Non-syndromic Cleft Palate: An Overview on Human Genetic and Environmental Risk Factors
- 1Department of Experimental, Diagnostic and Specialty Medicine, Alma Mater Studiorum – University of Bologna, Bologna, Italy
- 2Department of Morphology, Surgery and Experimental Medicine, University of Ferrara, Ferrara, Italy
The epithelial and mesenchymal cells involved in early embryonic facial development are guided by complex regulatory mechanisms. Any factor perturbing the growth, approach and fusion of the frontonasal and maxillary processes could result in orofacial clefts that represent the most common craniofacial malformations in humans. The rarest and, probably for this reason, the least studied form of cleft involves only the secondary palate, which is posterior to the incisive foramen. The etiology of cleft palate only is multifactorial and involves both genetic and environmental risk factors. The intention of this review is to give the reader an overview of the efforts made by researchers to shed light on the underlying causes of this birth defect. Most of the scientific papers suggesting potential environmental and genetic causes of non-syndromic cleft palate are summarized in this review, including genome-wide association and gene–environment interaction studies.
Introduction
Orofacial clefts are the most common orofacial malformations in humans and include cleft lip (CL), cleft lip with or without cleft palate (CL/P), and cleft palate only (CPO). CPO (MIM 119540) is a birth defect that occurs when only the secondary palate is involved and can affect the hard palate and/or the soft palate, sometimes limited to one cleft uvula. It represents one third of all oral clefts and affects about 1 to 25 per 10.000 newborns worldwide (Mossey et al., 2009). The incidence of CPO is highly influenced by ethnicity and race, with the highest rates observed in non-Hispanic Whites and the lowest in Africans (Mossey and Modell, 2012). Besides, females are more prone to the defect than males (1:1.075) (Mossey and Catilla, 2003).
Cleft palate only is a multifactorial disorder influenced by both genetic and environmental factors that act during palatogenesis (Meng et al., 2009; Dixon et al., 2011). Moreover, the local changes in growth factors, extracellular matrix (ECM), and cell adhesion molecules may also play a part in CPO onset. A variety of signaling pathways are involved in palate development and several mutations on developmental genes that have been found to contribute to CPO will be discussed.
Furthermore, the environmental contribution to CPO by tobacco, alcohol and multivitamin supplementation has been highlighted by epidemiological studies hereinafter detailed.
Classification
Cleft lip with or without cleft palate and CPO are considered as different congenital malformations, having distinct embryologic origins and recurrence risks. Hence, CPO refers to any cleft of the palate which is posterior to the palatine foramen, and which does not involve the alveolar process or lip. A number of different classifications have been proposed since Veau’s version in 1931 (Veau, 1931; Danescu et al., 2015). In Figure 1, a collection of different types of CPO, including the recently introduced submucous microform (Danescu et al., 2015).
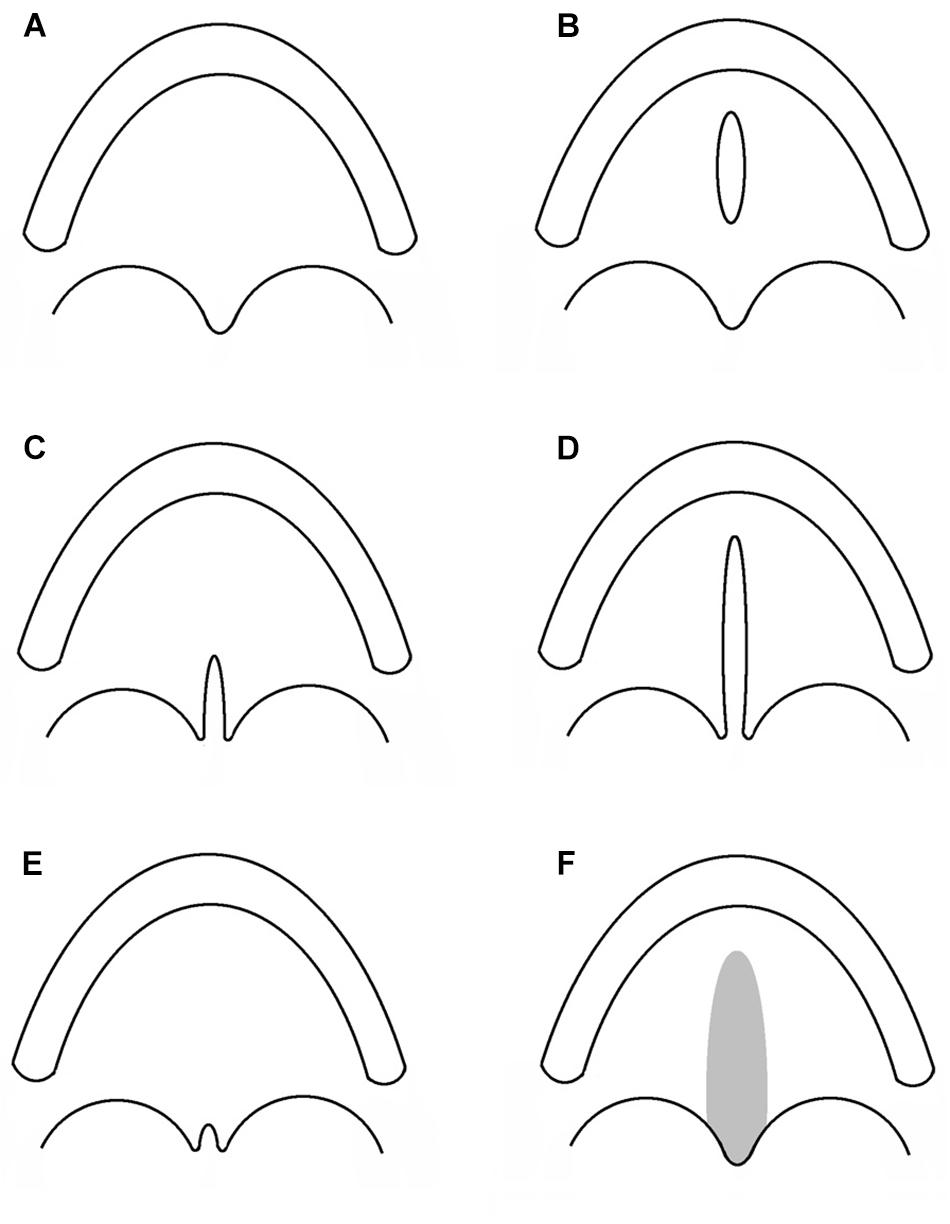
Figure 1. Subtypes of cleft palate only. Each sketch illustrates the alveolar process and the palatal rim. (A) Normal palate. (B) Cleft of the hard palate. (C) Cleft of the soft palate. (D) Cleft of the hard and soft palate. (E) Cleft uvula. (F) Submucous cleft palate.
Another kind of classification divides CPO into two major groups: (i) isolated CPO or non-syndromic CPO (NSCPO), when clefting is an isolated defect unassociated with any other additional anomalies, representing 54.8% of the total CPO cases; (ii) syndromic CPO, when clefting is associated with other anomalies in a recognizable (27.2%), or unrecognizable syndrome (18%) (Calzolari et al., 2004).
