- 1Department of Internal Medicine, São Paulo State University (UNESP-FMB), Botucatu, Brazil
- 2Department of Bioprocesses and Biotechnology, São Paulo State University (UNESP-FCA), Botucatu, Brazil
- 3Department of Structural and Functional Biology, São Paulo State University (UNESP-IBB), Botucatu, Brazil
Chronic myeloid leukemia (CML) results from a translocation between chromosomes 9 and 22, which generates the Philadelphia chromosome. This forms BCR/ABL1, an active tyrosine kinase protein that promotes cell growth and replication. Despite great progress in CML treatment in the form of tyrosine kinase inhibitors, allogeneic-hematopoietic stem cell transplantation (allo-HSCT) is currently used as an important treatment alternative for patients resistant to these inhibitors. Studies have shown that unregulated expression of microRNAs, which act as oncogenes or tumor suppressors, is associated with human cancers. This contributes to tumor formation and development by stimulating proliferation, angiogenesis, and invasion. Research has demonstrated the potential of microRNAs as biomarkers for cancer diagnosis, prognosis, and therapeutic targets. In the present study, we compared the circulating microRNA expression profiles of 14 newly diagnosed patients with chronic phase-CML and 14 Philadelphia chromosome-negative patients after allo-HSCT. For each patient, we tested 758 microRNAs by reverse transcription quantitative polymerase chain reaction (RT-qPCR) analysis. The global expression profile of microRNAs revealed 16 upregulated and 30 downregulated microRNAs. Target genes were analyzed, and key pathways were extracted and compared. Bioinformatics tools were used to analyze data. Among the downregulated miRNA target genes, some genes related to cell proliferation pathways were identified. These results reveal the comprehensive microRNA profile of CML patients and the main pathways related to the target genes of these miRNAs in cytogenetic remission after allo-HSCT. These results provide new resources for exploring stem cell transplantation-based CML treatment strategies.
Introduction
Chronic myeloid leukemia (CML) is a myeloproliferative disease accounting for ~20% of diagnosed adult cases (1, 2). CML was the first human malignant disease to be linked to a chromosomal abnormality. A translocation between chromosomes 9q34 and 22q11 generates the Philadelphia chromosome. This encodes the BCR/ABL1 oncoprotein, an active tyrosine kinase protein that is the main driver of CML pathogenesis (2, 3). There have been great developments and improvements in anticancer targeted therapy associated with CML (4). Imatinib was the first member of a class of small molecules that prevent tyrosine kinase activity to be developed, and acts by binding to the BCR-ABL1 protein. Tyrosine kinase inhibitors (TKIs) act upon the interaction between the BCR-ABL1 oncoprotein and ATP, blocking cellular proliferation of the malignant clone. However, ~2% of patients become resistant to TKIs. Allogeneic-hematopoietic stem cell transplantation (allo-HSCT) is the only curative treatment for CML and provides an important alternative for TKI-resistant or advanced phase CML patients (2). However, the mortality and morbidity of this method, as well as a lack of suitable donors, limits the application of allo-HSCT (5).
The natural course of CML begins in the chronic phase and progresses to the blast phase, passing through the accelerated phase. The transformation mechanisms involved with this process are varied and not yet fully understood. The interruption of differentiation, genomic instability, shortening of telomeres, and loss of tumor suppressor functions are among the steps of this transformation that have already been described (6, 7).
Recent advancements in gene expression profiling technology have demonstrated that microRNAs (miRNAs) are promising prognostic predictors of different types of cancers. miRNAs, which modulate post-transcriptional gene expression, are 18–25-nucleotide non-coding RNAs (8–10). They regulate gene expression and modify cancer processes such as differentiation, proliferation, and apoptosis. Previous studies have suggested that miRNAs play important roles in regulating angiogenesis and metastasis (11, 12). Additionally, miRNAs are very stable molecules in the blood, suggesting that they can be applied as molecular markers (13).
Bioinformatics is an important research approach that can be applied for understanding gene regulation pathways. Cancer bioinformatics is an emerging field that integrates knowledge from cancer and information technology. Integrating cancer research and bioinformatics is important for advancing the diagnosis, prognosis, and treatment of cancer (14). Additionally, bioinformatics analyses have contributed to the identification of candidate genes and miRNA-mRNA target pairs (15).
This study was conducted to determine the profiles of miRNAs and their target genes in CML patients treated with allo-HSCT. These profiles were then compared to those of a newly diagnosed and untreated patient group.
Patients and Methods
The study was previously approved by the Research Ethics Committee of the Dr. Amaral Carvalho Hospital, Jahu, SP, Brazil (CEPHAC-−2.917.389). The patients provided informed consent to participate in the study, in accordance with the Declaration of Helsinki.
Patients and Samples
A total of 28 patients diagnosed with chronic phase-CML and treated at the Dr. Amaral Carvalho Hospital were included in the study. The patients were separated into two groups: (1) 14 patients newly diagnosed with Philadelphia chromosome-positive (Ph+) CML who had not been treated with TKIs; and (2) 14 patients who achieved complete cytogenetic remission (Philadelphia chromosome-negative) post-allo-HSCT. Patient characteristics are described in Table 1. The present study used a leukocyte pool of 14 healthy blood donors as a control group. To determine the miRNA profiles of each patient group, the control group was compared to the patient groups to determine which miRNAs were upregulated or downregulated. All transplanted patients received BuCy-2 as a conditioning regimen and cyclosporine and methotrexate as graft-vs.-host disease prophylaxis (16, 17).
Table 1Patient clinical data.
Total RNA Isolation and RT-qPCR
Total RNA isolation was performed using a QIAamp® RNA Blood Mini Kit (QIAGEN, Hilden, Germany) with 5 mL peripheral blood. RNA integrity and quantity were evaluated by NanoDrop (Thermo Scientific, Waltham, MA, USA) and RNA 2100 Bioanalyzer (Agilent Technologies, Santa Clara, CA, USA) according to the manufacturer's instructions.
The total RNA was reverse-transcribed using a Taqman MicroRNA Reverse Transcription Kit, and a TaqManTM MGB probe was used for real-time qPCR according to the manufacturer's instructions. RT-qPCR and TaqMan® Low Density Array Human MicroRNA Arrays A v2.0 and B v3.0 (ABIV®, Life Technologies, Carlsbad, CA, USA) were performed on a ViiA7 platform (ABIV®) following the manufacturer's instructions. miRNAs were quantified using the comparative Ct-method (18). Each Human Pool Set contains 377 unique human miRNAs, three internal controls, and one negative control. A total of 758 miRNAs were analyzed.
