- 1Laboratory of Cellular and Molecular Radiation Oncology, Radiation Oncology Institute of Enze Medical Health Academy, Affiliated Taizhou Hospital of Wenzhou Medical University, Taizhou, China
- 2Department of Radiation Oncology, Affiliated Taizhou Hospital of Wenzhou Medical University, Taizhou, China
- 3Department of Radiation Medicine, University of Kentucky, Lexington, KY, United States
- 4Department of Clinical Oncology, Hong Kong University Shenzhen Hospital and Queen Mary Hospital, Hong Kong University Li Ka Shing Medical School, Hong Kong, China
- 5Department of Radiation Oncology, University Hospitals/Seidman Cancer Center and Case Comprehensive Cancer Center, Case Western Reserve University, Cleveland, OH, United States
Purpose: Although intensity-modulated radiotherapy (IMRT) is now a preferred option for conventionally fractionated RT in lung cancer, the commonly used cutoff values of the dosimetric constraints are still mainly derived from the data using three-dimensional conformal radiotherapy (3D-CRT). We aimed to compare the prediction performance among different dosimetric parameters for acute radiation pneumonitis (RP) in patients with lung cancer received IMRT.
Methods: A total of 236 patients treated with IMRT were retrospectively reviewed in two independent groups of lung cancer from January 2014 to August 2018. The primary endpoint was grade 2 or higher acute RP (RP2). Dose metrics were generated from the bilateral lung volume outside GTV (VdoseG) and PTV (VdoseP). The associations of RP2 with clinical variables, dose-volume parameters and mean lung dose (MLD) were analyzed by univariate and multivariate logistic regression. The power of discrimination among each predictor was assessed by employing the bootstrapped area under the receiver operating characteristic curve (AUC), net reclassification improvement (NRI), and the integrated discrimination improvement (IDI).
Results: Thirty-four (14.4%) out of 236 patients developed acute RP2 after the end of IMRT. The clinical parameters were identified as less important predictors for RP2 based on univariate and multivariate analysis. In both studied groups, the significance of association was more convincing in V20P, V30P, and MLDP (smaller Ps) than V5G and V5P. The largest bootstrapped AUC was identified for the V30P. We found a trend of better discriminating performance for the V20P and V30P, and MLDP than the V5G and V5P according to the higher values in AUC, IDI, and NRI analysis. To limit RP2 incidence less than 20%, the V30P cutoff was 14.5%.
Conclusions: This study identified the intermediate dose-volume parameters V20P and V30P with better prediction performance for acute RP2 than low-dose metrics V5G and V5P. Among all studied predictors, the V30P had the best discriminating power, and should be considered as a supplement to the traditional dose constraints in lung cancer treated with IMRT.
Introduction
Acute radiation pneumonitis (RP), a challenging dose-limiting toxicity, commonly occurs within 1 to 6 months (most often within 12 weeks) after the completion of thoracic radiotherapy (RT) (1, 2). It is also the main reason to preclude the initiation of consolidative immunotherapy for local-advanced unresectable non-small cell lung cancer (3).
The quantitative analyses of normal tissue effects in the clinic (QUANTEC) lung project reviewed over 70 articles published before 2010 and provided the most reliable dose-RP relationship models to overcome the inconsistency (4). Accordingly the guidelines recommend the cutoff values of lung dose constraints to be the bilateral lung volume exceeding 20 Gy (V20) ≤35% and mean lung dose (MLD) ≤20 Gy (5, 6). However, the majority of evidence in the QUANTEC was based on three-dimensional conformal radiation therapy (3D-CRT), which may not well represent the dose distributions delivered by the more advanced techniques, such as intensity-modulated radiotherapy (IMRT). Ten years have passed since the QUANTEC, and there is a need to investigate more accurate dose predictors based on new data emerging from IMRT.
In a secondary analysis of the Radiation Therapy Oncology Group (RTOG) 0617, the IMRT group had a significantly larger V5 (61.6% vs. 58.4%), similar V20 and MLD compared to the 3D-CRT group. However, on the contrary, the severe RP was found to be significantly lower in the IMRT group (3.5% vs. 7.9%) (7). The commonly used dose constraints, especially V5, could not provide a sufficient explanation of why the severe RP was much lower in the IMRT group.
We hypothesized that the dose distribution differences between 3D-CRT and IMRT might impact the plan optimization strategy. This study aimed to analyze the prediction performance for symptomatic RP using various dosimetric parameters in two independent groups of lung cancer treated with IMRT.
Materials and Methods
Study Population
In this study, we retrospectively reviewed a total of 236 patients treated with IMRT between January 2014 and August 2018. The primary IMRT group included 183 consecutive patients with lung cancer treated before September 2017. The key inclusion criteria were pathologically confirmed lung cancer, available dosimetric data, follow-up records of at least three months, conventional daily fraction, first time receiving thoracic RT, and only thoracic IMRT. Patients receiving a prescription dose of less than 50 Gy were excluded from this study.
Starting from November 2017, we prescribed a higher dose for definitive radiotherapy to patients with unresectable stage III NSCLC. In addition, we routinely acquired a mid-treatment computed tomography (CT) and planned a new adaptive radiotherapy (ART). An independent group of 53 consecutive patients treated with IMRT-ART were selected using the same inclusion criteria as for the primary IMRT group.
The bilateral lung volumes were delineated according to the RTOG 1106 atlas of organs at risk under the revision and supervision of a senior physician (8). An additional lung definition was created for each patient by excluding the PTV from the bilateral lung volume. For all included patients, the collected clinical variables included age, gender, smoking status, tumor histology and stage, chemotherapy, and surgery. Dose metrics generated from dose-volume histograms (DVHs) in this study were including V5G, V10G, V20G, V30G, V40G, V50G, and MLDG from the bilateral lung volume minus GTV, and V5P, V10P, V20P, V30P, V40P, V50P, and MLDP from the lung minus PTV. The absolute lung volumes spared from certain dose levels were collected, including 5, 10, 20, 30, 40, and 50 Gy (AV5-50Spared). The total dose metrics for adaptative plans were summed up by using rigid registration and slightly manual adjustment with initial plans.
