- 1Center for Comparative Medicine, University of California, Davis, Davis, CA, United States
- 2Department of Pathology and Laboratory Medicine, School of Medicine, University of California, Davis, Davis, CA, United States
Mouse models and genetically engineered mouse models (GEMM) are essential experimental tools for the understanding molecular mechanisms within complex biological systems. GEMM are especially useful for inferencing phenocopy information to genetic human diseases such as breast cancer. Human breast cancer modeling in mice most commonly employs mammary epithelial-specific promoters to investigate gene function(s) and, in particular, putative oncogenes. Models are specifically useful in the mammary epithelial cell in the context of the complete mammary gland environment. Gene targeted knockout mice including conditional targeting to specific mammary cells can reveal developmental defects in mammary organogenesis and demonstrate the importance of putative tumor suppressor genes. Some of these models demonstrate a non-traditional type of tumor suppression which involves interplay between the tumor susceptible cell and its host/environment. These GEMM help to reveal the processes of cancer progression beyond those intrinsic to cancer cells. Furthermore, the, analysis of mouse models requires appropriate consideration of mouse strain, background, and environmental factors. In this review, we compare aging-related factors in mouse models for breast cancer. We introduce databases of GEMM attributes and colony functional variations.
Preamble
Since the introduction of gland cleared mammary fat pad by DeOme and associates (Deome et al., 1959), mammary gland and mammary cell transplantation has been used to study aging in the mammary gland. One of the initial observations was that normal mammary epithelium had a limited capacity to fill the mammary fat pad and the original mammary epithelium would not grow after five or so serial transplants (Daniel et al., 1968). Serial transplant senescence defines a property of normal mammary epithelium, with “immortalization” or non-senescence proof of a neoplastic transformation (Daniel et al., 1968, 1975). Subsequently, this “test-by-transplantation” became the basis for the early descriptions of mammary gland stem cells their “committed” intermediates (Smith and Medina, 1988, 2008). This experiment laid the foundation for modern stem cell research. Although endocrine influence on the mammary gland was well-understood at the time, additional environmental factors were somewhat ignored (Young et al., 1971; Daniel et al., 1983) and not widely applied to problems in neoplasia.
Genetic engineering technology brought a new scientific generation into the study of experimental mammary tumorigenesis. The literature produced deals only indirectly with the problems of aging and mouse mammary tumorigenesis. Our objective is to organize and discuss this abundant data set specifically in the context of aging-related cancer.
Background
Breast cancer is an aging-related disease. According to American Cancer Society (www.cancer.org), multiple factors have been identified as risk factors. These factors include aging, gene mutations, ethnicity, breast tissue density, early menarche, menopause after age 55, exposure to certain chemicals and life style related factors (e.g., parity, breast feeding, birth control, hormone therapy, drinking alcohol, and obese). Each of the factors indicated above are also linking to aging. For example, first birth at an older age results in a higher risk (MacMahon et al., 1970) and the risk after parity is sustained in a woman who has her first pregnancy at an older age (Albrektsen et al., 2005; Meier-Abt and Bentires-Alj, 2014). Like the famous “nun study” suggesting that parity was protective, more recent studies comparing nulliparous and parous Norwegian women demonstrated that parity has a slight protective effect that increases with age (Albrektsen et al., 2005). The mechanism of increased cancer risk related to these proven epidemiological factors has been extensively investigated and debated. Clearly, endocrine changes with time affects incidence, but also raises a central conundrum of breast cancer risk—why does cancer risk increase with advancing age even as endogenous estrogen levels drop after menopause? And, for our purposes in this review, do mice model this change? Mice do not go through a “menopause” per se, but their reproductive capacity and hormonal levels, including estrogen, decline around 1 year of age (Nelson et al., 1982; Finch et al., 1984). Perhaps the better question is—do mice live long enough to get mammary cancer? This is where genetic engineering can help. Originally construed as a simple, cell intrinsic, proof of oncogene function, like a modern Koch's postulate for oncogenes, these genetic alterations, in many cases, instead result in a mild acceleration of the risk for developing mammary tumors. These genotypes are the important GEMM for studying breast cancer risk, risk modifiers, and aging.
A wide variety of GEMM have been generated to study beast and other cancers. Strong mammary epithelial specific-promoters driving potent oncogenes are most commonly used, with the obvious practical benefit of rapid and highly penetrant cancer development—a clear practical benefit to experimental design and execution (Borowsky, 2011). Mouse mammary tumor virus long terminal repeat (MMTV-LTR) (note: this construct does not include other viral elements, such as T antigens, and is not transforming by itself) and milk protein promoters such as whey acidic protein (Wap) and beta lactoglobulin (Blg) promoters are the most extensively used for transducing breast cancer-related genes in mouse mammary epithelial cells. However, the target molecule under MMTV-LTR promotion is transduced even in early developmental stages (Wagner et al., 2001), and most tumors with the MMTV-LTR as a promoter induce strong growth signals at a relatively early age in the mouse life span. On the other hand, Wap RNA expression is up-regulated by lactogenic hormones and is induced during the late stages of parturition and lactation (Pittius et al., 1988). This trait makes the Wap promoter a useful tool for analyzing the pregnancy-dependent effects. However, the transgene under Wap promoter activity is also activated in nulliparous mice and in early pre-lactation (Pittius et al., 1988) as well as during the early stages of involution (Wagner et al., 1997). The promoter activity is, however, the highest during pre-lactation/lactation.
Tumors arising in GEMM with transgenes constructed from strong epithelial promoters can be difficult to interpret. The promoters themselves are hormonally regulated, so that studying hormone blockers or depletion in these models can yield the trivial mechanistic result of down-regulation of the transgene. There is also the problem of simultaneous development and progression of multiple primary tumors, sometimes making the progression of a specific, single tumor impossible to study.
Gene knockout (GKO) mice also have been generated for breast cancer research and exhibit differences in mammary gland development and/or tumorigenesis. The GKO mice are generally designed to test for tumor suppressor type of genes. Therefore, the GKO does not usually result in the neoplastic alteration by itself. However, tumor suppressor GKOs often result in dramatic changes in tumor kinetics when crossed with an oncogenic transgene GEMM. Conditional gene knockouts using Cre-LOX technology and using mammary luminal specific (MMTV-LTR-Cre; Wap-Cre; Blg-Cre) or basal specific (K14- or K5-Cre) targeting are useful to focus on the molecular involvement in epithelial cells but may not reflect the importance of changes in the microenvironment during breast cancer progression.
The analysis of aging effects in breast cancer progression requires an understanding of senescence of any cell in the microenvironment as well as the cancer cell. Therefore, such phenomena as the immune cell activities on clearing damaged senescent cells must be considered. However, very little is known about how these microenvironment and immune cell activities are involved in GEMM. Variations in phenotypes depending on the mouse strain's background which also limits comparisons with other models. Further, the “environmental” factors, such as mouse housing, cycles of circadian rhythm, diet, viral—and bacterial-infections, can also affect the phenotype. However, environmental variables are almost completely ignored when models are compared. These environmental factors are also known as modifiers for immune cell activities and are important for immune cell-related aging. In this report, we introduce GEMM for breast cancer research which might be useful tools for analyzing the effects of aging and microenvironment.
Aging Parameters in GEMM
Aging in the mouse has been extensively described and discussed in literature and in monographic compendiums about pathobiology (Carlton et al., 2001) and physiology (Flurkey et al., 2007). The pathobiology has been updated for application to GEMM (Brayton et al., 2012). Numerous biomarkers for mouse aging and senescence have been described and applied. For example, the use of T-cell populations and IGF-1 levels have been widely used but other markers are also quite useful (Flurkey et al., 2007).
Endocrine factors have also been analyzed for aging related study in mouse (Flurkey et al., 2007). The status of ovarian hormone production is key to understanding aging-related hormone receptor positive neoplasms in mouse and human mammary neoplasms. Although estrogen receptor (ER) positive mammary tumors in GEMM have been analyzed in prior studies (reviewed in Dabydeen and Furth, 2014), not all models have been tested for hormone dependent- and independent-tumors actions using ovariectomy or hormone disrupting chemicals. Ovarian ablation experiments are important in GEMM because aged mice are not anovulatory as in humans (see the section below: “Aging in the human breast and mouse mammary gland and microenvironment”).
In the absence of biomarker data, life span is used for comparing potential aging effects on tumorigenesis in GEMM. Since the standard measurement of biomarkers have not been applied to GEMM of breast cancer and few, if any, laboratories keep colonies with sufficient populations to have accurate length of life data. We have arbitrarily adapted Harrison's life stages graph to estimate Mature (3–6 months), Middle Age (10–14 months), and Old (18–24 months) (Flurkey et al., 2007). Further, we have converted the numbers from months to weeks or days. The only data available, in most tumor related papers, is the T50 of tumorigenesis. These data can be misleading because of the chimeric populations used in some studies. However, the T50 is a useful metric for comparison.
Mammary Tumors in MMTV-LTR and Wap Promoter Driven Mouse Models
MMTV-LTR was first used to generate Tg(MMTV-Myc) in 1984 (Stewart et al., 1984). The activity of this promoter is sensitive to progesterone and dihydrotestosterone but not to estrogen (Otten et al., 1988). It can also be affected by the combinations of other factors, such as cell density, nutrition, growth factor, and hormones (Young et al., 1975; Cardiff et al., 1976). Currently, 114 GEMM lines targeting 45 genes (except for tTA, rtTA, and luc) have been registered in Mouse Genome Informatics (MGI) (Figure 1) (Bult et al., 2015; Blake et al., 2017; Finger et al., 2017). Of these 45 genes, human or mouse derived, 27 genes promoted by the MMTV-LTR developed mammary tumors. Whereas the median tumor latency time (a.k.a. median tumor-free latency; T50) is not always indicated or reported and the estimated T50 is variable ranging from 4 weeks to more than a year (52 weeks). Since MMTV-LTR is also active in embryonic tissues, the gene induction can be higher in developmental stages of mammary gland growth (Wagner et al., 2001). Transgenes with strong signaling inducing activity (i.e., PyMT, Erbb2, Catnb, Nras, Haras) form palpable tumors at relatively young ages. However, interestingly, the T50 in several MMTV-LTR driven GEMM are longer than a year. Remember that according to Flurkey et al. mice aged 10–14 months are equivalent to 38–47 years middle-aged human, and 18–24months are equivalent to 56–69 years older human (Flurkey et al., 2007).
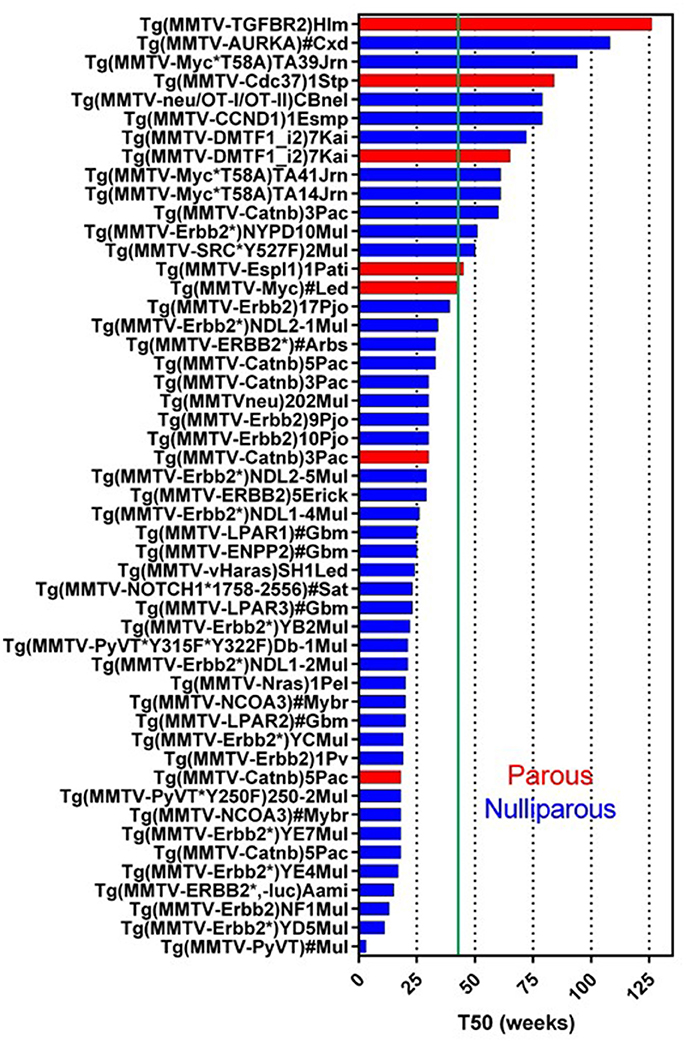
Figure 1. Tumor latency in MMTV-LTR driven GEMM. A graph indicates a tumor latency (T50, weeks) in each MMTV-LTR driven GEMM. GEMM in Mouse Gemone Informatics (http://www.informatics.jax.org/) are shown. Blue and red bars indicate nulliparous and multiparous, respectively. Green line indicates 43 weeks (10 months) when the mouse is in age equivalent in mid-age human (Flurkey et al., 2007). If each publication described T50 or a graph for percentage of tumor free, T50 is indicated as weeks. If the publication does not indicate these parameters but has enough information to predict T50, indicated T50 were calculated. MMTV-LTR driven GEMM, which does not have sufficient information to calculate T50 or have other information (e.g., non-palpable tumor but microscopic observation for hyperplastic region), are summarized in additional Supplementary Data Sheet 1.
In MMTV-LTR driven tumor-forming GEMM (Figure 1), mice with T50 longer than 10 months (43 weeks) or older number 13 including: Myc (Cardiff et al., 1991), Espl1 (Mukherjee et al., 2014), constitutively active SRC (Y527F) (Webster et al., 1995), auto-phosphorylation mutant Erbb2 (NYPD) (Dankort et al., 2001), Catnb (Imbert et al., 2001), DMP1β (Maglic et al., 2015), CCND1 (Wang et al., 1994), activated rat neu oncogene tagged with CD4 and CD8 epitopes (OT-I/OT-II) (Wall et al., 2007), Cdc37 (Stepanova et al., 2000), Myc mutant insensitive to KRas signal (T58A) (Andrechek et al., 2009), AURKA (Wang et al., 2006) and dominant negative type II TGFb receptor (TGFBR2) (Gorska et al., 1998).
Some models, when analyzed, demonstrate that parity facilitates tumorigenesis. MMTV-Catnb (Imbert et al., 2001), MMTV-Myc (Stewart et al., 1984; Cardiff et al., 1991), and MMTV-DMTF1 (Maglic et al., 2015) in parous mice have shorter T50 than nulliparous females. The MMTV-Espl GEMM forms tumor only with pregnancy (Mukherjee et al., 2014). Similarly, MMTV-PTGS2 nulliparous female mice do not develop tumors in, but 85% of multiparous female mice develop tumors (Liu et al., 2001).
