- 1Shaanxi Key Laboratory of Molecular Biology for Agriculture, College of Animal Science and Technology, Northwest A&F University, Yangling, China
- 2School of Medicine, Sun Yat-sen University, Guangzhou, China
- 3College of Food Science and Engineering, Northwest A&F University, Yangling, China
A new direction for the treatment of skeletal myopathies, which are mainly caused by abnormal mitochondrial metabolism, is the application of drugs and active substances to relieve oxidative stress in mitochondria. Phloretin, a dihydrochalcone active substance widely present in succulent fruits, has attracted attention for its strong antioxidant activity. This study aimed to investigate the potential antioxidant effects of phloretin and its potential mechanism of action in C2C12 mouse myoblasts. Under oxidative stress caused by 500 μmol/L H2O2, the addition of 10 μmol/L phloretin ameliorated the high level of reactive oxygen species, increased CuZn/Mn-dependent superoxide dismutase activities, and restored the loss of mitochondrial membrane potential. Additionally, apoptosis, necrocytosis, and the inhibition of cell proliferation caused by H2O2 stimulation were alleviated by phloretin. Moreover, phloretin significantly increased the expression of cyclin D1 and alleviated the stagnation trend of the G1 phase of cell proliferation caused by H2O2. Furthermore, the addition of phloretin simultaneously significantly increased the protein and mRNA expression of heme oxygenase-1 (HO-1) and alleviated the inhibitory phosphorylation of p-nuclear factor erythroid 2-related factor 2 (Nrf2), p-AMP-activated protein kinase (AMPK), and p-liver kinase B1 (LKB1) induced by H2O2. Moreover, the expression of nuclear Nrf2 was higher with phloretin treatment than without phloretin treatment. Overall, phloretin alleviated the proliferation inhibition and apoptosis induced by H2O2 and exerted antioxidant effects via the LKB1/AMPK/Nrf2/HO-1 pathway in C2C12 cells. These results provide insight for the application of phloretin to alleviate oxidative damage to muscle.
Introduction
Skeletal myopathies, which are common in children and adolescents, are characterized by the inability of the skeletal muscle to tolerate fatigue. Such muscle damage is caused by abnormal energy supply, mainly via abnormal mitochondrial metabolism in muscle cells (Tarnopolsky, 2016). The most common cause of mitochondrial metabolic abnormalities is oxidative damage owing to the excess production of reactive oxygen species (ROS) in the mitochondrial respiratory chain (Brand, 2016). Angiotensin II, the active component of the renin-angiotensin-aldosterone system, increases mitochondrial ROS production in skeletal muscle (Semprun-Prieto et al., 2011; Sukhanov et al., 2011). Oxidative stress in skeletal muscle induces proteolysis and leads to atrophy (Leitner et al., 2017). Therefore, the application of drugs and bioactive compounds to relieve oxidative stress caused by ROS is a new direction for the treatment of muscle diseases (Moulin and Ferreiro, 2017).
Owing to the side effects of drug treatment, more people are currently considering the use of active substances, especially active substances derived from succulent fruits. Phloretin, a naturally occurring flavonoid that belongs to the dihydrochalcone chemical class, is present in juicy fruits, fruit peels, and plant roots, such as those of apple and pear plants (Wang et al., 2014; Li et al., 2018). Recently, various biological activities of phloretin have been reported, including anti-tumor (Wu et al., 2018; Choi, 2019), anti-inflammatory (Alsanea et al., 2017; Wang G. et al., 2018), and anti-oxidation properties (Ren et al., 2016; Yang et al., 2018; Zhang et al., 2019). Phloretin has been reported to remove excess ROS from several types of cells through the redox signaling pathways, such as nuclear factor-κB/mitogen-activated protein kinase (Huang et al., 2015) and liver kinase B1 (LKB1)/AMP-activated protein kinase (AMPK) pathways (Yang et al., 2018), to exert antioxidant activity. However, whether the antioxidant activity of phloretin is exerted in skeletal muscle cells, and can be further used for the treatment of muscle diseases, such as skeletal myopathies, are still unknown.
A typical cell model for muscle development and differentiation research in vitro are C2C12 mouse myoblasts, precursor cells in adult mouse skeletal muscle tissue that rebuild muscle tissue after trauma, with excellent ability for growth and differentiation (Sondag et al., 2014). Therefore, in this study, C2C12 mouse myoblasts and a typical oxidative stress modeling method, hydrogen peroxide stimulation, were used to explore whether phloretin can effectively relieve oxidative damage in skeletal muscle cells. Furthermore, the related mechanism was explored to provide a new theoretical basis for the diet-based treatment of muscle metabolic diseases.
Materials and Methods
Materials
A stock solution of phloretin (analytical grade, purity 98%) was purchased from Yuanye Biotech. Co. (Shanghai, China) and Compound C (MedChemExpress, United States), an inhibitor of AMPK, was prepared in dimethyl sulfoxide (DMSO). In all experiments, DMSO was diluted to less than 0.1% (w/v) by phosphate buffered saline (PBS) and further diluted in growth medium (Yang et al., 2018).
Antibodies, including anti-phospho-AMPKα (Thr172), anti-proliferating cell nuclear antigen (PCNA), anti-Cyclin D1, anti-GAPDH, and goat anti-rabbit and anti-mouse secondary antibody, were purchased from Cell Signaling Technology (Shanghai, China); anti-β-actin, anti-caspase 3, anti-caspase 9, and anti-Keap1 were obtained from Proteintech (Hubei, China); and anti-nuclear factor erythroid 2-related factor 2 (Nrf2), anti-phospho-Nrf2 (Ser40), anti-phospho-LKB1 (Ser428), and anti-heme oxygenase-1 (HO-1) were obtained from Santa Cruz Biotechnology (United States).
Cell Culture and Oxidative Stress Modeling
In this study, the C2C12 mouse myoblast cell line was supplied by the Shanghai Cell Bank of the Chinese Academy of Sciences (Shanghai, China). Based on a previous study, C2C12 cells were cultured in Dulbecco’s modified Eagle’s growth medium containing 10% heat-inactivated fetal bovine serum and 1% penicillin/streptomycin, and maintained in a humidified atmosphere with 5% CO2 at 37°C (Zhang et al., 2018).
A previous study reported that the treatment of C2C12 cells with 200–1,000 μmol/L H2O2 causes oxidative stress in cells (Choi, 2018; Kim and Yi, 2018). Firstly, 500, 750, and 1,000 μmol/L H2O2 were added to C2C12 cells for 1 h in a preliminary experiment to stimulate oxidative stress. Secondly, based on cell morphology observation (Supplementary Figure S1), the final concentration of 500 μmol/L H2O2 was selected for subsequent experiments. Additionally, the 500 μmol/L H2O2 solution was generated as follows: the filtered 30% H2O2 solution was diluted with sterile PBS at a ratio of 1:199 and further diluted in growth medium, until the 500 μmol/L final concentration was achieved.
The four groups in this study were control group (treated with diluted DMSO), H2O2-stimulated group, phloretin-treated group, and 10 μmol/L phloretin and H2O2 co-treatment group.
Determination of ROS Production
C2C12 cells were inoculated into 12-well plates. When the density of cells reached 60–70%, the cells were washed with PBS. The experimental groups for ROS detection were as follows: control group treated with diluted DMSO, H2O2-stimulated group, and 10 μmol/L phloretin treatment followed by co-incubation with 500 μmol/L H2O2 in the final hour group. All treatments lasted for 12 h, after which the medium was discarded and the ROS detection probe 2′-7′dichlorofluorescin diacetate (10 μmol/mL) was added at 37°C. Subsequent processing steps were fully consistent with the instructions of the ROS Assay Kit (Beyotime Biotechnology, Shanghai, China). There were three biological replicates in each group, and each biological replicate was repeated six times.
Cell Counting Kit-8 (CCK8) Assay for Cell Activity and Cell Proliferation
To detect cell activity, C2C12 cells were seeded into 96-well plates. When the density of cells reached 40–60%, the specified dose of phloretin or diluted DMSO was added to the medium. Afterward, the cells in each well were treated according to instructions of CCK8 with WST-8 (Beyotime Biotechnology, Shanghai, China). After incubation for 24 h, cells were washed once with sterile PBS, and 10 μL of CCK8 staining solution and 100 μL of serum-free medium were added to each well. Incubation continued for 2–4 h in the dark, and the absorbance of WST-8, which is directly proportional to the viability of C2C12 cells, was measured at 450 nm.
Measurement of CuZn/Mn-Dependent Superoxide Dismutase (SOD) Activity and Mitochondrial Membrane Potential (MMP)
The CuZn/Mn-SOD activities in C2C12 cells were measured according to the instructions of the CuZn/Mn-SOD Assay Kit (Beyotime Biotechnology, Shanghai, China). The results were normalized with respect to the control group, and each treatment had three independent replicates (Han et al., 2020).
The MMP (ΔΨm) was detected using a fluorescent probe: 5,5′,6,6′-tetrachloro-1,1′,3,3′-tetraethyl benzimidazolyl carbocyanine iodide (JC-1; Beyotime Biotechnology, Shanghai, China). The increase in the number of JC-1 monomers and decrease in the number of J-aggregates, which are always accompanied by mitochondrial depolarization, were detected by a multi-detection microplate reader, following the manufacturer’s instructions.
Annexin-V-Fluorescein Isothiocyanate (FITC) and Propidium Iodide (PI) Double Staining Assay
Based on the instructions of the Annexin V-FITC Apoptosis Detection Kit (Beyotime Biotechnology, Shanghai, China), the cells were harvested, washed with PBS, and resuspended in a 500 μL of 1× binding buffer (5 μL of annexin-V-FITC and 5 μL of PI, Biobox). Subsequently, cells were incubated for 30 min at 20–25°C in the dark and analyzed by flow cytometry (BD FACSAria III, BD Biosciences). The data were analyzed using the FCS Express 5.0 Software (De Novo Software).
Cell Cycle Distribution
The cell cycle analysis method was based on a previous study (Chen et al., 2018). C2C12 cells, which were untreated or treated with 10 μmol/L phloretin for 24 h and stimulated with 500 μmol/L H2O2 for 1 h, were washed with PBS and digested with trypsin. Afterward, cells were centrifuged at 2,000 × g for 5 min and the collected cells were fixed using 1 mL of 70% pre-cooled ethanol diluted with PBS for 1 h at −20°C. After incubation with 50 μg/mL PI (Sigma-Aldrich, St. Louis, MI, United States), cells were analyzed by flow cytometry (BD FACSAria III, BD Biosciences) and the data were analyzed using the FCS Express 5.0 Software (De Novo Software) (Chen et al., 2018).