The institutional review board in our medical center waived the requirement of written informed consent because of the retrospective design in this study.
Radiotherapy
Conventional or four-dimensional (4D) computed tomography (CT) was performed for the radiotherapy simulations. The patients were immobilized in the supine position with their arms above their head. The CT scans were performed with 5 mm or less slice thickness and included the entire neck and lung. Pre-treatment positron emission tomography (PET)/CT was not routinely used in staging and tumor volume delineation.
All of the patients were treated with conventionally fractionated simultaneous integrated boost IMRT. The gross tumor volume (GTV) was defined as the visible primary tumor and positive mediastinal lymph node on the treatment planning CT or pre-treatment PET scan. The clinical target volume (CTV) was defined as GTV with a 0.5 cm to 1 cm margin and the region at high risk for lymph node involvement. Another 5 mm uniform expansion was delineated from the GTV and CTV to create the planning gross tumor volume (PGTV) and planning target volume (PTV). Image guidance was performed with an orthogonal megavoltage electronic portal imaging device (EPID) or a kilovoltage cone beam computed tomography (CBCT) for inter-fractional geometric assurance.
The prescriptions of conventionally fractionated IMRT were 54 to 66 Gy to the PGTV and 45 to 54 Gy to the PTV in 25 to 30 fractions for curative intent. The prescription dose was 50 Gy to the PTV for the patients receiving postoperative RT with negative margins or local palliative purposes. All treatment plans were designed with the goal of delivering the prescribed dose to at least 95% of the PGTV and PTV.
Endpoints and Follow-Ups
The primary endpoint was grade 2 or above acute radiation pneumonitis (RP2) within three months after radiotherapy. We graded RP according to the system described by Kong et al., which combines the considerations of SWOG, RTOG criteria, and CTCAE to provide an accurate assessment. The toxicities were prospectively evaluated during RT, and at 1 and 3 months of follow-up after the completion of IMRT. The diagnosis of acute RP was required to be distinguished from other causes, such as fibrosis, infection, or tumor recurrence.
Statistical Analysis
For a description of the population, we used the median and range for continuous variables and the percentage for categorical variables. Univariate and multivariate logistic regression were performed to analyze the correlation between predictors and RP2. The age and location factors will be included in the multivariate analysis since they were found associated with a higher risk of pneumonitis from several previous reports (9, 10). All factors with a P value less than 0.20 in the univariate analysis will be included in a multivariate analysis. Because the multicollinearity among dose metrics, only one parameter at a time will be put in each multivariate model with set clinical factors. The patients who died before a diagnosis of RP2 were not censored, since only the acute phase of RP after radiotherapy was considered. The Akaike information criterion (AIC) and Bayesian information criterion (BIC) were applied to assess the relative goodness of fit for each dose prediction model. We employed the area under the receiver operating characteristic curve (AUC) of the receiver operating characteristic curve (ROC) to assess the RP2 discrimination performance, with the 1000-sample bootstrap method to internal validate the stability of the predictors. The RP2 risk predictors were further compared by the integrated discrimination improvement (IDI) and net reclassification improvement (NRI) analysis. A positive value of NRI or IDI indicates a preferred model over the reference in discriminating of the events and non-events (11, 12). Differences were considered significant at P<0.05 (2-sided). GraphPad Prism, version 8.02 (GraphPad Software, San Diego, California) and R (R Foundation for Statistical Computing, Vienna, Austria) were used in this study.
Results
Baseline Characteristics and RP2 Association Analysis
A total of 236 patients were retrospectively reviewed in this study. The clinical characteristics and their association with RP2 are shown in Table 1. RP2 was found in 34 patients (14.4%); 26 out of 183 (14.2%) in the IMRT group, and 8 out of 53 (15.1%) in the IMRT-ART group. In the univariate logistic regression, none of the clinical factors was significantly associated with RP2, although the female gender (P=0.101) and the use of chemotherapy (P=0.107) had a trend of higher RP2 risk.
In the primary IMRT group, a significant association with RP2 was found for V5, V10, V20, V30, and MLD from two lung definitions. The significance was more convincing in V20P (P= 0.005, OR=1.204), V30P (P=0.003, OR=1.302) and MLDP (0.004, OR=1.421) than in the other parameters (Figure 1A). In the IMRT-ART group, only the parameters of V20, V30, and MLD from both lung volumes were confirmed to be significantly associated with RP2 (Figure 1B).