Although the presence of an evident pattern of anomalies makes the diagnosis easier, some features may not be fully expressed or could represent subclinical phenotypes more difficult to diagnose. Most frequently, the defects associated with CPO affect the heart (31.1%), the encephalon (hydrocephaly 11.2%), the urinary tract (9.7%), or digits (polydactyly 9.2%) (Mossey and Catilla, 2003). The number of syndromes involving cleft palate, among orofacial clefts, is subject to progressive implementation but already in 1978 it was quoted as 77 (Cohen, 1978).
The most common syndromes in which cleft palate deformity represents one of the features are: Apert Syndrome, Crouzon Syndrome, DiGeorge Syndrome, Loeys-Dietz Syndrome, Treacher Collins Syndrome, X-linked cleft palate Syndrome, Ankyloglossia, Bamforth-Lazarus Syndrome1. An exhaustive overview of the syndromes that count cleft palate among their features, as well as the responsible genes, can be found in a paper by Burg et al. (2016).
Palatogenesis
The human palate separates the nasal and oral cavities and consists of a bony hard palate (the anterior two thirds) and a fibromuscular soft palate (the posterior one third). The incisive foramen divides the hard palate into primary and secondary portions. The secondary portion separates the nasal passage from the pharynx and is marked by a median palatine raphe. The soft palate, or velum, is a mobile muscular fold forming a curtain posterior to the hard palate, ending with a conical mass called the uvula. The soft palate separates the nasopharynx from the oropharynx.
Palatal development takes place between the fifth and twelfth embryonic weeks, with the most critical period occurring during the sixth to ninth weeks (Merritt, 2005), when the lateral palatine processes fuse, after the fusion of the secondary palate to the primary palate with a junction marked by the incisive foramen. The palate body is composed by mesenchymal cells, mainly derived from migrating neural crest cells, layered by epithelial cells that surround the mesenchyme (Ito et al., 2003). The correct growth, migration, transition, differentiation, and apoptosis of these cells form the basis of a regular development of the palate. Palatal shelves, initially grown in a vertical position, have to rotate from the sides to the top of the tongue in order to acquire a horizontal position. Then, shelves approach and fuse with each other in an antero-posterior direction in the transient midline epithelial seam (Ferguson, 1988; Meng et al., 2009).
Conceivably, there are several molecular, mechanical and morphological steps, including the key step of epithelial to mesenchymal transition, involved in the orchestra of palate development which could go wrong (Diewert and Lozanoff, 2002). Clefting of the secondary palate may then arise in any one of these critical steps, and may be caused by failure in growth, elevation, adhesion or fusion of the palatal shelves.
Epidemiology
Cleft palate affects newborns with a world prevalence of about 3.1/10,000, which significantly varies depending on the geographical location. According to the Global Burden of Disease (GBD) study, the highest rates of CPO are found in the Oceanic continent, and the lowest in Sub-Saharan Africa, with overall values ranging from 0.4 to 11.3 every 10,000 births (Mossey and Modell, 2012). Nevertheless, there are very few epidemiological studies about OFC in African populations and estimated values of CPO incidence may not be accurate. Moreover, even inside the same continent, CPO prevalence values vary among different geographical areas, as in Europe. In fact, according to the EUROCAT registries Finland and Malta show the highest rates while Portugal and Romania the lowest, with more than three-times difference in rates (EUROCAT).
Besides geographical differences, there is also a disparity in the CPO incidence between genders. In fact, while for isolated CL/P cases the sex ratio was estimated at 1.81 (95% CI, 1.75–1.86), isolated CPO counts more females than males, with a sex ratio of 0.93 (95% CI, 0.89–0.96) in the world (Mossey and Catilla, 2003) and reported as 0.78 in Europe (Calzolari et al., 2004). This discrepancy is probably due to the delay of 1 week in the fusion of the palatal shelves in female embryos compared to males (Burdi and Faist, 1967). Possibly, in this period of time the morphogenesis could be more influenced by teratogens, thus justifying the higher CPO rate in girls.
Etiology
Environmental Factors
The complex etiology of NSCPO can be explained, beside genetic factors, by the intervention of unmodifiable (e.g., race/ethnicity, sex, family history of clefts) and modifiable factors, acting in the period from 1 month before through 2 months after conception. Fundamentally, maternal factors such as health/disease status, lifestyle, medication, exposure to environmental teratogens can affect/influence the intrauterine environment, more heavily during embryo development, and have been the topic of a number of studies looking at their association with NSCPO risk.
Surprisingly, it is the older age of the father that has been reported to increase the risk of NSCPO in the offspring, rather than that of the mother (Savitz et al., 1991; Bille et al., 2005) as confirmed by a meta-analysis (Herkrath et al., 2012).
Among the maternal behavioral factors, alcohol consumption during the first trimester of pregnancy has been suggested to have a correlation with oral cleft onset (Romitti et al., 2007; DeRoo et al., 2008). However, there are few evidences supporting a role in the CP etiology (DeRoo et al., 2008; Sabbagh et al., 2015). A recent meta-analysis (Yin et al., 2019) concluded that there is no evidence of a correlation between moderate periconceptional alcohol consumption and the risk of both non-syndromic CL/P and CP in infants. However, authors suggest that the potential risk of binge-drinking cannot be excluded, because of the heterogeneity of the threshold level for alcohol consumption in the different investigations and of the limited sample sizes of heavy drinker mother cohorts.
Also maternal tobacco smoking has been deeply investigated as an NSCPO risk factor. The studies were recently considered in two meta-analyses, which concluded by supporting a role for both maternal tobacco smoking (Little et al., 2004) and passive smoking (Sabbagh et al., 2015) in NSCPO etiology. Nevertheless, it should be taken into account that the results of meta-analyses could be biased because the sample size, ethnicity, and consumption levels vary widely from one study to another. Moreover, the alcohol and tobacco teratogenic dose-response effect is still a matter of debate.
A recent study examined the effect of maternal exposure to water disinfection by-products (DBP), a heterogeneous group of compounds that can originate from a combination of chemical disinfectants (e.g., chlorine, chloramine) and organic matter present in water (Kaufman et al., 2018). Studying the association between DBP and the risk of craniofacial malformation, the authors obtained evidence that some DBPs (specifically: DBP9, HAA5, trichloroacetic acid, and dichloroacetic acid) can increase the risk of NSCPO. Similarly, a previous study suggested an increased risk of NSCPO as a result of maternal exposure to total trihalomethanes, but at lower ranges than those of the above-mentioned work (Hwang et al., 2008). Moreover, nitrate intake from drinking water was seen to increase the risk of NSCPO in a large cohort study (OR, 1.9; 95% CI, 1.17–3.09) (Brender et al., 2013).
