, Mattson J, Rosales R, Vaisberg E, Bazan JF, et al. WSX-1 and glycoprotein 130 constitute a signal-transducing receptor for IL-27. J Immunol. (2004) 172:2225–31. doi: 10.4049/jimmunol.172.4.2225
24. Chen Q, Ghilardi N, Wang H, Baker T, Xie MH, Gurney A, et al. Development of Th1-type immune responses requires the type I cytokine receptor TCCR. Nature. (2000) 407:916–20. doi: 10.1038/35038103
25. Villarino AV, Larkin J 3rd, Saris CJ, Caton AJ, Lucas S, Wong T, et al. Positive and negative regulation of the IL-27 receptor during lymphoid cell activation. J Immunol. (2005) 174:7684–91. doi: 10.4049/jimmunol.174.12.7684
26. Dietrich C, Candon S, Ruemmele FM, Devergne O. A soluble form of IL-27Ralpha is a natural IL-27 antagonist. J Immunol. (2014) 192:5382–9. doi: 10.4049/jimmunol.1303435
27. Scheller J, Schuster B, Holscher C, Yoshimoto T, Rose-John S. No inhibition of IL-27 signaling by soluble gp130. Biochem Biophys Res Commun. (2005) 326:724–8. doi: 10.1016/j.bbrc.2004.11.098
28. Lokau J, Agthe M, Garbers C. Generation of soluble interleukin-11 and interleukin-6 receptors: a crucial function for proteases during inflammation. Mediators Inflamm. (2016) 2016:1785021. doi: 10.1155/2016/1785021
29. Owaki T, Asakawa M, Kamiya S, Takeda K, Fukai F, Mizuguchi J, et al. IL-27 suppresses CD28-mediated [correction of medicated] IL-2 production through suppressor of cytokine signaling 3. J Immunol. (2006) 176:2773–80. doi: 10.4049/jimmunol.176.5.2773
30. Larousserie F, Charlot P, Bardel E, Froger J, Kastelein RA, Devergne O. Differential effects of IL-27 on human B cell subsets. J Immunol. (2006) 176:5890–7. doi: 10.4049/jimmunol.176.10.5890
31. Yoshimura T, Takeda A, Hamano S, Miyazaki Y, Kinjyo I, Ishibashi T, et al. Two-sided roles of IL-27: induction of Th1 differentiation on naive CD4+ T cells versus suppression of proinflammatory cytokine production including IL-23-induced IL-17 on activated CD4+ T cells partially through STAT3-dependent mechanism. J Immunol. (2006) 177:5377–85. doi: 10.4049/jimmunol.177.8.5377
32. Kamakura M, Morisawa K, Komi H, Tomatani A, Saito F, Konishi Y, et al. Regulation of IL-27p28 gene by lipopolysaccharide in dendritic DC2.4 cells. Biochem Biophys Res Commun. (2006) 349:1372–7. doi: 10.1016/j.bbrc.2006.09.004
33. Molle C, Nguyen M, Flamand V, Renneson J, Trottein F, De Wit D, et al. IL-27 synthesis induced by TLR ligation critically depends on IFN regulatory factor 3. J Immunol. (2007) 178:7607–15. doi: 10.4049/jimmunol.178.12.7607
34. Villarino AV, Stumhofer JS, Saris CJ, Kastelein RA, de Sauvage FJ, Hunter CA. IL-27 limits IL-2 production during Th1 differentiation. J Immunol. (2006) 176:237–47. doi: 10.4049/jimmunol.176.1.237
35. Lucas S, Ghilardi N, Li J, de Sauvage FJ. IL-27 regulates IL-12 responsiveness of naive CD4+ T cells through Stat1-dependent and -independent mechanisms. Proc Natl Acad Sci USA. (2003) 100:15047–52. doi: 10.1073/pnas.2536517100
36. Diveu C, McGeachy MJ, Boniface K, Stumhofer JS, Sathe M, Joyce-Shaikh B, et al. IL-27 blocks RORc expression to inhibit lineage commitment of Th17 cells. J Immunol. (2009) 182:5748–56. doi: 10.4049/jimmunol.0801162
37. Hall AO, Beiting DP, Tato C, John B, Oldenhove G, Lombana CG, et al. The cytokines interleukin 27 and interferon-gamma promote distinct Treg cell populations required to limit infection-induced pathology. Immunity. (2012) 37:511–23. doi: 10.1016/j.immuni.2012.06.014
38. Kim G, Shinnakasu R, Saris CJ, Cheroutre H, Kronenberg M. A novel role for IL-27 in mediating the survival of activated mouse CD4 T lymphocytes. J Immunol. (2013) 190:1510–8. doi: 10.4049/jimmunol.1201017
39. Guzzo C, Ayer A, Basta S, Banfield BW, Gee K. IL-27 enhances LPS-induced proinflammatory cytokine production via upregulation of TLR4 expression and signaling in human monocytes. J Immunol. (2012) 188:864–73. doi: 10.4049/jimmunol.1101912
40. Petes C, Wynick C, Guzzo C, Mehta D, Logan S, Banfield BW, et al. IL-27 enhances LPS-induced IL-1beta in human monocytes and murine macrophages. J Leukoc Biol. (2017) 102:83–94. doi: 10.1189/jlb.3A0316-098R
41. Petes C, Mintsopoulos V, Finnen RL, Banfield BW, Gee K. The effects of CD14 and IL-27 on induction of endotoxin tolerance in human monocytes and macrophages. J Biol Chem. (2018) 293:17631–45. doi: 10.1074/jbc.RA118.003501
42. Petes C, Odoardi N, Plater SM, Martin NL, Gee K. IL-27 amplifies cytokine responses to Gram-negative bacterial products and Salmonella typhimurium infection. Sci Rep. (2018) 8:13704. doi: 10.1038/s41598-018-32007-y