Bioinformatic Analysis
The study followed the experimental procedure detailed in Figure 1. Expression Suite Software Version 1.1 Program was used to identify differentially expressed miRNAs. To identify possible differentially expressed miRNA targets, we conducted RT-qPCR using miRWalk 2.0, which includes target prediction data generated by different algorithms (including own algorithm) (19). The following algorithms were selected: miRWalk, miRDB, Micro T4, miRanda, RNAhybrid, and Targetscan. Only targets predicted by at least three of the selected algorithms were accepted. We then verified whether the predicted targets have been identified as being differentially expressed in CML using microarray data available from Gene Expression Omnibus (https://www.ncbi.nlm.nih.gov/geo), accession number GSE 43225. Microarray data were analyzed using the GEO2R script(https://www.ncbi.nlm.nih.gov/geo/geo2r/).
We considered genes to be differentially expressed when they showed a fold-change of at least 1.5. Gene Ontology (GO) (http://www.geneontology.org/) was used to search for enriched terms among differentially expressed genes, accepting only terms with P ≤ 0.05 and using Bonferroni's correction. Differentially expressed genes related to upregulated and downregulated miRNAs were analyzed according to EnrichR (https://amp.pharm.mssm.edu/Enrichr/) for enrichment analysis. Reactome was used for analysis. To assess the protein-protein interaction (PPI) network based on a list of genes, the online tool STRING Version 11.0 (https://string-db.org/) was utilized. Experiments, databases, co-expression data, neighborhood, and co-occurrence were considered active interaction sources. The minimum required interaction score was 0.700. Finally, we used Cytoscape Version 3.8.0 (http://www.cytoscape.org) software to visualize the enrichment results. Network nodes represent genes, while edges represent protein-protein associations.
Statistical Analysis
Comparative CT analysis was used to quantify miRNA gene expression. The differences were estimated by Student's t-test. Values of P < 0.05 were considered statistically significant.
Results
Differentially Expressed miRNAs
The miRNA expression data set is available in the NCBI GEO database (accession number GSE 148567). A total of 758 miRNAs were analyzed by RT-qPCR in peripheral blood samples from 14 newly diagnosed patients and untreated chronic phase CML patients, and 14 patients in cytogenetic remission after allo-HSCT. According to the cut-off criteria (fold-change ≤ 0.5 and fold-change ≥ 2.0), 46 differentially expressed miRNAs were identified. Sixteen (34.8%) miRNAs were upregulated, and thirty (65.2%) were downregulated (Table 2). Among them, miR-1260a was the most downregulated miRNA, whereas miR-409-3p was the most upregulated miRNA. The main functions of all differentially expressed miRNAs are listed in Table 3.
MiRNA Target Genes
Upregulated and downregulated miRNAs were analyzed in miRWalk to identify the miRNA target genes. Using microarray analysis, 1,069 genes were identified.
Gene Expression
We evaluated whether the identified target genes were previously differentially expressed in CML using available microarray data in the Gene Expression Omnibus. The microarray data were analyzed using GEO2R script. The identified genes were compared to our results. Of the 822 genes related to downregulated miRNA, 789 (96%) were also identified among the Gene Expression Omnibus microarray data genes. Among the genes associated with upregulated miRNAs, 247 genes were found in the patients included in the experiment, and 234 (95%) were found in the data searched.
Pathway Enrichment Analysis and PPI Network Construction
GO analysis (including Molecular Function, Biological Process and Cellular Component) was performed on 1,069 target genes. A total of 461 results were obtained from GO analysis. The top 30 terms are shown in Figure 2.
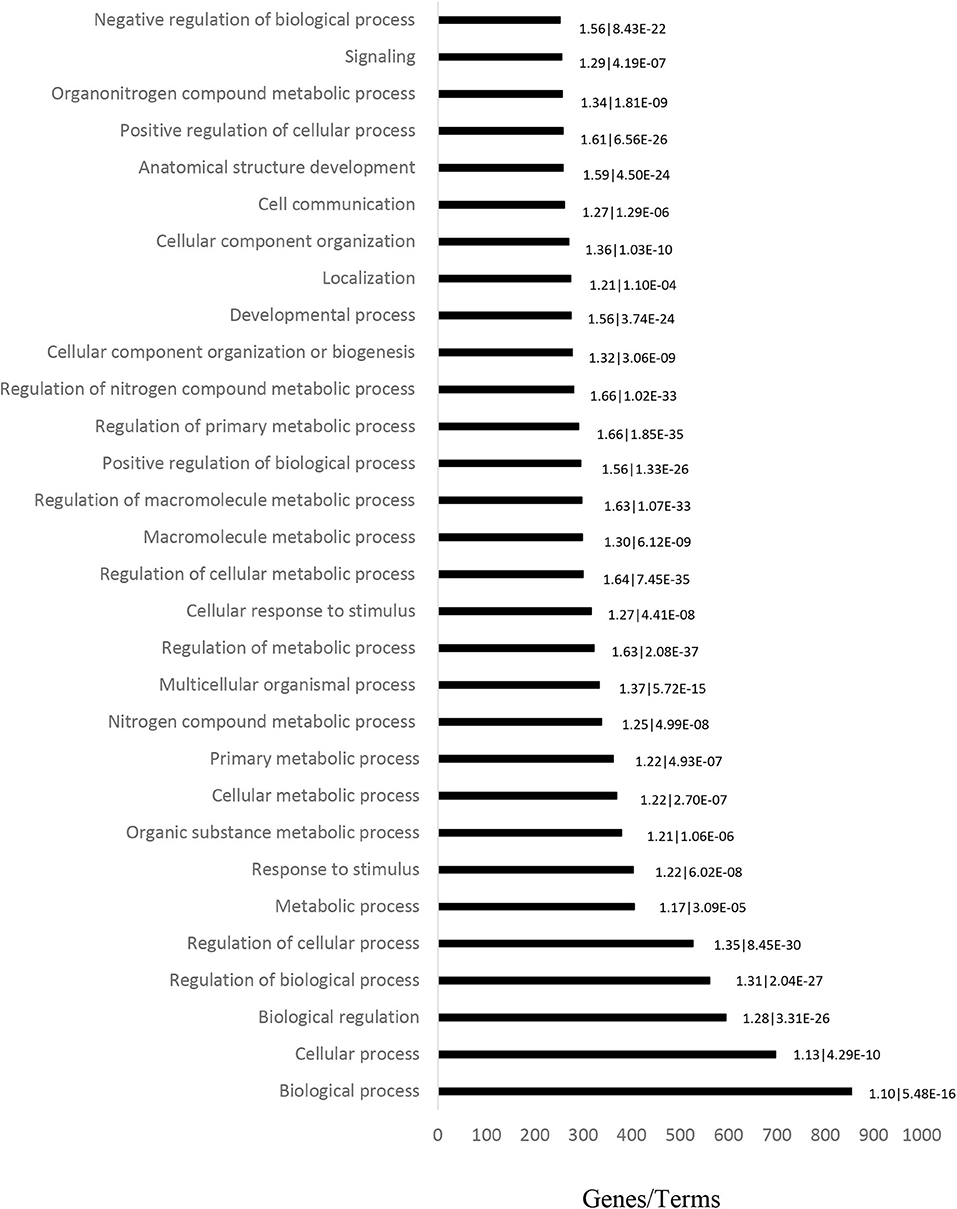
Figure 2. Ontology analysis of differentially expressed genes in CML patient blood samples, performed to determine the main altered pathways. Horizontal bars represent the number of genes found in each term. The fold-enrichment and P-values are displayed on the sides of the horizontal bars, and are separated by a vertical line.