Since the nutritional status of the embryo is fully dependent on maternal food intake and metabolism, unbalanced maternal nutrition during the first trimester of pregnancy can lead to birth defects. Several essential micronutrients, substances that cannot be synthesized by our body in sufficient amount, are needed for health maintenance, pregnancy progression and normal embryonic development. In case of maternal nutrition deficiencies, supplementation during pregnancy is strongly advised. It is commonly accepted that folic acid-fortified multivitamin supplementation before conception and continuing through the first trimester reduces the overall occurrence of several congenital anomalies. However, only few systematic reviews or meta-analyses regarding orofacial clefts have been conducted and findings on cleft palate are inconclusive. A systematic review analyzed data from five clinical trials in order to evaluate if supplementation with folic acid, alone or in combination with vitamins and minerals, can prevent the occurrence of neural tube defects (NTD) and other birth defects. No evidence of any preventive or negative effects on cleft palate by periconceptional oral folate supplementation was detected (RR, 0.73; 95% CI, 0.05–10.89) (De-Regil et al., 2015). A reduced risk of NSCPO resulted in a meta-analysis of case–control studies for mothers who took multivitamin supplementation starting from before pregnancy (OR, 0.76; 95% CI, 0.62–0.93) (Ingrid Goh et al., 2006). An observational study reported that after food grain fortification with folic acid, the prevalence of NSCPO in United States had a significant 12% reduction (Canfield et al., 2005). On the other hand, Sutton et al. (2011) measured levels of red cell folate (RCF), vitamin B12 and homocysteine (tHcy) in the blood of women who were pregnant with a malformed baby at a time when multivitamin supplementation or food fortification was still rare. They found that the level of B12, which plays a critical role in folate metabolism, was surprisingly higher in mothers expecting a baby with cleft palate than in mothers of unmalformed offspring, with no differences in levels of RCF and tHcy (Sutton et al., 2011).
Retinoic acid (RA), a derivate of Vitamin A, is an important regulator of processes that occur during embryogenesis, such as proliferation, differentiation, and apoptosis (Finnell et al., 2004). Only few epidemiological studies on human maternal intake of Vitamin A are available (Ackermans et al., 2011). Nevertheless, an excess of Vitamin A is considered teratogenic, causing congenital malformations, including cleft palate. On the other hand, Johansen et al. (2008) evidenced a substantial protective association between high (1.91–9.64 mg) maternal intake of Vitamin A and risk of NSCPO (adjusted OR [aOR], 0.47; 95% CI, 0.24–0.94), indicating that a deficit of Vitamin A is also to be considered a risk factor for NSCPO. The need for maternal zinc supplementation during pregnancy is still an unresolved issue with conflicting results from observational data. Higher maternal plasma zinc concentration was seen to be protective against NSCPO in a sample study of Filipino women (Tamura et al., 2005), whereas the same authors did not confirm the association in a sample study from Utah (Munger et al., 2009), probably attributable to the fact that zinc status in US mothers is not compromised to a certain severity as commonly seen in the Philippines.
Medicament intake during the periconceptional/first trimester period has long been generally ascertained to be correlated with an increased risk of adverse maternal outcomes. On the spectrum of congenital anomalies considered, cleft palate showed a significant increase in odd ratio with several treatments, including the use of inhaled β2-agonists as a medical treatment for asthma (OR, 1.63; 95% CI, 1.05–2.52) (Garne et al., 2015); use of valproic acid (OR, 5.8; 95% CI, 3.3–9.5) and carbamazepine (OR, 2.4; 95% CI, 1.1–4.5) as antiepileptic drugs (in the United States) (Gilboa et al., 2011); use of aspirin as analgesic (OR, 1.7; 95% CI, 1.0–2.9) (Hernandez et al., 2012); use of corticosteroids (OR, 5.3; 95% CI, 1.1–26.5) (Carmichael and Shaw, 1999); use of ondansetron to treat hyperemesis gravidarum (RR, 1.29; 95% CI, 1.00–1.65) (Huybrechts et al., 2018); and the use of nitrosatable amides as anti-infectives (aOR, 1.27, 95% CI, 1.00–1.62) (Brender et al., 2012).
Maternal sickness could influence the pregnancy outcome, as hypothesized for influenza, common cold and cystitis by Metneki et al. (2005). These authors highlighted the teratogenic effect of hyperthermia, which was confirmed the following year by Acs et al. (2006), who reported that the fever associated with acute respiratory infections seemed to increase the risk for posterior cleft palate in their Hungarian sample study. In 2020, in a Caucasian population-based case–control study with 751 NSCPO cases, again by Acs et al. (2020), analyzed the role of maternal diseases in the increased risk for developing NSCPO. Specifically, the authors evidenced significantly altered odd ratios for CP when mothers where affected by acute inflammatory diseases such as: influenza (OR, 1.8; 95% CI, 1.3–2.5), acute upper respiratory infections (OR, 2.5; 95% CI, 1.9–3.1), acute lower respiratory infections (OR, 2.4; 95% CI, 1.4–4.2), urogenital infections (OR, 2.0; 95% CI, 1.4–2.8), and unspecified high temperature (OR, 8.1; 95% CI, 2.9–22.6). Besides, they found a strong association with herpes simplex infection (OR, 14.8; CI, 5.7–38.5). The association between chronic maternal diseases and an increased risk for NSCPO in the offspring was observed for: Graves’ disease (OR, 4.3; 95% CI, 1.7–10.6), epilepsy (OR, 4.6; 95% CI, 2.4–8.8), migraine (OR, 2.8; 95% CI, 1.2–6.8), essential hypertension (OR, 1.7; 95% CI, 1.2–2.4) and neuro-musculoskeletal pain syndromes. However, these findings have to be considered along with the teratogenic effect of medicament assumption to alleviate the symptoms of such conditions (Acs et al., 2020). According to Acs et al. (2020) the significantly higher risk observed for anemic mothers to have an NSCPO child (OR, 1.84; 95% CI, 1.25–2.71) could be a consequence of embryonic hypoxia during the critical morphogenetic period.
A meta-analysis carried out to assess the association between maternal obesity and congenital anomalies, evidenced a barely but significantly increased risk of cleft palate (Stothard et al., 2009), a datum that was later confirmed by Block et al. (2013).
Gestational diabetes mellitus has been associated with an increased risk of cleft palate in the offspring (OR, 1.54; 95% CI, 1.01–2.37) of women with a pre-pregnancy BMI ≥ 25 kg/m2, but the authors stress the need to consider the possibility of undiagnosed type 2 diabetes mellitus and the subsequent hyperglycemia as a real cause of the embryo malformation (Correa et al., 2008). With regard to pregestational diabetes, a very large population-based birth defect case–control study, carried out this year in the United States, evidenced an association with cleft palate alone (OR, 4.3; 95% CI, 2.9–6.5), while a smaller increased risk was observed in association with gestational diabetes (OR, 1.4; 95% CI, 1.1–1.8) (Tinker et al., 2020).
Genetic Factors
Increased recurrence risk in relatives indicates a high level of heritability for NSCPO (Grosen et al., 2010). Hence, the evidence of a genetic component in the etiology of this birth defect and the challenge taken up by researchers to obtain a better understanding of the NSCPO molecular bases.
During secondary palate formation, the palatal shelves grow, approach and fuse. The cells need to activate a series of biological mechanisms, including cell migration, epithelial–mesenchymal transition, and apoptosis, necessary to remove epithelial cells from the palatal epithelia medial edge, leading to the continuity of the mesenchyme and to palatal formation. For this reason, it has been hypothesized that many genes that code for proteins involved in the formation of cytoskeleton filaments, for cell adhesion molecules, or for ECM components, may contribute, if altered, to the clefted palate phenotype (Gibbins et al., 1999; Tudela et al., 2002). Here, we browse through the association studies on genes involved in mechanisms crucial for palatal development (Table 1).
