- 1Department of Radiology and Nuclear Medicine, Amsterdam University Medical Center, University of Amsterdam, Amsterdam, Netherlands
- 2Brain Plasticity Group, Swammerdam Institute for Life Sciences, University of Amsterdam, Amsterdam, Netherlands
- 3Department of Child- and Adolescent Psychiatry, Triversum, Alkmaar, Netherlands
- 4Department of Psychology, University of Amsterdam, Amsterdam, Netherlands
- 5Academic Center for Child- and Adolescent Psychiatry, De Bascule, Duivendrecht, Netherlands
- 6Department of Sleep and Cognition, Netherlands Institute for Neuroscience, Amsterdam, Netherlands
- 7Departments of Integrative Neurophysiology and Psychiatry, Center for Neurogenomics and Cognitive Research (CNCR), VU University and Medical Center, Amsterdam, Netherlands
- 8Center for Sleep and Wake Disorders, Haaglanden Medical Center, The Hague, Netherlands
Methylphenidate (MPH) improves behavioral symptoms of attention-deficit/hyperactivity disorder (ADHD). Its effects on sleep, however, are insufficiently known, as trials with MPH in medication-naive children were so far restricted to relatively short trial durations. Here, we assessed effects of prolonged MPH treatment on sleep in medication-naive boys in a 16-weeks double-blind, placebo controlled, multicenter clinical trial with immediate-release MPH (ePOD-MPH trial, NTR3103). Seventy-five medication-naive boys, aged 10–12 years, were screened for eligibility using ADHD DSM-IV criteria. Sleep was assessed using actigraphy, diaries and questionnaires prior to randomization, in week 8, and 1 week after trial end. Fifty boys (mean age 11.4y, SD 0.9) were randomized to MPH or placebo. Linear mixed model analysis demonstrated a significant time-by-treatment interaction effect (p = 0.007) on sleep efficiency. Post-hoc analyses demonstrated that the two groups did not differ from each other (p = 0.94) during treatment (week 8), but that sleep efficiency was significantly improved in the MPH (p = 0.005), but not placebo group (p = 0.87) 1 week after trial end. The lack of MPH's negative effects on sleep during treatment differ from most previous studies and could be explained by the relatively long trial duration in our study and the medication-naive status of our sample; suggesting that evaluating sleep problems only shortly after treatment onset presents an incomplete picture, because it might not be representative for sleep quality after longer treatment periods. Our findings of improved sleep after trial end could be due to rebound effects or longer-term effects of MPH treatment and therefore require replication.
Clinical Trial Registration: Central Committee on Research Involving Human Subjects (an independent registry, identifier NL34509.000.10) before enrollment of the first subject and The Netherlands National Trial Register, identifier NTR3103.
Introduction
Attention-deficit/hyperactivity disorder (ADHD) is a neurodevelopmental disorder characterized by inattention, hyperactivity, and impulsivity (1). Methylphenidate (MPH) is the most frequently prescribed stimulant medication for ADHD treatment and is very effective in alleviating many ADHD symptoms. MPH blocks the dopamine (DA) transporter, thereby increasing extracellular DA levels, which is thought to underlie the changes in behavioral symptoms (2). Previous studies indicate a five-fold higher prevalence of sleep problems in children with ADHD compared to healthy children (3). These problems include high bedtime resistance, delayed sleep onset, frequent nocturnal awakenings, and excessive daytime sleepiness in ADHD (4–6). However, it is poorly understood whether these sleep problems are part of ADHD symptomatology per se or are induced by ADHD medication, and which neurobiological mechanisms underlie these sleep problems (7).
Although the possibility that sleep problems could be induced by ADHD medication often forms a concern for parents when they discuss the option of pharmacotherapy with the psychiatrist and/or pediatrician (8, 9), studies on the effects of ADHD medication on sleep have so far been inconclusive. Two meta-analyses (10, 11) on objective sleep estimates (including actigraphy and polysomnography) in children with ADHD have concluded that MPH delayed the sleep onset, reduced total sleep time and lowered sleep efficiency relative to placebo-treated patients. However, treatment duration in most earlier studies was relatively short; typically 1–7 weeks, whereas children are generally treated with MPH for months to years. In addition, in most studies children were not medication-naive, which makes it difficult to disentangle effects of ADHD per se from effects of previous medication on these sleep measures.
Neurobiologically, there is considerable support for an effect of MPH on sleep, given the role of the dopaminergic system in sleep-wake regulation. For instance, mice with a deleted dopamine transporter (DAT) gene are more wakeful compared to controls (12), and blocking the DAT with GBR-12909 dose-dependently increases wakefulness (13). This supports that MPH's short term effect is wake-promoting. When considering sleep quality in ADHD, it is important to consider the effects of MPH within the framework of the sleep/wake cycle model of Borbély (1982) and Schwartz and Roth (2008). This model proposes a process S (sleep) and a process C (circadian), that interact to regulate sleep. Process S is defined as sleep pressure (7), which accumulates during the day, and controls the need for sleep after a certain amount of time awake. On the other hand, process C is defined based on a circadian rhythm (14). Previous studies have emphasized that correct dosing as well as the timing of the medication during the day is important to mitigate MPH-related sleep problems (15). MPH administered at the right time and dose might reduce daytime sleepiness often reported in ADHD [as demonstrated in both children and adults (16, 17)] and result in build up of sleep pressure during the day, resulting in improved sleep at night. Unfortunately, most trials conducted so far are relatively short, whereas titration of the correct dose and dissipation of induced sleep problems may take some more time.
Therefore, we here investigated effects of immediate-release MPH on various sleep measures in a cohort of medication-naive ADHD children during a relatively long treatment period. These data were obtained from the “ePOD”-MPH randomized clinical trial (RCT) (Effect of Psychotropic Drugs on the Developing brain) in which medication-naive ADHD patients were randomly assigned to treatment with either MPH or placebo for a period of 16 weeks (18). Actigraphic measures were obtained at week 8 (during treatment) and week 17 (1 week after trial end). In line with previous literature described above, we expected MPH to negatively affect sleep at week 8 of treatment. The expected effect at 17 weeks is more difficult to predict, at least for the MPH group, because medication was stopped and this measurement can have been confounded by rebound effects. Nevertheless, we initially hypothesized that negative effects would be less pronounced or even absent at the end of the trial (week 17) compared to mid-trial (week 8), because one study found that with an increasing duration of the trial, fewer sleep problems were present (11), and because of the hypothesized positive effects of MPH on daytime sleepiness through increased DA turnover. For the placebo group, we expected no changes at week 8 or week 17.