43. Feng XM, Chen XL, Liu N, Chen Z, Zhou YL, Han ZB, et al. Interleukin-27 upregulates major histocompatibility complex class II expression in primary human endothelial cells through induction of major histocompatibility complex class II transactivator. Hum Immunol. (2007) 68:965–72. doi: 10.1016/j.humimm.2007.10.004
44. Feng XM, Liu N, Yang SG, Hu LY, Chen XL, Fang ZH, et al. Regulation of the class II and class I MHC pathways in human THP-1 monocytic cells by interleukin-27. Biochem Biophys Res Commun. (2008) 367:553–9. doi: 10.1016/j.bbrc.2007.12.154
45. Jung JY, Roberts LL, Robinson CM. The presence of interleukin-27 during monocyte-derived dendritic cell differentiation promotes improved antigen processing and stimulation of T cells. Immunology. (2015) 144:649–60. doi: 10.1111/imm.12417
46. Dibra D, Cutrera J, Xia X, Kallakury B, Mishra L, Li S. Interleukin-30: a novel antiinflammatory cytokine candidate for prevention and treatment of inflammatory cytokine-induced liver injury. Hepatology. (2012) 55:1204–14. doi: 10.1002/hep.24814
47. Zhang S, Liang R, Luo W, Liu C, Wu X, Gao Y, et al. High susceptibility to liver injury in IL-27 p28 conditional knockout mice involves intrinsic interferon-gamma dysregulation of CD4+ T cells. Hepatology. (2013) 57:1620–31. doi: 10.1002/hep.26166
48. Mitra A, Satelli A, Yan J, Xueqing X, Gagea M, Hunter CA, et al. IL-30 (IL27p28) attenuates liver fibrosis through inducing NKG2D-rae1 interaction between NKT and activated hepatic stellate cells in mice. Hepatology. (2014) 60:2027–39. doi: 10.1002/hep.27392
49. Yan J, Mitra A, Hu J, Cutrera JJ, Xia X, Doetschman T, et al. Interleukin-30 (IL27p28) alleviates experimental sepsis by modulating cytokine profile in NKT cells. J Hepatol. (2016) 64:1128–36. doi: 10.1016/j.jhep.2015.12.020
50. Stumhofer JS, Tait ED, Quinn WJ 3rd, Hosken N, Spudy B, Goenka R, et al. A role for IL-27p28 as an antagonist of gp130-mediated signaling. Nat. Immunol. (2010) 11:1119–26. doi: 10.1038/ni.1957
51. Crabe S, Guay-Giroux A, Tormo AJ, Duluc D, Lissilaa R, Guilhot F, et al. The IL-27 p28 subunit binds cytokine-like factor 1 to form a cytokine regulating NK and T cell activities requiring IL-6R for signaling. J Immunol. (2009) 183:7692–702. doi: 10.4049/jimmunol.0901464
52. Garbers C, Spudy B, Aparicio-Siegmund S, Waetzig GH, Sommer J, Holscher C, et al. An interleukin-6 receptor-dependent molecular switch mediates signal transduction of the IL-27 cytokine subunit p28 (IL-30) via a gp130 protein receptor homodimer. J Biol Chem. (2013) 288:4346–54. doi: 10.1074/jbc.M112.432955
53. Dibra D, Cutrera JJ, Li S. Coordination between TLR9 signaling in macrophages and CD3 signaling in T cells induces robust expression of IL-30. J Immunol. (2012) 188:3709–15. doi: 10.4049/jimmunol.1100883
54. Tormo AJ, Meliani Y, Beaupre LA, Sharma M, Fritz JH, Elson G, et al. The composite cytokine p28/cytokine-like factor 1 sustains B cell proliferation and promotes plasma cell differentiation. J Immunol. (2013) 191:1657–65. doi: 10.4049/jimmunol.1201595
55. Collison LW, Workman CJ, Kuo TT, Boyd K, Wang Y, Vignali KM, et al. The inhibitory cytokine IL-35 contributes to regulatory T-cell function. Nature. (2007) 450:566–9. doi: 10.1038/nature06306
56. Niedbala W, Wei XQ, Cai B, Hueber AJ, Leung BP, McInnes IB, et al. IL-35 is a novel cytokine with therapeutic effects against collagen-induced arthritis through the expansion of regulatory T cells and suppression of Th17 cells. Eur J Immunol. (2007) 37:3021–9. doi: 10.1002/eji.200737810
57. Collison LW, Vignali DA. Interleukin-35: odd one out or part of the family? Immunol Rev. (2008) 226:248–62. doi: 10.1111/j.1600-065X.2008.00704.x
58. Collison LW, Delgoffe GM, Guy CS, Vignali KM, Chaturvedi V, Fairweather D, et al. The composition and signaling of the IL-35 receptor are unconventional. Nat Immunol. (2012) 13:290–9. doi: 10.1038/ni.2227
59. Shen P, Roch T, Lampropoulou V, O'Connor RA, Stervbo U, Hilgenberg E, et al. IL-35-producing B cells are critical regulators of immunity during autoimmune and infectious diseases. Nature. (2014) 507:366–70. doi: 10.1038/nature12979
60. Collison LW, Chaturvedi V, Henderson AL, Giacomin PR, Guy C, Bankoti J, et al. IL-35-mediated induction of a potent regulatory T cell population. Nat Immunol. (2010) 11:1093–101. doi: 10.1038/ni.1952
61. Seyerl M, Kirchberger S, Majdic O, Seipelt J, Jindra C, Schrauf C, et al. Human rhinoviruses induce IL-35-producing Treg via induction of B7-H1 (CD274) and sialoadhesin (CD169) on DC. Eur J Immunol. (2010) 40:321–9. doi: 10.1002/eji.200939527
