- 1Anesthesia and Critical Care Department, Juan Ramón Jiménez Hospital, Huelva, Spain
- 2Fluid Therapy and Hemodynamic Group of the Hemostasia, Transfusion Medicine, Fluid Therapy Section of the Spanish Society of Anesthesia and Critical Care (SEDAR), Madrid, Spain
- 3School of Medicine and Health Sciences, International University of Catalonia (UIC), Barcelona, Spain
- 4Anesthesia and Critical Care Department, Infanta Leonor Hospital, Madrid, Spain
- 5Clinical Management Anesthesiology Unit, Resuscitation, and Pain Therapy, Virgen del Rocio Hospital, Sevilla, Spain
- 6Anesthesia and Critical Care Department, Virgen Macarena Hospital, Sevilla, Spain
- 7Anesthesia and Critical Care Department, Hospital Universitario SAS de Jerez, Jerez de la Frontera, Spain
- 8Anesthesia and Critical Care Department, Complejo Hospitalario de Badajoz, Badajoz, Spain
Background: Intraoperative hypotension (IOH) is associated with increased morbidity and mortality after major abdominal surgery but remains significant even when using goal-directed hemodynamic therapy (GDHT) protocols. The Hypotension Prediction Index (HPI) is a machine learning-derived parameter that predicts arterial hypotension. We tested the hypothesis that an HPI-based protocol reduces the duration and severity of hypotension compared with a GDHT protocol during major abdominal surgery.
Methods: This is a parallel-arm double-blinded multicenter randomized trial involving adult patients undergoing elective major abdominal surgery at five centers. Patients were optimized according to a previously recommended GDHT protocol (GDHT group) or the HPI value (HPI group). Hemodynamic optimization in both groups started 15 min after the surgical incision. The primary outcome was the intraoperative time-weighted average of mean arterial pressure under 65 mmHg (TWA-MAP < 65 mmHg). Other metrics for IOH and secondary outcomes, including TWA below individual baseline values of intraoperative tissue oxygenation (StO2), postoperative AKIRisk, postoperative complications, length of stay, and 30-day mortality, were explored.
Results: Eighty patients were randomized (40 patients in each group). TWA-MAP < 65 mmHg was 0.06 (25th–75th interquartile range: 0–0.27) mmHg in the GDTH group vs. 0 (0–0.04) mmHg in the HPI group (p = 0.015). Total time with MAP < 65 mmHg per patient was 4.6 (0–21) min in the GDHT group and 0 (0–3) min in the HPI group (p = 0.008). The TWA below the baseline StO2 was 0.40% (0.12%–2.41%) in the GDHT group and 0.95% (0.15%–3.20%) in the HPI group (p = 0.353). The AKIRisk values obtained in the GDHT group were 0.30 (0.14–0.53) and 0.34 (0.15–0.67) in the GDHT and HPI groups (p = 0.731), respectively. Both groups had similar postoperative complications, length of stay, and 30-day mortality.
Conclusions: An HPI-based protocol reduced intraoperative hypotension compared with a standard GDHT protocol, with no differences in tissue oxygenation and postoperative AKIRisk.
1. Introduction
More than 300 million patients undergo surgery worldwide each year (1). Despite technological advances and the adoption of perioperative care pathways, postoperative complications remain frequent, affecting quality of life and postoperative mortality (2).
Intraoperative goal-directed hemodynamic therapies (GDHT) have traditionally attempted to optimize oxygen delivery and may significantly improve outcomes in patients undergoing non-cardiac surgery (3–5). Adequate perfusion pressure is crucial for avoiding tissue hypoxia and maintaining organ function during the perioperative period (6) and is a fundamental part of most GDHT protocols (7). Although Goldman and Caldera already demonstrated the crucial role of intraoperative arterial pressure management (8), in 2007, Bijker et al. reported significant variability in published definitions of hypotension, describing 140 different definitions in 130 reviewed articles (9). More recently, based on the association between intraoperative mean arterial pressure (MAP) and adverse postoperative outcomes, a MAP < 65 mmHg is now recommended for defining intraoperative hypotension (IOH) (10). However, a unidimensional definition of IOH is incomplete, as both the severity and duration of IOH have been associated with poor postoperative outcomes (11, 12).
Arterial hypotension is frequent during the intraoperative period, with an incidence estimated between 5% and 99%, depending on the definition used (9). In a recent study, 87% of patients who underwent surgery experienced at least one episode of intraoperative hypotension, defined as a MAP < 65 mmHg for at least 1 min, even when receiving hemodynamic optimization according to a GDHT protocol (13).
The Hypotension Prediction Index (HPI) is a validated algorithm developed using machine learning techniques based on arterial pressure waveform analysis that predicts the occurrence of arterial hypotension (14). The use of HPI coupled with a proactive therapeutic attitude may reduce intraoperative hypotension in adults undergoing non-cardiac surgery (15). In this multicenter study, we hypothesize that an HPI-based therapeutic protocol will reduce the overall duration and severity of intraoperative hypotension and may improve tissue oxygenation and postoperative risk for kidney dysfunction in adult patients undergoing major abdominal surgery compared with a recommended GDHT protocol (16).
2. Material and methods
This manuscript was written according to the CONSORT statement (checklist available in Appendix A, Supplementary Material). The Predict-H trial was a parallel-arm double-blinded multicenter randomized controlled trial. The study was carried out at five Spanish hospitals: the Juan Ramón Jiménez University Hospital (Huelva), the Virgen del Rocío University Hospital (Sevilla), the Infanta Leonor University Hospital (Madrid), the Hospital Universitario SAS de Jerez (Jerez de la Frontera), and the Infanta Cristina University Hospital (Badajoz). The trial was registered in ClinicalTrials.gov, identifier NCT04301102; Principal Investigator, Juan Victor Lorente; March 10, 2020. Ethics Committee approval was obtained from the Ethics Committee of Hospital Gregorio Marañón (Madrid, Spain). The full trial protocol has been published previously (17). Written informed consent was obtained from all included patients.