Even GEMMs without tumor, crossbred to a GEMM with mammary tumors often results in different tumor kinetics. For example, the following crosses all resulted in accelerated tumor development: MMTV-MAT × MMTV-neu (Rudolph-Owen et al., 1998), MMTV-Vegfa × MMTV-neuYD (Oshima et al., 2004), MMTV-Akt1*T308D*S473D × MMTV-PyMT (Hutchinson et al., 2001). In contrast, MMTV-DTX1 × MMTV-HRas (or MMTV-cMyc) bigenic mice had better survival (Kiaris et al., 2004). Some GEMM publications which do not have sufficient information to estimate T50, are indicated in Supplementary Data Sheet 1.
Wap-promoter driven mouse model was first used to make Wap-HRAS (Andres et al., 1987; Nielsen et al., 1991). In 2017, 25 GEMM lines targeting 16 genes are registered in MGI. Wap promoter activity has been used to transactivate target cDNA in mammary epithelial cells during pre-lactation and lactation. These models might be useful for the study of parity-dependent tumorigenesis. In these GEMM, 13 genes transduced with Wap-promoter formed mammary tumor (Figure 2). Although the pregnancy usually occurs in younger, reproductive age mice, the T50s in Wap promoter driven GEMM vary widely from younger (21 weeks) to older (81 weeks) females. Examples include Wap promoter driving the expression of the intracellular domain of Notch4 with a longer T50 (43 weeks<) (nulliparous) (Gallahan et al., 1996), a truncated form of the SV40 T-antigen (T121) (Simin et al., 2004), SV40 large T (Tag) (Tzeng et al., 1993; Goetz et al., 2001), TDGF1 (Sun et al., 2005), SV40 small T (tAg) (Tzeng et al., 1993; Goetz et al., 2001) and constitutively active rat Mmp3 (Sympson et al., 1994).
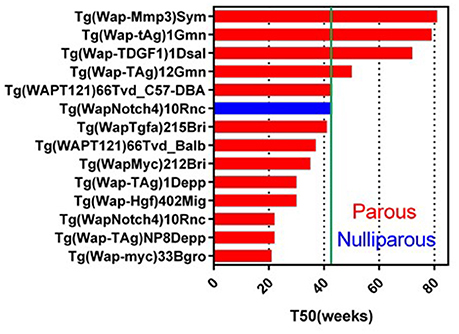
Figure 2. Tumor latency in Wap-promoter driven GEMM. A graph indicates a tumor latency (T50, weeks) in each Wap-promoter driven GEMM. Blue and red bars indicate nulliparous and multiparous, respectively. Green line indicates 43 weeks (10 months). As same as MMTV-LTR driven GEMM, if each publication does not indicate T50, the T50 is estimated from the information. Additional information for GEMM not listed on this graph is available in Supplementary Data Sheet 1.
Parity enhances tumorigenesis in the Wap-Notch4 (Gallahan et al., 1996). Wap-Shh is a Wap promoter driven GEMM which does not develop parity-dependent tumors other than in Cre expressing GEMM (García-Zaragoza et al., 2012). Interestingly, Wap-Myc showed the shortest T50 (21 weeks) (Schoenenberger et al., 1988) amongst the Wap-promoter driven GEMM. In contrast, MMTV-Myc showed longer latency (T50 = 43 weeks) (Stewart et al., 1984; Cardiff et al., 1991). This difference could reflect the transient Myc expression in Wap-Myc versus the sustained expression of Myc in MMTV-Myc. These Wap- promoted GEMM with longer T50s may provide useful clues for comparison of parity-related human breast cancer (Albrektsen et al., 2005; Meier-Abt and Bentires-Alj, 2014) and the effects of lactation in reducing human breast cancer risk (Ursin et al., 2004). For example, Wap-Myc (or other Wap-promoter driven) mice can have induced pregnancy at different ages and analyzed for tumorigenesis to identify the mechanism of higher risk in the first pregnancy in older age. The experiment can also be expanded to identify the mechanisms of multiparity dependent-or breast feeding dependent-risk reduction for breast cancer.
GKO Mouse Models and Mammary Tumors
GKO mice have also been used to analyze the molecular mechanisms in breast cancer. The Trp53 (p53) deletion is best known GKO mouse that develops mammary tumors. It is based on the study of heterozygous deletions found in Li-Fraumeni syndrome (Donehower, 1996). p53 heterozygous and homozygous knockout mice on mixed genetic backgrounds have tumor-free survivals (T50) of 78 and 18 weeks, respectively (Donehower, 1996). In contrast, on a pure Balb/c background p53 heterozygote and homozygous knockout mice have tumor-free survivals (T50) at 54 and 15.4 weeks respectively (Kuperwasser et al., 2000). Although these p53 GKO mice develop various types of tumor, palpable mammary tumors are rare on the mixed backgrounds. The Balb/c model had “mammary tumors” at the rate of 78%. However, microscopic examination of the masses revealed that 68% of “tumors” consisted of palpable masses were stromal abnormalities and sarcomas rather than epithelial tumors (Kuperwasser et al., 2000). Other studies with p53 deletions targeted to the mammary gland resulted in forming heterogeneous types of mammary tumors, suggesting a second event with different oncogenic drivers (Cardiff et al., 2006).
We reported that a signal transducer and activator of transcription 1 (Stat1) GKO in 129S6/SvEvTac background has a reduced branching morphogenesis during mammary gland development (Chen J. Q. et al., 2015). Most important, this GKO developed ER positive luminal-subtype mammary carcinomas with T50 at 91 weeks in nulliparous mice and 78 weeks in parous mice (Mori et al., 2017). This is a rare GKO which forms a morphologically uniform type of mammary tumor without crossing with the other GEMM or p53 GKO. This unique GKO will be discussed in more detail below.
Some studies using GKO crossed with tumorigenic GEMM targeting mammary epithelium have been instructive. Cell-cell association is also a microenvironmental signal to epithelial cells and provides important signals to polarize epithelial cells. Cdh1 f/f; Trp53 f/f is a Cre-dependent conditional deletion of E-cadherin (Cdh1) and p53 resulting in double GKO only in cells (and their progeny) that express the bacterial (exogenous) Cre recombinase. When crossed with K14-Cre (Derksen et al., 2006) or Wap-Cre (Derksen et al., 2011) to conditionally knockout Cdh1 and p53 in mammary basal epithelial cells or epithelial cells, respectively, the resulting K14-Cre; Cdh1 f/f; Trp53 f/+ developed mammary and skin tumors with T50 of 71 weeks. However, the homozygous deletions developed tumors with shorter T50s: K14-Cre; Cdh1 f/f; Trp53 f/f (T50 = 31 weeks) and K14-Cre; Cdh1 f/+ (or +/+); Trp53 f/f (T50 = 48 weeks) (Derksen et al., 2006). In contrast, the Wap promoter versions of similar crosses, Wap-Cre; Cdh1 f/f; Trp53 f/+, formed mammary tumor with T50 of 83 weeks (calculated from Kaplan-Meier tumor free survival) which was much longer latency than Wap-Cre; Cdh1 f/f; Trp53 f/f (T50 = 28 weeks), Wap-Cre; Cdh1 f/+; Trp53 f/f (T50 = 45 weeks), and Wap-Cre; Cdh1+/+; Trp53 f/f (T50 = 42 weeks) (Derksen et al., 2011). In both K14-Cre and Wap-Cre, genotype with Cdh1 f/f; Trp53 f/+ exhibited prolonged latency, suggesting that these GEMM might be useful for the study of aging-related mammary tumorigenesis. Cdh1 GKO model as another example of a homozygous GKO that is not sufficient for tumorigenesis but requires a cross with other GEMM to induce tumors.
Defects in BRCA1 and BRCA2 genes have been recognized as risk factors for human breast cancer and ovarian cancer (Narod and Foulkes, 2004). Brca1KO and Brca2KO have been developed with deletions in different exons. Most of the homozygous BrcaKO (mutants of Brca1KO and Brca2KO) were embryonic lethal (Evers and Jonkers, 2006). Conditional KO models of exon11 of Brca1 (Brca1Ko/Co: mouse carrying a Brca1-null allele Brca1Ko22 and a conditional allele Brca1Co crossed with WAP-Cre or MMTV-Cre) (Xu et al., 1999) have been analyzed for BRCA1 related studies. Females of these Brca1 GEMM do not form mammary tumors in the first 10 months of life. Within 10–13 months they do develop a tumor with low incidences of 2 of 13 Brca1Ko/Co; WAP-Cre and 3 of 10 Brca1Ko/Co; MMTV-Cre. These lower rates of tumorigenesis are increased and accelerated in crosses with Trp53 heterozygote: Brca1Ko/Co; MMTV-Cre; Trp53+/− mice (8 out of 11 between 6 and 8 months age) (Xu et al., 1999). The low incidence and prolonged latency suggest that these GEMMs could be useful for aging studies. However, like the majority of examples, to date these studies have not specifically emphasized “aging processes” in these models.
Mouse Strains and Their Basic Characteristics
Comparison of mouse models should be done in same background strain because of the intrinsic variation of phenotypes in each strain. Backcrossing GEMM to adjust the strain requires time, effort, and money. Any combination of these factors can be the reasons for avoiding breeding to homozygosity. But why this is important? Hunter's classical study of the MMTV- PyMT in 20 different background strains document the effect of genetic background a single transgene raised in the same colony (Lifsted et al., 1998). The F1 crosses exhibited different tumor latencies and different metastatic potentials. Studies from other facilities show that MMTV-PyMT had a T50 in C57BL/6: MMTV-PyMT of 92 days (14 weeks) as compared to the shorter T50 (43 days; 7 weeks) in FVB: MMTV-PyMT (Davie et al., 2007).
The normal life span in each colony is critical for the evaluation of any age-related study. Each inbred mouse strain has a different life span. Studies from the Jackson Laboratory have reported the comparison of survival rates from 12 to 20 months. They showed that the survival rate in C57BL/6, 129S1/SvlmJ, and FVB/NJ are almost identical (Sundberg et al., 2011). However, the survival rate at 20 months is lower in FVB/NJ (Sundberg et al., 2011). Therefore, the longevity is variable in different mouse strains. Comparison of life span in 32 inbred strains showed life spans that vary with gender and strain. For example, FVB/NJ (female = 760 days and male = 591 days), 129S1/SvlmJ (female = 819 days and male = 882 days) and C57BL/6J (female = 866 days and male 901 days) are life span in these mouse strains (Yuan et al., 2009).
The cause of the death is also variable in inbred mice. Age-related diseases and causes of death typical of some common strains have been documented (Brayton et al., 2012). Not surprisingly, their comparison of end of life neoplasms in three strains (B6, 129 and B6,129) in three institutions (UW, NTCR, NIH) also reveal differences incidence of neoplastic and non-neoplastic disease. These differences could be attributed to difference in mouse housing and maintenance.
Genetic drift in isolation is another factor. For example, some but not all FVB colonies have pituitary abnormalities. Shorter life spans in FVB might be partially related to the pituitary gland abnormalities (pituitary tumor 32%, pituitary hyperplasia 14%) in aging FVB/N mice. The study showed that about 30% of aged (>80 weeks) females also developed mammary gland tumors (Radaelli et al., 2009). Since FVB have been the most commonly used strain for transgenic targeting of the mammary epithelium, investigators using this strain need to collect pituitary at the end of the experiment.
The Brayton's study lists the factors which can affect mouse phenotype and experimental outcomes. Difference in diet (calories, its contents), enrichment, housing (cage type, density/cage, and pathogen status), ear tag, infectious agents, light cycle, and temperature can influence all phenotypes and experimental outcomes (Brayton et al., 2012). For example, studies with MMTV-neu show that diet alters the percent tumor free animals (Yang et al., 2003; Liu et al., 2005) and efficacy of tamoxifen (Liu et al., 2005).
The Microenvironment
The normal mammary microenvironment of the mouse has been extensively studied. However, most reviews emphasize the immune system or hypoxia (Rothschild and Banerjee, 2015). With the success of immunotherapy syngeneic tumor transplant models have become extremely useful. Without a doubt, all the other cell populations deserve mention. Whereas many factors play major roles in the epithelial-environmental, there is almost no information with the lack of mouse models of microenvironmental factor and aging. As examples of some of the molecules, we discuss herein the metalloproteinases and adhesion molecules.
Extracellular proteins, cell surface molecules and signaling molecules are the targets for the analysis of stromal-epithelial interactions. Some molecules involved in association between extracellular matrices (ECMs) and cell surface receptors have been analyzed in GKOs. These GKOs are often crossed with tumorigenic MMTV-LTR or Wap-promoter driven GEMM, because GKO of these molecules might not be sufficient to form palpable mammary tumors by themselves. As summarized in Supplementary Data Sheet 1, ECM molecule-related GKOs have been crossed mostly with MMTV-PyMT and MMTV-neu.
For example, metalloproteinases (matrix metalloproteinase: MMP; a disintegrin and metalloprotease: ADAM) are a family of zinc binding type extracellular proteinases which process ECM molecules and surface molecules (Wolfsberg et al., 1995; Nagase and Woessner, 1999). These molecules are known to be involved in various physiopathological activities including development, tissue remodeling and cancer cell invasion (Rudolph-Owen et al., 1998; Seiki et al., 2003; Fata et al., 2007; Reiss and Saftig, 2009; Mori et al., 2013). Some of these MMP genes (Mmp-8, and -14) and ADAMs (Adam-12 and -ts1) have been used in studies of mammary tumor progression by crossing GKO with MMTV-PyMT. Deletion of Adam genes delay PyMT tumor growth and metastasis (Fröhlich et al., 2011; Ricciardelli et al., 2011). Deletion of the Mmp genes had a different effect in PyMT tumorigenesis. Interestingly, both Mmp8KO and Mmp14KO in bigenic crosses with PyMT developed tumors earlier than WT-PyMT control (Szabova et al., 2008; Decock et al., 2015). Lung metastasis of PyMT tumor was reduced in bigenic mice with Mmp14KO (Szabova et al., 2008). In contrast, Mmp8 deletion enhanced PyMT pulmonary metastasis (Decock et al., 2015).