62. Tedder TF, Leonard WJ. Autoimmunity: regulatory B cells–IL-35 and IL-21 regulate the regulators. Nat Rev Rheumatol. (2014) 10:452–3. doi: 10.1038/nrrheum.2014.95
63. Terayama H, Yoshimoto T, Hirai S, Naito M, Qu N, Hatayama N, et al. Contribution of IL-12/IL-35 common subunit p35 to maintaining the testicular immune privilege. PLoS ONE. (2014) 9:e96120. doi: 10.1371/journal.pone.0096120
64. Wang RX, Yu CR, Dambuza IM, Mahdi RM, Dolinska MB, Sergeev YV, et al. Interleukin-35 induces regulatory B cells that suppress autoimmune disease. Nat Med. (2014) 20:633–41. doi: 10.1038/nm.3554
65. Dixon KO, van der Kooij SW, Vignali DA, van Kooten C. Human tolerogenic dendritic cells produce IL-35 in the absence of other IL-12 family members. Eur J Immunol. (2015) 45:1736–47. doi: 10.1002/eji.201445217
66. Shimizu M, Shimamura M, Owaki T, Asakawa M, Fujita K, Kudo M, et al. Antiangiogenic and antitumor activities of IL-27. J Immunol. (2006) 176:7317–24. doi: 10.4049/jimmunol.176.12.7317
67. Ho MY, Leu SJ, Sun GH, Tao MH, Tang SJ, Sun KH. IL-27 directly restrains lung tumorigenicity by suppressing cyclooxygenase-2-mediated activities. J Immunol. (2009) 183:6217–26. doi: 10.4049/jimmunol.0901272
68. Kachroo P, Lee MH, Zhang L, Baratelli F, Lee G, Srivastava MK, et al. IL-27 inhibits epithelial-mesenchymal transition and angiogenic factor production in a STAT1-dominant pathway in human non-small cell lung cancer. J Exp Clin Cancer Res. (2013) 32:97. doi: 10.1186/1756-9966-32-97
69. Cao JY, Yin HS, Li HS, Yu XQ, Han X. Interleukin-27 augments the inhibitory effects of sorafenib on bladder cancer cells. Braz J Med Biol Res. (2017) 50:e6207. doi: 10.1590/1414-431x20176207
70. Wang T, Chen Y, Nie H, Huang Y, Zhao Y, Yang J. IL-27 inhibits non-small-cell lung cancer cell metastasis by miR-935 in vitro. Onco Targets Ther. (2019) 12:1447–54. doi: 10.2147/OTT.S173207
71. Yoshimoto T, Morishima N, Mizoguchi I, Shimizu M, Nagai H, Oniki S, et al. Antiproliferative activity of IL-27 on melanoma. J Immunol. (2008) 180:6527–35. doi: 10.4049/jimmunol.180.10.6527
72. Cocco C, Giuliani N, Di Carlo E, Ognio E, Storti P, Abeltino M, et al. Interleukin-27 acts as multifunctional antitumor agent in multiple myeloma. Clin Cancer Res. (2010) 16:4188–97. doi: 10.1158/1078-0432.CCR-10-0173
73. Di Carlo E, Sorrentino C, Zorzoli A, Di Meo S, Tupone MG, Ognio E, et al. The antitumor potential of Interleukin-27 in prostate cancer. Oncotarget. (2014) 5:10332–41. doi: 10.18632/oncotarget.1425
74. Airoldi I, Tupone MG, Esposito S, Russo MV, Barbarito G, Cipollone G, et al. Interleukin-27 re-educates intratumoral myeloid cells and down-regulates stemness genes in non-small cell lung cancer. Oncotarget. (2015) 6:3694–708. doi: 10.18632/oncotarget.2797
75. Zhang Z, Zhou B, Zhang K, Song Y, Zhang L, Xi M. IL-27 suppresses SKOV3 cells proliferation by enhancing STAT3 and inhibiting the Akt signal pathway. Mol Immunol. (2016) 78:155–63. doi: 10.1016/j.molimm.2016.09.014
76. Chiba Y, Mizoguchi I, Mitobe K, Higuchi K, Nagai H, Nishigori C, et al. IL-27 enhances the expression of TRAIL and TLR3 in human melanomas and inhibits their tumor growth in cooperation with a TLR3 agonist poly(I:C) partly in a TRAIL-dependent manner. PLoS ONE. (2013) 8:e76159. doi: 10.1371/journal.pone.0076159
77. Kourko O, Smyth R, Cino D, Seaver K, Petes C, Eo SY, et al. Poly(I:C)-mediated death of human prostate cancer cell lines is induced by interleukin-27 treatment. J Interferon Cytokine Res. (2019). 39:483–94. doi: 10.1089/jir.2018.0166
78. Guzzo C, Che Mat NF, Gee K. Interleukin-27 induces a STAT1/3- and NF-kappaB-dependent proinflammatory cytokine profile in human monocytes. J Biol Chem. (2010) 285:24404–11. doi: 10.1074/jbc.M110.112599
79. Su Y, Yao H, Wang H, Xu F, Li D, Li D, et al. IL-27 enhances innate immunity of human pulmonary fibroblasts and epithelial cells through upregulation of TLR4 expression. Am J Physiol Lung Cell Mol Physiol. (2016) 310:L133–141. doi: 10.1152/ajplung.00307.2015
80. Boon T, Coulie PG, Van den Eynde B. Tumor antigens recognized by T cells. Immunol Today. (1997) 18:267–8. doi: 10.1016/S0167-5699(97)80020-5
81. Tanaka H, Yoshizawa H, Yamaguchi Y, Ito K, Kagamu H, Suzuki E, et al. Successful adoptive immunotherapy of murine poorly immunogenic tumor with specific effector cells generated from gene-modified tumor-primed lymph node cells. J Immunol. (1999) 162:3574–82.