This study was conducted in accordance with the original protocol from November 2020 to February 2022. Patients were eligible for enrollment if they were aged more than 65 years old and/or had American Society of Anesthesiologists (ASA) physical status III/IV and were scheduled for elective major abdominal surgery, including general surgery, urology, or gynecology, through a laparoscopic or open approach, with general or combined anesthesia. Surgery was considered major if the expected duration was >2 h, the estimated blood loss was >15% of blood volume, or if the expected required transfusion was ≥2 packed red blood cells. The exclusion criteria were pregnancy, preoperative glomerular filtrate of <60 ml/min/1.73 m2 according to the CKD-EPI 2009 formula (18), persistent atrial fibrillation, known cardiac shunts, right ventricular dysfunction, severe valvulopathy, kidney transplant recipient, and refusal to participate in the study.
2.1. Randomization
A research assistant at each center assessed patient eligibility and enrollment. This assistant obtained informed consent from all patients. The principal investigator at each center assigned patients to the intraoperative HPI protocol (HPI group) or a GDHT protocol (GDHT group) through a computer-generated 1:1 allocation sequence with a variable block randomization method through age strata. Patients were blinded to their randomization group.
2.2. Common perioperative measures
Patients from both groups received general or combined anesthesia. The neuraxial analgesia technique was performed according to the anesthesiologist's preference before induction. For pragmatic reasons, the administration of the drugs used in the anesthesia induction was at the anesthesiologist's discretion. Bispectral-index monitoring (BIS, Medtronic, Dublin, Ireland) was used to monitor the depth of anesthesia. Sevoflurane or propofol was used for hypnosis maintenance. After the induction of anesthesia, all patients received a radial artery catheter connected to a FloTrac® sensor (Edwards Lifesciences, Irvine, CA, USA) in the GDHT group or an AcumenIQ® sensor in the HPI group (Edwards Lifesciences).
All patients received standard measures to maintain oxygen saturation by pulse oximetry of >94%, normothermia (>36°C), and a heart rate of <100 beats/min. Ventilation with an inspired oxygen fraction of 60% was mechanically controlled to maintain PaCO2 between 4.7 and 6.0 kPa, with a positive end-expiratory pressure of 4–6 mmHg and a tidal volume of 8 ml/kg. For maintenance fluid therapy, a balanced crystalloid (Isofundin®/Plasmalyte®) was administered at 1–3 ml/kg/h for laparoscopic surgery and 5–7 ml/kg/h for open surgery. Packed red blood cells were transfused if the hemoglobin level was <8 g/dl. The choice and dose of the vasopressor and ionotropic drugs were determined by the anesthesiologist in charge of the patient in both groups.
Tissue oxygen saturation (StO2) was continuously recorded every 2 s by placing an adult sensor (ForeSight Elite® sensor, model FSESL, Edwards Lifesciences) over the brachioradialis muscle using near-infrared spectroscopy (NIRS) technology. StO2 values were hidden from the main screen in both groups but were recorded internally into the HemoSphere monitor. Urinary kidney stress biomarkers [TIMP-2]-[IGFBP7] were measured with an Astute140 Meter (BioMérieux). This device applies a sandwich immunoassay technique and converts the fluorescent signals from each immunoassay (TIMP-2 and IGFBP7) contained within the Nephrocheck test cartridge into a single numerical risk result (AKIRisk). The first urine sample was collected when performing the bladder catheterization after induction. The first postoperative sample was collected 4 h after the patient's admission to the intensive care unit. If the value of this sample was between 0.3 and 2, a second postoperative sample was collected 12 h after the first one (19). Urinary [TIMP-2]-[IGFBP7] was measured by the principal investigator at each center and blinded to the rest of the researchers.
The intervention period (optimization time) began 15 min after the surgical incision and continued until wound closure. The primary hemodynamic goal for both groups before this period was to maintain a mean arterial pressure (MAP) of >65 mmHg by administering vasopressors as necessary.
All hemodynamic data were recorded every 20 s using the HemoSphere system and were downloaded after surgery for offline analysis. Artifacts in blood pressure measurements were excluded if they met one or more of the following criteria: a null value was recorded because the monitor could not calculate MAP; SAP ≥300 mmHg or <20 mmHg; diastolic pressure ≥225 mmHg or ≤5 mmHg; systolic pressure ≤ diastolic pressure 5 mmHg; or if the absolute value of the second derivative of MAP >15 mmHg·s2. If missing values accounted for less than 1 min, MAP was linearly interpolated from the available values.
Data related to the total fluid therapy administered, the accumulated dose of vasoactive agents and inotropes, and the transfusion of whole-blood products during surgery were collected. A research assistant at each hospital, who was blinded to the group allocation, conducted daily follow-ups of patients until their discharge from the hospital.
2.3. Hemodynamic management
2.3.1. GDHT group
In the GDHT group, hemodynamic management was based on the variables provided by the HemoSphere monitor connected to a FloTrac sensor, including cardiac index (CI), stroke volume variation (SVV), and MAP. The hemodynamic optimization protocol for this group is depicted in Figure 1. This protocol is a previously published (16) and recommended algorithm for intraoperative flow optimization (GDHT), not specifically designed for IOH reduction. If the SVV value increased above 13%, a fluid bolus of 250 ml of colloid was administered and repeated until SVV was <13%. MAP was maintained above 65 mmHg by a vasoconstrictor drug after fluid optimization of SVV. An ionotropic agent was added if the CI persisted at <2.5 L/ml/m2 after the previous steps (16).
2.3.2. HPI group
In the HPI group, hemodynamic management was based on the hypotension alert provided by HPI in combination with SVV, the maximum rise of arterial pressure (dP/dtmax) as a surrogate for assessing left ventricular contractility (20), and dynamic arterial elastance (Eadyn) for determining the pressure-responsiveness (21). The hemodynamic optimization protocol on the HPI group aimed to prevent the main mechanisms leading to arterial hypotension: hypovolemia, impaired contractility, and vasoplegia (Figure 2). When HPI rose above 85%, SVV was checked. If SVV was <13% and dP/dtmax was >400 mmHg·s, a vasoconstrictor was administered, whereas an inotrope was administered if dP/dtmax was <400 mmHg·s. On the other hand, if SVV was >13% and the Eadyn value was >1, a fluid bolus of 250 ml of colloid was administered; a vasoconstrictor was administered if the Eadyn was <1.
