- 1Algae Biotechnology Laboratory, School of Agriculture and Food Sciences, The University of Queensland, Brisbane, QLD, Australia
- 2Plant Cell Biotechnology Department, CSIR-Central Food Technological Research Institute, Mysore, India
- 3Algae Laboratory, Botany Department, Faculty of Science, Mansoura University, Mansoura, Egypt
For efficient downstream processing, harvesting remains as one of the challenges in producing Nannochloropsis biomass, a microalga with high-value omega-3 oils. Flocculation is an effective, low-energy, low-cost method to harvest microalgae. Chitosan has been shown to be an effective food-grade flocculant; however, commercial chitosan is sourced from crustaceans, which has disadvantages including concerns over heavy-metal contamination. Thus, this study tests the flocculation potential of mushroom chitosan. Our results indicate a 13% yield of chitosan from mushroom. The identity of the prepared chitosan was confirmed by Fourier-transform infrared (FTIR) spectroscopy. Furthermore, results show that mushroom chitosan can be an alternative flocculant with >95% flocculation efficiency when tested in 100-mL jar and 200-L vertical column photobioreactor. Applications in a 2000-L raceway pond demonstrated that thorough mixing of mushroom chitosan with the algal culture is required to achieve efficient flocculation. With proper mixing, mushroom chitosan can be used to produce food-grade Nannochloropsis biomass suitable for the production of vegan omega-3 oils as a fish oil alternative.
Introduction
Microalgae are photosynthetic microorganisms that grow in various environments. In recent years, research on microalgae has shifted from their use as biofuel to the production of nutraceuticals such as omega-3 fatty acids, carotenoids, and protein. Nannochloropsis sp. is a marine microalga, which contains high amounts of omega-3 fatty acids in the form of eicosapentaenoic acid (EPA) that has been proposed as a suitable vegan alternative for fish oil (Chua and Schenk, 2017). Its protein content can reach 36% of the biomass (Schulze et al., 2016) and even 46% according to our own data. Because of these abundant high-value products, Nannochloropsis sp. has gained interest from investors for large-scale cultivation.
In large-scale microalgae cultivation systems, one of the main challenges is harvesting the cells (Mathimani and Mallick, 2018). Conventional methods of harvesting include filtration and centrifugation. However, because of the small cell size (2–5 μm) of Nannochloropsis, these conventional harvesting methods require expensive equipment, which are also energy-intensive. Hence, harvesting can cost up to 30% of the total capital investment (Milledge and Heaven, 2013). An easy, simple, and low-cost method of harvesting is by flocculation (Vandamme et al., 2013). The most common and cheapest flocculant used is alum (Vandamme et al., 2013). However, this method contaminates the final harvested biomass with high amounts of aluminum, which makes the product not suitable for human and animal consumption. Another well-studied flocculant is chitosan (Vandamme et al., 2013; Chua et al., 2019). Chitosan is a linear polysaccharide derived from the deacetylation of the abundant natural polymer chitin, which is mainly composed of N-acetyl-D-glucosamine monomer units (Dimzon and Knepper, 2015). Numerous research papers have already proven the effectivity of chitosan to flocculate microalgae cells (Şirin et al., 2012; Xu et al., 2013). We have previously shown the importance of the pH of the chitosan–microalgae mixture to have high flocculation efficiencies (Chua et al., 2019). All chitosan samples tested were sourced from crustacean shells. However, crustacean-sourced chitosan has several disadvantages including heavy metal contamination (Ghormade et al., 2017). Previous studies have shown that chitosan can also be extracted from mushrooms (Yen and Mau, 2006; Erdogan et al., 2017) or even mushroom wastes (Wu et al., 2004). Thus, in this study, we produced and tested the effectiveness of mushroom chitosan for flocculating Nannochloropsis cells. The results were further verified in large-scale cultures, i.e., a 200-L vertical column photobioreactor culture and a 2000-L raceway pond culture.
Materials and Methods
Microalgae Culture
Nannochloropsis oceanica BR2 (Genbank accession JQ423160) was obtained from the microalgae culture collection of the University of Queensland Algae Biotechnology culture collection (Lim et al., 2012; Brown et al., 2019). The species was initially grown in a 250-mL flask using 20 g/L Ocean Nature Sea Salt (Aquasonic Pty. Ltd., NSW, Australia) enriched with f/2 medium (per L water): 75 mg NaNO3, 5 mg NaH2PO4⋅H2O, 30 mg Na2SiO3⋅9H2O, 3.15 mg FeCl3⋅6H2O, 4.36 mg Na2EDTA⋅2H2O, 9.8 μg CuSO4⋅5H2O, 6.3 μg Na2MoO4⋅2H2O, 22 μg ZnSO4⋅7H2O, 10 μg CoCl2⋅6H2O, 180 μg MnCl2⋅4H2O, 200 mg thiamine HCl, 1 μg biotin, and 1 μg cyanocobalamin (Guillard, 1975; Ma et al., 2018; Chua et al., 2019). The culture was continuously illuminated with fluorescent light (70 μmol photons m–2s–1) and aerated with filtered (through 0.2-μm pore size membrane filter) air. The culture was then gradually scaled up to the larger volumes (2 and 20 L) until it was transferred to outdoor cultures with volumes of 200 and 2000 L. The 200-L culture was grown in a vertical column photobioreactor with a diameter of 36 cm and a height of 2 m. The culture was maintained at pH 8.2 for optimum growth by bubbling CO2 (food-grade, 99.99% pure) at 1 vvm. Apart from maintaining the pH, CO2 was also the sole source of carbon for the culture. On the other hand, the 2000-L culture was grown in a 10 m2 raceway pond that had 1 m wide channels and a depth of 10 cm. The pond was mixed using an air-lift system and pH was controlled and maintained at 8 by automatic additions of CO2.
