Detachment Activated CyPA/CD147 Induces Cancer Stem Cell Potential in Non-stem Breast Cancer Cells
- 1National Translational Science Center for Molecular Medicine, Department of Cell Biology, School of Basic Medicine, The Fourth Military Medical University, Xi’an, China
- 2Shaanxi Provincial Centre for Disease Control and Prevention, Xi’an, China
- 3Department of Urology, Xijing Hospital, The Fourth Military Medical University, Xi’an, China
- 4Department of Pathology, Fuzhou General Hospital of Nanjing Military Command, Fuzhou, China
- 5Department of Molecular Biosciences, The University of Kansas, Lawrence, KS, United States
- 6Department of Radiation Oncology, The University of Kansas, Lawrence, KS, United States
Background: Cancer stem cells (CSCs), responsible for cancer metastasis and recurrence, are generated from non-CSCs after chemo-radiation therapy. This study investigated the induction of CSC potential in non-stem breast cancer cells and the underlying molecular mechanisms in detachment culture.
Methods: Bulk breast cancer cells, or sorted non-CSCs and CSCs were cultured under an attached or detached condition to assess CSC numbers, ability to form tumor spheres, expression of stemness markers, and chemoresistance. Lentivirus carrying CD147 shRNA or cDNA was used to manipulate CD147 expression, while CD147 ligand recombinant cyclophilin A (CyPA) or its inhibitor was used to activate or inhibit CD147 signaling.
Results: Detachment promoted anoikis resistance, chemoresistance, sphere formation, self-renewal, and expression of stemness markers in breast cancer cells. Detachment increased functional ALDH+ or CD44highCD24–/low CSCs, and induced CSC potential in ALDH– or CD44lowCD24high non-CSCs. Upon detachment, both CD147 expression and CyPA secretion were enhanced, and CyPA-CD147 activation mediated detachment induced CSC potential in non-CSCs via STAT3 signaling. Clinically, CD147 and pSTAT3 were highly co-expressed and correlated with poor overall survival and tumor recurrence in breast cancer patients.
Conclusion: This study demonstrates that detachment induces the generation of CSCs from non-stem breast cancer cells via CyPA-CD147 signaling, indicating that targeting CD147 may serve as a potential novel therapeutic strategy for lethal metastatic breast cancer by eliminating induced CSCs.
Introduction
Breast cancer is the most commonly diagnosed cancer in women and the sixth leading cause of cancer-related deaths in Chinese women (Li et al., 2016). Approximately 80% of breast cancer-related deaths are due to cancer metastasis to distant organs, like the lung, bone, liver, or brain (Quaglino et al., 2020). To date, surgery is still the most effective treatment option to cure localized breast cancer, and chemo-radiotherapy shows efficacy for shrinking tumors; however, both treatment strategies fail to eradicate tumor metastasis. Thus, there is an urgent unmet need to develop novel and more effective strategies to control breast cancer metastasis.
Cancer cells can spread in the early stage of disease, such as cancer in situ or precancerous lesions, while clinically visible cancer metastasis usually occurs over time, typically 5–20 years (Visvader and Lindeman, 2012). In an experimental mouse model, the majority of injected metastatic cancer cells were shown to undergo apoptosis within 2 days and only 0.02% of cancer cells were able to form macro-metastases (Oskarsson et al., 2014; Celia-Terrassa and Kang, 2016). It is estimated that millions of cancer cells are released from a primary tumor mass into the circulation system daily; however, only a few of these cells can survive and successfully form metastatic lesions. This small portion of cancer cells are referred to as cancer stem cells (CSCs), which possess the ability to initiate tumor formation and growth or to initiate tumor metastasis and recurrence in patients (Batlle and Clevers, 2017; Clarke, 2019). Numerous reports have provided evidence that CSCs exist in the blood and solid tumor, and breast CSCs were among the earliest reported CSCs isolated from solid tumors (Al-Hajj et al., 2003). Breast CSCs were found to have higher specific cell surface antigen CD44 expression but no or lower CD24 expression, or to have higher aldehyde dehydrogenase 1 (ALDH1) activity (Celia-Terrassa and Kang, 2016; Cortes-Hernandez et al., 2019). Besides their tumorigenicity capability, breast CSCs exhibit enhanced in vitro invasiveness and in vivo metastasis compared with non-CSCs; and high numbers of CSCs have been associated with poor prognosis and distant metastasis in breast cancer patients (Pece et al., 2010).
Recently, it has become commonly accepted that not all CSCs have an equal capability to initiate metastasis, and that only a subset of CSCs, named as metastatic stem cell (MetSC) (Oskarsson et al., 2014) or metastasis-initiating cells (MICs) (Celia-Terrassa and Kang, 2016), possesses the ability to settle metastatic colonies in secondary organs. Beside inherited CSC potential, including self-renewal, differentiation, and tumorigenicity, MICs may acquire additional properties, such as resistance to anoikis, which is a detachment induced apoptosis that serves as the first major challenge and a critical barrier to metastasis. These properties provide the cells with a chance to survive in the circulation and form metastasis (Peitzsch et al., 2017). Moreover, MICs may have evolved from a subpopulation of circulating tumor cells (CTCs, cancer cells detached from a solid tumor mass into the blood circulatory system) by undergoing epithelial to mesenchymal transition (EMT) and obtaining induced stem cell-like potential, which provides them an extraordinary advantage to suppress anoikis and seed a metastatic lesion (Pece et al., 2010; Gkountela and Aceto, 2016; Peitzsch et al., 2017). In breast cancer CTC populations, the existence of CD44+Met+CD47+ MICs has been reported (Pece et al., 2010).
Thus, MICs with both CSC potential and anoikis resistance properties are likely driven by epigenetic changes and subsequent induction under extreme stress conditions, like detachment from the extracellular matrix (ECM) during dissemination (Peitzsch et al., 2017). Hypomethylation regions enriched with transcription factor binding sites for stemness genes, such as OCT4, NANOG, and SOX2, were identified in metastasis-prone CTC clusters (Gkountela et al., 2019). However, it remains unclear how the CTC epigenetic status or the induced CSC potential is established under the ECM detached condition. Therefore, to discover a novel anti-metastasis strategy against MICs, research exploring the impact of detachment on CSC potential and the corresponding molecular mechanisms is urgently needed. Furthermore, current research in the field has mainly focused on enrichment and assessment of CSCs using the sphere formation assay in a detached or non-adherent condition, but only a few studies have assessed the induction of CSC potential upon detachment.
Our previous studies demonstrated that breast cancer cells with high CD147 expression possess more malignant phenotypes, like cancer metastasis and recurrence, and are associated with poor overall survival and treatment outcomes (Li et al., 2009). CD147 promotes cancer cell invasion and metastasis by stimulating the secretion of matrix metalloproteinase (Xu et al., 2007b), enhancing cell motility (Zhao et al., 2011), and regulating the interaction between cancer and stroma (Xu et al., 2013). More importantly, CD147 expression can induce cancer cells to acquire CSC characteristics, such as EMT (Wu et al., 2011), anoikis resistance (Ma et al., 2010; Ke et al., 2012), and chemo-resistance (Tang et al., 2012). In addition, we previously reported that CD147 induces cell proliferation and invasion by activating pSTAT3Y705 signaling via interacting with CD44s (Li et al., 2013), while anti-CD147 inhibits CSC potential and sensitizes cancer cells to chemoradiotherapy by blocking CD44s-pSTAT3 signaling (Fan et al., 2019). In view of the role of CD147 in CSCs and anoikis resistance, we hypothesized that CD147 may be involved in the induction of breast CSC features under a detached condition.
We performed a systematic study to explore the impact of detachment on the potential of breast CSCs and the underlying cellular and molecular mechanisms. Our findings provide a promising therapeutic strategy to prevent breast cancer metastasis through disrupting detachment induced CSC potential via targeting CD147.
Materials and Methods
Cell Lines and Constructs
Human breast cancer T-47D, MCF7, and MDA-MB-231 cell lines were originally purchased from the American Type Culture Collection (Manassas, VA, United States) and cultured in Roswell Park Memorial Institute-1640 medium (RPMI-1640; HyClone, Logan, UT, United States) supplemented with 10% fetal bovine serum (FBS, HyClone) in a humidified incubator with 5% CO2 at 37°C. All cell lines were tested for mycoplasma and characterized by short tandem repeat (STR) profiling analysis (Cenvino, Beijing, China).
The pGIPZ empty vector, pGIPZ-CD147, and pLKO.1-CD147 lentiviral shRNA constructs were obtained from Open Biosystems (Lafayette, CO, United States). The MISSION® Non-Target shRNA control vector (pLKO.1-NTC) was obtained from Sigma Chemicals. CD147 overexpressed construct was established by subcloning human CD147 cDNA into the GV341 lentiviral expression vector (Genechem, Shanghai, China).
Establishment of Stable Cell Sublines
The lentivirus carrying CD147 shRNA (untagged pLKO CD147-shRNA, GFP tagged pGIPZ CD147-shRNA) or negative control shRNA, carrying CD147 cDNA or control vector was infected into breast cancer cells, and the stable cell subclones were selected by adding culture medium containing 3–6 μg/ml of puromycin. CD147 expression levels were analyzed using flow cytometry and immunoblotting.
Tumorsphere Culture and Passaging
Cells were suspended in Dulbecco’s modified Eagle’s medium/F12 (DMEM/F12, HyClone) serum-free medium containing 1% N2, 2% B27, 15% glucose (Invitrogen), 10 ng/ml human bFGF (Sigma Chemicals), 10 ng/ml EGF (Invitrogen), 1 μg/ml hydrocortisone (Sigma Chemicals), 5 μg/ml insulin (Sigma Chemicals), 5 μg/ml β-mercaptoethanol (Invitrogen), 0.2% heparin (Stem Cell Technologies, Cambridge, MA, United States) and seeded into six-well ultra-low attachment plates (Corning, NY, United States) in triplicate at a density of 2 × 104 cells per well and cultured for 10–14 days to form tumor spheres, which were quantified under an inverted microscope (Olympus). For tumorsphere passaging, we collected spheroids with a 40 μm Cell Strainer (Corning, San Jose, CA, United States) and disassociated them with TrypLETM (Thermo Fisher Scientific, Waltham, MA, United States) into a single cell suspension, and re-seeded the viable cells into six-well ultra-low attachment plates at a density of 2 × 104 cells per well and cultured for 10–14 days. Sphere formation efficiency (SFE) was calculated as the percentage of the number of spheres larger than 70 μm in the total number of original inoculated cells.