2.4. Outcomes
2.4.1. Primary outcome
The primary outcome was the time-weighted average (TWA) of arterial hypotension during surgery, which considers the duration and depth of arterial hypotension, the two main factors associated with the risk of developing tissue hypoperfusion (12).
The TWA for arterial hypotension was calculated as follows:
As TWA-MAP < 65 mmHg weights the amount of intraoperative hypotension by the total optimization time (defined from the onset of hemodynamic optimization until the closure of the surgical wound), it represents a more helpful variable for comparing the severity and duration of IOH among different populations.
2.4.2. Exploratory outcomes
2.4.2.1. Other metrics for IOH
As exploratory outcomes, we also analyzed the total hypotension time per case, the incidence of hypotension (the number of hypotensive events per patient), and arterial pressure variability. A hypotensive event was defined as a MAP value of <65 mmHg for at least 1 min. MAP variability, a variable that has been independently associated with 30-day mortality in the non-cardiac surgery population, was assessed using the generalized averaged real blood pressure variability (generalized aVR), an index of short-term blood pressure variation proposed by Mascha et al. and measured as the sum of consecutive jumps or drops across surgery (10):
where N is the number of arterial pressure measurements averaged every minute and T is the duration of the optimization period, expressed in minutes.
2.4.2.2. Secondary outcomes
We calculated the time-weighted average below an individual StO2-specific threshold obtained as the average StO2 value during the first minute of optimization. We also identified the minimum StO2 value in both groups, defined as the minimum value sustained for at least 5 min (22).
The time-weighted average of StO2 below this baseline reference value was measured by calculating the area under the threshold defined divided by the total duration of surgery. We also compared the AKIRisk at baseline and the evolution among both groups.
Secondary outcomes also included postoperative complications defined by the European Perioperative Clinical Outcome (EPCO) definitions (23), length of hospital stay, and 30-day mortality. A designated data collector was responsible for data collection, including blood sampling and AKIRisk measurements; this individual was blinded to patient allocation.
2.5. Sample size
The sample size was based on the previous report of the mean and standard deviation of TWA-MAP < 65 mmHg. According to Wijnberge et al., we estimated a mean and standard deviation TWA-MAP < 65 mmHg of 0.5 and 0.51 in the control group, respectively. For a 75% reduction in TWA-MAP < 65 mmHg, the calculated effect size was 0.735. A sample size of 80 patients, 40 in each group, would provide a 90% power to detect a two-sided 0.05 significance level. Sample size was calculated using G* Power software v3.9.7 (Universitat Kiel, Kiel, Germany).
2.6. Statistical analysis
The normality of data distribution was assessed using the Shapiro–Wilk test. Continuous data are presented as the mean and the standard deviation (SD) or the median (25th–75th interquartile range, IQR) when non-normally distributed.
Categorical variables are expressed as absolute counts and percentages. Differences in primary and secondary outcomes were assessed using the Mann–Whitney–Wilcoxon test. The median of differences and their 95% confidence intervals were calculated using the Hodges–Lehmann method. Chi-square or the exact Fisher test was used for the intergroup comparisons for categorical variables. We fitted a linear mixed model to detect differences in the AKIRisk, creatinine, and serum lactate evolution between the HPI and GDHT groups. The analysis was performed using restricted maximum likelihood (REML), including study group, time, and their interaction as fixed factors, and subjects as a random effect. The model employed a random intercept and slope (with an unstructured covariance structure) to allow for individual differences in baseline AKIRisk, creatinine, and serum lactate levels, as well as their changes over time. The quasipoisson regression model was used to compare the LOS between groups.
Two-tailed testing was conducted for all hypothesis testing, and p < 0.05 was considered statistically significant. All statistical analyses were performed by an external statistician using R (R Core Team: A language and environment for statistical computing. R Foundation for Statistical Computing, Vienna, Austria).
3. Results
One hundred and thirty-two patients were assessed for eligibility. A total of 80 patients were recruited at the five participating hospitals between November 2020 and February 2022. The study flowchart is shown in Figure 3. Table 1 shows the baseline characteristics of the study population. Most patients were male (64%), aged between 65 and 74 years (46%), and had an ASA status of III (58%). Colorectal surgery was the most frequent surgery in both groups. The neuroaxial analgesia technique was performed in 26 patients in the GDHT group vs. 25 patients in the HPI group (p = 0.8172). The duration of the optimization period was significantly lower in the HPI group [165 (212–190) min vs. 198 (143–241) min]; p = 0.027). Both groups had a comparable surgery duration (p = 0.323).
Table 2 shows both groups' cumulative doses of vasoactive and fluid therapy during the intraoperative period. Patients in the GDHT group received more colloids, and only one in the HPI group received a dobutamine infusion. We observed a trend increase in serum lactate levels but no significant differences between both groups (Figure 4). There were no differences in the total volume of fluid therapy or transfusion of whole-blood products during surgery nor in the accumulated dose of vasoactive agents or ionotropic drugs during the intraoperative period.
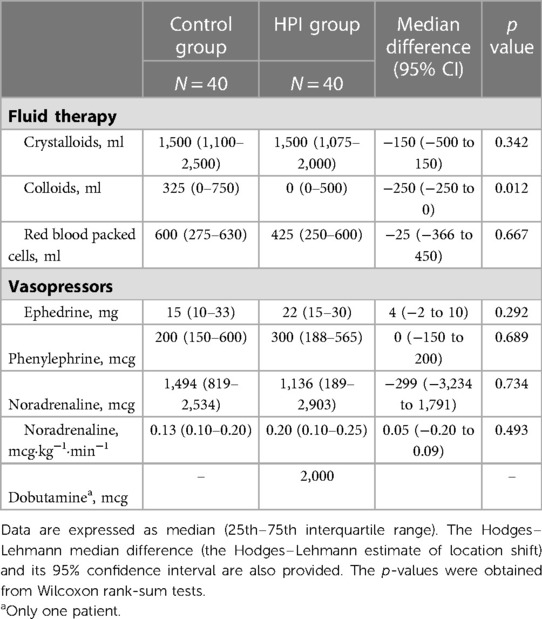
Table 2. Cumulative doses of fluids, blood transfusions, and vasoactive drugs administered intraoperatively.
