- 1School of Traditional Chinese Medicine, Beijing University of Chinese Medicine, Beijing, China
- 2Guang’anmen Hospital of Chinese Academy of Traditional Chinese Medicine, Beijing, China
Ischemic cardiomyopathy (ICM) is a special type or end stage of coronary heart disease or other irreversible ischemic myocardial injury. Inflammatory damage to coronary vessels is a crucial factor in causing stenosis or occlusion of coronary arteries, resulting in myocardial ischemia and hypoxia, but it is also an aspect of cardioprotection that is often overlooked. This review discusses the mechanisms of vascular injury during ICM, in which inflammation and oxidative stress interact and trigger cell death as the cause of coronary microvascular injury. Imbalances in endoplasmic reticulum function and mitochondrial quality control are important potential drivers of inflammation and oxidative stress. In addition, many studies have confirmed the therapeutic effects of Chinese herbal medicines and their natural monomeric components on vascular injuries. Their mitochondrial quality control and endoplasmic reticulum protection mechanisms as well as their role in combating improvements in vascular endothelial function and attenuating vascular injury are also summarized, with a perspective to provide a reference for pathologic understanding, drug research, and clinical application of ICM-associated coronary microvascular injury.
1 Introduction
Ischemic cardiomyopathy (ICM) is a specific type or end stage of coronary heart disease or other irreversible ischemic myocardial injury. It is mainly due to coronary atherosclerosis caused by long-term coronary artery stenosis or occlusion, resulting in long-term myocardial ischemia and hypoxia, irreversible damage to myocardial cells and irreversible cardiac remodelling (Chang et al., 2023a; Pastena et al., 2023). A series of clinical symptoms, such as angina pectoris, arrhythmia, heart failure, thrombosis and embolism follow (Panza et al., 2019), seriously threatening people’s physical and mental health (Khan et al., 2020; Virani et al., 2021).
Coronary blood flow is essential for the normal physiological function of the heart. Therefore, reduced local blood flow due to coronary artery stenosis or occlusion is often a key cause of the development of myocardial ischemia (Efentakis et al., 2024; Li S. Y. et al., 2024). Inflammatory damage to the coronary vasculature is one of the primary pathological changes in this process (Chang et al., 2023b; Zhang X. M. et al., 2023). Although the medical community has some understanding of the mechanism by which mitochondrial and endoplasmic reticulum (ER) abnormalities are involved in the occurrence and development of myocardial injury after myocardial infarction or ischemia-reperfusion (I/R), coronary circulation is still a neglected cardiac protection target so far. Its pathological mechanism in the process of ICM is not yet clear. The relevant targeted therapeutic drugs need to be further studied. A large number of studies have shown that mitochondrial quality control (MQC) and ER regulatory mechanisms are involved in regulating the whole process of vascular physiological and pathological functions (Tan et al., 2020; Hou D. et al., 2024; Li J. et al., 2024). However, in the development of ICM, the effects of both on vascular inflammatory injury are still unclear. Consequently, it is necessary to further explore the interaction mechanism of MQC and ER function involved in vascular physiology and pathology to directly link the ICM with ICM-related vascular injury (Figure 1).
Traditional Chinese medicine (TCM) has a long history of use in China, with rich drug resources and a unique theoretical system. In recent years, the research and clinical application of the main active monomers of TCM have also increased its vitality and value. Over the past two decades, many studies have demonstrated that TCM compounds, drug extracts, and related monomer drugs are able to reduce oxidative stress, inflammatory responses, and cell death by modulating MQC, ER function, and counteracting vascular injury. However, few studies have investigated the efficacy and mechanism of action of natural drugs for coronary microvascular injury during the development of ICM, which has great research value and clinical application prospects.
This article outlines the current understanding of ICM-associated inflammatory vascular injury, the interaction between oxidative stress and inflammation, and inflammation-mediated programmed cell death. The critical role of ER function and the MQC system in vascular pathology is a key focus. Furthermore, this article summarizes the vascular targeting effects of natural drugs and their monomer components that have been reported so far, with a perspective to provide a reference for drug research and clinical application in ICM-related coronary microvascular injury.
2 Pathologic mechanisms of inflammatory vascular injury
2.1 Inflammatory damage
The inflammatory process of ICM involves a variety of pathological mechanisms. Among them, oxidized LDL (ox-LDL) (Ammar et al., 2022), advanced glycation end products (AGEs) (Zhang J. et al., 2023), stable activation of hypoxia-inducible factor 1α (HIF-1α) caused by hypoxia (Du et al., 2018), and destruction of the endothelial glycocalyx barrier (Dehghani et al., 2022) are important pathological nodes for activating and promoting the vascular endothelial inflammatory response.
Endothelial cells (ECs) play a key role in maintaining vascular homeostasis by regulating vascular tone, permeability, and angiogenesis and are targets for anti-inflammatory and antithrombotic factors. It is both the target of inflammatory stimulation and the main promoter of inflammation. Under conditions of hypoxia, hyperlipidemia, hyperglycemia, oxidative stress and other adverse factors, ECs express vascular cell adhesion molecule-1 (VCAM-1), intercellular adhesion molecule-1 (ICAM-1), interleukin-6 (IL-6), IL-1, monocyte chemoattractant protein-1 (MCP-1), and other inflammatory cytokines (Schunk et al., 2021; Wang et al., 2021; Takenoshita et al., 2024). These inflammatory factors stimulate the activation, adhesion and infiltration of circulating leukocytes (including neutrophils, lymphocytes, and monocytes) (Tang et al., 2023), resulting in vascular endothelial injury. Damaged tissue further releases proinflammatory cytokines, resulting in reactivation of the proinflammatory signalling pathway and the enhancement of the inflammatory response (Zhao F. et al., 2023). In this process, toll-like receptor 4 (TLR4) and its downstream transcription factor nuclear factor kappa-B (NF-κB) perform essential functions. Adequate activation of NF-κB is necessary for the expression of major proinflammatory mediators such as tumor necrosis factor-α (TNF-α), IL-1, and IL-6 (Li et al., 2023b). TLR4, a typical pattern recognition receptor, is preferentially expressed on the surface of vascular ECs. When activated by MyD88-targeted NF-κB, TLR4 triggers the occurrence and cascade enhancement of the inflammatory response (Kiyan et al., 2019; Liu et al., 2021). Excessive inflammatory stimulation eventually leads to impaired vascular tone, increased permeability, increased procoagulant activity, and dysregulated angiogenesis (Zhao H. et al., 2023), worsening the progression of ICM.
2.2 Oxidative stress and inflammation
Oxidative stress refers to the process in which excessive reactive oxygen species (ROS), such as superoxide (O2−), peroxy radical (ROO−), and hydrogen peroxide (H2O2), are produced by the system under various pathological stimuli, which are strongly unbalanced with the antioxidant capacity of the biological system, causing toxic reactions leading to cell death and tissue damage (Ramachandra et al., 2020). During the ICM, mitochondrial reverse electron transfer (RET) and nicotinamide adenine dinucleotide phosphate (NADPH) oxidase (NOX) family enzymes are the main sources of ROS in the coronary microvascular (Manuneedhi Cholan et al., 2018; Feenstra et al., 2023).
There is a close relationship between oxidative stress and the inflammatory response. Through interaction, the two can promote the progression of a series of pathological processes of the ICM, such as abnormal vasoconstriction, fibrosis, and thrombosis (D’Onofrio et al., 2023; Kulkovienė et al., 2024). Recent studies have shown that TNF-α-treated human aortic endothelial cells (HAECs), coronary artery endothelial cells (HCAECs), and umbilical vein endothelial cells (HUVECs) all display activation of inflammatory pathways, mitochondrial abnormalities, and ROS accumulation (D’Onofrio et al., 2023). The inhibition of NF-κB p65 reduces ROS production in ox-LDL-stimulated HUVECs (Li Y. et al., 2021). In addition, neutrophils are one of the sources of ROS. ROS from ECs can amplify the inflammatory response and affect nearby neutrophils, further inducing a cascade of ROS generation (Nakamura et al., 1998; Hori and Nishida, 2008). At the same time, the increase in ROS production in inflammatory tissues during the inflammatory process significantly promotes the synthesis of proinflammatory mediators such as cytokines and chemokines involved in inflammatory cell migration (Shukla et al., 2020; Tan et al., 2022). Mitochondrial DNA (mtDNA) is oxidized and destroyed under oxidative stress, which escapes into the cytoplasm through the mitochondrial permeability transition pore (mPTP) and causes an inflammatory response (Xian et al., 2022; An et al., 2023). Excess ROS can also cause low-density lipoprotein (LDL) to peroxidize into ox-LDL (Olivares-Caro et al., 2020). Ox-LDL can upregulate the expression of p-selectin, VCAM, ICAM and MCP-1 on the cell surface, accelerate the adhesion and penetration of white blood cells to the vascular endothelium (Lei et al., 2008; Ammar et al., 2022; Luo et al., 2024), and lead to an enhanced inflammatory response. Studies have shown that by activating NF-κB, ROS can enhance the expression of the inflammatory cytokines ICAM-1, VCAM-1, IL-6 and TNF-α in vascular endothelial tissue (Kim et al., 2008; Han et al., 2015), and increase the adhesion characteristics of ECs (Alshabibi et al., 2018). In summary, there is a complex interaction between oxidative stress and inflammation, which is a key factor in promoting vascular injury and ICM progression.
2.3 Inflammation-mediated programmed cell death
In the ICM, the activation and amplification of the inflammatory response leads to programmed cell death of vascular ECs and inflammatory cells (such as macrophages and neutrophils), which involves mainly apoptosis, necroptosis, pyroptosis, ferroptosis and NETosis. Under oxidative stress, mitochondria produce excessive ROS and determine cell fate, which is the central link between inflammation-mediated apoptosis, necroptosis, pyroptosis, ferroptosis and other cell death pathways (Basit et al., 2017). Together, these processes lead to vascular tissue damage, plaque calcification, lesion thrombosis, and coronary artery stenosis (Zhang A. et al., 2023; Jiang et al., 2024; Lu et al., 2024), exacerbating the progression of ICM.
In coronary artery ECs, inflammation-mediated apoptosis is generally considered to be an indirect effect, that is, by inducing the explosive production of ROS, causing the destruction of mitochondrial membrane components, activating Bax, inducing the increase of mitochondrial membrane permeability and cytochrome C (cyt C) release (Salie et al., 2023). Cyt C binds to the C-terminal domain of apoptotic protease activating factor-1 (Apaf-1), induces conformational changes, activates the release of caspase-9 and caspase-3, and ultimately accelerates the apoptosis of ECs (Miao et al., 2018; Xue et al., 2023).
Necroptosis is programmed cell death induced by a class of death receptors (TNFR1, Fas and TRAIL-R). The inflammatory factor TNF-α can initiate necroptosis through TNFR1 (Nam et al., 2024). By binding to the extracellular part of TNFR1, TNF-α causes allosteric changes in the intracellular part of TNFR1 (Huang et al., 2022) and mediates the activation of downstream molecules such as receptor-interacting serine threonine kinase 1 (RIPK1), RIPK3, Fas-associated with death domain protein (FADD)/TNF receptor-associated death domain protein (TRADD), and caspases 8/10 (Xiao et al., 2020). Subsequently, RIPK1, RIPK3, and mixed lineage kinase domain like protein (MLKL) form a necrotic complex, which promotes the opening of the mitochondrial mPTP (Szobi et al., 2018; Qiang et al., 2022; Li Z. et al., 2023), mediates mitochondrial damage, and ultimately induces cell death (Pozzer et al., 2019). In addition, recent studies have shown that RIPK1 can drive NF-κB-dependent inflammation in early atherosclerotic lesions and mediate the initiation of necrotic apoptosis in macrophages and ECs (Karunakaran et al., 2021). At the same time, the expression of RIPK1 is also a key link in the process of TNF-induced necroptosis (Newton et al., 2019).
Pyroptosis is a form of cell death that is dependent on caspase-1 activation and is widely involved in the occurrence, progression and complications of ICM (Jin et al., 2023). It is characterized by rapid rupture of the plasma membrane and the release of proinflammatory substances in cells. Recent studies have shown that the classical pathway of pyroptosis is mediated by the NLRP3 inflammasome in ICM vascular injury (Shao et al., 2022; Li S. et al., 2024; Yang et al., 2024). The NLRP3 inflammasome is a macromolecular protein complex, and its three key components include the NLRP3 protein, apoptosis-associated speck-like protein containing a CARD (ASC), and procaspase-1. It can sense damage by activating caspase-1, and then stimulate the release of proinflammatory cytokines IL-1β and IL-18 and the cleavage of Gasdermin D (GSDMD), triggering and amplifying the inflammatory response. This process is one of the drivers of pyroptosis (Toldo and Abbate, 2024). Mitochondrial damage and dysfunction will increase the production of ROS and ox-LDL and K+ efflux (Jiang et al., 2023; Kan et al., 2024; Tao et al., 2024), which trigger the inflammatory response of NLRP3, induce pyroptosis of inflammatory cells and ECs, and aggravate vascular endothelial damage.
Ferroptosis is a new type of programmed cell death that has attracted wide attention in recent years. Excessive accumulation of lipid peroxides leads to cell death in the case of iron-dependent overload. Iron metabolism disorders, oxidative stress, lipid peroxidation, targeted induction of P53, and other factors are involved in the occurrence of ferroptosis. Studies have shown that TNF-α may be involved in the process of promoting ferroptosis in vascular ECs (Chen X. et al., 2023; Jankauskas et al., 2023). In addition, ferroptosis has been shown to be involved in the drive of proinflammatory response (Hou H. et al., 2024). Iron overload in the arterial wall results in increased expression of cytokines, increased expression of ICAM-1 and VCAM-1, extensive endothelial defects and increased permeability, and excessive oxidative damage (Vinchi et al., 2020). Ferroptosis inhibitors can reduce subsequent immune cell infiltration and the inflammatory response (Li et al., 2019; Zhu et al., 2024), which may have a timely preventive effect on the extensive vascular injury caused by inflammation.
NETosis is a neutrophil-specific form of programmed cell death involving the release of neutrophil extracellular traps (NETs) (MacArthur et al., 2024). NETs are reticular structures composed of DNA, histones and various antimicrobial proteins. They are released by neutrophils to capture and destroy extracellular pathogens such as bacteria and fungi. During NETosis, neutrophils undergo a series of morphological changes, leading to cell membrane rupture, and NETs are released into the surrounding environment, inducing the activation of ECs, antigen-presenting cells and platelets, leading to local tissue inflammation and promoting vascular injury and thrombosis (Sun et al., 2023). Moreover, dysfunctional ECs will enhance NETosis, amplify the damage to adjacent cells, and further damage the inner layer of the arterial endothelium (Nija et al., 2020). Peptide arginine deiminase 4 (PAD4) is a key enzyme in NETosis, which mediates the citrullination of histones and the decondensation of chromatin, resulting in DNA extrusion and NETs formation. Studies have shown that in the Ldlr−/− mouse model of blood flow-mediated superficial intimal erosion, the inactivation of the PAD4 gene can eliminate NETosis, reduce endothelial permeability, and reduce in situ thrombosis (Dou et al., 2021). In addition, a decrease in TUNEL+ apoptotic ECs was observed in mice treated with Pad4−/− bone marrow transplantation or PAD4 inhibitors (Franck et al., 2018). Recent studies have also shown that targeted delivery of PAD4 inhibitors in ApoE−/− mouse models can reduce the accumulation of NETs at the site of intimal injury and maintain endothelial continuity (Molinaro et al., 2021).
3 ER interacts with vascular physiology and pathology
3.1 ER physiological regulatory mechanism
The ER is a multifunctional organelle that plays an important role in a variety of physiological processes, which is involved in protein synthesis, folding and translocation, and regulates cellular Ca2+ uptake, storage and signalling. In addition, the ER is involved in the production of cellular lipids, such as cholesterol, glycerophospholipids, and ceramides, as well as the regulation of gene expression and energy metabolism, and the transmission of signals to the nucleus, cytoplasm, mitochondria, and plasma membrane (Dong et al., 2024).
Increased physiological and pathological changes, including fluctuations in intracellular Ca2+ levels, genetic or environmental damage, oxidative stress, inflammatory responses, and glycosylation, may disturb the steady state of the ER and impair its ability to fold and posttranslationally modify proteins. When the load of secreted proteins exceeds the folding capacity of the endoplasmic reticulum, it leads to the accumulation of misfolded proteins, which is called endoplasmic reticulum stress (ERS). In this case, transcription-induced endoplasmic reticulum chaperone genes such as glucose-regulated protein 78 (GRP78)/binding protein (BiP) or glucose-regulated protein 94 (GRP94) are activated to promote folding ability. ER-associated protein degradation pathway (ERAD) and the unfolded protein response (UPR) are activated to accelerate the degradation of unfolded proteins. Endoplasmic reticulum remodelling and ER autophagy will be initiated to remove excess ER membranes and proteins, fight ERS, and maintain normal ER structure and function (Figure 2).
3.2 ER is involved in regulating vascular endothelial physiology
The vascular endothelium is a unique, dynamically regulated organ composed of monolayer ECs that regulate blood flow and fibrosis, vascular tone, angiogenesis, vascular permeability, leukocyte adhesion, and platelet aggregation. It is essential for maintaining vascular homeostasis and adapting the cardiovascular system to environmental changes. Ca2+ is a ubiquitous secondary messenger that initiates signal transduction events in ECs. It regulates the synthesis and release of various vasoactive substances, such as nitric oxide (NO), prostacyclin (PGI2), endothelium-derived hyperpolarizing factor (EDHF), and endothelin, so that ECs can achieve normal physiological functions such as the regulation of vascular tension, endothelial permeability, and angiogenesis. As one of the intracellular organelles that regulate the uptake and storage of Ca2+, the ER plays an important role in the physiological regulation of the vascular endothelium (Suzuki et al., 2014). The ER in vascular ECs mainly controls Ca2+ efflux through inositol triphosphate receptor (IP3R) and Ca2+ influx through sarco/endoplasmic reticulum Ca2+-ATPase (SERCA) (Cohen and Jackson, 2005; Yan et al., 2015). Studies have shown that IP3R is involved in the negative feedback regulation of small artery muscle tone by regulating endothelial cell Ca2+ levels (Cohen and Jackson, 2005; Ledoux et al., 2008). Under the regulation of NO, SERCA can reduce the intracellular free Ca2+ by increasing the uptake of Ca2+ and play the role of reducing vascular tension (Adachi et al., 2002; Adachi et al., 2004). SERCA overexpression has been shown to have protective effects on endothelial function and barrier integrity in many studies (Li et al., 2020a; Tan et al., 2020). In addition, a Ca2+-binding protein with high affinity and Ca2+ buffering capacity, calreticulin (CRT), is present in the ER and plays a supportive role in adequate ER Ca2+ storage and stable Ca2+ levels (Wang et al., 2018; Wang et al., 2019b).