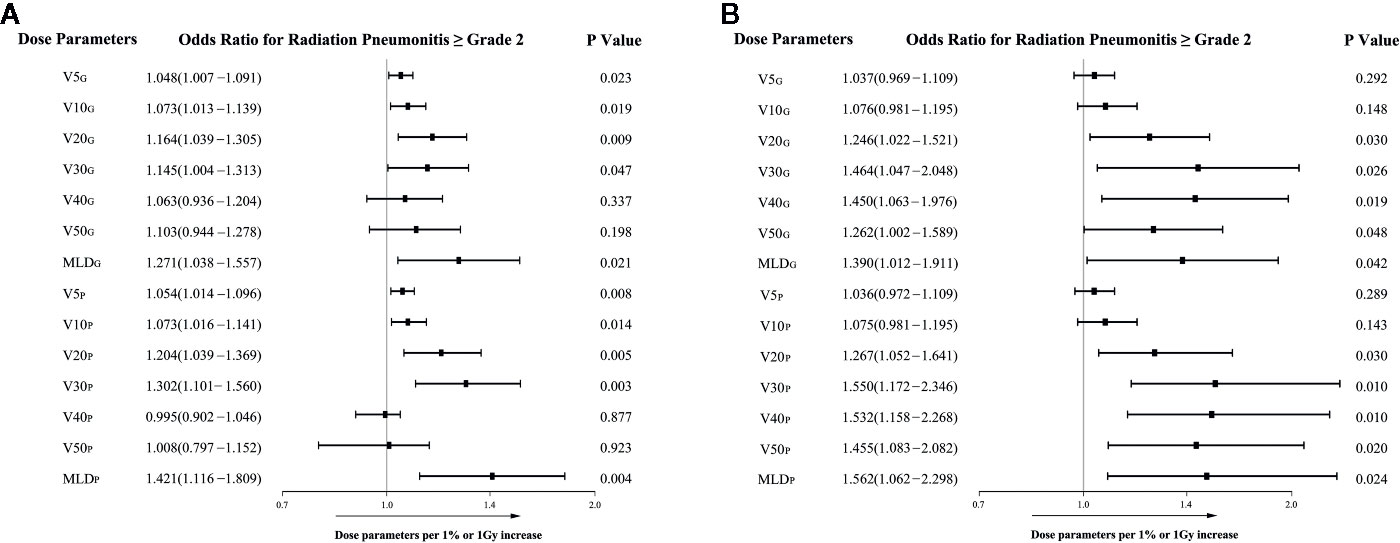
Figure 1 The associations of dosimetric parameters with grade ≥2 radiation pneumonitis in the univariate logistic regression analysis. (A) The associations in the primary IMRT group; (B) The associations in the IMRT-ART group.
In the multivariate analysis, age, tumor location, and chemotherapy did not reach significance (All Ps> 0.05). All of the dosimetric factors remained as independent predictors of RP2 in each of their multivariate models. Female gender was found significantly associated with RP2 in the models including V20G and V20P, but not had a significant association in those including other dosimetric parameters (Table S1). Given a very limited number of female patients were included in this study (9.7%), the gender factor will not be considered in the direct comparison of the prediction performance for RP2 using different dosimetric factors. Discrimination performance for RP2
We employed the bootstrapped area under the ROC (AUC) to evaluate the discrimination performance for RP2 using each dosimetric parameter in 236 patients. The V30P had the best prediction performance among all dose metrics (AUC=0.683). We found that the V5, V20, V30 and MLD from the bilateral lung volume minus PTV with larger AUCs than the ones from the lung minus GTV. The V20, V30 and MLD from both lung volumes showed a trend of better discriminating values than V5, even their confidence intervals of AUCs overlapped. The absolute volume of spared lung parameters showed lower prediction values for RP2 (All AUCs smaller than 0.55) compared with the dose-volume predictors (Table 2).
The V20P, V30P, and MLDP displayed a trend towards larger values of NRI and IDI than the most commonly used parameter, MLDG, in both the primary IMRT and IMRT-ART groups. The V5 and V10 from two lung volumes compared with the MLDG had less reliable prediction performance in IMRT-ART group, while V40 and V50 were significantly inferior in discrimination based on the primary IMRT data. Details of NRI and IDI analysis for each dose metrics are shown in Figure 2.
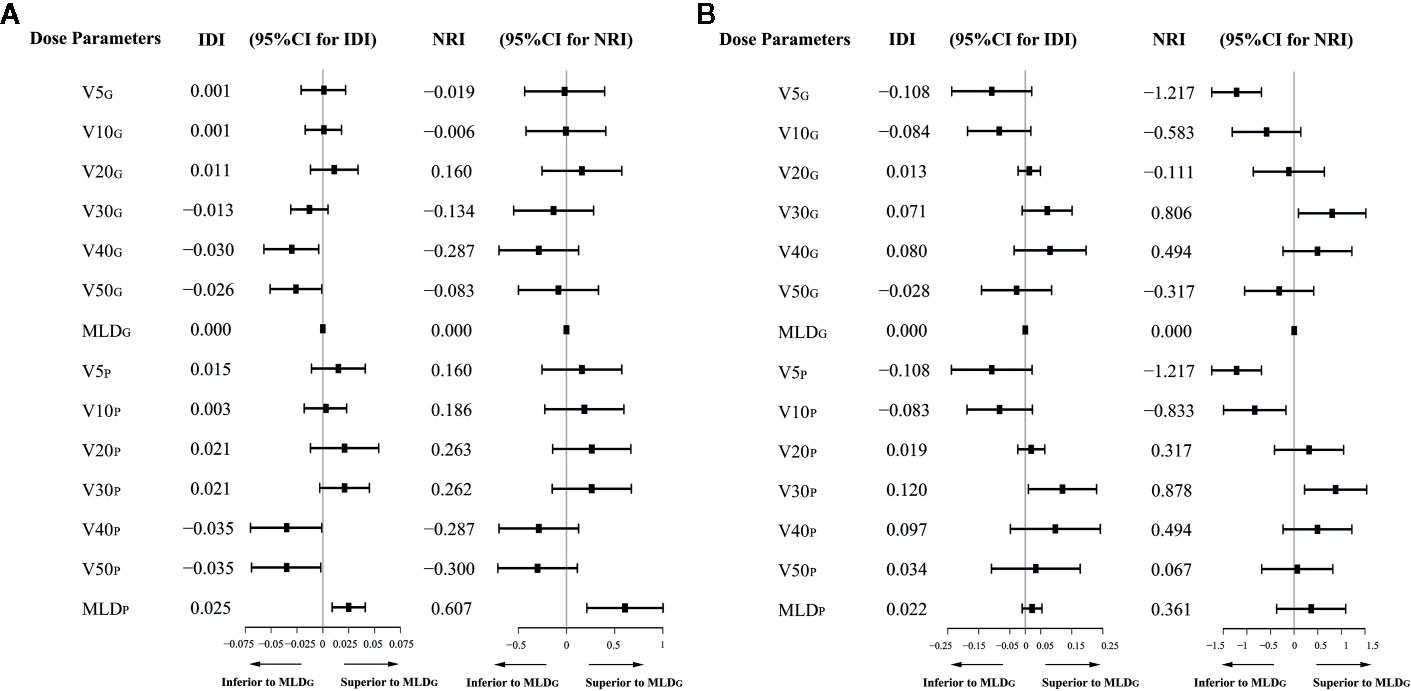
Figure 2 Integrated discrimination improvement (IDI) and net reclassification improvement (NRI) analysis for each dosimetric predictor compared with the MLDG. (A) IDI and NRI values in the primary IMRT group; (B) IDI and NRI values in the IMRT-ART group.