Isolation of Total RNA and Quantitative RT PCR Analysis
Following the manufacturer’s instructions, total cellular RNA was isolated using TRIzol reagent (Life Technologies, Carlsbad, CA, United States). Then, the cDNA Synthesis Kit (Life Technologies, Shanghai, China) was used for cDNA synthesis from isolated total RNA. Primers for cell proliferation and apoptosis marker genes (Table 1) were used for quantitative RT-PCR (qRT-PCR), and TB Green Master Mix (TaKaRa) and ABI 7300 software (Applied Biosystems, United States) were applied for the quantification of the results. Each treatment group contained three biological replicates, and each biological replicate consisted of three technical replicates. The 2–ΔΔCT method was selected to analyze relative fold changes of candidate genes (Zhang et al., 2016) and β-actin was used for normalization.
Western Blot Analysis
Protein was extracted from harvested cells using radioimmunoprecipitation assay buffer (Beyotime Biotechnology, Shanghai, China) based on a previously established procedure (Chen et al., 2018). After quantification by the BCA Protein Assay Kit (TaKaRa, T9300A), equal amounts of protein (30–50 μg) were separated by 10% sodium dodecyl sulfate-polyacrylamide gel electrophoresis and transferred onto polyvinylidene fluoride membranes (Millipore, Germany). Membranes were blocked with 4% bovine serum albumin and incubated with a specific primary antibody overnight at 4°C and incubated with a secondary antibody for 1 h at room temperature. Bands of proteins were visualized using a chemiluminescence assay system on a ChemiDoc Touch Imaging System (Bio-Rad). Finally, the relative densities of the individual bands were analyzed using the ImageJ software. GAPDH and β-actin were used as loading controls.
Statistical Analysis
All statistical analyses were performed using SPSS 18.0. Statistical analysis was performed using a one-way analysis of variance to compare the means among different groups with normally distributed data (Yang et al., 2018). For data that did not follow a normal distribution, the homogeneity of variances was analyzed using the non-parametric Kruskal–Wallis test (Chen et al., 2018). All data are presented as the mean ± SEM, and P < 0.05 was considered statistically significant.
Results
The Phloretin Treatment Concentration and Oxidative Stress Model Were Established
The results of cell viability assay (Figure 1) and cell morphology observation (Supplementary Figure S1) showed that phloretin had no toxic effects on cells when the concentration is less than 50 μmol/L. However, various concentrations of H2O2, including 500, 750, and 1,000 μmol/L, significantly altered cell morphology and reduced the number of living cells, indicating the success of the oxidative stress modeling (Figure 2 and Supplementary Figure S1A).
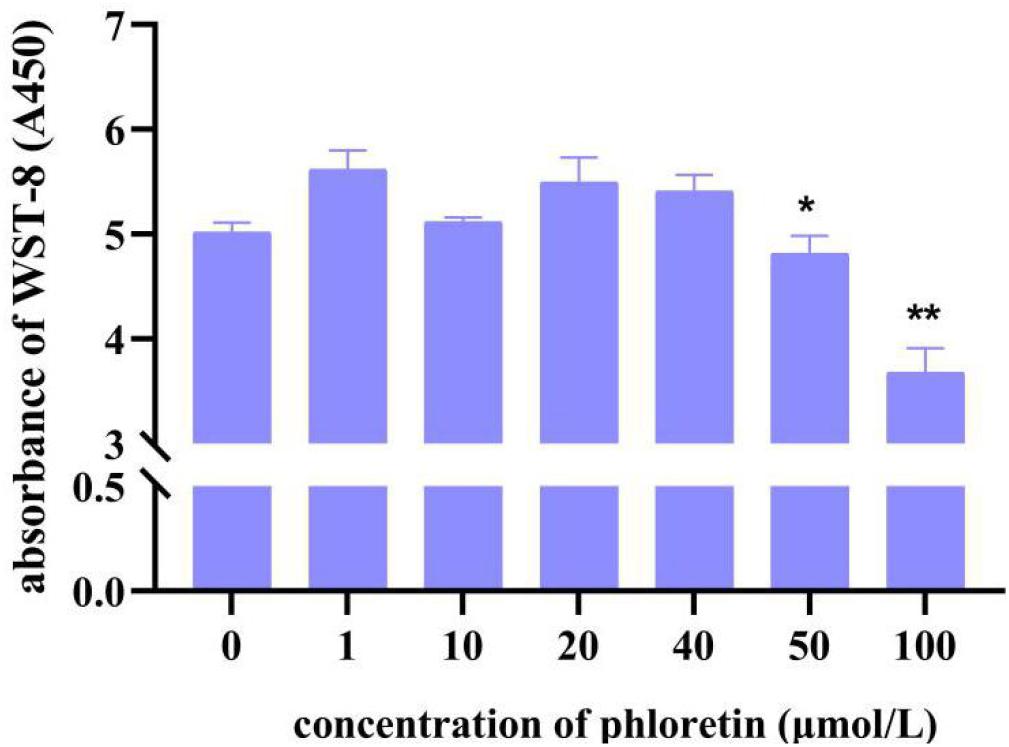
Figure 1. C2C12 cell viability of different concentrations of phloretin using the CCK8 assay. * and ** Mean significant difference refer to P < 0.05 and P < 0.01, respectively.
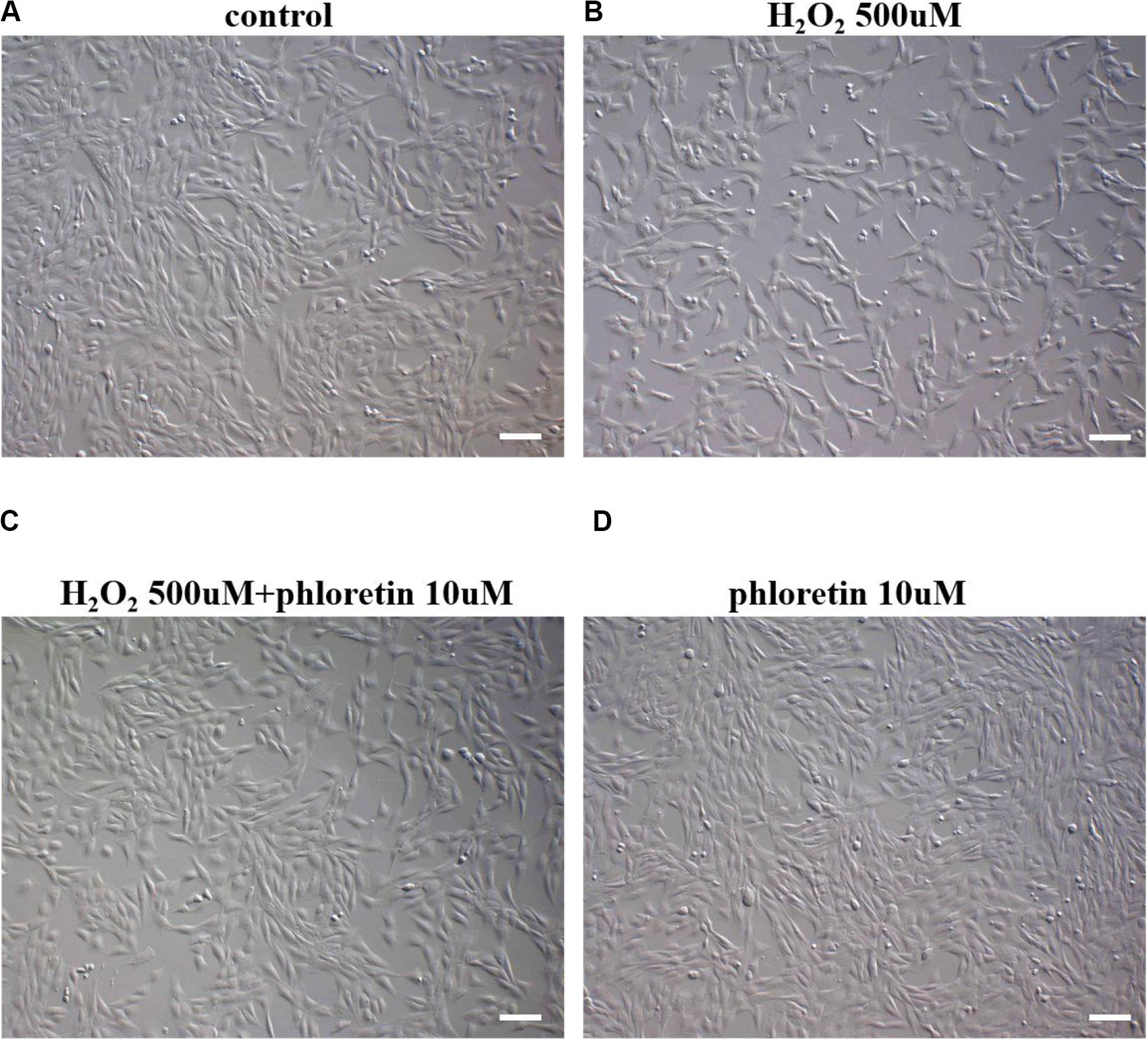
Figure 2. Morphology and numbers of C2C12 cells after supplementation with phloretin or H2O2 in different groups. When the density of cells reached approximately 30%, diluted DMSO was added to the (A) control group and (B) H2O2-stimulated group, whereas 10 μmol/L phloretin was added to the (C) co-induction group and (D) phloretin group for 24 h. For the final 1 h, 500 μmol/L H2O2 was added to (B,C). Scale bar = 100 μm.
The morphology of C2C12 cells treated with three concentrations of phloretin, 10, 20, and 40 μmol/L, and co-treated with 500, 750, and 1,000 μmol/L of H2O2 were observed. When the concentration of hydrogen peroxide was 1000 μmol/L, no concentration of phloretin could restore the morphology of the cells to normal (Supplementary Figure S1B); thus, the H2O2 concentrations of 500 and 750 μmol/L were selected for further experiments.
Based on the CCK8 assay results, in the co-treatment groups, the highest cell viability was detected in 10 μmol/L phloretin among 500 μmol/L H2O2 treated groups (Figure 3A), and 40 μmol/L phloretin among 750 μmol/L H2O2 treated groups (Supplementary Figure S2). Hence, the combination of 10 μmol/L phloretin and 500 μmol/L H2O2 was selected for subsequent experiments, whereas the combination of 40 μmol/L phloretin and 750 μmol/L H2O2, was used in a supplementary group for more pronounced results.