3.3 ER is involved in the mechanism of vascular endothelial pathological injury
The ER is involved mainly in vascular endothelial pathological damage through Ca2+ homeostasis and ERS.
Adverse factors such as high glucose, high fat, ischemia and hypoxia, and inflammatory infiltration that may exist in the pathological state of the ICM can stimulate the abnormal expression of IP3R and SERCA in the ER, causing a rapid increase in intracellular Ca2+ levels (Qin et al., 2014; Zhang et al., 2016; Yu et al., 2017; Zhou et al., 2018; Chen et al., 2021). This leads to Ca2+ -dependent xanthine oxidase (XO) activation and ROS production, which in turn triggers endothelial cell apoptosis (Liu M. et al., 2024). SERCA overexpression can reduce cardiac microvascular endothelial cell death and exert a protective effect against cardiac microvascular ischemia/reperfusion injury by improving mitochondrial quality control and inhibiting the expression of mitochondrial calcium-monotransporter protein (MCU) (Li et al., 2020a; Tan et al., 2020). In addition, inhibition of IP3R expression reduces microvascular damage caused by Ca2+ overload and related oxidative stress (Zhu et al., 2018).
At the same time, the above adverse factors and Ca2+ homeostasis itself are the reasons for the continuous accumulation of misfolded proteins. When the accumulation of misfolded proteins in the lumen of the ER is excessive and beyond the normal physiological regulation, the ERS and the persistence of the UPR have an impact on the cells and radicalize the pathological process.
In ICM vascular endothelial injury, ERS and UPR are involved in mediating the inflammatory response, oxidative stress and cell death (Wu L. et al., 2014; Huang et al., 2018; Wang F. et al., 2019). In particular, the UPR is controlled by three ER transmembrane proteins: protein kinase R-like endoplasmic reticulum kinase (PERK), inositol-requiring enzyme 1α (IRE1α), and activating transcription factor 6 (ATF6). Endoplasmic reticulum stress and subsequent endothelial cell apoptosis, involving activation of eukaryotic initiation factor 2α (EIF2α), X-box-binding protein 1 (XBP1), CCAAT-enhancer-binding protein homologous protein (CHOP), and NF-κB, can induce endothelial dysfunction. Correspondingly, ERS inhibition improves endothelial cell function directly or indirectly (Zhou et al., 2023). Upon UPR activation, IRE1α elevates the expression of TNF receptor-associated factor 2 (TRAF2), which, together with JNK and IκB kinases, initiates NF-κB and activates the expression of inflammatory cytokines (Lei et al., 2023). The ATF6 pathway has also been shown to trigger the NF-κB pathway (Nakajima et al., 2011; Chen et al., 2018). The IRE1 pathway induces an increase in thioredoxin-interacting protein (TXNIP), activates NLRP3 inflammatory vesicles, and promotes secretion of IL-1β and IL-18 (Hong et al., 2021). In addition, sustained ERS drives macrophage polarization to proinflammatory M1 morphology, causing organ inflammation during obesity and insulin resistance (Shan et al., 2017). Studies have shown that excess ER cholesterol triggers the macrophage UPR and activates the IκB/NF-κB, MAP kinase 3 (MKK3)/p38, extracellular regulated protein kinases (Erk) 1/2, and JNK1/2 signalling pathways, leading to elevated expression of the inflammatory molecules IL-8, IL-6, MCP-1 and TNF-α. This may be one of the mechanisms that exacerbates the vascular inflammatory response and macrophage accumulation in advanced atherosclerosis and ICM (Li et al., 2005).
ERS can activate ROS production in several ways: i) Activating of NOX, especially NOX2 and NOX4 (Lee et al., 2014; Vilas-Boas et al., 2021). ii) Ca2+ leaks from the ER lumen into the cytosol, which can increase mitochondrial ROS production (Zhang et al., 2016). iii) Increased energy consumption by the ER during protein folding and refolding stimulates mitochondrial oxidative phosphorylation to increase ATP and reactive oxygen species production, especially O2−. O2− can combine with NO synthesized by endothelial NO synthase (eNOS) to form peroxynitrite, leading to impaired endothelium-dependent vasodilatation and decreased NO bioavailability, which in turn leads to endothelial dysfunction (Cheng et al., 2021; Hassoun et al., 2021). Conversely, oxidative stress may similarly promote ERS directly or indirectly. Excess mitochondrial ROS promote ER Ca2+ release and activate ERS (Kim et al., 2016). In addition, ox-LDL, a product of ROS-promoted lipid peroxidation formation, attenuates cholesterol efflux from ECs, upregulates the expression of ERS-associated proteins (CHOP, p-PERK, GRP78, and p-IRE-1) (Hang et al., 2020), and mediates the onset of ERS, the eruption of an inflammatory response, and, ultimately, endothelial cell apoptosis (Li P. et al., 2021). Promoting SOD1 through activation of Nrf2, which in turn counteracts ROS production, effectively alleviates ERS-induced endothelial cell apoptosis (Ei et al., 2022). These studies all support the interaction and synergy between oxidative stress and ERS, with ROS being both a downstream product of ERS and a regulator of ERS.
High levels of chronic ERS induce apoptosis through all three branches of the UPR. IRE1α initiates apoptosis via TRAF2 (Ma et al., 2023). The IRE1α cytoplasmic structural domain recruits TRAF2, which activates apoptosis signal-regulated kinase 1 (ASK1)/cJUN nh2-terminal kinase (JNK) (Ji et al., 2020; Kang et al., 2024). By stimulating the proapoptotic protein Bax and inactivating the antiapoptotic protein Bcl-2, JNK plays a role in apoptosis. PERK/EIF2α and ATF6 activate an increase in the level of the proapoptotic transcription factor CHOP, which initiates apoptosis by downregulating the expression of Bcl-2 and activating the cascade of caspase-12/9/3 (Peng et al., 2022; Li H. et al., 2024). CHOP also stimulates endoplasmic reticulum Ca2+ release and triggers the calcium-sensitizing enzyme CaMKII, which induces a variety of downstream apoptotic mechanisms such as Fas death receptor, JNK activation, and the release of mitochondrial cytochrome c, triggering apoptosis (Dilshara et al., 2018; He et al., 2021; Mohan et al., 2023). In addition, it has also been recently shown that ERS is also involved in activating other forms of cell death. Under hypoxia induction, ERS may also be involved in the autophagy of ECs through the IRE1-mediated UPR (Tao et al., 2023). Cigarette tar mediates RIPK3-dependent necroptosis and accelerates the progression of atherosclerosis by activating the ERS PERK/EIF2α/CHOP axis in vascular smooth muscle cells (Bai et al., 2024).
4 Mitochondria interact with vascular pathology
Mitochondrial quality control (MQC) is a group of adaptive responses that regulate mitochondrial dynamics, mitophagy, and mitochondrial biogenesis (Figure 3). It is an important mechanism to ensure mitochondrial homeostasis and maintain vascular endothelial stability (Liu et al., 2023; Ronayne et al., 2023; Tahmaz et al., 2023).
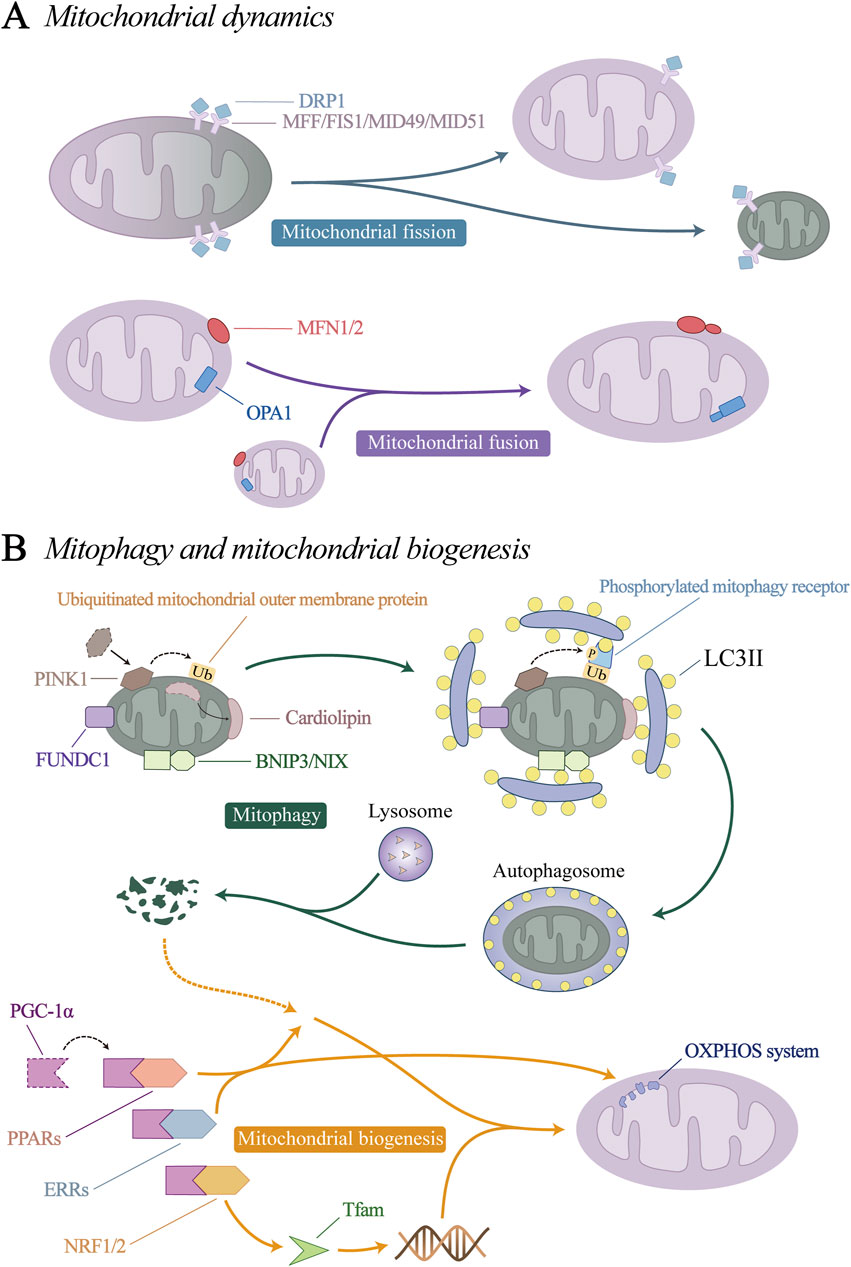
Figure 3. Mitochondrial quality control (MQC) mechanism. (A) Mitochondrial dynamics. (B) Mitophagy and mitochondrial biogenesis.
4.1 Mitochondrial dynamics and vascular pathology
Mitochondrial dynamics consists of mitochondrial fission and fusion processes. Through constant division and fusion, mitochondria maintain their integrity, distribution and size.
Mitochondrial fission can remove dysfunctional or damaged mitochondria from the mitochondrial network, which is important for maintaining the overall homeostasis and function of mitochondria (Suen et al., 2010; Kalkhoran et al., 2022). Mitochondrial fusion is a process of integrating several mitochondrial fragments into filamentous mitochondria, which can maintain the balance of mitochondrial components and the stability of function, weaken the damage to mtDNA and protein, and prevent the excessive division of mitochondria from causing apoptosis (Pirzeh et al., 2019; Gao et al., 2023; Noone et al., 2023). Mitochondrial dynamics not only determines mitochondrial homeostasis in many cell types, but also is considered to be a key requirement for vascular endothelial stability under normal conditions.
Mitochondrial fission is regulated by dynein-related protein 1 (DRP1) and its receptor mitochondrial fission factor (MFF), mitochondrial fission 1 (FIS1), mitochondrial dynamics protein 49 kDa (MID49) and MID51 (Ke et al., 2023; Ren et al., 2023). Under physiological conditions, DRP1 is mainly free in the cytoplasm in an inactive form, so mitochondrial fission is relatively rare. Under stress conditions, DRP1 undergoes conformational changes through posttranscriptional modification, such as ubiquitination, acetylation, and phosphorylation (Wang X. et al., 2019; Hu et al., 2023; Li et al., 2023c). Its binding sites are exposed and transported to the mitochondrial surface to bind to its receptors, where it mediates mitochondrial fission.
Mitochondrial fusion involves two processes. Mitofusin 1 and 2 (MFN1, MFN2) are located on the mitochondrial outer membrane (OMM) and mediate OMM fusion through homotypic or heterotypic coordination (Noone et al., 2023). Optic atrophy 1(OPA1) mediates endosomal fusion, which exists in two different forms, the long isomer of OPA1 (L-OPA1), and the short isomer (S-OPA1). Under the action of yeast mitochondrial escape 1 like 1 ATPase (YME1L1) and OMA1 zinc metallopeptidase (OMA1), L-OPA1 can be hydrolysed to S-OPA1 (Consolato et al., 2018; Jian et al., 2023). L- and S-OPA1 homeostasis coordinates to promote mitochondrial inner membrane fusion (Ge et al., 2021; Duan et al., 2023).
Studies have shown that mitochondrial dynamics are involved in mediating vascular pathology in the ICM, mainly through excessive mitochondrial fission, resulting in endothelial cell dysfunction and the development of vascular injury, degeneration, and fibrosis. In vitro studies have shown that DRP1 and FIS1 accumulate in HAECs exposed to high-glucose environments (Morales et al., 2014). In contrast, inhibition of DRP1 or FIS1 reduced mitochondrial fragmentation, attenuated mitochondria-derived ROS release, suppressed vascular inflammation and ameliorated endothelial dysfunction (Wang et al., 2017). MiD49/51 expression was elevated in aortic valve ECs from ApoE−/− mice with high-fat diet-induced atherosclerosis, which accelerated DRP1-mediated mitochondrial fission. Silencing MiD49/51 reduces atherosclerotic plaque size and increases collagen content (Ren et al., 2023). In addition, animal studies provide evidence that acute microvascular I/R injury in mice is accompanied by increased MFF expression, excessive mitochondrial division, and mitochondria-dependent microvascular endothelial cell apoptosis. Compared with wild-type mice, MFF-deficient mice presented more stable coronary blood flow, less microcirculatory perfusion injury, a smaller infarct size, and preserved cardiac function during acute microvascular I/R injury (Zhou et al., 2017a). Clinical evidence suggests that mitochondrial breaks, mitochondrial ROS levels, and FIS1 expression are increased in isolated venous ECs from diabetic patients with one of the cardiovascular risk factors, hyperglycemic state, compared to healthy subjects, which may contribute to the induction of cardiovascular pathologic changes (Shenouda et al., 2011).
In contrast, mitochondrial fusion has been noted in studies to play a role in delaying vascular injury and ICM progression. The mitochondrial fusion proteins MFN1 and MFN2 maintain the mitochondrial network and protect endothelial cell function by initiating OMM fusion.Reduced expression of MFN1 and MFN2 leads to endothelial dysfunction and inhibition of VSMC proliferation, promoting the progression of atherosclerosis (Lugus et al., 2011; Forrester et al., 2020). Knockout of MFN2 impairs gene expression of mitochondrial respiratory chain and oxidative metabolism-related transcription factors, while knockout of MFN1 reduces Akt-mediated eNOS expression and inhibits endothelial cell activity (Lugus et al., 2011). Animal studies have shown that during cardiac microvascular I/R, the expression of MFN2 and OPA1 mRNA in mouse cardiac microcirculation vascular ECs was reduced, mitochondrial fusion was inhibited, vascular lumen was narrowed, vascular wall thickening, and the risk of thrombosis were increased (Tan et al., 2020). A fish oil-rich diet can improve endothelium-dependent vascular relaxation and delay the progression of atherosclerosis by upregulating the expression of MFN2 and OPA1 in aortas of ApoE−/− mice fed with high-fat diet (Sun et al., 2014). In addition, the clinical study of Diaz-Morales et al. (Diaz-Morales et al., 2016) reported that compared with those in healthy volunteers, the mitochondrial fusion-related proteins MFN1/2 and OPA1 were significantly downregulated in circulating leukocytes isolated from diabetic patients. The decrease in leukocyte mitochondrial fusion and the increase in mitochondrial fission in diabetic patients were related to an increase in leukocyte-endothelial cell interaction, indicating that a higher degree of vascular inflammation is inseparable from the imbalance of endothelial cell mitochondrial dynamics.
4.2 Mitophagy and vascular pathology
Mitophagy is a type of organelle autophagy that prevents the accumulation of abnormal mitochondria (Jia et al., 2023). After mitochondrial degradation, amino acids and fatty acids are recycled to ensure the continuous growth and division of the existing mitochondrial network through mitochondrial biogenesis to meet the energy needs of cells (Wang L. et al., 2023). Mitochondrial autophagy is coordinated with mitochondrial biogenesis, ensuring a healthy mitochondrial network through the recycling of mitochondrial components (Wang et al., 2024).
Current researches have identified four initiation pathways for mitophagy: PTEN induced putative kinase 1 (PINK1)/Parkin pathway, BCL2/adenovirus E1B 19 kDa protein-interacting protein 3 (BNIP3)/NIP3-like protein X (NIX) pathway, the FUN14 domain containing 1 (FUNDC1) pathway, and the cardiolipin pathway. When mitophagy is initiated by the above-mentioned key proteins that initiate mitophagy, the autophagy-related protein microtubule-associated protein 1A/1B-light chain 3 (LC3) is processed into its cytoplasmic-localized LC3I, which is then combined with phosphatidylethanolamine (PE) on the phagosome and outer membrane to form LC3II (Chang et al., 2012; Zhu et al., 2022). LC3II binds to the mitophagy receptor protein, prompting the target mitochondria to be phagocytosed by phagosomes to form autophagosomes. Finally, lysosomes induce the hydrolysis and degradation of autophagosome proteins, nucleic acids and lipids, which are recovered by cells to restore balance.