For single cell tumorsphere formation, cells were seeded into 96-well ultra-low attachment plates (Corning) at a dilution of approximately one cell per well and maintained for 1 month with the tumorsphere medium refreshed every 7 days.
Anoikis Assay
Cells were seeded into 24-well ultra-low attachment plates (Corning, detached condition) or uncoated common plates (attached condition) at a density of 5 × 104 per well and cultured in RPMI-1640 medium containing 10% FBS for indicated periods of time. The cells were labeled with the Annexin V-FITC and propidium iodide (PI) double staining kit (BioLegend, San Diego, CA, United States) according to the manufacturer’s protocol. The labeled cells were immediately measured with a BD FACS Calibur Flow Cytometer (BD Biosciences, San Jose, CA, United States) and quantified with CellQuest software (BD Biosciences) for apoptosis (anoikis) levels.
ALDEFLUOR Assay and Sorting of ALDH± Subpopulations
The ALDEFLUOR kit (Stem Cell Technologies) was applied to analyze the population with ALDH1 enzymatic activity according to a previous study (Wu et al., 2016). In brief, 1 × 106 cells were incubated in the ALDEFLUOR assay buffer containing 1 μM ALDH1 substrate BAAA at 37°C for 45 min, whereas negative control cells were incubated with 50 mM of ALDH inhibitor diethylaminobenz aldehyde (DEAB) under the same conditions. Next, the cells were resuspended in 2% FBS/Hank’s Balanced Salt Solution (HBSS) containing 1 μg/ml 7-AAD to exclude dead cells. The sorting gates were established using DEAB negative cells, and positive and negative cells were sorted on a BD FACS Aria III (BD Biosciences). The purity of sorted cells was determined as >90% positive.
CD44+CD24– Staining and Sorting of CD44highCD24–/low or CD44lowCD24high Subpopulations
1 × 106 cells were incubated on ice with 200 μl of anti-CD24-PE and anti-CD44-APC in 2% FBS/HBSS in the dark for 20 min, and the antibody labeled cells were then analyzed using a FACS Calibur Flow Cytometer. The isotype-matched mouse immunoglobulin was used as a negative control. For cell sorting, cells were stained with the indicated antibodies and suspended in 2% FBS/HBSS with 1 μg/ml 7-AAD for gating viable cells. Positive and negative cells were sorted on a BD FACS Aria III. The purity of sorted cells was determined as >90% positive.
Patient Samples and Tissue Microarray
A total 138 breast cancer patients who were hospitalized between June 2007 and August 2016 were included in this retrospective study. Patients’ survival data were recorded for 26–132 months. All patients (100%) underwent primary surgical interventions. The median age of patients was 54 years (range 29–87 years). Clinicopathological data were collected from patients’ medical records, which included age of patients at diagnosis, tumor type and grade, TNM stage, AJCC stage, patient survival, recurrence time, and the expression of Her-2, ER, PR, P53 (Table 1). This study was approved by the Institutional Review Board of the Air Force Medical University. A tissue microarray (TMA) constructed from paraffin-embedded tissue blocks from above 138 patients was purchased from the National Engineering Center for Biochip (Shanghai, China). The study protocol was approved by the ethics committee of The Fourth Military Medical University (KY20183305-1).
Immunohistochemistry
The TMA sections were immunostained according to a standard staining procedure described previously (Li et al., 2013). Mouse or rabbit IgG was used as a negative control. The percentage and intensity of staining was independently scored by two pathologists in a blinded manner. The staining intensity was scored for four categories: 0 (no visible staining), 1 (light brown staining), 2 (medium brown staining), and 3 (dark brown staining), with the same intensity covering more than 75% of the stained area. CD147 staining was scored as negative (no positivity at all); weak (1 + positivity regardless of positive cell percentages or 2 + positivity of ≤30% of cells); moderate (2 + positivity of >30% of cells or 3 + positivity of ≤50% of cells); and strong (3+, positivity of >50% of cells). pSTAT3 staining was classified as negative (no staining); weak (1 + staining in ≤50% of cells or 2 + staining in ≤25% of cells); moderate (1 + staining in >50% of cells, 2 + staining in 26–75% of cells or 3 + staining in ≤25% of cells); strong (2 + staining in >75% of cells or 3 + staining in >25% of cells) (Li et al., 2009, 2013). For data analysis, the immunostained TMA-sections were divided into two groups of low- vs. high-expression. The low-expression group included cases with intensity scores indicating negative and weak staining, whereas the high-expression group included cases with intensity scores indicating moderate and strong staining.
Statistical Analysis
All data are expressed as mean ± standard deviation (SD) of three independent experiments. An independent Student’s t-test or one-way ANOVA was performed to compare the continuous variables between the two groups or more than two groups, respectively, while the categorical variables were analyzed using the χ2 test. The Kaplan–Meier method and the log-rank test were used to compare overall survival, defined as the time from a patient’s surgery until death, and recurrence survival, defined as the time of patients having recurrence until death. Patients who survived were censored at the time of their last follow-up. The Cox’s proportional hazards model was used to analyze the association of CD147 and/or pSTAT3 expression with patient survival/recurrence for the independent prognostic predictors. All statistical analyses were carried out with SPSS 22.0 (IBM) and Prism 6.0 software (GraphPad). ∗P < 0.05, ∗∗P < 0.01, and ∗∗∗P < 0.001 compared with the control group.
Results
Detachment Promotes CSC Features of Breast Cancer Cells
To detect the effect of detachment culture on induction of CSC potential, we first determined the ability of breast cancer cells to resist anoikis. As compared with cancer cells cultured under the attached condition, we found that cancer cells grown under the detached condition exhibited different degrees of apoptosis (Figure 1A). The detached MDA-MB-231 cells showed slight alterations in the apoptotic ratio compared with those of cells grown under the attached condition; however, both T-47D and MCF7 cells showed notable apoptosis on Day 3, which gradually reduced until Day 7, with 76–90% of cells surviving the detached condition. In the following experiments, we selected Day 3 as the cutoff time point for anoikis induction and Day 7 as the cut-off time point for cell survival (anoikis resistance).
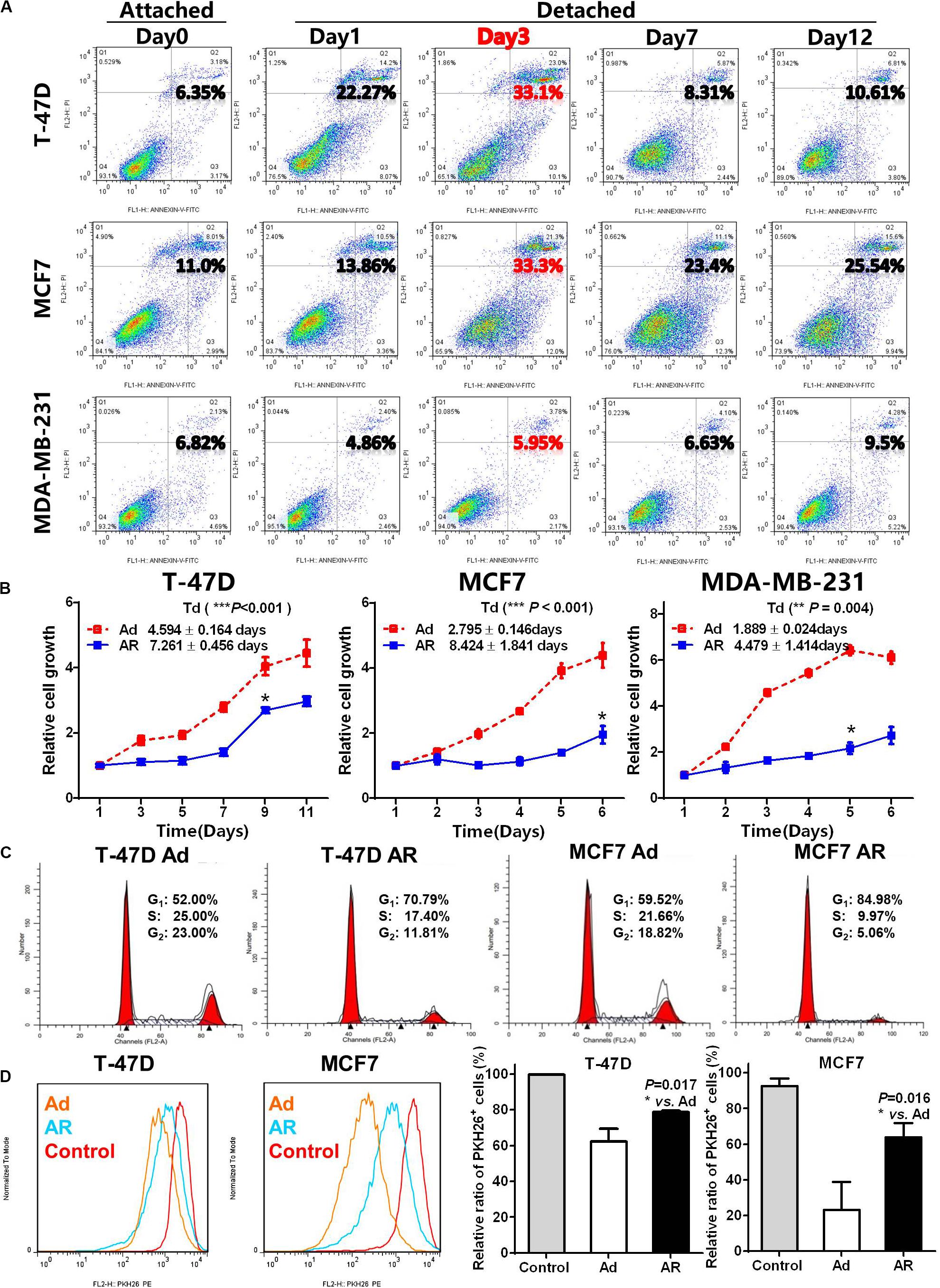
Figure 1. Detachment promotes CSC features of breast cancer cells. (A) Apoptosis analysis in breast cancer cells cultured under an attached condition in a common uncoated plate or a detached condition in ultra-low attachment plate for up to 12 days. (B) Cell growth measurement by detecting the WST-8 dye absorbance at 450 nm in breast cancer cells cultured under an adherent (Ad) or anoikis resistant (AR) condition for 7 days and then cultured under an adherent condition for up to 12 days. Td, doubling time. (C) Cell cycle distribution analysis in T-47D and MCF7 cells cultured under an Ad or AR condition for 7 days. (D) Cell proliferation analysis by PKH 26 staining in T-47D and MCF7 cells cultured under an Ad or AR condition for 7 days. Unlabeled cells were choosing as a control.