Tissue inhibitor for metalloproteinase 3 (Timp3) GKO when crossed with MMTV-PyMT and MMTV-neu inhibited tumor growth and lung metastasis (Jackson et al., 2015). The T50 in MMTV-neu in the absence of Timp3 was ~76 weeks compared with ~31 weeks in Timp3+/+; MMTV-neu (calculated from Kaplan-Meier curve of the age at first detection of tumor) (Jackson et al., 2015), suggesting that Timp3−/− MMTV-neu might be another suitable model for studying aging-related breast cancer progression. Whereas Timp3−/−; MMTV-PyMT tumor delayed T50 (~13 weeks compared with control: 10 weeks), tumor growth was accelerated once tumor was formed (Jackson et al., 2015). Since TIMP3 has broad-spectral inhibition activity on both MMPs and ADAMs (Brew and Nagase, 2010; Murphy, 2011), TIMP3 target protease activities might be differentially involved in tumor growth and metastatic conversion. Once spatiotemporal distributions of MMPs, ADAMs, and TIMP3 were identified during mammary tumor progression are revealed, the TIMP3 effects on each target protease can be understood. Nonetheless, the delay in tumorigenesis in Timp3−/−; MMTV-neu could be another useful model for studying age-related mammary tumorigenesis.
Other GKO mice crossed with MMTV-PyMT and -neu have also been used to study the functions of cell adhesion molecules' involvement during the neoplastic process. In particular, many of these molecules mediate the association between cells and microenvironment. Since PyMT tumors forms earlier than neu tumors, the PyMT tumor model may not be as good a model for the study of aging. However, accelerating or delaying tumorigenesis in neu- or PyMT-tumor might reveal the function of target molecules that may affect aging.
The models described here are GKO crossed with other mammary tumor models. To analyze the association between collagen and the receptor (integrin a2b1), Itga2KO was developed (Chen et al., 2002). This Itga2KO was then crossed with MMTV-neu (Ramirez et al., 2011). The bigenic cross showed the earlier T50 (31 weeks) with higher metastatic levels then the comparable Itga2+/+; MMTV-neu control (33 weeks). CD151, a tetraspanin molecule associating with laminin binding integrins (Hemler, 2005), was knocked out and the mice crossed with MMTV-neu to study the molecular interactions with anErbB2 driven cancer (Deng et al., 2012). The T50 of the bigenic CD151−/−; MMTV-neu was 48 weeks which indicates slower tumor growth compared with the control 38 weeks in the control CD151+/+; MMTV-neu mice.
The GKO of Hic5, a focal adhesion scaffold/adaptor protein, was crossed with MMTV-PyMT and the T50 was 9 weeks longer than the heterozygous knockouts with Hic5+/−; MMTV-PyMT (Goreczny et al., 2017). Protein-tyrosine phosphatase 1B (PTP1B), which is involved in regulating signals between cell and microenvironment, was knocked out and crossed with MMTV-PyMT and -neu (Bentires-Alj and Neel, 2007). Interestingly, Ptp1b−/−; MMTV-neu exhibited a greatly reduced tumorigenesis of neu tumor (27% mice formed tumors at 69 weeks) as comparing with Ptp1b+/−; MMTV-neu and Ptp1b+/+; MMTV-neu (T50 = 61 weeks and 57 weeks respectively). Ptp1b−/−; MMTV-PyMT did not, however, significantly delay PyMT tumor growth (Bentires-Alj and Neel, 2007). Some mammary tumor forming GEMM are also used to inhibit target molecule's activity (MMP9, Lysyl oxidase) or crossed with transgenic of mutant protein (e.g., collagenase resistant type I collagen alpha1 chain, Col1a1) are indicated in Supplementary Data Sheet 1.
These mouse models are useful for the study of various aspects of tumor progression. The mammary promoters (MMTV-LTR, Wap-, and K14-), however, are activated in other cells in different organs. MMTV-Cre; Gt(ROSA)26Sor transgenics demonstrate that the MMTV-LTR promoter actively transduces Cre in a variety of non-mammary tissues (e.g., salivary gland, skin, seminal vesicles, lymphocytes, megakaryocytes, erythroid cells, etc.) (Wagner et al., 2001). In addition, three MMTV-Cre promoted lines showed the defects in nursing pups; line A showed impairment in lactation, line F completely failed to nurse, and line D was normal.
Wap-cre; Tg(CMV-nlacZ)1Pgr showed Cre expression in brain, muscle and testes, and one line had Cre expression in mammary gland and the brain (Wagner et al., 1997). K14-Cre (Vasioukhin et al., 1999), K5-Cre (Tarutani et al., 1997), and Lgr-CreERT2 (Kinzel et al., 2014) have also been used to conditionally knockout gene targets in mammary epithelial cells (Taddei et al., 2008; Koren et al., 2015; Liu et al., 2016). These promoters were originally developed to study skin tissue (Tarutani et al., 1997; Vasioukhin et al., 1999; Kinzel et al., 2014) and other organs (gut and kidney) (Kinzel et al., 2014). Thus, these promoter activities are not specific to mammary epithelial cells. This suggests that the effect on mammary gland in GKOs crossed with these Cre expressing GEMM might be more complex than mammary epithelial-specific promoters. Once aging dependent or independent inflammatory activity occurs in one organ, the activity of immune cells will be modified and can affect other organs in the same animal. In addition, crossing these models with different mouse background strains can cause the variations in phenotypes (e.g., immune cell activity, drug efficacy, tumorigenesis). In next section, we summarize these parameters.
Aging in Immune Cells
The Jackson Laboratory's analysis of immune cell populations in peripheral blood in 6 months old inbred mice demonstrates that lymphocytes in female (F) and male (M) differ between strains and genders (Petkova et al., 2008). Examples include C57BL/6J which are F: 71.5% and M:78.2%, FVB/NJ are F:79.9% and M:52.5% and 129S1/SvlmJ are F:49.4% and M:60.5%(Petkova et al., 2008) Monocytes in C57BL/6J are F:4.47% and M:7.62%, FVB/NJ are F:5.79% and M:6.6% and 129S1/SvlmJ are F:8.3% and M:8.61% (Petkova et al., 2008). More importantly, these percentages of immune cells and the immune cell activities might also be different with age of the mouse strain. Studies of aging in immune cells, named “inflammaging” (Baylis et al., 2013; Salvioli et al., 2013; Franceschi and Campisi, 2014; Franceschi et al., 2017), indicate that each immune cell lineage and their activities change with age. These age-related changes can directly affect tumor initiation and progression. Since immune cells are a factor in “tumor microenvironment” and important in clearing damaged cells (such as senescence cells), immune cell activities in aged mice might also provide hints to decipher the link between aging and breast cancer.
Lymphocytes
Decreased lymphoid progenitors and involution of the thymus occur during aging (Linton and Dorshkind, 2004; Palmer, 2013). This results in a decrease in T-cell output and shifts in cell types from naïve (CD62Lhigh CD44low) to memory T-cells (CD62Llow CD44high) (Shimatani et al., 2009; Sprent and Surh, 2011; Goreczny et al., 2017). These aging memory T-cells are PD1+ and CD153+CD44high CD4+ (Sato et al., 2017). They have the same phenotype as senescence associated T-cells found in lupus pathogenesis (Tahir et al., 2015), suggesting that the aging dependent increase in CD4+ T-cells might also be involved in increased risk of autoimmunity. Memory CD8+T cells are also increased in aged mice (Chiu et al., 2013). The memory CD8+ T cells in older mouse expand the numbers of effector CD8+ T cells in LCMV infection compared with younger mouse derived memory CD8+ T cells (Eberlein et al., 2016). This critical change in T cells could be an anti-pathogen defense system in aged mice with less naïve T-cells.
In aged humans, decreased memory B-cells and increased naïve B-cells are observed (Chong et al., 2005). In mice, B-cells numbers are decreased with aging because of decreased precursors in bone marrow (Riley et al., 1991). This is further defined as the defect in the process between pro-B and pre-B maturation in aged mice (Stephan et al., 1996). The defect in pro-B to pre-B maturation in aged mice is attributed to insufficient levels of IL-7 (Fleming and Paige, 2001). In MMTV-PyMT mice, splenic B-cells are increased, This might be considered as evidence of inhibitory factors secreted from the PyMT tumor cells that inhibit B-cell progenitor proliferation and maturation (Moreau et al., 2016).
Regulatory T cells (Treg: a.k.a. suppressor T cells) are also altered in aged mice. CD4+/CD25+/Foxp3+ and CD8+/CD25+/Foxp3+ Treg cells are almost two times higher in spleens and lymph nodes from aged mice, and prevents the activation of immune response (Sharma et al., 2006). Increased Treg are also observed in both peripheral blood and tumor microenvironment of invasive breast cancers (Liyanage et al., 2002). C57BL/6 with Foxp3 knocked-out/knock-in human diphtheria toxin receptor (DTR; Foxp3DTR) (Kim et al., 2007) is a GEMM for analyzing Treg activities in the presence or the absence of diphtheria toxin (DT). Orthotopic injection of a C57BL/6; MMTV-PyMT derived mammary tumor into Foxp3DTR treated with DT results in reduction of PyMT tumor growth. Enhanced apoptosis was noted with proliferation of naïve CD4+ cells and CD8+ cells (Bos et al., 2013).
Macrophages
Macrophage phagocytosis is involved in clearing apoptotic cells and is important for maintaining tissue homeostasis. However, aging reduces the macrophage capacity for clearing apoptotic cells (Aprahamian et al., 2008). Moreover, macrophage treated with tunicamycin, an inducer for endoplasmic reticulum stress, showed that macrophages isolated aged mice have more apoptosis compared with macrophages from younger mice (Song et al., 2013). Macrophage activation is also altered in aging. Cytokine induction to direct macrophage to M1 is increased in aged mice, but the M2 induction is decreased. Conversely, induction to M1 is weaker in younger mice, but induction to M2 is stronger (Lee et al., 2013).
Deletion of p53 in hepatic stellate cells (HSCs) induces fibrotic liver tumors which have proliferating HSCs and senescence of HSCs (Lujambio et al., 2013). Interestingly, conditioned media from proliferating HSCs induces M2 polarization, and contrary, conditioned media from senescent HSCs induces M1 polarization of macrophage (Lujambio et al., 2013). The macrophage polarization in two studies suggest that aging reduces macrophage induction to M2 status. This might decrease the anti-tumor activity of macrophages and facilitate tumorigenesis. This secretion from senescence cells is called senescence associated secretory phenotype (SASP). SASP causes pro- and anti-tumor activities through affecting to different types of immune cells (Rao and Jackson, 2016). Since secretion from cells have soluble factors (e.g., cytokines, processed proteins from cell surface) and extracellular vesicles (exosomes, microvesicles, and apoptotic bodies) (El Andaloussi et al., 2013), the identification of the contents within each fraction, function, levels, and distribution is now required to understand SASP as a part of aged microenvironment.
Myeloid Derived Suppressor Cells (MDSCs)
MDSC is a key immune cell in the control of immune cell activities and reciprocally associates with Treg to regulate tumor progression (Hurez et al., 2012, 2017; Marvel and Gabrilovich, 2015). MDSCs are increased in aged mice (Grizzle et al., 2007; Enioutina et al., 2011) and human (Verschoor et al., 2013). This age dependent MDSC expansion is regulated by Nf-kB activity in aged mice (Flores et al., 2017). Aged mice have increased MDSCs and less T-cell cytotoxicity compared with young mice. The suppression of T-cell cytotoxicity is due to the high levels of arginase activity in aged MDSCs (Grizzle et al., 2007). Interestingly, the increase of MDSCs is also observed in bone marrow and spleen of tumor-bearing mice compared with naïve mice (Huang et al., 2006). These MDSCs from tumor-bearing mice induce the development of Treg (Huang et al., 2006) and T-cell tolerance (Kusmartsev et al., 2005; Huang et al., 2006). These age-related tumor permissive activities of MDSCs have been targeted for immunotherapy. Higher levels of tumor permissive activity in aged mice can be reduced with anti-GR1 targeting MDSCs, which reduces melanoma tumorigenesis (Hurez et al., 2012). Aged MDSCs have higher levels of B7-H1 (a.k.a. Programmed death ligand 1, PD-L1) induced by IL-10 activity, and the inhibition of B7-H1 reduced the tumorigenesis of Lewis lung cancer cells in aged mice (Chen S. et al., 2015).
Dendritic Cells
Dendritic cells (DCs) are antigen-presenting cells and categorized by differentiation status into conventional DCs (cDCs), plasmacytoid DCs (pDCs), and monocyte DCs (moDCs). The DCs are involved in a key function on T-cell activation (Gardner and Ruffell, 2016). Twenty six-month-old male C57BL/6 mice (from Jackson Laboratory and maintained at Oregon State Univ) had decreased splenic pDCs and CD8+ cDC (Wong et al., 2010). Another study with 18-month-old female C57BL/6 mice (from State Foundation for Production and Health Research and maintained at Instituto de Pesquisas Biomédicas, Brazil) found reduced numbers of CD11c+ cells but only cDCs were significantly reduced as compared with young mice (Pereira et al., 2011). However, C57BL/6 mice (from Animal Resources Center and maintained at The Centenary Institute for Cancer Medicine and Cell Biology, Australia) older than 18 months had increased cDCs in spleen and lung as compared to young mice. In contrast, Balb/c mice did not show the same difference between aged and young mice (Tan et al., 2012). This study also showed by comparison of T-cell activation of DCs from aged- and young- C57BL/6 mice no differences in co-stimulatory molecules (CD80, CD40, and CD70) except for CD86 (B7-2) which is reduced in aged cDCs with influenza infection (Tan et al., 2012). However, aging effect on CD86 levels are not differ in cDCs in above mentioned two other studies (Wong et al., 2010; Pereira et al., 2011) and without influenza infection (Tan et al., 2012). Thus, the difference in cDCs might be affected by variations in mouse strain background, gender, age, housing environment and pathogen activities. In a ovarian tumor model (C57BL6: LSL-K-rasG12D/+p53loxP/loxP generated from Krastm4Tyj and Trp53tm1Brn mice), DCs changed the phenotype from immunosuppressive in the earlier stage of tumor expansion to immunogenic in the advanced stage of cancer (Scarlett et al., 2012), This switch suggests that the status of DCs might indicate the checkpoint for neoplasia as it become malignant.
Natural Killer Cells
Natural killer (NK) cell is a type of lymphocyte and an important cell for innate immunity.
A comparison of NK cells in healthy people from 4 to 106 years of age showed that NK cells were increased in older age, but NK activity in middle-aged subjects was weaker compared to young subjects and to centenarians (Sansoni et al., 1993). In mice, there were strain dependent and gender variations in a percentage of peripheral NK cells (Petkova et al., 2008). Whereas C57BL/6 and 129S1/SvlmJ mice have similar percentages of NK cells between the genders (C57 female: 3.74%, male: 4.02%; 129 female: 5.5%, male: 4.66%), FBV/NJ male mice have a significantly fewer NK cells (3.49%) than females (7.15%) (Petkova et al., 2008). NK cells are increased in bone marrow in aged Balb/c mice, resulting in decreased pro-B cells in aged animals (King et al., 2009). NK cells (with different levels of CD56 and CD16) were increased in the peripheral blood from breast cancer patients with advanced cancer but were mostly immature and non-cytotoxic subsets (Mamessier et al., 2013). Spleen tissues in FVB; MMTV-neu mice have almost similar number of NK compared with wild type (WT) FVB mice while other immune cells (T cells, DCs, MDSCs, and Tregs) are increased in FVB; MMTV-neu (Abe et al., 2010). In C57BL/6; MMTV-PyMT, tumor derived NK cells are immature compared with splenic NK cells. An adaptive transplantation of splenic NK cells into tumor resulted in decreased NK maturation, suggesting that tumor microenvironment in MMTV-PyMT tumor determines the NK cell maturation. The authors suggested that IL12 and TGFb are involved in NK maturation (Krneta et al., 2016). Thus, although NK cell numbers and activities differ in human and mouse, levels of immaturity in NK cells is similar in human breast cancers and mouse mammary tumors.