82. Halle S, Halle O, Forster R. Mechanisms and dynamics of T cell-mediated cytotoxicity in vivo. Trends Immunol. (2017) 38:432–43. doi: 10.1016/j.it.2017.04.002
83. Durgeau A, Virk Y, Corgnac S, Mami-Chouaib F. Recent advances in targeting CD8 T-cell immunity for more effective cancer immunotherapy. Front Immunol. (2018) 9:14. doi: 10.3389/fimmu.2018.00014
84. Zamora AE, Crawford JC, Thomas PG. Hitting the target: how T cells detect and eliminate tumors. J Immunol. (2018) 200:392–9. doi: 10.4049/jimmunol.1701413
85. Salcedo R, Stauffer JK, Lincoln E, Back TC, Hixon JA, Hahn C, et al. IL-27 mediates complete regression of orthotopic primary and metastatic murine neuroblastoma tumors: role for CD8+ T cells. J Immunol. (2004) 173:7170–82. doi: 10.4049/jimmunol.173.12.7170
86. Liu Z, Liu JQ, Talebian F, Wu LC, Li S, Bai XF. IL-27 enhances the survival of tumor antigen-specific CD8+ T cells and programs them into IL-10-producing, memory precursor-like effector cells. Eur J Immunol. (2013) 43:468–79. doi: 10.1002/eji.201242930
87. Schneider R, Yaneva T, Beauseigle D, El-Khoury L, Arbour N. IL-27 increases the proliferation and effector functions of human naive CD8+ T lymphocytes and promotes their development into Tc1 cells. Eur J Immunol. (2011) 41:47–59. doi: 10.1002/eji.201040804
88. Morishima N, Owaki T, Asakawa M, Kamiya S, Mizuguchi J, Yoshimoto T. Augmentation of effector CD8+ T cell generation with enhanced granzyme B expression by IL-27. J Immunol. (2005) 175:1686–93. doi: 10.4049/jimmunol.175.3.1686
89. Pennock ND, Gapin L, Kedl RM. IL-27 is required for shaping the magnitude, affinity distribution, and memory of T cells responding to subunit immunization. Proc Natl Acad Sci USA. (2014) 111:16472–7. doi: 10.1073/pnas.1407393111
90. Liu Z, Wu L, Zhu J, Zhu X, Zhu J, Liu JQ, et al. Interleukin-27 signalling induces stem cell antigen-1 expression in T lymphocytes in vivo. Immunology. (2017) 152:638–47. doi: 10.1111/imm.12805
91. Zhang P, Lee JS, Gartlan KH, Schuster IS, Comerford I, Varelias A, et al. Eomesodermin promotes the development of type 1 regulatory T (TR1) cells. Sci Immunol. (2017) 2:eaah7152. doi: 10.1126/sciimmunol.aah7152
92. Trinchieri G. Interleukin-12 and the regulation of innate resistance and adaptive immunity. Nat Rev Immunol. (2003) 3:133–46. doi: 10.1038/nri1001
93. Artis D, Villarino A, Silverman M, He W, Thornton EM, Mu S, et al. The IL-27 receptor (WSX-1) is an inhibitor of innate and adaptive elements of type 2 immunity. J Immunol. (2004) 173:5626–34. doi: 10.4049/jimmunol.173.9.5626
94. Yoshimoto T, Yoshimoto T, Yasuda K, Mizuguchi J, Nakanishi K. IL-27 suppresses Th2 cell development and Th2 cytokines production from polarized Th2 cells: a novel therapeutic way for Th2-mediated allergic inflammation. J Immunol. (2007) 179:4415–23. doi: 10.4049/jimmunol.179.7.4415
95. Beyer M, Schultze JL. Regulatory T cells in cancer. Blood. (2006) 108:804–11. doi: 10.1182/blood-2006-02-002774
96. Huber M, Steinwald V, Guralnik A, Brustle A, Kleemann P, Rosenplanter C, et al. IL-27 inhibits the development of regulatory T cells via STAT3. Int Immunol. (2008) 20:223–34. doi: 10.1093/intimm/dxm139
97. Wojno ED, Hosken N, Stumhofer JS, O'Hara AC, Mauldin E, Fang Q, et al. A role for IL-27 in limiting T regulatory cell populations. J Immunol. (2011) 187:266–73. doi: 10.4049/jimmunol.1004182
98. Facciabene A, Motz GT, Coukos G. T-regulatory cells: key players in tumor immune escape and angiogenesis. Cancer Res. (2012) 72:2162–71. doi: 10.1158/0008-5472.CAN-11-3687
99. Zhu J, Liu JQ, Shi M, Cheng X, Ding M, Zhang JC, et al. IL-27 gene therapy induces depletion of Tregs and enhances the efficacy of cancer immunotherapy. JCI Insight. (2018) 3:e98745. doi: 10.1172/jci.insight.98745
100. Mulder R, Banete A, Seaver K, Basta S. M(IL-4) Tissue macrophages support efficient interferon-gamma production in antigen-specific CD8(+) T cells with reduced proliferative capacity. Front Immunol. (2017) 8:1629. doi: 10.3389/fimmu.2017.01629