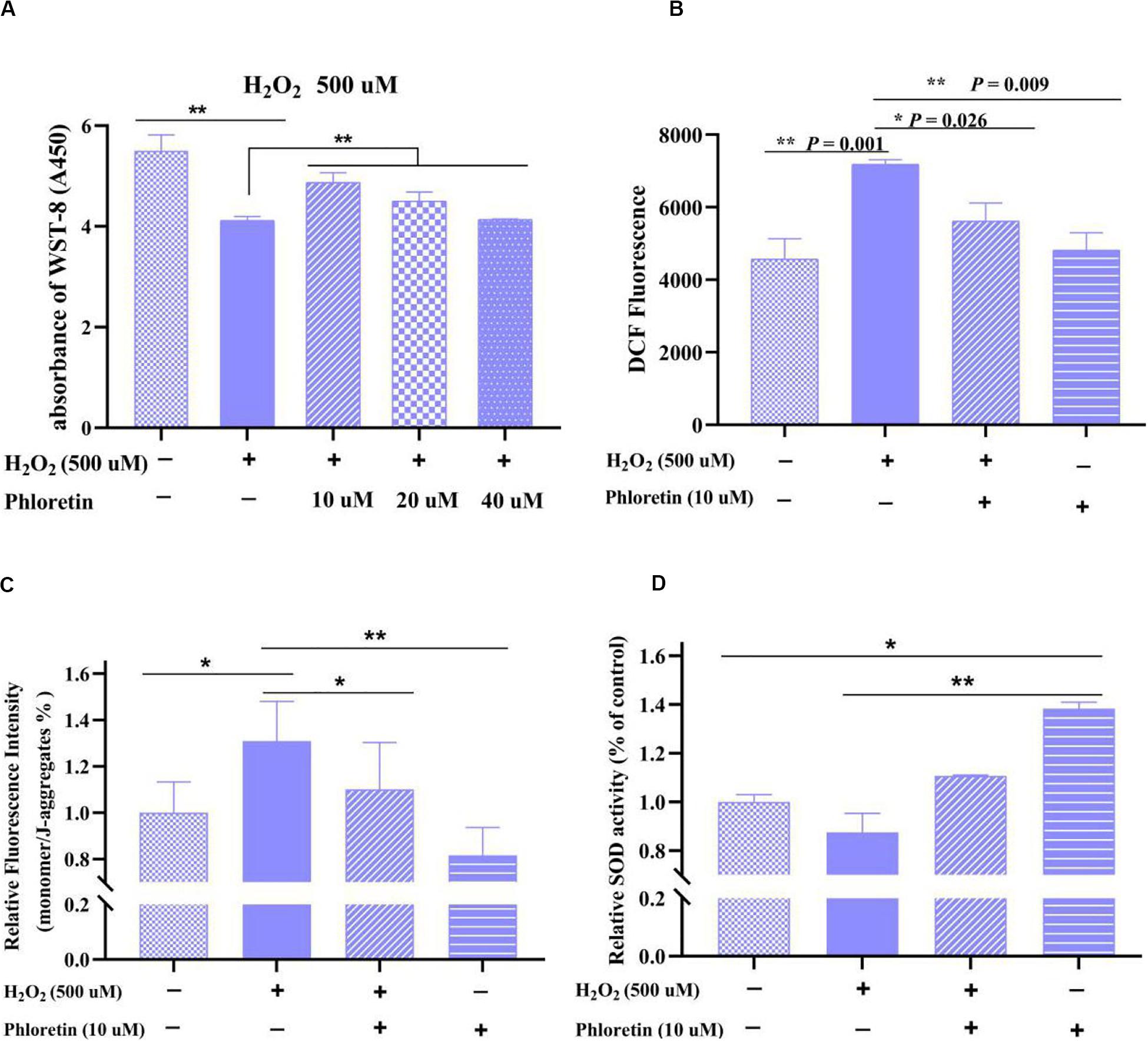
Figure 3. Detection of (A) cell viability, (B) ROS level, (C) mitochondrial membrane potential, and (D) superoxide dismutase activity of C2C12 cells in different groups. ** and * represent great significant difference (P < 0.01) and significant difference (P < 0.05), respectively.
Phloretin Attenuated Oxidative Stress and Restored the MMP Loss in C2C12 Cells
Based on cell morphology observation, the damage to cell morphology by H2O2 was alleviated by phloretin (Figures 2B,C). Furthermore, based on the results of CCK8 and ROS detection, ROS production in the phloretin and H2O2 co-treatment group was significantly lower than those in the H2O2 stimulation group, while trends of cell viability were contrary (Figures 3A,B).
In normal cells, the electron transport chain in mitochondria is one of the main sources of ROS and the excessive generation of ROS by oxidative stress causes the MMP level to drop, which seriously disrupts the function of cells (Brand, 2016). Herein, consistent with these previous findings (Yang et al., 2018), the MMP in H2O2-stimulated cells was significantly lower than that in control. However, this decrease was significantly reversed by 10 μmol/L phloretin preconditioning, which revealed that phloretin restores the loss of MMP caused by excessive ROS in C2C12 cells (Figure 3C).
Phloretin Increased the Activity and Gene Expression of Antioxidant Enzymes
The antioxidant enzymes in the body, such as SOD, play key roles in the removal of excessive ROS to relieve oxidative stress. In this study, co-treatment with phloretin and H2O2 significantly reversed the decrease of CuZn/Mn-dependent SOD enzyme activity caused by H2O2 (Figure 3D). Additionally, the activity of SOD in C2C12 cells treated with phloretin was significantly higher than that in control cells (Figure 3D).
Correspondingly, changes in the transcription levels of the coding genes SOD1 and SOD2 were detected. The trend of SOD1 expression in each group was consistent with that of SOD enzyme activity, but there was no significant difference in SOD1 expression among groups; contrarily, the trend of SOD2 expression was the opposite to that of SOD enzyme activity (Figures 4A,B). This revealed that phloretin does not increase SOD enzyme activity via the promotion of SOD expression.
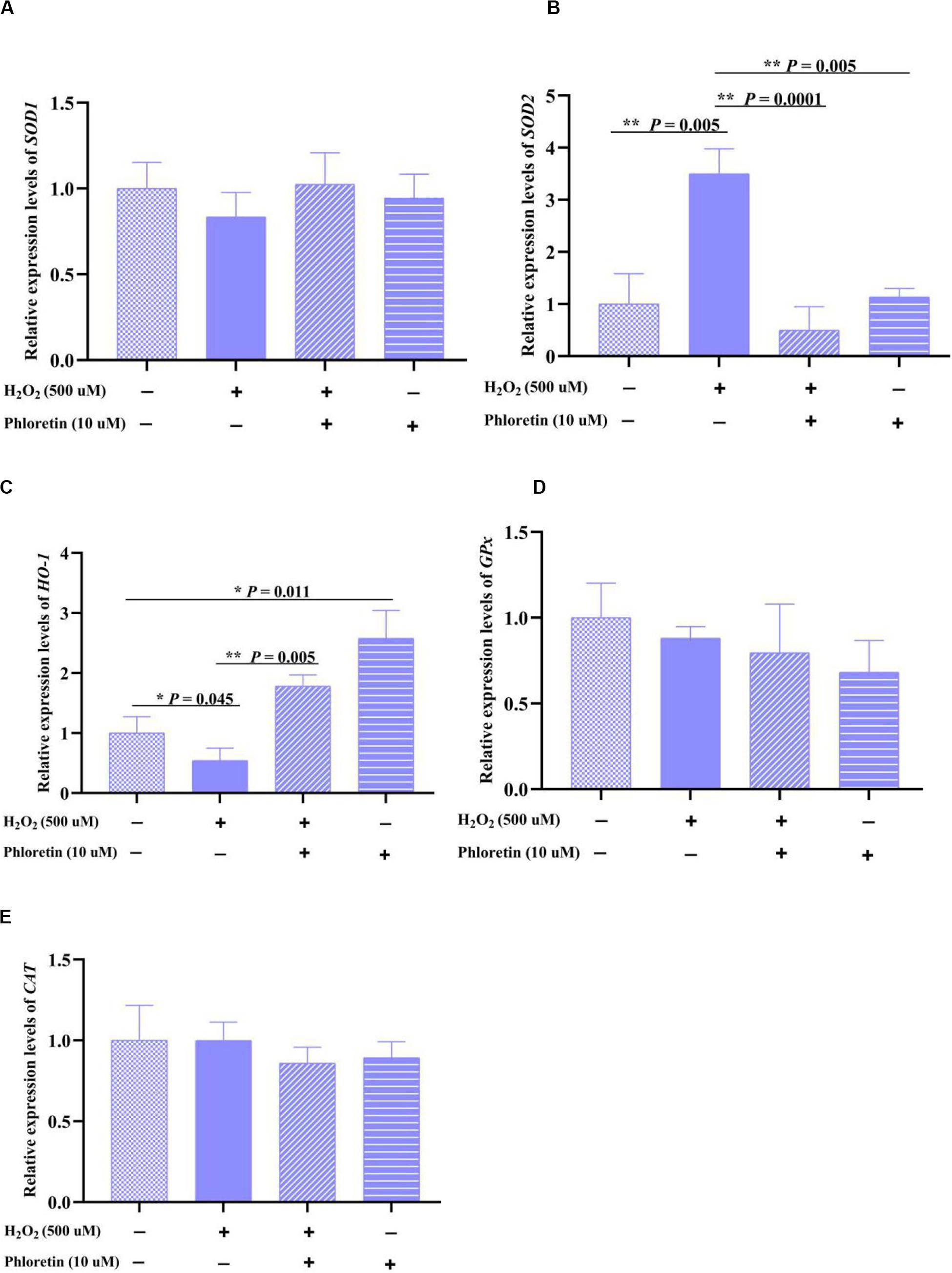
Figure 4. Relative expression levels of oxidation-reduction enzyme-encoding genes, (A) SOD1, (B) SOD2, (C) HO-1, (D) Gpx and (E) CAT in different treatment groups. ** and * represent great significant difference (P < 0.01) and significant difference (P < 0.05), respectively.
The expression of other oxidation-reduction enzyme-encoding genes, GPx, CAT, and HO-1, was also explored. Phloretin significantly alleviated the decrease in HO-1 expression caused by H2O2 stimulation (Figure 4C), whereas it did not significantly affect the expression of GPx and CAT (Figures 4D,E).
Phloretin Alleviated Cell Proliferation Inhibition Under Oxidative Stress
In addition to the attenuation of oxidative stress, in the phloretin and H2O2 co-treatment group, the cell proliferation inhibition caused by H2O2 was alleviated (Figures 2B,C). To uncover the process involved in the alleviation of cell proliferation inhibition by phloretin, cell cycle analysis was performed. After pre-protective treatment with 10 μmol/L phloretin for 24 h, the C2C12 cells were stimulated with 500 μmol/L H2O2 for 1 h, and the cell cycle was analyzed. There was no statistically significant difference in the proportion of cells in the S phase among groups (Figure 5 and Table 2). However, the difference in the proportion of cells in the G1 phase among groups revealed that H2O2 stimulation tended to arrest cell proliferation in the G1 phase, and this arrest was significantly alleviated with the addition of phloretin (Figure 5).