3.1. Primary outcomes
The TWA-MAP < 65 mmHg was 0.06 (0–0.27) mmHg in the GDHT group vs. 0.00 (0.00–0.04) mmHg in the HPI group [median difference: −0.01 (95% CI: 0.09–0), p = 0.015].
3.2. Exploratory outcomes
3.2.1. Other metrics for IOH
The total time of MAP < 65 mmHg per patient was 4.61 (0.0–20.9) min in the GDHT group and 0.00 (0.0–3.2) min in the HPI group [median difference: −2.23 (95% CI: 9.08–0), p = 0.008]. The number of hypotensive events was significantly lower in the HPI group [0 (0–1) vs. 1 (0–4)], [median difference: 0 (95% CI: −2 to 0), p < 0.010]. The AUC MAP < 65 mmHg was 9.80 (0.00–42.80) mmHg per min in the GDHT group and 0.00 [0.00–6.97] mmHg per min in the HPI-group [median difference: −2.28 (95% CI: −21.03 to 0), p = 0.015]. No significant differences were observed in minimum MAP values and arterial pressure variability (aVR) (Table 3, Figure 5).
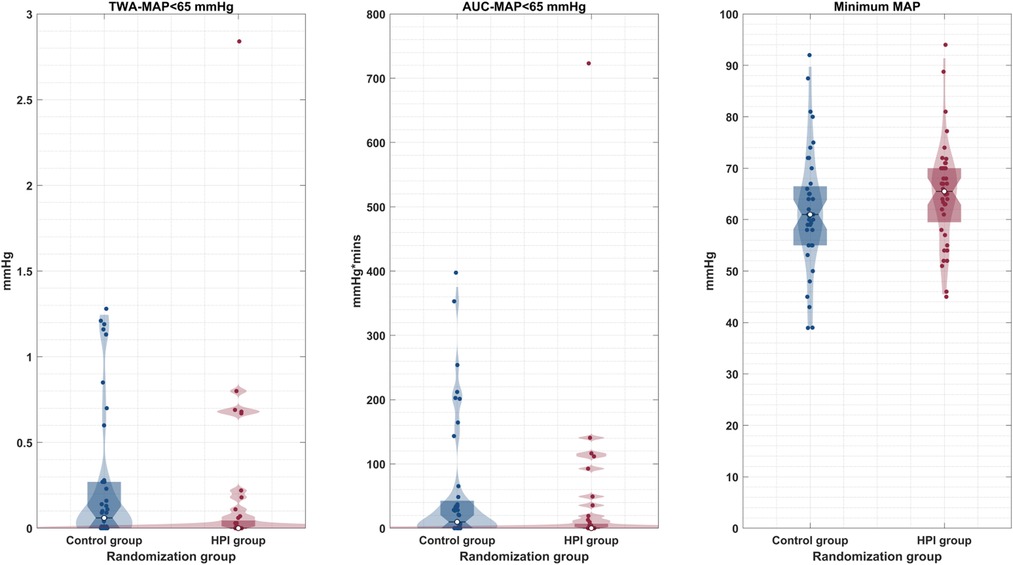
Figure 5. Distribution of minimum mean arterial pressure (MAP), time-weighted average MAP < 65 mmHg, and area under the curve MAP < 65 mmHg in both study groups.
3.2.2. Secondary outcomes
3.2.2.1. Tissue oxygenation
The results of intraoperative StO2 can be found in Table 4. The averaged StO2 value during the first minute of optimization was 73% (65%–83%) in the GDHT group and 74% (67%–82%) in the HPI group [median difference: 0 (95% CI: −6 to 6), p = 0.836]. The TWA below this baseline value was 0.40% (0.12%–2.41%) in the GDHT group and 0.95 (0.15%–3.20%) in the HPI group [median difference: −0.13 (95% CI: −0.13 to 0.85), p = 0.353]. The AUC under the baseline reference value in the GDHT group was 90% (18%–487%) and 160% (22–447) per min in the GDHT and HPI groups, respectively [median difference: 6 (95% CI: −47 to 92), p = 0.676]. The minimum StO2 value sustained at least for 5 min in the GDHT group was 65% (59%–76%) vs. 66% (58%–73%) in the HPI group [median difference: −1 (95% CI: −6 to 4), p = 0.840] (Figure 6).
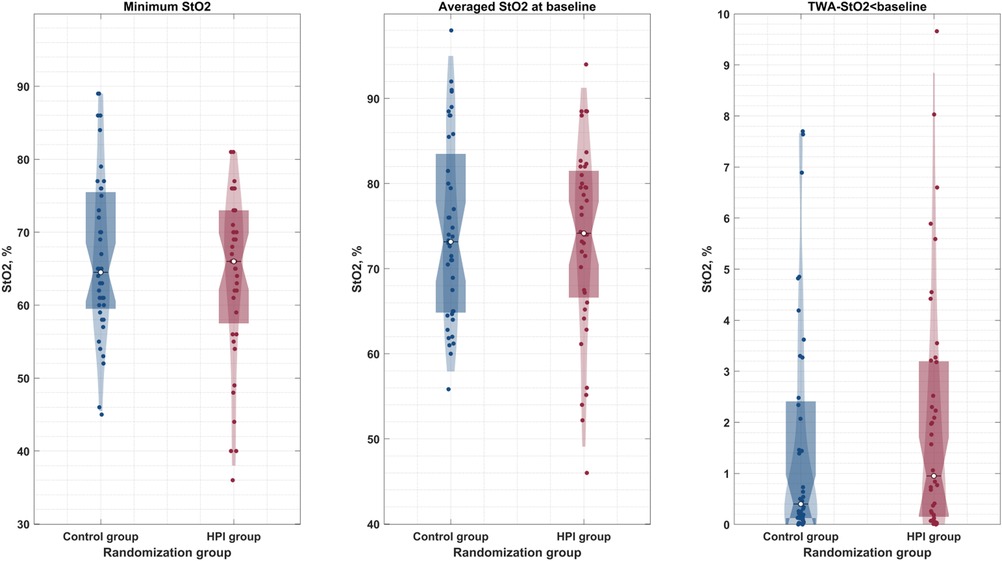
Figure 6. Distribution of minimum StO2, averaged StO2 at baseline, and time-weighted average StO2 <baseline in both study groups.