Under pathological conditions, mitophagy is generally considered to be a protective mechanism. When damaged mitochondria cannot be repaired by mitochondrial fission or fusion, mitophagy is activated to remove damaged mitochondria and prevent the apoptosis caused by excessive mitochondrial damage. During atherosclerosis, excessive ROS production in macrophages has been shown to activate NLRP3 inflammasome formation and cause mitochondrial damage. Promoting Parkin-mediated mitophagy can reduce mitochondrial ROS production and NLRP3 inflammasome-induced mitochondrial damage and inhibit oxidative stress and the inflammatory response (Jin et al., 2022a). The inhibition of BNIP3-related mitophagy exacerbates oxidative stress-induced vascular injury and promotes osteoblast phenotype transformation and calcium deposition in vascular smooth muscle cells (Zhu et al., 2020). In the process of cardiac microvascular I/R, it was also found that the number of mitochondrial-lysosomal complexes in mouse cardiac microcirculation vascular ECs was significantly reduced, and the expression of Parkin was decreased, indicating that mitophagy was inhibited. The activation of mitophagy can protect ECs (Li et al., 2020a). However, some studies have shown that excessive activation of mitophagy under pathological conditions may play a role in promoting cell death. In a study of microcirculatory I/R, I/R activated Drp1-dependent mitochondrial fission, followed by upregulation of PINK1/Parkin, which was mediated by voltage-dependent anion channel 1 (VDAC1)/hexokinase 2 (HK2), and ultimately activated mitophagy-mediated cardiac thrombotic ECs death (Zhou et al., 2017b). An appropriate amount of mitophagy plays a protective role in the process of vascular pathology and helps to maintain the normal function and cell viability of vascular ECs. When mitophagy is overactivated, or still unable to correct mitochondrial damage, it has a synergistic trend with vascular endothelial damage.
4.3 Mitochondrial biogenesis and vascular pathology
Mitochondrial biogenesis is a dynamic process that stabilizes mitochondria to maintain their structure. Mitochondrial biogenesis is activated to ensure the function and quantity of normal cellular metabolism when the demand for cellular energy metabolism increases, or when increased activity or proliferation is required. Peroxisome proliferator-activated receptor coactivator (PGC-1α) is considered to be the main regulator of mitochondrial biogenesis, and can activate the expression of a variety of downstream transcription factors, including nuclear respiratory factors (NRF1/2), peroxisome proliferator-activated receptors (PPARs) and estrogen-related receptors (ERRs). NRF1/2 promotes the expression of nuclear-encoded mitochondrial transcription factor A (Tfam), which is responsible for the transcription of mtDNA (Gleyzer et al., 2005; Xiang D. et al., 2022). PPARs and ERRs are involved in the generation of nuclear proteins and control many aspects of mitochondrial oxidative metabolism, including fatty acid transport and oxidation, glucose utilization, the TCA cycle, and oxidative phosphorylation (OXPHOS) (Kim et al., 2021; Li Z. et al., 2021; McMeekin et al., 2021; Tian et al., 2023).
Under various pathological stimuli during ICM, mitochondrial biogenesis function is mainly impaired. Studies have shown that under constant high glucose induction, the level of ROS in ECs increases, the activation of PGC-1α is inhibited (Abdelzaher et al., 2016), and the expression levels of NRF1 and TFAM decrease (Xu et al., 2014). After exposure to cardiac microvascular I/R injury, the expression of PGC-1α and Tfam in mouse cardiac microcirculation vascular ECs is inhibited, and the function of mitochondrial biogenesis is impaired (Tan et al., 2020). In oxygen-glucose deprivation-mediated endothelial cell injury, promoting the activation of PGC-1α via therapeutic measures can upregulate the activity and expression of eNOS and improve vasodilation function (Wei et al., 2019). In addition, studies have shown that hypoxia/reoxygenation injury induces increased mitochondrial fission, decreased fusion, and impaired mitochondrial biogenesis in microvascular ECs. The activation of mitophagy can improve these conditions, upregulate SIRT3 and PGC-1α, and restore endothelial cell viability and proliferation (Wu et al., 2022). The results of this study reflect the mutual regulation between mitochondrial quality control links in the pathological process. Mitochondrial dynamics maintains the stability of mitochondrial morphology and function, while the cycle of mitophagy and mitochondrial biogenesis effectively promote the recovery of damaged mitochondria and the timely supplement of mitochondrial number. By regulating some of these links, ICM intervention and treatment can be achieved.
5 Targeted therapy of natural medicines
Studies have shown that many Chinese herbal medicines and their natural medicinal active ingredients have MQC and ER protection functions, which can resist oxidative stress, alleviate vascular inflammation, directly or indirectly improve vascular endothelial function and reduce vascular injury (Table 1).
5.1 Mitochondrial quality control protection function
The mechanisms involved in mitochondrial dynamics, mitophagy and mitochondrial biogenesis mentioned above are all possible targets for natural drugs to improve mitochondrial quality control disorders during ICM.
For mitochondrial dynamics, natural drugs mainly inhibit excessive mitochondrial fission in pathological conditions by regulating the expression of DRP1 and FIS1 or promote mitochondrial fusion by regulating the expression of MFN1, MFN2 and OPA1, thereby exerting a protective effect on mitochondria and cells. Corosolic acid is a natural triterpene with antioxidant activity. In ECs, it activates AMPK in a IKB1-dependent manner, induces phosphorylation of the Ser637 site of DRP1, thereby inhibiting mitochondrial fission and resisting subsequent oxidative stress. Oral administration of corosolic acid in high-fat diet mice can replicate similar regulation in aortic endothelium (Li et al., 2016). Diallyl trisulfide is an organic polysulfide found in Allium sativum L. Studies have shown that Diallyl trisulfide can inhibit high glucose-induced HUVECs apoptosis in an AMPK-dependent manner by inhibiting DRP1-mediated mitochondrial fission (Hao et al., 2019). Isorhapontigenin is extracted from Gnetum cleistostachyum C.Y.Cheng, and has been proved to have good anti-inflammatory and anti-oxidative stress effects in many studies. Chen Y. et al., 2023) pointed out that isorhapontigenin promoted mitochondrial fusion through peroxiredoxin 2 (PRDX2)/MFN2, inhibited ACSL4-mediated ferroptosis of mitochondria-associated ECs and improved microvascular density and perfusion in db/db mice. Polydatin, which is extracted from Polygonum cuspidatum Sieb. et Zucc., enhances DRP1-mediated mitochondrial fission, reduces ROS production, and improves pyroptosis of HUVECs and the aorta in diabetic rats through the NLRP3/Caspase1/IL-1β pathway (Shah et al., 2023).
Buyang huanwu decoction (BYHWD, a TCM prescription) has been shown to reduce mitochondrial fission (decreased expression levels of DRP1 and FIS1) through AMPK activation, reduce ROS production, and improve NO production in diabetic ApoE mouse models and HUVECs exposed to high glucose (Tong et al., 2023). Shuangshen ningxin formula, another TCM prescription, has also been shown to reduce mitochondrial fission by inhibiting the nuclear receptor subfamily 4 group A member 1 (NR4A1)/MFF/Drp1 pathway in vivo and in vitro experiments, and plays a protective role in cardiac microvessels (Liu Z. et al., 2024).
Owing to the “double-sided” characteristics of mitophagy, either overexcitation or inhibition of mitophagy may be responsible for the pathological state. Thus, there are two trends in the action of natural drugs. Scutellarin is one of the active components of Scutellariae Radix. It can upregulate mitophagy through the PINK1/Parkin signalling pathway, fight against excessive ROS production, and protect vascular ECs from hyperglycemia-induced damage (Xi et al., 2021). Ginseng-Sanqi-Chuanxiong (GSC) extracts, a composite extract of Ginseng Radix Et Rhizoma, Notoginseng Radix Et Rhizoma, and Chuanxiong Rhizoma, was shown to activate mitophagy via the AMPK pathway, and was able to reduce the significant increase in mitochondrial ROS levels in HAECs under high glucose and palmitate stress conditions (Wang et al., 2020). Shenlian extract is a combination of Salviae miltiorrhizae Radix et Rhizoma and Andrographis Herba. In vivo and in vitro experiments have shown that Shenlian extract can regulate mitochondrial dysfunction and protect microvascular function by inhibiting mitophagy via the PINK/Parkin pathway (Li J. et al., 2023). Yi Mai granule is a TCM combination preparation, which regulates proinflammatory factors and blood lipid levels by promoting PINK1/MFN2/Parkin-mediated mitophagy, plays an endothelial protective role in vivo and in vitro experiments (Kong et al., 2024).
In terms of mitochondrial biogenesis, the mitochondrial biogenesis factors PGC-1α, Nrf-1, and Tfam are the main intervention targets of natural drugs and their monomers. Improvements in mitochondrial quality, mtDNA content, ATP production, oxidative phosphorylation function, and antioxidant capacity are the main follow-up effects. In the fight against ICM inflammatory vascular injury, improving mitochondrial biogenesis seems to be one of the most important ways for natural drugs to exert their efficacy.
As the main active ingredient of Coptidis Rhizoma, berberine has been shown to promote mitochondrial biogenesis in an AMPK-dependent manner in ApoE−/− mice, increase the level of the mitochondrial-derived ROS regulator uncoupling protein 2 (UCP2), and resist oxidative stress (Arsenijevic et al., 2000; Wang et al., 2011). (−)-Epicatechin is a natural flavanol compound that enhances citrate synthase activity in bovine coronary artery ECs, increases the levels of oxidatively phosphorylated proteins (complexes I and II), activates mitochondrial biogenesis, and stimulates mitochondrial function (Moreno-Ulloa et al., 2013). In addition, (−)-epicatechinis ameliorated the hyperglycemia-induced decrease in eNOS activity in HCAECs by activating mitochondrial biogenesis pathway (Ramírez-Sánchez et al., 2016), and was found to regulate the activity of mitochondrial complexes and reduce high glucose-mediated increasing ROS levels in HUVECs (Keller et al., 2020).
Chlorogenic acid, hydroxytyrosol and punicalagin are all natural polyphenolic compounds that are widely found in a variety of natural plant medicines. Chlorogenic acid pretreatment can increase the activity level of SIRT1 deacetylase, reverse the SIRT1/AMPK/PGC-1 activity damaged by ox-LDL, and reduce the degree of oxidative stress in HUVECs (Tsai et al., 2018). Hydroxytyrosol has been shown to promote mitochondrial biogenesis, increase mtDNA content, and attenuate endothelial dysfunction and pathological angiogenesis (Calabriso et al., 2018). Punicalagin, which is extracted from Punica granatum L., can promote mitochondrial biogenesis through the forkhead box O1 (FOXO1) pathway and improve hyperlipidemia-induced endothelial dysfunction (Liu et al., 2019). Vanillic acid is a phenolic acid compound widely distributed in natural plants. In palmitic acid-stimulated HUVECs, vanillic acid promoted the expression of p-Nrf2, HO-1, SIRT1, and PGC-1α through the LKB1/AMPK signalling pathway, and reduced the levels of ROS and malondialdehyde (MDA) to alleviate oxidative stress (Ma et al., 2019). Naringenin is a natural flavonoid widely distributed in plants. Its treatment can increase the expression of genes related to mitochondrial biogenesis (SIRT1, FOXO3a and PGC1α) in the aorta of ApoE−/− mice and improve vascular aging and atherosclerosis (Wang J. et al., 2023).
Dangua Fang, a TCM compound, can increase the expression of PGC-1α in ECs, regulate mitochondrial respiratory chain function, restore the mitochondrial membrane potential (MMP), and resist oxidative stress-induced endothelial cell injury (Xianpei et al., 2022).
In addition, the effects of some natural medicines on various aspects of MQC mechanisms have been comprehensively studied.
Resveratrol is a natural polyphenolic compound found in a variety of plants such as Polygoni Cuspidati Rhizoma et Radix and Vitis. In vitro studies revealed that resveratrol was able to increase the mitochondrial mass and mtDNA content and regulate the bioavailability of NO by inducing the upregulation of mitochondrial biogenesis factors (PGC-1α, Nrf-1, and Tfam) in HCAECs. Long-term resveratrol treatment corrects damage to mitochondrial biogenesis in the aorta of type 2 diabetic mice (Csiszar et al., 2009). Similarly, in HUVECs, the co-administration of resveratrol and equol, a natural flavonoid compound, induced the expression of the mitochondrial biogenesis factors PGC1-α, Tfam, and Nrf-1 via SIRT1, and increased mitochondrial mass and mitochondrial DNA content (Davinelli et al., 2013). In addition, resveratrol increased the protein levels of MFN1, MFN2 and OPA1, promoted mitochondrial fusion, and resisted palmitic acid-mediated oxidative damage to ECs (Yang et al., 2019). It can upregulate BNIP3-related mitophagy through HIF1/AMPK signaling pathway and prevent ox-LDL-mediated mitochondrial respiratory complex inactivation to promote endothelial cell survival (Li et al., 2020b).
Ferulic acid is the main active ingredient of Chuanxiong Rhizoma. It can inhibit the downregulation of vascular endothelial mitochondrial biogenesis markers (PGC-1α, PGC-1β, and NRF-1) and the decrease in fusion (Mfn1and Mfn2 levels decreased) and fission (FIS1 levels decreased) in ApoE−/− mice induced by a high-fat diet, thereby inhibiting the development of aortic atherosclerotic plaques and oxidative stress in mice. Clinical studies have shown that the intake of ferulic acid in healthy volunteers can reduce the activity of NADPH oxidase, superoxide release and apoptosis of peripheral blood monocytes, and improve the differentiation and proliferation of endothelial progenitor cells (Perez-Ternero et al., 2017).
5.2 ER protection function
The TCM plays an ER protection role mainly by resisting ERS. It can also inhibit the upstream and downstream oxidative stress of ERS and subsequently mediate the inflammatory response and programmed cell death and reduce vascular injury. Its ER protection function is reflected mainly in the regulation of ERS markers.
Taurine is one of the active components of Bovis Calculus, a natural Chinese medicine derived from animals, and is also found in a variety of animal foods. Studies have shown that taurine can improve homocysteine-induced vascular smooth muscle cells (VSMCs) ERS, downregulate the expression of GRP78 mRNA, inhibit PERK, and restore extracellular superoxide dismutase (EC-SOD) secretion to reduce oxidative stresss (Nonaka et al., 2001). In in vivo experiments, dietary taurine supplementation can reduce the expression of CHOP protein in ECs, inhibit the ERS-mediated apoptosis of ECs, and reduce the atherosclerosis of left main coronary artery in rabbits (Zulli et al., 2009). Ursodeoxycholic acid (UDCA) is a widely used drug and the main active ingredient of the precious traditional animal medicine Fel Ursi. A study by Chung et al. (2015), Chung et al. (2016) revealed out that UCDA blocked ERS and its downstream signalling pathways (p-PERK, XBP1, ATF6 and CHOP) in high glucose-treated ECs, inhibited subsequent oxidative stress and pro-inflammatory responses (ROS production and NF-κB activation), and inhibited the formation of atherosclerotic plaques. In in vivo experiments, UCDA has been shown to exert anti-atherosclerotic activity by inhibiting ERS in atherosclerotic mouse models caused by blood flow disorders and diabetic mouse models. Propolis is a colloidal substance formed by mixing plant resin collected by bees with secretions such as maxillary glands and wax glands and a small amount of pollen. It has a long history of application in traditional medicine. A study by Hua et al. (Tian et al., 2015) showed that ethanol extract of propolis inhibited ERS/CHOP pathway-mediated apoptosis by reducing the expression of PERK, eIF2α, GRP78 and CHOP, and played a protective role in ox-LDL-induced macrophage toxicity.
Apocynin, which is isolated from Picrorhiza Kurroa, is widely used as an inhibitor of NADPH oxidase (NOX). Studies have shown that Apocynin can participate in protecting ECs from ERS-induced apoptosis by promoting the expression of IRE1α at the mRNA and protein levels (Wu et al., 2018). Knockdown of IRE1α relieved this protective effect.
Astragaloside IV and cycloastragenol are the main active components of Astragali Radix. Zhao et al. (2015) reported that both of them could inhibit the production of ROS, the phosphorylation of IRE1α and the subsequent activation of TXNIP/NLRP3, inhibit ROS/ERS/inflammation, and play an anti-apoptotic role in palmitic acid-stimulated ECs.
Mangiferin is the active ingredient of traditional medicine Anemarrhenae Rhizoma and Belamcandae Rhizoma. In high glucose-treated ECs, mangiferin can effectively inhibit ERS and ERS-related oxidative stress and inflammation. The specific mechanism involves reducing reduce the phosphorylation of IRE1α through the AMPK pathway, inhibiting the expression of TXNIP and NLRP3, and reducing the production of ROS, IL-1β and IL-6 (Song et al., 2015).
Paeoniflorin is the main active ingredient of Paeoniae Radix Alba. Through the IRE1α/NF-κb pathway, paeoniflorin inhibited the overexpression of ERS markers (GRP78 and CHOP) and inflammatory cytokines (IL-6 and MCP-1) and reduced lipopolysaccharide (LPS) -induced HUVECs injury (Chen et al., 2018).
Paeonolis is present in a variety of plant medicines and is the main active ingredient of Moutan Cortex. It can inhibit the protein expression of ERS markers (eIF2α, ATF6, and GRP78) in the vascular wall through the AMPK/PPARδ signalling pathway, reduce ROS production, ameliorate the damage of aortic endothelium-dependent relaxation in mice, and increase the bioavailability of NO (Choy et al., 2016; Choy et al., 2017).