Materials and Methods
Trial Design
The ePOD-MPH RCT was a 16-weeks double-blind, randomized, placebo-controlled, multicenter trial with immediate-release MPH treatment in medication-naive children, and adults with ADHD with a blinded end-point evaluation (17). The primary outcome measure of the ePOD-MPH trial was to report on the modification by age of MPH treatment on the outgrowth of the DA system, using state-of-the-art magnetic resonance imaging (MRI) techniques. Our hypothesis was that MPH would induce lasting effects on DA function, one week after treatment end in children, but not in adults, reflecting presumed “neurochemical imprinting” effects, which have been found in rats with the same technique (19). These DA findings have been reported elsewhere (20).
The two secondary outcome measures were: (a) 2 functional outcome measures (fMRI and a neuropsychological test battery (18) and (b) effects of MPH on sleep, which we report here. Because of incomplete sleep data in the adult groups, only the children with complete data sets were included in the current study.
The institutional review board of the Academic Medical Center (AMC) approved the study. The trial ended on June 15, 2015 and was monitored by the Clinical Research Unit of the AMC. Written informed consent was obtained from the legal representatives of all participants.
Participants
Participants were 50 medication-naive boys (10–12 years old) recruited through clinical programs at the Departments of Child and Adolescent Psychiatry at Triversum (Alkmaar, the Netherlands) and De Bascule Academic Center for Child and Adolescent Psychiatry (Amsterdam, the Netherlands). All participants were diagnosed with ADHD according to DSM-IV (1) by an experienced psychiatrist (MB). The Diagnostic Interview Schedule for Children (authorized Dutch translation)(21) was used to confirm the diagnosis. Subjects with comorbid Axis-I psychiatric disorders requiring medication treatment, a history of major neurological or medical illness, or a history of clinical treatment with drugs influencing the DAergic system (e.g., stimulants, neuroleptics, antipsychotics, and DA receptor type 2 and 3 agonists) were excluded.
Intervention, Randomization, and Blinding
Participants were randomly assigned (1:1) using a permuted block randomization scheme generated by the local Clinical Research Unit to either immediate-release MPH or matching placebo (PLAC) treatment for 16 weeks. Allocation was concealed for all parties. Placebo and MPH tablets were similar in appearance and were manufactured according to Good Manufacturing Practice criteria. After study end, blinding was checked with the patient and his psychiatrist as well as the study investigators.
The hospital pharmacy (Alkmaar) assigned participants to a specific allocation, using sequentially numbered containers. Participants as well as care providers and research personnel were blinded. The placebo tablet was identical to the MPH tablet with respect to appearance and was manufactured and labeled according to GMP guidelines (2003/94/EG). The treating physician prescribed the medication under double-blind conditions on clinical guidance (reduction in ADHD symptoms), in accordance with Dutch treatment guidelines. That is, children received oral dosages of MPH starting with 0.3 mg/kg day in 1–2 doses and dosages were increased weekly with 5–10 mg/day to a maximum of 40 mg daily until target clinical dosage was reached. If, after in- or decreasing the dosage, serious side effects occur, the patient returned to the previous dosage and dosage modifications will be more gradual thereafter. Decisions about dosage modifications were always and only taken by the treating psychiatrist. Parents of the children received psycho-education. Adherence to the study medication was monitored at 5 control visits (in week 1, 2, 3, 8, and 12).
Outcomes
Our main outcome measure was estimated sleep efficiency (SE) prior to-, during-, and one week after treatment discontinuation, assessed using actigraphy. SE best summarizes the quality, composition, continuity, and consolidation of sleep (22). Secondary sleep outcome measures were; sleep onset latency (SOL), total sleep time (TST), total in-bed time (TIB), objective and subjective sleep start time (SST and SST-SUBJ), objective and subjective final wake time (Wake time and Wake-subj), wake after sleep onset (WASO), number of wake bouts (WBnumber), mean wake bout time (WBmean), interdaily stability (IS), intradaily variability (IV), the amount of activity during the 5 h with the lowest activity (L5) and during the 10 h with the highest activity (M10), the amplitude of the sleep-wake rhythm (AMP) (Table 1).
To obtain these data, subjects wore an Actiwatch AW4 (CamNtech Ltd., Cambridge, UK) on the non-dominant wrist for 24 h/day for five consecutive nights at three time-points during the 16-week treatment: 1 week before randomization (baseline, BL), during the eighth week of treatment (during treatment, DT) and during the week after treatment, i.e., in week 17 (post-treatment, PT) (Figure 1). Actiwatch Sleep Analysis 5.0 Software (CNT, UK) was used to calibrate the actiwatches. Epoch length was set to 30 sec. In addition, subjects were instructed to fill in a sleep-diary for five days during each actigraphy period. The actimetry sensor of the ActiWatch measures the gross motor activity of the wrist, with high sensitivity to a palmar-dorsal movement (z-axis). Raw ActiWatch data were extracted and transformed into raw three-dimensional accelerometry data in the unit of counts and were analyzed using MATLAB (23) using the Oakley algorithm (24). In short, a 3–11 Hz band-pass filter was applied prior to sampling at 50 Hz and the data were converted to 128 bins with a resolution of approximately 25 counts/g. Any negative values were set to zero and residual baseline noise was removed. The signal was then converted to unit counts by taking the peak value of each second and summing across the epoch length (i.e., 30 sec). For identifying immobile-mobile (or sleep-wake) pattern, the threshold for MOBILE was set to a medium sensitivity for a window period of 10 min so that the algorithm scores any first 10-min period with more than 1 mobile epoch with activity counts exceeding threshold as MOBILE (or wake). Therefore, the algorithm accommodates only 1 mobile epoch for the given time window during the estimation of sleep onset time and latency. The sleep logs were entered into the program to obtain the subjective sleep parameters for analysis.