Immune cells' variations and activities different between mouse strains, genders, and age. The spatial density and activity of each type of immune cell within the tissue is critical to understanding associations between immune cells and immune targeted cells (e.g., tumor cells and cells in senescence). These associations are important for understanding age related tumorigenesis. They are equally critical for understanding the process of immunoediting from “elimination,” “equilibrium” to “escape” (Schreiber et al., 2011; Mittal et al., 2014). The challenge is now to relate the two phenomena.
Aging in the Human Breast and Mouse Mammary Gland and Microenvironment
Human breasts and mouse mammary glands are functionally similar. However, these tissues have distinct structural differences especially during the aging process (Cardiff et al., 2018). In puberty, human breast completes the formation of branching collecting ducts with terminal duct lobular unit (TDLU) in collagen fiber rich stroma. In contrast, mouse mammary glands form a ductal branching tree without TDLU structures and primarily embedded in adipose tissue. Mouse mammary gland develops lobulo-alveolar structures with pregnancy. The murine lobulo-alveolar units quickly involute within a week of weaning (Cardiff and Wellings, 1999). Human breast also expands lobulo-alveolar units with endocrine signals during pregnancy and parturition. In contrast to the mouse, the human involution is slow and the TDLU structures remain (Cardiff and Wellings, 1999) with some structural variations, which correlate with time, after the postpartum (Jindal et al., 2014). Age- related involution in human breast occurs during perimenopause (or surgical- or chemical induced menopause) with the atrophy of senescence, which results in major reduction in TDLU structures (Cardiff and Wellings, 1999; Cardiff et al., 2018). In contrast, aged mice continue to ovulate and have estrus cycles. This limits the use of mice to model human peri- and post-menopause conditions (Nelson et al., 1982; Finch et al., 1984). In order to analyze menopause dependent effects, the investigator can us surgery- and chemical treatment-dependent induced menopause in mice. One approach might be ovariectomy (Finch et al., 1984) which quickly shuts down the 17β-Estradiol levels, stops the cyclicity and quickly maximizes FSH levels (www.jax.org). Another is 4-vynylcyclohexene diepoxide (VCD) treatment, which slowly increases FSH levels and slows down cyclicity (Brooks et al., 2016).
Studying Parity-Related Breast Cancer and Aging
Although multiple-pregnancies reduce the incidence rate of human breast cancer (Albrektsen et al., 2005), the risk reduction is sustained longer in women having a first pregnancy after 35 years old (Albrektsen et al., 2005; Meier-Abt and Bentires-Alj, 2014). Women having a first pregnancy after 30 years old also have higher risk for breast cancer and the risk is sustained higher until 65 years old compared with woman with nulliparous status (Albrektsen et al., 2005; Schedin, 2006; Meier-Abt and Bentires-Alj, 2014). The many factors (stem cells, hormone and hormone receptor status, growth factors, cytokines, ECM, and immune cell activities) which might be involved in these age- and parity-dependent breast cancer are discussed elsewhere (Schedin, 2006; Meier-Abt and Bentires-Alj, 2014).
The duration of breast-feeding is also an important factor. Prolonged nursing (lactation) reduces the risk of breast cancer even in aged cohort (50–64 years old)(Ursin et al., 2004). Analyzing involvement of each factor which functions as cancer promoting or suppressing will be a challenge for future research. The effects of parity have been well-documented in mouse models. Although some GEMM have prolonged tumor latency, factors relating to aging have not been recorded in many of the candidate models. But the current GEMM or newly generated GEMM combined with analysis indicated in this manuscript might give a hint for modeling the experiments. For example, the analysis of microenvironmental factors in parity facilitated, syngeneic orthotopic transplants in mice (Maglione et al., 2004; Namba et al., 2004, 2005; Borowsky et al., 2005) under different well-controlled conditions of age, parity status, parity cycles, and milk feeding duration. Since tumors from some GEMM grow too aggressively, tumors with slower tumor kinetics will be more suitable for analyzing aging and parity related tumorigenesis.
Stat1 GKO as a Model for Analysis For Age Related Mammary Tumorigenesis
The focus on mammary cancer in mice has been, quite correctly, the mechanisms that make the neoplastic epithelial cell autonomous. The transgenic mouse has provided the “Koch's Postulate” for modern genomic science and cancer by defining the neoplastic potential of numerous genes and/or characterizing genes that modify neoplastic progression. In spite of a plethora of these mice, they have not been used to address the issue of aging in breast cancer. Although research on the cancer microenvironment has blossomed, the issue of aging in GEMM has not been effectively addressed.
We have studied in detail a GEMM that includes prolonged tumor latency, 129S6/SvEvTac: Stat1tm1Rds (129: Stat1−/−) mice (Meraz et al., 1996), which is a unique GKO that rarely develops mammary cancer before 1 year and has a T50 of 91 weeks (Mori et al., 2017). The Stat1−/− females were held as long as 120 weeks (840 days) which exceeded the expected 817-day life span of wild type 129 females. From this perspective, the T50 was reached at 78% of the total life span. The 129: Stat1−/− provides some insight into many of the factors considered in the review above and the opportunity to consider the complex issues in such models.
Other groups have demonstrated that deletion of Stat1 leads to an increased susceptibility to mammary tumors (Klover et al., 2010; Raven et al., 2011; Schneckenleithner et al., 2011; Chan et al., 2012). The 129: Stat1−/− model forms mammary tumors with a T50 at 91 weeks in nulliparous and at 78 weeks in parous females, similar to the report from the colony in Washington University (Chan et al., 2012). Mice younger than 32 weeks old in our colony did not have detectable neoplastic histopathology. Mice older than 52 weeks nulliparous without tumors had microscopic MIN (mammary intraepithelial neoplasia; 3/32 cases), and 23/32 cases had preneoplastic MIN or tumors.
Tumor-bearing nulliparous (9/13 cases) showed lobulo-alveolar hyperplasia even without pregnancy or exposure to the pregnancy-associated hormones and these mice had dilated mammary duct filled with milky white fluid. The older tumor-bearing nulliparous, with stunted growth in mammary gland (4/32 cases) still had underdeveloped ductal network (Chen J. Q. et al., 2015).
The strain variation is also an important issue in Stat1 GKO. Other Stat1 GKO lines, Stat1tm1Dlv, backcrossed into Balb/c strain also shows tumorigenesis in multiparous Stat1-null condition with mammary tumor and disease onset at 57 weeks which is shorter latency compared with Stat1+/+ (69 weeks) (Durbin et al., 1996). Balb/c: Stat1tm1Dlv crossed with Balb/c: MMTV-Neu shows shorter latency of Neu type tumors as compared with Balb/c: MMTV-Neu nulliparous mice (42 vs. 50 weeks) (Raven et al., 2011). Similarly, Stat1f/f was crossed with FVB: Tg(MMTV-Erbb2*,-cre)1Mul (Ursini-Siegel et al., 2008) to conditionally knock out Stat1 gene and simultaneously transduce neu in mammary epithelial cells, which also results in an earlier T50 in the Stat1-null condition (50 vs. 63 weeks) (Klover et al., 2010). These two studies (Klover et al., 2010; Raven et al., 2011) suggest STAT1 as a tumor suppressing molecule, and the work of Klover et al. (2010) indicates that STAT1 function in mammary epithelium is anti-tumorigenic.
Interestingly, mammary gland development in Stat1 GKO is only impaired in 129S6/SvEvTac (Chen J. Q. et al., 2015) but the defect has not been observed in GKO in the Balb/c background (Schneckenleithner et al., 2011). Mammary tumorigenesis is also different in these two strains: 129:Stat1−/− forms homogeneous mammary tumors with or without parity (Chan et al., 2012; Mori et al., 2017), but Balb/c: Stat1−/− formed heterogeneous tumors only with multiparity (Schneckenleithner et al., 2011). However, these animals may not have been maintained long enough to develop tumors. For example, in one study, the Balb/c: Stat1−/− females developed mammary tumors only after being force-bred seven times.
The interpretation of these observations is compounded by the use of different molecular constructs for the knockout of Stat1, which further could have influenced the outcome. The Meraz construct deletes the N-terminus of STAT1 in 129/Sv mice, whereas residual low expression level C-terminus fragment remains (Meraz et al., 1996). The other construct deleting exon 18–20 in unknown background mouse strain does not have this fragment detected by anti-C-terminus STAT1 (Bailey et al., 2012). However, exon 18-20 deleted Stat1−/− mice still need to be investigated with anti-N-terminus STAT1 and analyzed for any defect. Thus, mechanisms leading to tumor development in 129: Stat1−/− remain unclear.
The initial study of normal mammary development in the 129: Stat1−/− females revealed a defect in branching morphogenesis accompanied by abnormal terminal end buds (Chen J. Q. et al., 2015). Given the type of molecular signaling, this could be expected. The DeOme's “Test-By-Transplantation” (Deome et al., 1959) with reciprocal transplants into gland-cleared mammary fat pads was used. The GKO epithelium developed normally in wild type mammary fat pad. Thus, the developmental phenotype was not intrinsic to the mammary epithelium.
Subsequent experiments proved that the GKO fat pad lacked cytokines needed for growth. Therefore, we concluded that the microenvironment of the fat pad was responsible for the growth defect. Interestingly, the GKO fat pad could be stimulated to produce adequate levels of cytokines using injections of a progesterone and prolactin. Unfortunately, we were unable to perform similar experiments using fat pads from older GKO females. These experiments, however, point to the importance of the microenvironment and the endocrine system in mammary development and demonstrate the consequences when they are defective.
The 129: Stat1−/− mammary tumors are morphologically and molecularly unique, ovarian dependent tumors. The tumors involve the neoplastic transformation of a stem cell population that is FoxA1+, ER+, PR+ with unique atypical cells with large, oval, moderately pleomorphic nuclei with an open chromatin and prominent nucleoli with pale cytoplasm, named large oval pale (LOP) cells. The characteristic of LOP cell is similar to “committed progenitor cells” in mouse mammary gland (Smith and Medina, 1988; Chepko and Smith, 1999) and suprabasal clear human breast cells (Toker, 1967; Stirling and Chandler, 1976, 1977; Smith et al., 1984). In addition, FoxA1 is known as a key factor (“pioneer transcription factor”) (Zaret and Carroll, 2011; Jozwik and Carroll, 2012; Zhang et al., 2016) which regulates the expression of estrogen receptor alpha (Bernardo and Keri, 2012; Liu et al., 2016; Zhang et al., 2016) and is an essential factor for mammary branching morphogenesis (Liu et al., 2016). The cell of origin is readily identified as the LOP cell. In the early manifestations, the LOP cell can be found distributed along ducts. Later, the LOP cell forms large, invasive neoplasms. The epithelial intrinsic mechanisms have been described including activation of the JAK2 axis, deregulation of SOCS3 and mutation of the prolactin receptor (Chan et al., 2014; Griffith et al., 2016).
The differences between two GKO Stat1-null strains might be reflecting the differences in immune cells: percentages of CD4+ T cells, CD8+ T, cells and NK cells in peripheral blood are higher in Balb/cByJ, and granulocytes, eosinophils, monocytes and B-cells are higher in 129S1/SvlmJ (Brayton et al., 2012). Because of STAT1 involvement in interleukin 2 signal in T lymphocytes and NK cell lines (Frank et al., 1995), the Stat1−/− mice were originally generated to analyze the STAT1 involvement in NK cell activity via interferon (IFN) dependent signals and in other cytokines' signals (Meraz et al., 1996). The study showed that STAT1 deficiency leads to inactivation in IFNa and IFNg signals (Durbin et al., 1996; Meraz et al., 1996) and has a defect in responding to microbial pathogens (Meraz et al., 1996) and viruses (Durbin et al., 1996; Meraz et al., 1996), but the response for other signals (growth hormone, EGF and IL-10) were not impaired (Meraz et al., 1996). In later study, 129: Stat1−/− was shown to have a defect in cytolysis activity in NK cells (Lee et al., 2000).
Our pathological analyses of the three cohorts of older 129: wild type (WT), tumor free 129: Stat1−/− and tumor-bearing 129: Stat1−/− revealed characteristic lesions of aging: including. ovaries with luteinized stroma and fewer Graafian follicles. However, the hormone regulating glands (adrenal gland, thyroid gland, and pituitary gland) were all histologically normal. Evidence active estrus cycle was still found in 96 weeks old mice, indicating the ovarian hormones were still active. Although some 129: Stat1−/− showed the defects such as ovarian cysts, not all female with ovarian cysts developed mammary tumor, and one tumor-bearing female had histologically normal ovaries. These observations on ovaries and estrus cycle indicate that the tumorigenesis in 129: Stat1−/− is not due to the lack of ovarian hormones.
To understand the effect of the microenvironmental factors in Stat1-null tumorigenesis, syngeneic orthotopic transplants of Stat1-null tumor and MIN tissue into younger 129 WT and KO were performed. Tumors were palpable earlier in WT host. However, the tumor growth rates were almost the same in both hosts. This still resulted in 10-100 folds larger tumor in WT at the termination time point.
The analysis primary tumors in 129:Stat1−/− showed that stroma adjacent to the tumor had larger infiltrates of inflammatory cells (macrophage and T-lymphocytes) with fibrosis as compared to other GEMM (Cardiff et al., 2006). These observations of immune cell activities suggested that Stat1-null tumor makes an immune-attractive tumor-adjacent microenvironment and has an “excluded infiltrate” phenotype (Hegde et al., 2016). To investigate the possible mechanisms, we analyzed factors secreted from Stat1-null cell lines and found that soluble factors inhibit macrophage migration whereas the extracellular vesicle fraction stimulates macrophage migration. This indicates that SASP activity in Stat1-null tumors involves with cross talk with the microenvironment.