Luteolin and epigallocatechin gallate (EGCG) are natural flavonoids. EGCG is present mainly in Camellia sinensis (L.) Kuntze, and luteolin can be found in a variety of plant medicines. Both of them can reduce the production of ROS and the activation of TXNIP through the activation of AMPK signalling pathway, which means that they play a role in resisting oxidative stress and ERS. They can also inhibit the subsequent expression of the NLRP3 inflammasome and IL-1β, and protect HUVECs from apoptosis by restoring MMP and inhibiting caspase-3 activity (Wu J. et al., 2014).
Caffeic acid is a phenolic acid widely found in many plants. By inhibiting receptor for advanced glycation endproducts (RAGE), NOX4-dependent oxidative stress, and ERS (promoting GRP78 protein expression, reducing EIF2α phosphorylation and CHOP expression), caffeic acid can reduce the secretion of CRP, VCAM-1 and MCP-1 in human ECs treated with glycosylated low-density lipoprotein (g-LDL) (Toma et al., 2017).
Ginseng Radix Et Rhizoma is the dried root and rhizome of Panax ginseng C. A. Mey., which is a valuable Chinese herbal medicine containing a large number of saponins (Yu et al., 2020). Under LPS conditions, ginsenoside Rb2 can reduce inflammation and ERS in HUVECs and THP-1 monocytes, thereby inhibiting the apoptosis and adhesion of THP-1 monocytes to HUVECs (Sun et al., 2020). Ginsenoside Rh1 has been shown to reduce LPS-induced ECs inflammation and apoptosis by blocking the binding of LPS to TLR2 and TLR4, thus inhibiting STAT3/NF-κB inflammatory pathway and PERK/CHOP/ERO1-α ERS signaling pathway. In the in vivo model, ginsenoside Rh1 can also effectively protect the ER and rescue the tight junctions of ECs damaged by LPS (Jin et al., 2022b). Ginsentide TP1 is a recently discovered heat-stable microprotein in Ginseng Radix Et Rhizoma. It has been shown to protect HUVECs from hypoxia and ERS, restore NO signal transduction and bioavailability, and reduce oxidative stress (Dutta et al., 2023).
Guanxinkang decoction and Danzhi jiangtang capsule are both TCM prescriptions. Studies have shown that Guanxinkang decoctioncan can inhibit endoplasmic reticulum stress (reduce GRP78 expression), increase the MMP, and reverse homocysteine-mediated HUVECs apoptosis. In addition, its antagonistic effect increased with the increase of concentration and action time (Wang et al., 2014). In high-fat diet-fed rats and palmitic acid-treated HUVECs, Danzhi jiangtang capsule can effectively reduce oxidative stress and ER stress (decreased expression of IRE1α, XBP1, CHOP and GRP78), and reduce lipid deposition and the inflammatory response (Lu et al., 2018).
5.3 The regulatory function of the interaction mechanism
Previous studies have shown that some natural drugs can play regulatory roles in both MQC and ER function.
Salidroside is the main active ingredient of the natural medicine Rhodiolae Crenulatae Radix et Rhizoma. Salidroside pretreatment of HUVECs significantly upregulated PGC-1 and Tfam, promoted mitochondrial biogenesis, and improved endothelial cell mitochondrial quality and ATP production. The toxicity of H2O2 to cells was reduced, and endothelium-dependent vascular relaxation was restored (Xing et al., 2014). In addition, salidroside can inhibit homocysteine-induced activation of Bip and CHOP, as well as phosphorylation of PERK and IRE1α, and regulate ERS to exert a protective effect on HUVECs (Zhu et al., 2017).
Tetramethylpyrazine is one of the active components of the natural medicine Chuanxiong Rhizoma. It can reverse the inhibition of sirtuin 1 (SIRT1), PGC-1α, Nrf-1, and Tfam in ECs caused by high glucose and play an antioxidant role (Xu et al., 2014). In addition, studies by Mak et al. (2017) showed that tetramethylpyrazine alleviated endothelium-dependent vasodilatation injury caused by angiotensin-II (Ang-II) by inhibiting the expression of the ERS-related markers GRP78, ATF6, pPERK and p-IRE1 in porcine coronary ECs.
Ilexgenin A, which is extracted from Ilex hainanensis Merr., activated NRF2 expression by promoting mitochondrial biogenesis and inhibited palmitate-induced excessive mitochondrial fission (increasing DRP1 expression level) and oxidative stress (Zhu et al., 2019). In addition, Ilexgenin A can attenuate IRE1 and PERK phosphorylation by regulating AMPK, inhibit palmitate-induced ERS and subsequent TXNIP/NLRP3 inflammasome activation, and improve endothelial dysfunction (Li et al., 2015).
Triptolide is a diterpene lactone epoxide compound extracted from Tripterygium wilfordii Hook. f. that can regulate the activation of the PPARs/PGC-1α pathway, play a role in ERS (downregulation of GRP78, XBP1, CHOP levels) and apoptosis (downregulation of Bax and caspase-3, and upregulation of Bcl-2 levels) of HUVECs (Xiang et al., 2020).
Icariin is the main active ingredient of Epimedii Folium, which can enhance mitophagy by promoting autophagosome-lysosome fusion and inhibit ferroptosis induced by ox-LDL (Wang X. et al., 2023). In addition, icariin exerted a dose-dependent protective effect on H2O2-induced vascular ECs injury by inhibiting ERS (reducing GRP78, ATF4 and eIF2α protein expression), enhancing SOD and glutathione peroxidase (GSH-Px) activities, and alleviating oxidative stress injury (Wang F. et al., 2019). In vivo and in vitro studies have shown that the protective effect of icariin on ER is related to the activation of PPARα/Sirt1/AMPKα pathway (Yao et al., 2021).
Salvianolic acid B is the main active ingredient of traditional natural medicine Salviae miltiorrhizae Radix et Rhizoma, which has a good protective effect on MQC and ER function. Studies have shown that salvianolic acid B can inhibit the increase in p-DRP1 and FIS1 levels induced by oxLDL and high glucose, which means that it can inhibit the mitochondrial fission of ECs (Ko et al., 2020). In the thoracic aorta of diabetic mice and HUVECs induced by HG-carbonyl cyanide m-chlorophenyl hydrazone (CCCP), Salvianolic acid B can significantly increase the expression of Bcl-2, reduce the expression of BAX, Beclin1, Parkin and Pink1, which protected ECs from mitophagy and apoptosis (Xiang J. et al., 2022). In addition, by regulating the AMPK/FoxO4/KLF2 and Syndecan-4/Ras-related C3 botulinum toxin substrate 1 (Rac1)/ATF2 pathways, salvianolic acid B can alleviate ERS and oxidative stress, and inhibit NLRP3 inflammasome-mediated ECs pyroptosis (Tang et al., 2022).
Quercetin is a flavonoid widely found in a variety of natural plant medicines. It can reduce mitochondrial fragmentation and restore vascular endothelial insulin sensitivity by inhibiting Drp1 levels and serine 616 phosphorylation in the ECs of obese mice (Chen et al., 2019). In addition, quercetin was shown to reduce the expression levels of GRP78 and CHOP to inhibit ERS in HUVECs, reduce the expression of the NLRP3 inflammasome and IL-1β and inhibit apoptosis. AMPK signaling pathway may be involved in mediating this effect (Suganya et al., 2014; Wu J. et al., 2014). For HUVECs treated with high glucose, quercetin also played a role in inhibiting ERS and the subsequent inflammatory response (Cai et al., 2017).
In general, the regulatory mechanisms of some natural drugs on both mitochondria and ER have been relatively well studied. However, further research is necessary to determine whether there are upstream and downstream correlations between the two regulatory mechanisms and whether there is a common upstream regulatory pathway.
Additionally, the crosstalk between oxidative stress and ERS intervened by TCM has been widely studied in recent years. Among these, Nrf2 is a crucial target for natural drugs to regulate both oxidative stress and ERS. Rhei Radix et Rhizoma, derived from the dried roots and rhizomes of Rheum palmatum L., is a traditional Chinese herbal medicine with a long history of application. Piceatannol is its active ingredient. Studies have shown that piceatannol pretreatment of ECs can activate Nrf2/heme oxygenase-1 (HO-1) expression to reduce GRP78 and CHOP expression and XBP1 mRNA splicing. Sulfur-containing amino acid homocysteine-induced oxidative stress, ERS and apoptosis were therefore inhibited (Kil et al., 2017). Similarly, as one of the active components of Rhei Radix et Rhizoma, physcion can increase the activation of eNOS/Nrf2 signalling, inhibit palmitic acid-induced oxidative stress and ERS in HUVECs (inhibit the expression of GRP78 and its downstream proteins PERK, p-EIF2α, ATF4, and CHOP), and prevent the damage to endothelium-dependent relaxation (Wang Y.-H. et al., 2023). Rosolic acid is an important polyphenol extracted from Plantago asiatica L.with good cardiovascular benefits (Foresti et al., 2005). Naresh Amin et al. (2021) pointed out that rosolic acid can increase the activity of antioxidant enzymes SOD, catalase (CAT) and GSH-Px by activating Nrf2, restore redox homeostasis, weaken endoplasmic reticulum stress in ECs, and exert endothelial protection.
6 Conclusion
ICM has always been a clinical concern and research challenge. Despite significant advances in the treatment of myocardial ischemic injury, coronary vascular injury, which is a key pathological change in the progression of ICM, remains to be focused on. In this process, inflammation and oxidative stress interact with each other and trigger cell death, which is responsible for coronary microvascular injury. Imbalances in ER function and MQC are important potential factors for inflammation and oxidative stress.
However, current research on coronary artery injury focuses on the early stages of ICM, such as atherosclerosis. The research objects are mainly ECs treated with high glucose, palmitic acid, and homocysteine, or animal models of diabetes and atherosclerosis, with fewer explorations of vascular injury in the later stages of ICM. Therefore, it is necessary to pay more attention to the study of coronary microvascular or microcirculation injury in subjects more closely related to the later stage of ICM, such as vascular ECs and vascular smooth muscle cells treated with glucose/oxygen deprivation or hypoxia/reoxygenation and animal models of cardiac microcirculation I/R or myocardial infarction. This can provide a comprehensive understanding of the role of inflammation and oxidative stress in ICM.
Currently, although many studies have identified markers of ER or mitochondrial dysfunction, there are no specific biomarkers that can target early detection of ICM-related vascular injury. Therefore, the identification of specific ER or mitochondrial dysfunction biomarkers closely related to ICM vascular injury is critical for a comprehensive study of this process.
In addition, owing to the wide variety of TCMs and their the complex chemical composition, although many basic studies have focused on the targeted regulation of vascular injury by natural drugs, few drugs have really entered the clinical research stage. Therefore, it is necessary to focus on a specific prescription, drug, or natural monomer, and actively carry out clinical research. This can accelerate the process of drug realization from basic research to clinical application, and realize the translation of findings on herbal medicines into standardized treatment protocols for ICM.
According to this review, among the TCM monomers that have been reported to improve vascular injury, flavonoids and polyphenols account for a large proportion. They have good intervention effects on oxidative stress and ERS, and are widely found in plant-derived TCMs. Therefore, it is possible to consider the screening and in-depth study of natural plant medicines rich in flavonoids and polyphenols. These findings provide fast and valuable ideas for the screening of effective monomer drugs and the development of new drugs against ICM. Finally, ICM patients must follow their doctor’s guidance for long-term use of herbal therapies and have regular follow-up visits to evaluate the efficacy and determine the appropriate treatment
Author contributions
CZ: Investigation, Writing–original draft. XC: Writing–original draft, Writing–review and editing. DZ: Visualization, Writing–original draft. YH: Visualization, Writing–original draft. GD: Writing–original draft, Writing–review and editing. LG: Investigation, Writing–original draft.
Funding
The author(s) declare that financial support was received for the research, authorship, and/or publication of this article. This study is supported by the NSFC (NO. 82074235) and the Central Universities(2023-JYB-JBQN-041). The funders had no role in paper design, data collection, data analysis, interpretation, and writing of the paper.
Conflict of interest
The authors declare that the research was conducted in the absence of any commercial or financial relationships that could be construed as a potential conflict of interest.
Generative AI statement
The author(s) declare that no Generative AI was used in the creation of this manuscript.
Publisher’s note
All claims expressed in this article are solely those of the authors and do not necessarily represent those of their affiliated organizations, or those of the publisher, the editors and the reviewers. Any product that may be evaluated in this article, or claim that may be made by its manufacturer, is not guaranteed or endorsed by the publisher.
References
Abdelzaher, L. A., Imaizumi, T., Suzuki, T., Tomita, K., Takashina, M., and Hattori, Y. (2016). Astaxanthin alleviates oxidative stress insults-related derangements in human vascular endothelial cells exposed to glucose fluctuations. Life Sci. 150, 24–31. doi:10.1016/j.lfs.2016.02.087
Adachi, T., Matsui, R., Xu, S., Kirber, M., Lazar, H. L., Sharov, V. S., et al. (2002). Antioxidant improves smooth muscle sarco/endoplasmic reticulum Ca 2+ -ATPase function and lowers tyrosine nitration in hypercholesterolemia and improves nitric oxide–induced relaxation. Circ. Res. 90, 1114–1121. doi:10.1161/01.RES.0000019757.57344.D5
Adachi, T., Weisbrod, R. M., Pimentel, D. R., Ying, J., Sharov, V. S., Schöneich, C., et al. (2004). S-Glutathiolation by peroxynitrite activates SERCA during arterial relaxation by nitric oxide. Nat. Med. 10, 1200–1207. doi:10.1038/nm1119
Alshabibi, M. A., Khatlani, T., Abomaray, F. M., AlAskar, A. S., Kalionis, B., Messaoudi, S. A., et al. (2018). Human decidua basalis mesenchymal stem/stromal cells protect endothelial cell functions from oxidative stress induced by hydrogen peroxide and monocytes. Stem Cell Res. Ther. 9, 275. doi:10.1186/s13287-018-1021-z
Ammar, R. B., Mohamed, M. E., Alfwuaires, M., Abdulaziz Alamer, S., Bani Ismail, M., Veeraraghavan, V. P., et al. (2022). Anti-inflammatory activity of geraniol isolated from lemon grass on ox-LDL-stimulated endothelial cells by upregulation of heme oxygenase-1 via PI3K/Akt and nrf-2 signaling pathways. Nutrients 14, 4817. doi:10.3390/nu14224817
An, C., Sun, F., Liu, C., Huang, S., Xu, T., Zhang, C., et al. (2023). IQGAP1 promotes mitochondrial damage and activation of the mtDNA sensor cGAS-STING pathway to induce endothelial cell pyroptosis leading to atherosclerosis. Int. Immunopharmacol. 123, 110795. doi:10.1016/j.intimp.2023.110795