Cell–Cell Adhesion
The epithelial adhesion molecule cadherin (CDH1) participates actively in the epithelial–mesenchymal transition, the developmental step when the epithelial cells at the palatal midline disappear, allowing mesenchymal continuity and palatal fusion. Alterations in the CDH1 gene contribute to the complex events that drive orofacial cleft, as demonstrated for the onset of non-syndromic cleft lip with or without cleft palate (NSCL/P) (Letra et al., 2009). Some studies have recently hypothesized that this gene may also represent a risk factor for NSCPO (Song and Zhang, 2011; Rafighdoost et al., 2013; Song et al., 2017).
Another gene involved in cell adhesion and motility that is crucial for craniofacial development is CRISPLD2. It contains an LCCL domain, common to other proteins involved in cellular migration (Chiquet et al., 2007). CRISPLD2 product has also been shown to be important for normal migration and differentiation of neural crest cells during early palate formation (Swindell et al., 2015). A significant association between allelic variants at rs1546124 and rs4783099 in the CRISPLD2 gene and the risk for NSCPO, has been reported in case–control studies (Shen et al., 2011; Gowans et al., 2016; Messetti et al., 2017).
One of the genes that participate in the signaling mechanism required for normal palate development is JAG2, which encodes a ligand for Notch family transmembrane receptors. JAG2 and NOTCH1 are spatio-temporally activated in the oral epithelia during palate development for the correct cell adhesion of the elevated palatal shelves, preventing their premature adhesion to other oral tissues (Casey et al., 2006). Nevertheless, only few studies investigated a possible involvement of JAG2 in the onset of NSCPO, with controversial results. JAG2 collaborates with IRF6 on the same molecular pathway during oral epithelial differentiation. This coordination is essential for the control of palatal adhesion and fusion competence (Richardson et al., 2009). IRF6 plays an important role in the formation and maintenance of the oral periderm, the spatiotemporal regulation of which is essential to ensure appropriate palatal adhesion (Richardson et al., 2009). Mutations in IRF6 cause two common forms of syndromic cleft, known as Van der Woude Syndrome (VWS) and popliteal pterygium syndrome (Kondo et al., 2002). The crucial role of IRF6 in the genesis of NSCL/P has been widely demonstrated in several studies. Zucchero et al. (2004), in a vast NSCL/P sample study from different countries, first demonstrated that genetic variants in IRF6, underlying VWS, might also be involved in the etiology of isolated clefts. However, although a strong association between genetic variants at IRF6 and NSCL/P risk was successively confirmed by independent studies (Blanton et al., 2005; Ghassibe et al., 2005; Scapoli et al., 2005; Srichomthong et al., 2005), the existing results regarding NSCPO are contradictory (Table 1). This could be due to the low power of the studies because of small sample sizes, but also to the widely demonstrated different etiology of NSCPO with respect to NSCL/P.
A number of scientific evidences suggest that FOXE1 is one of the most consistent genetic factors in the NSCPO etiology. Mutations of FOXE1 cause Bamforth-Lazarus, a recessive syndrome characterized by cleft palate and congenital hypothyroidism (Castanet et al., 2002). Mice lacking Foxe1 express a similar phenotype (De Felice et al., 1998). The FOXE1 is a transcription factor expressed in the shelf epithelium of the secondary palate during the fusion and regulates both MSX1 and TGFB3, which are required for proper palate formation (Venza et al., 2011). Various studies reported a strong association between FOXE1 and both NSCL/P or NSCPO (Moreno et al., 2009; Nikopensius et al., 2011; Lammer et al., 2016). No common missense mutations in FOXE1 can explain the association signal evidenced by different authors, suggesting the hypothesis of a common variant in a regulatory region (Lidral et al., 2015). Sequence analysis identified a novel non-coding polymorphism c.-1204C>G in the promoter of FOXE1 in 11 Italian NSCPOs (Venza et al., 2009), while Srichomthong et al. (2013) identified five non-synonymous mutations, none of which was present in the control population.
In craniofacial development, TGFA is expressed during the fusion of the palatine shelves at the level of the medial edge epithelium. Evidence of the involvement of TGFA in NSCPO etiology was found by several authors investigating different populations, but not by others, as detailed in Table 1.
Cell Proliferation
TCOF1 actively participates in the formation of neural crests. Alterations of this gene cause development anomalies that lead to craniofacial malformations (Dixon et al., 2006). Indeed, TCOF1 is a causative gene for Treacher Collins syndrome, characterized by hypoplasia of the facial bones, cleft palate, and middle and external ear defects. Sull et al. (2008) identified the SNP rs15251 variant as a possible risk factor for NSCPO.
The homeobox gene MSX1 actively participates in cell proliferation, differentiation, and apoptosis, all crucial processes for normal embryonic development. This gene plays a critical role in the epithelial–mesenchymal interaction during the formation of craniofacial bones (Satokata and Maas, 1994). Many studies on animal models have amply demonstrated MSX1 involvement in palatal, facial and dental development (Satokata and Maas, 1994; Jumlongras et al., 2001). Genetic studies conducted on humans have ascertained a specific role for MSX1 in the orofacial cleft (Jezewski et al., 2003; Modesto et al., 2006; Tasanarong et al., 2019). However, the association studies specifically dedicated to NSCPO cohorts obtained conflicting evidence, possibly due to the small sample sizes and the different ethnic groups considered (Table 1). A recent meta-analysis performed by Gu et al. (2018) of investigations published till 2017, indicated the SNP rs12532, able to affect mRNA expression and stability of MSX1, as a possible risk factor for NSCPO but not for NSCL/P.
TBX22 encodes a transcription factor important for the mesenchymal proliferation and elevation of palatal shelves before their fusion. Its normal expression is related to a correct development of the palate, while TBX22 mutations cause the hereditary X-linked disorder, cleft palate with ankyloglossia (CPX, OMIM 303400) (Marcano et al., 2004; Stanier and Moore, 2004). Marcano et al. (2004) screened for TBX22 mutations in 238 NSCPOs from the Philippines, North America, and Brazil, and found 15 different variants: five mutations affecting the coding region, as well as several putative splice site mutations. Suphapeetiporn et al. (2007) by sequencing the TBX22 gene in 53 NSCPO cases in the Thai population, found three missense mutations and a deletion in four affected individuals. Fu et al. (2015) reported a functional -73G>A mutation in the promoter of TBX22, in all five affected males of a six-generation Chinese family of NSCPO (Fu et al., 2015). Both TBX22 and MSX1 are post-translationally modified by SUMO1, a regulating factor that participates in the modulation of many other genes, with evidence of a role in human oral clefts (Dobreva et al., 2003; Ghioni et al., 2005; Lee et al., 2006; Andreou et al., 2007).
Shi et al. (2009) identified a de novo deletion on chromosome 2 in a Danish child affected by NSCPO. The estimated size of the deletion is 124.2 kb and contains SUMO1 (Shi et al., 2009). In a large Irish study population composed by 383 NSCPO patients, the SNP rs3769817 located in SUMO1 intron 2 was associated with an increased risk for cleft palate (Carter et al., 2010).