The point of introducing our study here is to provide an example of analyzing aging- related tumorigenesis in the mammary gland. First, as we discussed in above, environmental factors (housing, cycles of circadian rhythm controlled by light/dark cycles, diet, viral- and bacterial-infections and any other pathogens) for maintaining mouse colony can affect mouse phenotype. It is not easy to adjust these parameters in every institution to compare mouse models, at least checking viral- and bacterial-infection should be done to consider if immune cell activity is different or not in each mouse colony. Our colony ismonitored using a serology profile (UC Davis mouse level2 serogenic profile: Mouse Hepatitis Virus, Sendai, Pneumonia Virus of Mice Reo-3, MPV, Minute Virus of Mice (parvovirus type) NS-1, M.pul and arth, Theiler's Murine Encephalomyelitis Virus part of GDVII strain, Reo-3, Lymphocytic Choriomeningitis Virus, Epidemic Diarrhea of Infant Mice Virus, Mouse Adeno DNA Virus 1 and 2, Mouse Noro Virus). In addition, bacterial pathogens are tested on cecum or nasopharynx and pinworms or fur mites are also routinely checked (Mori et al., 2015). In our case, although specific immune cell activities are not compared, 129: Stat1−/− at UC Davis and Washington University showed identical mammary tumorigenesis phenotypes, especially for T50 in nulliparous and parous dependent and tumor subtypes (Chan et al., 2012; Mori et al., 2017).
Secondly, wild type and KO were necropsied to find causative factors of mammary tumorigenesis and cause of death (COD) especially for evidence associated with aging. The guidelines of for determination for of COD contributing to COD (CCOD) or cause of morbidity are as suggested by The National Center for Toxicological Research (NCTR), which is the important for aging studies (Kodell et al., 1995; Brayton et al., 2012).
Third, syngeneic orthotropic transplantation was used to understand some microenvironmental effects. Since many of GEMM have targeted gene regulation for embryonic stages or hormone dependency or inducible gene expression system multiple cell types could be targeted in these GEMM. In our case, Stat1 gene deletion could affect any Stat1 expressing cells. Therefore, the tissue microenvironment developed abnormally, or the tumor cells generated a unique microenvironment, or gene modification affected the microenvironment in Stat1 GKO. Some the non-epithelial effects can be eliminated using syngeneic orthotopic transplantation. This approach worked very well for the early experiments with younger host. Unfortunately, only young hosts were available for transplantation. Testing hypothesis in aged host mice will have to held for our future experiments. Syngeneic orthotopic transplantation is also useful for studying spatiotemporal factors in aging or cancer research.
Fourth, the understanding of targeted aging or microenvironmental factors in mammary tumorigenesis, can be analyzed applying these techniques. These can provide a broad-context picture of the biology of aging and cancer.
Summary
GEMM have been generated for analyzing functions of a gene in physiopathological events and are often crossed with other GEMM to further investigate context dependent biological phenomena. In breast cancer research, GEMM made for analyzing the gene involvement for mammary tumorigenesis have been used to study tumor progression in mammary glands and metastasis. Although a longer tumor latency (T50 value) suggests involvement of the target molecule in aging-dependent mammary tumorigenesis, few studies have defined how aging and impacts tumor initiation or tumor progression. This lack research effort might be explained by multiple issues: difficulties in separating the aging effect from tumorigenesis; the commitment of time to complete a project; and the cost and the effort required to model aging are all confounding. Tumorigenesis in most GEMM is too rapid to permit aging related comparisons. Complex parameters must be considered to understand entire in vivo biological system. Finally, lack of good GEMM for studying aging and mammary tumorigenesis simultaneously has hindered progress in this area.
129: Stat1−/− is a model for the study of age-related mammary tumorigenesis. The frequency of tumorigenesis, T50 and tumor subtype of the model provides a model that is an age-related ER+ tumor model. The origin and the fate of LOP cells needs to be identified and coupled with immunoediting and microenvironment factors. One envisions that experiments with this model will provide a complex but informative picture of breast cancer. This will be major challenge for investigators including us but will lead to much sounder understanding. Fortunately, these issues are not limited to the 129: Stat1−/− model but also applicable to many other GEMM.
The challenge is to understand the complex biology in each GEMM. It very important to share experimental information, data, and materials in addition to each publication. This will facilitate collaborative research by reducing time, cost and mouse usage and lead to an improved understanding of the biology of cancer in aging.
Sharing GEMM Derived Information and Materials on Aging
Databases available for sharing information:
Genome informatics (MGI: http://www.informatics.jax.org/), Mouse tumor biology database (http://tumor.informatics.jax.org/mtbwi/index.do), European mutant mouse pathology database (http://pathbase.net/), National Cancer Informatics Program (NCIP) hub for a collaboratory for cancer research (https://nciphub.org/). These databases give basic information of registered mouse models. However, each institution's environmental information for maintaining mouse colony and serogenic profile for each GEMM is not well-documented. Available tissue samples with detailed conditions have not been organized. There is an activity of “Sharing Experimental Animal Recourses, Coordinating Holdings,” which suggests sharing the materials from experimental animal models to reduce animal use and accelerate collaborative research. Especially for sharing aging research models, sharing hub website has been developed (www.sharmuk.org). Our website, Center for Genomic Pathology, additionally provides online courses for educating researchers (www.ctrgenpath.org). These are important to further understand each GEMM characteristics by comparing with a certain condition, which facilitate the cancer and aging related research.
Author Contributions
HM and RC: Conceptualized this review article, preparing the manuscript; AB: Preparing the manuscript.
Conflict of Interest Statement
The authors declare that the research was conducted in the absence of any commercial or financial relationships that could be construed as a potential conflict of interest.
Acknowledgments
Our research was supported by grants U01 CA196406 (AB), U01 CA141541, and U01 CA141582 (RC, AB) from the National Cancer Institute's Mouse Models of Human Cancers Consortium.
Supplementary Material
The Supplementary Material for this article can be found online at: https://www.frontiersin.org/articles/10.3389/fcell.2018.00035/full#supplementary-material
Supplementary Data Sheet 1. Excel files are summarizing GEMM with following categories. Page1: a list of MMTV-LTR driven mouse models, page2: a list of Wap-promoter driven mouse models, and page3: a list of GKO and other models. Each mouse model is indicated as Symbol (registered name for mouse model), name (transgene information and registered person's name), background strain, mammary tumor, latency, and estimated T50. A list of GKO has PMID or PMCID as additional information.
References
Abe, F., Younos, I., Westphal, S., Samson, H., Scholar, E., Dafferner, A., et al. (2010). Therapeutic activity of sunitinib for Her2/neu induced mammary cancer in FVB mice. Int. Immunopharmacol. 10, 140–145. doi: 10.1016/j.intimp.2009.09.023
Albrektsen, G., Heuch, I., Hansen, S., and Kvåle, G. (2005). Breast cancer risk by age at birth, time since birth and time intervals between births: exploring interaction effects. Br. J. Cancer 92, 167–175. doi: 10.1038/sj.bjc.6602302
Andrechek, E. R., Cardiff, R. D., Chang, J. T., Gatza, M. L., Acharya, C. R., Potti, A., et al. (2009). Genetic heterogeneity of Myc-induced mammary tumors reflecting diverse phenotypes including metastatic potential. Proc. Natl. Acad. Sci. U.S.A. 106, 16387–16392. doi: 10.1073/pnas.0901250106
Andres, A. C., Schönenberger, C. A., Groner, B., Hennighausen, L., LeMeur, M., and Gerlinger, P. (1987). Ha-ras oncogene expression directed by a milk protein gene promoter: tissue specificity, hormonal regulation, and tumor induction in transgenic mice. Proc. Natl. Acad. Sci. U.S.A. 84, 1299–1303. doi: 10.1073/pnas.84.5.1299
Aprahamian, T., Takemura, Y., Goukassian, D., and Walsh, K. (2008). Ageing is associated with diminished apoptotic cell clearance in vivo. Clin. Exp. Immunol. 152, 448–455. doi: 10.1111/j.1365-2249.2008.03658.x
Bailey, S. G., Cragg, M. S., and Townsend, P. A. (2012). Role of STAT1 in the breast. JAKSTAT 1, 197–199. doi: 10.4161/jkst.20967
Baylis, D., Bartlett, D. B., Patel, H. P., and Roberts, H. C. (2013). Understanding how we age: insights into inflammaging. Longev Healthspan 2:8. doi: 10.1186/2046-2395-2-8
Bentires-Alj, M., and Neel, B. G. (2007). Protein-tyrosine phosphatase 1B is required for HER2/Neu-induced breast cancer. Cancer Res. 67, 2420–2424. doi: 10.1158/0008-5472.CAN-06-4610
Bernardo, G. M., and Keri, R. A. (2012). FOXA1: a transcription factor with parallel functions in development and cancer. Biosci. Rep. 32, 113–130. doi: 10.1042/BSR20110046
Blake, J. A., Eppig, J. T., Kadin, J. A., Richardson, J. E., Smith, C. L., Bult, C. J., et al. (2017). Mouse Genome Database (MGD)-2017: community knowledge resource for the laboratory mouse. Nucleic Acids Res. 45, D723–D729. doi: 10.1093/nar/gkw1040
Borowsky, A. D. (2011). Choosing a mouse model: experimental biology in context–the utility and limitations of mouse models of breast cancer. Cold Spring Harb. Perspect. Biol. 3:a009670. doi: 10.1101/cshperspect.a009670
Borowsky, A. D., Namba, R., Young, L. J., Hunter, K. W., Hodgson, J. G., Tepper, C. G., et al. (2005). Syngeneic mouse mammary carcinoma cell lines: two closely related cell lines with divergent metastatic behavior. Clin. Exp. Metastasis 22, 47–59. doi: 10.1007/s10585-005-2908-5
Bos, P. D., Plitas, G., Rudra, D., Lee, S. Y., and Rudensky, A. Y. (2013). Transient regulatory T cell ablation deters oncogene-driven breast cancer and enhances radiotherapy. J. Exp. Med. 210, 2435–2466. doi: 10.1084/jem.20130762
Brayton, C. F., Treuting, P. M., and Ward, J. M. (2012). Pathobiology of aging mice and GEM: background strains and experimental design. Vet. Pathol. 49, 85–105. doi: 10.1177/0300985811430696
Brew, K., and Nagase, H. (2010). The tissue inhibitors of metalloproteinases (TIMPs): an ancient family with structural and functional diversity. Biochim. Biophys. Acta 1803, 55–71. doi: 10.1016/j.bbamcr.2010.01.003
Brooks, H. L., Pollow, D. P., and Hoyer, P. B. (2016). The VCD Mouse Model of Menopause and perimenopause for the study of sex differences in cardiovascular disease and the metabolic syndrome. Physiology 31, 250–257. doi: 10.1152/physiol.00057.2014
Bult, C. J., Krupke, D. M., Begley, D. A., Richardson, J. E., Neuhauser, S. B., Sundberg, J. P., et al. (2015). Mouse Tumor Biology (MTB): a database of mouse models for human cancer. Nucleic Acids Res 43, D818–D824. doi: 10.1093/nar/gku987
Cardiff, R. D., Jindal, S., Treuting, P. M., Going, J. J., Gusterson, B., and Thompson, H. J. (2018). “23 – Mammary Gland,” in Comparative Anatomy and Histology, 2nd Edn, eds P. M. Treuting, S. M. Dintzis, and K. S. Montine (Cambridge, MA: Academic Press), 487–509.
Cardiff, R. D., Munn, R. J., and Galvez, J. J. (2006). “The tumor pathology of genetically engineered mice: a new approach to molecular pathology,” in The Mouse in Biomedical Research: Experimental Biology and Oncology, 2nd Edn, eds J. G. Fox, M. T. Davisson, F. W. Quimby, S. W. Barthold, C. E. Newcomer, and A. L. Smith (New York, NY: Elsevier, Inc), 581–622.
Cardiff, R. D., Sinn, E., Muller, W., and Leder, P. (1991). Transgenic oncogene mice. Tumor phenotype predicts genotype. Am. J. Pathol. 139, 495–501.
Cardiff, R. D., and Wellings, S. R. (1999). The comparative pathology of human and mouse mammary glands. J. Mammary Gland Biol. Neoplasia 4, 105–122. doi: 10.1023/A:1018712905244
Cardiff, R. D., Young, L. J., and Ashley, R. L. (1976). Hormone synergism in the in vitro production of the mouse mammary tumor virus. J. Toxicol. Environ. Health Suppl. 1, 117–129.