Preparation of Chitosan From Mushroom
Chitosan was prepared from 50 g of Shiitake mushroom powder (Austral Herbs, NSW, Australia) following the method by Mohammed et al. (2013) with some modifications. Briefly, the mushroom powder was mixed with 5% NaOH solution in 1:8 ratio of powder to NaOH solution. The mixture was stirred at 120 r/min for 2 h at 60°C. Then, the sample was washed three times with distilled water. The crude chitin was deacetylated by refluxing in 50% (w/v) NaOH for 2 h at 100°C. The resulting liquor was then centrifuged, and the pellet was continuously washed until the pH was neutral. Finally, the pellet was lyophilized to obtain the crude chitosan. The entire procedure was carried out with 500 g mushroom powder for testing in the large-scale microalgal cultures.
Characterization of the Prepared Mushroom Chitosan
The crude mushroom chitosan was characterized using a Fourier-transform infrared (FTIR) spectrophotometer (Thermo Scientific Nicolet 700) fitted with an attenuated total reflectance accessory and a diamond crystal internal reflection element. The resulting spectrum was compared to the commercial chitosan (Sigma).
Elemental Analyses of Chitosan
Elemental analyses for heavy metals in chitosan samples were performed in duplicates as previously described (Aslam et al., 2019). Included in the analyses were two crustacean chitosan samples (Sample 1: Biomedical Chitosan, Australia; Sample 2: Qingdao Yunzhou Biochemistry Co., Ltd., China) and the mushroom-derived chitosan from the present study (Sample 3).
Testing the Prepared Mushroom Chitosan for Nannochloropsis Flocculation
A similar method was used to test for the flocculation efficiency of the prepared mushroom chitosan as described in Chua et al. (2019). The optimized parameters (chitosan concentration of 25 ppm, culture optical density of 2, adjustment of pH to 6 after chitosan addition, and increase of final pH to 10 after mixing the chitosan) were used for the test.
The prepared mushroom chitosan was compared to commercial chitosan and mushroom powder. All samples were suspended in 1% acetic acid. Samples were collected at mid-height at 5, 15, and 30 min and the absorbance of the samples was measured at 440 nm to evaluate the flocculation efficiency. The flocculation efficiency was calculated using Eq. 1:
where OD0 and ODt are the OD values of the cultures before and after the flocculation test, respectively. The culture absorbance was measured at 440 nm since this is the absorption maximum of chlorophyll a which is abundantly present in Nannochloropsis.
Further, a pilot scale testing of mushroom chitosan for flocculation was performed on Nannochloropsis cultivated in a 200 L vertical column photo-bioreactor and 2000 L open raceway pond. Both these cultures were maintained at pH 8 through CO2 supplementation. The final pH of the cultures was set by adding KOH. The same method was used to calculate the flocculation efficiency.
Fatty Acid Quantification and Profiling
Fatty acid methyl esters from chitosan-harvested N. oceanica BR2 biomass were quantified by gas chromatography-mass spectrometry (GC-MS) as previously described (Ma et al., 2018). The analysis was done in triplicates.
Statistical Analysis
The lab scale flocculation test was performed in triplicates. Tukey’s multiple comparisons test was used to test the significance among groups. Comparisons with p-values < 0.05 considered as statistically significant.
Results
Preparation of Mushroom Chitosan
Mushroom chitosan was prepared from mushroom chitin via alkaline treatment. From the mushroom powder used, 17.22 g of crude extract was obtained after the first alkaline treatment. The first alkaline treatment was necessary to remove the protein contaminants (Wu et al., 2004; Mohammed et al., 2013; Erdogan et al., 2017). The amount of crude extract was further reduced after the second alkaline treatment to 6.16 g, which equates to a 12.32% yield. For the 500 g-mushroom powder, 157.02 g of crude chitin were obtained resulting in a 31.4% yield. After the second alkaline treatment, 68 g of crude chitosan were obtained for a final yield of 13.60%. Figure 1A shows the appearance of the mushroom chitosan after it has been lyophilized. The final yields obtained for both the small scale and large-scale extractions were comparable to those in literature (Yen and Mau, 2006; Di Mario et al., 2008).