Evaluation of the Goodness of Fit for Prediction Models
The Akaike information criterion (AIC) and Bayesian information criterion (BIC) were used to evaluate the relative values of the goodness of fit for RP2 prediction models in two independent groups. Among all candidate models, better data fitness with the smallest values of AIC and BIC were found in the model with V30P in both IMRT and IMRT-ART groups. The models with V20P, V30P, and MLDP had relatively smaller values of AIC and BIC than V5G and V5P in both groups of patients (Figure 3).
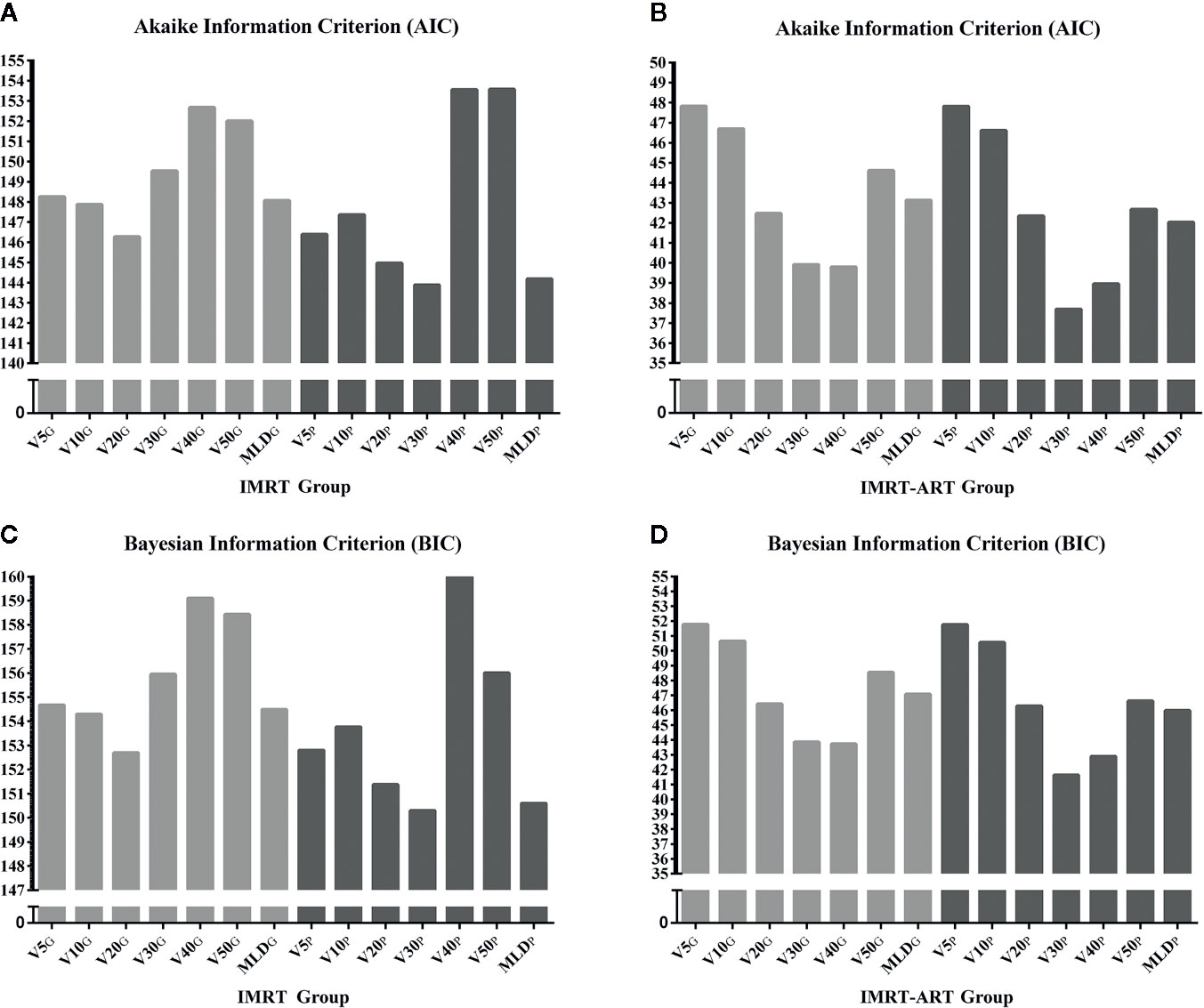
Figure 3 The relative evaluation of goodness of fit test for a model selection using Akaike information criterion (AIC) and Bayesian information criterion (BIC). (A) The relative values of the AIC in the IMRT group; (B) The relative values of the AIC in the IMRT-ART group; (C) The relative values of the BIC in the IMRT group; (D) The relative values of the BIC in the IMRT-ART group.
Prediction Model With V30P
The probability of RP2 from 236 included patients can be estimated from V30P by a fitted logistic formula: Logit(P) = −4.84+0.238X; P (% of RP2) =1/[1+exp (−0.238*V30P + 4.84)]. The prediction curve was plotted in Figure 4. The Hosmer-Lemeshow test showed no significant departure from a well-fitted model (P=0.968). To limit the probability of RP2 less than 20%, the V30P should be controlled to under 14.5%. According to current data, when the V30P <14.5%, the RP2 incidence was 11.2%; and when the V30P >14.5%, the RP2 incidence was 26.5%.