101. Poh AR, Ernst M. Targeting macrophages in cancer: from bench to bedside. Front Oncol. (2018) 8:49. doi: 10.3389/fonc.2018.00049
102. Najafi M, Hashemi Goradel N, Farhood B, Salehi E, Nashtaei MS, Khanlarkhani N, et al. Macrophage polarity in cancer: a review. J Cell Biochem. (2019) 120:2756–65. doi: 10.1002/jcb.27646
103. Yao L, Wang M, Niu Z, Liu Q, Gao X, Zhou L, et al. Interleukin-27 inhibits malignant behaviors of pancreatic cancer cells by targeting M2 polarized tumor associated macrophages. Cytokine. (2017) 89:194–200. doi: 10.1016/j.cyto.2015.12.003
104. Liu L, Wang S, Shan B, Shao L, Sato A, Kawamura K, et al. IL-27-mediated activation of natural killer cells and inflammation produced antitumour effects for human oesophageal carcinoma cells. Scand J Immunol. (2008) 68:22–9. doi: 10.1111/j.1365-3083.2008.02111.x
105. Ziblat A, Domaica CI, Spallanzani RG, Iraolagoitia XL, Rossi LE, Avila DE, et al. IL-27 stimulates human NK-cell effector functions and primes NK cells for IL-18 responsiveness. Eur J Immunol. (2015) 45:192–202. doi: 10.1002/eji.201444699
106. Nicholson SE, Keating N, Belz GT. Natural killer cells and anti-tumor immunity. Mol Immunol. (2017). 110:40–7. doi: 10.1016/j.molimm.2017.12.002
107. Oniki S, Nagai H, Horikawa T, Furukawa J, Belladonna ML, Yoshimoto T, et al. Interleukin-23 and interleukin-27 exert quite different antitumor and vaccine effects on poorly immunogenic melanoma. Cancer Res. (2006) 66:6395–404. doi: 10.1158/0008-5472.CAN-05-4087
108. Hu P, Hu HD, Chen M, Peng ML, Tang L, Tang KF, et al. Expression of interleukins-23 and 27 leads to successful gene therapy of hepatocellular carcinoma. Mol Immunol. (2009) 46:1654–62. doi: 10.1016/j.molimm.2009.02.025
109. Matsui M, Kishida T, Nakano H, Yoshimoto K, Shin-Ya M, Shimada T, et al. Interleukin-27 activates natural killer cells and suppresses NK-resistant head and neck squamous cell carcinoma through inducing antibody-dependent cellular cytotoxicity. Cancer Res. (2009) 69:2523–30. doi: 10.1158/0008-5472.CAN-08-2793
110. Cocco C, Pistoia V, Airoldi I. Anti-leukemic properties of IL-12, IL-23 and IL-27: differences and similarities in the control of pediatric B acute lymphoblastic leukemia. Crit Rev Oncol Hematol. (2012) 83:310–8. doi: 10.1016/j.critrevonc.2011.11.006
111. Jia H, Dilger P, Bird C, Wadhwa M. IL-27 promotes proliferation of human leukemic cell lines through the MAPK/ERK signaling pathway and suppresses sensitivity to chemotherapeutic drugs. J Interferon Cytokine Res. (2016) 36:302–16. doi: 10.1089/jir.2015.0091
112. Larousserie F, Bardel E, Coulomb L'Hermine A, Canioni D, Brousse N, Kastelein RA, et al. Variable expression of Epstein-Barr virus-induced gene 3 during normal B-cell differentiation and among B-cell lymphomas. J Pathol. (2006) 209:360–8. doi: 10.1002/path.1995
113. Diakowska D, Lewandowski A, Markocka-Maczka K, Grabowski K. Concentration of serum interleukin-27 increase in patients with lymph node metastatic gastroesophageal cancer. Adv Clin Exp Med. (2013) 22:683–91.
114. Gonin J, Carlotti A, Dietrich C, Audebourg A, Radenen-Bussiere B, Caignard A, et al. Expression of IL-27 by tumor cells in invasive cutaneous and metastatic melanomas [corrected]. PLoS ONE. (2013) 8:e75694. doi: 10.1371/journal.pone.0075694
115. Sekar D, Hahn C, Brune B, Roberts E, Weigert A. Apoptotic tumor cells induce IL-27 release from human DCs to activate Treg cells that express CD69 and attenuate cytotoxicity. Eur J Immunol. (2012) 42:1585–98. doi: 10.1002/eji.201142093
116. Park YJ, Ryu H, Choi G, Kim BS, Hwang ES, Kim HS, et al. IL-27 confers a protumorigenic activity of regulatory T cells via CD39. Proc Natl Acad Sci USA. (2019) 116:3106–11. doi: 10.1073/pnas.1810254116
117. Carbotti G, Barisione G, Airoldi I, Mezzanzanica D, Bagnoli M, Ferrero S, et al. IL-27 induces the expression of IDO and PD-L1 in human cancer cells. Oncotarget. (2015) 6:43267–80. doi: 10.18632/oncotarget.6530