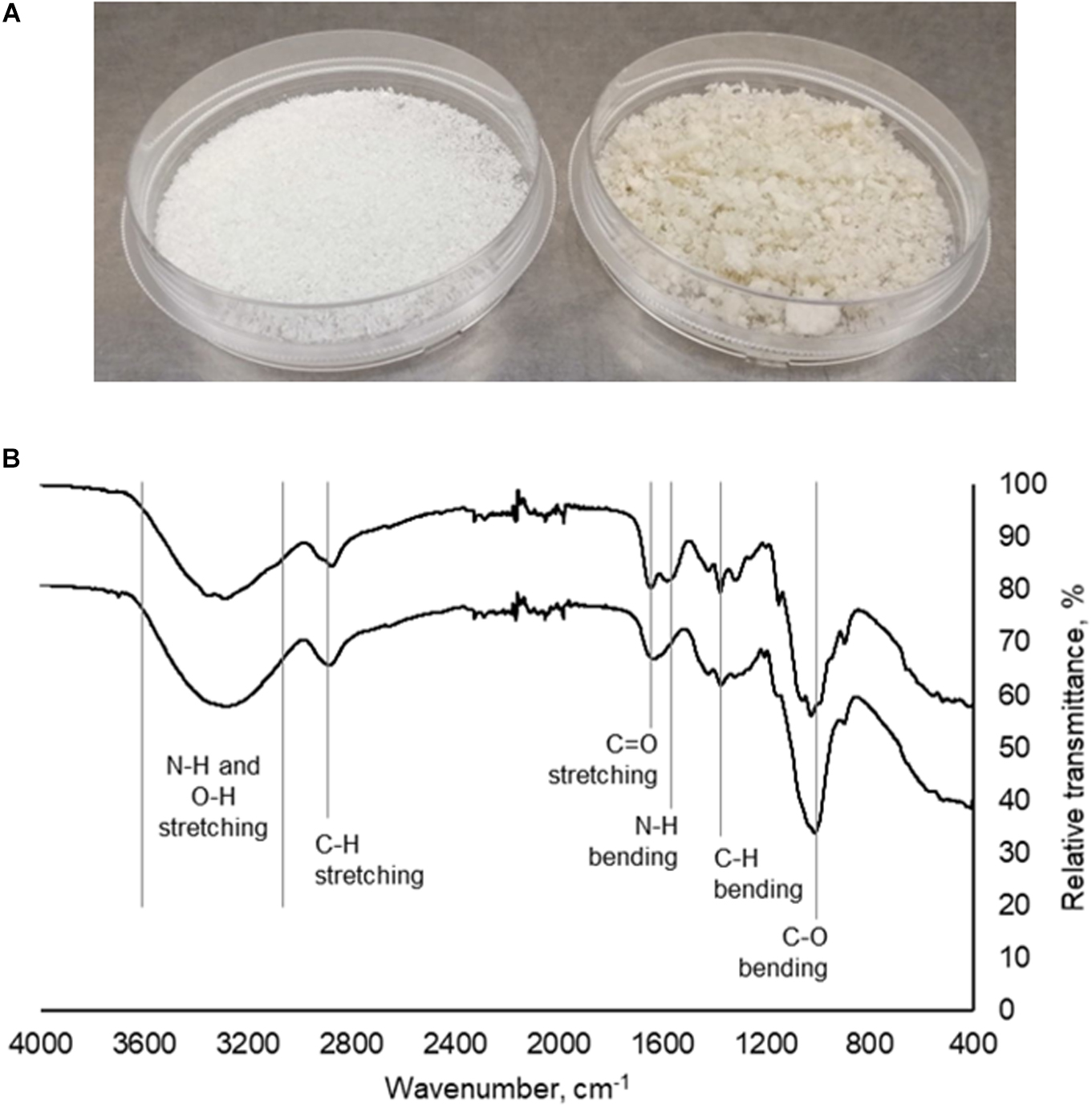
Figure 1. (A) Commercial chitosan (left) and crude mushroom chitosan (right). (B) Infrared spectra of commercial chitosan (top) and mushroom chitosan (bottom).
Infrared spectroscopy (Figure 1B) results indicated that chitosan was successfully prepared from the extracted mushroom chitin. Peaks at 3400–3200 cm–1 correspond to the N–H and O–H stretching. The peaks around the 1660 cm–1 region correspond to the C = O stretching from the amide group while the peak at 1600 cm–1 is the amine peak (Dimzon and Knepper, 2015). Finally, the peaks at 1024, 1373, and 2870 cm–1 correspond to the C–O bending, C–H bending, and C–H stretching from the polymer backbone, respectively.
Flocculation Efficiency of the Prepared Crude Mushroom Chitosan
The crude mushroom chitosan was tested on N. oceanica BR2 using previously optimized conditions which were: culture OD of 2, 25 ppm chitosan, adjusting the pH to 6 after chitosan addition, and increasing the final pH to 10 after mixing the chitosan into the culture (Chua et al., 2019). Similar to the results in Chua et al. (2019), no flocculation was observed without increasing the final pH to 10. Results indicated that that the crude mushroom chitosan can induce flocculation similar to the commercial chitosan (p > 0.05) with flocculation efficiency values > 94% after 5 min (Figure 2). On the other hand, the mushroom powder only yielded an average flocculation efficiency value of 66% even after 30 min.