Compared with the adherent (Ad) cells, anoikis resistant (AR) cells had a restoration of cell growth after 1–4 days of latent phase with varying degrees of prolonged doubling time (Td, Figure 1B). The reduced AR cell growth coincided with a higher proportion of cell in the G1-phase (Figure 1C and Supplementary Figure S1A), and a significantly higher number of PKH26 positive slow-cycling or quiescent cells compared to the Ad cells (Figure 1D). As anoikis resistance, slow-cycling, and quiescence are essential features of CSCs, we hypothesized that detachment may promote the acquisition of CSC potential in AR cells.
Detachment Increases Functional ALDH+ or CD44highCD24–/low CSCs
To assess whether AR cells acquire CSC potential upon detachment, we assayed the ratio of ALDH+ or CD44highCD24–/low CSCs. As compared with that of attached cells, detached cells had a time-dependent increase in the ALDH+ subpopulation (Figure 2A and Supplementary Figure S1B). Under the detached condition, the ratio of CD44highCD24–/low subpopulation increased in a time-dependent manner in the anoikis-sensitive T-47D and MCF7 cells but not in anoikis-insensitive MDA-MB-231 cells, which have a higher percentage of the CD44highCD24–/low subpopulation (over 90%, Figure 2B and Supplementary Figure S1C). We further isolated fresh ex vivo malignant pleural effusion (MPE) cells from two breast cancer patients and cultured cells under attached or detached conditions for 14 days (Supplementary Figure S2A). Upon detachment, the ratio of the ALDH+ subpopulation increased by 1.91- and 2.12-fold in both MPE cells, while the ratio of the CD44highCD24–/low subpopulation increased by threefold in the MPE cells (Supplementary Figures S2B,C). These data suggest that breast cancer cells cultured under the detached condition have increased CSCs.
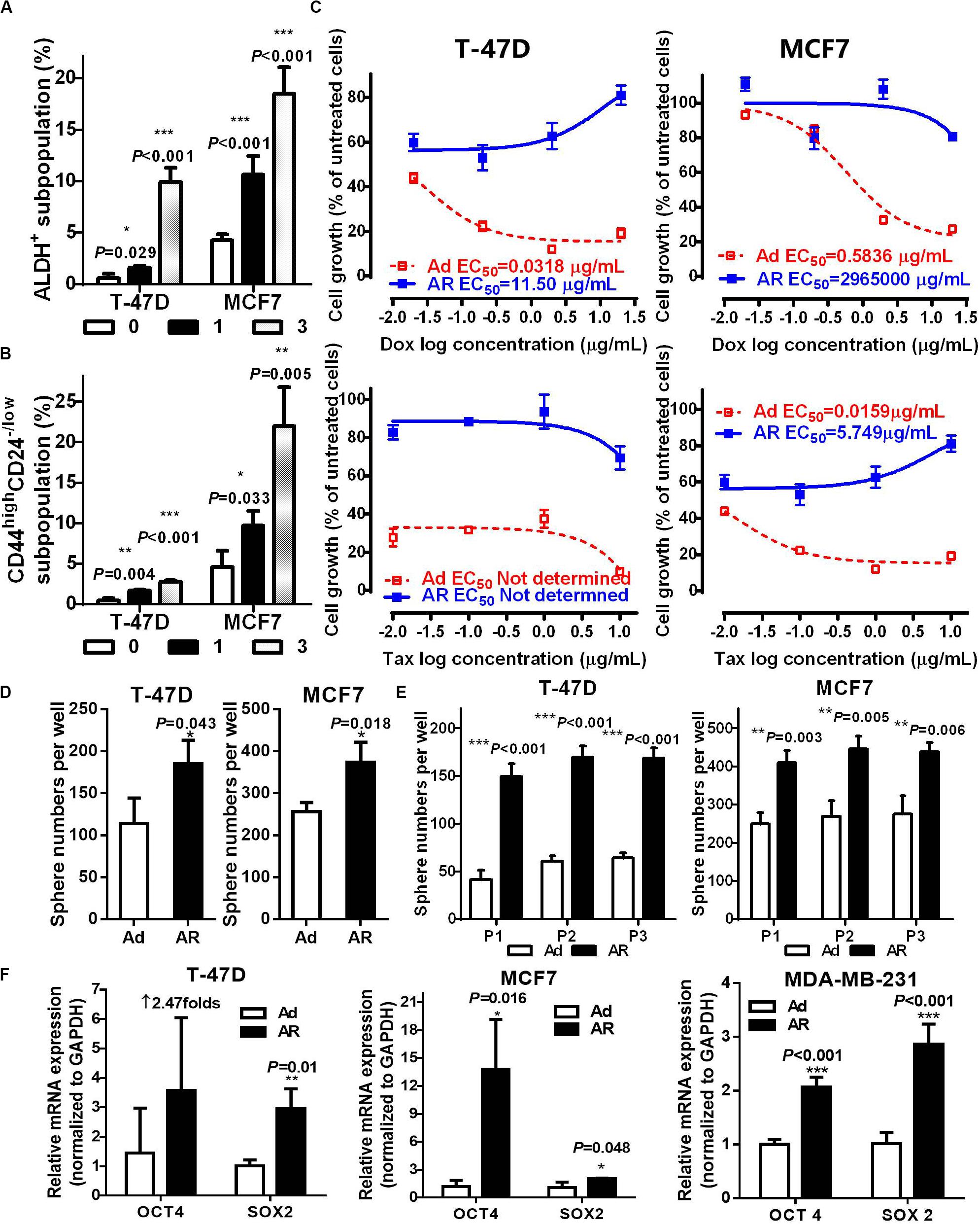
Figure 2. Detachment increases functional ALDH+ or CD44highCD24– /low CSCs. ALDH+ (A) and CD44highCD24– /low (B) subpopulation analysis in T-47D and MCF7 cells cultured under an attached or detached condition for 0, 1, or 3 days. (C–F) Cells were cultured under an Ad or AR condition for 7 days, and then used for the following experiments: (C) Cytotoxicity of Doxorubicin (Dox) and Taxol (Tax) in T-47D and MCF7 cells. (D,E) Tumorsphere numbers without or with passaging in T-47D and MCF7 cells. (F) qRT-PCR analysis of OCT4 and SOX2 mRNA levels in T-47D, MCF7, and MDA-MB-231 cells.
Next, we determined the influence of detachment on CSC traits, including drug sensitivity, spherogenesis, and stemness. Compared to those of Ad cells treated with either Doxorubicin or Taxol, AR cells showed a significant increase of 50% effective concentration (EC50, more than 360-fold inductions; Figure 2C and Supplementary Figure S2D). AR cells from the T-47D and MCF7 were capable of growing in the medium containing Doxorubicin and Taxol, respectively. AR cells also formed significantly more tumor spheres compared to the Ad cells (1.66 ± 0.19 and 1.47 ± 0.30-fold increases in T-47D and MCF7 cells, respectively, Figure 2D), and had higher spherogenesis capacity that was maintained after the third passage of tumorsphere culture (Figure 2E), implying that detached culture enhanced the self-renewal potential of CSCs. Moreover, AR cells expressed a significantly higher level of stemness genes like OCT4 and SOX2 compared to the Ad cells (Figure 2F). These results demonstrate that detachment enhances functional CSCs in AR cells.
Detachment Induces CSC Potential in ALDH– or CD44lowCD24high Non-CSCs
Previous studies revealed that differentiated cancer cells were able to acquire stem cell potential under environmental stress, such as hypoxia (Gammon et al., 2013), radiation (Peitzsch et al., 2016; Chen et al., 2017), and chemotherapy (Hu et al., 2012). In the following study, we explored whether non-stem breast cancer cells acquire CSC potential under a detached condition. We isolated non-CSCs (ALDH– or CD44lowCD24high) and CSCs (ALDH+ or CD44highCD24–/low), cultured them under either attached or detached conditions for 7 days, and then analyzed the CSC ratios (Figure 3A). Our data showed that upon detachment, both CSC and non-CSC subpopulations had significant increases in the ratio of CSCs (Figures 3B,C). However, the fold increases of CSC ratios were much higher in non-CSCs than in CSCs; the average increases of CSC ratios in CD44lowCD24high non-CSCs and in CD44highCD24–/low CSCs obtained from T-47D cells were 7.90 ± 2.68 and 3.77 ± 2.68-fold, respectively; and 3.61 ± 1.57 and 1.90 ± 0.42-fold, respectively, for MCF-7 cells. These findings were similar to the results in the ALDH– and ALDH+ subpopulations. Next, we isolated ALDH– non-CSCs and ALDH+ CSCs, and cultured them under Ad or AR conditions for tumorsphere formation and stemness gene analysis. We found that tumorsphere formation ability and stemness gene expression, especially SOX2 mRNA levels, were significantly increased both in the ALDH– non-CSCs and ALDH+ CSCs upon detachment, while OCT4 mRNA levels were significantly increased only in the ALDH+ CSCs upon detachment (Figures 3D,E). These data demonstrate that the CSC phenotypes and traits can be induced from non-CSCs under a detached condition.