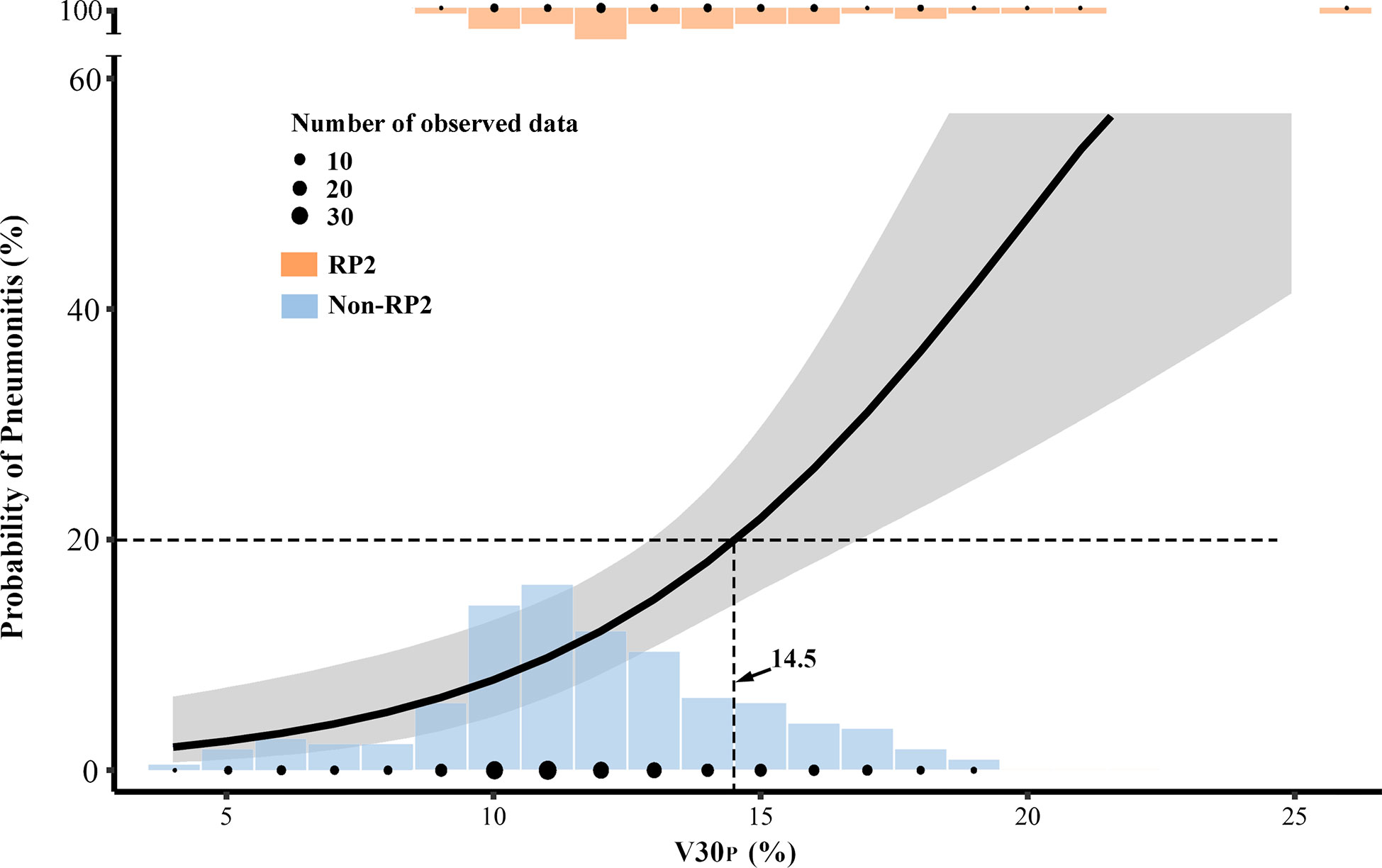
Figure 4 The prediction model with V30P was plotted in a solid curve with a 95% confidential interval for the probability of grade≥2 acute radiation pneumonitis (RP2). The V30P cutoff was 14.5% for limiting 20% RP2. The plotted dots and columns represented the number of observed data at each dose level.
Discussion
This study demonstrated that the V30P had the best RP2 prediction performance among all dosimetric parameters in two independent groups. None of the clinical factors showed a significant correlation with RP2 in univariate and multivariate analysis. The best prediction performance for RP2 was found with V30P, based on better goodness of fit in AIC and BIC, the largest bootstrapped AUC, and an upward trend towards higher NRI and IDI compared to other dosimetric predictors.
To the best of our knowledge, when using IMRT, this is one of the first studies to compare the prediction performance for RP2 among dosimetric factors from two lung definitions. The results showed that putting a higher priority on the V30P over V5 in planning optimization may better shape the DVHs, which may lead to a lower RP2 probability.
The normal lung volume definition currently recommended by both the RTOG and EORTC guidelines is total bilateral lung volume minus GTV (5, 8). QUANTEC also recommended using the definition of excluding GTV for the consideration of inconsistent delineation of the PTV from one institution to another (4). Therefore, most studies using IMRT from the past several years only report dose data from the lung volume minus GTV (13–15). However, our previous studies demonstrated that the dosimetric parameters from the lung minus PTV are not inferior to those in the lung minus GTV for RP2 prediction (16). In this study, we further demonstrated that V30P might be the best predictor among all parameters. Reducing the lung dose inside the PTV may not be reasonable during the optimization process. The conflict between getting 95% of the PTV covered with prescription and simultaneously reducing the dose to the lung in the PTV may complicate the optimization process.