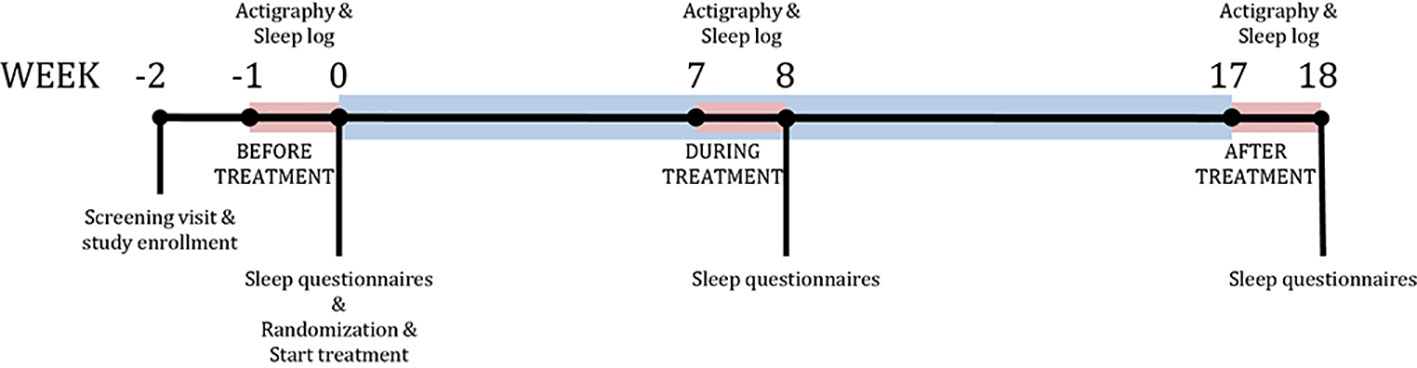
Figure 1 Study design. Timeline of the study. Blue bar represents treatment period. Red bars represent sleep measurement periods using the actigraph.
In addition to actigraphic sleep estimates, several self-reported questionnaires were administered (Supplementary Methods and Results): the Holland Sleep Disorder Questionnaire (HSDQ), used to screen for sleep disorders at baseline; the Epworth Sleepiness Scale (ESS); and the Evaluation List Insomnia Therapy (ELIT) were assessed at all three time points as subjective sleep questionnaire. Severity of restless legs syndrome (RLS), often associated with sleep problems in ADHD, was measured with the Johns Hopkins RLS severity scale (JHRLSS) during the trial. ADHD symptom severity was assessed using the Disruptive Behavior Disorder Rating Scale (DBD-RS).
Statistical Analysis
A per protocol analysis was conducted, with significance level set at p < 0.05 (2-sided). A linear mixed model [using SPSS 22.0 (IBM, 2013)] was used to estimate the time-by-treatment interaction effect. An autoregressive covariance matrix was assumed, with a fixed intercept and the model was estimated using maximum likelihood. Follow-up pairwise comparisons were corrected for multiple testing using Sidak's correction. As some subjects used melatonin (BL, DT, and PT n = 9) and/or wore the actigraph during holiday periods (BL n = 5, DT n = 10, PT n = 8), these variables were added to the model as dummy-coded covariates. Furthermore, JHRLSS scores were added as covariate. Since there were several missing values for this covariate (18.80% at BL, 22.90% DT and 20.80% PT), we replaced these using logistic regression imputation based on group, age, and JHRLSS score at the two other time-points.
For the sleep variables that were calculated per day (SE, SOL, TST, SST, SST-SUBJ, TIB, Wake time, Wake-SUBJ, WASO, WBnumber, WBmean), days were added as a secondary repeated variable to the model. The ESS and ELIT were not normally distributed and therefore log-transformed. Exploratory analyses were performed to assess possible correlations between the sleep variables and to distinguish between- from within-subject effects (Supplementary Methods and Results).
Results
Randomisation and Baseline Characteristics
Seventy-five children were screened for study inclusion. Fifty subjects met the inclusion criteria and were randomly assigned (1:1) to receive either MPH or placebo treatment (Figure 2). Baseline characteristics of the two groups are reported in Table 2. DBD-RS scores of attention and hyperactivity did not differ between the two randomisation groups at baseline (attention p = 0.20, hyperactivity p = 0.61). According to the HSDQ, severe sleep problems were absent from our sample at baseline. Adherence to the trial medication did not differ between the two randomisation groups (mean MPH 84.89%, PLAC 79.68%, p = 0.31). The final titrated dose in the placebo group was higher than the one prescribed in the MPH group, but this difference was not statistically different (mean dose MPH 29.13 mg (0.86 mg/kg), mean PLAC 34.40 mg (0.88 mg/kg), p = 0.07).