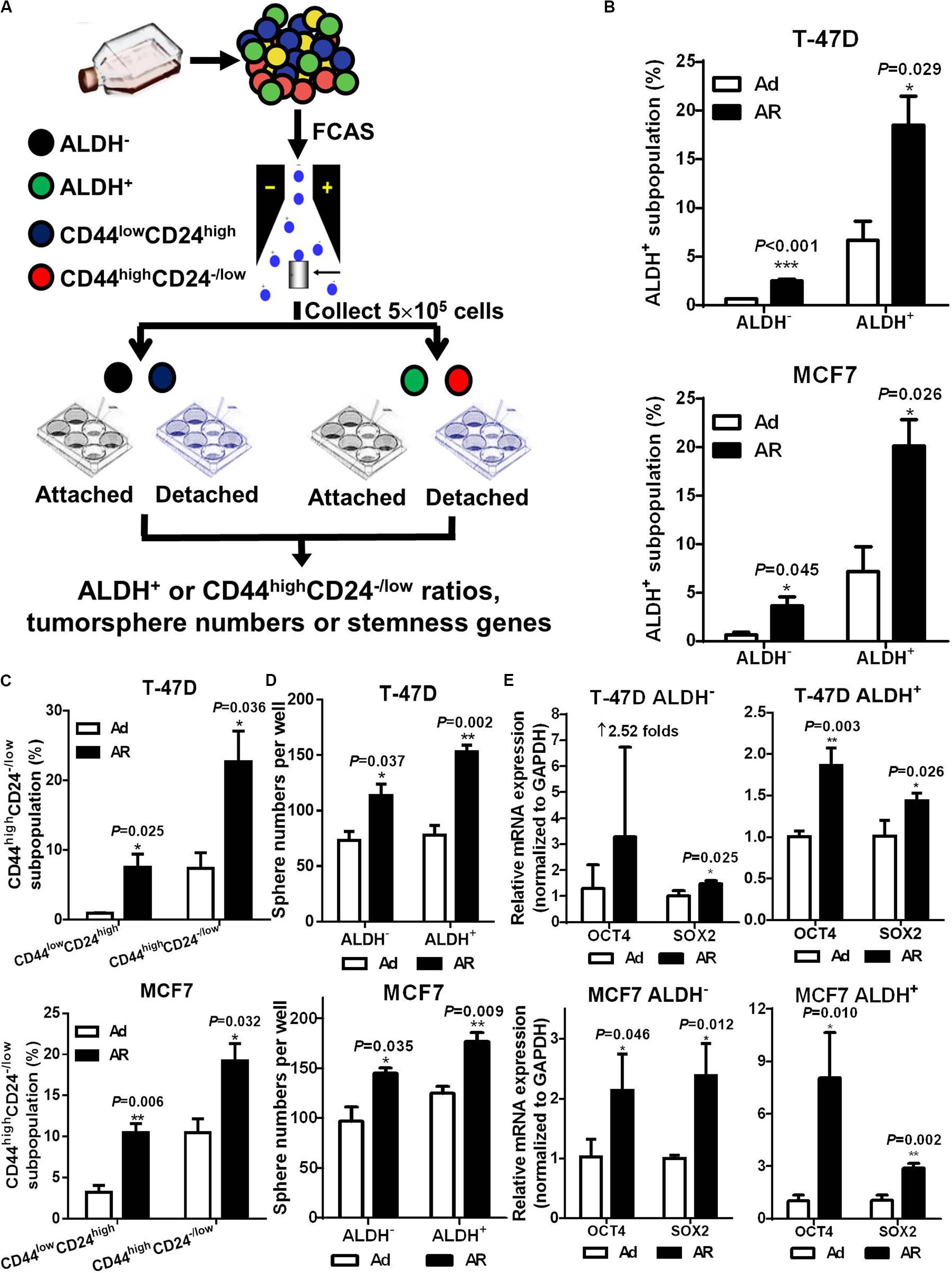
Figure 3. Detachment induces CSC potential in ALDH– or CD44lowCD24high non-CSCs. (A) Study design. Non-CSCs (ALDH– or CD44lowCD24high) and CSCs (ALDH+ or CD44highCD24– /low) were sorted from T-47D and MCF7 cells and cultured under an Ad or AR condition for 7 days, then the ratio of ALDH+ or CD44highCD24– /low subpopulation were analyzed. Flow cytometry analysis of ALDH+ (B) and CD44highCD24– /low (C) subpopulation in non-CSCs and CSCs. Tumorsphere formation (D) and qRT-PCR analysis (E) of OCT4 and SOX2 mRNA levels in ALDH– or ALDH+ subpopulation cultured under an Ad or AR condition for 7 days.
CD147 Mediates Detachment Induced CSC Potential in Non-CSCs
Our previous studies revealed that highly expressed CD147 in breast cancer tissues is an independent prognostic predictor for cancer recurrence and metastasis (Li et al., 2009), and CD147 induces the anoikis resistance of transformed HEK293 (Ma et al., 2010) and liver cancer cells (Ke et al., 2012). In our current breast cancer studies, we further confirmed that CD147 expression was associated with the ability to resist anoikis, with AR MDA-MB-231 cells having significantly higher CD147 expression compared to the anoikis sensitive T-47D and MCF7 cells (Supplementary Figures S2E,F, S3A left). Furthermore, upon detachment, CD147 protein levels were upregulated in a time-dependent manner in all three cell lines, although CD147 mRNA levels only increased in the T-47D and MCF7 cells (Figures 4A,B and Supplementary Figure S3A right).
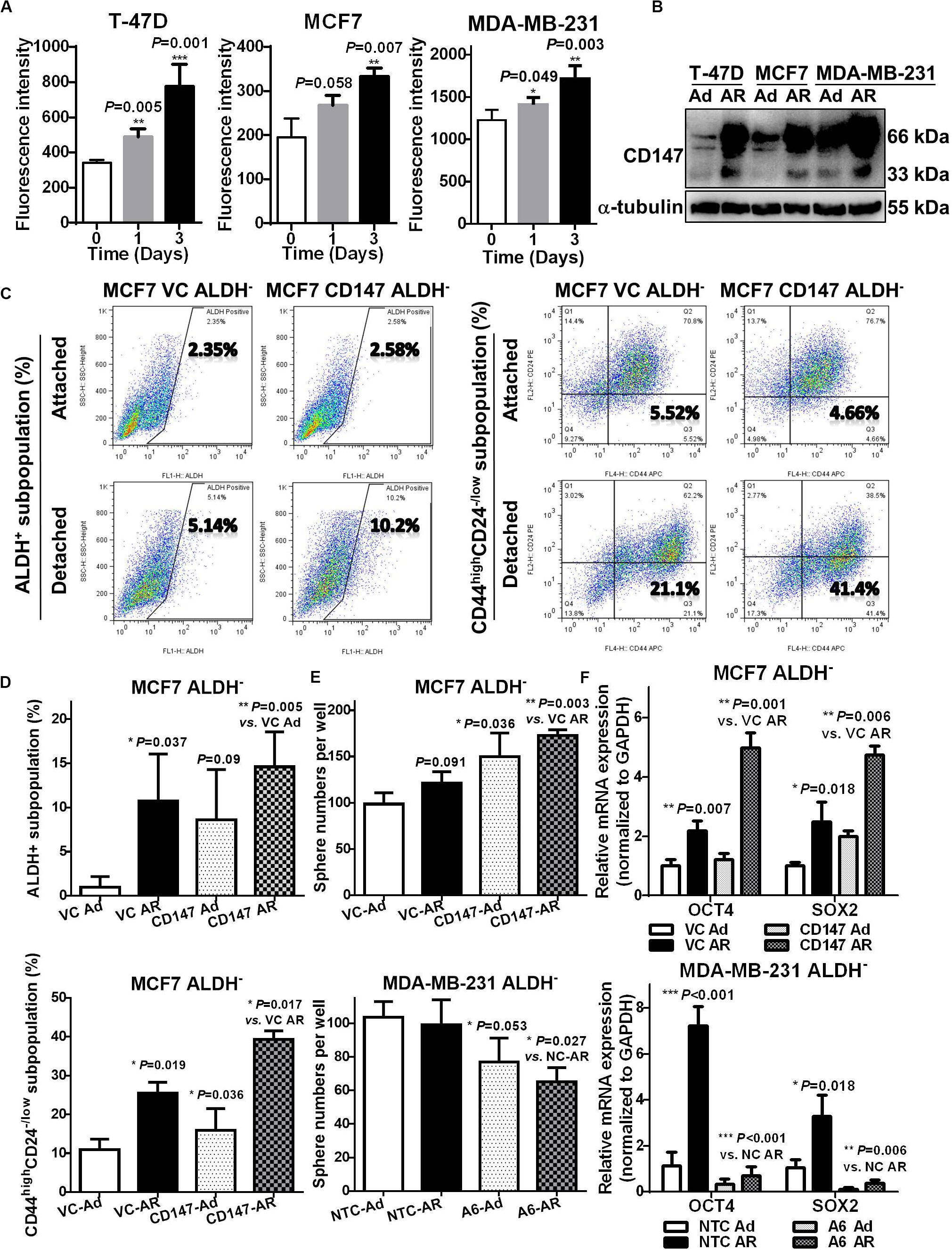
Figure 4. CD147 mediates detachment induced CSC potential in non-CSCs. (A) Flow cytometry analysis of membranous CD147 levels in T-47D, MCF7, and MDA-MB-231 cells cultured under an attached or detached condition for 0, 1, and 3 days. (B) Immunoblot assay of CD147 protein levels in T-47D, MCF7, and MDA-MB-231 cultured under an Ad or AR condition for 7 days. (C,D) ALDH+ and CD44highCD24– /low subpopulation analysis in ALDH– non-CSC subpopulation from CD147 knock-in (CD147) and control vector (VC) cells cultured under an Ad or AR condition for 7 days. Tumorsphere numbers (E) and OCT4 and SOX2 mRNA levels (F) in ALDH– subpopulation with or without CD147 knock-in or knockdown (A6 and NTC) cultured under an Ad or AR condition for 7 days.