Carlton, W. W., Boitnott, J. K., Dungworth, D. L., Ernst, H., Hayashi, Y., Mohr, U., et al. (2001). Assessment of the morphology and significance of the lymph nodal and hepatic lesions produced in rats by the feeding of certain mineral oils and waxes. Proceedings of a pathology workshop held at the Fraunhofer Institute of Toxicology and Aerosol Research Hannover, Germany, May 7-9, 2001. Exp. Toxicol. Pathol. 53, 247–255. doi: 10.1078/0940-2993-00198
Chan, S. R., Rickert, C. G., Vermi, W., Sheehan, K. C., Arthur, C., Allen, J. A., et al. (2014). Dysregulated STAT1-SOCS1 control of JAK2 promotes mammary luminal progenitor cell survival and drives ERalpha(+) tumorigenesis. Cell Death Differ. 21, 234–246. doi: 10.1038/cdd.2013.116
Chan, S. R., Vermi, W., Luo, J., Lucini, L., Rickert, C., Fowler, A. M., et al. (2012). STAT1-deficient mice spontaneously develop estrogen receptor alpha-positive luminal mammary carcinomas. Breast Cancer Res. 14:R16. doi: 10.1186/bcr3100
Chen, J., Diacovo, T. G., Grenache, D. G., Santoro, S. A., and Zutter, M. M. (2002). The alpha(2) integrin subunit-deficient mouse: a multifaceted phenotype including defects of branching morphogenesis and hemostasis. Am. J. Pathol. 161, 337–344. doi: 10.1016/S0002-9440(10)64185-5
Chen, J. Q., Mori, H., Cardiff, R. D., Trott, J. F., Hovey, R. C., Hubbard, N. E., et al. (2015). Abnormal mammary development in 129:STAT1-null mice is stroma-dependent. PLoS ONE 10:e0129895. doi: 10.1371/journal.pone.0129895
Chen, S., Liu, H., Su, N., Zhang, G., and Wang, L. (2015). Myeloid-derived suppressor cells promote age-related increase of lung cancer growth via B7-H1. Exp. Gerontol. 61, 84–91. doi: 10.1016/j.exger.2014.12.001
Chepko, G., and Smith, G. H. (1999). Mammary epithelial stem cells: our current understanding. J. Mammary Gland Biol. Neoplasia 4, 35–52. doi: 10.1023/A:1018752519356
Chiu, B. C., Martin, B. E., Stolberg, V. R., and Chensue, S. W. (2013). Cutting edge: central memory CD8 T cells in aged mice are virtual memory cells. J. Immunol. 191, 5793–5796. doi: 10.4049/jimmunol.1302509
Chong, Y., Ikematsu, H., Yamaji, K., Nishimura, M., Nabeshima, S., Kashiwagi, S., et al. (2005). CD27(+) (memory) B cell decrease and apoptosis-resistant CD27(−) (naive) B cell increase in aged humans: implications for age-related peripheral B cell developmental disturbances. Int. Immunol. 17, 383–390. doi: 10.1093/intimm/dxh218
Dabydeen, S. A., and Furth, P. A. (2014). Genetically engineered ERalpha-positive breast cancer mouse models. Endocr. Relat. Cancer 21, R195–R208. doi: 10.1530/ERC-13-0512
Daniel, C. W., Aidells, B. D., Medina, D., and Faulkin, L. J. Jr. (1975). Unlimited division potential of precancerous mouse mammary cells after spontaneous or carcinogen-induced transformation. Fed. Proc. 34, 64–67. doi: 10.1007/978-1-4684-2631-1_13
Daniel, C. W., De Ome, K. B., Young, J. T., Blair, P. B., and Faulkin, L. J. Jr. (1968). The in vivo life span of normal and preneoplastic mouse mammary glands: a serial transplantation study. Proc. Natl. Acad. Sci. U.S.A. 61, 53–60. doi: 10.1073/pnas.61.1.53
Daniel, C. W., Shannon, J. M., and Cunha, G. R. (1983). Transplanted mammary epithelium grows in association with host stroma: aging of serially transplanted mammary gland is intrinsic to epithelial cells. Mech. Ageing Dev. 23, 259–264. doi: 10.1016/0047-6374(83)90026-X
Dankort, D., Maslikowski, B., Warner, N., Kanno, N., Kim, H., Wang, Z., et al. (2001). Grb2 and Shc adapter proteins play distinct roles in Neu (ErbB-2)-induced mammary tumorigenesis: implications for human breast cancer. Mol. Cell. Biol. 21, 1540–1551. doi: 10.1128/MCB.21.5.1540-1551.2001
Davie, S. A., Maglione, J. E., Manner, C. K., Young, D., Cardiff, R. D., MacLeod, C. L., et al. (2007). Effects of FVB/NJ and C57Bl/6J strain backgrounds on mammary tumor phenotype in inducible nitric oxide synthase deficient mice. Transgenic Res. 16, 193–201. doi: 10.1007/s11248-006-9056-9
Decock, J., Hendrickx, W., Thirkettle, S., Gutiérrez-Fernández, A., Robinson, S. D., and Edwards, D. R. (2015). Pleiotropic functions of the tumor- and metastasis-suppressing matrix metalloproteinase-8 in mammary cancer in MMTV-PyMT transgenic mice. Breast Cancer Res. 17:38. doi: 10.1186/s13058-015-0545-8
Deng, X., Li, Q., Hoff, J., Novak, M., Yang, H., Jin, H., et al. (2012). Integrin-associated CD151 drives ErbB2-evoked mammary tumor onset and metastasis. Neoplasia 14, 678–689. doi: 10.1593/neo.12922
Deome, K. B., Faulkin, L. J. Jr., Bern, H. A., and Blair, P. B. (1959). Development of mammary tumors from hyperplastic alveolar nodules transplanted into gland-free mammary fat pads of female C3H mice. Cancer Res. 19, 515–520.
Derksen, P. W., Braumuller, T. M., van der Burg, E., Hornsveld, M., Mesman, E., Wesseling, J., et al. (2011). Mammary-specific inactivation of E-cadherin and p53 impairs functional gland development and leads to pleomorphic invasive lobular carcinoma in mice. Dis. Model. Mech. 4, 347–358. doi: 10.1242/dmm.006395
Derksen, P. W., Liu, X., Saridin, F., van der Gulden, H., Zevenhoven, J., Evers, B., et al. (2006). Somatic inactivation of E-cadherin and p53 in mice leads to metastatic lobular mammary carcinoma through induction of anoikis resistance and angiogenesis. Cancer Cell 10, 437–449. doi: 10.1016/j.ccr.2006.09.013
Donehower, L. A. (1996). The p53-deficient mouse: a model for basic and applied cancer studies. Semin. Cancer Biol. 7, 269–278. doi: 10.1006/scbi.1996.0035
Durbin, J. E., Hackenmiller, R., Simon, M. C., and Levy, D. E. (1996). Targeted disruption of the mouse Stat1 gene results in compromised innate immunity to viral disease. Cell 84, 443–450. doi: 10.1016/S0092-8674(00)81289-1
Eberlein, J., Davenport, B., Nguyen, T., Victorino, F., Haist, K., Jhun, K., et al. (2016). Aging promotes acquisition of naive-like CD8+ memory T cell traits and enhanced functionalities. J. Clin. Invest. 126, 3942–3960. doi: 10.1172/JCI88546
El Andaloussi, S., Mäger, I., Breakefield, X. O., and Wood, M. J. (2013). Extracellular vesicles: biology and emerging therapeutic opportunities. Nat. Rev. Drug Discov. 12, 347–357. doi: 10.1038/nrd3978
Enioutina, E. Y., Bareyan, D., and Daynes, R. A. (2011). A role for immature myeloid cells in immune senescence. J. Immunol. 186, 697–707. doi: 10.4049/jimmunol.1002987
Evers, B., and Jonkers, J. (2006). Mouse models of BRCA1 and BRCA2 deficiency: past lessons, current understanding and future prospects. Oncogene 25, 5885–5897. doi: 10.1038/sj.onc.1209871
Fata, J. E., Mori, H., Ewald, A. J., Zhang, H., Yao, E., Werb, Z., et al. (2007). The MAPK(ERK-1,2) pathway integrates distinct and antagonistic signals from TGFalpha and FGF7 in morphogenesis of mouse mammary epithelium. Dev. Biol. 306, 193–207. doi: 10.1016/j.ydbio.2007.03.013
Finch, C. E., Felicio, L. S., Mobbs, C. V., and Nelson, J. F. (1984). Ovarian and steroidal influences on neuroendocrine aging processes in female rodents. Endocr. Rev. 5, 467–497. doi: 10.1210/edrv-5-4-467
Finger, J. H., Smith, C. M., Hayamizu, T. F., McCright, I. J., Xu, J., Law, M., et al. (2017). The mouse Gene Expression Database (GXD): 2017 update. Nucleic Acids Res. 45, D730–D736. doi: 10.1093/nar/gkw1073
Fleming, H. E., and Paige, C. J. (2001). Pre-B cell receptor signaling mediates selective response to IL-7 at the pro-B to pre-B cell transition via an ERK/MAP kinase-dependent pathway. Immunity 15, 521–531. doi: 10.1016/S1074-7613(01)00216-3
Flores, R. R., Clauson, C. L., Cho, J., Lee, B. C., McGowan, S. J., Baker, D. J., et al. (2017). Expansion of myeloid-derived suppressor cells with aging in the bone marrow of mice through a NF-kappaB-dependent mechanism. Aging Cell 16, 480–487. doi: 10.1111/acel.12571
Flurkey, K., Currer, J. M., and Harrison, D. E. (2007). “The mouse in aging research,” in The Mouse in Biomedical Research, 2nd Edn, ed J. G. Fox (Amsterdam; Boston, MA: Elsevier), 637–672.
Franceschi, C., and Campisi, J. (2014). Chronic inflammation (inflammaging) and its potential contribution to age-associated diseases. J. Gerontol A Biol. Sci. Med. Sci. 69(Suppl.1), S4–S9. doi: 10.1093/gerona/glu057
Franceschi, C., Garagnani, P., Vitale, G., Capri, M., and Salvioli, S. (2017). Inflammaging and ‘Garb-aging’. Trends Endocrinol. Metab. 28, 199–212. doi: 10.1016/j.tem.2016.09.005
Frank, D. A., Robertson, M. J., Bonni, A., Ritz, J., and Greenberg, M. E. (1995). Interleukin 2 signaling involves the phosphorylation of Stat proteins. Proc. Natl. Acad. Sci. U.S.A. 92, 7779–7783. doi: 10.1073/pnas.92.17.7779
Fröhlich, C., Nehammer, C., Albrechtsen, R., Kronqvist, P., Kveiborg, M., Sehara-Fujisawa, A., et al. (2011). ADAM12 produced by tumor cells rather than stromal cells accelerates breast tumor progression. Mol. Cancer Res. 9, 1449–1461. doi: 10.1158/1541-7786.MCR-11-0100
Gallahan, D., Jhappan, C., Robinson, G., Hennighausen, L., Sharp, R., Kordon, E., et al. (1996). Expression of a truncated Int3 gene in developing secretory mammary epithelium specifically retards lobular differentiation resulting in tumorigenesis. Cancer Res. 56, 1775–1785.
García-Zaragoza, E., Pérez-Tavarez, R., Ballester, A., Lafarga, V., Jiménez-Reinoso, A., Ramírez, A., et al. (2012). Intraepithelial paracrine Hedgehog signaling induces the expansion of ciliated cells that express diverse progenitor cell markers in the basal epithelium of the mouse mammary gland. Dev. Biol. 372, 28–44. doi: 10.1016/j.ydbio.2012.09.005
Gardner, A., and Ruffell, B. (2016). Dendritic Cells and Cancer Immunity. Trends Immunol. 37, 855–865. doi: 10.1016/j.it.2016.09.006
Goetz, F., Tzeng, Y. J., Guhl, E., Merker, J., Graessmann, M., and Graessmann, A. (2001). The SV40 small t-antigen prevents mammary gland differentiation and induces breast cancer formation in transgenic mice; truncated large T-antigen molecules harboring the intact p53 and pRb binding region do not have this effect. Oncogene 20, 2325–2332. doi: 10.1038/sj.onc.1204355
Goreczny, G. J., Ouderkirk-Pecone, J. L., Olson, E. C., Krendel, M., and Turner, C. E. (2017). Hic-5 remodeling of the stromal matrix promotes breast tumor progression. Oncogene 36, 2693–2703. doi: 10.1038/onc.2016.422
Gorska, A. E., Joseph, H., Derynck, R., Moses, H. L., and Serra, R. (1998). Dominant-negative interference of the transforming growth factor beta type II receptor in mammary gland epithelium results in alveolar hyperplasia and differentiation in virgin mice. Cell Growth Differ. 9, 229–238.
Griffith, O. L., Chan, S. R., Griffith, M., Krysiak, K., Skidmore, Z. L., Hundal, J., et al. (2016). Truncating prolactin receptor mutations promote tumor growth in murine estrogen receptor-alpha mammary carcinomas. Cell Rep. 17, 249–260. doi: 10.1016/j.celrep.2016.08.076
Grizzle, W. E., Xu, X., Zhang, S., Stockard, C. R., Liu, C., Yu, S., et al. (2007). Age-related increase of tumor susceptibility is associated with myeloid-derived suppressor cell mediated suppression of T cell cytotoxicity in recombinant inbred BXD12 mice. Mech. Ageing Dev. 128, 672–680. doi: 10.1016/j.mad.2007.10.003
Hegde, P. S., Karanikas, V., and Evers, S. (2016). The where, the when, and the how of immune monitoring for cancer immunotherapies in the era of checkpoint inhibition. Clin. Cancer Res. 22, 1865–1874. doi: 10.1158/1078-0432.CCR-15-1507
Hemler, M. E. (2005). Tetraspanin functions and associated microdomains. Nat. Rev. Mol. Cell Biol. 6, 801–811. doi: 10.1038/nrm1736
Huang, B., Pan, P. Y., Li, Q., Sato, A. I., Levy, D. E., Bromberg, J., et al. (2006). Gr-1+CD115+ immature myeloid suppressor cells mediate the development of tumor-induced T regulatory cells and T-cell anergy in tumor-bearing host. Cancer Res. 66, 1123–1131. doi: 10.1158/0008-5472.CAN-05-1299
Hurez, V., Daniel, B. J., Sun, L., Liu, A. J., Ludwig, S. M., Kious, M. J., et al. (2012). Mitigating age-related immune dysfunction heightens the efficacy of tumor immunotherapy in aged mice. Cancer Res. 72, 2089–2099. doi: 10.1158/0008-5472.CAN-11-3019
Hurez, V., Padrón, ÁS., Svatek, R. S., and Curiel, T. J. (2017). Considerations for successful cancer immunotherapy in aged hosts. Clin. Exp. Gerontol. 187, 53–63. doi: 10.1111/cei.12875
Hutchinson, J., Jin, J., Cardiff, R. D., Woodgett, J. R., and Muller, W. J. (2001). Activation of Akt (protein kinase B) in mammary epithelium provides a critical cell survival signal required for tumor progression. Mol. Cell. Biol. 21, 2203–2212. doi: 10.1128/MCB.21.6.2203-2212.2001
Imbert, A., Eelkema, R., Jordan, S., Feiner, H., and Cowin, P. (2001). Delta N89 beta-catenin induces precocious development, differentiation, and neoplasia in mammary gland. J. Cell Biol. 153, 555–568. doi: 10.1083/jcb.153.3.555
Jackson, H. W., Hojilla, C. V., Weiss, A., Sanchez, O. H., Wood, G. A., and Khokha, R. (2015). Timp3 deficient mice show resistance to developing breast cancer. PLoS ONE 10:e0120107. doi: 10.1371/journal.pone.0120107
Jindal, S., Gao, D., Bell, P., Albrektsen, G., Edgerton, S. M., Ambrosone, C. B., et al. (2014). Postpartum breast involution reveals regression of secretory lobules mediated by tissue-remodeling. Breast Cancer Res. 16:R31. doi: 10.1186/bcr3633
Jozwik, K. M., and Carroll, J. S. (2012). Pioneer factors in hormone-dependent cancers. Nat. Rev. Cancer 12, 381–385. doi: 10.1038/nrc3263
Kiaris, H., Politi, K., Grimm, L. M., Szabolcs, M., Fisher, P., Efstratiadis, A., et al. (2004). Modulation of notch signaling elicits signature tumors and inhibits hras1-induced oncogenesis in the mouse mammary epithelium. Am. J. Pathol. 165, 695–705. doi: 10.1016/S0002-9440(10)63333-0
Kim, J. M., Rasmussen, J. P., and Rudensky, A. Y. (2007). Regulatory T cells prevent catastrophic autoimmunity throughout the lifespan of mice. Nat. Immunol. 8, 191–197. doi: 10.1038/ni1428
King, A. M., Keating, P., Prabhu, A., Blomberg, B. B., and Riley, R. L. (2009). NK cells in the CD19- B220+ bone marrow fraction are increased in senescence and reduce E2A and surrogate light chain proteins in B cell precursors. Mech. Ageing Dev. 130, 384–392. doi: 10.1016/j.mad.2009.03.002
Kinzel, B., Pikiolek, M., Orsini, V., Sprunger, J., Isken, A., Zietzling, S., et al. (2014). Functional roles of Lgr4 and Lgr5 in embryonic gut, kidney and skin development in mice. Dev. Biol. 390, 181–190. doi: 10.1016/j.ydbio.2014.03.009
Klover, P. J., Muller, W. J., Robinson, G. W., Pfeiffer, R. M., Yamaji, D., and Hennighausen, L. (2010). Loss of STAT1 from mouse mammary epithelium results in an increased Neu-induced tumor burden. Neoplasia 12, 899–905. doi: 10.1593/neo.10716
Kodell, R. L., Blackwell, B. N., Bucci, T. J., and Greenman, D. L. (1995). Cause-of-death assignment at the national center for toxicological research. Toxicol. Pathol. 23, 241–247. doi: 10.1177/019262339502300301
Koren, S., Reavie, L., Couto, J. P., De Silva, D., Stadler, M. B., Roloff, T., et al. (2015). PIK3CA(H1047R) induces multipotency and multi-lineage mammary tumours. Nature 525, 114–118. doi: 10.1038/nature14669
Krneta, T., Gillgrass, A., Chew, M., and Ashkar, A. A. (2016). The breast tumor microenvironment alters the phenotype and function of natural killer cells. Cell. Mol. Immunol. 13, 628–639. doi: 10.1038/cmi.2015.42
Kuperwasser, C., Hurlbut, G. D., Kittrell, F. S., Dickinson, E. S., Laucirica, R., Medina, D., et al. (2000). Development of spontaneous mammary tumors in BALB/c p53 heterozygous mice. A model for Li-Fraumeni syndrome. Am. J. Pathol. 157, 2151–2159. doi: 10.1016/S0002-9440(10)64853-5
Kusmartsev, S., Nagaraj, S., and Gabrilovich, D. I. (2005). Tumor-associated CD8+ T cell tolerance induced by bone marrow-derived immature myeloid cells. J. Immunol. 175, 4583–4592. doi: 10.4049/jimmunol.175.7.4583
Lee, C. K., Rao, D. T., Gertner, R., Gimeno, R., Frey, A. B., and Levy, D. E. (2000). Distinct requirements for IFNs and STAT1 in NK cell function. J. Immunol. 165, 3571–3577. doi: 10.4049/jimmunol.165.7.3571
Lee, D. C., Ruiz, C. R., Lebson, L., Selenica, M. L., Rizer, J., Hunt, J. B. Jr., et al. (2013). Aging enhances classical activation but mitigates alternative activation in the central nervous system. Neurobiol. Aging 34, 1610–1620. doi: 10.1016/j.neurobiolaging.2012.12.014
Lifsted, T., Le Voyer, T., Williams, M., Muller, W., Klein-Szanto, A., Buetow, K. H., et al. (1998). Identification of inbred mouse strains harboring genetic modifiers of mammary tumor age of onset and metastatic progression. Int. J. Cancer 77, 640–644.