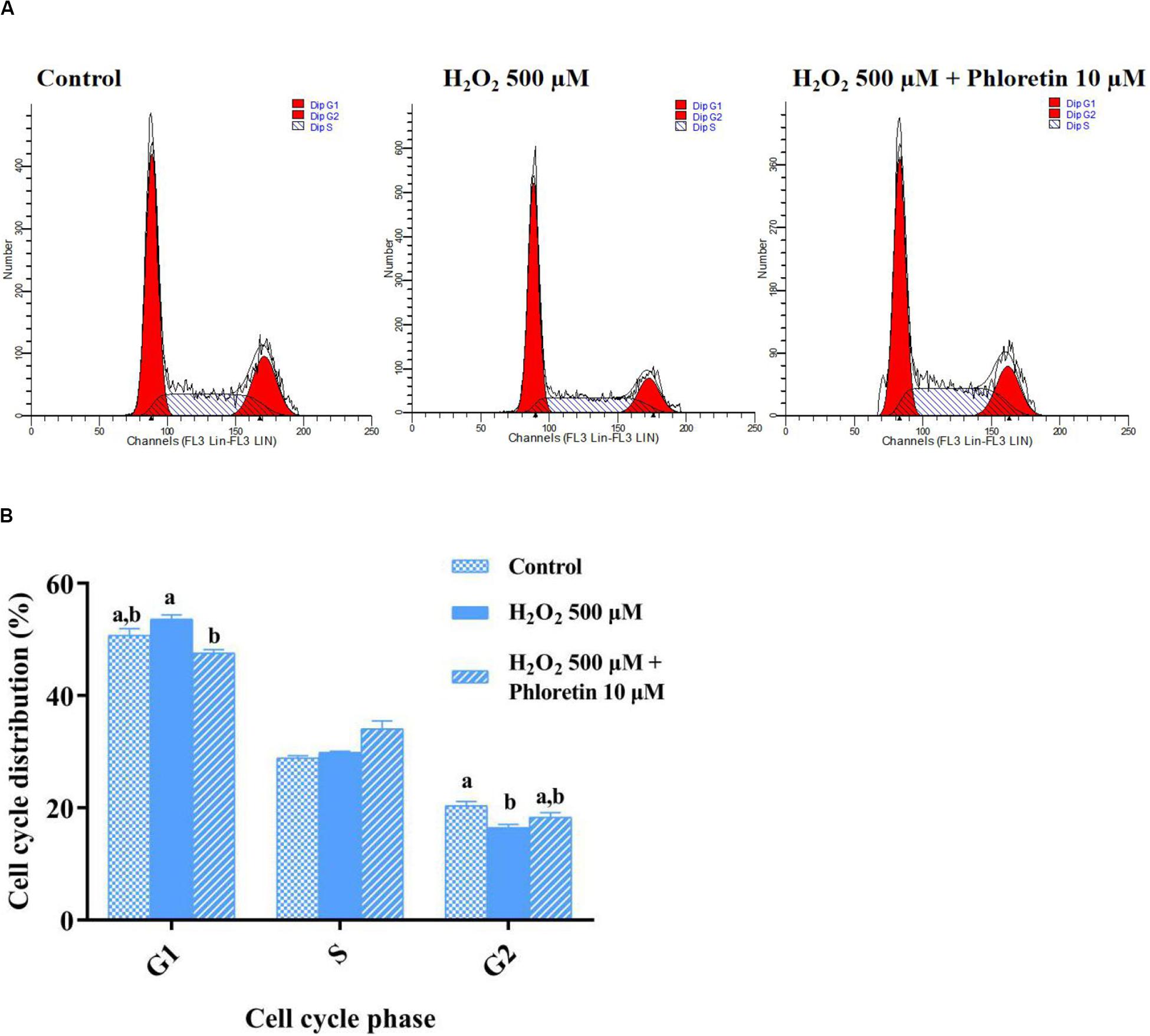
Figure 5. Effect of phloretin on the cell cycle distribution of C2C12 cells. (A) Representative diagram of cell cycle with different treatment. (B) Histogram of each cell cycle in different groups. a and b represent significant difference with P < 0.05.
Phloretin Altered the Expression of Cell Cycle Proteins
To further understand the cell cycle was modulated by phloretin, the expression levels of cell cycle proteins and their coding genes were investigated by western blotting and qRT-PCR, respectively. The protein expressions of cyclin D1 and PCNA were significantly higher in the phloretin and H2O2 co-treatment group than H2O2 stimulation group, which showed that phloretin alleviates proliferation inhibition under oxidative stress (Figure 6B). However, no significant differences were detected among groups in the transcription levels of the genes coding these proteins. As the main inhibitors upstream of cyclinD, the expression levels of P21 and P53 in the phloretin and H2O2 co-treatment group were lower than those in the H2O2 stimulation group, and P53 expressions were significantly different (Figure 6A and Supplementary Figure S3). This indicated that the inhibition of P21 and P53 by phloretin promotes the expression of the cell cycle protein cyclin D1, which plays a key role in the G1 phase.
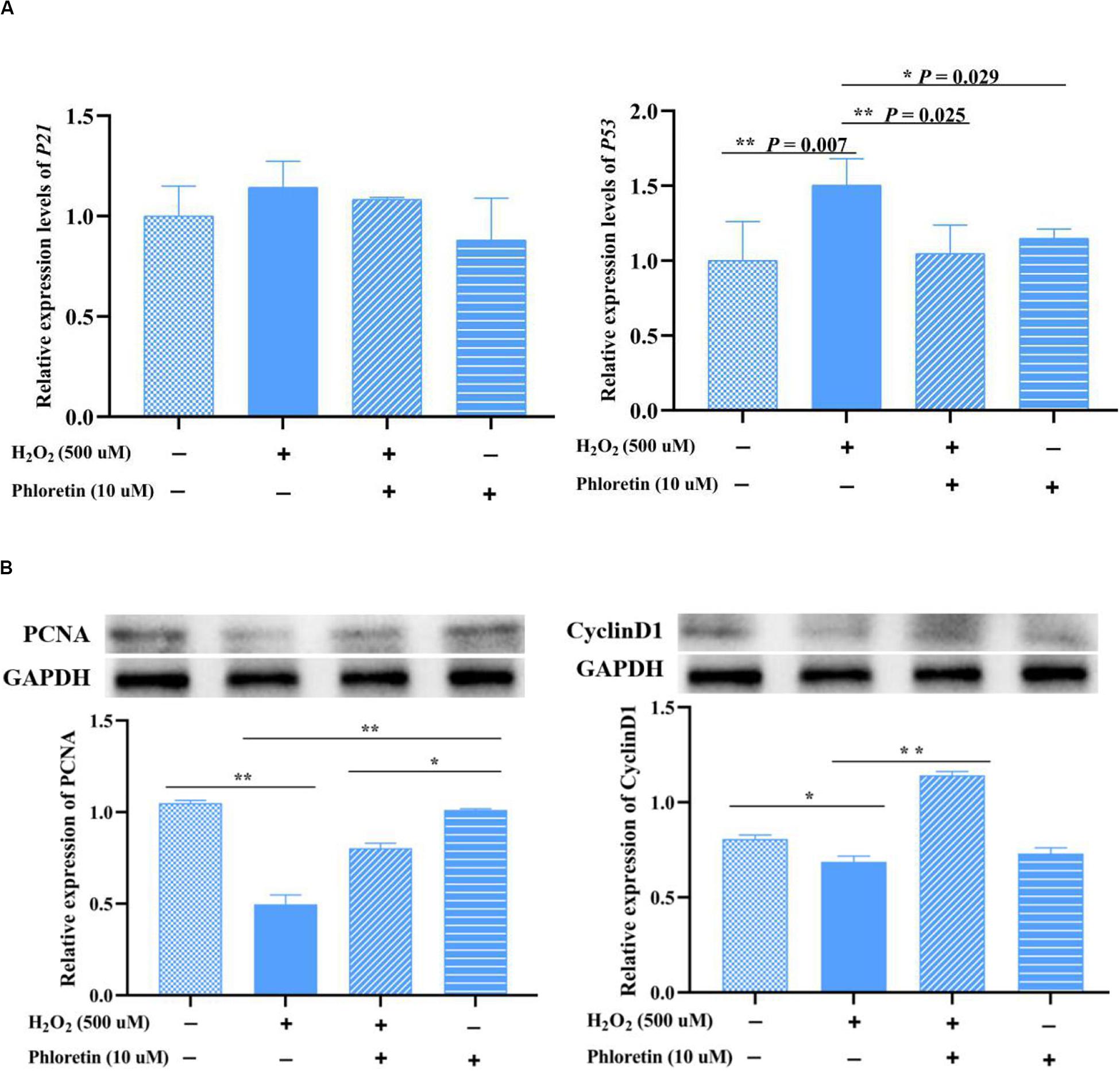
Figure 6. Effect of phloretin and H2O2 on the expression of cell proliferation genes at the transcriptional (A) and translational (B) levels. ** and * represent great significant difference (P < 0.01) and significant difference (P < 0.05), respectively.
Phloretin Reduced the Percentages of H2O2-Induced Apoptotic and Necrotic Cells
As oxidative stress causes apoptosis and necrosis, flow cytometry was used to explore apoptotic and necrotic cells among different groups. The percentages of necrotic and early and late apoptotic cells were markedly higher, whereas the percentage of living cells was significantly lower in the H2O2 stimulation group than in the control group. However, in the phloretin and H2O2 co-treatment group, phloretin significantly alleviated the percentages of apoptotic and necrotic cells (Figure 7).