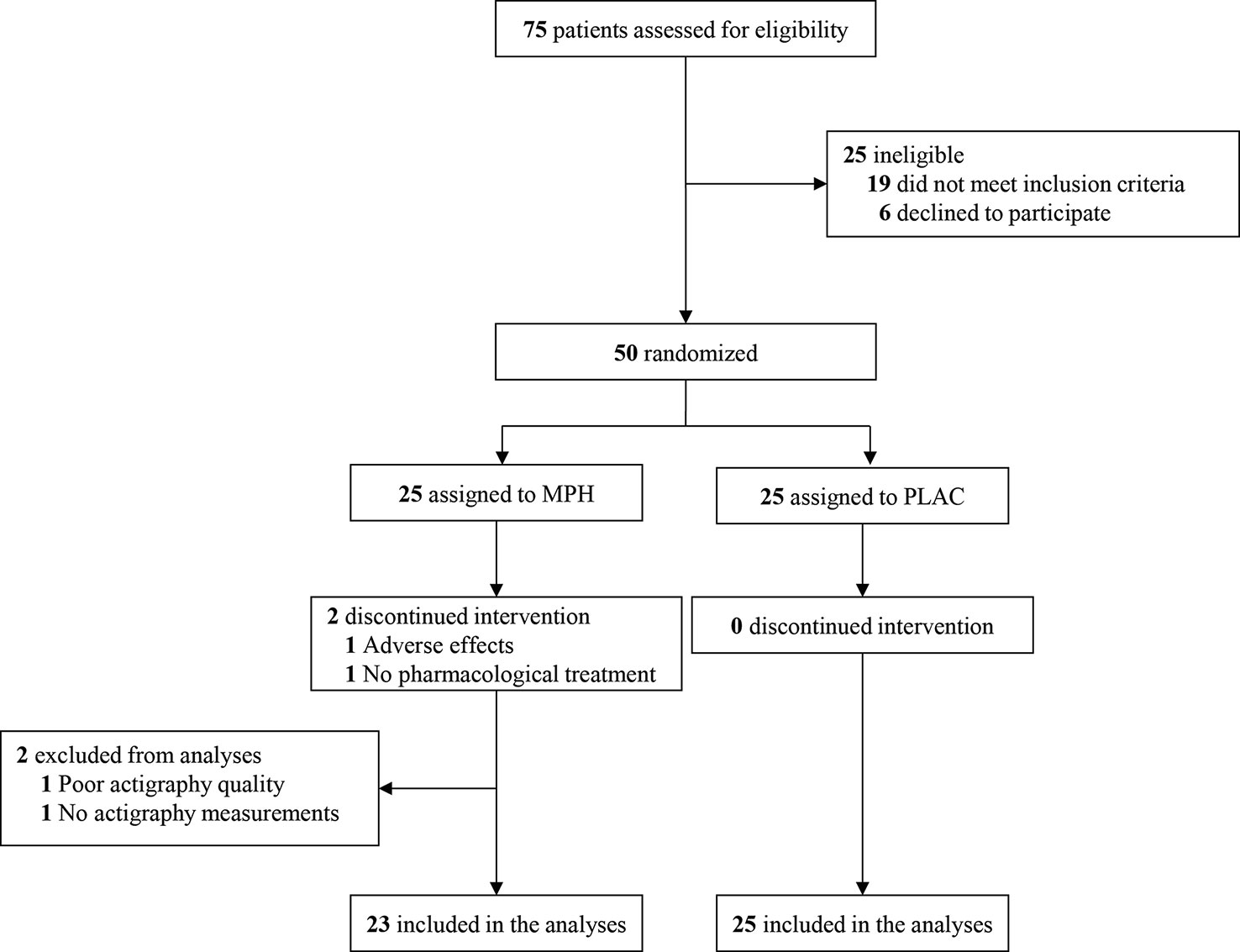
Figure 2 Consolidated standards of reporting trials flow diagram. MPH, methylphenidate condition; PLAC, placebo condition.
Main Outcome Measure SE
Due to logistical problems, sleep data was missing from two participants. Linear mixed model analysis showed a significant time-by-treatment interaction effect on our primary outcome variable SE (F(2,240) = 5.07, p = 0.007). MPH induced an increase in SE at post-treatment when compared to baseline (mean difference 4.94%; 95%CI, 1.18-8.70; p = 0.005). Also, the MPH group had higher SE values than the placebo group (mean difference 5.84%; 95%CI, 2.79-8.88; p < 0.01). At baseline and during treatment (week 8), no differences were found in SE between the MPH and placebo group (BL, mean difference 0.07%, 95%CI -2.88-2.74, p = 0.962; DT, mean difference 0.11%, 95%CI -2.81-3.03, p = 0.94)(Figure 3 and Table S1). Addition of the covariates holiday, melatonin and RLS to the model did not affect our results, although both holiday and melatonin had a main negative effect (holiday F(1,219) = 4.47, p = 0.04, melatonin F(1,145) = 17.68, p < 0.01).
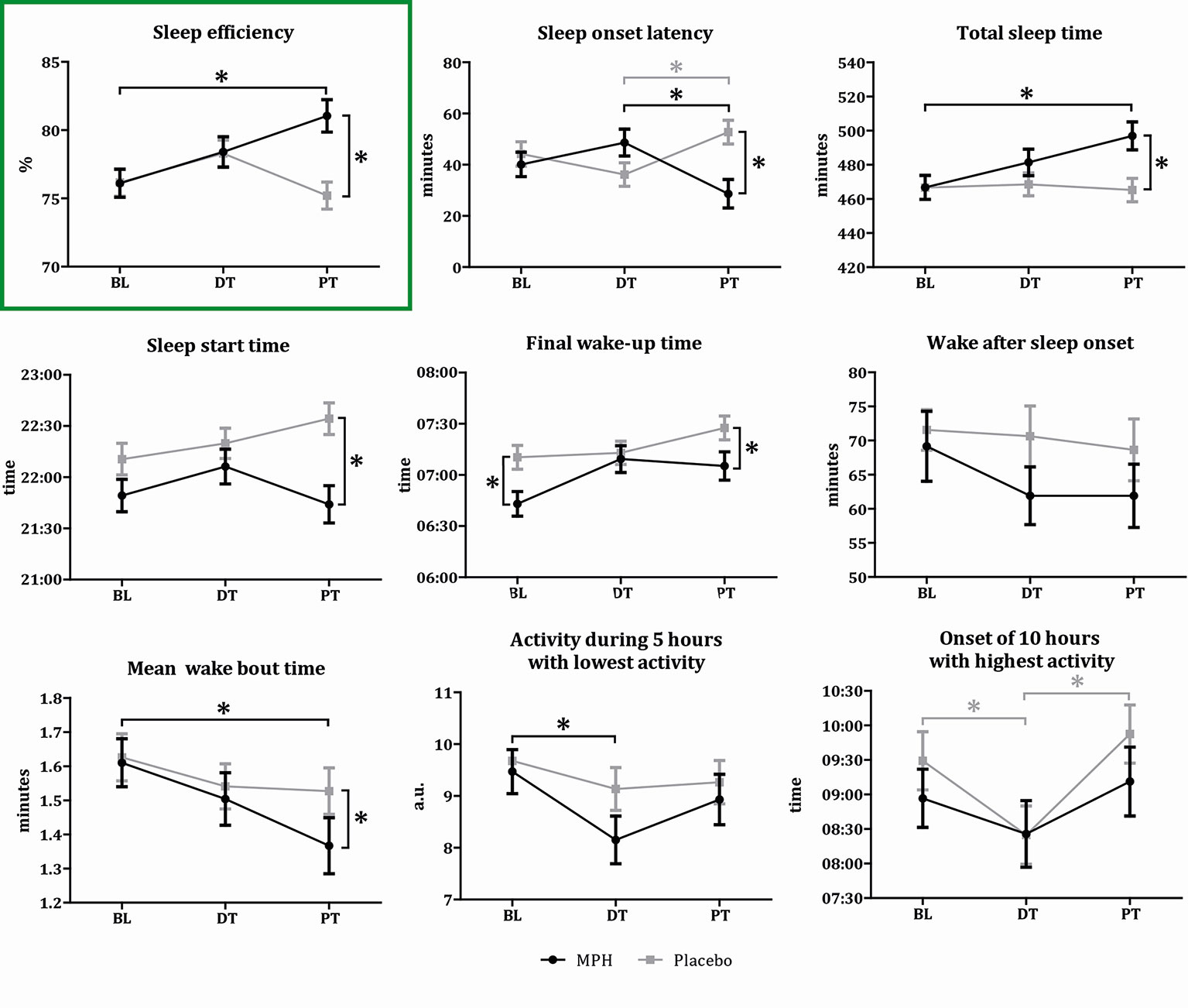
Figure 3 Results sleep variables. Graphical representation of the sleep variable outcomes for the two conditions on three time points (BL, baseline; DT, during treatment; PT, post-treatment). Asterisks indicate a significant difference between the two conditions (p < 0.05). Values represent means per condition for each time point, bars represent the standard deviation. Black lines indicate the MPH treated subjects, gray lines the placebo treated subjects.
Secondary Outcome Measures
Significant time-by-treatment interaction effects, or main effects of time or treatment, were found for our secondary outcome variables SOL, TST, SST, Wake time, Wake-SUBJ, M10 onset, and L5. Significance values and post-hoc comparisons for these sleep variables are presented in Figure 3 and Tables S1 and S2. All these variables demonstrated positive effects in the MPH group at trial end when compared to baseline values as well as to the placebo group. The addition of the covariates did not affect these results, although a time-by-treatment interaction effect for WASO was apparent after addition of the covariates, and not before (Figure 3 and Tables S1 and S2). For the ELIT sleep subscore as well as for the ELIT wake subscore, we observed a main effect of time, indicating reduced sleep complaints during the trial in both treatment groups. For the ESS questionnaire, we observed a main effect of treatment, which was the result of a decrease in excessive daytime sleepiness induced by MPH when compared to the placebo group (Table S3 and Figure S1).
ADHD Symptom Severity