EnrichR is a free web-based gene signature search tool. It was used to evaluate the 247 target genes of upregulated miRNAs, among which 573 terms were identified. Among the 822 target genes of downregulated miRNAs, 1,017 terms were found. EnrichR provides a visualization summary of the pathways based on a collective gene function list. The free pathway database tool Reactome is available for online use, and provides biological interpretation and visualization models for network analysis. STRING analysis was performed on the target genes of upregulated miRNAs (Figure 3) and downregulated miRNAs (Figure 4). The results were visualized using Cytoscape.
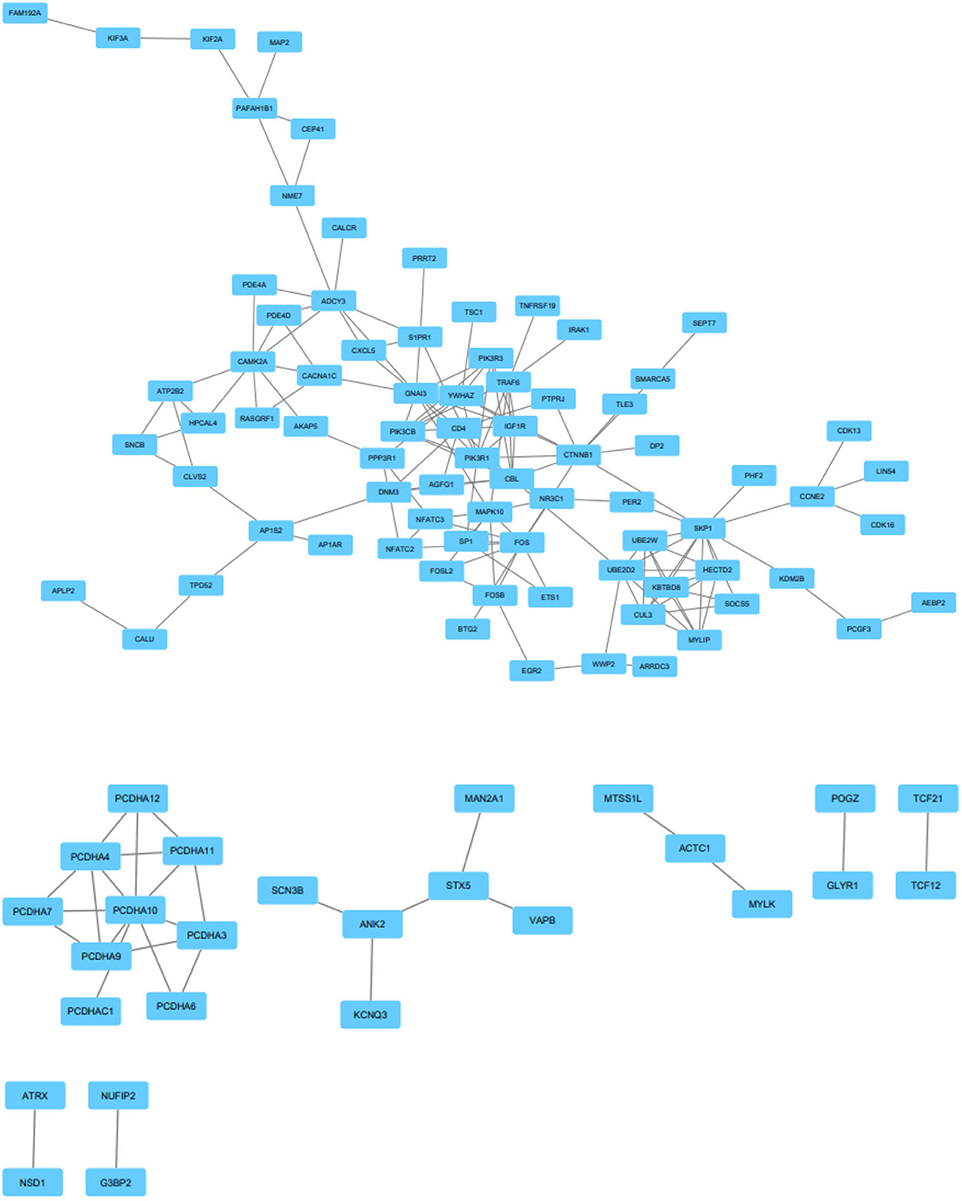
Figure 3. Protein-protein interaction network analysis of the target genes of upregulated miRNAs. Gene interactions were constructed using the STRING online database and Cytoscape. Network nodes represent genes, and the edges represent protein-protein associations.