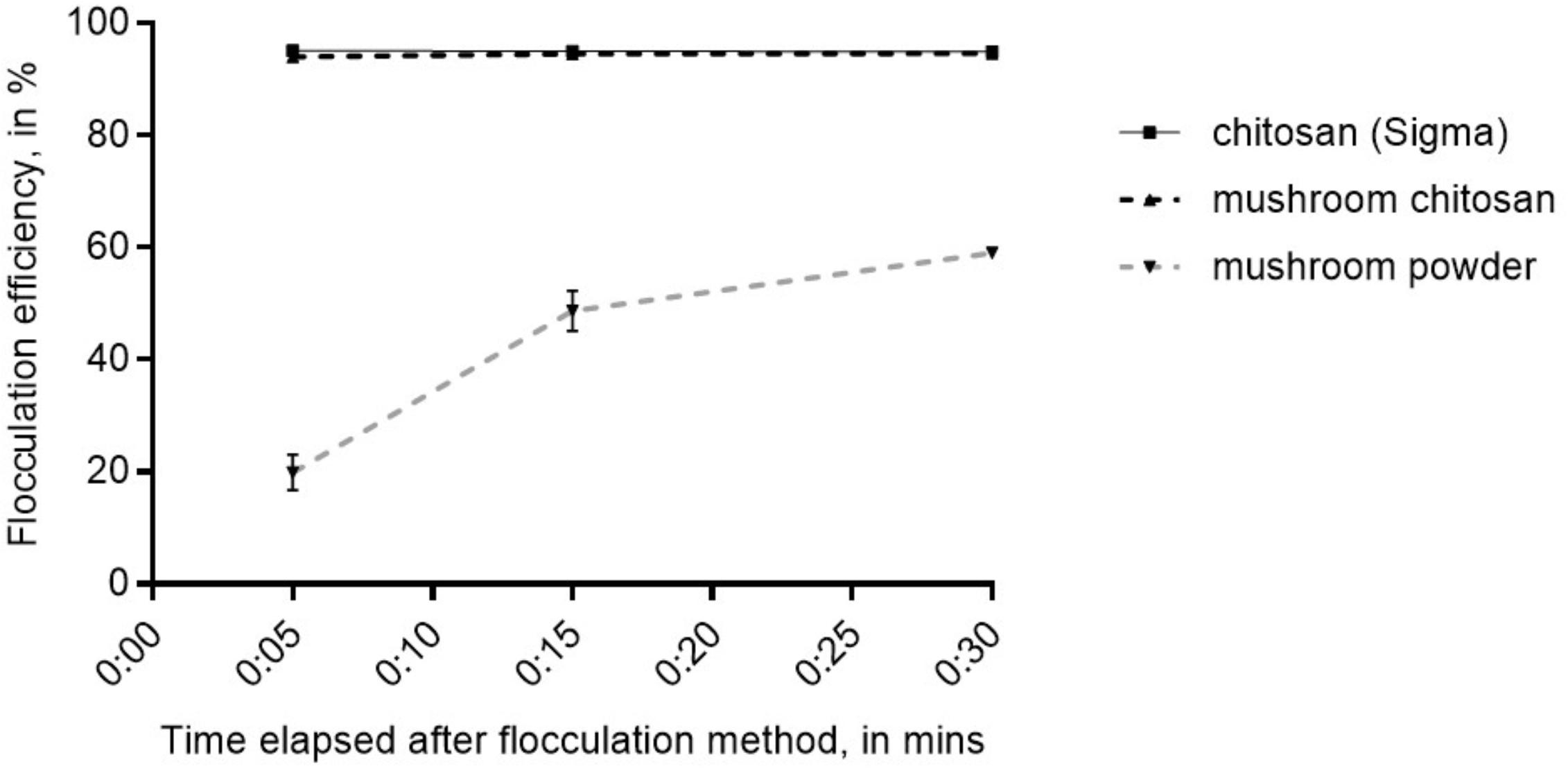
Figure 2. Flocculation efficiency for mushroom chitosan compared to the commercial chitosan from crustacean. Shown are mean values ± SE of three replicates.
Testing of Mushroom Chitosan in a Pilot-Scale Harvesting
The effectiveness of mushroom chitosan to induce flocculation of N. oceanica BR2 was tested at pilot scale using a 200-L vertical column photo-bioreactor and a 2000-L open raceway pond. Figures 3A,B show the 200-L column photo-bioreactor before and after the flocculation procedure, respectively. In this case, a flocculation efficiency of 98.3% was achieved. As for the 2000-L raceway pond, it had an initial OD of 2.9. So, a 50-mL sample was obtained before testing. Flocculation was observed after adding chitosan into the 50-mL sample as shown in Figure 3D indicating that 25 ppm of mushroom chitosan is still effective. Mixing was performed by using an air-lift system, and the chitosan was poured in and mixed for 10 min. Figures 3F,G show some of the flocs that formed after the chitosan was added and these flocs were not present before chitosan addition as shown in Figure 3E. The entire procedure yielded 64% flocculation efficiency after 1 h of settling. After 24 h, samples were collected at different points of the pond, and the average OD was 0.787, yielding 73% flocculation efficiency. Figure 3C summarizes the flocculation results of the 200- and 2000-L culture.
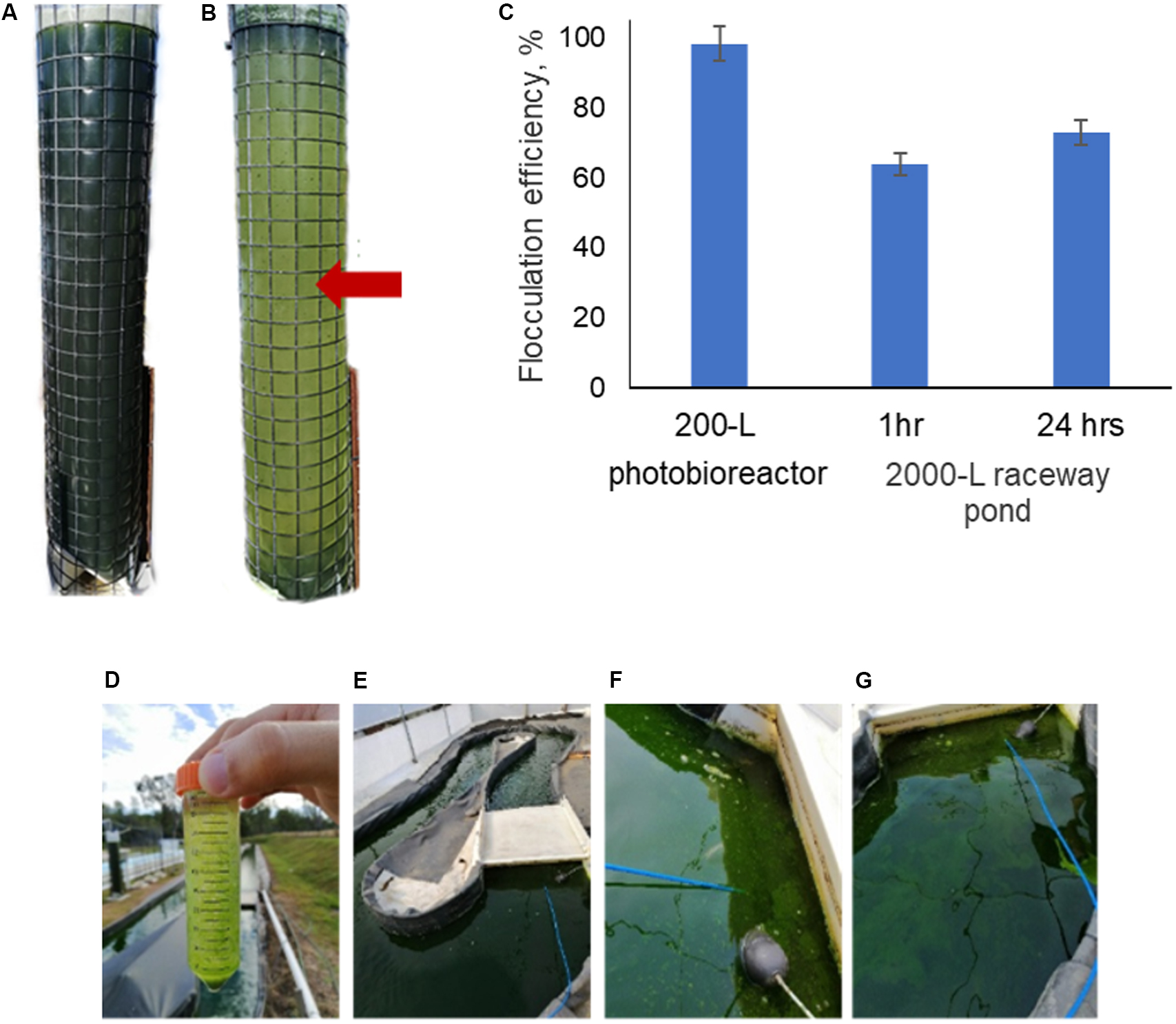
Figure 3. Mushroom chitosan-mediated flocculation of N. oceanica in a 200-L tower culture: (A) before flocculation and (B) after flocculation. The resulting flocculation efficiencies are presented in (C). The red arrow indicates the sampling point; and in a 2000-L raceway pond: (D) testing on a 50-mL sample, (E) before flocculation, (F,G) after flocculation.
To determine which type of chitosan (mushroom- or crustaceae-derived) is a safer option for human consumption, elemental analyses were performed using two samples of crustacean and one sample of mushroom chitosan from the present study. These showed that heavy metals varied greatly for the two crustacean-derived samples, with chromium and nickel levels as high as 47.44 and 27.21 mg/kg, respectively, while mushroom-derived chitosan did not contain any concerning heavy metal contamination (Supplementary Table S1).
Fatty Acid Profiling of Mushroom Chitosan-Harvested N. oceanica BR2 Biomass