3.2.2.2. AKIRisk
Perioperative AKIRisk values are shown in Table 4. AKIRisk obtained just after induction was similar in both groups. The AKIRisk values collected 4 h after surgery were 0.30 (0.14–0.53) and 0.34 (0.15–0.67) in the GDHT and HPI groups, respectively [median difference: 0.01 (95% CI: −0.11 to 0.17), p = 0.731]. There was no significant association between the AKIRisk value or the creatinine level and the study group, time, and the interaction between both factors in the linear mixed model analysis (Figure 7).
3.2.2.3. Other postoperative outcomes
Table 5 shows the results for postoperative complications. The percentage of patients who experienced postoperative complications was similar in both groups: 32.5% vs. 22.5% [χ2 (1, N = 80) = 0.991, p = 0.3196]. LOS was similar in both groups: 10.2 (8.5) days in the GDHT group vs. 10.8 (16.1) days in the HPI group [OR 1.06 (95% confidence interval: 0.642–1.732, p = 0.828)]. All patients included in both groups were alive 30 days after the surgery.
4. Discussion
In this multicenter trial, an HPI-based therapeutic protocol significantly reduced intraoperative hypotension compared with a goal-directed hemodynamic therapy protocol in adult patients undergoing major abdominal surgery. However, there were no differences between intraoperative StO2 and postoperative AKIRisk values.
Intraoperative hypotension has been increasingly recognized as a preventable and potentially avoidable phenomenon associated with worse postoperative outcomes, such as acute kidney injury, myocardial injury, and mortality (11, 12). Clinical decision support systems that are designed to continuously monitor and identify patients at a high risk of developing hemodynamic instability have the potential to improve the timely recognition of the need for immediate hemodynamic support (24). Multiparameter systems using machine learning to analyze complex associations between physiological variables and the occurrence of hemodynamic instability have been proposed as a way to accurately stratify patients at risk (25). Our results corroborate previous studies on the usefulness of HPI for reducing IOH (15, 26) and extend those reports by comparing its performance against a standard goal-directed therapy protocol. An intraoperative hemodynamic protocol based on proactive management aimed at preventing arterial hypotension offered a significant reduction in the severity and duration of IOH compared with a flow-optimization protocol without affecting tissue perfusion. However, the contribution of this reduction in IOH to postoperative outcomes is yet to be determined.
GDHT algorithms have not been designed explicitly for IOH reduction. Their implementation is not the definitive solution to this problem. In a recent study, a significant hypotensive burden was accumulated during surgery despite using an intraoperative GDHT protocol (13). Moreover, considering that the association between IOH and postoperative outcomes is independent of the optimal cardiac index (27), the application of an HPI-based protocol provides an additional benefit, reflected in the IOH reduction and the lower duration of IOH per case in the HPI group. The HPI provides time for the clinician to select the adequate treatment based on advanced hemodynamic parameters before hypotension occurs. In this study, once the HPI value rose above 85%, the anesthesiologist started a protocol-guided treatment that provides three options, fluid administration, vasoconstrictor, or inotropes, thus allowing a proactive approach to the intraoperative management of IOH based on the main mechanisms leading to arterial hypotension.
Compared with similar studies evaluating the impact of the HPI algorithm (15, 28) on IOH, the TWA-MAP < 65 mmHg in our GDHT group was significantly lower. In the HYPE study, the median TWA-MAP < 65 mmHg in the control group was 0.44 (0.23–0.72) mmHg, whereas Maheshwari K et al. reported a median of 0.14 (0.03–0.39) mmHg in the unguided group. However, we must consider that, in these studies, patients in the control group received standard care, which usually means no specific hemodynamic protocol was used. In our GDHT group, however, a widely accepted hemodynamic optimization protocol (16) was employed. In daily practice, less than 30% of patients undergoing colorectal surgery receive an intraoperative GDHT protocol (29). Therefore, the differences in IOH observed in our study groups could have been even greater if a control group closer to these real-life conditions was used.
The TWA-MAP < 65 mmHg in the HPI group was lower than in two previous randomized controlled trials using an HPI-based protocol to reduce IOH in patients undergoing abdominal surgery (15, 30). In these trials, the preoperative comorbidity of the included patients, represented by ASA status, was similar. In the HYPE trial (15), the authors reported a TWA-MAP < 65 mmHg higher than our GDHT group [0.1 (0–0.43) mmHg]. The use of a relatively complex hemodynamic diagnostic and treatment guide and the inclusion of esophageal surgery patients in their intervention group may have influenced our better results. Murabito et al. (30) also reported a higher TWA-MAP < 65 mmHg [0.12 (0.35) mmHg]. The arterial line was placed before anesthesia induction in contrast to our study.
Our results contrast with the lack of benefit of using an intraoperative HPI-guided protocol found in the Maheshwari et al. study (28). However, as acknowledged by their authors, the lack of benefits in this study could be explained by several factors: an excessively complicated HPI treatment protocol, poor adherence to the recommendation suggested by the protocol, and the lack of use of lower HPI values for alerting the physician of increased hemodynamic instability and recommending checking the potential cause, as in our protocol.