Arsenijevic, D., Onuma, H., Pecqueur, C., Raimbault, S., Manning, B. S., Miroux, B., et al. (2000). Disruption of the uncoupling protein-2 gene in mice reveals a role in immunity and reactive oxygen species production. Nat. Genet. 26, 435–439. doi:10.1038/82565
Bai, X., Wang, Y., Luo, X., Bao, X., Weng, X., Chen, Y., et al. (2024). Cigarette tar accelerates atherosclerosis progression via RIPK3-dependent necroptosis mediated by endoplasmic reticulum stress in vascular smooth muscle cells. Cell Commun. Signal. 22, 41. doi:10.1186/s12964-024-01480-6
Basit, F., Van Oppen, L. M., Schöckel, L., Bossenbroek, H. M., Van Emst-de Vries, S. E., Hermeling, J. C., et al. (2017). Mitochondrial complex I inhibition triggers a mitophagy-dependent ROS increase leading to necroptosis and ferroptosis in melanoma cells. Cell Death Dis. 8, e2716. doi:10.1038/cddis.2017.133
Cai, X., Bao, L., Ding, Y., Dai, X., Zhang, Z., and Li, Y. (2017). Quercetin alleviates cell apoptosis and inflammation via the ER stress pathway in vascular endothelial cells cultured in high concentrations of glucosamine. Mol. Med. Rep. 15, 825–832. doi:10.3892/mmr.2016.6054
Calabriso, N., Gnoni, A., Stanca, E., Cavallo, A., Damiano, F., Siculella, L., et al. (2018). Hydroxytyrosol ameliorates endothelial function under inflammatory conditions by preventing mitochondrial dysfunction. Oxid. Med. Cell. Longev. 2018, 9086947–9087014. doi:10.1155/2018/9086947
Chang, X., Liu, J., Wang, Y., Guan, X., and Liu, R. (2023a). Mitochondrial disorder and treatment of ischemic cardiomyopathy: potential and advantages of Chinese herbal medicine. Biomed. Pharmacother. 159, 114171. doi:10.1016/j.biopha.2022.114171
Chang, X., Liu, R., Li, R., Peng, Y., Zhu, P., and Zhou, H. (2023b). Molecular mechanisms of mitochondrial quality control in ischemic cardiomyopathy. Int. J. Biol. Sci. 19, 426–448. doi:10.7150/ijbs.76223
Chang, Y., Yan, W., He, X., Zhang, L., Li, C., Huang, H., et al. (2012). miR-375 inhibits autophagy and reduces viability of hepatocellular carcinoma cells under hypoxic conditions. Gastroenterology 143, 177–187. doi:10.1053/j.gastro.2012.04.009
Chen, C., Huang, J., Shen, J., and Bai, Q. (2019). Quercetin improves endothelial insulin sensitivity in obese mice by inhibiting Drp1 phosphorylation at serine 616 and mitochondrial fragmentation. Acta Biochim. Biophys. Sin. 51, 1250–1257. doi:10.1093/abbs/gmz127
Chen, J., Zhang, M., Zhu, M., Gu, J., Song, J., Cui, L., et al. (2018). Paeoniflorin prevents endoplasmic reticulum stress-associated inflammation in lipopolysaccharide-stimulated human umbilical vein endothelial cells via the IRE1α/NF-κB signaling pathway. Food Funct. 9, 2386–2397. doi:10.1039/C7FO01406F
Chen, L., Xu, T., Qiu, Y., Liu, N., Ke, X., Fang, L., et al. (2021). Homocysteine induced a calcium-mediated disruption of mitochondrial function and dynamics in endothelial cells. J. Biochem. Mol. Toxicol. 35, e22737. doi:10.1002/jbt.22737
Chen, X., Liu, C., Yu, R., Gan, Z., Zhang, Z., Chen, Z., et al. (2023a). Interaction between ferroptosis and TNF -α: impact in obesity-related osteoporosis. FASEB J. 37, e22947. doi:10.1096/fj.202201958R
Chen, Y., Li, S., Yin, M., Li, Y., Chen, C., Zhang, J., et al. (2023b). Isorhapontigenin attenuates cardiac microvascular injury in diabetes via the inhibition of mitochondria-associated ferroptosis through PRDX2-MFN2-ACSL4 pathways. Diabetes 72, 389–404. doi:10.2337/db22-0553
Cheng, C. K., Luo, J.-Y., Lau, C. W., Cho, W. C., Ng, C. F., Ma, R. C. W., et al. (2021). A GLP-1 analog lowers ER stress and enhances protein folding to ameliorate homocysteine-induced endothelial dysfunction. Acta Pharmacol. Sin. 42, 1598–1609. doi:10.1038/s41401-020-00589-x
Choy, K. W., Lau, Y. S., Murugan, D., and Mustafa, M. R. (2017). Chronic treatment with paeonol improves endothelial function in mice through inhibition of endoplasmic reticulum stress-mediated oxidative stress. PLOS ONE 12, e0178365. doi:10.1371/journal.pone.0178365
Choy, K.-W., Mustafa, M. R., Lau, Y. S., Liu, J., Murugan, D., Lau, C. W., et al. (2016). Paeonol protects against endoplasmic reticulum stress-induced endothelial dysfunction via AMPK/PPARδ signaling pathway. Biochem. Pharmacol. 116, 51–62. doi:10.1016/j.bcp.2016.07.013
Chung, J., An, S. H., Kang, S. W., and Kwon, K. (2016). Ursodeoxycholic acid (UDCA) exerts anti-atherogenic effects by inhibiting RAGE signaling in diabetic atherosclerosis. PLOS ONE 11, e0147839. doi:10.1371/journal.pone.0147839
Chung, J., Kim, K. H., Lee, S. C., An, S. H., and Kwon, K. (2015). Ursodeoxycholic acid (UDCA) exerts anti-atherogenic effects by inhibiting endoplasmic reticulum (ER) stress induced by disturbed flow. Mol. Cells 38, 851–858. doi:10.14348/molcells.2015.0094
Cohen, K. D., and Jackson, W. F. (2005). Membrane hyperpolarization is not required for sustained muscarinic agonist-induced increases in intracellular Ca 2+ in arteriolar endothelial cells. Microcirculation 12, 169–182. doi:10.1080/10739680590904973
Consolato, F., Maltecca, F., Tulli, S., Sambri, I., and Casari, G. (2018). m-AAA and i-AAA complexes coordinate to regulate OMA1, the stress-activated supervisor of mitochondrial dynamics. J. Cell Sci. 131, jcs213546. doi:10.1242/jcs.213546
Csiszar, A., Labinskyy, N., Pinto, J. T., Ballabh, P., Zhang, H., Losonczy, G., et al. (2009). Resveratrol induces mitochondrial biogenesis in endothelial cells. Am. J. Physiol.-Heart Circ. Physiol. 297, H13–H20. doi:10.1152/ajpheart.00368.2009
Davinelli, S., Sapere, N., Visentin, M., Zella, D., and Scapagnini, G. (2013). Enhancement of mitochondrial biogenesis with polyphenols: combined effects of resveratrol and equol in human endothelial cells. Immun. Ageing 10, 28. doi:10.1186/1742-4933-10-28
Dehghani, T., Thai, P. N., Sodhi, H., Ren, L., Sirish, P., Nader, C. E., et al. (2022). Selectin-targeting glycosaminoglycan-peptide conjugate limits neutrophil-mediated cardiac reperfusion injury. Cardiovasc. Res. 118, 267–281. doi:10.1093/cvr/cvaa312
Diaz-Morales, N., Rovira-Llopis, S., Bañuls, C., Escribano-Lopez, I., De Marañon, A. M., Lopez-Domenech, S., et al. (2016). Are mitochondrial fusion and fission impaired in leukocytes of type 2 diabetic patients? Antioxid. Redox Signal. 25, 108–115. doi:10.1089/ars.2016.6707
Dilshara, M. G., Jayasooriya, R. G. P. T., Molagoda, I. M. N., Jeong, J.-W., Lee, S., Park, S. R., et al. (2018). Silibinin sensitizes TRAIL-mediated apoptosis by upregulating DR5 through ROS-induced endoplasmic reticulum stress-Ca2+-CaMKII-Sp1 pathway. Oncotarget 9, 10324–10342. doi:10.18632/oncotarget.23129
Dong, J., Chen, L., Ye, F., Tang, J., Liu, B., Lin, J., et al. (2024). Mic19 depletion impairs endoplasmic reticulum-mitochondrial contacts and mitochondrial lipid metabolism and triggers liver disease. Nat. Commun. 15, 168. doi:10.1038/s41467-023-44057-6
D’Onofrio, N., Prattichizzo, F., Martino, E., Anastasio, C., Mele, L., La Grotta, R., et al. (2023). MiR-27b attenuates mitochondrial oxidative stress and inflammation in endothelial cells. Redox Biol. 62, 102681. doi:10.1016/j.redox.2023.102681
Dou, H., Kotini, A., Liu, W., Fidler, T., Endo-Umeda, K., Sun, X., et al. (2021). Oxidized phospholipids promote NETosis and arterial thrombosis in LNK(SH2B3) deficiency. Circulation 144, 1940–1954. doi:10.1161/CIRCULATIONAHA.121.056414
Du, Y., Ge, Y., Xu, Z., Aa, N., Gu, X., Meng, H., et al. (2018). Hypoxia-inducible factor 1 alpha (HIF-1α)/Vascular endothelial growth factor (VEGF) pathway participates in angiogenesis of myocardial infarction in muscone-treated mice: preliminary study. Med. Sci. Monit. 24, 8870–8877. doi:10.12659/MSM.912051
Duan, W., Liu, C., Zhou, J., Yu, Q., Duan, Y., Zhang, T., et al. (2023). Upregulation of mitochondrial calcium uniporter contributes to paraquat-induced neuropathology linked to Parkinson’s disease via imbalanced OPA1 processing. J. Hazard. Mater. 453, 131369. doi:10.1016/j.jhazmat.2023.131369
Dutta, B., Loo, S., Kam, A., Sze, S. K., and Tam, J. P. (2023). Ginsentide TP1 protects hypoxia-induced dysfunction and ER stress-linked apoptosis. Cells 12, 1401. doi:10.3390/cells12101401
Efentakis, P., Choustoulaki, A., Kwiatkowski, G., Varela, A., Kostopoulos, I. V., Tsekenis, G., et al. (2024). Early microvascular coronary endothelial dysfunction precedes pembrolizumab-induced cardiotoxicity. Preventive role of high dose of atorvastatin. Basic Res. Cardiol. 120, 263–286. doi:10.1007/s00395-024-01046-0
Ei, Z. Z., Hutamekalin, P., Prommeenate, P., Singh, A., Benjakul, S., Visuttijai, K., et al. (2022). Chitooligosaccharide prevents vascular endothelial cell apoptosis by attenuation of endoplasmic reticulum stress via suppression of oxidative stress through Nrf2-SOD1 up-regulation. Pharm. Biol. 60, 2155–2166. doi:10.1080/13880209.2022.2133150
Feenstra, L., Kutikhin, A. G., Shishkova, D. K., Buikema, H., Zeper, L. W., Bourgonje, A. R., et al. (2023). Calciprotein particles induce endothelial dysfunction by impairing endothelial nitric oxide metabolism. Arterioscler. Thromb. Vasc. Biol. 43, 443–455. doi:10.1161/ATVBAHA.122.318420
Foresti, R., Hoque, M., Monti, D., Green, C. J., and Motterlini, R. (2005). Differential activation of heme oxygenase-1 by chalcones and rosolic acid in endothelial cells. J. Pharmacol. Exp. Ther. 312, 686–693. doi:10.1124/jpet.104.074153
Forrester, S. J., Preston, K. J., Cooper, H. A., Boyer, M. J., Escoto, K. M., Poltronetti, A. J., et al. (2020). Mitochondrial fission mediates endothelial inflammation. Hypertension 76, 267–276. doi:10.1161/HYPERTENSIONAHA.120.14686
Franck, G., Mawson, T. L., Folco, E. J., Molinaro, R., Ruvkun, V., Engelbertsen, D., et al. (2018). Roles of PAD4 and NETosis in experimental atherosclerosis and arterial injury: implications for superficial erosion. Circ. Res. 123, 33–42. doi:10.1161/CIRCRESAHA.117.312494
Gao, J., Su, H.-X., Li, P.-B., Shi, K.-N., and Li, H.-H. (2023). TCH-165 attenuates cardiac ischaemia/reperfusion injury by balancing mitochondrial dynamics via increasing proteasome activity. Eur. J. Pharmacol. 957, 176011. doi:10.1016/j.ejphar.2023.176011
Ge, Y., Boopathy, S., Nguyen, T. H., Lugo, C. M., and Chao, L. H. (2021). Absence of cardiolipin from the outer leaflet of a mitochondrial inner membrane mimic restricts opa1-mediated fusion. Front. Mol. Biosci. 8, 769135. doi:10.3389/fmolb.2021.769135
Gleyzer, N., Vercauteren, K., and Scarpulla, R. C. (2005). Control of mitochondrial transcription specificity factors (TFB1M and TFB2M) by nuclear respiratory factors (NRF-1 and NRF-2) and PGC-1 family coactivators. Mol. Cell. Biol. 25, 1354–1366. doi:10.1128/MCB.25.4.1354-1366.2005
Han, S., Wu, H., Li, W., and Gao, P. (2015). Protective effects of genistein in homocysteine-induced endothelial cell inflammatory injury. Mol. Cell. Biochem. 403, 43–49. doi:10.1007/s11010-015-2335-0
Hang, L., Peng, Y., Xiang, R., Li, X., and Li, Z. (2020). Ox-LDL causes endothelial cell injury through ASK1/NLRP3-mediated inflammasome activation via endoplasmic reticulum stress. Drug Des. devel. Ther. 14, 731–744. doi:10.2147/DDDT.S231916
Hao, Y., Liu, H.-M., Wei, X., Gong, X., Lu, Z.-Y., and Huang, Z.-H. (2019). Diallyl trisulfide attenuates hyperglycemia-induced endothelial apoptosis by inhibition of Drp1-mediated mitochondrial fission. Acta Diabetol. 56, 1177–1189. doi:10.1007/s00592-019-01366-x
Hassoun, R., Budde, H., Zhazykbayeva, S., Herwig, M., Sieme, M., Delalat, S., et al. (2021). Stress activated signalling impaired protein quality control pathways in human hypertrophic cardiomyopathy. Int. J. Cardiol. 344, 160–169. doi:10.1016/j.ijcard.2021.09.009
He, P.-Y., Hou, Y.-H., Yang, Y., and Li, N. (2021). The anticancer effect of extract of medicinal mushroom Sanghuangprous vaninii against human cervical cancer cell via endoplasmic reticulum stress-mitochondrial apoptotic pathway. J. Ethnopharmacol. 279, 114345. doi:10.1016/j.jep.2021.114345
Hong, J., Park, E., Lee, J., Lee, Y., Rooney, B. V., and Park, Y. (2021). Exercise training mitigates ER stress and UCP2 deficiency-associated coronary vascular dysfunction in atherosclerosis. Sci. Rep. 11, 15449. doi:10.1038/s41598-021-94944-5
Hori, M., and Nishida, K. (2008). Oxidative stress and left ventricular remodelling after myocardial infarction. Cardiovasc. Res. 81, 457–464. doi:10.1093/cvr/cvn335
Hou, D., Liu, R., Hao, S., Dou, Y., Chen, G., Liu, L., et al. (2024a). Notoginsenoside R1 improves intestinal microvascular functioning in sepsis by targeting Drp1-mediated mitochondrial quality imbalance. Pharm. Biol. 62, 250–260. doi:10.1080/13880209.2024.2318349
Hou, H., Qin, X., Li, G., Cui, Z., Zhang, J., Dong, B., et al. (2024b). Nrf2-mediated redox balance alleviates LPS-induced vascular endothelial cell inflammation by inhibiting endothelial cell ferroptosis. Sci. Rep. 14, 3335. doi:10.1038/s41598-024-53976-3
Hu, Z., Zhang, H., Wang, Y., Li, B., Liu, K., Ran, J., et al. (2023). Exercise activates Sirt1-mediated Drp1 acetylation and inhibits hepatocyte apoptosis to improve nonalcoholic fatty liver disease. Lipids Health Dis. 22, 33. doi:10.1186/s12944-023-01798-z
Huang, J., Wan, L., Lu, H., and Li, X. (2018). High expression of active ATF6 aggravates endoplasmic reticulum stress-induced vascular endothelial cell apoptosis through the mitochondrial apoptotic pathway. Mol. Med. Rep. 17, 6483–6489. doi:10.3892/mmr.2018.8658
Huang, W.-Y., Lai, Y.-L., Liu, K.-H., Lin, S., Chen, H.-Y., Liang, C.-H., et al. (2022). TNFα-mediated necroptosis in brain endothelial cells as a potential mechanism of increased seizure susceptibility in mice following systemic inflammation. J. Neuroinflammation 19, 29. doi:10.1186/s12974-022-02406-0
Jankauskas, S. S., Kansakar, U., Sardu, C., Varzideh, F., Avvisato, R., Wang, X., et al. (2023). COVID-19 causes ferroptosis and oxidative stress in human endothelial cells. Antioxidants 12, 326. doi:10.3390/antiox12020326
Ji, M., Niu, S., Guo, J., Mi, H., and Jiang, P. (2020). Silencing RNF13 alleviates Parkinson’s disease – like problems in mouse models by regulating the endoplasmic reticulum stress–mediated ire1α-TRAF2-ASK1-JNK pathway. J. Mol. Neurosci. 70, 1977–1986. doi:10.1007/s12031-020-01599-4
Jia, F., Chen, Y., Xin, G., Li, L., Liu, Z., Xu, S., et al. (2023). Shuangshen Ningxin capsule alleviates myocardial ischemia–reperfusion injury in miniature pigs by modulating mitophagy: network pharmacology and experiments in vivo. Chin. Med. 18, 120. doi:10.1186/s13020-023-00810-z
Jian, Y., Yang, Y., Cheng, L., Yang, X., Liu, H., Li, W., et al. (2023). Sirt3 mitigates LPS -induced mitochondrial damage in renal tubular epithelial cells by deacetylating YME1L1. Cell Prolif. 56, e13362. doi:10.1111/cpr.13362
Jiang, X., Ma, C., Gao, Y., Cui, H., Zheng, Y., Li, J., et al. (2023). Tongxinluo attenuates atherosclerosis by inhibiting ROS/NLRP3/caspase-1-mediated endothelial cell pyroptosis. J. Ethnopharmacol. 304, 116011. doi:10.1016/j.jep.2022.116011
Jiang, Y., Dong, B., Jiao, X., Shan, J., Fang, C., Zhang, K., et al. (2024). Nano-selenium alleviates the pyroptosis of cardiovascular endothelial cells in chicken induced by decabromodiphenyl ether through ERS-TXNIP-NLRP3 pathway. Sci. Total Environ. 915, 170129. doi:10.1016/j.scitotenv.2024.170129
Jin, Y., Liu, Y., Xu, L., Xu, J., Xiong, Y., Peng, Y., et al. (2022a). Novel role for caspase 1 inhibitor VX765 in suppressing NLRP3 inflammasome assembly and atherosclerosis via promoting mitophagy and efferocytosis. Cell Death Dis. 13, 512. doi:10.1038/s41419-022-04966-8