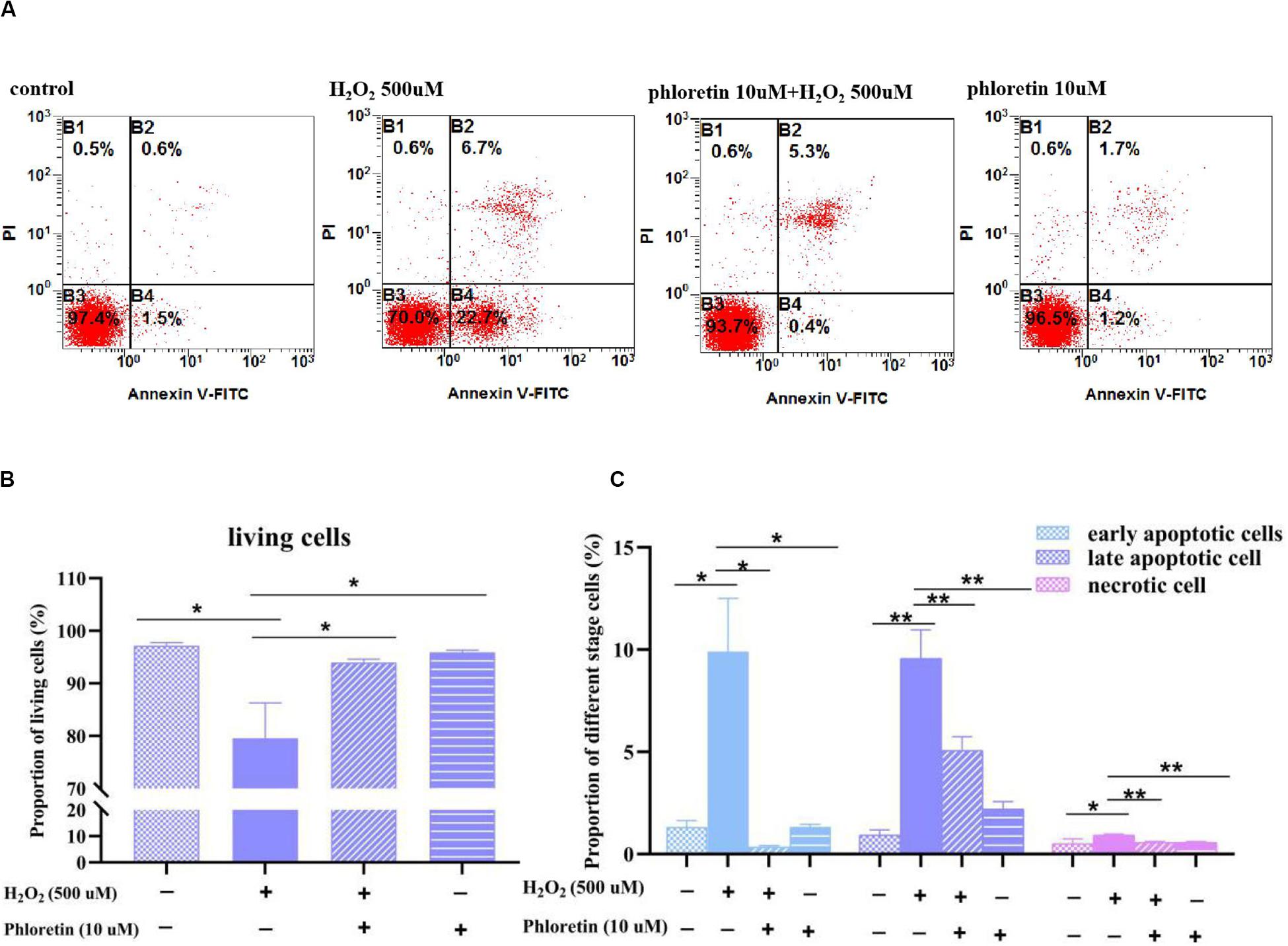
Figure 7. Effect of phloretin and H2O2 on the percentages of apoptotic and necrotic cells. (A) Representative diagram of Annexin-V-FITC&PI with different treatment. Histogram of percentages of (B) living cells, (C) apoptotic and necrotic cells in different groups. * and ** represent significant difference with P < 0.05 and P < 0.01, respectively.
Phloretin Alleviated the H2O2-Induced Expression of Apoptosis-Related Genes and Proteins
To explore the process involved in apoptosis and necrosis due to oxidative stress, the expressions of related genes and proteins were detected. qRT-PCR showed that the expression levels of caspase3 and caspase9 were significantly lower with phloretin and H2O2 co-treatment than H2O2 stimulation (Figure 8A). However, phloretin was not involved in the transcriptional and translational regulation of caspase3, caspase9, and their activated forms (Figure 8B). Nevertheless, the decline in the expression of Bcl-2 induced by H2O2 was alleviated by phloretin (Figure 8B).
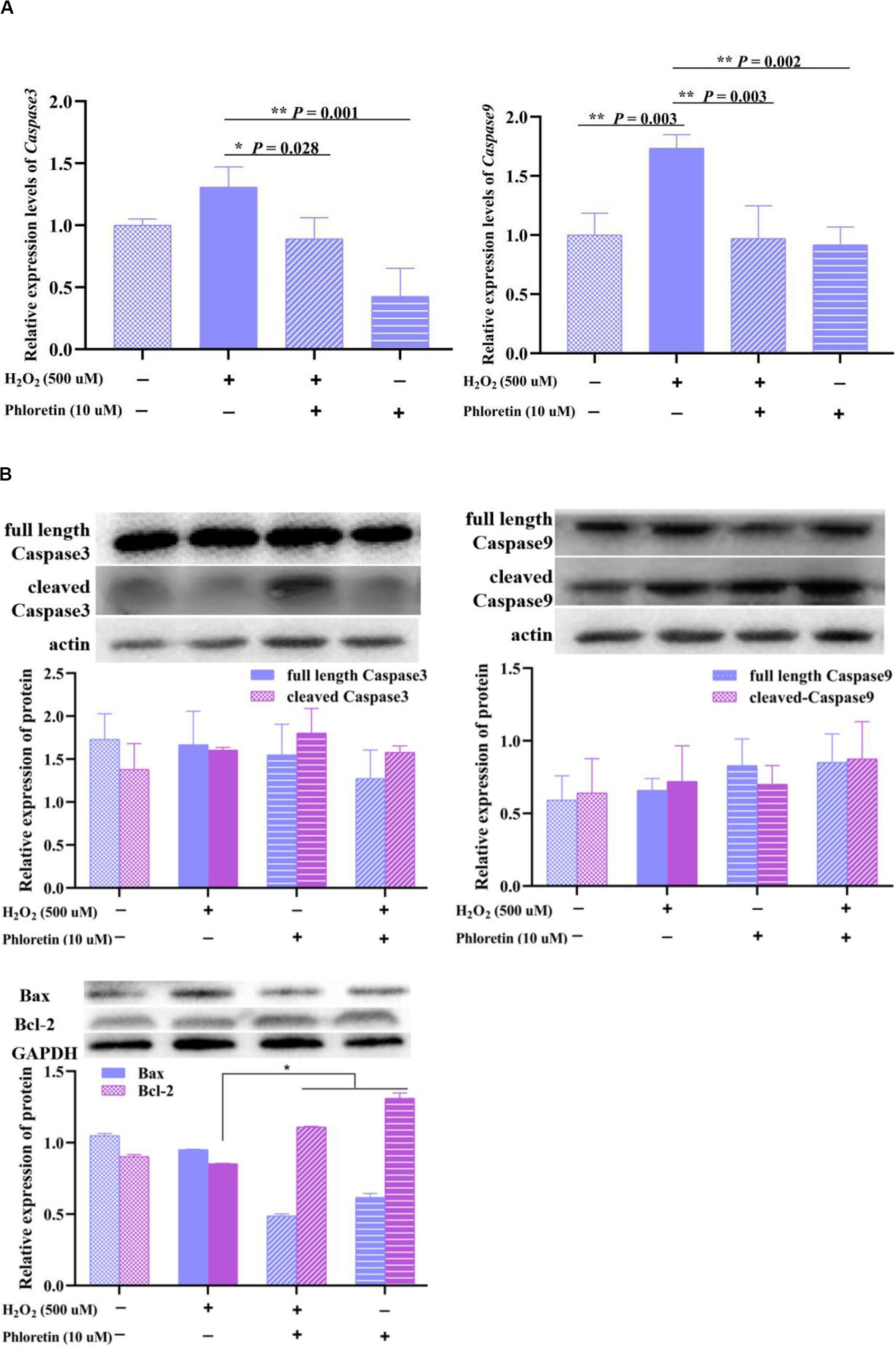
Figure 8. Effect of phloretin and H2O2 on C2C12 apoptosis. (A) q-RT PCR assay, (B) western blot analysis.
These results indicated that phloretin exerts antioxidant functions by the transcriptional and translational regulation of cell proliferation and apoptosis.
Phloretin Exerted Antioxidant Effects via the AMPK/Nrf2/HO-1 Pathway
HO-1, an enzyme with extremely strong antioxidant activity that plays a vital role in the alleviation of increased intercellular ROS levels, was detected in different treatment groups. The expression of HO-1 in the H2O2 stimulation group was significantly lower than that in the control group. In contrast, with the addition of phloretin, the expression of HO-1 was promoted (Figure 9A), consistent with the changes in the transcription level of HO-1 (Figure 4C).