Linton, P. J., and Dorshkind, K. (2004). Age-related changes in lymphocyte development and function. Nat. Immunol. 5, 133–139. doi: 10.1038/ni1033
Liu, B., Edgerton, S., Yang, X., Kim, A., Ordonez-Ercan, D., Mason, T., et al. (2005). Low-dose dietary phytoestrogen abrogates tamoxifen-associated mammary tumor prevention. Cancer Res. 65, 879–886. Available online at: http://cancerres.aacrjournals.org/content/65/3/879.long
Liu, C. H., Chang, S. H., Narko, K., Trifan, O. C., Wu, M. T., Smith, E., et al. (2001). Overexpression of cyclooxygenase-2 is sufficient to induce tumorigenesis in transgenic mice. J. Biol. Chem. 276, 18563–18569. doi: 10.1074/jbc.M010787200
Liu, Y., Zhao, Y., Skerry, B., Wang, X., Colin-Cassin, C., Radisky, D. C., et al. (2016). Foxa1 is essential for mammary duct formation. Genesis 54, 277–285. doi: 10.1002/dvg.22929
Liyanage, U. K., Moore, T. T., Joo, H. G., Tanaka, Y., Herrmann, V., Doherty, G., et al. (2002). Prevalence of regulatory T cells is increased in peripheral blood and tumor microenvironment of patients with pancreas or breast adenocarcinoma. J. Immunol. 169, 2756–2761. doi: 10.4049/jimmunol.169.5.2756
Lujambio, A., Akkari, L., Simon, J., Grace, D., Tschaharganeh, D. F., Bolden, J. E., et al. (2013). Non-cell-autonomous tumor suppression by p53. Cell 153, 449–460. doi: 10.1016/j.cell.2013.03.020
MacMahon, B., Cole, P., Lin, T. M., Lowe, C. R., Mirra, A. P., Ravnihar, B., et al. (1970). Age at first birth and breast cancer risk. Bull. World Health Organ. 43, 209–221.
Maglic, D., Stovall, D. B., Cline, J. M., Fry, E. A., Mallakin, A., Taneja, P., et al. (2015). DMP1beta, a splice isoform of the tumour suppressor DMP1 locus, induces proliferation and progression of breast cancer. J. Pathol. 236, 90–102. doi: 10.1002/path.4504
Maglione, J. E., McGoldrick, E. T., Young, L. J., Namba, R., Gregg, J. P., Liu, L., et al. (2004). Polyomavirus middle T-induced mammary intraepithelial neoplasia outgrowths: single origin, divergent evolution, and multiple outcomes. Mol. Cancer Ther. 3, 941–953. Available online at: http://mct.aacrjournals.org/content/3/8/941.long
Mamessier, E., Pradel, L. C., Thibult, M. L., Drevet, C., Zouine, A., Jacquemier, J., et al. (2013). Peripheral blood NK cells from breast cancer patients are tumor-induced composite subsets. J. Immunol. 190, 2424–2436. doi: 10.4049/jimmunol.1200140
Marvel, D., and Gabrilovich, D. I. (2015). Myeloid-derived suppressor cells in the tumor microenvironment: expect the unexpected. J. Clin. Invest. 125, 3356–3364. doi: 10.1172/JCI80005
Meier-Abt, F., and Bentires-Alj, M. (2014). How pregnancy at early age protects against breast cancer. Trends Mol. Med. 20, 143–153. doi: 10.1016/j.molmed.2013.11.002
Meraz, M. A., White, J. M., Sheehan, K. C., Bach, E. A., Rodig, S. J., Dighe, A. S., et al. (1996). Targeted disruption of the Stat1 gene in mice reveals unexpected physiologic specificity in the JAK-STAT signaling pathway. Cell 84, 431–442. doi: 10.1016/S0092-8674(00)81288-X
Mittal, D., Gubin, M. M., Schreiber, R. D., and Smyth, M. J. (2014). New insights into cancer immunoediting and its three component phases–elimination, equilibrium and escape. Curr. Opin. Immunol. 27, 16–25. doi: 10.1016/j.coi.2014.01.004
Moreau, J. M., Mielnik, M., Berger, A., Furlonger, C., and Paige, C. J. (2016). Tumor-secreted products repress B-cell lymphopoiesis in a murine model of breast cancer. Eur. J. Immunol. 46, 2835–2841. doi: 10.1002/eji.201646552
Mori, H., Chen, J. Q., Cardiff, R. D., Pénzváltó, Z., Hubbard, N. E., Schuetter, L., et al. (2017). Pathobiology of the 129:Stat1 (−/−) mouse model of human age-related ER-positive breast cancer with an immune infiltrate-excluded phenotype. Breast Cancer Res. 19:102. doi: 10.1186/s13058-017-0892-8
Mori, H., Lo, A. T., Inman, J. L., Alcaraz, J., Ghajar, C. M., Mott, J. D., et al. (2013). Transmembrane/cytoplasmic, rather than catalytic, domains of Mmp14 signal to MAPK activation and mammary branching morphogenesis via binding to integrin beta1. Development 140, 343–352. doi: 10.1242/dev.084236
Mori, H., Soonsawad, P., Schuetter, L., Chen, Q., Hubbard, N. E., Cardiff, R. D., et al. (2015). Introduction of zinc-salt fixation for effective detection of immune cell-related markers by immunohistochemistry. Toxicol. Pathol. 43, 883–889. doi: 10.1177/0192623315587593
Mukherjee, M., Ge, G., Zhang, N., Edwards, D. G., Sumazin, P., Sharan, S. K., et al. (2014). MMTV-Espl1 transgenic mice develop aneuploid, estrogen receptor alpha (ERalpha)-positive mammary adenocarcinomas. Oncogene 33, 5511–5522. doi: 10.1038/onc.2013.493
Murphy, G. (2011). Tissue inhibitors of metalloproteinases. Genome Biol. 12, 233. doi: 10.1186/gb-2011-12-11-233
Nagase, H., and Woessner, J. F. Jr. (1999). Matrix metalloproteinases. J. Biol. Chem. 274, 21491–21494. doi: 10.1074/jbc.274.31.21491
Namba, R., Maglione, J. E., Young, L. J., Borowsky, A. D., Cardiff, R. D., MacLeod, C. L., et al. (2004). Molecular characterization of the transition to malignancy in a genetically engineered mouse-based model of ductal carcinoma in situ. Mol. Cancer Res. 2, 453–463. Available online at: http://mcr.aacrjournals.org/content/2/8/453.long
Namba, R., Young, L. J., Maglione, J. E., McGoldrick, E. T., Liu, S., Wurz, G. T., et al. (2005). Selective estrogen receptor modulators inhibit growth and progression of premalignant lesions in a mouse model of ductal carcinoma in situ. Breast Cancer Res. 7, R881–R889. doi: 10.1186/bcr1317
Narod, S. A., and Foulkes, W. D. (2004). BRCA1 and BRCA2: 1994 and beyond. Nat. Rev. Cancer 4, 665–676. doi: 10.1038/nrc1431
Nelson, J. F., Felicio, L. S., Randall, P. K., Sims, C., and Finch, C. E. (1982). A longitudinal study of estrous cyclicity in aging C57BL/6J mice: I. Cycle frequency, length and vaginal cytology. Biol Reprod 27, 327–339. doi: 10.1095/biolreprod27.2.327
Nielsen, L. L., Discafani, C. M., Gurnani, M., and Tyler, R. D. (1991). Histopathology of salivary and mammary gland tumors in transgenic mice expressing a human Ha-ras oncogene. Cancer Res. 51, 3762–3767.
Oshima, R. G., Lesperance, J., Munoz, V., Hebbard, L., Ranscht, B., Sharan, N., et al. (2004). Angiogenic acceleration of Neu induced mammary tumor progression and metastasis. Cancer Res. 64, 169–179. doi: 10.1158/0008-5472.CAN-03-1944
Otten, A. D., Sanders, M. M., and McKnight, G. S. (1988). The MMTV LTR promoter is induced by progesterone and dihydrotestosterone but not by estrogen. Mol. Endocrinol. 2, 143–147. doi: 10.1210/mend-2-2-143
Palmer, D. B. (2013). The effect of age on thymic function. Front. Immunol. 4:316. doi: 10.3389/fimmu.2013.00316
Pereira, L. F., de Souza, A. P., Borges, T. J., and Bonorino, C. (2011). Impaired in vivo CD4+ T cell expansion and differentiation in aged mice is not solely due to T cell defects: decreased stimulation by aged dendritic cells. Mech. Ageing Dev. 132, 187–194. doi: 10.1016/j.mad.2011.03.005
Petkova, S. B., Yuan, R., Tsaih, S. W., Schott, W., Roopenian, D. C., and Paigen, B. (2008). Genetic influence on immune phenotype revealed strain-specific variations in peripheral blood lineages. Physiol. Genomics 34, 304–314. doi: 10.1152/physiolgenomics.00185.2007
Pittius, C. W., Sankaran, L., Topper, Y. J., and Hennighausen, L. (1988). Comparison of the regulation of the whey acidic protein gene with that of a hybrid gene containing the whey acidic protein gene promoter in transgenic mice. Mol. Endocrinol. 2, 1027–1032. doi: 10.1210/mend-2-11-1027
Radaelli, E., Arnold, A., Papanikolaou, A., Garcia-Fernandez, R. A., Mattiello, S., Scanziani, E., et al. (2009). Mammary tumor phenotypes in wild-type aging female FVB/N mice with pituitary prolactinomas. Vet. Pathol. 46, 736–745. doi: 10.1354/vp.08-VP-0280-R-FL
Ramirez, N. E., Zhang, Z., Madamanchi, A., Boyd, K. L., O'Rear, L. D., Nashabi, A., et al. (2011). The α2β1 integrin is a metastasis suppressor in mouse models and human cancer. J. Clin. Invest. 121, 226–237. doi: 10.1172/JCI42328
Rao, S. G., and Jackson, J. G. (2016). SASP: tumor suppressor or promoter? Yes! Trends Cancer 2, 676–687. doi: 10.1016/j.trecan.2016.10.001
Raven, J. F., Williams, V., Wang, S., Tremblay, M. L., Muller, W. J., Durbin, J. E., et al. (2011). Stat1 is a suppressor of ErbB2/Neu-mediated cellular transformation and mouse mammary gland tumor formation. Cell Cycle 10, 794–804. doi: 10.4161/cc.10.5.14956
Reiss, K., and Saftig, P. (2009). The “a disintegrin and metalloprotease” (ADAM) family of sheddases: physiological and cellular functions. Semin. Cell Dev. Biol. 20, 126–137. doi: 10.1016/j.semcdb.2008.11.002
Ricciardelli, C., Frewin, K. M., Tan Ide, A., Williams, E. D., Opeskin, K., Pritchard, M. A., et al. (2011). The ADAMTS1 protease gene is required for mammary tumor growth and metastasis. Am. J. Pathol. 179, 3075–3085. doi: 10.1016/j.ajpath.2011.08.021
Riley, R. L., Kruger, M. G., and Elia, J. (1991). B cell precursors are decreased in senescent BALB/c mice, but retain normal mitotic activity in vivo and in vitro. Clin. Immunol. Immunopathol. 59, 301–313. doi: 10.1016/0090-1229(91)90026-7
Rothschild, E., and Banerjee, D. (2015). Subverting subversion: a review on the breast cancer microenvironment and therapeutic opportunities. Breast Cancer 9(Suppl. 2), 7–15. doi: 10.4137/BCBCR.S29423
Rudolph-Owen, L. A., Cannon, P., and Matrisian, L. M. (1998). Overexpression of the matrix metalloproteinase matrilysin results in premature mammary gland differentiation and male infertility. Mol. Biol. Cell 9, 421–435. doi: 10.1091/mbc,.9.2.421
Salvioli, S., Monti, D., Lanzarini, C., Conte, M., Pirazzini, C., Bacalini, M. G., et al. (2013). Immune system, cell senescence, aging and longevity–inflamm-aging reappraised. Curr. Pharm. Des. 19, 1675–1679. doi: 10.2174/1381612811319090015
Sansoni, P., Cossarizza, A., Brianti, V., Fagnoni, F., Snelli, G., Monti, D., et al. (1993). Lymphocyte subsets and natural killer cell activity in healthy old people and centenarians. Blood 82, 2767–2773.