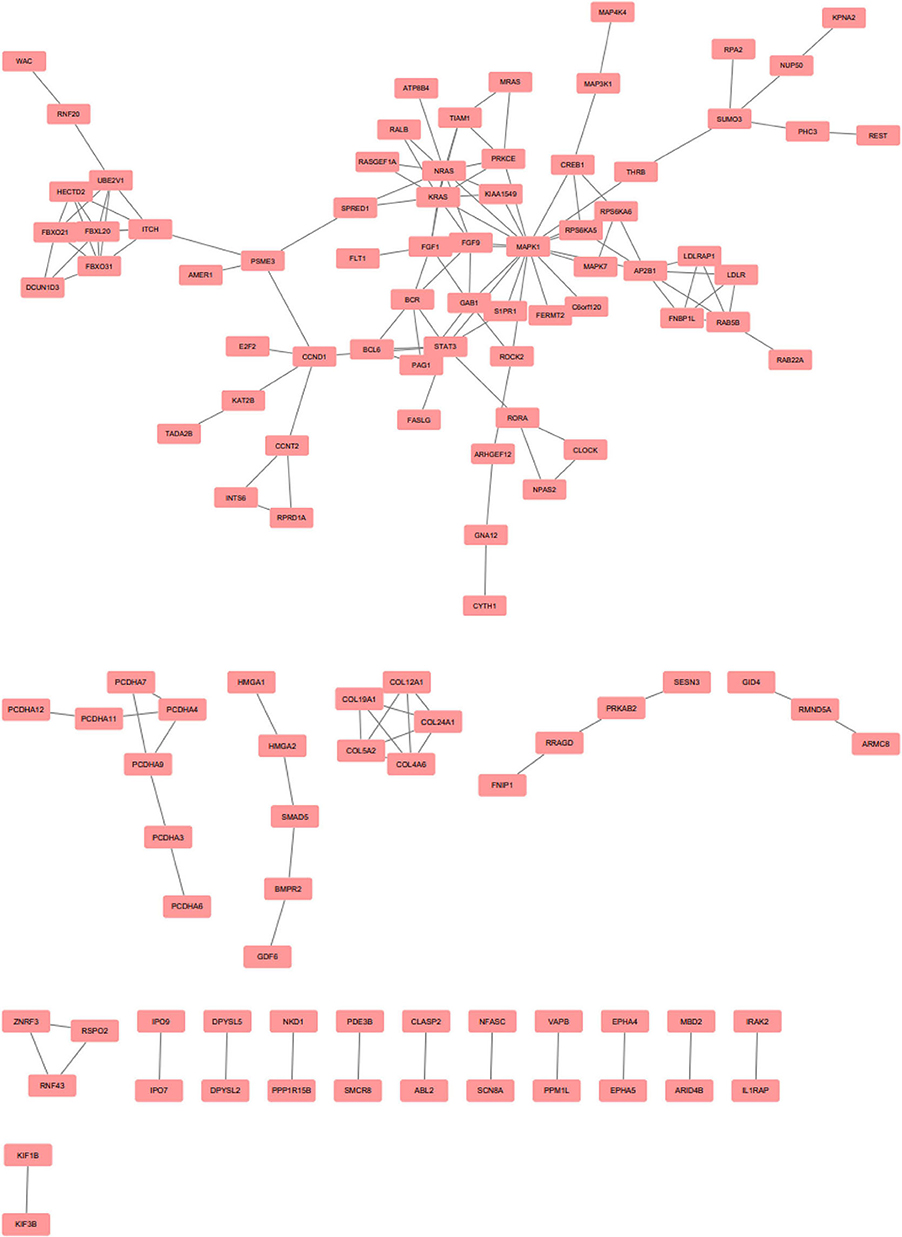
Figure 4. Protein-protein interaction network analysis of the target genes of downregulated microRNAs. Gene interactions were constructed using the STRING online database and Cytoscape. Network nodes represent genes, and the edges represent protein-protein associations.
Discussion
Differentially Expressed miRNAs
MiRNAs can act as oncogenes or tumor suppressor genes in CML, contributing to the pathogenesis, disease progression, and therapeutic responses (1, 52). Following the advent of TKIs as specific target drugs, hematopoietic stem cell transplantation began to play an important role in treating CML patients that are in the disease phase or are resistant to TKIs. This study investigated the miRNA profile of a group of 14 CML patients treated with allo-HSCT, who were in complete cytogenetic remission at the time of sample collection. Among the evaluated group, five patients underwent transplantation due to disease progression (patients 1, 3, 7, 10, and 14) and four others underwent transplantation due to a failed therapeutic response (patients 2, 5, 8, and 9). These comprised a total of 64.3% of the evaluated patients.
miR-10a
MiR-10a has been extensively studied due to its potential as a CML marker. Flamant et al. suggested the relevance of miR-10a as a drug response biomarker. By means of microarray analysis, a significant increase in miR-10a was observed in patient samples 2 weeks post-imatinib treatment (34). miR-10a downregulation was also detected in the transplanted patient group in the present study.
miR-17/92 Cluster
The miR-17/92 cluster is another potential biomarker for CML progression, and may be detected from the chronic phase to the blastic phase. This cluster is comprised of miR-17, miR18a, miR-19a, miR19b-1, miR20a, and miR92a-1 (32). In our study, a downregulation in the expression of miR-18a and miR-19a was observed, both of which are present in this cluster.
miR-328 and miR150
miR-328 and miR-150 are also related to disease progression (32, 48, 54, 64). Eiring et al. (48) have previously demonstrated the loss of miR-328 in blastic crisis CD34+ cells, which did not otherwise occur in chronic phase CD34+ myeloid cells. Poláková et al. (52) detected a negative correlation between the levels of BCR-ABL transcript and miR-150, further substantiating previous findings by Agirre et al. (64) and Fallah et al. (54) suggested that the downregulation of miR-150 is a potential diagnostic marker of CML. RT-PCR was performed for 50 samples from patients newly diagnosed with CML, revealing a downregulation in miR-150. In our study, both miRNAs were found to be upregulated, suggesting that miR-328 and miR-150 are useful as molecular biomarkers of the treatment response.
miR-486
The results of previous studies have also suggested miR-486 as an effective treatment response marker. Wang et al. compared miRNA expression in normal human CD34+ cells with that in CML CD34+ cells, using human leukemia cell lines and CD34+ cells isolated from chronic phase CML patients who had not been treated with imatinib. The results showed that miR-486 was overexpressed in CML cells, especially in megakaryocytes and erythroid progenitor cells (36). In our study, miR-486 was downregulated, further suggesting the potential of this miRNA to act as a biomarker of the treatment response.
miR-181c and miR-30
According to studies carried out in the K562 cell line, miR-181c, and miR-30 downregulation is related to imatinib resistance (30, 31). Yu et al. demonstrated that the downregulation of miR-30 in this CML cell line was related to the autophagy process and imatinib resistance mechanisms (30, 31). These results were reflected in the current study, with our findings demonstrating that miR-181c and miR-30 were downregulated.