118. Rolvering C, Zimmer AD, Ginolhac A, Margue C, Kirchmeyer M, Servais F, et al. The PD-L1- and IL6-mediated dampening of the IL27/STAT1 anticancer responses are prevented by alpha-PD-L1 or alpha-IL6 antibodies. J Leukoc Biol. (2018) 104:969–85. doi: 10.1002/JLB.MA1217-495R
119. Ribeiro ST, Ribot JC, Silva-Santos B. Five layers of receptor signaling in gammadelta T-cell differentiation and activation. Front Immunol. (2015) 6:15. doi: 10.3389/fimmu.2015.00015
120. Horlad H, Ma C, Yano H, Pan C, Ohnishi K, Fujiwara Y, et al. An IL-27/Stat3 axis induces expression of programmed cell death 1 ligands (PD-L1/2) on infiltrating macrophages in lymphoma. Cancer Sci. (2016) 107:1696–704. doi: 10.1111/cas.13065
121. Versteven M, Van den Bergh JMJ, Marcq E, Smits ELJ, Van Tendeloo VFI, Hobo W, et al. Dendritic cells and programmed death-1 blockade: a joint venture to combat cancer. Front Immunol. (2018) 9:394. doi: 10.3389/fimmu.2018.00394
122. DeLong JH, O'Hara Hall A, Rausch M, Moodley D, Perry J, Park J, et al. IL-27 and TCR stimulation promote T cell expression of multiple inhibitory receptors. Immunohorizons. (2019) 3:13–25. doi: 10.4049/immunohorizons.1800083
123. Di Meo S, Airoldi I, Sorrentino C, Zorzoli A, Esposito S, Di Carlo E. Interleukin-30 expression in prostate cancer and its draining lymph nodes correlates with advanced grade and stage. Clin Cancer Res. (2014) 20:585–94. doi: 10.1158/1078-0432.CCR-13-2240
124. Airoldi I, Cocco C, Sorrentino C, Angelucci D, Di Meo S, Manzoli L, et al. Interleukin-30 Promotes Breast Cancer Growth and Progression. Cancer Res. (2016) 76:6218–29. doi: 10.1158/0008-5472.CAN-16-0189
125. Liu JQ, Liu Z, Zhang X, Shi Y, Talebian F, Carl JWJr, et al. Increased Th17 and regulatory T cell responses in EBV-induced gene 3-deficient mice lead to marginally enhanced development of autoimmune encephalomyelitis. J Immunol. (2012) 188:3099–106. doi: 10.4049/jimmunol.1100106
126. Liu Z, Liu JQ, Shi Y, Zhu X, Liu Z, Li MS, et al. Epstein-Barr virus-induced gene 3-deficiency leads to impaired antitumor T-cell responses and accelerated tumor growth. Oncoimmunology. (2015) 4:e989137. doi: 10.4161/2162402X.2014.989137
127. Niedobitek G, Pazolt D, Teichmann M, Devergne O. Frequent expression of the Epstein-Barr virus (EBV)-induced gene, EBI3, an IL-12 p40-related cytokine, in Hodgkin and Reed-Sternberg cells. J Pathol. (2002) 198:310–6. doi: 10.1002/path.1217
128. Jiang J, Liu X. Upregulated EBI3 correlates with poor outcome and tumor progression in breast cancer. Oncol Res Treat. (2018) 41:111–5. doi: 10.1159/000484935
129. Nishino R, Takano A, Oshita H, Ishikawa N, Akiyama H, Ito H, et al. Identification of Epstein-Barr virus-induced gene 3 as a novel serum and tissue biomarker and a therapeutic target for lung cancer. Clin Cancer Res. (2011) 17:6272–86. doi: 10.1158/1078-0432.CCR-11-0060
130. Pylayeva-Gupta Y, Das S, Handler JS, Hajdu CH, Coffre M, Koralov SB, et al. IL35-producing B cells promote the development of pancreatic neoplasia. Cancer Discov. (2016) 6:247–55. doi: 10.1158/2159-8290.CD-15-0843
131. Sauer KA, Maxeiner JH, Karwot R, Scholtes P, Lehr HA, Birkenbach M, et al. Immunosurveillance of lung melanoma metastasis in EBI-3-deficient mice mediated by CD8+ T cells. J Immunol. (2008) 181:6148–57. doi: 10.4049/jimmunol.181.9.6148
132. Wu H, Li P, Shao N, Ma J, Ji M, Sun X, et al. Aberrant expression of Treg-associated cytokine IL-35 along with IL-10 and TGF-beta in acute myeloid leukemia. Oncol Lett. (2012) 3:1119–23. doi: 10.3892/ol.2012.614
133. Zeng JC, Zhang Z, Li TY, Liang YF, Wang HM, Bao JJ, et al. Assessing the role of IL-35 in colorectal cancer progression and prognosis. Int J Clin Exp Pathol. (2013) 6:1806–16.
134. Jin P, Ren H, Sun W, Xin W, Zhang H, Hao J. Circulating IL-35 in pancreatic ductal adenocarcinoma patients. Hum Immunol. (2014) 75:29–33. doi: 10.1016/j.humimm.2013.09.018
135. Jin L, Xu X, Ye B, Pan M, Shi Z, Hu Y. Elevated serum interleukin-35 levels correlate with poor prognosis in patients with clear cell renal cell carcinoma. Int J Clin Exp Med. (2015) 8:18861–6.