Various dosimetric parameters are highly correlated with each other (17–19). The relative priority of reducing one dose parameter at the expense of increasing another is still unknown. However, with IMRT and VMAT, we have more freedom to optimize the shape of the DVH by reducing the volume of the intermediate dose region by irradiating a more substantial volume with a low dose bath. A few studies discussed the question of whether to deliver a low dose to a larger volume (“a little to a lot”) or a high dose to a smaller volume (“a lot to a little”) to further reduce the symptomatic RP probability. Willner et al. concluded that a small dose to a large volume was preferable to a large dose to a small lung volume (20). Multiple studies, on the other hand, highlighted the importance of the V5 or other low dose predictors. Metha et al. argued that “a little to a lot” could be worse than “a lot to a little” because the loss of carbon monoxide diffusing capacity occurs at 13 Gy (21). Wang et al. analyzed 223 patients treated with 3D-CRT and found a cutoff point of 42% in the V5 to have the best discrimination power for severe RP (18). Yorke et al. concluded that the low dose from V5 to V13 in the total and ipsilateral lung volume were more strongly correlated with severe RP than the V20 and higher dose parameters (19). In the IMRT era, however, the lung V5 did not show a higher priority than other dose metrics for lung toxicity prevention based on most studies (7, 22). Tucker et al. analyzed the differences in RP risk for patients receiving the same MLD but with different shapes of the DVHs. They suggested that the high dose region plays a more important role than the mean lung dose in the risk of severe RP; “a lot to a little” is associated with a higher risk of severe RP than “a little to a lot.” (23) These findings were also confirmed in their later validation study (24). Our results found an inferior predictive value for the V5, which was consistent with the IMRT studies above. We further demonstrated a better RP2 prediction performance with the V30 in the lung region outside the PTV. Adding V30P along with the traditional V20G and MLDG constraints in treatment planning optimization may better shape the DVH to further reduce the RP2 probability.
We recognized that this study is limited in several aspects. First, the patients in the IMRT- ART group had a mid-treatment CT scan and a treatment replanning. The difference in dose calculation methods between non-adaptive and adaptive RT could have resulted in variation in the overall lung dose estimation. However, the prediction performance for each dose parameter was always directly compared in a single patient. Different approaches could have impacted the exact cutoff value, but they would not have changed the relative predictive power regarding which predictor is better. Second, this was a single-institution retrospective study; 236 patients were still a small sample size considering that only 34 patients developed acute RP2. Third, immunotherapy after definitive concurrent chemoradiotherapy is considered a standard routine practice for unresectable locally advanced NSCLC (25). However, during the time of this study, our patients did not have access to PD-1 or PD-L1 inhibitors. The influence of immunotherapy on RP toxicity was not considered in this study.
Ideally, the individual sensitivity to RP2 should be identified before determining the RT prescription. Some investigators have focused on the impact of clinical factors on RP (9, 26, 27). In the current study, only the gender and chemotherapy showed a trend of association with RP2. The older age and lower lobe location were not identified as high-risk factors, and they may not significantly impact the comparison results of dosimetric predictors. Correlations between biological markers and an increased risk of RP have also been found in several studies (28–30). However, none of these risk predictors has been applied and proved in a prospective clinical trial yet. The conventionally fractionated definitive RT for lung cancer is generally prescribed at 60 to 70 Gy with no further escalation in routine practice (31, 32). The most important tool to prevent symptomatic RP is not only to keep it under “safety” criteria, but also to optimize the lung dose as low as reasonably. Our results suggested that the V30P should be weighted as a higher priority dose constraint in the treatment planning optimization in order to lower the RP2 risk further. A large external dataset from other institutions is needed in the future to further validate the superior RP2 predictive value of the V30 from the lung volume outside the PTV.
Data Availability Statement
The raw data supporting the conclusions of this article will be made available by the authors, without undue reservation.
Ethics Statement
The studies involving human participants were reviewed and approved by the institutional review board of Taizhou Hospital. Written informed consent for participation was not required for this study in accordance with the national legislation and the institutional requirements.
Author Contributions
HY, F-MSK, and WL had the ideas and contributed to the study design. YM, WW, HY, and LH carried out the statistical analysis. YM, CZ, SZ, WL, and WW contributed to the literature search. YM, LH, and XT contributed to the treatment planning and dosimetry data. CZ, SZ, and HY contributed to the evaluation of radiation pneumonitis and clinical data. YM, WL, F-MSK, and HY were major contributors to the writing of the manuscript. All authors contributed to the article and approved the submitted version.
Funding
This work was funded by the National Natural Science Foundation of China (81874221), and Taizhou Science and Technology Bureau (20ywa09 and 1802ky07).
Conflict of Interest
The authors declare that the research was conducted in the absence of any commercial or financial relationships that could be construed as a potential conflict of interest.
Supplementary Material
The Supplementary Material for this article can be found online at: https://www.frontiersin.org/articles/10.3389/fonc.2020.584756/full#supplementary-material
References
1. Kim TH, Cho KH, Pyo HR, Lee JS, Zo JI, Lee DH, et al. Dose-volumetric parameters for predicting severe radiation pneumonitis after three-dimensional conformal radiation therapy for lung cancer. Radiology (2005) 235(1):208–15. doi: 10.1148/radiol.2351040248
2. Hanania AN, Mainwaring W, Ghebre YT, Hanania NA, Ludwig M. Radiation-Induced Lung Injury: Assessment and Management. Chest (2019) 156(1):150–62. doi: 10.1016/j.chest.2019.03.033
3. Shaverdian N, Offin MD, Rimner A, Shepherd AF, Wu AJ, Rudin CM, et al. Utilization and factors precluding the initiation of consolidative durvalumab in unresectable stage III non-small cell lung cancer. Radiother Oncol (2020) 144:101–4. doi: 10.1016/j.radonc.2019.11.015
4. Marks LB, Bentzen SM, Deasy JO, Kong FM, Bradley JD, Vogelius IS, et al. Radiation dose-volume effects in the lung. Int J Radiat Oncol Biol Phys (2010) 76(3 Suppl):S70–76. doi: 10.1016/j.ijrobp.2009.06.091
5. De Ruysscher D, Faivre-Finn C, Moeller D, Nestle U, Hurkmans CW, Le Péchoux C, et al. European Organization for Research and Treatment of Cancer (EORTC) recommendations for planning and delivery of high-dose, high precision radiotherapy for lung cancer. Radiother Oncol (2017) 124(1):S0167814017304036. doi: 10.1016/j.radonc.2017.06.003
6. National Comprehensive Cancer Network. Non-small cell lung Cancer. Version 1. (2020). Available at: http://www.nccn.org.