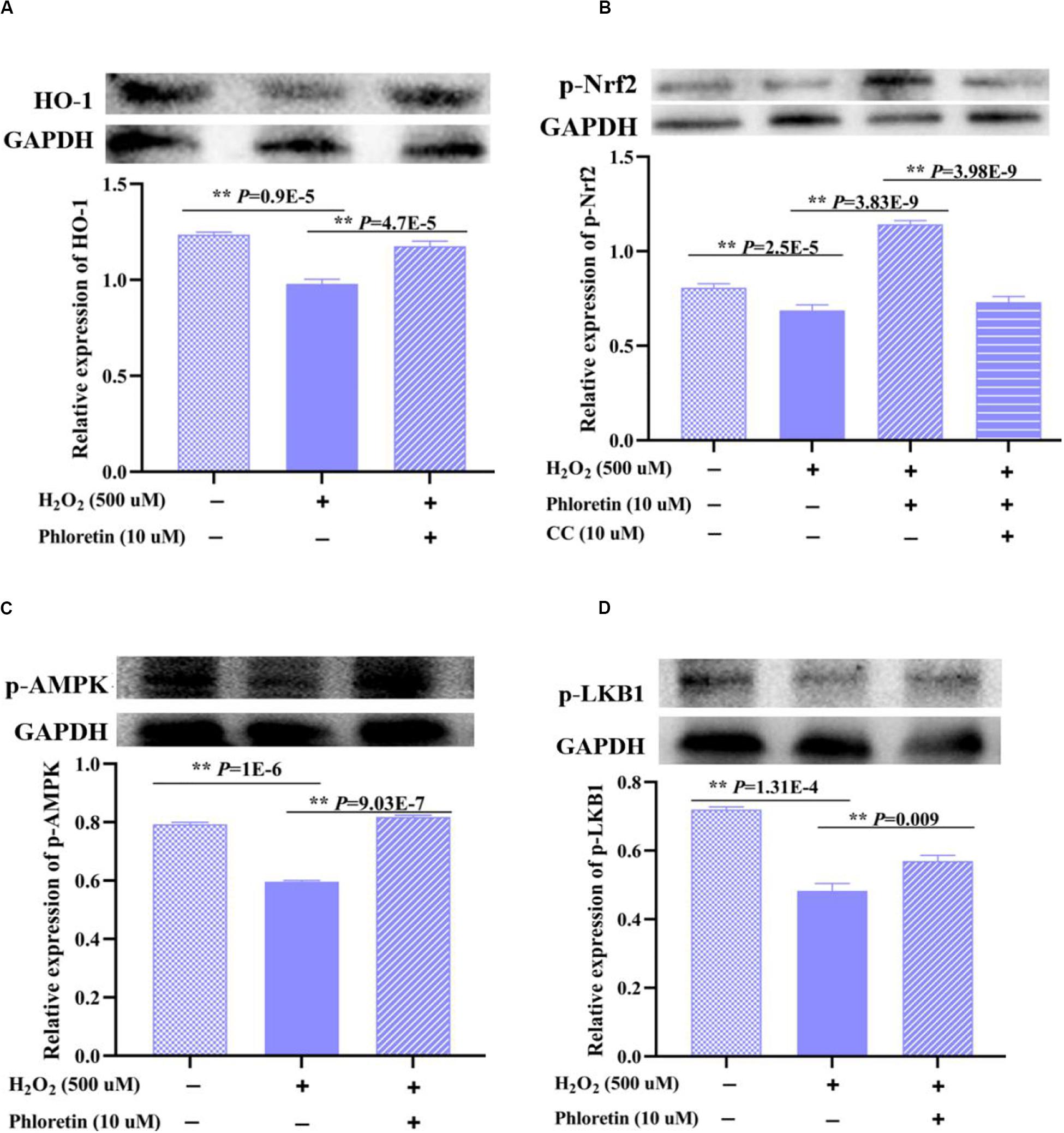
Figure 9. Effect of phloretin on LKB1/AMPK/Nrf2/HO-1 activity in C2C12 cells. Cells at a density of 70% were treated (or not treated) with 10 μmol/L phloretin and 500 μmol/L H2O2. (A) Protein expression of HO-1 and phosphorylation levels of (B) p-Nrf2, (C) p-AMPK, and (D) p-LKB1 were detected. Cells were cultured with compound C (10 μmol/L), an inhibitor of AMPK, for 3 h in (B).
Subsequently, Nrf2, an upstream regulator of HO-1, was examined. The expression trend of the phosphorylated Nrf2 protein was consistent with that of the HO-1 protein among groups (Figure 9B).
There are various upstream regulators of Nrf2, such as AMPK (Nguyen et al., 2015). After treatment with phloretin and the AMPK protein inhibitor compound C, phosphorylated Nrf2 protein was significantly decreased compared to that in the cells treated with phloretin only (Figure 9B). Furthermore, the addition of phloretin significantly alleviated the H2O2-induced inhibition of AMPK phosphorylation (Figure 9C), indicating that phloretin exerts antioxidant effects via the activation of the AMPK/Nrf2/HO-1 pathway.
As a vital regulatory protein of AMPK, the expression trend of p-LKB1 was consistent with that of p-AMPK among groups (Figure 9D).
Phloretin Increased the Expression of Nuclear Nrf2
Nrf2 is sequestered by cytoplasmic Keap1 and targeted for proteasomal degradation under basal conditions; under oxidative stress, Nrf2 detaches from Keap1 and translocates to the nucleus (Bellezza et al., 2018). In this study, although no significant difference existed in total and cytoplasmic Nrf2 and Keap1 proteins expression among groups (Figure 10A). The expression of endonuclear Nrf2 in the H2O2 stimulation group was lower than that in the control group. Additionally, the expression of endonuclear Nrf2 in the phloretin and H2O2 co-treatment group increased significantly compared with H2O2 stimulation group (Figure 10B), which was in accordance with the expression trend of the phosphorylated Nrf2 protein.
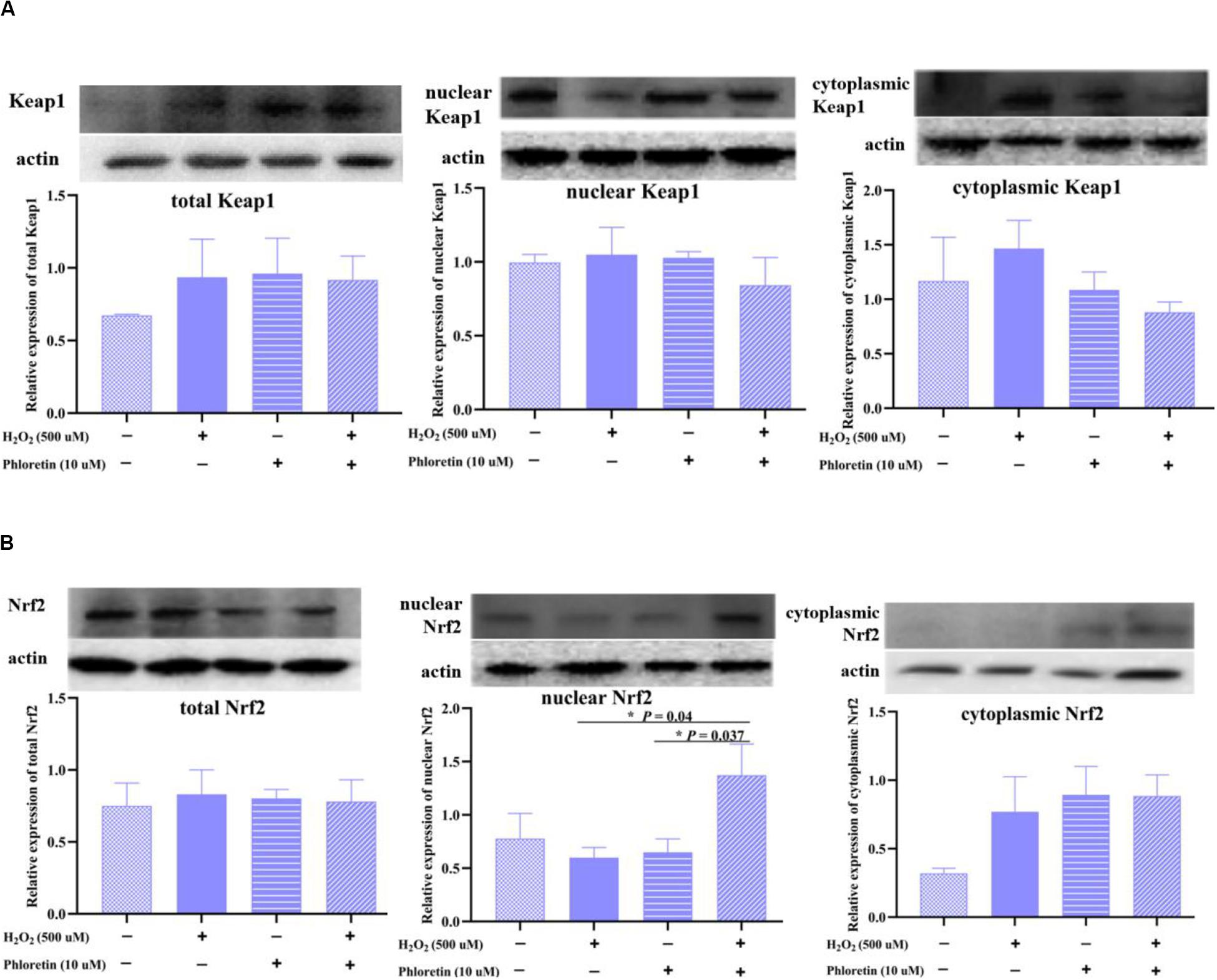
Figure 10. Effect of phloretin on the expression of (A) Keap1 protein and (B) Nrf2 protein in different components of cells. * Mean significant difference (P < 0.05).
The above results indicated that phloretin alleviates the proliferation inhibition and apoptosis of C2C12 cells induced by H2O2 and exerts antioxidant effects via the LKB1/AMPK/Nrf2/HO-1 pathway in C2C12 cells (Figure 11).
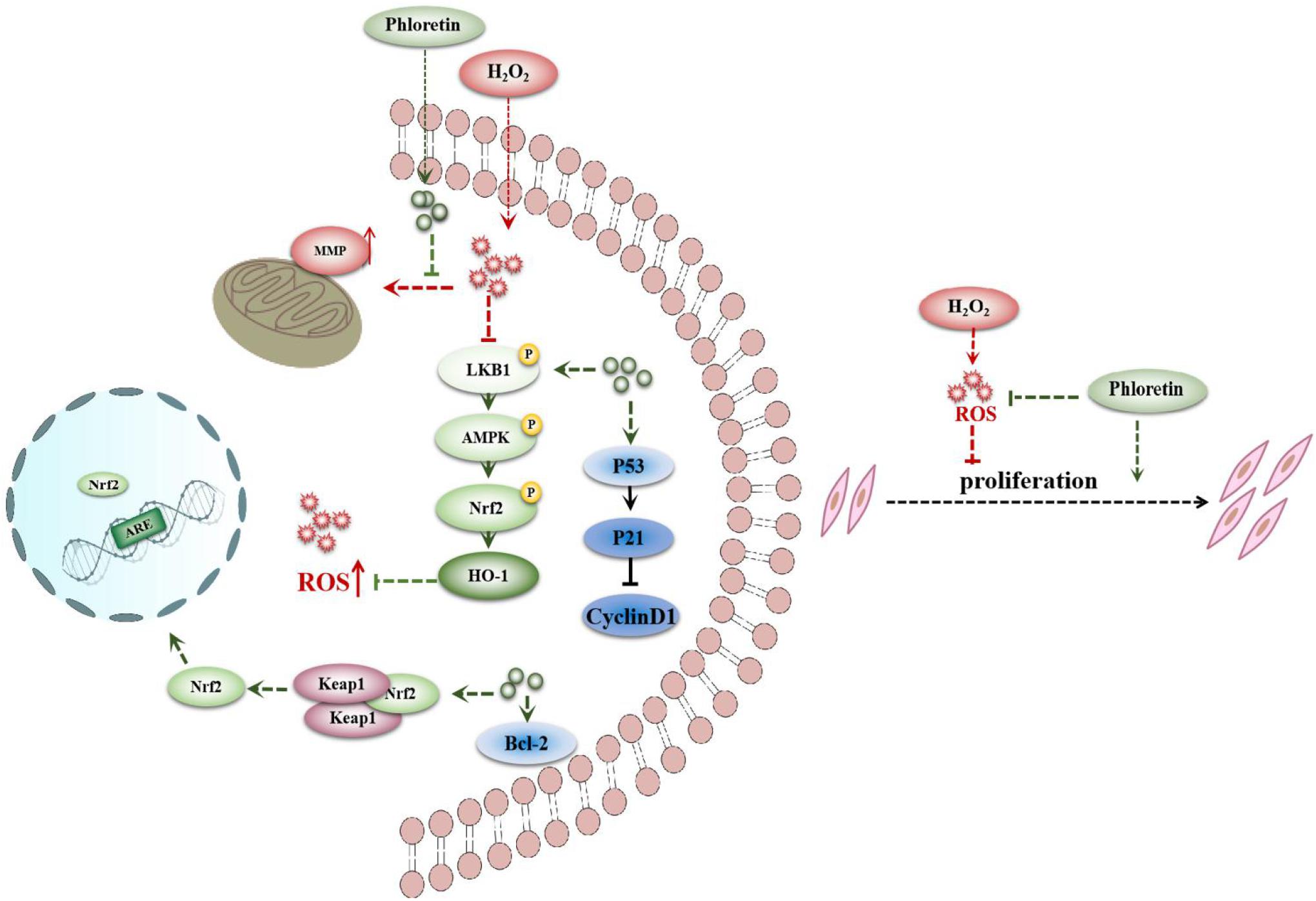
Figure 11. Proposed signaling pathways for phloretin to ameliorate oxidative stress in C2C12 myoblasts.