Jin, Y., Nguyen, T. L. L., Myung, C.-S., and Heo, K.-S. (2022b). Ginsenoside Rh1 protects human endothelial cells against lipopolysaccharide-induced inflammatory injury through inhibiting TLR2/4-mediated STAT3, NF-κB, and ER stress signaling pathways. Life Sci. 309, 120973. doi:10.1016/j.lfs.2022.120973
Jin, Z., Liu, F., Zhang, G., Zhang, J., Zhao, X., Huo, X., et al. (2023). An effective disease diagnostic model related to pyroptosis in ischemic cardiomyopathy. J. Cell. Mol. Med. 27, 3816–3826. doi:10.1111/jcmm.17957
Kalkhoran, S. B., Kriston-Vizi, J., Hernandez-Resendiz, S., Crespo-Avilan, G. E., Rosdah, A. A., Lees, J. G., et al. (2022). Hydralazine protects the heart against acute ischaemia/reperfusion injury by inhibiting Drp1-mediated mitochondrial fission. Cardiovasc. Res. 118, 282–294. doi:10.1093/cvr/cvaa343
Kan, H., Zhao, M., Wang, W., and Sun, B. (2024). Understanding propofol’s protective mechanism in tubular epithelial cells: mitigating pyroptosis via the miR-143-3p/ATPase Na +/K + transporting subunit alpha 2 pathway in renal ischemia–reperfusion. Mol. Biotechnol. 67, 1165–1177. doi:10.1007/s12033-024-01116-7
Kang, K., Chen, S.-H., Wang, D.-P., and Chen, F. (2024). Inhibition of endoplasmic reticulum stress improves chronic ischemic hippocampal damage associated with suppression of ire1α/TRAF2/ASK1/JNK-dependent apoptosis. Inflammation 47, 1479–1490. doi:10.1007/s10753-024-01989-5
Karunakaran, D., Nguyen, M.-A., Geoffrion, M., Vreeken, D., Lister, Z., Cheng, H. S., et al. (2021). RIPK1 expression associates with inflammation in early atherosclerosis in humans and can Be therapeutically silenced to reduce NF-κB activation and atherogenesis in mice. Circulation 143, 163–177. doi:10.1161/CIRCULATIONAHA.118.038379
Ke, W., Wang, B., Liao, Z., Song, Y., Li, G., Ma, L., et al. (2023). Matrix stiffness induces Drp1-mediated mitochondrial fission through Piezo1 mechanotransduction in human intervertebral disc degeneration. J. Transl. Med. 21, 711. doi:10.1186/s12967-023-04590-w
Keller, A., Hull, S. E., Elajaili, H., Johnston, A., Knaub, L. A., Chun, J. H., et al. (2020). (–)-Epicatechin modulates mitochondrial redox in vascular cell models of oxidative stress. Oxid. Med. Cell. Longev. 2020, 6392629–6392712. doi:10.1155/2020/6392629
Khan, M. A., Hashim, M. J., Mustafa, H., Baniyas, M. Y., Al Suwaidi, S. K. B. M., AlKatheeri, R., et al. (2020). Global epidemiology of ischemic heart disease: results from the global burden of disease study. Cureus 12, e9349. doi:10.7759/cureus.9349
Kil, J.-S., Jeong, S.-O., Chung, H.-T., and Pae, H.-O. (2017). Piceatannol attenuates homocysteine-induced endoplasmic reticulum stress and endothelial cell damage via heme oxygenase-1 expression. Amino Acids 49, 735–745. doi:10.1007/s00726-016-2375-0
Kim, M., Kim, J., Moon, S., Choi, B. Y., Kim, S., Jeon, H. S., et al. (2021). Korean red ginseng improves astrocytic mitochondrial function by upregulating HO-1-Mediated ampkα–PGC-1α–errα circuit after traumatic brain injury. Int. J. Mol. Sci. 22, 13081. doi:10.3390/ijms222313081
Kim, S.-H., Kim, K.-Y., Yu, S.-N., Seo, Y.-K., Chun, S.-S., Yu, H.-S., et al. (2016). Silibinin induces mitochondrial NOX4-mediated endoplasmic reticulum stress response and its subsequent apoptosis. BMC Cancer 16, 452. doi:10.1186/s12885-016-2516-6
Kim, S.-R., Bae, Y.-H., Bae, S.-K., Choi, K.-S., Yoon, K.-H., Koo, T. H., et al. (2008). Visfatin enhances ICAM-1 and VCAM-1 expression through ROS-dependent NF-kappaB activation in endothelial cells. Biochim. Biophys. Acta BBA - Mol. Cell Res. 1783, 886–895. doi:10.1016/j.bbamcr.2008.01.004
Kiyan, Y., Tkachuk, S., Kurselis, K., Shushakova, N., Stahl, K., Dawodu, D., et al. (2019). Heparanase-2 protects from LPS-mediated endothelial injury by inhibiting TLR4 signalling. Sci. Rep. 9, 13591. doi:10.1038/s41598-019-50068-5
Ko, Y. S., Jin, H., Park, S. W., and Kim, H. J. (2020). Salvianolic acid B protects against oxLDL-induced endothelial dysfunction under high-glucose conditions by downregulating ROCK1-mediated mitophagy and apoptosis. Biochem. Pharmacol. 174, 113815. doi:10.1016/j.bcp.2020.113815
Kong, D. Z., Sun, P., Lu, Y., Yang, Y., Min, D. Y., Zheng, S. C., et al. (2024). Yi Mai granule improve energy supply of endothelial cells in atherosclerosis via miRNA-125a-5p regulating mitochondrial autophagy through Pink1-Mfn2-Parkin pathway. J. Ethnopharmacol. 319, 117114. doi:10.1016/j.jep.2023.117114
Kulkovienė, G., Narauskaitė, D., Tunaitytė, A., Volkevičiūtė, A., Balion, Z., Kutakh, O., et al. (2024). Differential mitochondrial, oxidative stress and inflammatory responses to SARS-CoV-2 spike protein receptor binding domain in human lung microvascular, coronary artery endothelial and bronchial epithelial cells. Int. J. Mol. Sci. 25, 3188. doi:10.3390/ijms25063188
Ledoux, J., Taylor, M. S., Bonev, A. D., Hannah, R. M., Solodushko, V., Shui, B., et al. (2008). Functional architecture of inositol 1,4,5-trisphosphate signaling in restricted spaces of myoendothelial projections. Proc. Natl. Acad. Sci. 105, 9627–9632. doi:10.1073/pnas.0801963105
Lee, W.-J., Huey-Herng Sheu, W., Liu, S.-H., Yi, Y.-C., Chen, W.-C., Lin, S.-Y., et al. (2014). Nε-carboxymethyllysine-mediated endoplasmic reticulum stress promotes endothelial cell injury through Nox4/MKP-3 interaction. Free Radic. Biol. Med. 74, 294–306. doi:10.1016/j.freeradbiomed.2014.06.015
Lei, N., Song, H., Zeng, L., Ji, S., Meng, X., Zhu, X., et al. (2023). Persistent lipid accumulation leads to persistent exacerbation of endoplasmic reticulum stress and inflammation in progressive NASH via the ire1α/TRAF2 complex. Molecules 28, 3185. doi:10.3390/molecules28073185
Lei, Y.-P., Chen, H.-W., Sheen, L.-Y., and Lii, C.-K. (2008). Diallyl disulfide and Diallyl trisulfide suppress oxidized LDL–induced vascular cell adhesion molecule and E-selectin expression through protein kinase A– and B–dependent signaling pathways. J. Nutr. 138, 996–1003. doi:10.1093/jn/138.6.996
Li, C., Ma, Q., Toan, S., Wang, J., Zhou, H., and Liang, J. (2020a). SERCA overexpression reduces reperfusion-mediated cardiac microvascular damage through inhibition of the calcium/MCU/mPTP/necroptosis signaling pathways. Redox Biol. 36, 101659. doi:10.1016/j.redox.2020.101659
Li, C., Tan, Y., Wu, J., Ma, Q., Bai, S., Xia, Z., et al. (2020b). Resveratrol improves bnip3-related mitophagy and attenuates high-fat-induced endothelial dysfunction. Front. Cell Dev. Biol. 8, 796. doi:10.3389/fcell.2020.00796
Li, H., Pan, W., Li, C., Cai, M., Shi, W., Ren, Z., et al. (2024a). Heat stress induces calcium dyshomeostasis to subsequent cognitive impairment through ERS-mediated apoptosis via SERCA/PERK/eIF2α pathway. Cell Death Discov. 10, 280. doi:10.1038/s41420-024-02047-7
Li, J., Wang, Y., Wang, C., Li, Y., Yang, Q., Cai, W., et al. (2023a). Shenlian extract decreases mitochondrial autophagy to regulate mitochondrial function in microvascular to alleviate coronary artery no-reflow. Phytother. Res. 37, 1864–1882. doi:10.1002/ptr.7703
Li, J., Zhang, J., Yu, P., Xu, H., Wang, M., Chen, Z., et al. (2024b). ROS-responsive and scavenging NO nanomedicine for vascular diseases treatment by inhibiting endoplasmic reticulum stress and improving NO bioavailability. Bioact. Mater. 37, 239–252. doi:10.1016/j.bioactmat.2024.03.010
Li, P., Xie, C., Zhong, J., Guo, Z., Guo, K., and Tu, Q. (2021a). Melatonin attenuates ox-LDL-induced endothelial dysfunction by reducing ER stress and inhibiting JNK/mff signaling. Oxid. Med. Cell. Longev. 2021, 5589612–5589710. doi:10.1155/2021/5589612
Li, S., Li, Q., Zhou, Q., Li, S., Wang, S., Yao, Q., et al. (2024c). Attenuating atherosclerosis through inhibition of the NF-κB/NLRP3/IL-1β pathway-mediated pyroptosis in vascular smooth muscle cells (VSMCs). Cardiovasc. Ther. 2024, 1506083–1506118. doi:10.1155/2024/1506083
Li, S. Y., Zhong, J., Qiao, H. Y., Schoepf, U. J., Emrich, T., Butler, W. N., et al. (2024d). FFRCT and static computed tomography myocardial perfusion imaging for therapeutic decision-making and prognosis in patients with coronary artery disease. J. Thorac. Imaging 39, 101–110. doi:10.1097/RTI.0000000000000718
Li, W., Feng, G., Gauthier, J. M., Lokshina, I., Higashikubo, R., Evans, S., et al. (2019). Ferroptotic cell death and TLR4/Trif signaling initiate neutrophil recruitment after heart transplantation. J. Clin. Invest. 129, 2293–2304. doi:10.1172/JCI126428
Li, X., Chen, X., Zheng, L., Chen, M., Zhang, Y., Zhu, R., et al. (2023b). Non-canonical STING–PERK pathway dependent epigenetic regulation of vascular endothelial dysfunction via integrating IRF3 and NF-κB in inflammatory response. Acta Pharm. Sin. B 13, 4765–4784. doi:10.1016/j.apsb.2023.08.015
Li, X., Liu, X., Liu, W., Lin, Y., Liu, J., Peng, Y., et al. (2023c). Inhibition of TMEM16A improves cisplatin-induced acute kidney injury via preventing DRP1-mediated mitochondrial fission. Acta Pharmacol. Sin. 44, 2230–2242. doi:10.1038/s41401-023-01122-6
Li, Y., Schwabe, R. F., DeVries-Seimon, T., Yao, P. M., Gerbod-Giannone, M.-C., Tall, A. R., et al. (2005). Free cholesterol-loaded macrophages are an abundant source of tumor necrosis factor-alpha and interleukin-6: model of NF-kappaB- and map kinase-dependent inflammation in advanced atherosclerosis. J. Biol. Chem. 280, 21763–21772. doi:10.1074/jbc.M501759200
Li, Y., Tang, J., Gao, H., Xu, Y., Han, Y., Shang, H., et al. (2021b). Ganoderma lucidum triterpenoids and polysaccharides attenuate atherosclerotic plaque in high-fat diet rabbits. Nutr. Metab. Cardiovasc. Dis. 31, 1929–1938. doi:10.1016/j.numecd.2021.03.023
Li, Y., Yang, J., Chen, M.-H., Wang, Q., Qin, M.-J., Zhang, T., et al. (2015). Ilexgenin A inhibits endoplasmic reticulum stress and ameliorates endothelial dysfunction via suppression of TXNIP/NLRP3 inflammasome activation in an AMPK dependent manner. Pharmacol. Res. 99, 101–115. doi:10.1016/j.phrs.2015.05.012
Li, Y., Zhou, Z.-H., Chen, M.-H., Yang, J., Leng, J., Cao, G.-S., et al. (2016). Inhibition of mitochondrial fission and NOX2 expression prevent NLRP3 inflammasome activation in the endothelium: the role of corosolic acid action in the amelioration of endothelial dysfunction. Antioxid. Redox Signal. 24, 893–908. doi:10.1089/ars.2015.6479
Li, Z., Dai, R., Chen, M., Huang, L., Zhu, K., Li, M., et al. (2023d). p55γ degrades RIP3 via MG53 to suppress ischaemia-induced myocardial necroptosis and mediates cardioprotection of preconditioning. Cardiovasc. Res. 119, 2421–2440. doi:10.1093/cvr/cvad123
Li, Z., Zhang, Y., Zheng, Y., Liu, W., Zhang, X., Li, W., et al. (2021c). Intranasal 15d-PGJ2 ameliorates brain glucose hypometabolism via PPARγ-dependent activation of PGC-1α/GLUT4 signalling in APP/PS1 transgenic mice. Neuropharmacology 196, 108685. doi:10.1016/j.neuropharm.2021.108685
Liu, H., Sun, Y., Xu, H., Tan, B., Yi, Q., Tian, J., et al. (2023). PTEN-induced putative kinase 1 regulates mitochondrial quality control and is essential for the maturation of human induced pluripotent stem cell-derived cardiomyocytes. Genes Dis. 10, 2151–2166. doi:10.1016/j.gendis.2022.08.023
Liu, M., Li, S., Yin, M., Li, Y., Chen, J., Chen, Y., et al. (2024a). Pinacidil ameliorates cardiac microvascular ischemia–reperfusion injury by inhibiting chaperone-mediated autophagy of calreticulin. Basic Res. Cardiol. 119, 113–131. doi:10.1007/s00395-023-01028-8
Liu, X., Cao, K., Lv, W., Feng, Z., Liu, J., Gao, J., et al. (2019). Punicalagin attenuates endothelial dysfunction by activating FoxO1, a pivotal regulating switch of mitochondrial biogenesis. Free Radic. Biol. Med. 135, 251–260. doi:10.1016/j.freeradbiomed.2019.03.011
Liu, X., Lu, B., Fu, J., Zhu, X., Song, E., and Song, Y. (2021). Amorphous silica nanoparticles induce inflammation via activation of NLRP3 inflammasome and HMGB1/TLR4/MYD88/NF-kb signaling pathway in HUVEC cells. J. Hazard. Mater. 404, 124050. doi:10.1016/j.jhazmat.2020.124050
Liu, Z., Han, X., You, Y., Xin, G., Li, L., Gao, J., et al. (2024b). Shuangshen ningxin formula attenuates cardiac microvascular ischemia/reperfusion injury through improving mitochondrial function. J. Ethnopharmacol. 323, 117690. doi:10.1016/j.jep.2023.117690
Lu, L., Ye, Y., Chen, Y., Feng, L., Huang, J., Liang, Q., et al. (2024). Oxidized phospholipid POVPC contributes to vascular calcification by triggering ferroptosis of vascular smooth muscle cells. FASEB J. 38, e23592. doi:10.1096/fj.202302570R
Lu, Y., Chen, Y., Li, R., Liu, Q., Wang, N., Zhang, Y., et al. (2018). Protective effects of Danzhi jiangtang capsule on vascular endothelial damages induced by high-fat diet and palmitic acid. Biomed. Pharmacother. 107, 1631–1640. doi:10.1016/j.biopha.2018.08.129
Lugus, J. J., Ngoh, G. A., Bachschmid, M. M., and Walsh, K. (2011). Mitofusins are required for angiogenic function and modulate different signaling pathways in cultured endothelial cells. J. Mol. Cell. Cardiol. 51, 885–893. doi:10.1016/j.yjmcc.2011.07.023
Luo, H., Zhao, L., Dong, B., and Liu, Y. (2024). MiR-375 inhibitor alleviates inflammation and oxidative stress by upregulating the GPR39 expression in atherosclerosis. Int. Heart. J. 65, 135–145. doi:10.1536/ihj.23-155
Ma, L., Hua, L., Yu, W., Ke, L., and Li, L. (2023). TSG -6 inhibits hypertrophic scar fibroblast proliferation by regulating IRE1α/TRAF2/NF-κB signalling. Int. Wound J. 20, 1008–1019. doi:10.1111/iwj.13950
Ma, W., Duan, X., Han, L., Zhang, L., Meng, X., Li, Y., et al. (2019). Vanillic acid alleviates palmitic acid-induced oxidative stress in human umbilical vein endothelial cells via Adenosine Monophosphate-Activated Protein Kinase signaling pathway. J. Food Biochem. 43, e12893. doi:10.1111/jfbc.12893
MacArthur, T. A., Goswami, J., Navarro, S. M., Spears, G. M., Bailey, K. R., Thompson, R., et al. (2024). A murine multiple-injury model for the study of thromboinflammation. J. Trauma Acute Care Surg. 96, 203–208. doi:10.1097/TA.0000000000004179
Mak, S.-K., Yu, C.-M., Sun, W.-T., He, G.-W., Liu, X.-C., and Yang, Q. (2017). Tetramethylpyrazine suppresses angiotensin II-induced soluble epoxide hydrolase expression in coronary endothelium via anti-ER stress mechanism. Toxicol. Appl. Pharmacol. 336, 84–93. doi:10.1016/j.taap.2017.10.016
Manuneedhi Cholan, P., Cartland, S. P., Dang, L., Rayner, B. S., Patel, S., Thomas, S. R., et al. (2018). TRAIL protects against endothelial dysfunction in vivo and inhibits angiotensin-II-induced oxidative stress in vascular endothelial cells in vitro. Free Radic. Biol. Med. 126, 341–349. doi:10.1016/j.freeradbiomed.2018.08.031
McMeekin, L. J., Joyce, K. L., Jenkins, L. M., Bohannon, B. M., Patel, K. D., Bohannon, A. S., et al. (2021). Estrogen-related receptor alpha (ERRα) is required for PGC-1α-dependent gene expression in the mouse brain. Neuroscience 479, 70–90. doi:10.1016/j.neuroscience.2021.10.007
Miao, J., Huang, Z., Liu, S., Li, X., Jia, P., Guo, Y., et al. (2018). Hydroxytyrosol protects against myocardial ischemia reperfusion injury by inhibiting mitochondrial permeability transition pore opening. Exp. Ther. Med. 17, 671–678. doi:10.3892/etm.2018.7016