7. Chun SG, Hu C, Choy H, Komaki RU, Timmerman RD, Schild SE, et al. Impact of Intensity-Modulated Radiation Therapy Technique for Locally Advanced Non-Small-Cell Lung Cancer: A Secondary Analysis of the NRG Oncology RTOG 0617 Randomized Clinical Trial. J Clin Oncol (2017) 35(1):56–62. doi: 10.1200/JCO.2016.69.1378
8. Kong FM, Ritter T, Quint DJ, Senan S, Gaspar LE, Komaki RU, et al. Consideration of dose limits for organs at risk of thoracic radiotherapy: atlas for lung, proximal bronchial tree, esophagus, spinal cord, ribs, and brachial plexus. Int J Radiat Oncol Biol Phys (2011) 81(5):1442–57. doi: 10.1016/j.ijrobp.2010.07.1977
9. Vogelius IR, Bentzen SRM. A literature-based meta-analysis of clinical risk factors for development of radiation induced pneumonitis. Acta Oncol (2012) 51(8):975–83. doi: 10.3109/0284186X.2012.718093
10. Kong FM, Wang S. Nondosimetric risk factors for radiation-induced lung toxicity. Semin Radiat Oncol (2015) 25(2):100–9. doi: 10.1016/j.semradonc.2014.12.003
11. Pencina MJ, D’ Agostino RB Sr., D’ Agostino RB Jr., Vasan RS. Evaluating the added predictive ability of a new marker: From area under the ROC curve to reclassification and beyond. Stat Med (2008) 27(2):157–72. doi: 10.1002/sim.2929
12. Pencina MJ, D’Agostino RB, Vasan RS. Statistical methods for assessment of added usefulness of new biomarkers. Clin Chem Lab Med (2010) 48(12):1703–11. doi: 10.1515/CCLM.2010.340
13. Khalil AA, Hoffmann L, Moeller DS, Farr KP, Knap MM. New dose constraint reduces radiation-induced fatal pneumonitis in locally advanced non-small cell lung cancer patients treated with intensity-modulated radiotherapy. Acta Oncol (2015) 54(9):1343–9. doi: 10.3109/0284186X.2015.1061216
14. Wijsman R, Dankers F, Troost EGC, Hoffmann AL, van der Heijden EHFM, de Geus-Oei L-F, et al. Comparison of toxicity and outcome in advanced stage non-small cell lung cancer patients treated with intensity-modulated (chemo-)radiotherapy using IMRT or VMAT. Radiother Oncol (2017) 122(2):295–9. doi: 10.1016/j.radonc.2016.11.015
15. Wijsman R, Dankers FJWM, Troost EGC, Hoffmann AL, van der Heijden EHFM, de Geus-Oei L-F, et al. Inclusion of Incidental Radiation Dose to the Cardiac Atria and Ventricles Does Not Improve the Prediction of Radiation Pneumonitis in Advanced-Stage Non-Small Cell Lung Cancer Patients Treated With Intensity Modulated Radiation Therapy. Int J Radiat Oncol Biol Physics (2017) 99(2):434–41. doi: 10.1016/j.ijrobp.2017.04.011
16. Meng Y, Yang H, Wang W, Tang X, Jiang C, Shen Y, et al. Excluding PTV from lung volume may better predict radiation pneumonitis for intensity modulated radiation therapy in lung cancer patients. Radiat Oncol (2019) 14(1):7. doi: 10.1186/s13014-018-1204-x
17. Kong FM, Hayman JA, Griffith KA, Kalemkerian GP, Arenberg D, Lyons S, et al. Final toxicity results of a radiation-dose escalation study in patients with non-small-cell lung cancer (NSCLC): predictors for radiation pneumonitis and fibrosis. Int J Radiat Oncol Biol Phys (2006) 65(4):1075–86. doi: 10.1016/j.ijrobp.2006.01.051
18. Wang S, Liao Z, Wei X, Liu HH, Tucker SL, Hu C-S, et al. Analysis of clinical and dosimetric factors associated with treatment-related pneumonitis (TRP) in patients with non–small-cell lung cancer (NSCLC) treated with concurrent chemotherapy and three-dimensional conformal radiotherapy (3D-CRT). Int J Radiat Oncol Biol Phys (2006) 66(5):1399–407. doi: 10.1016/j.ijrobp.2006.07.1337
19. Yorke ED, Jackson A, Rosenzweig KE, Braban L, Leibel SA, Ling CC. Correlation of dosimetric factors and radiation pneumonitis for non-small-cell lung cancer patients in a recently completed dose escalation study. Int J Radiat Oncol Biol Physics (2004) 63(3):672–82. doi: 10.1016/j.ijrobp.2005.03.026
20. Willner J, Jost A, Baier K, Flentje M. A Little to a Lot or a Lot to a Little? Strahlenther Und Onkol (2003) 179(8):548–56. doi: 10.1007/s00066-003-1078-0
21. Mehta V. Radiation pneumonitis and pulmonary fibrosis in non–small-cell lung cancer: Pulmonary function, prediction, and prevention. Int J Radiat Oncol Biol Phys (2005) 63(1):5–24. doi: 10.1016/j.ijrobp.2005.03.047
22. Yom SS, Liao Z, Liu HH, Tucker SL, Hu CS, Wei X, et al. Initial evaluation of treatment-related pneumonitis in advanced-stage non-small-cell lung cancer patients treated with concurrent chemotherapy and intensity-modulated radiotherapy. Int J Radiat Oncol Biol Phys (2007) 68(1):94–102. doi: 10.1016/j.ijrobp.2006.12.031