We observed a significant time-by-treatment interaction (F(2,87) = 3.33, p = 0.04) on attention symptom severity, indicative of lower ADHD symptoms scores in the MPH group compared to placebo during and after treatment (mean difference DT 4.64, 95%CI 2.14-7.15, p < 0.01, mean difference PT 4.61, 95% CI 2.23-6.98, p < 0.01). Additionally, in both groups, the attention symptom severity was lower during treatment and post-treatment, compared to baseline (DT vs. BL; MPH, mean difference 8.62, 95%CI 6.19–11.06, p < 0.01; PLAC, mean difference 5.29, 95%CI 2.98–7.60, p < 0.01; PT vs. BL; MPH, mean difference 8.5, 95%CI 6.42–10.58, p < 0.01; PLAC, mean difference 5.14, 95%CI 3.15–7.13, p < 0.01). For hyperactivity scores, only a main effect of time was found (F(2,82) = 23.09, p < 0.01), indicating decreased hyperactivity scores in both groups during treatment and post-treatment when compared to baseline (DT vs. BL; mean difference 4.7, 95%CI 2.98–6.43, p < 0.01; PT vs. BL; mean difference 4.62, 95%CI 2.48–6.76, p < 0.01) (see Table 2).
Discussion
In this RCT, we investigated the effects of MPH treatment on various sleep measures in medication-naive boys diagnosed with ADHD. MPH did not affect objective sleep quality mid-treatment (i.e., at week 8). Moreover, children in the MPH group demonstrated a higher sleep efficiency one week after the end of the trial, had a shorter sleep onset latency and slept longer as compared to both the placebo group and baseline. As expected, no changes in sleep efficiency were observed in the placebo group, either at week 8 or week 17. After trial end, participants in both the placebo and MPH groups reported less sleep-related problems. Additionally, MPH treatment improved the attention subscores compared to placebo, whereas hyperactivity improved in both the MPH and placebo group.
The absence of an effect of MPH mid-treatment (week 8) on several sleep variables in boys with ADHD is in contrast with two meta-analyses in children, that found MPH to worsen sleep; which was concluded based on increases in SOL, decreases in SE and an increased number of wake bouts in these studies (10, 11). This discrepancy may be explained by the fact that our first measurement on medication was after 8 weeks, whereas previous studies conducted actigraphy or polosomnography after 1–7 weeks of medication. Interestingly, in line with our observations, one study indeed reported that SE improved when ADHD medication was used for longer periods of time (11). Moreover, the fact that most of these studies had included already medicated children with ADHD may underlie the inconsistency with our current findings. The inclusion of medication-naive subjects in the current study is a critical element in its design, as this excludes any possible interacting effects of prior medication. Using a post-hoc power analysis we found that the effect size of the difference between placebo and MPH at week 8 to have a Cohen's d of 0.01 (resulting in a required sample size >1,000 children per group), suggesting that the likelihood of our finding to be the result of a type-II error to be very small.