Let-7g and miR-16
Let-7a and miR-16 also have important functions in myeloid leukemogenesis, such as regulating the cell cycle and apoptosis. Let-7a regulates oncogenes such as RAS and HMGA, while Mir-16 targets MCL1, BCL2, WNT3A, and CCNDI (35). Let-7a is also a member of the let-7 family, and it has been found that it may suppress CML via CRKL (20). Zuo et al. (35) examined the plasma levels of miR-16 and let-7a in a group of 50 patients with myelodysplastic syndrome (MDS). After comparing these results with those from a group of 76 healthy donors, it was found that both miRNAs were significantly lower in MDS patients than in healthy controls. In the present study, we found that let-7g and miR-16 were downregulated.
Pathway Enrichment Analysis and PPI Network Construction
In the analysis conducted using STRING and EnrichR, it was possible to determine which pathways were enriched by observing the target genes of unregulated miRNAs. Through our analysis, we found that MAPK, NRAS, KRAS, and ROCK had important functions related to CML regulation pathways.
The mitogen-activated protein kinase (MAPK) pathway is an important signaling cascade in several types of cancer, including CML (65). This pathway controls fundamental cellular processes such as cell proliferation, migration, growth, differentiation, and death (66). The MAPK pathway therefore plays a fundamental role in cell growth and survival, and irregularities in this pathway can lead to cells developing cancerous properties. This may include exacerbated cell proliferation, metastasis, and evasion of apoptosis (67).
RAS proteins are involved in signal transduction, and are mutated in different types of human cancer. The RAS family comprises three genes associated with carcinogenesis: HRAS, KRAS, and NRAS (68). These three genes encode a protein located on the inner cell membrane, which has GTPase activity. This protein participates in extracellular signal transduction into the cell, and the signal is transmitted by a cascade of kinases. As a result, MAPK is activated, which then activates transcription factors. Mutations in the RAS genes in human cancer inhibit GTP hydrolysis, and mutated RAS proteins remain in their GTP-linked active form. This, leads to disordered cell proliferation (69). KRAS and NRAS mutations are frequently found in myeloid disorders, including CML (70).
ROCK proteins perform different functions in cells. They are involved in organization of the actin cytoskeleton, human tumor pathogenesis, and in the signaling pathways that lead to cell proliferation. The RAS and ROCK pathways are interconnected, with RAS activating PI3K, which then activates the ROCK pathway. This interaction network can lead to continuous cell proliferation and survival (68).
The MAPK, RAS, and ROCK genes had increased expression in the patient group of our study. As these genes are involved in cell proliferation during leukemogenesis, it can be concluded that these genes play an important role in post-transplant evolution. Nine of the 14 patients (64.3%) had leukemia relapse, and the other five (35.7%) did not achieve a deep molecular response (BCR-ABL transcript level ≤ 0.01%).
This study explored miRNAs in CML patients and their target genes, and analyzed the pathways involved. Our study was based on original and clinical samples. However, as there were scarce samples available and these were insufficient to validate the 46 altered miRNAS, the study was limited by this factor. The bioinformatics analyses predicted interactions that require further biological validation before their therapeutic application.
Despite the small sample size, these findings showed that aggressive therapy such as transplantation did not alter the disease course in these group of CML patients. Furthermore, the demonstrated pattern of gene expression is suggestive of disease progression.
Data Availability Statement
All datasets generated for this study are included in the article/Supplementary Material.
Ethics Statement
This study was reviewed and approved by Research Ethics Committee of Dr. Amaral Carvalho Hospital–Jahu-SP- Brazil (CEPHAC—2.917.389). The patients/participants provided their written informed consent to participate in this study.
Author Contributions
PH, NH, and CN designed and coordinated the study and prepared the manuscript. JM, LM, and SC performed bioinformatics analysis and prepared the manuscript. JD and JC collected and analyzed the data. RC offered laboratory support and helped with discussions about the study. All authors critically reviewed and approved the final version of the manuscript.
Funding
This study received financial support by São Paulo Research Foundation (FAPESP 2011/50629-7).
Conflict of Interest
The authors declare that the research was conducted in the absence of any commercial or financial relationships that could be construed as a potential conflict of interest.
The handling editor declared a shared affiliation, though no other research collaboration, with the authors.