Our results highlight the value of our HPI protocol. First, most situations contemplated in our HPI protocol involve treatment, not just observation, encouraging a proactive attitude toward reducing IOH. Second, its simplicity allows the anesthesiologist in charge to act quickly in case of an alarm. In addition, it offers a tailored treatment based on the main pathophysiological mechanisms related to IOH according to the continuous assessment of SVV, dP/dtmax, and Eadyn. The HPI protocol in our intervention group provided time for the anesthesiologist to perform the most physiologically adequate treatment for the patient.
Undetected tissue hypoxia may contribute to postoperative organ dysfunction (31). Therefore, evaluating whether a potential reduction of IOH in the HPI group was associated with better intraoperative perfusion was another element of our study. However, no significant differences in StO2 or AKIRisk values were observed between both groups, despite the overall reduction in IOH compared with the control group.
Our results reinforce the value of the GDHT protocol for preventing organ hypoperfusion. The low IOH exposure achieved in both groups, despite using different strategies (flow optimization in the control group vs. pressure optimization in the intervention group), may explain the absence of improvement in tissue oxygenation and postoperative AKIRisk values in the HPI group. However, our study was not primarily aimed at detecting such differences, which would require a much larger study population. Finally, LOS was longer compared with large observational cohorts in abdominal surgery (29, 32) and similar in both study groups, despite the low incidence of postoperative complications, probably due to the high preoperative patient comorbidity and the high-risk surgery performed.
Our study has several limitations that should be considered. First, the arterial line was placed after anesthesia induction, according to the standard care of all participating centers. It should be considered a limitation because up to one-third of IOH occurs shortly after induction (33). As the HPI algorithm cannot predict hypotension derived from external pharmacological and mechanical interventions, such as induction and laryngoscopy, differences in MAP values and their contributions to the incidence and severity of hypotension in this period were not analyzed. Second, we do not report clinician response time following the HPI alarm nor the percentage of intraoperative time with values above the targets set by both protocols. However, even if the adherence to the study protocol was not recorded, it was carefully checked by one of the PIs in each case. Moreover, all participating centers have previous experience performing intraoperative GDHT and using HPI. Thus, all of them were previously trained before the study started. Third, the optimization duration was lower in the HPI group, which could explain the lower AUC and duration of MAP < 65. However, as TWA-MAP < 65 mmHg considers the duration and severity of hypotension, this limitation does not affect differences in the primary outcome. Fourth, the measurement of StO2 by NIRS is not free of limitations, such as the interference of myoglobin in the measurements, which may overestimate the value of tissue saturation (34). Finally, our sample size was insufficient to demonstrate any significant difference in the secondary outcomes. Thus, these results should only be considered exploratory. As for strengths of this study, this is the first multicenter approach to evaluate the effect of an HPI-based algorithm in reducing IOH when compared with a group optimized with a widely accepted and recommended GDHT protocol (16).
5. Conclusion
In conclusion, our multicenter study involving patients undergoing elective major abdominal surgery demonstrated that the use of an HPI-based therapeutic protocol significantly reduced the intraoperative time-weighted average of arterial hypotension compared with a control group managed according to a well-established GDHT protocol. Despite the reduction in IOH, there were no differences in intraoperative tissue oxygenation and postoperative AKIRisk values. To better understand the effect of an intraoperative HPI-based therapeutic protocol on postoperative clinical outcomes, further research is warranted with a larger multicenter randomized controlled trial.
Data availability statement
The datasets presented in this article are not readily available because they are involved in ongoing substudies. Requests to access the datasets should be directed to Juan Víctor Lorente, anVhbnZpY3Rvci5sb3JlbnRlQGdtYWlsLmNvbQ==.
Ethics statement
The studies involving human participants were reviewed and approved by Ethics Committee of Hospital Gregorio Marañón (Madrid, Spain). The patients/participants provided their written informed consent to participate in this study.
Author contributions
Conception and study design: JL, JR, IJ, and MM. Acquisition of data: JL, JR, IJ, AB, IM, PF, MF, AM, MA, AV, AE, AC, RV, AQ, GA, AM, PG, AG, and MM. Analysis of data and interpretation of data: JL, JM, IJ, AB, IM, PF, MF, AM, MA, AV, AE, AC, RV, AQ, GA, AM, PG, AG, and MM. Review of the content of paper: JL, JM, IJ, AB, IM, PF, MF, AM, MA, AV, AE, AC, RV, AQ, GA, AM, PG, AG, and MM. All authors contributed to the article and approved the submitted version.
Funding
This study was funded by Edwards Lifesciences (Irvine, USA) (HCP-8200579). The study's design, the collection and interpretation of data, and the writing of the manuscript were carried out independently by the research team without any intervention from the funding body.
Conflict of interest
JVL: Edwards Lifesciences, Fresenius Kabi, Baxter and Vifor Pharma conference fees. Clinical consultant for bioMérieux. Financial support for Edwards Lifesciences research was obtained through the Grant Portal of the company. Economic research support from bioMérieux. JRM: Edwards Lifesciences, bioMérieux, Fresenius Kabi conference fees. IJ: Edwards Lifesciences conference fees. AAG: Edwards Lifesciences and 3M conference fees. MIMG: Clinical consultant for Edwards Lifesciences and Dextera Medical.
The remaining authors declare that the research was conducted in the absence of any commercial or financial relationships that could be construed as a potential conflict of interest.
Publisher's note
All claims expressed in this article are solely those of the authors and do not necessarily represent those of their affiliated organizations, or those of the publisher, the editors and the reviewers. Any product that may be evaluated in this article, or claim that may be made by its manufacturer, is not guaranteed or endorsed by the publisher.
Supplementary material
The Supplementary Material for this article can be found online at: https://www.frontiersin.org/articles/10.3389/fanes.2023.1193886/full#supplementary-material.
References
1. Weiser TG, Haynes AB, Molina G, Lipsitz SR, Esquivel MM, Uribe-Leitz T, et al. Estimate of the global volume of surgery in 2012: an assessment supporting improved health outcomes. Lancet. (2015) 385:S11. doi: 10.1016/s0140-6736(15)60806-6
2. Pearse RM, Moreno RP, Bauer P, Pelosi P, Metnitz P, Spies C, et al. Mortality after surgery in Europe: a 7 day cohort study. Lancet. (2012) 380:1059–65. doi: 10.1016/S0140-6736(12)61148-9
3. Brienza N, Biancofiore G, Cavaliere F, Corcione A, De Gasperi A, De Rosa RC, et al. Clinical guidelines for perioperative hemodynamic management of non cardiac surgical adult patients. Minerva Anestesiol. (2019) 85(12):1315–33. doi: 10.23736/S0375-9393.19.13584-5. 31213042.