One week after trial end, we did observe a positive effect of prolonged MPH treatment on sleep, indicating that the current effects of MPH on sleep outlast the acute effects of the drug. This could result from lasting reductions in ADHD symptoms, e.g., a reduction in attentional problems and thus an altered activity during day time. Indeed, the increased ability to concentrate during the day and a decreased day-time sleepiness may have resulted in an increased build-up of “sleep pressure” (process S), which may have then improved sleep quality at night. Nevertheless, we cannot rule out the possibility that these positive effects result from a “rebound” effect, i.e., a recovery from prior sleep loss. Indeed, an increased sleep propensity (i.e., a shorter sleep onset, longer sleep time) is a hallmark of sleep recovery. However, rebound effects are more likely to occur in the case of sleep deprivation or sleep problems, which were not found in our study for the MPH or placebo group. Unfortunately, in the absence of sleep measures collected in the week prior to trial end we cannot draw firm conclusions based on these findings. Of course, within subject crossover designs would be advantageous in terms of power, but are not ethical with such long treatment durations—therefore most studies using these designs limit the placebo period to 1–2 weeks. The time that children in this study received placebo was therefore dictated by the length of the waiting list (4 months) in the Netherlands at that time (i.e., meaning that children were not withheld effective treatment for longer than in standard clinical care. Notwithstanding, it would be interesting to study children at even longer follow up times whether enduring effects of MPH on sleep are still present after medication is stopped. Preclinical literature on the DA system suggest this might be the case, acting via lasting MPH-induced changes in the developing DA system (‘neurochemical imprinting') (19, 25). Consistent with this, we did observe lasting changes in DA function studied before in the same children (20).
The current effects of MPH can be interpreted within the framework of the sleep/wake cycle model of Borbély (14) and Schwartz and Roth (7). Sleep problems in children with ADHD have been previously attributed to changes in either process S (26) or C (27), and increases in SE could be due to changes in either process. However, based on our significant effects of MPH on SE, SOL, TST, SST, and wake time, and due to a lack of effects on the M10 and L5 circadian rhythm sleep variables, it seems more likely that in particular process S is involved in the current effects of MPH on sleep quality in ADHD.
The results of our RCT are of considerable clinical relevance, as possible effects on sleep quality are often an important concern when parents discuss MPH prescription to their children with their psychiatrist or pediatrician (8, 9). Becker et al. (15) reported that children with pre-existing sleep problems can benefit from MPH when titrated at the right dose. Moreover, our results add that the evaluation of sleep problems only shortly after treatment onset might be inappropriate, or at least might not be representative for sleep quality when measured after longer treatment periods (28). Additional studies are needed to further establish the optimal time window for sleep-related effects of MPH, what the effect of drug “holidays” is on sleep, and whether the cessation of medication can change sleep.
There are also some limitations to our study. First, we only included boys of around 11 years old and it thus remains to be demonstrated whether our findings can be generalized to females and other age groups. Although our sample may seem somewhat atypical, it does represent the age group with the highest prevalence of ADHD in boys in the Netherlands. Our sample presented with mild-to-moderate symptoms and therefore the MPH dose prescribed was low-to-moderate. Additionally, based on the HSDQ, no severe (subjective) sleep problems were present in our sample. This could have resulted in more neutral or positive effects of MPH on sleep. It is possible that more severe cases (and those with comorbidities) present at an earlier age and that higher dose regimens result in more negative effects of MPH on sleep. Future studies should investigate whether the current positive effects of MPH on sleep can be extended also to children with ADHD who suffer from more severe ADHD or sleep problems. Moreover, we adminisitered immediate release MPH in this trial and results cannot directly be generalized to extended release medications. For example, Faraone et al. (29) have demonstrated in a recent meta-analysis that the risk for MPH-associated sleep disorders was largest for extended release medications, likely due to pharmacological effects that extend into bedtime. Indeed, using actigraphy it was already demonstrated that triple dosing vs double dosing with MPH decreases total sleep time in children with ADHD, presumably due to a later dosing in time (30); clearly this is a factor that needs to be taken into account both in clinical studies and clinical practice. A second limitation is that some subjects additionally received melatonin during the measurement periods (n = 9 for all three time points). Melatonin is known to positively affect process C (26), and has also been shown to have an inhibitory effect on presynaptic dopamine release (31). Therefore, it was considered a potential confounder and included as a significant covariate in our statistical model for SE, SOL, WASO, WBmean, and IV. This did not change the main results, however, and is therefore unlikely to have influenced our main results. This suggests that the pro-dopaminergic effect of MPH is stronger than the dopamine inhibiting effect of melatonin. Nevertheless, as melatonin use was only present in nine subjects, it is difficult to test its specific effects for this sample and future studies should test interactions between stimulants and melatonin in relation to sleep. Another limitation is that despite our randomization, baseline, and post-treatment wake time differed between the two groups (Table S1). Since wake time was significantly later during a holiday than during a school-week (p = 0.009), we evaluated whether treatment groups were differentially measured during holidays. This was however not the case (at baseline: MPH n = 2, placebo n = 3; at follow-up: MPH, n = 4, placebo n = 4) and adding holiday to the model did not alter our findings either.
Conclusion
In this cohort of medication-naive children with ADHD we show that treatment with MPH does not negatively affect sleep during (week 8) or 1 week after treatment (week 17). Moreover, sixteen weeks of MPH treatment actually increased the efficiency and duration of sleep at 1 week after trial end, but this study could not confirm whether this was a rebound effect or just a long-term effect of treatment. Nevertheless, evaluation of sleep problems associated with ADHD medication should not only be assessed shortly after the onset of treatment, but also after longer treatment periods. Our findings underscore the need for further research, especially in studies with longer MPH treatment durations.
Author’s Note
The study was carried out at Amsterdam University Medical Centers, University of Amsterdam.
Data Availability Statement
The datasets generated for this study are available on request to the corresponding author.
Ethics Statement
The study involving human participants were reviewed and approved by Central Committee on Research Involving Human Subjects The Netherlands. Written informed consent to participate in this study was provided by the participants' legal guardian/next of kin.