The PAX transcription factors are critical for neural crest induction (Monsoro-Burq, 2015). In particular, Pax7 regulates the cell cycle of embryonic stem cells and mouse embryonic fibroblasts (Czerwinska et al., 2016). The intronic rs742071 PAX7 polymorphism, previously proposed as a risk factor for NSCL/P (Ludwig et al., 2012), was also found significantly associated with NSCPO in a family-based association study carried out with 266 European triads (Bohmer et al., 2013). The association with NSCPO was then confirmed in the Chinese and sub-Saharan African populations (Duan et al., 2017; Butali et al., 2019).
Another transcriptional factor widely involved in the processes of closure of the neural tube and craniofacial development is encoded by GRHL3. In families that do not show mutations at the IRF6 locus, GRHL3 seems to be the second candidate gene in VWS (Peyrard-Janvid et al., 2014). Functional analyses demonstrate that GRHL3 is a target of IRF6 in the processes of periderm differentiation (de la Garza et al., 2013). In a genome-wide association study, Leslie et al. (2016) identified an association between the rs41268753 SNP and NSCPO in two independent populations. The importance of GRHL3 in NSCPO etiology was independently confirmed by Mangold et al. (2016), who collected evidences supporting the non-synonymous polymorphism rs41268753 as a susceptibility factor for NSCPO and described rare mutations of this gene in patients. COL2A1 is a cartilage-specific marker involved in cranial neural crest differentiation by complex mechanisms that involve epithelial–mesenchymal transition, migration, and differentiation (Ghassibe-Sabbagh et al., 2011). Mutations in genes coding for cartilage collagens II cause syndromes that are often associated with cleft palate or micrognathia, such as Pierre-Robin sequence and Stickler syndrome (Kannu et al., 2012). Evidence of an association between COL2A1 alleles and NSCPO risk was found (Nikopensius et al., 2010), though not confirmed by others (Machado et al., 2016).
Cell Migration
The two Rho kinase isoforms (ROCK1 and ROCK2) play essential roles in fundamental cellular processes such as contraction, adhesion, migration, apoptosis, and proliferation. ROCK regulates stress fiber and focal adhesion assembly (Phillips et al., 2012) and modulates cytoskeleton organization by phosphorylating different substrates, mainly myosin light chain and myosin phosphatase, involved in the contractility pathway that leads to normal palate development. A recent family-based association study carried out in two cohorts from Italy and Iran, showed a significant level of association between ROCK1 rs35996865-G and NSCPO (Palmieri et al., 2020). Currently, there are no reports demonstrating the involvement of ROCK2 in the onset of orofacial cleft, however, some studies show that the loss of both chromosome 18q and 2p (where ROCK1 and ROCK2 reside, respectively) determines a series of anomalies including defects of the palate and micrognathia (Suzuki et al., 2016).
FLNB belongs to a family of actin-binding proteins. Filamins are also able to interact with receptors and intracellular proteins involved in cytoskeleton-dependent cell proliferation, differentiation, and migration (Hu et al., 2014). Mutations in this gene cause malformations that include cleft palate as a feature, as reported by Jugessur et al. (2010) who suggest a maternal effect for variants mapping in this gene, in their NSCPO cohort (Jugessur et al., 2010).
Genes Involved in Folate and Homocysteine Metabolism
Vitamin B9 or folate is not synthesized by our organism but is present, for example, in legumes, leafy green vegetables, some fruits, and eggs. Folic acid is the most stable form of folate, synthetically produced and usually used in vitamin supplements and fortified foods (Bailey et al., 2015). Adequate folate intake is essential for cell division and homeostasis. Indeed, the folate pathway plays a crucial role in several strategic biochemical processes such as DNA biosynthesis, methionine regeneration, amino acid metabolism, mitochondrial protein translation, and DNA methylation. Folate deficiency and/or aberrant folate metabolism during embryogenesis were therefore assumed to cause congenital malformations because of the alteration of the precise interplay of cell proliferation and death, migration, and differentiation needed in this delicate moment of development. However, as above documented, there is no strong association between folate supplementation during the periconceptional period and a decreased risk of having an NSCPO baby (Johnson and Little, 2008). Nevertheless, the folate pathway, with its enzymes and substrates, was suspected to have a role in the NSCPO etiology and several studies investigated associations between polymorphisms in genes related to one-carbon metabolism and cleft palate risk. The most investigated gene is MTHFR, with its C677T polymorphism. However, in a meta-analysis based on five studies, with a total of 576 CP cases and 2587 controls, no statistical significances were observed for the risk of NSCPO for heterozygotes, neither for homozygotes (Luo et al., 2012).
Table 2 reports information on folate and homocysteine metabolism gene polymorphisms investigated for their potential association with cleft palate, published until June 2020 in PubMed. Only single studies with data from a cohort or a subset of non-syndromic cleft palate cases have been considered in the table.
Genes Responsible for Syndromic Forms as Candidate for NSCPO
As mentioned above, referring to Burg et al.’s (2016) work, the responsible genes have been identified in a number of syndromes that include cleft palate among their features. Based on the assumption that genes implicated in syndromic forms of cleft could also have a role in non-syndromic phenotypes, the variant association of such genes has been questioned in case control and family based studies on different NSCPO worldwide cohorts.
The attempts of researchers to confirm this hypothesis are summarized in Table 3, where genes already mentioned in a previous section (FLNB) or in Table 1 (TBX22, FOXE1 COL2A1, IRF6, TCOF1) are omitted.
Genome-Wide Analysis
The rapid improvement, in recent years, of technological tools for genotyping and sequencing has allowed researchers to approach whole genome studies. The aim is to identify as many susceptibility genes as possible, while the challenge is to collect and analyze dataset large enough to deliver the required statistical power. The genome-wide association studies (GWAS) approach is an indirect mapping technique, able to identify associated polymorphisms, while exome/genome sequencing (WES/WGS) studies are more focused on rare genetic mutations. Table 4 reports genes and polymorphisms associated with NSCPO detected by GWAS. Besides IRF6, an already known genetic risk factor for orofacial clefts, the list includes several new candidate genes. Among these GRHL3, whose involvement in NSCPO etiology was confirmed in an independent European cohort (Leslie et al., 2016) and by Mangold et al. (2016) in a subsequent investigation in which they evidenced common and rare causative mutations.
Whole exome sequencing (WES) searching for NSCPO mutations was performed in affected individuals from multiplex families with NSCPO (Hoebel et al., 2017). A probable deleterious mutation in ARHGAP29, a gene expressed in developing palate, was found in four individuals of a single family affected by cleft of the soft palate (Liu et al., 2017). The WES of 16 individuals from 8 multiplex families allowed Hoebel et al. (2017) to select 26 candidate genes that were sequenced in additional 132 NSCPO cases. The investigation failed to identify genes with recurrent deleterious mutations but produced data that may be useful for subsequent investigations.
The potential role of epigenetic regulation in NSCPO has been recently explored by two groups (Sharp et al., 2017; Xu et al., 2019). In these investigations, epigenome-wide association studies (EWAS) were conducted to test whether DNA methylation of blood, or other relevant tissues, was associated with orofacial clefting. These preliminary investigations suggest differences in methylation profiles of patients and unaffected controls, as well as between different types of clefts. However, future result replications may be difficult because methylation status may consistently vary as a function of kind of investigated tissue, and of patient age at the moment of sampling.