To explore the influence of CD147 on detachment-induced CSC potential, we constructed the CD147 knockdown (A6)/negative control (NTC) or CD147 overexpressing (CD147)/vector control (VC) breast cancer cells and cultured them in a detached condition. As shown in Supplementary Figures S3B–G, effectively silencing CD147 expression (>70%) resulted in sensitizing MDA-MB-231 cells to detachment-induced apoptosis, while CD147 overexpression greatly enhanced the MCF7 cells’ ability to resist anoikis. CD147 knockdown significantly decreased tumorsphere numbers both in a passage-dependent manner and at a single cell level (Supplementary Figure S4A). Compared to NTC cells, CD147 knockdown A6 cells had a much lower tumor incidence (2/8 vs. 5/8), an increased median tumor formation time (>44 vs. 25 days), and significantly smaller tumors (77.05–81.72% reduction between Day 20 and Day 44, Supplementary Figure S4B). CD147 knockdown also abolished the detachment-induced ALDH+ subpopulation (0.43-fold vs. 1.63-fold), while CD147 overexpression promoted the detachment-induced stemness by upregulating SOX2 expression (Supplementary Figures S4C,D).
Next, we examined whether detachment-induced CD147 expression could help generate CSCs in non-CSCs. As shown in Figures 4C,D, CD147 knock-in significantly boosted detachment in both ALDH+ and CD44highCD24–/low subpopulation in MCF7 ALDH– cells. Moreover, CD147 overexpression significantly increased whereas CD147 knockdown remarkably abolished the detachment-induced spherogenesis and stemness in ALDH– MCF7 and MDA-MB-231 cells (Figures 4E,F). These data suggest that CD147 is associated with CSC traits in AR breast cancer cells, and that it plays an essential role in detachment-induced conversion of non-CSCs to CSCs.
CyPA Induces CSC Potential in Non-CSCs via Activating CD147
Cyclophilin A (CyPA) is a natural CD147 ligand and functions as an autocrine/paracrine chaperone molecule to facilitate CD147 membrane expression and stabilization for CD147 activation in cells (Zhu et al., 2015). Therefore, we explored whether CyPA induced CSC potential in breast cancer cells that were cultured under a detached condition. We found that the conditioned medium of AR cells had more extracellular CyPA (eCyPA) compared to the conditioned medium of the Ad cells (Figure 5A and Supplementary Figure S4E). We further found that recombinant CyPA dose-dependently increased the CSC subpopulation, tumorsphere formation, and stemness gene expression (Figures 5B–D and Supplementary Figure S4F). Together, these results suggest that eCyPA induces CSC potential.
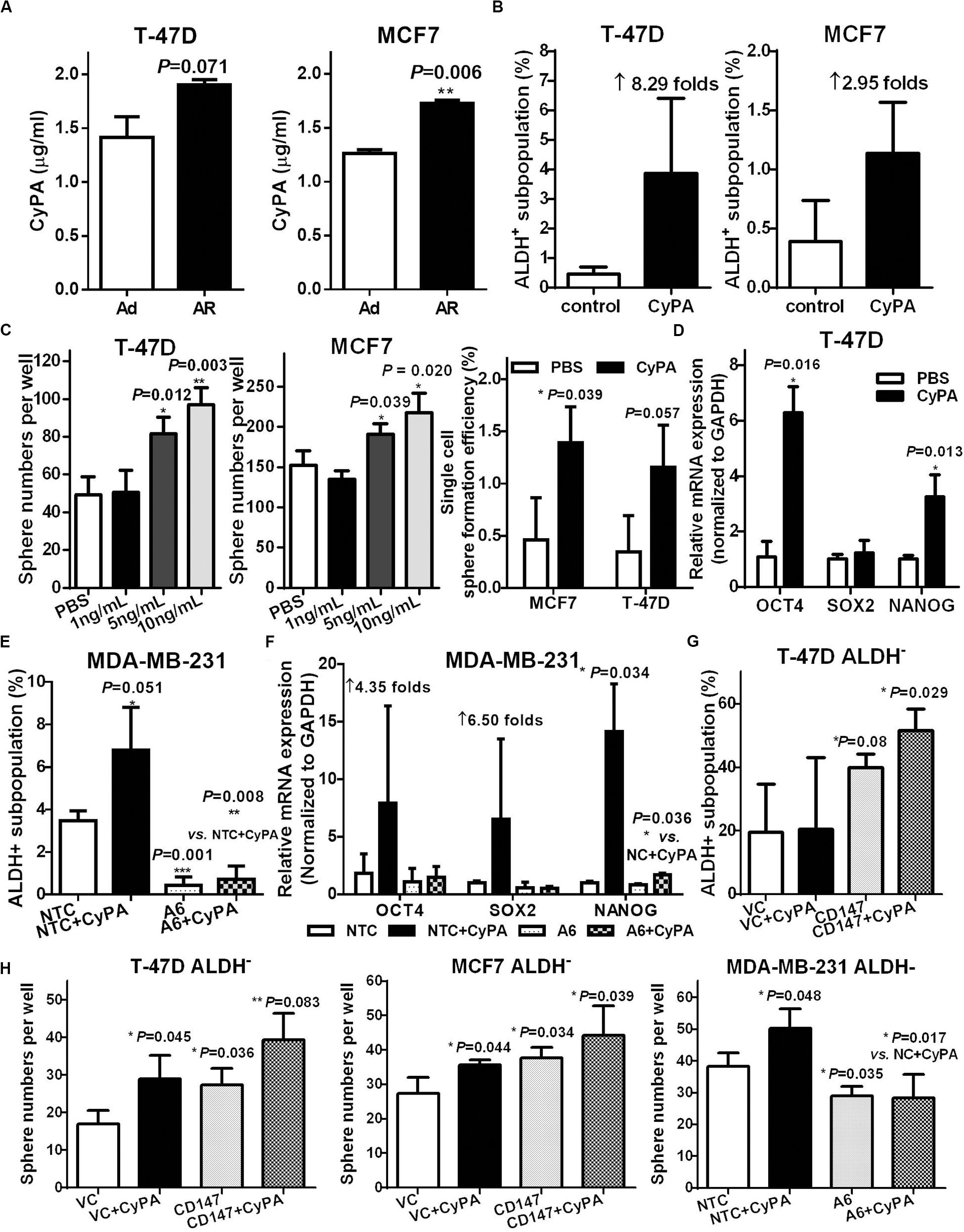
Figure 5. CyPA induces CSC potential in non-CSCs via activating CD147. (A) Supernatant CyPA levels from T-47D and MCF7 cells cultured under an attached or detached condition for 3 days. ALDH+ subpopulation analysis (B) and tumorsphere formation (C) in T-47D and MCF7 cells cultured with or without CyPA. (D) OCT4, SOX2, and NANOG mRNA level detection in T-47D cells cultured with or without CyPA. The ratio of ALDH+ subpopulation (E) and OCT4, SOX2, and NANOG mRNA level (F) analysis in MDA-MB-231 A6 and NTC cells cultured with or without CyPA. (G) ALDH+ subpopulation analysis in ALDH– subpopulation from T47D CD147 and VC cells cultured with or without CyPA. (H) Tumorsphere number analysis in ALDH– subpopulation with or without CD147 knock-in or knockdown cultured with or without CyPA. Apart from the dose-dependent tumorsphere formation assay, all cells used for the above experiments were treated with 20 ng/mL CyPA for 24 h.
We next investigated whether the CyPA-induced CSC potential was mediated by CD147 activation through altering the level or activity of CD147 or CyPA. Our data showed that knockdown of CD147 significantly restrained the CyPA induced ALDH+/CD44+CD24–/low subpopulation and stemness gene expression (Figures 5E,F and Supplementary Figures S5A,B). Conversely, CyPA inhibition reduced the CD147-overexpression increased ALDH+ and CD44+CD24–/low subpopulations (Supplementary Figures S5C–F). More importantly, CD147 overexpression further increased, whereas CD147 knockdown significantly blocked the CyPA-induced ALDH+ subpopulations and spherogenesis in ALDH– non-CSCs (Figures 5G,H). These data support that CyPA-activated CD147 mediates detachment-induced CSC potential in non-stem breast cancer cells.
CD147-STAT3 Signaling Contributes to Induced CSC Potential and Predicts Poor Prognosis and Recurrence in Breast Cancer Patients
We previously reported that CD147 induces cell proliferation and invasion by activating STAT3 signaling (Li et al., 2013; Xu et al., 2016), while others showed that STAT3 is associated with anoikis resistance (Fofaria and Srivastava, 2015) and the conversion of non-CSCs to CSCs (Kim et al., 2013). Thus, we next determined the role of STAT3 signaling in CD147 mediated conversion of non-CSCs to CSCs upon detachment. We observed that, compared to anoikis-sensitive T-47D and MCF7 Ad cells, AR cells had upregulated protein levels of total and phosphorylated STAT3 as well as its downstream target Bcl-xL (Figure 6A). We also found that the detachment-increased STAT3 signaling occurred in a CD147-dependent manner, as CD147 overexpression or knockdown dramatically enhanced or reduced the detachment-induced pSTAT3/Bcl-xL level, respectively (Figure 6B and Supplementary Figure S6A). Furthermore, the STAT3 inhibitor WP1066 significantly attenuated the detachment-induced spherogenesis and stemness by downregulating SOX2 protein expression in the detached ALDH– non-CSCs (Figures 6C,D). We also found that HAb18IgG, a CD147 antibody, significantly reversed CyPA-induced STAT3 signaling activation, downstream SOX2/OCT4 stemness gene expression, and sphere formation in ALDH– non-CSCs, which demonstrates that targeting CyPA-CD147 signaling could suppress STAT3 signaling and inhibit the generation of CSCs (Figures 6E,F). Taken together, our data indicate that CD147 promotes detachment-induced conversion of non-CSCs to CSCs by activating STAT3 signaling.