Author Contributions
AS, CB, and HT were responsible for the data collection, with support from MB and RR. HB and MS were responsible for the data processing. MS was responsible for the statistical analyses. AS, CB, HT, ES, LR, MS, and PL interpreted the data. MS, AS, RR, ES, LR, and PL wrote the manuscript. All authors reviewed and approved of the manuscript.
Funding
This study was funded by faculty resources of the Academic Medical Center, University of Amsterdam, and grant 11.32050.26 from the European Research Area Network Priority Medicines for Children (Sixth Framework Programme). Also the Swammerdam Institute for Life Sciences of the University of Amsterdam supported the study. PJL, AS and LR are also supported by Amsterdam Brain & Cognition.
Conflict of Interest
The authors declare that the research was conducted in the absence of any commercial or financial relationships that could be construed as a potential conflict of interest.
Acknowledgments
The authors thank the participants and their parents for their contribution to the study.
Supplementary Material
The Supplementary Material for this article can be found online at: https://www.frontiersin.org/articles/10.3389/fpsyt.2020.00082/full#supplementary-material
Figure S1 | Results subjective sleep subscores and questionnaire. ESS, Epworth Sleepiness Scale; ELIT, Evaluation List Insomnia Therapy.
Abbreviations
ADHD, Attention-Deficit/Hyperactivity Disorder; AMC, Academic Medical Center; AMP, amplitude of the sleep-wake rhythm; BL, baseline; DA, dopamine; DBD-RS, Disruptive Behavior Disorder Rating Scale; DT, during treatment; ELIT, Evaluation List Insomnia Therapy; ePOD-MPH, Effect of Psychotropic Drugs on the Developing brain MPH trial; ESS, Epworth Sleepiness Scale; HSDQ, Holland Sleep Disorder Questionnaire; IS, interdaily stability; IV, intradaily variability; JHRLSS, Johns Hopkins RLS severity scale; L5, amount of activity during the 5 h with the lowest activity; MPH, methylphenidate; M10, amount of activity during the 10 h with the highest activity; PLAC, placebo; Process C, process circadian; Process S, process sleep; PT, post-treatment; RCT, randomized controlled trial; RLS, restless legs syndrome; RLS-HTDI, Johns Hopkins telephone diagnostic interview for RLS; SE, sleep efficiency; SOL, sleep onset latency; SST, objective sleep start time; SST-SUBJ, subjective sleep start time; TIB, total in-bed time; TST, total sleep time; Wake time, objective final wake time; Wake-subj, subjective final wake time; WASO, wake after sleep onset; WBmean, mean wake bout time; WBnumber, number of wake bouts; 95%CI, 95% confidence interval.
References
1. American Psychiatric Association. Diagnostic and Statistical Manual of Mental Disorders 5th Edition. 5th ed. Washington (2013).
2. MTA study, MTA group, MTA-Cooperative-Group, The MTA group, MTA-Cooperative-Group. A 14-month randomized clinical trial of treatment strategies for attention-deficit/hyperactivity disorder. The MTA Cooperative Group. Multimodal Treatment Study of Children with ADHD. Arch Gen Psychiatry (1999) 56:1073–86. doi: 10.1001/archpsyc.56.12.1073
3. Corkum P, Panton R, Ironside S, MacPherson M, Williams T. Acute Impact of Immediate Release Methylphenidate Administered Three Times a Day on Sleep in Children with Attention-Deficit/Hyperactivity Disorder. J Pediatr Psychol (2007) 33:368–79. doi: 10.1093/jpepsy/jsm106
4. Cortese S, Faraone SV, Konofal E, Lecendreux M. Sleep in Children With Attention-Deficit/Hyperactivity Disorder: Meta-Analysis of Subjective and Objective Studies. J Am Acad Child Adolesc Psychiatry (2009) 48:894–908. doi: 10.1097/CHI.0b013e3181ae09c9
5. Gruber R, Sadeh A. Sleep and neurobehavioral functioning in boys with attention-deficit/hyperactivity disorder and no reported breathing problems. Sleep (2004) 27:267–73. doi: 10.1093/sleep/27.2.267
6. O'Brien LM, Gozal D. Sleep in children with attention deficit/hyperactivity disorder. Minerva Pediatr (2004) 56:585–601.
7. Schwartz JRL, Roth T. Neurophysiology of sleep and wakefulness: basic science and clinical implications. Curr Neuropharmacol (2008) 6:367–78. doi: 10.2174/157015908787386050
8. Hvolby A, Jørgensen J, Bilenberg N. Actigraphic and parental reports of sleep difficulties in children with attention-deficit/hyperactivity disorder. Arch Pediatr Adolesc Med (2008) 162:323–9. doi: 10.1001/archpedi.162.4.323
9. Owens JA, Maxim R, Nobile C, McGuinn M, Msall M. Parental and self-report of sleep in children with attention-deficit/hyperactivity disorder. Arch Pediatr Adolesc Med (2000) 154:549–55. doi: 10.1001/archpedi.154.6.549
10. De Crescenzo F, Licchelli S, Ciabattini M, Menghini D, Armando M, Alfieri P, et al. The use of actigraphy in the monitoring of sleep and activity in ADHD: A meta-analysis. Sleep Med Rev (2014) 26:9–20. doi: 10.1016/j.smrv.2015.04.002
11. Kidwell KM, Van Dyk TR, Lundahl A, Nelson TD. Stimulant Medications and Sleep for Youth With ADHD: A Meta-analysis. Pediatrics (2015) 136:1144–53. doi: 10.1542/peds.2015-1708
12. Wisor JP, Nishino S, Sora I, Uhl GH, Mignot E, Edgar DM. Dopaminergic role in stimulant-induced wakefulness. J Neurosci (2001) 21:1787–94. doi: 10.1523/JNEUROSCI.21-05-01787.2001