Supplementary Material
The Supplementary Material for this article can be found online at: https://www.frontiersin.org/articles/10.3389/fonc.2020.01544/full#supplementary-material
References
1. Wang K, Xu Z, Wang N, Tian Y, Sun X, Ma Y. Analysis of microRNA and gene networks in human chronic myelogenous leukemia. Mol Med Rep. (2016) 13:453–60. doi: 10.3892/mmr.2015.4502
2. Jabbour E, Kantarjian H. Chronic myeloid leukemia: 2018 update on diagnosis, therapy and monitoring. Am J Hematol. (2018) 93:442–59. doi: 10.1002/ajh.25011
3. Spagnuolo M, Regazzo G, De Dominici M, Sacconi A, Pelosi A, Korita E, et al. Transcriptional activation of the miR-17-92 cluster is involved in the growth-promoting effects of MYB in human Ph-positive leukemia cells. Haematologica. (2019) 104:82–92. doi: 10.3324/haematol.2018.191213
4. Baccarani M, Pane F. A critical history of chronic myeloid leukemia. Mediterr J Hematol Infect Dis. (2014) 6:1 doi: 10.4084/MJHID.2014.010
5. Goldman JM. Chronic myeloid leukemia: a historical perspective. Semin Hematology. (2010) 47:302–11. doi: 10.1053/j.seminhematol.2010.07.001
6. Garcia-Manero G, Faderl S, O'Brien S, Cortes J, Talpaz M, Kantarjian HM. Chronic myelogenous leucemia: a review and update of therapeutic strategies. Cancer. (2003) 98:437–57. doi: 10.1002/cncr.11520
7. Chakraborty C, Sharma AR, Patra BC, Bhattacharya M, Sharma G, Lee SS. MicroRNAs mediated regulation of MAPK signaling pathways in chronic myeloid leukemia. Oncotarget. (2016) 7:42683–97. doi: 10.18632/oncotarget.7977
8. Liang L, Wei DM, Li JJ, Luo DZ, Chen G, Dang YW, et al. Prognostic microRNAs and their potential molecular mechanism in pancreatic cancer: a study based on The cancer genome atlas and bioinformatics investigation. Mol Med Rep. (2018) 17:939–51. doi: 10.3892/mmr.2017.7945
9. Keklikoglou I, Koerner C, Schmidt C, Zhang JD, Heckmann D, Shavinskaya A, et al. MicroRNA-520/373 family functions as a tumor suppressor in estrogen receptor negative breast cancer by targeting NF-κB and TGF-β signaling pathways. Oncogene. (2012) 31:4150–63. doi: 10.1038/onc.2011.571
10. Cao Z, Moore BT, Wang Y, Peng XH, Lappe JM, Recker RR, et al. MiR-422a as a potential cellular microRNA biomarker for postmenopausal osteoporosis. PLoS ONE. (2014) 9:e97098 doi: 10.1371/jornal.pone.0097098
11. Guessous F, Velez MA, Marcinkiewicz L, Zhang Y, Kim J, Heister S, et al. Oncogenic effects of miR-10b in glioblastoma stem cells. J Neurooncol. (2013) 112:153–63. doi: 10.1007/s11060-013-1047-0
12. Garofalo M, Croce CM. Role of microRNAs in mantaining cancer stem cells. Adv Drug Deliv Rev. (2015) 81:53–61. doi: 10.1016/j.addr.2014.11.014
13. Li A, Yu J, Kim H, Wolfgang CL, Canto MI, Hruban RH, et al. MicroRNA array analysis finds elevated serum miR-1290 accurately distinguishes patients with low-stage pancreatic cancer from healthy and disease controls. Clin Cancer Res. (2013) 19:3600–10. doi: 10.1158/1078-0432.CCR-12-3092
14. Banwait JK, Bastola DR. Contribution of bioinformatics prediction in microRNA-based cancer therapeutics. Adv Drug Deliv Rev. (2015) 81:94–103. doi: 10.1016/j.addr.2014.10.030
15. Gao C, Zhou C, Zhuang J, Liu L, Wei J, Liu C, et al. Identification of key candidate genes and miRNA-mRNA target pairs in chronic lymphocytic leukemia by integrated bioinformatics analysis. Mol Med Rep. (2019) 19:362–74. doi: 10.3892/mmr.2018.9636
16. Tutscha PJ, Copelan EA, Klein JP. Bone marrow transplantation for leukemia following a new busulfan and cyclosphosphamide regimen. Blood. (1987) 70:1382–8.
17. Storb R, Deeg HJ, Whitehead J, Appelbaum F, Beatty P, Bensinger W, et al. Methotrexate and cyclosporine alone for prophylaxis of acute graft versus host disease after marrow transplantation for leukemia. N Engl J Med. (1986) 314:729–35. doi: 10.1056/NEJM198603203141201
18. Schmittgen T, Livak K. Analyzing real-time PCR data by the comparative CT method. Nat Protoc. (2008) 3:1101–8. doi: 10.1038/nprot.2008.73
19. Dweep H, Gretz N. MiRWalk2.0: a comprehensive atlas of microRNA-target interactions. Nat Methods. (2015) 12:697. doi: 10.1038/nmeth.3485
20. Rokah OH, Geva P, Divon MS, Shpilberg O, Aronov SL. Network analysis of microRNAs, genes and their regulation in diffuse and follicular B-cell lymphomas. Oncotarget. (2018) 9:7928–41. doi: 10.18632/oncotarget.23974
21. Li X, Xu M, Ding L, Tang J. miR-27a: A novel biomarker and potential therapeutic target in tumors. J Cancer. (2019) 10:2836–48. doi: 10.7150/jca.31361
22. Song B, Wang Y, Xi Y, Kudo K, Bruheim S, Botchkina G I, et al. Mechanism of chemoresistance mediated by miR-140 in human osteosarcoma and colon cancer cells. Oncogene. (2009) 28:4065–74. doi: 10.1038/onc.2009.274
23. Xia N, Tan WF, Peng QZ, Lai HN. MiR-374b reduces cell proliferation and cell invasion of cervical cancer through regulating FOXM1. Eur Rev Med Pharmacol Sci . (2019) 23:513–21. doi: 10.26355/eurrev_201901_16863
24. Takai T, Tsujino T, Yoshikawa Y, Inamoto T, Sugito N, Kuranaga Y. Synthetic miR-143 exhibited an anti-cancer effect via the downregulation of K-RAS networks of renal cell cancer cells in vitro and in vivo. Mol Ther. (2019) 27:1017–27. doi: 10.1016/j.ymthe.2019.03.004
25. Zhao L, Li Y, Song X, Zhou H, Li N, Miao Y, et al. Upregulation of miR-181c inhibits chemoresistance by targeting ST8SIA4 in chronic myelocytic leukemia. Oncotarget. (2016) 7:60074–86. doi: 10.18632/oncotarget.11054