23. Tucker SL, Liu HH, Liao Z, Wei X, Wang S, Jin H, et al. Analysis of radiation pneumonitis risk using a generalized Lyman model. Int J Radiat Oncol Biol Phys (2008) 72(2):568–74. doi: 10.1016/j.ijrobp.2008.04.053
24. Tucker SL, Xu T, Paganetti H, Deist T, Verma V, Choi N, et al. Validation of Effective Dose as a Better Predictor of Radiation Pneumonitis Risk Than Mean Lung Dose: Secondary Analysis of a Randomized Trial. Int J Radiat Oncol Biol Phys (2019) 103(2):403–10. doi: 10.1016/j.ijrobp.2018.09.029
25. Antonia SJ, Villegas A, Daniel D, Vicente D, Murakami S, Hui R, et al. Overall Survival with Durvalumab after Chemoradiotherapy in Stage III NSCLC. N Engl J Med (2018) 379(24):2342–50. doi: 10.1056/NEJMoa1809697
26. Palma DA, Senan S, Tsujino K, Barriger RB, Rengan R, Moreno M, et al. Predicting Radiation Pneumonitis After Chemoradiation Therapy for Lung Cancer: An International Individual Patient Data Meta-analysis. Int J Radiat Oncol Biol Phys (2013) 85(2):444–50. doi: 10.1016/j.ijrobp.2012.04.043
27. Appelt AL, Bentzen SM, Farr KP, Khalil AA, Vogelius IR. OC-0077: Towards individualized dose constraints: The QUANTEC radiation pneumonitis model with clinical risk factors. Radiother Oncol (2013) 106(5):605. doi: 10.1016/S0167-8140(15)32383-5
28. Stenmark MH, Cai X-W, Shedden K, Hayman JA, Yuan S, Ritter T, et al. Combining Physical and Biologic Parameters to Predict Radiation-Induced Lung Toxicity in Patients With Non-Small-Cell Lung Cancer Treated With Definitive Radiation Therapy. Int J Radiat Oncol Biol Phys (2012) 84(2):e217–22. doi: 10.1016/j.ijrobp.2012.03.067
29. Hawkins PG, Boonstra PS, Hobson ST, Jwd H, Hayman JA, Ten Haken RK, et al. Radiation-induced lung toxicity in non-small-cell lung cancer: Understanding the interactions of clinical factors and cytokines with the dose-toxicity relationship. Radiother Oncol J Eur Soc Ther Radiol Oncol (2017) 125(1):66. doi: 10.1016/j.radonc.2017.09.005
30. Wang S, Campbell J, Stenmark MH, Zhao J, Stanton P, Matuszak MM, et al. Plasma Levels of IL-8 and TGF-β1 Predict Radiation Induced Lung Toxicity in Non-Small Cell Lung Cancer Patients: A Validation Study. Int J Radiat Oncol Biol Phys (2017) 98(3):615. doi: 10.1016/j.ijrobp.2017.03.011
31. Bezjak A, Temin S, Franklin G, Giaccone G, Govindan R, Johnson ML, et al. Definitive and Adjuvant Radiotherapy in Locally Advanced Non-Small-Cell Lung Cancer: American Society of Clinical Oncology Clinical Practice Guideline Endorsement of the American Society for Radiation Oncology Evidence-Based Clinical Practice Guideline. J Clin Oncol (2015) 33(18):2100–5. doi: 10.1200/JCO.2014.59.2360
32. Bradley PJD, Rebecca Paulus BS, Komaki PR, Gregory Masters MD, MD PHC. Standard-dose versus high-dose conformal radiotherapy with concurrent and consolidation carboplatin plus paclitaxel with or without cetuximab for patients with stage IIIA or IIIB non-small-cell lung cancer (RTOG 0617): a randomised, two-by-two factorial ph. Lancet Oncol (2015) 16(2):187–99. doi: 10.1016/S1470-2045(14)71207-0
Keywords: lung cancer, intensity modulated radiotherapy (IMRT), radiation pneumonitis (RP), dosimetric parameters, prediction model
Citation: Meng Y, Luo W, Wang W, Zhou C, Zhou S, Tang X, Hou L, Kong FS and Yang H (2020) Intermediate Dose-Volume Parameters, Not Low-Dose Bath, Is Superior to Predict Radiation Pneumonitis for Lung Cancer Treated With Intensity-Modulated Radiotherapy. Front. Oncol. 10:584756. doi: 10.3389/fonc.2020.584756
Received: 18 July 2020; Accepted: 22 September 2020;
Published: 15 October 2020.
Edited by:
Tiziana Rancati, Istituto Nazionale dei Tumori (IRCCS), ItalyReviewed by:
Gilles Defraene, KU Leuven, BelgiumZachary D Horne, Allegheny Health Network, United States
Copyright © 2020 Meng, Luo, Wang, Zhou, Zhou, Tang, Hou, Kong and Yang. This is an open-access article distributed under the terms of the Creative Commons Attribution License (CC BY). The use, distribution or reproduction in other forums is permitted, provided the original author(s) and the copyright owner(s) are credited and that the original publication in this journal is cited, in accordance with accepted academic practice. No use, distribution or reproduction is permitted which does not comply with these terms.
*Correspondence: Haihua Yang, yhh93181@hotmail.com