4. Chong MA, Wang Y, Berbenetz NM, McConachie I. Does goal-directed haemodynamic and fluid therapy improve peri-operative outcomes? Eur J Anaesthesiol. (2018) 35:469–83. doi: 10.1097/EJA.0000000000000778
5. Giglio MT, Marucci M, Testini M, Brienza N. Goal-directed haemodynamic therapy and gastrointestinal complications in major surgery : a meta-analysis of randomized controlled trials. Br J Anaesth. (2009) 103:637–46. doi: 10.1093/bja/aep279
6. Sessler DI, Bloomstone JA, Aronson S, Berry C, Gan TJ, Kellum JA, et al. Perioperative quality initiative consensus statement on intraoperative blood pressure, risk and outcomes for elective surgery. Br J Anaesth. (2019) 122:563–74. doi: 10.1016/j.bja.2019.01.013
7. Futier E, Lefrant J-Y, Guinot P-G, Godet T, Lorne E, Cuvillon P, et al. Effect of individualized vs standard blood pressure management strategies on postoperative organ dysfunction among high-risk patients undergoing Major surgery A randomized clinical trial. JAMA. (2017) 318(14):1346–57. doi: 10.1001/jama.2017.14172
8. Goldman L, Caldera DL. Risks of general anesthesia and elective operation in the hypertensive patient. Anesthesiology. (1979) 50:285–92. doi: 10.1097/00000542-197904000-00002
9. Bijker JB, Klei WA, Van Kappen TH, Wolfswinkel L, Van Moons KGM, Kalkman CJ. Incidence of intraoperative hypotension as a function of the chosen definition. J Am Soc Anesthesiol. (2007) 107(2):213–20. doi: 10.1097/01.anes.0000270724.40897.8e. 17667564.
10. Mascha EJ, Yang D, Weiss S, Sessler DI. Intraoperative mean arterial pressure variability and 30-day mortality in patients having noncardiac surgery. Anesthesiology. (2015) 123:79–91. doi: 10.1097/ALN.0000000000000686
11. Monk TG, Bronsert MR, Henderson WG, Mangione MP, Sum-Ping STJ, Bentt DR, et al. Association between intraoperative hypotension and hypertension and 30-day postoperative mortality in noncardiac surgery. Anesthesiology. (2015) 123:307–19. doi: 10.1097/ALN.0000000000000756
12. Salmasi V, Maheshwari K, Yang D, Mascha EJ, Singh A, Sessler DI, et al. Relationship between intraoperative hypotension, defined by either reduction from baseline or absolute thresholds, and acute kidney and myocardial injury after noncardiac surgery. Anesthesiology. (2017) 126:47–65. doi: 10.1097/ALN.0000000000001432
13. Davies SJ, Vistisen ST, Jian Z, Hatib F, Scheeren TWL. Ability of an arterial waveform analysis–derived hypotension prediction index to predict future hypotensive events in surgical patients. Anesth Analg. (2020) 130:352–9. doi: 10.1213/ANE.0000000000004121
14. Hatib F, Jian Z, Buddi S, Lee C, Settels J, Sibert K, et al. Machine-learning algorithm to predict hypotension based on high-fidelity arterial pressure waveform analysis. Anesthesiology. (2018) 129:663–74. doi: 10.1097/ALN.0000000000002300
15. Wijnberge M, Geerts BF, Hol L, Lemmers N, Mulder MP, Berge P, et al. Effect of a machine learning–derived early warning system for intraoperative hypotension vs standard care on depth and duration of intraoperative hypotension during elective noncardiac surgery the HYPE randomized clinical trial. JAMA. (2020) 323:1052–60. doi: 10.1001/jama.2020.0592
16. Heming N, Moine P, Coscas R, Annane D. Perioperative fluid management for major elective surgery. Br J Surg. (2020) 107:e56–62. doi: 10.1002/bjs.11457
17. Lorente JV, Jimenez I, Ripollés-Melchor J, Becerra A, Wesselink W, Reguant F, et al. Intraoperative haemodynamic optimisation using the hypotension prediction Index and its impact on tissular perfusion: a protocol for a randomised controlled trial. BMJ Open. (2022) 12:e051728. doi: 10.1136/bmjopen-2021-051728
18. Michels WM, Grootendorst DC, Verduijn M, Elliott EG, Dekker FW, Krediet RT. Performance of the cockcroft-gault, MDRD, and new CKD-EPI formulas in relation to GFR, age, and body size. Clin J Am Soc Nephrol. (2010) 5:1003. doi: 10.2215/CJN.06870909
19. Engelman DT, Crisafi C, Germain M, Greco B, Nathanson BH, Engelman RM, et al. Utilizing urinary biomarkers to reduce acute kidney injury following cardiac surgery. J Thorac Cardiovasc Surg. (2020) 160(5):1235–1246.e2. doi: 10.1016/j.jtcvs.2019.10.034. 31757451.
20. Monge Garcia MI, Jian Z, Settels JJ, Hunley C, Cecconi M, Hatib F, et al. Performance comparison of ventricular and arterial dP/dtmax for assessing left ventricular systolic function during different experimental loading and contractile conditions. Crit Care. (2018) 22(1):325. doi: 10.1186/s13054-018-2260-1
21. Monge García MI, Jian Z, Hatib F, Settels JJ, Cecconi M, Pinsky MR. Dynamic arterial elastance as a ventriculo-arterial coupling index: an experimental animal study. Front Physiol. (2020) 11:284. doi: 10.3389/fphys.2020.00284. 32327999; 153496.