Gene × Gene Interaction
Gene–gene epistatic interaction (GxG) analysis has been successfully adopted for other diseases in order to narrow a critical region (Cox et al., 1999) or to identify additional loci (Pankratz et al., 2003). A small number of researchers tested for GxG in cohorts that do not include syndromic forms of CPO nor patients with CL. In detail, Jugessur et al. (2003a) found a significant 6.3-fold increased risk of NSCPO in patients carrying the TGFA Taq I A2A2 genotype in combination with one or two copies of the T-allele at MTHFR C677T polymorphism. This same gene–gene interaction was investigated by Mossey et al. (2017), evidencing an opposite effect when considering the combination Taq I A2 and one or two copies of MTHFR variant for the child genotype, with a 6 and 12.5-fold reduced risk, respectively. Lastly, Duan et al. (2017) evidenced an epistatic interaction between rs4844913 (43 kb 3′ of DIEXF) and rs11119388 (SYT14) and between rs6072081 (53 kb 3′ of MAFB) and rs6102085 (33 kb 3′ of MAFB).
Gene × Environment Interactions
Orofacial clefts, including NSCPO, are considered as typical complex diseases caused by several genetic and environmental factors. Although many candidate gene studies and GWAS have been performed to reveal genetic factors of NSCPO, the genetic variants identified so far can explain only a small fraction of heritability of this common malformation. This has opened the hypothesis that the effect of genetic factors on a disorder can be modulated by environmental factors and vice versa. Several different models of gene–environment interactions (GxE) were postulated; in some cases it could be easier to detect the effect of genetic factors if the effect of environmental factors is also considered (Ottman, 1996).
The first attempts to investigate GxE interaction in OFC were reviewed by Murray (2002). Most of these studies included NSCL/P cases only, or NSCL/P cases together with a small fraction of NSCPO cases. Some possible GxE interactions were suggested but not confirmed (Murray, 2002).
An interaction between the rs7205289 polymorphism and passive smoking in NSCPO was observed in the Chinese population (Li et al., 2011). The rs7205289 lies in the microRNA-140 gene and can reduce its expression (Li et al., 2011). Interestingly, tobacco smoke had the same effect in cultured mouse palatal mesenchymal cells (Li et al., 2011). This data is particularly relevant because dysregulation of miRNA-140 was found to cause palatal malformations in zebrafish by targeting Pdgfra (Eberhart et al., 2008; Li et al., 2011). The Pdgfra has a critical role in neural crest development, mesenchymal cell migration, and palatogenesis also in mice (McCarthy et al., 2016). Finally, mutations in PDGFRA were found in NSCPO patients (Rattanasopha et al., 2012).
In a GxE study on OFC cases, Shi et al. (2007) investigated the interaction between maternal smoking and 16 candidate genes involved in detoxification. An interaction was found with GSTT1 among OFC, with a higher effect in the NSCPO subgroup. In particular, the combination of fetal GSTT1-null genotype and maternal smoking provided the highest risk of cleft. This finding was important, because it confirmed a previous study involving an OFC sample from The Netherlands (van Rooij et al., 2001).
A GWAS conducted with 550 patient-parents triads supported the importance of a GxE interaction investigation. Indeed, while no individual gene reached the statistical threshold of significance in the test of association with NSCPO, several genes demonstrated an association when their interaction was considered. In particular, polymorphisms of MLLT3 and SMC2 interacted with maternal alcohol consumption; TBK1 and ZNF236 with maternal smoking; and BAALC with multivitamin supplementation (Beaty et al., 2011). Data were further examined by stratifying the sample by European and Asian ancestry (Wu et al., 2014). Several polymorphisms of a chromosome 4 region that includes SLC2A9 and WDR1 genes were associated with NSCPO in Asians when the interaction with environmental tobacco smoke exposure was tested.
The same dataset was investigated with an alternative model that includes the parent of origin (PoO) effect, i.e., the risk in the child varies on whether the allele is inherited from the mother or the father. The hypothesis of interaction between PoO and maternal exposure (PoOxE) provided the evidence for new genes involved in NSCPO etiology. Indeed, several polymorphisms in ICE2 and NAALADL2 showed PoOxSmoke interaction in European families (Haaland et al., 2017).
Discussion
Defects of the closure of the secondary palate only are, among orofacial clefts, the rarest, the least studied, and those with the least obvious nature. CPO can occur as a feature of Mendelian single gene disorders or of several chromosomal syndromes. On the other hand, the isolated form of CPO is considered to have a multifactorial etiology, and in the last decades researchers have attempted to elucidate all the teratogenic and genetic factors causing this common malformation. The identification of causal factors and understanding of the molecular mechanisms of cleft formation would be an invaluable aid for clinicians in optimizing prevention approaches, providing genetic counseling, and planning personalized surgical and medical treatments.
Several evidences have highlighted the fact that NSCPO and NSCL/P are different malformations with different, or slightly overlapping, causes. Scientific research has been mainly focused on NSCL/P, while NSCPO study has been marginal, maybe owing to the lower incidence that makes harder to collect cohorts of comparable size. In recent years, this gap has been reducing, but new insights on CPO are accumulating more slowly than expected, especially with regards GWAS. A possible explanation is a limited contribution of common genetic variants in NSCPO etiology, which lower the success rate of association analyses. Genetic investigations have provided convincing evidences for a limited number of heritable factors that do not account for the high level of hereditability of NSCPO. These include FOXE1, GRHL3, and PAX7, while a number of investigations provided support for transcription and regulatory factors necessary for the normal development of neural crests. If rare variants play a major role in CPO etiology, future large-scale sequencing efforts may represent a promising approach to detect genetic factors. Emerging trends of research regard more complex models, including gene–gene interaction, gene–environment interaction, and epigenetic analyses, which could be more powerful to explain missing heritability, but all of these require larger sample sizes. However, such approaches need a deeper investigation, in order to earlier predict NSCPO risk and to plan healthcare strategies aimed at improving environmental conditions and reducing exposure to potential epigenetic factors.
Considering the long, but not exhaustive, list of potential susceptibility factors for NSCPO, a wide range prevention could appear unrealistic, also because palate closure occurs during a gestation period in which the mother is often unaware she is pregnant. It is in any case crucial to identify exposure risks acting around the conception time (1 month before, 3 months after) that may impact embryonic development. A consistent bulk of data supports an epidemiological role for both active and passive tobacco smoking, while contrasting evidences have been collected for moderate alcohol consumption. On the contrary, folate fortification and multivitamin supplementation seem to decrease the risk of NSCPO.
Author Contributions
MM conceived the idea and design of the manuscript, acquired most of the references and data, and contributed to the drafting, revision, and final approval of the manuscript. AP and FC contributed to the manuscript design and critical revisions and final approval of the manuscript. LS contributed to the design, drafting, acquisition of statistical data, revision, and final approval of the manuscript. All authors contributed to the article and approved the submitted version.
Funding
This work was supported in part by the Alma Mater Studiorum – Università di Bologna (RFO2017_MARTINELLI_MARCELLA) and by the University of Ferrara (FIR2019 to FC).