The mushroom chitosan-harvested biomass has EPA levels up to 41.3(±0.3)% of the total fatty acid content. Other fatty acids detected were palmitic acid (C16:0, 15.0 ± 0.6%), palmitoleic acid (C16:1, 34.4 ± 0.8%), oleic acid (C18:1, 2.8 ± 0.3%), linoleic acid (C18:2, 1.8 ± 0.2%), and arachidonic acid (C20:4n-6, 4.7 ± 0.3%). Supplementary Table S2 lists the fatty acid profile of the mushroom-harvested N. oceanica BR2 biomass together with profiles from other studies for comparison.
Discussion
Chitin is popularly known to be extracted from the shells of crustaceans such as crabs and shrimps. Earlier estimates have shown that more than 80,000 tons of chitin is obtained from marine by-products (Ghormade et al., 2017). However, there are some disadvantages to marine-derived chitosan including seasonal variation and possible heavy metal contamination (Ghormade et al., 2017; Abo Elsoud and El Kady, 2019). This was also confirmed in the present study (Supplementary Table S1). In addition, fungal chitosan is suitable for vegans and is free from allergenic shrimp protein, which can be included in the final harvested biomass (Arcidiacono and Kaplan, 1992; Dhillon et al., 2013).
To theoretically estimate the comparative production expenses of chitosan from mushroom and crustaceans, the literature outlines that due to the inconsistent structure of chitin and chitosan from crustaceans, fungal (mushroom in this case) may represent a better alternative (Di Mario et al., 2008; Hassainia et al., 2018; Jones et al., 2020). Furthermore, fluctuations in seasonal supply of various animal sources and challenges in raw material standardization cause high variability in terms of deacetylation degree and molecular mass. These results may interfere in final flocculation efficiency of the chitosan. Unlike crustacean chitin, fungal chitin has more consistent physical and chemical properties, is not limited by seasonal and regional variation, and does not require the aggressive acid treatment that crustacean chitin needs for purification and demineralization to remove calcium carbonate and other minerals (Di Mario et al., 2008; Hassainia et al., 2018; Jones et al., 2020). In addition, from an environmental economics and sustainability point of view, crustacean chitosan production is likely to generate more waste than fungal chitosan. In the traditional process of chitin extraction from crustaceans, calcium and proteins are removed by HCl and NaOH, respectively. The remaining material is usually bleached with KMnO4 or H2O2 and deacetylation is performed with hot concentrated alkaline or acidic solution. These harsh treatments can result in considerable amounts of wastes and deleterious trace contaminants (Bierhalz et al., 2016). Therefore, chitosan produced from mushroom waste is safer, more environmentally friendly, more reliable in its supply, and suitable for vegetarians.