136. Huang A, Cheng L, He M, Nie J, Wang J, Jiang K. Interleukin-35 on B cell and T cell induction and regulation. J Inflamm. (2017) 14:16. doi: 10.1186/s12950-017-0164-5
137. Zhao Z, Chen X, Hao S, Jia R, Wang N, Chen S, et al. Increased interleukin-35 expression in tumor-infiltrating lymphocytes correlates with poor prognosis in patients with breast cancer. Cytokine. (2017) 89:76–81. doi: 10.1016/j.cyto.2016.09.012
138. Zhou C, Zhang J, Chen Y, Wang H, Hou J. Interleukin-35 as a predictor of prostate cancer in patients undergoing initial prostate biopsy. Onco Targets Ther. (2017) 10:3485–91. doi: 10.2147/OTT.S135873
139. Nicholl MB, Ledgewood CL, Chen X, Bai Q, Qin C, Cook KM, et al. IL-35 promotes pancreas cancer growth through enhancement of proliferation and inhibition of apoptosis: evidence for a role as an autocrine growth factor. Cytokine. (2014) 70:126–33. doi: 10.1016/j.cyto.2014.06.020
140. Wang Z, Liu JQ, Liu Z, Shen R, Zhang G, Xu J, et al. Tumor-derived IL-35 promotes tumor growth by enhancing myeloid cell accumulation and angiogenesis. J Immunol. (2013) 190:2415–23. doi: 10.4049/jimmunol.1202535
141. Lee CC, Lin JC, Hwang WL, Kuo YJ, Chen HK, Tai SK, et al. Macrophage-secreted interleukin-35 regulates cancer cell plasticity to facilitate metastatic colonization. Nat Commun. (2018) 9:3763. doi: 10.1038/s41467-018-06268-0
142. Zou JM, Qin J, Li YC, Wang Y, Li D, Shu Y, et al. IL-35 induces N2 phenotype of neutrophils to promote tumor growth. Oncotarget. (2017) 8:33501–14. doi: 10.18632/oncotarget.16819
143. Wang HM, Zhang XH, Feng MM, Qiao YJ, Ye LQ, Chen J, et al. Interleukin-35 suppresses the antitumor activity of t cells in patients with non-small cell lung cancer. Cell Physiol Biochem. (2018) 47:2407–19. doi: 10.1159/000491615
144. Yang L, Shao X, Jia S, Zhang Q, Jin Z. Interleukin-35 dampens CD8+ T cells activity in patients with non-viral hepatitis-related hepatocellular carcinoma. Front Immunol. (2019) 10:1032. doi: 10.3389/fimmu.2019.01032
145. Hao S, Chen X, Wang F, Shao Q, Liu J, Zhao H, et al. Breast cancer cell-derived IL-35 promotes tumor progression via induction of IL-35-producing induced regulatory T cells. Carcinogenesis. (2018) 39:1488–96. doi: 10.1093/carcin/bgy136
146. Turnis ME, Sawant DV, Szymczak-Workman AL, Andrews LP, Delgoffe GM, Yano H, et al. Interleukin-35 limits anti-tumor immunity. Immunity. (2016) 44:316–29. doi: 10.1016/j.immuni.2016.01.013
147. Huang C, Li N, Li Z, Chang A, Chen Y, Zhao T, et al. Tumour-derived Interleukin 35 promotes pancreatic ductal adenocarcinoma cell extravasation and metastasis by inducing ICAM1 expression. Nat Commun. (2017) 8:14035. doi: 10.1038/ncomms14035
148. Sun M, Zheng X, Meng Q, Dong Y, Zhang G, Rao D, et al. Interleukin-35 expression in non-small cell lung cancer is associated with tumor progression. Cell Physiol Biochem. (2018) 51:1839–51. doi: 10.1159/000495706
149. Chiba Y, Mizoguchi I, Furusawa J, Hasegawa H, Ohashi M, Xu M, et al. Interleukin-27 exerts its antitumor effects by promoting differentiation of hematopoietic stem cells to M1 macrophages. Cancer Res. (2018) 78:182–94. doi: 10.1158/0008-5472.CAN-17-0960
150. Waight JD, Netherby C, Hensen ML, Miller A, Hu Q, Liu S, et al. Myeloid-derived suppressor cell development is regulated by a STAT/IRF-8 axis. J Clin Invest. (2013) 123:4464–78. doi: 10.1172/JCI68189
151. Netherby CS, Messmer MN, Burkard-Mandel L, Colligan S, Miller A, Cortes Gomez E, et al. The granulocyte progenitor stage is a key target of IRF8-mediated regulation of myeloid-derived suppressor cell production. J Immunol. (2017) 198:4129–39. doi: 10.4049/jimmunol.1601722
152. Hellsten R, Lilljebjorn L, Johansson M, Leandersson K, Bjartell A. The STAT3 inhibitor galiellalactone inhibits the generation of MDSC-like monocytes by prostate cancer cells and decreases immunosuppressive and tumorigenic factors. Prostate. (2019). doi: 10.1002/pros.23885
153. Lee MH, Kachroo P, Pagano PC, Yanagawa J, Wang G, Walser TC, et al. Combination treatment with apricoxib and IL-27 enhances inhibition of epithelial-mesenchymal transition in human lung cancer cells through a STAT1 dominant pathway. J Cancer Sci Ther. (2014) 6:468–77. doi: 10.4172/1948-5956.1000310
154. Wei J, Xia S, Sun H, Zhang S, Wang J, Zhao H, et al. Critical role of dendritic cell-derived IL-27 in antitumor immunity through regulating the recruitment and activation of NK and NKT cells. J Immunol. (2013) 191:500–8. doi: 10.4049/jimmunol.1300328
155. Kumar V, Patel S, Tcyganov E, Gabrilovich DI. The nature of myeloid-derived suppressor cells in the tumor microenvironment. Trends Immunol. (2016) 37:208–20. doi: 10.1016/j.it.2016.01.004
156. Gordon SR, Maute RL, Dulken BW, Hutter G, George BM, McCracken MN, et al. PD-1 expression by tumour-associated macrophages inhibits phagocytosis and tumour immunity. Nature. (2017) 545:495–9. doi: 10.1038/nature22396
157. Jovanovic D, Roksandic Milenkovic M, Kotur Stevuljevic J, Markovic J, Ceriman V, Kontic M, et al. Membrane PD-L1 expression and soluble PD-L1 plasma levels in idiopathic pulmonary fibrosis-a pilot study. J Thorac Dis. (2018) 10:6660–9. doi: 10.21037/jtd.2018.11.16