Discussion
As a chalcone compound, phloretin is present in various succulent fruits. Studies have shown that phloretin relieves oxidative damage in certain types of cells, such as myocardial (Vineetha et al., 2015) and smooth muscle (Wang et al., 2015) cells. To investigate whether its effects are extensive, the effects of phloretin on oxidative stress in skeletal muscle cells was explored. In contrast to the promotion of the activity of caspase 3 in myocardial cells (Vineetha et al., 2015), phloretin was not involved in the expression of caspases in this study. It is speculated that these differences may be caused by different cell types or different phloretin concentrations. Moreover, although phloretin promoted C2C12 cells proliferation in this study, phloretin has been shown to inhibit abnormal cell proliferation in rat aortic smooth muscle cells (Wang et al., 2015). However, consistent with our results, the oxidative stress caused by H2O2 has been shown to inhibit cell proliferation, and phloretin attenuated this inhibition via the promotion of the expression of cell proliferation-associated proteins (Dimozi et al., 2015). Furthermore, phloretin ameliorates mitochondrial dysfunction in cardiomyoblasts (Vineetha et al., 2015). This is consistent with the results of this study and reflects the consistency and breadth of the anti-oxidation effect of phloretin.
Oxidative stress can cause abnormalities in the mitochondrial oxidative respiratory chain, which in turn, can aggravate the occurrence of muscle metabolic diseases. In this study, phloretin restored the loss of MMP and significantly decreased the level of excessive ROS in C2C12 cells, which is beneficial to maintain a steady electron transport chain in mitochondria. Clinically, skeletal myopathies mainly manifest as a progressive aggravation of skeletal muscle atrophy and weakness, with varying degrees and distribution. The current drug treatment for muscle atrophy is mainly focused on improvement of appetite, modulation of inflammation, and interference with anabolic and catabolic reactions using candidate medications, such as thalidomide (Cao et al., 2018). However, no medication has been shown to be effective in clinical trials so far. The use of a phloretin-based therapeutic regimen will likely be safe and reliable for the treatment of muscular atrophy. In skeletal muscle and C2C12 myoblasts, in addition to phloretin, other plant-derived bioactive substances abundant in fruits, such as myricitrin (Ahangarpour et al., 2018) and resveratrol (Haramizu et al., 2017), may exert antioxidant effects. Currently, various studies have linked healthy foods to the alleviation of degenerative diseases (Panche et al., 2016). However, the potential interactions among different antioxidant bioactive substances in a single type of cell or tissue are worth further exploration to provide insight into appropriate fruit and dietary combinations.
Furthermore, here, phloretin inhibited oxidation via the regulation of the LKB1/AMPK/Nrf2/HO-1 pathway. Phloretin has also been identified to exert antioxidant effects via the Nrf2 pathway in other cells, such as human umbilical vein endothelial cells (Yang et al., 2018). Nrf2, a key nuclear transcription factor, is a core regulator in the oxidative stress pathway (Chen and Maltagliati, 2018). Its upstream regulatory protein, AMPK, plays a major role in the maintenance of the intracellular energy balance and regulation of whole-body energy metabolism (Carling, 2017). Hence, the AMPK-Nrf2-dependent pathway is involved in the antioxidant and anti-inflammatory functions of other plant-derived bioactive substances, including quercetin (Wang D. et al., 2018), anthocyanins (Ho et al., 2017), procyanidin (Lu et al., 2018), salvianolic acid C (Song et al., 2018), and fortunellin (Zhao et al., 2017). For instance, phloretin may prevent diabetic cardiomyopathy via the dissociation of the Keap1/Nrf2 complex and inhibition of oxidative stress (Ying et al., 2018). In contrast to these studies, this study revealed a more comprehensive signaling pathway for the role of phloretin in oxidative stress regulation.
Additionally, previous intervention study has examined the effect of fresh apple consumption on oxidative markers in humans. The daily intake of fresh apple at 2 g/kg body weight for 30 days effectively increases the levels of antioxidant enzymes in tested participants (Avci et al., 2007). Therefore, it is speculated that a patient with muscle atrophy can effectively reduce oxidative stress by the ingestion of approximately two normal size apples per day (Hyson, 2011; Tu et al., 2017). For patients who cannot consume apples, the direct intake of pure phloretin products with liquid food or the consumption of freshly squeezed apple juice is recommended. Regardless of the method of intake, the intake of phloretin from succulent fruits undoubtedly provides a healthy and feasible treatment for patients with muscle diseases. However, the antioxidant mechanism of phloretin in skeletal muscle cells needs further validation in vivo.
Conclusion
Phloretin mitigates oxidative damage in C2C12 cells by the alleviation of proliferation inhibition and apoptosis via the AMPK/Nrf2/HO-1 signaling pathway. The results of this study provide a strong scientific basis for the proper application of phloretin to promote the intake of healthy dietary combinations for treating skeletal myopathies.
Data Availability Statement
All datasets generated for this study are included in the article/Supplementary Material.
Author Contributions
JL: writing – original draft and validation. QY and LH: validation. CP: supervision, writing – review and editing. CL and HC: writing – review and editing. XL: supervision and project administration. All authors read and approved the final manuscript.
Funding
This study was supported by the National Natural Science Foundation of China (No. 31872331).
Conflict of Interest
The authors declare that the research was conducted in the absence of any commercial or financial relationships that could be construed as a potential conflict of interest.
Supplementary Material
The Supplementary Material for this article can be found online at: https://www.frontiersin.org/articles/10.3389/fcell.2020.541260/full#supplementary-material
FIGURE S1 | Morphology and numbers of C2C12 cells after supplementation of phloretin and H2O2 with various concentrations in different groups. Scale bar = 100 μm. “∗” Means significant difference with P < 0.05.
FIGURE S2 | Ros level of C2C12 cells under induction with 750 μmol/L H2O2 in different groups. “∗∗” and “∗” represent great significant difference (P < 0.01) and significant difference (P < 0.05), respectively.
FIGURE S3 | The relative expression levels of P21 and P53 genes in different treatment groups (under induction with 750 μmol/L H2O2). “∗∗” and “∗” represent great significant difference (P < 0.01) and significant difference (P < 0.05), respectively.
References
Ahangarpour, A., Oroojan, A. A., Khorsandi, L., Kouchak, M., and Badavi, M. (2018). Antioxidant effect of myricitrin on hyperglycemia-induced oxidative stress in C2C12 cell. Cell Stress Chaperones. 23, 773–781. doi: 10.1007/s12192-018-0888-zwi
Alsanea, S., Gao, M., and Liu, D. (2017). Phloretin prevents high-fat diet-induced obesity and improves metabolic homeostasis. AAPS J. 19, 797–805. doi: 10.1208/s12248-017-0053-0
Avci, A., Atli, T., Ergüder, I. B., Varli, M., Devrim, E., Turgay, S. A., et al. (2007). Effects of apple consumption on plasma and erythrocyte antioxidant parameters in elderly subjects. Exp. Aging. Res. 33, 429–437. doi: 10.1080/03610730701525352
Bellezza, I., Giambanco, I., Minelli, A., and Donato, R. (2018). Nrf2-Keap1 signaling in oxidative and reductive stress. Biochim. Biophys. Acta Mol. Cell Res. 1865, 721–733. doi: 10.1016/j.bbamcr.2018.02.010
Brand, M. D. (2016). Mitochondrial generation of superoxide and hydrogen peroxide as the source of mitochondrial redox signaling. Free Radic. Biol. Med. 100, 14–31. doi: 10.1016/j.freeradbiomed.2016.04.001
Cao, R. Y., Li, J., Dai, Q., Li, Q., and Yang, J. (2018). Muscle atrophy: present and future. Adv. Exp. Med. Biol. 1088, 605–624. doi: 10.1007/978-981-13-1435-3_29
Carling, D. (2017). AMPK signalling in health and disease. Curr. Opin. Cell. Biol. 45, 31–37. doi: 10.1016/j.ceb.2017.01.005
Chen, Q. M., and Maltagliati, A. J. (2018). Nrf2 at the heart of oxidative stress and cardiac protection. Physiol. Genomics 50, 77–97. doi: 10.1152/physiolgenomics.00041.2017
Chen, R., Cui, Y., Zhang, X., Zhang, Y., Chen, M., Zhou, T., et al. (2018). Chlorpyrifos induction of testicular-cell apoptosis through generation of reactive oxygen species and phosphorylation of AMPK. J. Agr. Food. Chem. 66, 12455–12470. doi: 10.1021/acs.jafc.8b03407
Choi, B. Y. (2019). Biochemical basis of anti-cancer-effects of phloretin-A natural dihydrochalcone. Molecules 24:E278. doi: 10.3390/molecules24020278
Choi, Y. H. (2018). Schisandrin A prevents oxidative stress-induced DNA damage and apoptosis by attenuating ROS generation in C2C12 cells. Biomed. Pharmacother. 106, 902–909. doi: 10.1016/j.biopha.2018.07.035