A difference in color between the crude mushroom chitosan and the commercial chitosan was observed, which could be because the mushroom chitosan was not purified. The yellowish color was also obtained by Yen and Mau (2006). Further purification may be conducted by refluxing the crude powder in HCl or acetic acid (Wu et al., 2004; Darwesh et al., 2018). Decolorization may also be performed to improve the color (Yen and Mau, 2006; Mohammed et al., 2013). However, these processes will increase the cost of producing the chitosan flocculant. Nevertheless, even without the purification step, IR spectroscopy revealed the successful preparation of mushroom chitosan. The same characteristic peaks were observed in the IR spectrum of the mushroom chitosan when compared to the commercial chitosan.
Chitosan has been demonstrated as a good alternative bio-flocculant (Xu et al., 2013; Chua et al., 2019). Our flocculation test results confirm that mushroom chitosan was successfully prepared and has similar flocculation properties as the commercial chitosan. Mushroom powder did not flocculate the cells, which indicates that chitosan is the active ingredient and can only be obtained after deacetylation. Recently, Pugazhendhi et al. (2019) discussed the chemical mechanism of cationic polymers for microalgae flocculation. Because chitosan is cationic at acidic pH; thus, the lowering of the pH was necessary. In another study by Blockx et al. (2018), they demonstrated that high pH is necessary to flocculate microalgae in seawater medium. Thus, it was necessary to increase the pH after chitosan addition. This demonstrates that mushroom chitosan can indeed be used to harvest Nannochloropsis biomass that would then be suitable for vegetarians or even vegans. The fatty acid profile, which was not different from those in literature, further supports that the mushroom chitosan-harvested biomass can indeed serve as an alternative source of fish oil.
Previous studies (Table 1) have tested different flocculants to harvest Nannochloropsis spp. In the current study, mushroom chitosan has resulted in almost similar flocculation efficiency of >94% as compared to the previously reported chemical-based flocculants such as Al2(SO4)3 and FeCl3, but at much lower concentrations. Therefore, in this case, chitosan has advantage over chemical flocculants, which are not recommended for food-grade applications of harvested biomass. On the other hand, mung bean protein concentrate can be avoided since it can drive the food debate over its use for harvesting purpose. Tanfloc showed a comparable flocculation efficiency and at an even lower concentration compared to the mushroom chitosan concentration used in this study. However, it is not clear if Tanfloc can be used in food production as it is currently sold for water and wastewater treatment (TANAC, S.A.1). To our knowledge, this is the first study to report the use of mushroom chitosan for microalgae flocculation.
Higher flocculation efficiencies were observed in the 200-L vertical column photobioreactor compared to the air-lift raceway pond. The low flocculation efficiency was likely caused by the suboptimal mixing of the chitosan into the pond culture, as the mixing was significantly more efficient in the 50-mL and 200-L cultures (>95 and 98.3% yield, respectively). The raceway pond was slowly mixed using an air-lift system achieved with microbubbles, which may have also affected the performance since the bubbles disrupted the large flocs. Even after 24 h, the flocculation efficiency did not reach >90%. This result clearly indicated that the cells have not interacted properly with the chitosan. Bleeke et al. (2015) and Pugazhendhi et al. (2019) discussed the importance of mixing speed, intensity, and time. Mixing using a paddle-wheel system may provide a better flocculation performance as the mechanism less disruptive to the large flocs as they pass through. Koley et al. (2017) have demonstrated the effectivity of chitosan with flocculation efficiencies of ∼90% to flocculate Scenedesmus obliquus and Chlorella vulgaris cultured in raceway ponds. However, the cultures had to be pumped into 1000-L tanks, which was easier to mix with a large motor-driven stirrer. Further optimization on the mixing of the chitosan into the pond culture would be necessary along with economic feasibility studies to improve the attractiveness of chitosan for use in microalgae harvesting.
Conclusion
Mushroom chitosan was prepared by extracting and deacetylating chitin from mushroom powder and was verified using FTIR. Results showed that the prepared mushroom chitosan had similar flocculation efficiency as commercial crustacean-derived chitosan. Furthermore, chitosan can be sustainably prepared utilizing the wastes from mushroom industries and using it for harvesting promotes the chemical-free harvesting protocol for microalgae for food and/or feed applications. While chitosan was also found suitable for harvesting of large culture volumes, the requirement for efficient mixing should be considered. The availability of mushroom chitosan harvested Nannochloropsis offer an affordable and sustainable fish oil replacement product suitable for vegans.
Data Availability Statement
The original contributions presented in the study are included in the article/Supplementary Material. Further inquiries can be directed to the corresponding author.
Author Contributions
EC conducted the experiments, analyzed the results, and wrote the original draft. AS assisted in the large-scale experiments. EE assisted in preparing the mushroom chitosan. ST-H prepared the resources for the large-scale experiments. PS supervised the entire project and acquired the necessary funding. All authors reviewed the manuscript.
Funding
This work was supported by a Cooperative Research Centre Project (CRC-P50438) jointly funded by the Australian Government, Qponics Ltd., Nutrition Care Pharmaceuticals, and The University of Queensland, and an Advance Queensland Biofutures Commercialization Program (AQBCP00516-17RD1) jointly funded by the Queensland Government, Woods Grain Pty Ltd., and The University of Queensland.
Conflict of Interest
The authors declare that the research was conducted in the absence of any commercial or financial relationships that could be construed as a potential conflict of interest.
Acknowledgments
The authors would like to thank Dr. Idriss Blakey for assisting in the FTIR analysis of the chitosan samples and the Australian Endeavour Leadership Program for providing a scholarship for EC.