Sato, K., Kato, A., Sekai, M., Hamazaki, Y., and Minato, N. (2017). Physiologic thymic involution underlies age-dependent accumulation of senescence-associated CD4(+) T Cells. J. Immunol. 199, 138–148. doi: 10.4049/jimmunol.1602005
Scarlett, U. K., Rutkowski, M. R., Rauwerdink, A. M., Fields, J., Escovar-Fadul, X., Baird, J., et al. (2012). Ovarian cancer progression is controlled by phenotypic changes in dendritic cells. J. Exp. Med. 209, 495–506. doi: 10.1084/jem.20111413
Schedin, P. (2006). Pregnancy-associated breast cancer and metastasis. Nat. Rev. Cancer 6, 281–291. doi: 10.1038/nrc1839
Schneckenleithner, C., Bago-Horvath, Z., Dolznig, H., Neugebauer, N., Kollmann, K., Kolbe, T., et al. (2011). Putting the brakes on mammary tumorigenesis: loss of STAT1 predisposes to intraepithelial neoplasias. Oncotarget 2, 1043–1054. doi: 10.18632/oncotarget.371
Schoenenberger, C. A., Andres, A. C., Groner, B., van der Valk, M., LeMeur, M., and Gerlinger, P. (1988). Targeted c-myc gene expression in mammary glands of transgenic mice induces mammary tumours with constitutive milk protein gene transcription. EMBO J. 7, 169–175.
Schreiber, R. D., Old, L. J., and Smyth, M. J. (2011). Cancer immunoediting: integrating immunity's roles in cancer suppression and promotion. Science 331, 1565–1570. doi: 10.1126/science.1203486
Seiki, M., Mori, H., Kajita, M., Uekita, T., and Itoh, Y. (2003). Membrane-type 1 matrix metalloproteinase and cell migration. Biochem. Soc. Symp. 70, 253–262. doi: 10.1042/bss0700253
Sharma, S., Dominguez, A. L., and Lustgarten, J. (2006). High accumulation of T regulatory cells prevents the activation of immune responses in aged animals. J. Immunol. 177, 8348–8355. doi: 10.4049/jimmunol.177.12.8348
Shimatani, K., Nakashima, Y., Hattori, M., Hamazaki, Y., and Minato, N. (2009). PD-1+ memory phenotype CD4+ T cells expressing C/EBPα underlie T cell immunodepression in senescence and leukemia. Proc. Natl. Acad. Sci. U.S.A. 106, 15807–15812. doi: 10.1073/pnas.0908805106
Simin, K., Wu, H., Lu, L., Pinkel, D., Albertson, D., Cardiff, R. D., et al. (2004). pRb inactivation in mammary cells reveals common mechanisms for tumor initiation and progression in divergent epithelia. PLoS Biol. 2:E22. doi: 10.1371/journal.pbio.0020022
Smith, C. A., Monaghan, P., and Neville, A. M. (1984). Basal clear cells of the normal human breast. Virchows Arch. A Pathol. Anat. Histopathol. 402, 319–329. doi: 10.1007/BF00695085
Smith, G. H., and Medina, D. (1988). A morphologically distinct candidate for an epithelial stem cell in mouse mammary gland. J. Cell Sci. 90 (Pt 1), 173–183.
Smith, G. H., and Medina, D. (2008). Re-evaluation of mammary stem cell biology based on in vivo transplantation. Breast Cancer Res. 10:203. doi: 10.1186/bcr1856
Song, Y., Shen, H., Du, W., and Goldstein, D. R. (2013). Inhibition of x-box binding protein 1 reduces tunicamycin-induced apoptosis in aged murine macrophages. Aging Cell 12, 794–801. doi: 10.1111/acel.12105
Sprent, J., and Surh, C. D. (2011). Normal T cell homeostasis: the conversion of naive cells into memory-phenotype cells. Nat. Immunol. 12, 478–484. doi: 10.1038/ni.2018
Stepanova, L., Finegold, M., DeMayo, F., Schmidt, E. V., and Harper, J. W. (2000). The oncoprotein kinase chaperone CDC37 functions as an oncogene in mice and collaborates with both c-myc and cyclin D1 in transformation of multiple tissues. Mol. Cell. Biol. 20, 4462–4473. doi: 10.1128/MCB.20.12.4462-4473.2000
Stephan, R. P., Sanders, V. M., and Witte, P. L. (1996). Stage-specific alterations in murine B lymphopoiesis with age. Int. Immunol. 8, 509–518. doi: 10.1093/intimm/8.4.509
Stewart, T. A., Pattengale, P. K., and Leder, P. (1984). Spontaneous mammary adenocarcinomas in transgenic mice that carry and express MTV/myc fusion genes. Cell 38, 627–637. doi: 10.1016/0092-8674(84)90257-5
Stirling, J. W., and Chandler, J. A. (1976). The fine structure of the normal, resting terminal ductal-lobular unit of the female breast. Virchows Arch. A Pathol. Anat. Histol. 372, 205–226. doi: 10.1007/BF00433280
Stirling, J. W., and Chandler, J. A. (1977). The fine structure of ducts and subareolar ducts in the resting gland of the female breast. Virchows Arch. A Pathol. Anat. Histol. 373, 119–132. doi: 10.1007/BF00432157
Sun, Y., Strizzi, L., Raafat, A., Hirota, M., Bianco, C., Feigenbaum, L., et al. (2005). Overexpression of human Cripto-1 in transgenic mice delays mammary gland development and differentiation and induces mammary tumorigenesis. Am. J. Pathol. 167, 585–597. doi: 10.1016/S0002-9440(10)63000-3
Sundberg, J. P., Berndt, A., Sundberg, B. A., Silva, K. A., Kennedy, V., Bronson, R., et al. (2011). The mouse as a model for understanding chronic diseases of aging: the histopathologic basis of aging in inbred mice. Pathobiol Aging Age Relat Dis 1. doi: 10.3402/pba.v1i0.7179
Sympson, C. J., Talhouk, R. S., Alexander, C. M., Chin, J. R., Clift, S. M., Bissell, M. J., et al. (1994). Targeted expression of stromelysin-1 in mammary gland provides evidence for a role of proteinases in branching morphogenesis and the requirement for an intact basement membrane for tissue-specific gene expression. J. Cell Biol. 125, 681–693. doi: 10.1083/jcb.125.3.681
Szabova, L., Chrysovergis, K., Yamada, S. S., and Holmbeck, K. (2008). MT1-MMP is required for efficient tumor dissemination in experimental metastatic disease. Oncogene 27, 3274–3281. doi: 10.1038/sj.onc.1210982
Taddei, I., Deugnier, M. A., Faraldo, M. M., Petit, V., Bouvard, D., Medina, D., et al. (2008). Beta1 integrin deletion from the basal compartment of the mammary epithelium affects stem cells. Nat. Cell Biol. 10, 716–722. doi: 10.1038/ncb1734
Tahir, S., Fukushima, Y., Sakamoto, K., Sato, K., Fujita, H., Inoue, J., et al. (2015). A CD153+CD4+ T follicular cell population with cell-senescence features plays a crucial role in lupus pathogenesis via osteopontin production. J. Immunol. 194, 5725–5735. doi: 10.4049/jimmunol.1500319
Tan, S. Y., Cavanagh, L. L., d'Advigor, W., Shackel, N., Fazekas de St Groth, B., and Weninger, W. (2012). Phenotype and functions of conventional dendritic cells are not compromised in aged mice. Immunol. Cell Biol. 90, 722–732. doi: 10.1038/icb.2011.104
Tarutani, M., Itami, S., Okabe, M., Ikawa, M., Tezuka, T., Yoshikawa, K., et al. (1997). Tissue-specific knockout of the mouse Pig-a gene reveals important roles for GPI-anchored proteins in skin development. Proc. Natl. Acad. Sci. U.S.A. 94, 7400–7405. doi: 10.1073/pnas.94.14.7400
Toker, C. (1967). Observations on the ultrastructure of a mammary ductule. J. Ultrastruct. Res. 21, 9–25. doi: 10.1016/S0022-5320(67)80003-0
Tzeng, Y. J., Guhl, E., Graessmann, M., and Graessmann, A. (1993). Breast cancer formation in transgenic animals induced by the whey acidic protein SV40 T antigen (WAP-SV-T) hybrid gene. Oncogene 8, 1965–1971.
Ursin, G., Bernstein, L., Wang, Y., Lord, S. J., Deapen, D., Liff, J. M., et al. (2004). Reproductive factors and risk of breast carcinoma in a study of white and African-American women. Cancer 101, 353–362. doi: 10.1002/cncr.20373
Ursini-Siegel, J., Hardy, W. R., Zuo, D., Lam, S. H., Sanguin-Gendreau, V., Cardiff, R. D., et al. (2008). ShcA signalling is essential for tumour progression in mouse models of human breast cancer. EMBO J. 27, 910–920. doi: 10.1038/emboj.2008.22
Vasioukhin, V., Degenstein, L., Wise, B., and Fuchs, E. (1999). The magical touch: genome targeting in epidermal stem cells induced by tamoxifen application to mouse skin. Proc. Natl. Acad. Sci. U.S.A. 96, 8551–8556. doi: 10.1073/pnas.96.15.8551
Verschoor, C. P., Johnstone, J., Millar, J., Dorrington, M. G., Habibagahi, M., Lelic, A., et al. (2013). Blood CD33(+)HLA-DR(−) myeloid-derived suppressor cells are increased with age and a history of cancer. J. Leukoc. Biol. 93, 633–637. doi: 10.1189/jlb.0912461
Wagner, K. U., McAllister, K., Ward, T., Davis, B., Wiseman, R., and Hennighausen, L. (2001). Spatial and temporal expression of the Cre gene under the control of the MMTV-LTR in different lines of transgenic mice. Transgenic Res. 10, 545–553. doi: 10.1023/A:1013063514007
Wagner, K. U., Wall, R. J., St-Onge, L., Gruss, P., Wynshaw-Boris, A., Garrett, L., et al. (1997). Cre-mediated gene deletion in the mammary gland. Nucleic Acids Res. 25, 4323–4330. doi: 10.1093/nar/25.21.4323
Wall, E. M., Milne, K., Martin, M. L., Watson, P. H., Theiss, P., and Nelson, B. H. (2007). Spontaneous mammary tumors differ widely in their inherent sensitivity to adoptively transferred T cells. Cancer Res. 67, 6442–6450. doi: 10.1158/0008-5472.CAN-07-0622
Wang, T. C., Cardiff, R. D., Zukerberg, L., Lees, E., Arnold, A., and Schmidt, E. V. (1994). Mammary hyperplasia and carcinoma in MMTV-cyclin D1 transgenic mice. Nature 369, 669–671. doi: 10.1038/369669a0
Wang, X., Zhou, Y. X., Qiao, W., Tominaga, Y., Ouchi, M., Ouchi, T., et al. (2006). Overexpression of aurora kinase A in mouse mammary epithelium induces genetic instability preceding mammary tumor formation. Oncogene 25, 7148–7158. doi: 10.1038/sj.onc.1209707
Webster, M. A., Cardiff, R. D., and Muller, W. J. (1995). Induction of mammary epithelial hyperplasias and mammary tumors in transgenic mice expressing a murine mammary tumor virus/activated c-src fusion gene. Proc. Natl. Acad. Sci. U.S.A. 92, 7849–7853. doi: 10.1073/pnas.92.17.7849
Wolfsberg, T. G., Primakoff, P., Myles, D. G., and White, J. M. (1995). ADAM, a novel family of membrane proteins containing A Disintegrin And Metalloprotease domain: multipotential functions in cell-cell and cell-matrix interactions. J. Cell Biol. 131, 275–278. doi: 10.1083/jcb.131.2.275
Wong, C. P., Magnusson, K. R., and Ho, E. (2010). Aging is associated with altered dendritic cells subset distribution and impaired proinflammatory cytokine production. Exp. Gerontol. 45, 163–169. doi: 10.1016/j.exger.2009.11.005
Xu, X., Wagner, K. U., Larson, D., Weaver, Z., Li, C., Ried, T., et al. (1999). Conditional mutation of Brca1 in mammary epithelial cells results in blunted ductal morphogenesis and tumour formation. Nat. Genet. 22, 37–43. doi: 10.1038/8743
Yang, X., Edgerton, S. M., Kosanke, S. D., Mason, T. L., Alvarez, K. M., Liu, N., et al. (2003). Hormonal and dietary modulation of mammary carcinogenesis in mouse mammary tumor virus-c-erbB-2 transgenic mice. Cancer Res. 63, 2425–2433. Available online at: http://cancerres.aacrjournals.org/content/63/10/2425.long
Young, L. J., Cardiff, R. D., and Ashley, R. L. (1975). Long-term primary culture of mouse mammary tumor cells: production of virus. J. Natl. Cancer Inst. 54, 1215–1221. doi: 10.1093/jnci/54.5.1215
Young, L. J., Medina, D., DeOme, K. B., and Daniel, C. W. (1971). The influence of host and tissue age on life span and growth rate of serially transplanted mouse mammary gland. Exp. Gerontol. 6, 49–56. doi: 10.1016/0531-5565(71)90048-9
Yuan, R., Tsaih, S. W., Petkova, S. B., Marin de Evsikova, C., Xing, S., Marion, M. A., et al. (2009). Aging in inbred strains of mice: study design and interim report on median lifespans and circulating IGF1 levels. Aging Cell 8, 277–287. doi: 10.1111/j.1474-9726.2009.00478.x
Zaret, K. S., and Carroll, J. S. (2011). Pioneer transcription factors: establishing competence for gene expression. Genes Dev. 25, 2227–2241. doi: 10.1101/gad.176826.111
Keywords: genetically engineered mouse models (GEMMs), gene knockout mice, aging, mammary tumorigenesis, mouse strain
Citation: Mori H, Cardiff RD and Borowsky AD (2018) Aging Mouse Models Reveal Complex Tumor-Microenvironment Interactions in Cancer Progression. Front. Cell Dev. Biol. 6:35. doi: 10.3389/fcell.2018.00035
Received: 02 January 2018; Accepted: 15 March 2018;
Published: 29 March 2018.
Edited by:
Mark A. LaBarge, Irell & Manella Graduate School of Biological Sciences, United StatesReviewed by:
A. Emre Sayan, University of Southampton, United KingdomDeyin Xing, School of Medicine, Johns Hopkins University, United States
Copyright © 2018 Mori, Cardiff and Borowsky. This is an open-access article distributed under the terms of the Creative Commons Attribution License (CC BY). The use, distribution or reproduction in other forums is permitted, provided the original author(s) and the copyright owner are credited and that the original publication in this journal is cited, in accordance with accepted academic practice. No use, distribution or reproduction is permitted which does not comply with these terms.
*Correspondence: Hidetoshi Mori, aG1vcmlAdWNkYXZpcy5lZHU=