Dimozi, A., Mavrogonatou, E., Sklirou, A., and Kletsas, D. (2015). Oxidative stress inhibits the proliferation, induces premature senescence and promotes a catabolic phenotype in human nucleus pulposus intervertebral disc cells. Eur. Cells Mater. 30, 89–102. doi: 10.22203/ecm.v030a07
Han, L., Li, J., Li, J., Pan, C. Y., Xiao, Y., Lan, X. Y., et al. (2020). Activation of AMPK/Sirt3 pathway by phloretin reduces mitochondrial ROS in vascular endothelium by increasing the activity of MnSOD via deacetylation. Food Funct. 11, 3073–3083. doi: 10.1039/c9fo02334h
Haramizu, S., Asano, S., Butler, D. C., Stanton, D. A., Hajira, A., Mohamed, J. S., et al. (2017). Dietary resveratrol confers apoptotic resistance to oxidative stress in myoblasts. J. Nutr. Biochem. 50, 103–115. doi: 10.1016/j.jnutbio.2017.08.008
Ho, G. T., Kase, E. T., Wangensteen, H., and Barsett, H. (2017). Phenolic elderberry extracts, anthocyanins, procyanidins, and metabolites influence glucose and fatty acid uptake in human skeletal muscle cells. J. Agric. Food Chem. 65, 2677–2685. doi: 10.1021/acs.jafc.6b05582
Huang, W. C., Dai, Y. W., Peng, H. L., Kang, C. W., Kuo, C. Y., and Liou, C. J. (2015). Phloretin ameliorates chemokines and ICAM-1 expression via blocking of the NF-κB pathway in the TNF-α-induced HaCaT human keratinocytes. Int. Immunopharmacol. 27, 32–37. doi: 10.1016/j.intimp.2015.04.024
Hyson, D. A. (2011). A comprehensive review of apples and apple components and their relationship to human health. Adv. Nutr. 2, 408–420. doi: 10.3945/an.111.000513
Kim, J. S., and Yi, H. K. (2018). Schisandrin C enhances mitochondrial biogenesis and autophagy in C2C12 skeletal muscle cells: potential involvement of anti-oxidative mechanisms. N. S. Arch. Pharmacol. 391, 197–206. doi: 10.1007/s00210-017-1449-1
Leitner, L. M., Wilson, R. J., Yan, Z., and Gödecke, A. (2017). Reactive oxygen species/nitric oxide mediated inter-organ communication in skeletal muscle wasting diseases. Antioxid. Redox. Sign. 26, 700–717. doi: 10.1089/ars.2016.6942
Li, X., Chen, B., Xie, H., He, Y., Zhong, D., and Chen, D. (2018). Antioxidant structure-activity relationship analysis of five dihydrochalcones. Molecules 23:E1162. doi: 10.3390/molecules23051162
Lu, J., Jiang, H., Liu, B., Baiyun, R., Li, S., Lv, Y., et al. (2018). Grape seed procyanidin extract protects against Pb-induced lung toxicity by activating the AMPK/Nrf2/p62 signaling axis. Food Chem. Toxicol. 116, 59–69. doi: 10.1016/j.fct.2018.03.034
Moulin, M., and Ferreiro, A. (2017). Muscle redox disturbances and oxidative stress as pathomechanisms and therapeutic targets in early-onset myopathies. Semin. Cell. Dev. Biol. 64, 213–223. doi: 10.1016/j.semcdb.2016.08.003
Nguyen, T. M., Seigneurin, F., Froment, P., Combarnous, Y., and Blesbois, E. (2015). The 5’-AMP-Activated protein kinase (AMPK) is involved in the augmentation of antioxidant defenses in cryopreserved chicken sperm. PLoS One 10:e0134420. doi: 10.1371/journal.pone.0134420
Panche, A. N., Diwan, A. D., and Chandra, S. R. (2016). Flavonoids: an overview. J. Nutr. Sci. 5:e47. doi: 10.1017/jns.2016.41
Ren, D., Liu, Y., Zhao, Y., and Yang, X. (2016). Hepatotoxicity and endothelial dysfunction induced by high choline diet and the protective effects of phloretin in mice. Food Chem. Toxicol. 94, 203–212. doi: 10.1016/j.fct.2016.06.004
Semprun-Prieto, L. C., Sukhanov, S., Yoshida, T., Rezk, B. M., Gonzalez-Villalobos, R. A., Vaughn, C., et al. (2011). Angiotensin II induced catabolic effect and muscle atrophy are redox dependent. Biochem. Bioph. Res. 409, 217–221. doi: 10.1016/j.bbrc.2011.04.122
Sondag, G. R., Salihoglu, S., Lababidi, S. L., Crowder, D. C., Moussa, F. M., Abdelmagid, S. M., et al. (2014). Osteoactivin induces transdifferentiation of C2C12 myoblasts into osteoblasts. J. Cell. Physiol. 229, 955–966. doi: 10.1002/jcp.24512
Song, J., Zhang, W., Wang, J., Yang, H., Zhao, X., Zhou, Q., et al. (2018). Activation of Nrf2 signaling by salvianolic acid C attenuates NF-κB mediated inflammatory response both in vivo and in vitro. Int. Immunopharmacol. 63, 299–310. doi: 10.1016/j.intimp.2018.08.004
Sukhanov, S., Semprun-Prieto, L., Yoshida, T., Michael, T. A., Higashi, Y., Galvez, S., et al. (2011). Angiotensin II, oxidative stress and skeletal muscle wasting. Am. J. Med. Sci. 342, 143–147. doi: 10.1097/MAJ.0b013e318222e620
Tarnopolsky, M. A. (2016). Metabolic myopathies. Continuum 22, 1829–1851. doi: 10.1212/CON.0000000000000403
Tu, S. H., Chen, L. C., and Ho, Y. S. (2017). An apple a day to prevent cancer formation: reducing cancer risk with flavonoids. J. Food Drug Anal. 25, 119–124. doi: 10.1016/j.jfda.2016.10.016
Vineetha, V. P., Soumya, R. S., and Raghu, K. G. (2015). Phloretin ameliorates arsenic trioxide induced mitochondrial dysfunction in H9c2 cardiomyoblasts mediated via alterations in membrane permeability and ETC complexes. Eur. J. Pharmacol. 754, 162–172. doi: 10.1016/j.ejphar.2015.02.036
Wang, D., Wang, Q., Yan, G., Qiao, Y., and Tang, C. (2015). Phloretin inhibits platelet-derived growth factor-BB-induced rat aortic smooth muscle cell proliferation, migration, and neointimal formation after carotid injury. J. Cardiovasc. Pharm. 65, 444–455. doi: 10.1097/FJC.0000000000000213
Wang, D., Zhao, J., Li, S., Shen, G., and Hu, S. (2018). Quercetin attenuates domoic acid-induced cognitive deficits in mice. Nutr. Neurosci. 21, 123–131. doi: 10.1080/1028415X.2016.1231438
Wang, G., Gao, Y., Wang, H., Wang, J., and Niu, X. (2018). Phloretin reduces cell injury and inflammation mediated by staphylococcus aureus via targeting sortase B and the molecular mechanism. Appl. Microbiol. Biot. 102, 10665–10674. doi: 10.1007/s00253-018-9376-8
Wang, L., Li, Z. W., Zhang, W., Xu, R., Gao, F., Liu, Y. F., et al. (2014). Synthesis, crystal structure, and biological evaluation of a series of phloretin derivatives. Molecules 19, 16447–16457. doi: 10.3390/molecules191016447
Wu, K. H., Ho, C. T., Chen, Z. F., Chen, L. C., Whang-Peng, J., Lin, T. N., et al. (2018). The apple polyphenol phloretin inhibits breast cancer cell migration and proliferation via inhibition of signals by type 2 glucose transporter. J. Food Drug. Anal. 26, 221–231. doi: 10.1016/j.jfda.2017.03.009
Yang, Q., Han, L., Li, J., Xu, H., Liu, X., Wang, X., et al. (2018). Activation of Nrf2 by phloretin attenuates palmitic acid-induced endothelial cell oxidative stress via AMPK-dependent signaling. J. Agric. Food Chem. 67, 120–131. doi: 10.1021/acs.jafc.8b05025
Ying, Y., Jin, J., Ye, L., Sun, P., Wang, H., and Wang, X. (2018). Phloretin prevents diabetic cardiomyopathy by dissociating Keap1/Nrf2 complex and inhibiting oxidative stress. Front. Endocrinol. 9:774. doi: 10.3389/fendo.2018.00774
Zhang, Z., Li, S., Cao, H., Shen, P., Liu, J., Fu, Y., et al. (2019). The protective role of phloretin against dextran sulfate sodium-induced ulcerative colitis in mice. Food Funct. 10, 422–431. doi: 10.1039/c8fo01699b
Zhang, Z. K., Li, J., Guan, D., Liang, C., Zhuo, Z., Liu, J., et al. (2018). A newly identified lncRNA MAR1 acts as a miR-487b sponge to promote skeletal muscle differentiation and regeneration. J. Cachexia Sarcopeni 9, 613–626. doi: 10.1002/jcsm.12281
Zhang, Z. K., Li, J., Liu, J., Guo, B., Leung, A., Zhang, G., et al. (2016). Icaritin requires phosphatidylinositol 3 kinase (PI3K)/Akt signaling to counteract skeletal muscle atrophy following mechanical unloading. Sci. Rep. 6:20300. doi: 10.1038/srep20300
Zhao, C., Zhang, Y., Liu, H., Li, P., Zhang, H., and Cheng, G. (2017). Fortunellin protects against high fructose-induced diabetic heart injury in mice by suppressing inflammation and oxidative stress via AMPK/Nrf-2 pathway regulation. Biochem. Biophys. Res. 490, 552–559. doi: 10.1016/j.bbrc.2017.06.076
Keywords: phloretin, skeletal myopathies diseases, C2C12 myoblasts, AMPK, antioxidative
Citation: Li J, Yang Q, Han L, Pan C, Lei C, Chen H and Lan X (2020) C2C12 Mouse Myoblasts Damage Induced by Oxidative Stress Is Alleviated by the Antioxidant Capacity of the Active Substance Phloretin. Front. Cell Dev. Biol. 8:541260. doi: 10.3389/fcell.2020.541260
Received: 10 March 2020; Accepted: 25 August 2020;
Published: 11 September 2020.
Edited by:
Eleonora Napoli, University of California, Davis, United StatesReviewed by:
H. Susana Marinho, University of Lisbon, PortugalClaudia Penna, University of Turin, Italy
Stephan Krähenbühl, University of Basel, Switzerland
Jamal Bouitbir, University Hospital of Basel, Switzerland
Copyright © 2020 Li, Yang, Han, Pan, Lei, Chen and Lan. This is an open-access article distributed under the terms of the Creative Commons Attribution License (CC BY). The use, distribution or reproduction in other forums is permitted, provided the original author(s) and the copyright owner(s) are credited and that the original publication in this journal is cited, in accordance with accepted academic practice. No use, distribution or reproduction is permitted which does not comply with these terms.
*Correspondence: Xianyong Lan, lanxianyong79@nwsuaf.edu.cn; lanxianyong79@126.com