Conflict of Interest
The authors declare that the research was conducted in the absence of any commercial or financial relationships that could be construed as a potential conflict of interest.
Supplementary Material
The Supplementary Material for this article can be found online at: https://www.frontiersin.org/articles/10.3389/fcell.2020.592271/full#supplementary-material
Footnotes
References
Ackermans, M. M., Zhou, H., Carels, C. E., Wagener, F. A., and Von den Hoff, J. W. (2011). Vitamin A and clefting: putative biological mechanisms. Nutr. Rev. 69(10), 613–624. doi: 10.1111/j.1753-4887.2011.00425.x
Acs, L., Banyai, D., Nemes, B., Nagy, K., Acs, N., Banhidy, F., et al. (2020). Maternal-related factors in the origin of isolated cleft palate-A population-based case-control study. Orthod. Craniofac. Res. 23(2), 174–180. doi: 10.1111/ocr.12361
Acs, N., Banhidy, F., Puho, E. H., and Czeizel, A. E. (2006). Acute respiratory infections during pregnancy and congenital abnormalities: a population-based case-control study. Cong. Anom 46(2), 86–96. doi: 10.1111/j.1741-4520.2006.00108.x
Andreou, A. M., Pauws, E., Jones, M. C., Singh, M. K., Bussen, M., Doudney, K., et al. (2007). TBX22 missense mutations found in patients with X-linked cleft palate affect DNA binding, sumoylation, and transcriptional repression. Am. J. Hum. Genet 81(4), 700–712. doi: 10.1086/521033
Bailey, L. B., Stover, P. J., McNulty, H., Fenech, M. F., Gregory, J. F. 3rd, Mills, J. L., et al. (2015). Biomarkers of Nutrition for Development-Folate Review. J. Nutr. 145(7), 1636S–1680S. doi: 10.3945/jn.114.206599
Beaty, T. H., Ruczinski, I., Murray, J. C., Marazita, M. L., Munger, R. G., Hetmanski, J. B., et al. (2011). Evidence for gene-environment interaction in a genome wide study of nonsyndromic cleft palate. Genet Epidemiol. 35(6), 469–478. doi: 10.1002/gepi.20595
Bezerra, J. F., Silva, H., Bortolin, R. H., Luchessi, A. D., Ururahy, M. A. G., Loureiro, M. B., et al. (2019). IRF6 polymorphisms in Brazilian patients with non-syndromic cleft lip with or without palate. Braz J. Otorhinolaryngol. 18, S1808–8694. doi: 10.1016/j.bjorl.2019.04.011
Bille, C., Skytthe, A., Vach, W., Knudsen, L. B., Andersen, A. M., Murray, J. C., et al. (2005). Parent’s age and the risk of oral clefts. Epidemiology 16(3), 311–316. doi: 10.1097/01.ede.0000158745.84019.c2
Blanton, S. H., Cortez, A., Stal, S., Mulliken, J. B., Finnell, R. H., and Hecht, J. T. (2005). Variation in IRF6 contributes to nonsyndromic cleft lip and palate. Am. J. Med. Genet A 137A(3), 259–262. doi: 10.1002/ajmg.a.30887
Block, S. R., Watkins, S. M., Salemi, J. L., Rutkowski, R., Tanner, J. P., Correia, J. A., et al. (2013). Maternal pre-pregnancy body mass index and risk of selected birth defects: evidence of a dose-response relationship. Paediatr. Peri. Epidemiol. 27(6), 521–531. doi: 10.1111/ppe.12084
Bohmer, A. C., Mangold, E., Tessmann, P., Mossey, P. A., Steegers-Theunissen, R. P., Lindemans, J., et al. (2013). Analysis of susceptibility loci for nonsyndromic orofacial clefting in a European trio sample. Am. J. Med. Genet A 161A(10), 2545–2549. doi: 10.1002/ajmg.a.36141
Boyles, A. L., Wilcox, A. J., Taylor, J. A., Meyer, K., Fredriksen, A., Ueland, P. M., et al. (2008). Folate and one-carbon metabolism gene polymorphisms and their associations with oral facial clefts. Am. J. Med. Genet A 146A(4), 440–449. doi: 10.1002/ajmg.a.32162
Boyles, A. L., Wilcox, A. J., Taylor, J. A., Shi, M., Weinberg, C. R., Meyer, K., et al. (2009). Oral facial clefts and gene polymorphisms in metabolism of folate/one-carbon and vitamin A: a pathway-wide association study. Genet Epidemiol. 33(3), 247–255. doi: 10.1002/gepi.20376
Brender, J. D., Werler, M. M., Shinde, M. U., Vuong, A. M., Kelley, K. E., and Huber, J. C. Jr., et al. (2012). Nitrosatable drug exposure during the first trimester of pregnancy and selected congenital malformations. Bir. Def. Res. A Clin. Mol. Teratol. 94(9), 701–713. doi: 10.1002/bdra.23060
Brender, J. D., Weyer, P. J., Romitti, P. A., Mohanty, B. P., Shinde, M. U., Vuong, A. M., et al. (2013). Prenatal nitrate intake from drinking water and selected birth defects in offspring of participants in the national birth defects prevention study. Environ. Health Perspect. 121(9), 1083–1089. doi: 10.1289/ehp.1206249
Burdi, A.R., and Faist, K. (1967). Morphogenesis of the palate in normal human embryos with special emphasis on the mechanisms involved. Am. J. Anat. 120, 149–159
Burg, M. L., Chai, Y., Yao, C. A., Magee, W. 3rd, and Figueiredo, J. C. (2016). Epidemiology, Etiology, and Treatment of Isolated Cleft Palate. Front. Physiol. 7:67. doi: 10.3389/fphys.2016.00067
Butali, A., Mossey, P. A., Adeyemo, W. L., Eshete, M. A., Gowans, L. J. J., Busch, T. D., et al. (2019). Genomic analyses in African populations identify novel risk loci for cleft palate. Hum. Mol. Genet. 28(6), 1038–1051. doi: 10.1093/hmg/ddy402
Butali, A., Suzuki, S., Cooper, M. E., Mansilla, A. M., Cuenco, K., Leslie, E. J., et al. (2013). Replication of genome wide association identified candidate genes confirm the role of common and rare variants in PAX7 and VAX1 in the etiology of nonsyndromic CL(P). Am. J. Med. Genet. A 161A(5), 965–972. doi: 10.1002/ajmg.a.35749
Calzolari, E., Bianchi, F., Rubini, M., Ritvanen, A., Neville, A. J., and Group, E. W. (2004). Epidemiology of cleft palate in Europe: implications for genetic research. Cleft. Palate Craniofac. J. 41(3), 244–249. doi: 10.1597/02-074.1
Canfield, M. A., Collins, J. S., Botto, L. D., Williams, L. J., Mai, C. T., Kirby, R. S., et al. (2005). Changes in the birth prevalence of selected birth defects after grain fortification with folic acid in the United States: findings from a multi-state population-based study. Birt. Def. Res. A Clin. Mol. Teratol. 73(10), 679–689. doi: 10.1002/bdra.20210
Carinci, F., Palmieri, A., Scapoli, L., Cura, F., Borelli, F., Morselli, P. G., et al. (2019). Non-syndromic cleft palate: association analysis on three gene polymorphisms of the folate pathway in Asian and Italian populations. Int, J. Immunopathol. Pharmacol. 33:2058738419858572. doi: 10.1177/2058738419858572
Carmichael, S. L., and Shaw, G. M. (1999). Maternal corticosteroid use and risk of selected congenital anomalies. Am. J. Med. Genet 86(3), 242–244.