Mohan, S., Nair, A., Poornima, M. S., and Raghu, K. G. (2023). Vanillic acid mitigates hyperinsulinemia induced ER stress mediated altered calcium homeostasis, MAMs distortion and surplus lipogenesis in HepG2 cells. Chem. Biol. Interact. 375, 110365. doi:10.1016/j.cbi.2023.110365
Molinaro, R., Yu, M., Sausen, G., Bichsel, C. A., Corbo, C., Folco, E. J., et al. (2021). Targeted delivery of protein arginine deiminase-4 inhibitors to limit arterial intimal NETosis and preserve endothelial integrity. Cardiovasc. Res. 117, 2652–2663. doi:10.1093/cvr/cvab074
Morales, P. E., Torres, G., Sotomayor-Flores, C., Peña-Oyarzún, D., Rivera-Mejías, P., Paredes, F., et al. (2014). GLP-1 promotes mitochondrial metabolism in vascular smooth muscle cells by enhancing endoplasmic reticulum–mitochondria coupling. Biochem. Biophys. Res. Commun. 446, 410–416. doi:10.1016/j.bbrc.2014.03.004
Moreno-Ulloa, A., Cid, A., Rubio-Gayosso, I., Ceballos, G., Villarreal, F., and Ramirez-Sanchez, I. (2013). Effects of (−)-epicatechin and derivatives on nitric oxide mediated induction of mitochondrial proteins. Bioorg. Med. Chem. Lett. 23, 4441–4446. doi:10.1016/j.bmcl.2013.05.079
Nakajima, S., Hiramatsu, N., Hayakawa, K., Saito, Y., Kato, H., Huang, T., et al. (2011). Selective abrogation of BiP/GRP78 blunts activation of NF-κB through the ATF6 branch of the UPR: involvement of C/EBPβ and mTOR-dependent dephosphorylation of Akt. Mol. Cell. Biol. 31, 1710–1718. doi:10.1128/MCB.00939-10
Nakamura, K., Fushimi, K., Kouchi, H., Mihara, K., Miyazaki, M., Ohe, T., et al. (1998). Inhibitory effects of antioxidants on neonatal rat cardiac myocyte hypertrophy induced by tumor necrosis factor-alpha and angiotensin II. Circulation 98, 794–799. doi:10.1161/01.CIR.98.8.794
Nam, Y. W., Shin, J.-H., Kim, S., Hwang, C. H., Lee, C.-S., Hwang, G., et al. (2024). EGFR inhibits TNF-α-mediated pathway by phosphorylating TNFR1 at tyrosine 360 and 401. Cell Death Differ. 31, 1318–1332. doi:10.1038/s41418-024-01316-3
Naresh Amin, K., Rajagru, P., Sarkar, K., Ganesh, M. R., Suzuki, T., Ali, D., et al. (2021). Pharmacological activation of Nrf2 by rosolic acid attenuates endoplasmic reticulum stress in endothelial cells. Oxid. Med. Cell. Longev. 2021, 2732435–2732520. doi:10.1155/2021/2732435
Newton, K., Wickliffe, K. E., Dugger, D. L., Maltzman, A., Roose-Girma, M., Dohse, M., et al. (2019). Cleavage of RIPK1 by caspase-8 is crucial for limiting apoptosis and necroptosis. Nature 574, 428–431. doi:10.1038/s41586-019-1548-x
Nija, R. J., Sanju, S., Sidharthan, N., and Mony, U. (2020). Extracellular trap by blood cells: clinical implications. Tissue Eng. Regen. Med. 17, 141–153. doi:10.1007/s13770-020-00241-z
Nonaka, H., Tsujino, T., Watari, Y., Emoto, N., and Yokoyama, M. (2001). Taurine prevents the decrease in expression and secretion of extracellular superoxide dismutase induced by homocysteine: amelioration of homocysteine-induced endoplasmic reticulum stress by taurine. Circulation 104, 1165–1170. doi:10.1161/hc3601.093976
Noone, J., Rochfort, K. D., O’Sullivan, F., and O’Gorman, D. J. (2023). SIRT4 is a regulator of human skeletal muscle fatty acid metabolism influencing inner and outer mitochondrial membrane-mediated fusion. Cell. Signal. 112, 110931. doi:10.1016/j.cellsig.2023.110931
Olivares-Caro, L., Radojkovic, C., Chau, S. Y., Nova, D., Bustamante, L., Neira, J. Y., et al. (2020). Berberis microphylla G. Forst (calafate) berry extract reduces oxidative stress and lipid peroxidation of human LDL. Antioxidants 9, 1171. doi:10.3390/antiox9121171
Panza, J. A., Ellis, A. M., Al-Khalidi, H. R., Holly, T. A., Berman, D. S., Oh, J. K., et al. (2019). Myocardial viability and long-term outcomes in ischemic cardiomyopathy. N. Engl. J. Med. 381, 739–748. doi:10.1056/NEJMoa1807365
Pastena, P., Frye, J. T., Ho, C., Goldschmidt, M. E., and Kalogeropoulos, A. P. (2023). Ischemic cardiomyopathy: epidemiology, pathophysiology, outcomes, and therapeutic options. Heart fail. Rev. 29, 287–299. doi:10.1007/s10741-023-10377-4
Peng, W., Wu, Y., Peng, Z., Qi, W., Liu, T., Yang, B., et al. (2022). Cyanidin-3-glucoside improves the barrier function of retinal pigment epithelium cells by attenuating endoplasmic reticulum stress-induced apoptosis. Food Res. Int. 157, 111313. doi:10.1016/j.foodres.2022.111313
Perez-Ternero, C., Werner, C. M., Nickel, A. G., Herrera, M. D., Motilva, M.-J., Böhm, M., et al. (2017). Ferulic acid, a bioactive component of rice bran, improves oxidative stress and mitochondrial biogenesis and dynamics in mice and in human mononuclear cells. J. Nutr. Biochem. 48, 51–61. doi:10.1016/j.jnutbio.2017.06.011
Pirzeh, L., Babapour, V., Badalzadeh, R., and Panahi, N. (2019). Pretreatment with vildagliptin boosts ischemic-postconditioning effects on cardioprotection and expression profile of genes regulating autophagy and mitochondrial fission/fusion in diabetic heart with reperfusion injury. Naunyn. Schmiedeb. Arch. Pharmacol. 392, 1371–1382. doi:10.1007/s00210-019-01660-z
Pozzer, D., Varone, E., Chernorudskiy, A., Schiarea, S., Missiroli, S., Giorgi, C., et al. (2019). A maladaptive ER stress response triggers dysfunction in highly active muscles of mice with SELENON loss. Redox Biol. 20, 354–366. doi:10.1016/j.redox.2018.10.017
Qiang, M., Hao, J., Liu, H., Yin, J., Zhang, H., Yang, J., et al. (2022). Er-xian ameliorates myocardial ischemia-reperfusion injury in rats through RISK pathway involving estrogen receptors. Chin. J. Nat. Med. 20, 902–913. doi:10.1016/S1875-5364(22)60213-9
Qin, Z., Hou, X., Weisbrod, R. M., Seta, F., Cohen, R. A., and Tong, X. (2014). Nox2 mediates high fat high sucrose diet-induced nitric oxide dysfunction and inflammation in aortic smooth muscle cells. J. Mol. Cell. Cardiol. 72, 56–63. doi:10.1016/j.yjmcc.2014.02.019
Ramachandra, C. J. A., Hernandez-Resendiz, S., Crespo-Avilan, G. E., Lin, Y.-H., and Hausenloy, D. J. (2020). Mitochondria in acute myocardial infarction and cardioprotection. EBioMedicine 57, 102884. doi:10.1016/j.ebiom.2020.102884
Ramírez-Sánchez, I., Rodríguez, A., Moreno-Ulloa, A., Ceballos, G., and Villarreal, F. (2016). (-)-Epicatechin-induced recovery of mitochondria from simulated diabetes: potential role of endothelial nitric oxide synthase. Diab. Vasc. Dis. Res. 13, 201–210. doi:10.1177/1479164115620982
Ren, J., Liu, J., Zhang, J., Hu, X., Cui, Y., Wei, X., et al. (2023). Dynamin-related protein 1 binding partners MiD49 and MiD51 increased mitochondrial fission in vitro and atherosclerosis in high-fat-diet-fed ApoE-/- mice. Int. J. Mol. Sci. 25, 244. doi:10.3390/ijms25010244
Ronayne, C. T., Jackson, T. D., Bennett, C. F., Perry, E. A., Kantorovic, N., and Puigserver, P. (2023). Tetracyclines activate mitoribosome quality control and reduce ER stress to promote cell survival. EMBO Rep. 24, e57228. doi:10.15252/embr.202357228
Salie, R., Lopes, J., Kotze, L., and Van Aarde, R. (2023). The cardioprotective effect of S. africana caerulea/Blue Sage in ischaemia and reperfusion induced oxidative stress. Front. Pharmacol. 14, 1254561. doi:10.3389/fphar.2023.1254561
Schunk, S. J., Triem, S., Schmit, D., Zewinger, S., Sarakpi, T., Becker, E., et al. (2021). Interleukin-1α is a central regulator of leukocyte-endothelial adhesion in myocardial infarction and in chronic kidney disease. Circulation 144, 893–908. doi:10.1161/CIRCULATIONAHA.121.053547
Shah, W., Zhao, Q., Wang, S., Zhang, M., Ma, H., Guan, Y., et al. (2023). Polydatin improves vascular endothelial function by maintaining mitochondrial homeostasis under high glucose conditions. Sci. Rep. 13, 16550. doi:10.1038/s41598-023-43786-4
Shan, B., Wang, X., Wu, Y., Xu, C., Xia, Z., Dai, J., et al. (2017). The metabolic ER stress sensor IRE1α suppresses alternative activation of macrophages and impairs energy expenditure in obesity. Nat. Immunol. 18, 519–529. doi:10.1038/ni.3709
Shao, X., Huang, B., Tan, H., Wang, R., Huang, X., Diao, H., et al. (2022). Fu Fang zhen Zhu tiao zhi capsules protect against myocardial ischemia by inhibiting cardiomyocyte pyroptosis. Evid. Based Complement. Altern. Med. 2022, 4752360–4752415. doi:10.1155/2022/4752360
Shenouda, S. M., Widlansky, M. E., Chen, K., Xu, G., Holbrook, M., Tabit, C. E., et al. (2011). Altered mitochondrial dynamics contributes to endothelial dysfunction in diabetes mellitus. Circulation 124, 444–453. doi:10.1161/CIRCULATIONAHA.110.014506
Shukla, H., Chitrakar, R., Bibi, H. A., Gaje, G., Koucheki, A., Trush, M. A., et al. (2020). Reactive oxygen species production by BP-1,6-quinone and its effects on the endothelial dysfunction: involvement of the mitochondria. Toxicol. Lett. 322, 120–130. doi:10.1016/j.toxlet.2020.01.011
Song, J., Li, J., Hou, F., Wang, X., and Liu, B. (2015). Mangiferin inhibits endoplasmic reticulum stress-associated thioredoxin-interacting protein/NLRP3 inflammasome activation with regulation of AMPK in endothelial cells. Metabolism 64, 428–437. doi:10.1016/j.metabol.2014.11.008
Suen, D.-F., Narendra, D. P., Tanaka, A., Manfredi, G., and Youle, R. J. (2010). Parkin overexpression selects against a deleterious mtDNA mutation in heteroplasmic cybrid cells. Proc. Natl. Acad. Sci. 107, 11835–11840. doi:10.1073/pnas.0914569107
Suganya, N., Bhakkiyalakshmi, E., Suriyanarayanan, S., Paulmurugan, R., and Ramkumar, K. M. (2014). Quercetin ameliorates tunicamycin-induced endoplasmic reticulum stress in endothelial cells. Cell Prolif. 47, 231–240. doi:10.1111/cpr.12102
Sun, J. L., Abd El-Aty, A. M., Jeong, J. H., and Jung, T. W. (2020). Ginsenoside Rb2 ameliorates LPS-induced inflammation and ER stress in HUVECs and THP-1 cells via the AMPK-mediated pathway. Am. J. Chin. Med. 48, 967–985. doi:10.1142/S0192415X20500469
Sun, R., Wang, X., Liu, Y., and Xia, M. (2014). Dietary supplementation with fish oil alters the expression levels of proteins governing mitochondrial dynamics and prevents high-fat diet-induced endothelial dysfunction. Br. J. Nutr. 112, 145–153. doi:10.1017/S0007114514000701
Sun, S., Zou, X., Wang, D., Liu, Y., Zhang, Z., Guo, J., et al. (2023). IRGM/Irgm1 deficiency inhibits neutrophil-platelet interactions and thrombosis in experimental atherosclerosis and arterial injury. Biomed. Pharmacother. 158, 114152. doi:10.1016/j.biopha.2022.114152
Suzuki, J., Kanemaru, K., Ishii, K., Ohkura, M., Okubo, Y., and Iino, M. (2014). Imaging intraorganellar Ca2+ at subcellular resolution using CEPIA. Nat. Commun. 5, 4153. doi:10.1038/ncomms5153
Szobi, A., Farkašová-Ledvényiová, V., Lichý, M., Muráriková, M., Čarnická, S., Ravingerová, T., et al. (2018). Cardioprotection of ischaemic preconditioning is associated with inhibition of translocation of MLKL within the plasma membrane. J. Cell. Mol. Med. 22, 4183–4196. doi:10.1111/jcmm.13697
Tahmaz, I., Shahmoradi Ghahe, S., Stasiak, M., Liput, K. P., Jonak, K., and Topf, U. (2023). Prefoldin 2 contributes to mitochondrial morphology and function. BMC Biol. 21, 193. doi:10.1186/s12915-023-01695-y
Takenoshita, Y., Tokito, A., and Jougasaki, M. (2024). Inhibitory effects of eicosapentaenoic acid on vascular endothelial growth factor-induced monocyte chemoattractant protein-1, interleukin-6, and interleukin-8 in human vascular endothelial cells. Int. J. Mol. Sci. 25, 2749. doi:10.3390/ijms25052749
Tan, J., Yadav, M. K., Devi, S., and Kumar, M. (2022). Neuroprotective effects of arbutin against oxygen and glucose deprivation-induced oxidative stress and neuroinflammation in rat cortical neurons. Acta Pharm. 72, 123–134. doi:10.2478/acph-2022-0002
Tan, Y., Mui, D., Toan, S., Zhu, P., Li, R., and Zhou, H. (2020). SERCA overexpression improves mitochondrial quality control and attenuates cardiac microvascular ischemia-reperfusion injury. Mol. Ther. - Nucleic Acids 22, 696–707. doi:10.1016/j.omtn.2020.09.013
Tang, C., Chen, G., Wu, F., Cao, Y., Yang, F., You, T., et al. (2023). Endothelial CCRL2 induced by disturbed flow promotes atherosclerosis via chemerin-dependent β2 integrin activation in monocytes. Cardiovasc. Res. 119, 1811–1824. doi:10.1093/cvr/cvad085
Tang, Y., Wa, Q., Peng, L., Zheng, Y., Chen, J., Chen, X., et al. (2022). Salvianolic acid B suppresses ER stress-induced NLRP3 inflammasome and pyroptosis via the AMPK/FoxO4 and syndecan-4/rac1 signaling pathways in human endothelial progenitor cells. Oxid. Med. Cell. Longev. 2022, 8332825–8332922. doi:10.1155/2022/8332825
Tao, T., Zhu, Y., Shi, Y., Sun, B., Gu, Y., and Xu, S. (2024). Unveiling the role of PD-L1 in vascular endothelial dysfunction: insights into the mtros/NLRP3/caspase-1 mediated pyroptotic pathway. Exp. Cell Res. 438, 114047. doi:10.1016/j.yexcr.2024.114047
Tao, Z., Wei, B., Zhao, M., Zhang, X., Zhong, Y., and Wan, J. (2023). Hypoxia affects autophagy in human umbilical vein endothelial cells via the IRE1 unfolded protein response. Curr. Med. Sci. 43, 689–695. doi:10.1007/s11596-023-2749-y
Tian, H., Sun, H.-W., Zhang, J.-J., Zhang, X.-W., Zhao, L., Guo, S.-D., et al. (2015). Ethanol extract of propolis protects macrophages from oxidized low density lipoprotein-induced apoptosis by inhibiting CD36 expression and endoplasmic reticulum stress-C/EBP homologous protein pathway. BMC Complement. Altern. Med. 15, 230. doi:10.1186/s12906-015-0759-4
Tian, Y., Shi, D., Liao, H., Lu, B., and Pang, Z. (2023). The role of Huidouba in regulating skeletal muscle metabolic disorders in prediabetic mice through AMPK/PGC-1α/PPARα pathway. Diabetol. Metab. Syndr. 15, 145. doi:10.1186/s13098-023-01097-8
Toldo, S., and Abbate, A. (2024). The role of the NLRP3 inflammasome and pyroptosis in cardiovascular diseases. Nat. Rev. Cardiol. 21, 219–237. doi:10.1038/s41569-023-00946-3
Toma, L., Sanda, G. M., Niculescu, L. S., Deleanu, M., Stancu, C. S., and Sima, A. V. (2017). Caffeic acid attenuates the inflammatory stress induced by glycated LDL in human endothelial cells by mechanisms involving inhibition of AGE-receptor, oxidative, and endoplasmic reticulum stress. BioFactors 43, 685–697. doi:10.1002/biof.1373
Tong, W., Leng, L., Wang, Y., Guo, J., Owusu, F. B., Zhang, Y., et al. (2023). Buyang huanwu decoction inhibits diabetes-accelerated atherosclerosis via reduction of AMPK-Drp1-mitochondrial fission axis. J. Ethnopharmacol. 312, 116432. doi:10.1016/j.jep.2023.116432
Tsai, K., Hung, C., Chan, S., Hsieh, P., Ou, H., Cheng, Y., et al. (2018). Chlorogenic acid protects against oxLDL-induced oxidative damage and mitochondrial dysfunction by modulating SIRT1 in endothelial cells. Mol. Nutr. Food Res. 62, 1700928. doi:10.1002/mnfr.201700928
Vilas-Boas, E. A., Carlein, C., Nalbach, L., Almeida, D. C., Ampofo, E., Carpinelli, A. R., et al. (2021). Early cytokine-induced transient NOX2 activity is ER stress-dependent and impacts β-cell function and survival. Antioxidants 10, 1305. doi:10.3390/antiox10081305
Vinchi, F., Porto, G., Simmelbauer, A., Altamura, S., Passos, S. T., Garbowski, M., et al. (2020). Atherosclerosis is aggravated by iron overload and ameliorated by dietary and pharmacological iron restriction. Eur. Heart J. 41, 2681–2695. doi:10.1093/eurheartj/ehz112
Virani, S. S., Alonso, A., Aparicio, H. J., Benjamin, E. J., Bittencourt, M. S., Callaway, C. W., et al. (2021). Heart disease and stroke statistics—2021 update: a report from the American heart association. Circulation 143, e254–e743. doi:10.1161/CIR.0000000000000950