158. Rolvering C, Zimmer AD, Kozar I, Hermanns HM, Letellier E, Vallar L, et al. Crosstalk between different family members: IL27 recapitulates IFN gamma responses in HCC cells, but is inhibited by IL6-type cytokines. Biochim Biophys Acta-Mol Cell Res. (2017) 1864:516–26. doi: 10.1016/j.bbamcr.2016.12.006
159. Farhood B, Najafi M, Mortezaee K. Cancer-associated fibroblasts: Secretions, interactions, and therapy. J Cell Biochem. (2019) 120:2791–800. doi: 10.1002/jcb.27703
160. Dong Z, Zhao X, Tai W, Lei W, Wang Y, Li Z, et al. IL-27 attenuates the TGF-beta1-induced proliferation, differentiation and collagen synthesis in lung fibroblasts. Life Sci. (2016) 146:24–33. doi: 10.1016/j.lfs.2016.01.004
161. Dong S, Zhang X, He Y, Xu F, Li D, Xu W, et al. Synergy of IL-27 and TNF-alpha in regulating CXCL10 expression in lung fibroblasts. Am J Respir Cell Mol Biol. (2013) 48:518–30. doi: 10.1165/rcmb.2012-0340OC
162. Fridlender ZG, Sun J, Kim S, Kapoor V, Cheng G, Ling L, et al. Polarization of tumor-associated neutrophil phenotype by TGF-beta: “N1” versus “N2” TAN. Cancer Cell. (2009) 16:183–94. doi: 10.1016/j.ccr.2009.06.017
163. Shaul ME, Levy L, Sun J, Mishalian I, Singhal S, Kapoor V, et al. Tumor-associated neutrophils display a distinct N1 profile following TGFbeta modulation: a transcriptomics analysis of pro- vs. antitumor TANs. Oncoimmunology. (2016) 5:e1232221. doi: 10.1080/2162402X.2016.1232221
164. Granot Z, Jablonska J. Distinct functions of neutrophil in cancer and its regulation. Mediators Inflamm. (2015) 2015:701067. doi: 10.1155/2015/701067
165. Bin Dhuban K, Bartolucci S, d'Hennezel E, Piccirillo CA. Signaling through gp130 compromises suppressive function in human FOXP3(+) regulatory T cells. Front Immunol. (2019) 10:1532. doi: 10.3389/fimmu.2019.01532
166. Morishima N, Mizoguchi I, Okumura M, Chiba Y, Xu M, Shimizu M, et al. A pivotal role for interleukin-27 in CD8+ T cell functions and generation of cytotoxic T lymphocytes. J Biomed Biotechnol. (2010) 2010:605483. doi: 10.1155/2010/605483
167. Felices M, Chu S, Kodal B, Bendzick L, Ryan C, Lenvik AJ, et al. IL-15 super-agonist (ALT-803) enhances natural killer (NK) cell function against ovarian cancer. Gynecol Oncol. (2017) 145:453–61. doi: 10.1016/j.ygyno.2017.02.028
168. Greppi M, Tabellini G, Patrizi O, Candiani S, Decensi A, Parolini S, et al. Strengthening the antitumor NK cell function for the treatment of ovarian cancer. Int J Mol Sci. (2019) 20:890. doi: 10.3390/ijms20040890
169. Choi YH, Lim EJ, Kim SW, Moon YW, Park KS, An HJ. IL-27 enhances IL-15/IL-18-mediated activation of human natural killer cells. J Immunother Cancer. (2019) 7:168. doi: 10.1186/s40425-019-0652-7
170. Sorrentino C, Yin Z, Ciummo S, Lanuti P, Lu LF, Marchisio M, et al. Targeting interleukin(IL)-30/IL-27p28 signaling in cancer stem-like cells and host environment synergistically inhibits prostate cancer growth and improves survival. J Immunother Cancer. (2019) 7:201. doi: 10.1186/s40425-019-0668-z
171. Hasegawa H, Mizoguchi I, Chiba Y, Ohashi M, Xu M, Yoshimoto T. Expanding diversity in molecular structures and functions of the IL-6/IL-12 heterodimeric cytokine family. Front Immunol. (2016) 7:479. doi: 10.3389/fimmu.2016.00479
172. Wang X, Wei Y, Xiao H, Liu X, Zhang Y, Han G, et al. A novel IL-23p19/Ebi3 (IL-39) cytokine mediates inflammation in Lupus-like mice. Eur J Immunol. (2016) 46:1343–50. doi: 10.1002/eji.201546095
173. Wang X, Zhang Y, Wang Z, Liu X, Zhu G, Han G, et al. AntiIL39 (IL23p19/Ebi3) polyclonal antibodies ameliorate autoimmune symptoms in lupuslike mice. Mol Med Rep. (2018) 17:1660–6. doi: 10.3892/mmr.2017.8048
174. Manning AA, Zhao L, Zhu Z, Xiao H, Redington CG, Ding VA, et al. IL-39 acts as a friend to pancreatic cancer. Med Oncol. (2018) 36:12. doi: 10.1007/s12032-018-1236-y
175. Wang RX, Yu CR, Mahdi RM, Egwuagu CE. Novel IL27p28/IL12p40 cytokine suppressed experimental autoimmune uveitis by inhibiting autoreactive Th1/Th17 cells and promoting expansion of regulatory T cells. J Biol Chem. (2012) 287:36012–21. doi: 10.1074/jbc.M112.390625
Keywords: cytokines, signaling, immunotherapy, tumor microenvironment, cancer
Citation: Kourko O, Seaver K, Odoardi N, Basta S and Gee K (2019) IL-27, IL-30, and IL-35: A Cytokine Triumvirate in Cancer. Front. Oncol. 9:969. doi: 10.3389/fonc.2019.00969
Received: 06 June 2019; Accepted: 12 September 2019;
Published: 01 October 2019.
Edited by:
Alexandr Bazhin, Hospital of the University of Munich, GermanyReviewed by:
Robin Parihar, Baylor College of Medicine, United StatesOnur Boyman, University of Zurich, Switzerland
Copyright © 2019 Kourko, Seaver, Odoardi, Basta and Gee. This is an open-access article distributed under the terms of the Creative Commons Attribution License (CC BY). The use, distribution or reproduction in other forums is permitted, provided the original author(s) and the copyright owner(s) are credited and that the original publication in this journal is cited, in accordance with accepted academic practice. No use, distribution or reproduction is permitted which does not comply with these terms.
*Correspondence: Katrina Gee, a2dlZUBxdWVlbnN1LmNh
†These authors have contributed equally to this work