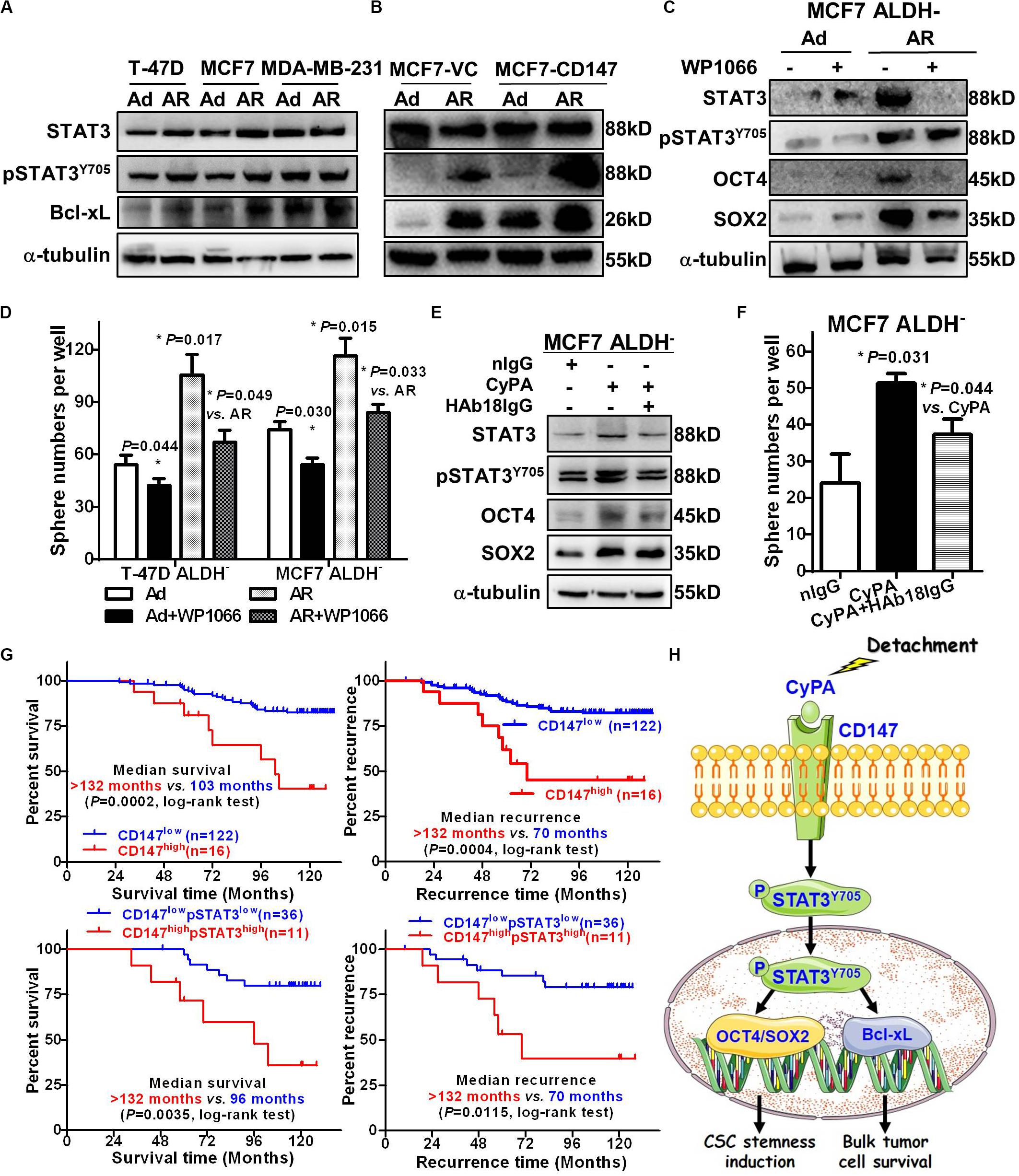
Figure 6. CD147-STAT3 signaling contributes to induced CSC potential and predicts poor prognosis and recurrence in breast cancer patients. STAT3, pSTAT3Y705, and Bcl-xL protein levels in T-47D, MCF7, and MDA-MB-231 cells (A) and MCF7-CD147/VC cells (B) cultured under an Ad or AR condition for 7 days. α-tubulin was used as a loading control and one representative of three biological replicates is shown. STAT3, pSTAT3Y705, OCT4, and SOX2 protein levels (C) and tumorsphere numbers (D) in ALDH– cells treated with or without 1 μM WP1066 for 24 h and then cultured under an Ad or AR condition for 7 days. STAT3, pSTAT3Y705, OCT4, and SOX2 protein levels (E) and tumorsphere numbers (F) in ALDH– cells treated with or without 20 ng/mL CyPA and 10 μg/mL HAb18IgG for 24 h. (G) Kaplan–Meier curve analysis of overall survival and tumor recurrence time in breast cancer patients stratified by level of cytoplasmic CD147 and/or pSTAT3. Up: Overall survival (left) and tumor recurrence time (right) in 138 patients stratified by low- vs. high CD147 expression. Down: Overall survival (left) and tumor recurrence time (right) in 47 patients stratified by low- vs. high CD147/pSTAT3 expression. (H) A proposed working model for detachment-induces CSC potential in non-stem breast cancer cells via CyPA-CD147-pSTAT3 signaling.
To investigate whether CD147-pSTAT3 signaling has a clinicopathological connection, we correlated patient survival and recurrence data with CD147 and pSTAT3 levels in breast cancer tissues from 138 patients. The patients with high cytoplasmic but not membranous CD147 expression had poorer overall survival and tumor recurrence (Figure 6G and Supplementary Figure S6B). The double immunofluorescence staining showed that CD147 and pSTAT3Y705 were co-expressed in breast cancer tissues (Supplementary Figure S6C). Furthermore, the co-expression of CD147 and pSTAT3Y705 was higher in detached cells than in adherent cells (Supplementary Figure S6D). These data are consistent with our recent reports that CD147 undergoes endocytosis, which removes membrane proteins in the cytoplasm, releases the intracellular domains in the nucleus, and contributes to liver cancer progression and poor prognosis (Wu et al., 2017; Yong et al., 2019). Moreover, cytoplasmic CD147 expression was significantly associated with pSTAT3 expression (Spearman r = 0.184, P = 0.03; Supplementary Figure S6E). Patients with high cytoplasmic CD147 and pSTAT3 co-expressed tumors had worse overall survival and tumor recurrence (Figure 6G and Table 2). The multi-variant Cox proportional hazards analysis shows that co-expression of cytoplasmic CD147 and pSTAT3 was an independent predictor for poor overall survival after adjusting for tumor type/grade, pT/pN/pM/stage, and clinical stages (hazard ratio = 31.85; 95% confidence interval, 2.67–380.02; P = 0.006, Table 3).
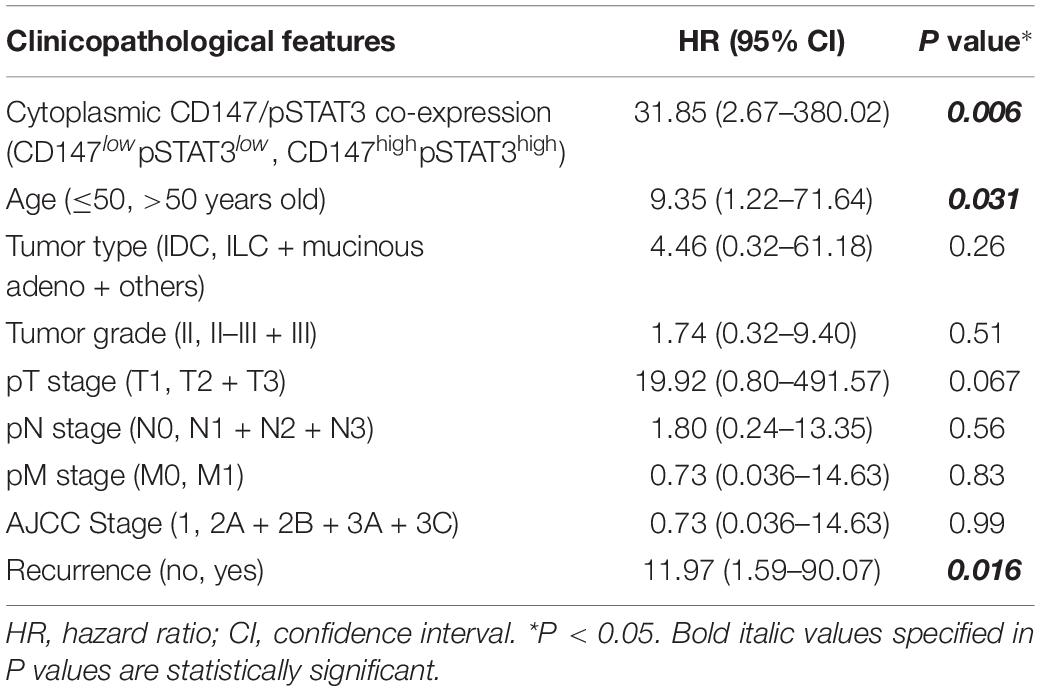
Table 3. The Cox proportional hazard analysis of independent predicators for overall survival of breast cancer patients.
Discussion
In the current study, we investigated the generation of induced breast cancer CSCs and the underlying molecular mechanisms under detachment culture conditions. We found that the resistance to detachment induced anoikis was associated with CSC characteristics, and detached culture was able to increase functional ALDH+ or CD44highCD24–/low CSC subpopulations and to induce CSC potential in ALDH– or CD44lowCD24high non-CSCs. Our data further demonstrated that the expression of CD147 and the secretion of its ligand CyPA were both enhanced in the detachment cultured breast cancer cells or supernatants, respectively, and high levels of CyPA/CD147 mediated the conversion of non-CSCs into CSCs via activating pSTAT3 signaling. In breast cancer tissue specimens, cytoplasmic CD147 and pSTAT3 were both highly expressed, and their concurrent expression was correlated with patient poor overall survival and tumor recurrence. In conclusion, our study provides exciting novel evidence that cancer cells cultured in a detached condition acquire stemness traits via upregulating CD147 expression. This suggests that detachment cultured non-stem cancer cells resistant to anoikis could be a source of induced CSCs with metastatic potential or MICs, and also indicates that targeting CD147 could be a promising therapeutic strategy for lethal metastatic breast cancer by eliminating induced CSCs.