26. Verghese ET, Drury R, Green CA, Holliday DL, Lu X, Nash C. MiR-26b is down-regulated in carcinoma-associated fibroblasts from ER-positive breast cancers leading to enhanced cell migration and invasion. J Pathol. (2013) 231:388–99. doi: 10.1002/path.4248
27. Wu X, Chen H, Zhang G, Wu J, Zhu W, Gu Y, et al. MiR-212-3p inhibits cell proliferation and promotes apoptosis by targeting nuclear factor IA in bladder cancer. J Biosci. (2019) 44:80. doi: 10.1007/s12038-019-9903-5
28. Fiserova B, Kubiczkova L, Sedlarikova L, Hajek R, Sevcikova S. The miR-29 family in hematological malignancies. Biomed Pap Med Fac Univ Palacky Olomouc Czech Repub. (2015) 159:184–91. doi: 10.5507/bp.2014.037
29. Wen L, Cheng F, Zhou Y, Yin C. MiR-26a enhances the sensitivity of gastric cancer cells to cisplatin by targeting NRAS and E2F2. Saudi J Gastroenterol. (2015) 21:313–9. doi: 10.4103/1319-3767.166206
30. Zimmerman EI, Dollins CM, Crawford M, Grant S, Sinkam SPN, Richards KL, et al. Lyn kinase-dependent regulation of miR181 and myeloid cell leukemia-1 expression: Implications for drug resistance in myelogenous leukemia. Mol Pharmacol. (2010) 78:811–7. doi: 10.1124/mol.110.066258
31. Yu Y, Yang L, Zhao M, Zhu S, Kang R, Vernon P, et al. Targeting microRNA-30a-mediated autophagy enhances imatinib activity against human chronic myeloid leukemia cells. Leukemia. (2012) 26:1752–60. doi: 10.1038/leu.2012.65
32. Venturini L, Battmer K, Castoldi M, Schultheis B, Hochhaus A, Muckenthaler MU, et al. Expression of the miR-17-92 polycistron in chronic myeloid leukemia (CML) CD34+ cells. Blood. (2007) 109:4399–405. doi: 10.1182/blood-2006-09-045104
33. Song B, Yan J, Liu C, Zhou H, Zheng Y. Tumor suppressor role of miR-363-3p in gastric cancer. Med Sci Monit. (2015) 21:4074–80. doi: 10.12659/MSM.896556
34. Flamant S, Ritchie W, Guilhot J, Holst J, Bonnet ML, Chomel JC, et al. Micro-RNA response to imatinib mesylate in patients with chronic myeloid leukemia. Haematologica. (2010) 95:1325–33. doi: 10.3324/haematol.2009.020636
35. Zuo Z, Calin GA, de Paula HM, Medeiros LJ, Fernandez MH, Shimizu M, et al. Circulating microRNAs let-7a and miR-16 predict progression-free survival and overall survival in patients with myelodysplastic syndrome. Blood. (2011) 118:413–5. doi: 10.1182/blood-2011-01-330704
36. Wang LS, Li L, Li L, Chu S, Shiang KD, Li M, et al. MicroRNA-486 regulates normal erythropoiesis and enhances growth and modulates drug response in CML progenitors. Blood. (2015) 125:1302–13. doi: 10.1182/blood-2014-06-581926
37. Mou T, Xie F, Zhong P, Hua H, Lai L, Yang Q, et al. MiR-345-5p functions as a tumor suppressor in pancreatic cancer by directly targeting CCL8. Biomed Pharmacother. (2019) 111:891–900. doi: 10.1016/j.biopha.2018.12.121
38. Hannafon BN, Cai A, Calloway CL, Xu YF, Zhang R, Fung KM, et al. miR-23b and miR-27b are oncogenic microRNAs in breast cancer: evidence from a CRISPR/Cas9 deletion study. BMC Cancer. (2019) 19:642. doi: 10.1186/s12885-019-5839-2
39. Ji R, Zhang X, Gu H, Ma J, Wen X, Zhou J, et al. miR-374a-5p: A new target for diagnosis and drug resistance therapy in gastric cancer. Mol Ther Nucleic Acids. (2019) 18:320–31. doi: 10.1016/j.omtn.2019.07.025
40. Xia J T, Chen L Z, Jian W H, Wang K B, Yang Y Z, He W L. MicroRNA-362 induces cell proliferation and apoptosis resistance in gastric cancer by activation of NF-κB signaling. J Transl Med. (2014) 12:33. doi: 10.1186/1479-5876-12-33
41. Chadrashekar K, Jayaram S. Role of let-7 family microRNA in breast cancer. Non-Cod RNA Res. (2016) 1:77–82. doi: 10.1016/j.ncrna.2016.10.003
42. Sun GL, Li Z, Wang WZ, Chen Z, Zhang L, Li Q. miR-324-3p promotes gastric cancer development by activating Smad4-mediated Wnt/beta-catenin signaling pathway. J Gastroenterol. (2018) 53:725–39. doi: 10.1007/s00535-017-1408-0
43. Choe MH, Yoon Y, Kim J, Hwang SG, Han YH, Kim JS. miR-550a-3-5p acts as a tumor suppressor and reverses BRAF inhibitor resistance through the direct targeting of YAP. Cell Death Dis. (2018) 9:640. doi: 10.1038/s41419-018-0698-3
44. Pan L, Zhou L, Yin W, Bai J, Liu R. miR-125a induces apoptosis, metabolism disorder and migration impairment in pancreatic cancer cells by targeting Mfn2-related mitochondrial fission. Int J Oncol. (2018) 53:124–136. doi: 10.3892/ijo.2018.4380
45. Ni S, Weng W, Xu M, Wang Q, Ian C, Sun H. miR-106b-5p inhibits the invasion and metastasis of colorectal cancer by targeting CTSA. Onco Targets Ther. (2018) 11:3835–45. doi: 10.2147/OTT.S172887
46. Xue J, Yang M, Hua L-H, Wang Z-P. MiRNA-191 functions as an oncogene in primary glioblastoma by directly targeting NDST1. Eur Rev Med Pharmacol Sci. (2019) 23:6242–9. doi: 10.26355/eurrev_201907_18443
47. Ji WB, Liu X, Luo Y, Zhang WZ. High expression of miR-15b predicts poor prognosis for hepatocellular carcinoma after curative hepatectomy. Oncol Rep. (2016) 36:1901–8. doi: 10.3892/or.2016.4982
48. Eiring AM, Harb JG, Neviani P, Garton C, Oaks JJ, Spizzo R, et al. MiR-328 functions as an RNA decoy to modulate hnRNP E2 regulation of mRNA translation in leukemic blasts. Cell. (2010) 140:652–65. doi: 10.1016/j.cell.2010.01.007
49. Garofalo M, Quintavalle C, Romano G, Croce CM, Condorelli G. miR221/222 in cancer: their role in tumor progression and response to therapy. Curr Mol Med. (2012) 12:27–33. doi: 10.2174/156652412798376170