13. Qu W, Xu X, Yan M, Wang Y-Q, Urade Y, Huang Z-L. Essential Role of Dopamine D2 Receptor in the Maintenance of Wakefulness, But Not in Homeostatic Regulation of Sleep, in Mice. J Neurosci (2010) 30:4382–9. doi: 10.1523/JNEUROSCI.4936-09.2010
15. Becker SP, Froehlich TE, Epstein JN. Effects of methylphenidate on sleep functioning in children with attention-deficit/hyperactivity disorder. J Dev Behav Pediatr (2016) 37:395–404. doi: 10.1097/DBP.0000000000000285
16. Golan N, Shahar E, Ravid S, Pillar G. Sleep disorders and daytime sleepiness in children with attention-deficit/hyperactive disorder. Sleep (2004) 27:261–6. doi: 10.1093/sleep/27.2.261
17. Puymirat J, Bouchard JP, Mathieu J. Efficacy and Tolerability of a 20-mg Dose of Methylphenidate for the Treatment of Daytime Sleepiness in Adult Patients With Myotonic Dystrophy Type 1: A 2-Center, Randomized, Double-Blind, Placebo-Controlled, 3-Week Crossover Trial. Clin Ther (2012) 34:1103–11. doi: 10.1016/j.clinthera.2012.03.060
18. Bottelier MA, Schouw MLJ, Klomp A, Tamminga HGH, Schrantee AGM, Bouziane C, et al. The effects of Psychotropic drugs On Developing brain (ePOD) study: methods and design. BMC Psychiatry (2014) 14:48. doi: 10.1186/1471-244X-14-48
19. Andersen SL, Napierata L, Brenhouse HC, Sonntag KC. Juvenile methylphenidate modulates reward-related behaviors and cerebral blood flow by decreasing cortical D3 receptors. Eur J Neurosci (2008) 27:2962–72. doi: 10.1111/j.1460-9568.2008.06254.x
20. Schrantee A, Tamminga HGH, Bouziane C, Bottelier MA, Bron EE, Mutsaerts HJMM, et al. Age-dependent effects of methylphenidate on the human dopaminergic system in young vs adult patients with attention-deficit/hyperactivity disorder. JAMA Psychiatry (2016) 73:955–62. doi: 10.1001/jamapsychiatry.2016.1572
21. Ferdinand R, van der Ende J. NIMH DISC-IV: Diagnostic Interview Schedule for Children [in Dutch]. Rotterdam, Department of Child- and Adolescent Psychiatry, Sophia Children Hospital/Academic Hospital Rotterdam: The Netherlands (2000).
22. Weitzman ED. Electroencephalography (EEG) of Human Sleep: Clinical Applications. JAMA J Am Med Assoc (1974) 230:753. doi: 10.1001/jama.1974.03240050073035
23. te Lindert BHW, van Someren EJW. Sleep estimates using microelectromechanical systems (MEMS). Sleep (2013) 36:781–9. doi: 10.5665/sleep.2648
24. Oakley N. Validation with polysomnography of the sleep watch: Sleep/Wake scoring algorithm used by the Actiwatch Activity Monitoring System. Technical report to Mini Mitter Co., Inc.(1997).
25. Moll GHGH, Hause S, Rüther E, Rothenberger A, Huether G, Al MET, et al. Early methylphenidate administration to young rats causes a persistent reduction in the density of striatal dopamine transporters. J Child Adolesc Psychopharmacol (2001) 11:15–24. doi: 10.1089/104454601750143366
26. Schwartz G, Ben AL, Grizenko N, Lageix P, Baron C, DB B, et al. Actigraphic monitoring during sleep of children with ADHD on methylphenidate and placebo. J Am Acad Child Adolesc Psychiatry (2004) 43:1276–82. doi: 10.1097/01.chi.0000135802.94090.93
27. Mohammadi MR, Mostafavi SA, Keshavarz SA, Eshraghian MR, Hosseinzadeh P, Hosseinzadeh-Attar MJ, et al. Melatonin effects in methylphenidate treated children with attention deficit hyperactivity disorder: A randomized double blind clinical trial. Iran J Psychiatry (2012) 7:87–92.
28. Vitiello B, Severe JB, Greenhill LL, Arnold LE, Abikoff HB, Bukstein OG, et al. Methylphenidate dosage for children with ADHD over time under controlled conditions: Lessons from the MTA. J Am Acad Child Adolesc Psychiatry (2001) 40:188–96. doi: 10.1097/00004583-200102000-00013
29. Faraone SV, Po MD, Komolova M, Cortese S. Sleep-associated adverse events during methylphenidate treatment of attention-deficit/hyperactivity disorder: A meta-analysis(2019). J Clin Psychiatry (2019) 80. doi: 10.4088/JCP.18r12210
30. Stein MA, Blondis TA, Schnitzler ER, O'Brien T, Fishkin J, Blackwell B, et al. Methylphenidate Dosing: Twice Daily Versus Three Times Daily. Pediatrics (1996) 98:748–56.
Keywords: sleep, ADHD, methylphenidate, actigraphy, randomized clinical trial
Citation: Solleveld MM, Schrantee A, Baek HK, Bottelier MA, Tamminga HGH, Bouziane C, Stoffelsen R, Lucassen PJ, Van Someren EJW, Rijsman RM and Reneman L (2020) Effects of 16 Weeks of Methylphenidate Treatment on Actigraph-Assessed Sleep Measures in Medication-Naive Children With ADHD. Front. Psychiatry 11:82. doi: 10.3389/fpsyt.2020.00082
Received: 13 September 2019; Accepted: 03 February 2020;
Published: 28 February 2020.
Edited by:
Lino Nobili, University of Genoa, ItalyReviewed by:
Elisa De Grandis, University of Genoa, ItalyMark Stein, University of Washington, United States
Copyright © 2020 Solleveld, Schrantee, Baek, Bottelier, Tamminga, Bouziane, Stoffelsen, Lucassen, Van Someren, Rijsman and Reneman. This is an open-access article distributed under the terms of the Creative Commons Attribution License (CC BY). The use, distribution or reproduction in other forums is permitted, provided the original author(s) and the copyright owner(s) are credited and that the original publication in this journal is cited, in accordance with accepted academic practice. No use, distribution or reproduction is permitted which does not comply with these terms.
*Correspondence: Liesbeth Reneman, bC5yZW5lbWFuQGFtc3RlcmRhbXVtYy5ubA==
†These authors have contributed equally to this work