In the metastasis cascade, the spread of a detached primary tumor cells through the bloodstream as CTCs is a critical step, and only CTCs enriched with stem-like features are considered the precursors of metastasis lesions. Therefore, the majority of studies have focused on the contribution of stem-like properties in CTCs to the metastatic initiating capacity (Gkountela and Aceto, 2016; Cortes-Hernandez et al., 2019). For example, the study by Gkountela et al. displayed the dedication of epigenetic regulation of stemness in detached CTC clusters on their metastasis-prone properties (Cortes-Hernandez et al., 2019; Gkountela et al., 2019). However, it is still not well known how CTCs acquire stemness potential. Using patient MPE derived cell lines and MPE cells grown in aggregates under detached conditions as a “surrogate” model for CTC clusters (May et al., 2018), we revealed that the CSC potential could be induced in breast cancer cells under a detached condition. Our current data indicate that detachment acts as a harsh microenvironmental pressure to induce CTC stemness potential. Therefore, the detailed signaling and molecular profiling involved in detachment induced stem-like potential holds exceptional promise to develop metastasis-tailored therapies.
Recently, the concept of phenotypic plasticity in cancer cells has been well established. Several studies confirmed that both CSCs and non-CSCs were capable of undergoing phenotypic transitions in response to microenvironmental stresses, with a dynamic equilibrium of dedifferentiation-redifferentiation between non-CSCs and induced CSCs (Batlle and Clevers, 2017; O’Brien-Ball and Biddle, 2017; May et al., 2018; Clarke, 2019). Specifically, non-CSCs can be induced or dedifferentiate into induced CSCs by radio-chemotherapy (Peitzsch et al., 2016; Chen et al., 2017) and also by detachment condition, as shown in this paper. As we prepared this manuscript for publication, Fumagalli et al. (2020) reported that Lgr5– non-CSCs are able to convert into Lgr5+ CSCs at the metastatic site for efficient colorectal cancer metastatic outgrowth. Based on these finding, it seems that a successful cancer cure can be achieved only by killing both preexisting CSCs and induced CSCs. The key point to eradicate the induced CSCs is to prevent the formation of induced CSCs and to block the signaling pathways involved in de-differentiation of non-CSCs to CSCs. Thus, blocking the detachment of cancer cells from the ECM and the subsequent induction of CSC potential could be a novel strategy for therapeutic intervention of cancer metastasis.
Due to their plasticity, CSCs will always be activated or recreated on the condition that the CSC niche remains intact. Thus, a better understanding of the underlying genetic and epigenetic factors in the niche where cancer cells adopt phenotypic plasticity could help us to effectively control cancer progression and recurrence (O’Brien-Ball and Biddle, 2017). For example, a cancer pre-metastatic niche, like the MPE, may function as a dynamic reservoir for invasive cancer cells, where cancer cells may establish a malignant cytokine network for their survival and metastasis (Giarnieri et al., 2015; Bruschini et al., 2020). In our current study, we revealed that the levels of eCyPA in the conditioned medium of detachment cultured cells were significantly higher than that in cells growing under the attached condition; while the addition of recombinant CyPA promoted the conversion of cancer cells to CSCs in a CD147-dependent manner. Previous studies showed that IL-6 can induce conversion of non-CSCs to CSCs (Iliopoulos et al., 2011; Kim et al., 2013), but we did not detect any increases in IL-6 levels in our experimental conditions (data not shown). Although cytoplasmic CyPA is reported to play a role in maintaining glioma CSC stemness (Wang et al., 2017), our study is the first report of such effort to focus on the effects of extracellular secreted CyPA on induction of CSC potential. Unfortunately, it remains unknown how and why non-CSCs were able to produce eCyPA and overexpress CD147 upon detachment. Future studies are needed to identify the upstream signaling cascade that triggers the expression and activation of eCyPA and CD147 in breast cancer cells growing under the detached condition.
Previous studies revealed that high CD147 expression on cancer cells is linked to CSC characteristics, such as EMT, anoikis-resistance, and chemoresistance (Ma et al., 2010; Wu et al., 2011; Ke et al., 2012; Dai et al., 2013), indicating that cancer cells expression high levels of CD147 could be more aggressive and prone to cancer metastasis and recurrence. Indeed, our previous findings indicated that anti-CD147 HAb18IgG sensitized pancreatic cancer cells to chemo-radiotherapy and reduced CSC potential by inhibiting pSTAT3 signaling (Fan et al., 2019), and the CD147 antibody drug, namely, Licartin, was successfully used to prevent tumor recurrence after liver transplantation or radiofrequency ablation in advanced hepatocellular carcinoma patients (Xu et al., 2007a; Bian et al., 2014). Here, we demonstrate that CD147 expression on breast cancer cells was upregulated when culturing cells under a detached condition, and CD147 expression boosted the detachment-induced conversion of non-CSCs to CSCs by activating STAT3-SOX2 signaling. In this regard, blocking circulating breast cancer cells with an anti-CD147 antibody could be clinically effective at suppressing the induced CSCs (or MICs), and subsequently preventing the generation of metastatic tumor mass.
Conclusion
In summary, our current study demonstrates that detachment induced breast cancer cells to express CD147 and secrete CyPA, which played an important role in the conversion of non-CSCs to CSCs through activation of the STAT3-SOX2 signaling pathway (Figure 6H). Thus, our findings uncovered a pivotal role of the eCyPA-CD147 axis in the functional link between anoikis resistance and induced CSC potential. The translational extension of these studies into the clinical setting could include the development of novel therapies of breast cancer metastasis by targeting the eCyPA–CD147 interaction.
Data Availability Statement
All datasets presented in this study are included in the article/Supplementary Material.
Ethics Statement
The animal study was reviewed and approved by the Animal Care and Use Committee of The Fourth Military Medical University.
Author Contributions
LL, J-LJ, and Z-NC conceived the study and participated in the study design, performance, coordination, and manuscript writing. YM, X-YF, L-JY, B-QX, DH, H-YC, S-JW, and L-JW obtained the samples, performed the experiments, interpreted the data, and prepared the figures. X-QW and LX revised the manuscript. All authors reviewed and approved the final manuscript.
Funding
This study was supported in part by grants from the National Basic Research Program (#2015CB553700), National Science and Technology Major Project (#2015ZX09501-009), and National Natural Science Foundation of China (#31571469 and #81872349).
Conflict of Interest
The authors declare that the research was conducted in the absence of any commercial or financial relationships that could be construed as a potential conflict of interest.
The reviewer YL declared a shared affiliation with the authors to the handling editor at the time of review.
Acknowledgments
We thank Ms. Tianjiao Zhang of the Department of Cell Biology, The Fourth Military Medical University (Xi’an, China) for assistance in animal experiments. We also thank Medjaden Bioscience Ltd. for scientific editing of this manuscript (MJD1806125).
Supplementary Material
The Supplementary Material for this article can be found online at: https://www.frontiersin.org/articles/10.3389/fcell.2020.543856/full#supplementary-material
References
Al-Hajj, M., Wicha, M. S., Benito-Hernandez, A., Morrison, S. J., and Clarke, M. F. (2003). Prospective identification of tumorigenic breast cancer cells. Proc. Natl. Acad. Sci. U.S.A. 100, 3983–3988. doi: 10.1073/pnas.0530291100
Batlle, E., and Clevers, H. (2017). Cancer stem cells revisited. Nat. Med. 23, 1124–1134. doi: 10.1038/nm.4409
Bian, H., Zheng, J. S., Nan, G., Li, R., Chen, C., Hu, C. X., et al. (2014). Randomized trial of [131I] metuximab in treatment of hepatocellular carcinoma after percutaneous radiofrequency ablation. J. Natl. Cancer Inst. 106:dju239. doi: 10.1093/jnci/dju239
Bruschini, S., di Martino, S., Pisanu, M. E., Fattore, L., De Vitis, C., Laquintana, V., et al. (2020). CytoMatrix for a reliable and simple characterization of lung cancer stem cells from malignant pleural effusions. J. Cell Physiol. 235, 1877–1887. doi: 10.1002/jcp.29121
Celia-Terrassa, T., and Kang, Y. (2016). Distinctive properties of metastasis-initiating cells. Genes Dev. 30, 892–908. doi: 10.1101/gad.277681.116
Chen, X., Liao, R., Li, D., and Sun, J. (2017). Induced cancer stem cells generated by radiochemotherapy and their therapeutic implications. Oncotarget 8, 17301–17312. doi: 10.18632/oncotarget.14230
Clarke, M. F. (2019). Clinical and therapeutic implications of cancer stem cells. N. Engl. J. Med. 380, 2237–2245. doi: 10.1056/NEJMra1804280
Cortes-Hernandez, L. E., Eslami, S. Z., and Alix-Panabieres, C. (2019). Circulating tumor cell as the functional aspect of liquid biopsy to understand the metastatic cascade in solid cancer. Mol. Aspects Med. 72:100816. doi: 10.1016/j.mam.2019.07.008
Dai, L., Guinea, M. C., Slomiany, M. G., Bratoeva, M., Grass, G. D., Tolliver, L. B., et al. (2013). CD147-dependent heterogeneity in malignant and chemoresistant properties of cancer cells. Am. J. Pathol. 182, 577–585. doi: 10.1016/j.ajpath.2012.10.011
Fan, X. Y., He, D., Sheng, C. B., Wang, B., Wang, L. J., Wu, X. Q., et al. (2019). Therapeutic anti-CD147 antibody sensitizes cells to chemoradiotherapy via targeting pancreatic cancer stem cells. Am. J. Transl. Res. 11, 3543–3554.