Supplementary Material
The Supplementary Material for this article can be found online at: https://www.frontiersin.org/articles/10.3389/fbioe.2020.00771/full#supplementary-material
Footnotes
References
Abo Elsoud, M. M., and El Kady, E. M. (2019). Current trends in fungal biosynthesis of chitin and chitosan. Bull. Natl. Res. Cent. 43:59. doi: 10.1186/s42269-019-0105-y
Arcidiacono, S., and Kaplan, D. L. (1992). Molecular weight distribution of chitosan isolated from Mucor rouxii under different culture and processing conditions. Biotechnol. Bioeng. 39, 281–286. doi: 10.1002/bit.260390305
Aslam, A., Thomas-Hall, S. R., Mughal, T., Zaman, Q. U., Ehsan, N., Javied, S., et al. (2019). Heavy metal bioremediation of coal-fired flue gas using microalgae under different CO2 concentrations. J. Environ. Manag. 241, 243–250. doi: 10.1016/j.jenvman.2019.03.118
Bierhalz, A. C. K., Westin, C. B., and Moraes, A. M. (2016). Comparison of the properties of membranes produced with alginate and chitosan from mushroom and from shrimp. Int. J. Biol. Macromol. 91, 496–504. doi: 10.1016/j.ijbiomac.2016.05.095
Bleeke, F., Milas, M., Winckelmann, D., and Klöck, G. (2015). Optimization of freshwater microalgal biomass harvest using polymeric flocculants. Int. Aquat. Res. 7, 235–244. doi: 10.1007/s40071-015-0108-8
Blockx, J., Verfaillie, A., Thielemans, W., and Muylaert, K. (2018). Unravelling the mechanism of chitosan-driven flocculation of microalgae in seawater as a function of pH. ACS Sustainab. Chem. Eng. 6, 11273–11279. doi: 10.1021/acssuschemeng.7b04802
Brown, R. B., Wass, T. J., Thomas-Hall, S. R., and Schenk, P. M. (2019). Chromosome-scale genome assembly of two Australian Nannochloropsis oceanica isolates exhibiting superior lipid characteristics. Microbiol. Resour. Announc. 8:48. doi: 10.1128/MRA.01288-19
Chua, E. T., Eltanahy, E., Jung, H., Uy, M., Thomas-Hall, S. R., and Schenk, P. M. (2019). Efficient harvesting of Nannochloropsis microalgae via optimized chitosan-mediated flocculation. Glob. Challeng. 3:1800038. doi: 10.1002/gch2.201800038
Chua, E. T., and Schenk, P. M. (2017). A biorefinery for Nannochloropsis: induction, harvesting, and extraction of EPA-rich oil and high-value protein. Bioresour. Technol. 244, 1416–1424. doi: 10.1016/j.biortech.2017.05.124
Darwesh, O. M., Sultan, Y. Y., Seif, M. M., and Marrez, D. A. (2018). Bio-evaluation of crustacean and fungal nano-chitosan for applying as food ingredient. Toxicol. Rep. 5, 348–356. doi: 10.1016/j.toxrep.2018.03.002
Dhillon, G. S., Kaur, S., Brar, S. K., and Verma, M. (2013). Green synthesis approach: extraction of chitosan from fungus mycelia. Crit. Rev. Biotechnol. 33, 379–403. doi: 10.3109/07388551.2012.717217
Di Mario, F., Rapanà, P., Tomati, U., and Galli, E. (2008). Chitin and chitosan from Basidiomycetes. Int. J. Biol. Macromol. 43, 8–12. doi: 10.1016/j.ijbiomac.2007.10.005
Dimzon, I. K. D., and Knepper, T. P. (2015). Degree of deacetylation of chitosan by infrared spectroscopy and partial least squares. Int. J. Biol. Macromol. 72, 939–945. doi: 10.1016/j.ijbiomac.2014.09.050
Erdogan, S., Kaya, M., and Akata, I. (2017). Chitin extraction and chitosan production from cell wall of two mushroom species (Lactarius vellereus and Phyllophora ribis). AIP Conf. Proc. 1809:020012. doi: 10.1063/1.4975427
Fuad, N., Omar, R., Kamarudin, S., Harun, R., Idris, A., and Wan Azlina, W. A. K. G. (2018). Effective use of tannin based natural biopolymer, AFlok-BP1 to harvest marine microalgae Nannochloropsis sp. J. Environ. Chem. Eng. 6, 4318–4328. doi: 10.1016/j.jece.2018.06.041
Ghormade, V., Pathan, E. K., and Deshpande, M. V. (2017). Can fungi compete with marine sources for chitosan production? Int. J. Biol. Macromol. 104, 1415–1421. doi: 10.1016/j.ijbiomac.2017.01.112
Guillard, R. R. L. (1975). Culture of Phytoplankton For Feeding Marine Invertebrates, In Culture Of Marine Invertebrate Animals. New York, NY: Plenum Press.