22. Hammer SM, Hueber DM, Townsend DK, Huckaby LM, Alexander AM, Didier KD, et al. Effect of assuming constant tissue scattering on measured tissue oxygenation values during tissue ischemia and vascular reperfusion. J Appl Physiol. (2019) 127:22–30. doi: 10.1152/japplphysiol.01138.2018
23. Jammer I, Wickboldt N, Sander M, Smith A, Schultz MJ, Pelosi P, et al. Standards for definitions and use of outcome measures for clinical effectiveness research in perioperative medicine : european perioperative clinical outcome (EPCO) definitions A statement from the ESA-ESICM joint taskforce on perioperative outcome meas. Eur J Anaesthesiol. (2015) 32(2):88–105. doi: 10.1097/EJA.0000000000000118
24. Nair BG, Horibe M, Newman SF, Wu WY, Peterson GN, Schwid HA. Anesthesia information management system-based near real-time decision support to manage intraoperative hypotension and hypertension. Anesth Analg. (2014) 118(1):206–14. doi: 10.1213/ANE.0000000000000027
25. Yoon JH, Pinsky MR. Predicting adverse hemodynamic events in critically ill patients. Curr Opin Crit Care. (2018) 24(3):196–203. doi: 10.1097/MCC.0000000000000496
26. Schneck E, Schulte D, Habig L, Ruhrmann S, Edinger F, Markmann M, et al. Hypotension prediction Index based protocolized haemodynamic management reduces the incidence and duration of intraoperative hypotension in primary total hip arthroplasty: a single centre feasibility randomised blinded prospective interventional trial. J Clin Monit Comput. (2020) 34(6):1149–58. doi: 10.1007/s10877-019-00433-6. Epub 2019 Nov 29. 31784852.31784852
27. Maheshwari K, Pu X, Rivas E, Saugel B, Turan A, Schmidt MT, et al. Association between intraoperative mean arterial pressure and postoperative complications is independent of cardiac index in patients undergoing noncardiac surgery. Br J Anaesth. (2021) 127:e102–104. doi: 10.1016/j.bja.2021.06.024
28. Maheshwari K, Shimada T, Yang D, Khanna S, Cywinski JB, Irefin SA, et al. Hypotension prediction Index for prevention of hypotension during moderate- to high-risk noncardiac surgery: a pilot randomized trial. Anesthesiology. (2020) 133(6):1214–22. doi: 10.1097/ALN.0000000000003557. 32960954.32960954
29. Ripollés-Melchor J, Ramírez-Rodríguez JM, Casans-Francés R, Aldecoa C, Abad-Motos A, Logroño-Egea M, et al. Association between use of enhanced recovery after surgery protocol and postoperative complications in colorectal surgery: the postoperative outcomes within enhanced recovery after surgery protocol (POWER) study. JAMA Surg. (2019) 154:725–36. doi: 10.1001/jamasurg.2019.0995
30. Murabito P, Astuto M, Sanfilippo F, La Via L, Vasile F, Basile F, et al. Proactive management of intraoperative hypotension reduces biomarkers of organ injury and oxidative stress during elective non-cardiac surgery: a pilot randomized controlled trial. J Clin Med. (2022) 11(2):392. doi: 10.3390/jcm11020392. 35054083; 8777609.35054083
31. Barstow TJ. Understanding near infrared spectroscopy and its application to skeletal muscle research. J Appl Physiol. (2019) 126:1360–76. doi: 10.1152/japplphysiol.00166.2018
32. Ripollés-Melchor J, Abad-Motos A, Cecconi M, Pearse R, Jaber S, Slim K, et al. Association between use of enhanced recovery after surgery protocols and postoperative complications in colorectal surgery in Europe: the EuroPOWER international observational study. J Clin Anesth. (2022) 80:110752. doi: 10.1016/j.jclinane.2022.110752
33. Jor O, Maca J, Koutna J, Gemrotova M, Vymazal T, Litschmannova M, et al. Hypotension after induction of general anesthesia: occurrence, risk factors, and therapy. A prospective multicentre observational study. J Anesth. (2018) 32:673–80. doi: 10.1007/s00540-018-2532-6
Keywords: intraoperative hypotension, postoperative complications, hypotension prediction index, goal-directed hemodynamic therapy, tissue oxygenation
Citation: Lorente JV, Ripollés-Melchor J, Jiménez I, Becerra AI, Mojarro I, Fernández-Valdes-Bango P, Fuentes MA, Moreno A, Agudelo ME, Villar-Pellit de la Vega A, Ruiz-Escobar A, Cortés A, Venturoli R, Quintero A, Acedo GM, Abad-Motos A, Gómez P, Abad-Gurumeta A and Monge-García MI (2023) Intraoperative hemodynamic optimization using the hypotension prediction index vs. goal-directed hemodynamic therapy during elective major abdominal surgery: the Predict-H multicenter randomized controlled trial. Front. Anesthesiol. 2:1193886. doi: 10.3389/fanes.2023.1193886
Received: 25 March 2023; Accepted: 8 May 2023;
Published: 23 May 2023.
Edited by:
Matthieu Jabaudon, Université Clermont Auvergne, FranceReviewed by:
Vedat Eljezi, Centre Hospitalier Universitaire de Clermont-Ferrand, FranceMarc-Olivier Fischer, Clinique Saint Augustin, France
© 2023 Lorente, Ripollés-Melchor, Jiménez, Becerra, Mojarro, Fernández-Valdes-Bango, Fuentes, Moreno, Agudelo, Villar-Pellit de la Vega, Ruiz-Escobar, Cortés, Venturoli, Quintero, Acedo, Abad-Motos, Gómez, Abad-Gurumeta and Monge García. This is an open-access article distributed under the terms of the Creative Commons Attribution License (CC BY). The use, distribution or reproduction in other forums is permitted, provided the original author(s) and the copyright owner(s) are credited and that the original publication in this journal is cited, in accordance with accepted academic practice. No use, distribution or reproduction is permitted which does not comply with these terms.
*Correspondence: Juan V. Lorente anVhbnZpY3Rvci5sb3JlbnRlQGdtYWlsLmNvbQ==