Fofaria, N. M., and Srivastava, S. K. (2015). STAT3 induces anoikis resistance, promotes cell invasion and metastatic potential in pancreatic cancer cells. Carcinogenesis 36, 142–150. doi: 10.1093/carcin/bgu233
Fumagalli, A., Oost, K. C., Kester, L., Morgner, J., Bornes, L., Bruens, L., et al. (2020). Plasticity of Lgr5-negative cancer cells drives metastasis in colorectal cancer. Cell Stem Cell 26, 569–578.e7. doi: 10.1016/j.stem.2020.02.008
Gammon, L., Biddle, A., Heywood, H. K., Johannessen, A. C., and Mackenzie, I. C. (2013). Sub-sets of cancer stem cells differ intrinsically in their patterns of oxygen metabolism. PLoS One 8:e62493. doi: 10.1371/journal.pone.0062493
Giarnieri, E., Bellipanni, G., Macaluso, M., Mancini, R., Holstein, A. C., Milanese, C., et al. (2015). Review: cell dynamics in malignant pleural effusions. J. Cell Physiol. 230, 272–277. doi: 10.1002/jcp.24806
Gkountela, S., and Aceto, N. (2016). Stem-like features of cancer cells on their way to metastasis. Biol. Direct. 11:33. doi: 10.1186/s13062-016-0135-4
Gkountela, S., Castro-Giner, F., Szczerba, B. M., Vetter, M., Landin, J., Scherrer, R., et al. (2019). Circulating tumor cell clustering shapes DNA methylation to enable metastasis seeding. Cell 176, 98–112.e14. doi: 10.1016/j.cell.2018.11.046
Hu, X., Ghisolfi, L., Keates, A. C., Zhang, J., Xiang, S., Lee, D. K., et al. (2012). Induction of cancer cell stemness by chemotherapy. Cell Cycle 11, 2691–2698. doi: 10.4161/cc.21021
Iliopoulos, D., Hirsch, H. A., Wang, G., and Struhl, K. (2011). Inducible formation of breast cancer stem cells and their dynamic equilibrium with non-stem cancer cells via IL6 secretion. Proc. Natl. Acad. Sci. U.S.A. 108, 1397–1402. doi: 10.1073/pnas.1018898108
Ke, X., Li, L., Dong, H. L., and Chen, Z. N. (2012). Acquisition of anoikis resistance through CD147 upregulation: a new mechanism underlying metastasis of hepatocellular carcinoma cells. Oncol. Lett. 3, 1249–1254. doi: 10.3892/ol.2012.658
Kim, S. Y., Kang, J. W., Song, X., Kim, B. K., Yoo, Y. D., Kwon, Y. T., et al. (2013). Role of the IL-6-JAK1-STAT3-Oct-4 pathway in the conversion of non-stem cancer cells into cancer stem-like cells. Cell Signal. 25, 961–969. doi: 10.1016/j.cellsig.2013.01.007
Li, L., Tang, W., Wu, X., Karnak, D., Meng, X., Thompson, R., et al. (2013). HAb18G/CD147 promotes pSTAT3-mediated pancreatic cancer development via CD44s. Clin. Cancer Res. 19, 6703–6715. doi: 10.1158/1078-0432.CCR-13-0621
Li, T., Mello-Thoms, C., and Brennan, P. C. (2016). Descriptive epidemiology of breast cancer in China: incidence, mortality, survival and prevalence. Breast Cancer Res. Treat. 159, 395–406. doi: 10.1007/s10549-016-3947-0
Li, Y., Xu, J., Chen, L., Zhong, W. D., Zhang, Z., Mi, L., et al. (2009). HAb18G (CD147), a cancer-associated biomarker and its role in cancer detection. Histopathology 54, 677–687. doi: 10.1111/j.1365-2559.2009.03280.x
Ma, X. K., Wang, L., Li, Y., Yang, X. M., Zhao, P., Tang, H., et al. (2010). HAb18G/CD147 cell-cell contacts confer resistance of a HEK293 subpopulation to anoikis in an E-cadherin-dependent manner. BMC Cell Biol. 11:27. doi: 10.1186/1471-2121-11-27
May, A. N., Crawford, B. D., and Nedelcu, A. M. (2018). In vitro model-systems to understand the biology and clinical significance of circulating tumor cell clusters. Front. Oncol. 8:63. doi: 10.3389/fonc.2018.00063
O’Brien-Ball, C., and Biddle, A. (2017). Reprogramming to developmental plasticity in cancer stem cells. Dev. Biol. 430, 266–274. doi: 10.1016/j.ydbio.2017.07.025
Oskarsson, T., Batlle, E., and Massague, J. (2014). Metastatic stem cells: sources, niches, and vital pathways. Cell Stem Cell 14, 306–321. doi: 10.1016/j.stem.2014.02.002
Pece, S., Tosoni, D., Confalonieri, S., Mazzarol, G., Vecchi, M., Ronzoni, S., et al. (2010). Biological and molecular heterogeneity of breast cancers correlates with their cancer stem cell content. Cell 140, 62–73. doi: 10.1016/j.cell.2009.12.007
Peitzsch, C., Cojoc, M., Hein, L., Kurth, I., Mabert, K., Trautmann, F., et al. (2016). An Epigenetic reprogramming strategy to resensitize radioresistant prostate cancer cells. Cancer Res. 76, 2637–2651. doi: 10.1158/0008-5472.CAN-15-2116
Peitzsch, C., Tyutyunnykova, A., Pantel, K., and Dubrovska, A. (2017). Cancer stem cells: the root of tumor recurrence and metastases. Semin. Cancer Biol. 44, 10–24. doi: 10.1016/j.semcancer.2017.02.011
Quaglino, E., Conti, L., and Cavallo, F. (2020). Breast cancer stem cell antigens as targets for immunotherapy. Semin. Immunol. 47:101386. doi: 10.1016/j.smim.2020.101386
Tang, J., Guo, Y. S., Zhang, Y., Yu, X. L., Li, L., Huang, W., et al. (2012). CD147 induces UPR to inhibit apoptosis and chemosensitivity by increasing the transcription of Bip in hepatocellular carcinoma. Cell Death Differ. 19, 1779–1790. doi: 10.1038/cdd.2012.60
Visvader, J. E., and Lindeman, G. J. (2012). Cancer stem cells: current status and evolving complexities. Cell Stem Cell 10, 717–728. doi: 10.1016/j.stem.2012.05.007
Wang, G., Shen, J., Sun, J., Jiang, Z., Fan, J., Wang, H., et al. (2017). Cyclophilin A maintains Glioma-initiating cell stemness by regulating Wnt/beta-catenin signaling. Clin. Cancer Res. 23, 6640–6649. doi: 10.1158/1078-0432.CCR-17-0774
Wu, B., Cui, J., Yang, X. M., Liu, Z. Y., Song, F., Li, L., et al. (2017). Cytoplasmic fragment of CD147 generated by regulated intramembrane proteolysis contributes to HCC by promoting autophagy. Cell Death Dis. 8:e2925. doi: 10.1038/cddis.2017.251
Wu, J., Ru, N. Y., Zhang, Y., Li, Y., Wei, D., Ren, Z., et al. (2011). HAb18G/CD147 promotes epithelial-mesenchymal transition through TGF-beta signaling and is transcriptionally regulated by Slug. Oncogene 30, 4410–4427. doi: 10.1038/onc.2011.149
Wu, X., Tang, W., Marquez, R. T., Li, K., Highfill, C. A., He, F., et al. (2016). Overcoming chemo/radio-resistance of pancreatic cancer by inhibiting STAT3 signaling. Oncotarget 7, 11708–11723. doi: 10.18632/oncotarget.7336
Xu, B. Q., Fu, Z. G., Meng, Y., Wu, X. Q., Wu, B., Xu, L., et al. (2016). Gemcitabine enhances cell invasion via activating HAb18G/CD147-EGFR-pSTAT3 signaling. Oncotarget 7, 62177–62193. doi: 10.18632/oncotarget.11405
Xu, J., Lu, Y., Qiu, S., Chen, Z. N., and Fan, Z. (2013). A novel role of EMMPRIN/CD147 in transformation of quiescent fibroblasts to cancer-associated fibroblasts by breast cancer cells. Cancer Lett. 335, 380–386. doi: 10.1016/j.canlet.2013.02.054
Xu, J., Shen, Z. Y., Chen, X. G., Zhang, Q., Bian, H. J., Zhu, P., et al. (2007a). A randomized controlled trial of Licartin for preventing hepatoma recurrence after liver transplantation. Hepatology 45, 269–276. doi: 10.1002/hep.21465
Xu, J., Xu, H. Y., Zhang, Q., Song, F., Jiang, J. L., Yang, X. M., et al. (2007b). HAb18G/CD147 functions in invasion and metastasis of hepatocellular carcinoma. Mol. Cancer Res. 5, 605–614. doi: 10.1158/1541-7786.MCR-06-0286
Yong, Y. L., Zhang, R. Y., Liu, Z. K., Wei, D., Shang, Y. K., Wu, J., et al. (2019). Gamma-secretase complex-dependent intramembrane proteolysis of CD147 regulates the Notch1 signaling pathway in hepatocellular carcinoma. J. Pathol. 249, 255–267. doi: 10.1002/path.5316
Zhao, P., Zhang, W., Wang, S. J., Yu, X. L., Tang, J., Huang, W., et al. (2011). HAb18G/CD147 promotes cell motility by regulating annexin II-activated RhoA and Rac1 signaling pathways in hepatocellular carcinoma cells. Hepatology 54, 2012–2024. doi: 10.1002/hep.24592
Keywords: breast cancer, detachment culture, induced cancer stem cells, CD147, CyPA
Citation: Meng Y, Fan X-Y, Yang L-J, Xu B-Q, He D, Xu Z, Wu D, Wang B, Cui H-Y, Wang S-J, Wang L-J, Wu X-Q, Jiang J-L, Xu L, Chen Z-N and Li L (2020) Detachment Activated CyPA/CD147 Induces Cancer Stem Cell Potential in Non-stem Breast Cancer Cells. Front. Cell Dev. Biol. 8:543856. doi: 10.3389/fcell.2020.543856
Received: 18 March 2020; Accepted: 17 September 2020;
Published: 16 October 2020.
Edited by:
Dong-Ming Kuang, Sun Yat-sen University, ChinaReviewed by:
Dong-Ping Chen, Sun Yat-sen University, ChinaYuanyuan Lu, Fourth Military Medical University, Xi’an, China
Copyright © 2020 Meng, Fan, Yang, Xu, He, Xu, Wu, Wang, Cui, Wang, Wang, Wu, Jiang, Xu, Chen and Li. This is an open-access article distributed under the terms of the Creative Commons Attribution License (CC BY). The use, distribution or reproduction in other forums is permitted, provided the original author(s) and the copyright owner(s) are credited and that the original publication in this journal is cited, in accordance with accepted academic practice. No use, distribution or reproduction is permitted which does not comply with these terms.
*Correspondence: Ling Li, liling25@fmmu.edu.cn; Zhi-Nan Chen, znchen@fmmu.edu.cn
†These authors have contributed equally to this work