Wang, D., Yang, L., Ding, W., Chen, Z., Yang, X., Jiang, Y., et al. (2024). Licochalcone A alleviates abnormal glucolipid metabolism and restores energy homeostasis in diet-induced diabetic mice. Phytother. Res. 38, 196–213. doi:10.1002/ptr.8044
Wang, F., Jia, J., Song, H., Jia, C., Chen, C., and Ma, J. (2019a). Icariin protects vascular endothelial cells from oxidative stress through inhibiting endoplasmic reticulum stress. J. Integr. Med. 17, 205–212. doi:10.1016/j.joim.2019.01.011
Wang, H., Zheng, Z.-M., and Gao, B.-L. (2014). Guanxinkang decoction exerts its antiatherosclerotic effect partly through inhibiting the endoplasmic reticulum stress. Evid. Based Complement. Altern. Med. 2014, 465640–465648. doi:10.1155/2014/465640
Wang, J., Wu, R., Hua, Y., Ling, S., and Xu, X. (2023a). Naringenin ameliorates vascular senescence and atherosclerosis involving SIRT1 activation. J. Pharm. Pharmacol. 75, 1021–1033. doi:10.1093/jpp/rgad053
Wang, L., Astone, M., Alam, Sk. K., Zhu, Z., Pei, W., Frank, D. A., et al. (2021). Suppressing STAT3 activity protects the endothelial barrier from VEGF-mediated vascular permeability. Dis. Model. Mech. 14, dmm049029. doi:10.1242/dmm.049029
Wang, L., Hilander, T., Liu, X., Tsang, H. Y., Eriksson, O., Jackson, C. B., et al. (2023b). GTPBP8 is required for mitoribosomal biogenesis and mitochondrial translation. Cell. Mol. Life Sci. 80, 361. doi:10.1007/s00018-023-05014-0
Wang, Q., Zhang, M., Liang, B., Shirwany, N., Zhu, Y., and Zou, M.-H. (2011). Activation of AMP-activated protein kinase is required for berberine-induced reduction of atherosclerosis in mice: the role of uncoupling protein 2. PLoS ONE 6, e25436. doi:10.1371/journal.pone.0025436
Wang, Q., Zhang, M., Torres, G., Wu, S., Ouyang, C., Xie, Z., et al. (2017). Metformin suppresses diabetes-accelerated atherosclerosis via the inhibition of drp1-mediated mitochondrial fission. Diabetes 66, 193–205. doi:10.2337/db16-0915
Wang, X., Tao, T., Song, D., Mao, H., Liu, M., Wang, J., et al. (2019b). Calreticulin stabilizes F-actin by acetylating actin and protects microvascular endothelial cells against microwave radiation. Life Sci. 232, 116591. doi:10.1016/j.lfs.2019.116591
Wang, X., Zhang, J.-Q., Xiu, C.-K., Yang, J., Fang, J.-Y., and Lei, Y. (2020). Ginseng-sanqi-chuanxiong (GSC) extracts ameliorate diabetes-induced endothelial cell senescence through regulating mitophagy via the AMPK pathway. Oxid. Med. Cell. Longev. 2020, 7151946–7152022. doi:10.1155/2020/7151946
Wang, X., Zhang, M., Mao, C., Zhang, C., Ma, W., Tang, J., et al. (2023c). Icariin alleviates ferroptosis-related atherosclerosis by promoting autophagy in xo-LDL -induced vascular endothelial cell injury and atherosclerotic mice. Phytother. Res. 37, 3951–3963. doi:10.1002/ptr.7854
Wang, Y., Tao, T.-Q., Song, D.-D., and Liu, X.-H. (2018). Calreticulin ameliorates hypoxia/reoxygenation-induced human microvascular endothelial cell injury by inhibiting autophagy. Shock 49, 108–116. doi:10.1097/SHK.0000000000000905
Wang, Y.-H., Liu, Y.-P., Zhu, J.-Q., Zhou, G. H., Zhang, F., An, Q., et al. (2023d). Physcion prevents high-fat diet-induced endothelial dysfunction by inhibiting oxidative stress and endoplasmic reticulum stress pathways. Eur. J. Pharmacol. 943, 175554. doi:10.1016/j.ejphar.2023.175554
Wei, H., Zhan, L.-P., Zhang, B., Li, Y.-P., Pei, Z., and Li, L. (2019). dl-3n-butylphthalide reduces oxygen-glucose deprivation-induced endothelial cell damage by increasing PGC-1α. Eur. Rev. Med. Pharmacol. Sci. 23, 4481–4490. doi:10.26355/eurrev_201905_17960
Wu, D., Ji, H., Du, W., Ren, L., and Qian, G. (2022). Mitophagy alleviates ischemia/reperfusion-induced microvascular damage through improving mitochondrial quality control. Bioengineered 13, 3596–3607. doi:10.1080/21655979.2022.2027065
Wu, J., Xu, X., Li, Y., Kou, J., Huang, F., Liu, B., et al. (2014a). Quercetin, luteolin and epigallocatechin gallate alleviate TXNIP and NLRP3-mediated inflammation and apoptosis with regulation of AMPK in endothelial cells. Eur. J. Pharmacol. 745, 59–68. doi:10.1016/j.ejphar.2014.09.046
Wu, J., Zhang, W., Liu, X., Wu, L., He, G., Li, P., et al. (2018). Apocynin protects endothelial cells from endoplasmic reticulum stress-induced apoptosis via IRE1α engagement. Mol. Cell. Biochem. 449, 257–265. doi:10.1007/s11010-018-3362-4
Wu, L., Wang, D., Xiao, Y., Zhou, X., Wang, L., Chen, B., et al. (2014b). Endoplasmic reticulum stress plays a role in the advanced glycation end product-induced inflammatory response in endothelial cells. Life Sci. 110, 44–51. doi:10.1016/j.lfs.2014.06.020
Xi, J., Rong, Y., Zhao, Z., Huang, Y., Wang, P., Luan, H., et al. (2021). Scutellarin ameliorates high glucose-induced vascular endothelial cells injury by activating PINK1/Parkin-mediated mitophagy. J. Ethnopharmacol. 271, 113855. doi:10.1016/j.jep.2021.113855
Xian, H., Watari, K., Sanchez-Lopez, E., Offenberger, J., Onyuru, J., Sampath, H., et al. (2022). Oxidized DNA fragments exit mitochondria via mPTP- and VDAC-dependent channels to activate NLRP3 inflammasome and interferon signaling. Immunity 55, 1370–1385.e8. doi:10.1016/j.immuni.2022.06.007
Xiang, D., Yang, W., Fang, Z., Mao, J., Yan, Q., Li, L., et al. (2022a). Agrimol B inhibits colon carcinoma progression by blocking mitochondrial function through the PGC-1α/NRF1/TFAM signaling pathway. Front. Oncol. 12, 1055126. doi:10.3389/fonc.2022.1055126
Xiang, J., Zhang, C., Di, T., Chen, L., Zhao, W., Wei, L., et al. (2022b). Salvianolic acid B alleviates diabetic endothelial and mitochondrial dysfunction by down-regulating apoptosis and mitophagy of endothelial cells. Bioengineered 13, 3486–3502. doi:10.1080/21655979.2022.2026552
Xiang, Y., Peng, J., Nie, H., and Ou, B. (2020). In vitro investigation of protective mechanisms of triptolide against coronary heart disease by regulating miR-24-3p-BCL2L11 axis and PPARs-PGC1α pathway. Am. J. Transl. Res. 12, 7982–7994.
Xianpei, H., Liang, L. I., Liuqin, Y., and Zhita, W. (2022). Efficacy of Dangua Fang on endothelial cells damaged by oxidative stress. J. Tradit. Chin. Med. Chung Tsa Chih Ying Wen Pan 42, 900–907. doi:10.19852/j.cnki.jtcm.20220815.001
Xiao, P., Wang, C., Li, J., Su, H., Yang, L., Wu, P., et al. (2020). COP9 signalosome suppresses RIPK1-RIPK3–mediated cardiomyocyte necroptosis in mice. Circ. Heart Fail. 13, e006996. doi:10.1161/CIRCHEARTFAILURE.120.006996
Xing, S., Yang, X., Li, W., Bian, F., Wu, D., Chi, J., et al. (2014). Salidroside stimulates mitochondrial biogenesis and protects against H 2 O 2 -induced endothelial dysfunction. Oxid. Med. Cell. Longev. 2014, 1–13. doi:10.1155/2014/904834
Xu, Q., Xia, P., Li, X., Wang, W., Liu, Z., and Gao, X. (2014). Tetramethylpyrazine ameliorates high glucose-induced endothelial dysfunction by increasing mitochondrial biogenesis. PLoS ONE 9, e88243. doi:10.1371/journal.pone.0088243
Xue, X., Xi, W., Li, W., Xiao, J., Wang, Z., and Zhang, Y. (2023). Hydrogen-rich saline alleviates cardiomyocyte apoptosis by reducing expression of calpain1 via miR-124-3p. Esc. Heart Fail 10, 3077–3090. doi:10.1002/ehf2.14492
Yan, Z., Bai, X., Yan, C., Wu, J., Li, Z., Xie, T., et al. (2015). Structure of the rabbit ryanodine receptor RyR1 at near-atomic resolution. Nature 517, 50–55. doi:10.1038/nature14063
Yang, H., Xu, G., Li, Q., and Zhu, L. (2024). Ligustrazine alleviates the progression of coronary artery calcification by inhibiting caspase-3/GSDME mediated pyroptosis. Biosci. Trends 2024, 482–491. doi:10.5582/bst.2024.01096
Yang, J., Zhou, X., Zeng, X., Hu, O., Yi, L., and Mi, M. (2019). Resveratrol attenuates oxidative injury in human umbilical vein endothelial cells through regulating mitochondrial fusion via TyrRS-PARP1 pathway. Nutr. Metab. 16, 9. doi:10.1186/s12986-019-0338-7
Yao, W., Wang, K., Wang, X., Li, X., Dong, J., Zhang, Y., et al. (2021). Icariin ameliorates endothelial dysfunction in type 1 diabetic rats by suppressing ER stress via the PPARα/Sirt1/AMPKα pathway. J. Cell. Physiol. 236, 1889–1902. doi:10.1002/jcp.29972
Yu, S., Wang, S., Huang, S., Wang, W., Wei, Z., Ding, Y., et al. (2020). Radix et Rhizoma Ginseng chemoprevents both initiation and promotion of cutaneous carcinoma by enhancing cell-mediated immunity and maintaining redox homeostasis. J. Ginseng Res. 44, 580–592. doi:10.1016/j.jgr.2019.05.004
Yu, W., Liu, X., Feng, L., Yang, H., Yu, W., Feng, T., et al. (2017). Glycation of paraoxonase 1 by high glucose instigates endoplasmic reticulum stress to induce endothelial dysfunction in vivo. Sci. Rep. 7, 45827. doi:10.1038/srep45827
Zhang, A., Liu, K., Liang, X., Li, H., Fu, X., Zhu, N., et al. (2023a). Metal-phenolic capsules with ROS scavenging reshape the oxidative microenvironment of atherosclerosis. Nanomedicine Nanotechnol. Biol. Med. 53, 102700. doi:10.1016/j.nano.2023.102700
Zhang, J., Qiao, S. L., Han, Y. W., Xu, S. X., Lee, S. C., Wei, Z. Y., et al. (2023b). Advanced glycation end products initiate the mutual promoting cycle between centrosome amplification and the release of inflammatory cytokines in human vascular endothelial cells. Biochem. Biophys. Res. Commun. 681, 232–241. doi:10.1016/j.bbrc.2023.09.085
Zhang, X. M., Ma, Z., Mao, C., Zhang, J., and Ma, Z. (2023c). Effect of ligustrazine on lipopolysaccharide-induced inflammatory response of vascular endothelial cells and its possible mechanism. Pharmacogn. Mag. 19, 994–1002. doi:10.1177/09731296231197077
Zhang, Y., Zhou, H., Wu, W., Shi, C., Hu, S., Yin, T., et al. (2016). Liraglutide protects cardiac microvascular endothelial cells against hypoxia/reoxygenation injury through the suppression of the SR-Ca2+–XO–ROS axis via activation of the GLP-1R/PI3K/Akt/survivin pathways. Free Radic. Biol. Med. 95, 278–292. doi:10.1016/j.freeradbiomed.2016.03.035
Zhao, F., Jiang, H., Zhang, T., Chen, H., Li, W., Li, X., et al. (2023a). Mechanism repositioning based on integrative pharmacology: anti-inflammatory effect of safflower in myocardial ischemia–reperfusion injury. Int. J. Mol. Sci. 24, 5313. doi:10.3390/ijms24065313
Zhao, H., Wang, P., Wang, X., Du, W., Yang, H.-H., Liu, Y., et al. (2023b). Lipocalin 10 is essential for protection against inflammation-triggered vascular leakage by activating LDL receptor-related protein 2-slingshot homologue 1 signalling pathway. Cardiovasc. Res. 119, 1981–1996. doi:10.1093/cvr/cvad105
Zhao, Y., Li, Q., Zhao, W., Li, J., Sun, Y., Liu, K., et al. (2015). Astragaloside IV and cycloastragenol are equally effective in inhibition of endoplasmic reticulum stress-associated TXNIP/NLRP3 inflammasome activation in the endothelium. J. Ethnopharmacol. 169, 210–218. doi:10.1016/j.jep.2015.04.030
Zhou, H., Hu, S., Jin, Q., Shi, C., Zhang, Y., Zhu, P., et al. (2017a). Mff-dependent mitochondrial fission contributes to the pathogenesis of cardiac microvasculature ischemia/reperfusion injury via induction of mROS-mediated cardiolipin oxidation and HK2/VDAC1 disassociation-involved mPTP opening. J. Am. Heart Assoc. 6, e005328. doi:10.1161/JAHA.116.005328
Zhou, H., Wang, J., Zhu, P., Hu, S., and Ren, J. (2018). Ripk3 regulates cardiac microvascular reperfusion injury: the role of IP3R-dependent calcium overload, XO-mediated oxidative stress and F-action/filopodia-based cellular migration. Cell. Signal. 45, 12–22. doi:10.1016/j.cellsig.2018.01.020
Zhou, H., Zhang, Y., Hu, S., Shi, C., Zhu, P., Ma, Q., et al. (2017b). Melatonin protects cardiac microvasculature against ischemia/reperfusion injury via suppression of mitochondrial fission- VDAC 1- HK 2- mPTP -mitophagy axis. J. Pineal Res. 63, e12413. doi:10.1111/jpi.12413
Zhou, Y., Wang, L., Liu, Y., Fan, L., Zhang, X., Shi, Q., et al. (2023). Homoplantaginin attenuates high glucose-induced vascular endothelial dysfunction via inhibiting store-operated calcium entry channel and endoplasmic reticulum stress. J. Pharm. Pharmacol. 75, 1530–1543. doi:10.1093/jpp/rgad087
Zhu, H., Jin, Q., Li, Y., Ma, Q., Wang, J., Li, D., et al. (2018). Melatonin protected cardiac microvascular endothelial cells against oxidative stress injury via suppression of IP3R-[Ca2+]c/VDAC-[Ca2+]m axis by activation of MAPK/ERK signaling pathway. Cell Stress Chaperones 23, 101–113. doi:10.1007/s12192-017-0827-4
Zhu, L., Bao, Y., Liu, Z., Liu, J., Li, Z., Sun, X., et al. (2024). Gualou-Xiebai herb pair ameliorate atherosclerosis in HFD-induced ApoE−/− mice and inhibit the ox-LDL-induced injury of HUVECs by regulating the Nrf2-mediated ferroptosis. J. Ethnopharmacol. 326, 117892. doi:10.1016/j.jep.2024.117892
Zhu, L., Jia, F., Wei, J., Yu, Y., Yu, T., Wang, Y., et al. (2017). Salidroside protects against homocysteine-induced injury in human umbilical vein endothelial cells via the regulation of endoplasmic reticulum stress. Cardiovasc. Ther. 35, 33–39. doi:10.1111/1755-5922.12234
Zhu, S., Li, X., Dang, B., Wu, F., Wang, C., and Lin, C. (2022). Lycium Barbarum polysaccharide protects HaCaT cells from PM2.5-induced apoptosis via inhibiting oxidative stress, ER stress and autophagy. Redox Rep. 27, 32–44. doi:10.1080/13510002.2022.2036507
Zhu, Y., Han, X.-Q., Sun, X.-J., Yang, R., Ma, W.-Q., and Liu, N.-F. (2020). Lactate accelerates vascular calcification through NR4A1-regulated mitochondrial fission and BNIP3-related mitophagy. Apoptosis 25, 321–340. doi:10.1007/s10495-020-01592-7
Zhu, Y., Li, M., Lu, Y., Li, J., Ke, Y., and Yang, J. (2019). Ilexgenin A inhibits mitochondrial fission and promote Drp1 degradation by Nrf2-induced PSMB5 in endothelial cells. Drug Dev. Res. 80, 481–489. doi:10.1002/ddr.21521
Zulli, A., Lau, E., Wijaya, B. P. P., Jin, X., Sutarga, K., Schwartz, G. D., et al. (2009). High dietary taurine reduces apoptosis and atherosclerosis in the left main coronary artery: association with reduced CCAAT/enhancer binding protein homologous protein and total plasma homocysteine but not lipidemia. Hypertension 53, 1017–1022. doi:10.1161/HYPERTENSIONAHA.109.129924
Keywords: ischemic cardiomyopathy, inflammatory vascular injury, mitochondrial quality control, endoplasmic reticulum, oxidative stress, traditional chinese medicine
Citation: Zhang C, Chang X, Zhao D, He Y, Dong G and Gao L (2025) Decoding interaction between mitochondria and endoplasmic reticulum in ischemic myocardial injury: targeting natural medicines. Front. Pharmacol. 16:1536773. doi: 10.3389/fphar.2025.1536773
Received: 29 November 2024; Accepted: 07 February 2025;
Published: 28 February 2025.
Edited by:
Fang-Rong Chang, Kaohsiung Medical University, TaiwanReviewed by:
Priyanka Choudhury, Medical College of Wisconsin, United StatesYing Xie, Case Western Reserve University, United States
Copyright © 2025 Zhang, Chang, Zhao, He, Dong and Gao. This is an open-access article distributed under the terms of the Creative Commons Attribution License (CC BY). The use, distribution or reproduction in other forums is permitted, provided the original author(s) and the copyright owner(s) are credited and that the original publication in this journal is cited, in accordance with accepted academic practice. No use, distribution or reproduction is permitted which does not comply with these terms.
*Correspondence: Guangtong Dong, MTAwNzgwOTIwNkBxcS5jb20=; Lin Gao, Z2FvbGluOTNAYWxpeXVuLmNvbQ==
†These authors have contributed equally to this work