Hassainia, A., Satha, H., and Boufi, S. (2018). Chitin from agaricus bisporus: extraction and characterization. Int. J. Biol. Macromol. 117, 1334–1342. doi: 10.1016/j.ijniomax.2017.11.172
Hulatt, C. J., Wijffels, R. H., Bolla, S., and Kiron, V. (2017). Production of fatty acids and protein by Nannochloropsis in flat-plate photobioreactors. PLoS One 12:e0170440. doi: 10.1371/journal.pone.0170440
Jones, M., Kujundzic, M., John, S., and Bismarck, A. (2020). Crab vs. mushroom: a review of crustacean and fungal chitin in wound treatment. Mar. Drugs 18:64. doi: 10.3390/md18010064
Kandasamy, G., and Shaleh, S. R. M. (2017). Harvesting of the microalga Nannochloropsis sp. by bioflocculation with mung bean protein extract. Appl. Biochem. Biotech. 182, 587–597. doi: 10.1007/s12010-016-2346-7
Koley, S., Prasad, S., Bagchi, S. K., and Mallick, N. (2017). Development of a harvesting technique for large-scale microalgal harvesting for biodiesel production. RSC Adv. 7, 7227–7237. doi: 10.1039/C6RA27286J
Lim, D. K. Y., Garg, S., Timmins, M., Zhang, E. S. B., Thomas-Hall, S. R., Schuhmann, H., et al. (2012). Isolation and evaluation of oil-producing microalgae from subtropical coastal and brackish waters. PLoS One 7:e40751. doi: 10.1371/journal.pone.0040751
Ma, R., Thomas-Hall, S. R., Chua, E. T., Eltanahy, E., Netzel, M. E., Netzel, G., et al. (2018). LED power efficiency of biomass, fatty acid, and carotenoid production in Nannochloropsis microalgae. Bioresour. Technol. 252, 118–126. doi: 10.1016/j.biortech.2017.12.096
Mathimani, T., and Mallick, N. (2018). A comprehensive review on harvesting of microalgae for biodiesel - key challenges and future directions. Renew. Sustain. Energy Rev 91, 1103–1120. doi: 10.1016/j.rser.2018.04.083
Milledge, J. J., and Heaven, S. (2013). A review of the harvesting of micro-algae for biofuel production. Rev. Environ. Sci. Biotechnol. 12, 165–178. doi: 10.1007/s11157-012-9301-z
Mohammed, M. H., Williams, P. A., and Tverezovskaya, O. (2013). Extraction of chitin from prawn shells and conversion to low molecular mass chitosan. Food Hydrocoll. 31, 166–171. doi: 10.1016/j.foodhyd.2012.10.021
Pugazhendhi, A., Shobana, S., Bakonyi, P., Nemestothy, N., Xia, A., Banu, J., et al. (2019). A review on chemical mechanism of microalgae flocculation via polymers. Biotechnol. Rep. 21:e00302. doi: 10.1016/j.btre.2018.e00302
Roselet, F., Burkert, J., and Abreu, P. C. (2016). Flocculation of Nannochloropsis oculata using a tannin-based polymer: bench scale optimisation and pilot scale reproducibility. Biomass Bioenergy 87, 55–60. doi: 10.1016/j.biombioe.2016.02.015
Schulze, P. S. C., Pereira, H. G. C., Santos, T. F. C., Schueler, L., Guerra, R., Barreira, L. A., et al. (2016). Effect of light quality supplied by light emitting diodes (LEDs) on growth and biochemical profiles of Nannochloropsis oculata and Tetraselmis chuii. Algal Res. 16, 387–398. doi: 10.1016/j.algal.2016.03.034
Shen, P.-L., Wang, H.-T., Pan, Y.-F., Meng, Y.-Y., Wu, P.-C., and Xue, S. (2016). Identification of characteristic fatty acids to quantify triacylglycerols in microalgae. Front. Plant Sci. 7:162. doi: 10.3389/fpls.2016.00162
Şirin, S., Trobajo, R., Ibanez, C., and Salvadó, J. (2012). Harvesting the microalgae Phaeodactylum tricornutum with polyaluminum chloride, aluminium sulphate, chitosan and alkalinity-induced flocculation. J. Appl. Phycol. 24, 1067–1080. doi: 10.1007/s10811-011-9736-6
Vandamme, D., Foubert, I., and Muylaert, K. (2013). Flocculation as a low-cost method for harvesting microalgae for bulk biomass production. Trends Biotechnol. 31, 233–239. doi: 10.1016/j.tibtech.2012.12.005
Wu, T., Zivanovic, S., Draughon, F. A., and Sams, C. E. (2004). Chitin and chitosan value-added products from mushroom waste. J. Agric. Food Chem. 52, 7905–7910. doi: 10.1021/jf0492565
Xu, Y., Purton, S., and Baganz, F. (2013). Chitosan flocculation to aid the harvesting of the microalga Chlorella sorokiniana. Bioresour. Technol. 129, 296–301. doi: 10.1016/j.biortech.2012.11.068
Yen, M.-T., and Mau, J.-L. (2006). Preparation of fungal chitin and chitosan from shiitake stipes. Fung. Sci. 21, 1–11. doi: 10.7099/FS.200612.0001
Keywords: Nannochloropsis, flocculation, mushroom, chitosan, harvesting, vegan, omega-3
Citation: Chua ET, Shekh AY, Eltanahy E, Thomas-Hall SR and Schenk PM (2020) Effective Harvesting of Nannochloropsis Microalgae Using Mushroom Chitosan: A Pilot-Scale Study. Front. Bioeng. Biotechnol. 8:771. doi: 10.3389/fbioe.2020.00771
Received: 30 March 2020; Accepted: 18 June 2020;
Published: 14 July 2020.
Edited by:
Zhengquan Gao, Shandong University of Technology, ChinaReviewed by:
Jian Li, Panzhihua University, ChinaHongli Cui, Shanxi Agricultural University, China
Manoranjan Nayak, Korea Advanced Institute of Science and Technology, South Korea
Copyright © 2020 Chua, Shekh, Eltanahy, Thomas-Hall and Schenk. This is an open-access article distributed under the terms of the Creative Commons Attribution License (CC BY). The use, distribution or reproduction in other forums is permitted, provided the original author(s) and the copyright owner(s) are credited and that the original publication in this journal is cited, in accordance with accepted academic practice. No use, distribution or reproduction is permitted which does not comply with these terms.
*Correspondence: Elvis T. Chua, ZS5jaHVhQHVxLmVkdS5hdQ==