50. Zhang H, Jiang L, Sun D, Li J, Tang J. MiR-139-5p: promising biomarker for cancer. Tumor Biol. (2015) 36:1355–65. doi: 10.1007/s13277-015-3199-3
51. Li M, Guan X, Sun Y, Mi J, Shu X, Liu F, et al. miR-92a family and their target genes in tumorigenesis and metastasis. Exp Cell Res. (2014) 323:1–6. doi: 10.1016/j.yexcr.2013.12.025
52. Poláková KM, Lopotová T, Klamová H, Burda P, Trněný M, Stopka T, et al. Expression patterns of micro-RNAs associated with CML phases and their disease related targets. Mol Cancer. (2011) 10:41. doi: 10.1186/1476-4598-10-41
53. Chen L, Jiang X, Chen H, Han Q, Liu C, Sun M. microRNA-628 inhibits the proliferation of acute myeloid leukemia cells by directly targeting IGF-1R. Onco Targets Ther. (2019) 12:907–19. doi: 10.2147/OTT.S192137
54. Fallah P, Amirizadeh N, Poopak B, Toogeh G, Arefian E, Kohram F, et al. Expression pattern of key microRNAs in patients with newly diagnosed chronic myeloid leukemia in chronic phase. Int J Lab Hematol. (2015) 37:560–8. doi: 10.1111/ijlh.12351
55. Zheng J, Zhou Y, Li XJ, Hu JM. MiR-574-3p exerts as a tumor suppressor in ovarian cancer through inhibiting MMP3 expression. Eur Rev Med Pharmacol Sci. (2019) 23:6839–48. doi: 10.26355/eurrev_201908_18723
56. Zearo S, Kim E, Zhu Y, Zhao JT, Sidhu SB, Robinson BG, et al. MicroRNA-484 is more highly expressed in serum of early breast cancer patients compared to healthy volunteers. BMC Cancer. (2014) 14:200. doi: 10.1186/1471-2407-14-200
57. Guo LH, Li H, Wang F, Yu J, He JS. The tumor suppressor roles of miR-433 and miR-127 in gastric cancer. Int J Mol Sci. (2013) 14:14171–84. doi: 10.3390/ijms140714171
58. Labbaye C, Testa U. The emerging role of MIR-146A in the control of hematopoiesis, immune function and cancer. J Hematol Oncol. (2012) 5:13. doi: 10.1186/1756-8722-5-13
59. Chen J, Gao S, Wang C, Wang Z, Zhang H, Huang K, et al. Pathologically decreased expression of miR-193a contributes to metastasis by targeting WT1-E-cadherin axis in non-small cell lung cancers. J Exp Clin Cancer Res. (2016) 35:173. doi: 10.1186/s13046-016-0450-8
60. Wang H, He H, Yang C. miR-342 suppresses the proliferation and invasion of acute myeloid leukemia by targeting Naa10p. Artif Cell Nanomed Biotechnol. (2019) 47:3671–6. doi: 10.1080/21691401.2019.1596930
61. Ravichandran K, Sekar D, Subramanian G, Devanathan S. Role of microRNA-7-1-3p in prostate cancer progression. HIV Adv Res Dev. (2014) 1:1–4. doi: 10.14437/HARDOA-1-103
62. Pan JY, Zhang F, Sun CC, Li SJ, Li G, Gong FY, et al. miR-134: A human cancer suppressor? Mol Ther Nucl Acids. (2017) 6:140–9. doi: 10.1016/j.omtn.2016.11.003
63. Zhang C, Wang B, Wu L. MicroRNA-409 may function as a tumor suppressor in endometrial carcinoma cells by targeting Smad2. Mol Med Rep. (2019) 19:622–8. doi: 10.3892/mmr.2018.9642
64. Agirre X, Velasco AJ, Enériz ESJ, Garate L, Bandrés E, Cordeu L, et al. Down-regulation of hsa-miR-10a in chronic myeloid leukemia CD34+ cells increases USF2-mediated cell growth. Mol Cancer Res. (2008) 6:1830–40. doi: 10.1158/1541-7786.MCR-08-0167
65. Koul HK, Pal M, Koul S. Role of p38 MAP kinase signal transduction in solid tumors. Genes Cancer. (2013) 4:342–59. doi: 10.1177/1947601913507951
66. Meister M, Tomasovic A, Banning A, Tikkanen R. Mitogen-activated protein (MAP) kinase scaffolding proteins: a recount. Int J Mol Sci. (2013) 14:4854–84. doi: 10.3390/ijms14034854
67. Dhillon AS, Hagan S, Rath O, Kolch W. MAP kinase signalling pathways in cancer. Oncogene. (2007) 26:3279–90. doi: 10.1038/sj.onc.1210421
68. Rocca S, Carrà G, Poggio P, Morotti A, Brancaccio M. Targeting few to help hundreds: JAK, MAPK and ROCK pathways as druggable targets in atypical chronic myeloid leukemia. Mol Cancer. (2018) 17:40. doi: 10.1186/s12943-018-0774-4
69. Zhang F, Cheong JK. The renewed battle against RAS-mutant cancers. Cell Mol Life Sci. (2016) 73:1845–58. doi: 10.1007/s00018-016-2155-8
Keywords: miRNAs, chronic myeloid leukemia, allogeneic hematopoietic stem cell transplantation, biomarkers, Philadelphia chromosome
Citation: Martins JRB, Moraes LN, Cury SS, Dadalto J, Capannacci J, Carvalho RF, Nogueira CR, Hokama NK and Hokama POM (2020) Comparison of microRNA Expression Profile in Chronic Myeloid Leukemia Patients Newly Diagnosed and Treated by Allogeneic Hematopoietic Stem Cell Transplantation. Front. Oncol. 10:1544. doi: 10.3389/fonc.2020.01544
Received: 13 July 2019; Accepted: 20 July 2020;
Published: 04 September 2020.
Edited by:
Deilson Elgui De Oliveira, São Paulo State University, BrazilReviewed by:
Mohammad Sarwar Jamal, King Abdulaziz University, Saudi ArabiaClement M. Lee, Icahn School of Medicine at Mount Sinai, United States
Copyright © 2020 Martins, Moraes, Cury, Dadalto, Capannacci, Carvalho, Nogueira, Hokama and Hokama. This is an open-access article distributed under the terms of the Creative Commons Attribution License (CC BY). The use, distribution or reproduction in other forums is permitted, provided the original author(s) and the copyright owner(s) are credited and that the original publication in this journal is cited, in accordance with accepted academic practice. No use, distribution or reproduction is permitted which does not comply with these terms.
*Correspondence: Paula de Oliveira Montandon Hokama, paula.hokama@unesp.br