Carter, T. C., Molloy, A. M., Pangilinan, F., Troendle, J. F., Kirke, P. N., Conley, M. R., et al. (2010). Testing reported associations of genetic risk factors for oral clefts in a large Irish study population. Bir. Def. Res. A. Clin. Mol. Teratol. 88(2), 84–93. doi: 10.1002/bdra.20639
Casey, L. M., Lan, Y., Cho, E. S., Maltby, K. M., Gridley, T., and Jiang, R. (2006). Jag2-Notch1 signaling regulates oral epithelial differentiation and palate development. Dev. Dyn. 235(7), 1830–1844. doi: 10.1002/dvdy.20821
Castanet, M., Park, S. M., Smith, A., Bost, M., Leger, J., Lyonnet, S., et al. (2002). A novel loss-of-function mutation in TTF-2 is associated with congenital hypothyroidism, thyroid agenesis and cleft palate. Hum. Mol. Genet 11(17), 2051–2059. doi: 10.1093/hmg/11.17.2051
Chevrier, C., Perret, C., Bahuau, M., Zhu, H., Nelva, A., Herman, C., et al. (2007). Fetal and maternal MTHFR C677T genotype, maternal folate intake and the risk of nonsyndromic oral clefts. Am. J. Med. Genet A 143, 248–257.
Chiquet, B. T., Lidral, A. C., Stal, S., Mulliken, J. B., Moreno, L. M., Arcos-Burgos, M., et al. (2007). CRISPLD2: a novel NSCLP candidate gene. Hum. Mol. Genet 16(18), 2241–2248. doi: 10.1093/hmg/ddm176
Correa, A., Gilboa, S. M., Besser, L. M., Botto, L. D., Moore, C. A., Hobbs, C. A., et al. (2008). Diabetes mellitus and birth defects. Am. J. Obstet. Gynecol. 199(3), e231–239. doi: 10.1016/j.ajog.2008.06.028
Cox, N. J., Frigge, M., Nicolae, D. L., Concannon, P., Hanis, C. L., Bell, G. I., et al. (1999). Loci on chromosomes 2 (NIDDM1) and 15 interact to increase susceptibility to diabetes in Mexican Americans. Nat. Genet 21(2), 213–215. doi: 10.1038/6002
Czerwinska, A. M., Nowacka, J., Aszer, M., Gawrzak, S., Archacka, K., Fogtman, A., et al. (2016). Cell cycle regulation of embryonic stem cells and mouse embryonic fibroblasts lacking functional Pax7. Cell Cycle 15(21), 2931–2942. doi: 10.1080/15384101.2016.1231260
Danescu, A., Mattson, M., Dool, C., Diewert, V. M., and Richman, J. M. (2015). Analysis of human soft palate morphogenesis supports regional regulation of palatal fusion. J. Anat. 227(4), 474–486. doi: 10.1111/joa.12365
De Felice, M., Ovitt, C., Biffali, E., Rodriguez-Mallon, A., Arra, C., Anastassiadis, K., et al. (1998). A mouse model for hereditary thyroid dysgenesis and cleft palate. Nat. Genet. 19(4), 395–398. doi: 10.1038/1289
de la Garza, G., Schleiffarth, J. R., Dunnwald, M., Mankad, A., Weirather, J. L., Bonde, G., et al. (2013). Interferon regulatory factor 6 promotes differentiation of the periderm by activating expression of Grainyhead-like 3. J. Invest. Dermatol. 133(1), 68–77. doi: 10.1038/jid.2012.269
De-Regil, L. M., Pena-Rosas, J. P., Fernandez-Gaxiola, A. C., and Rayco-Solon, P. (2015). Effects and safety of periconceptional oral folate supplementation for preventing birth defects. Cochrane. Datab. Syst. Rev. 12:CD007950. doi: 10.1002/14651858.CD007950.pub3
DeRoo, L. A., Wilcox, A. J., Drevon, C. A., and Lie, R. T. (2008). First-trimester maternal alcohol consumption and the risk of infant oral clefts in Norway: a population-based case-control study. Am. J. Epidemiol. 168(6), 638–646. doi: 10.1093/aje/kwn186
Diewert, V.M., and Lozanoff, S. (2002). “Animal models of facial clefting: experimental, congenital, and transgenic,” in Understanding Craniofacial Anomalies: The Etiopathogenesis of Craniosynostoses and Facial Clefting, eds. M.P. Mooney and M. I. Siegel (New York, NY: Wiley-Liss), 251–272.
Dixon, J., Jones, N. C., Sandell, L. L., Jayasinghe, S. M., Crane, J., Rey, J. P., et al. (2006). Tcof1/Treacle is required for neural crest cell formation and proliferation deficiencies that cause craniofacial abnormalities. Proc. Natl. Acad. Sci. U S A. 103(36), 13403–13408. doi: 10.1073/pnas.0603730103
Dixon, M. J., Marazita, M. L., Beaty, T. H., and Murray, J. C. (2011). Cleft lip and palate: understanding genetic and environmental influences. Nat. Rev. Genet. 12, 167–178.
Dobreva, G., Dambacher, J., and Grosschedl, R. (2003). SUMO modification of a novel MAR-binding protein, SATB2, modulates immunoglobulin mu gene expression. Genes. Dev. 17(24), 3048–3061. doi: 10.1101/gad.1153003
Duan, S. J., Huang, N., Zhang, B. H., Shi, J. Y., He, S., Ma, J., et al. (2017). New insights from GWAS for the cleft palate among han Chinese population. Med. Oral. Patol. Oral. Cir. Bucal. 22(2), e219–e227. doi: 10.4317/medoral.21439
Eberhart, J. K., He, X., Swartz, M. E., Yan, Y. L., Song, H., Boling, T. C., et al. (2008). MicroRNA Mirn140 modulates Pdgf signaling during palatogenesis. Nat. Genet. 40(3), 290–298. doi: 10.1038/ng.82
Fan, R., Lee, A., Lu, Z., Liu, A., Troendle, J. F., and Mills, J. L. (2013). Association analysis of complex diseases using triads, parent-child dyads and singleton monads. BMC Genet. 14:78. doi: 10.1186/1471-2156-1478
Finnell, R. H., Shaw, G. M., Lammer, E. J., Brandl, K. L., Carmichael, S. L., and Rosenquist, T. H. (2004). Gene-nutrient interactions: importance of folates and retinoids during early embryogenesis. Toxicol. Appl. Pharmacol. 198(2), 75–85. doi: 10.1016/j.taap.2003.09.031
Fu, X., Cheng, Y., Yuan, J., Huang, C., Cheng, H., and Zhou, R. (2015). Loss-of-function mutation in the X-linked TBX22 promoter disrupts an ETS-1 binding site and leads to cleft palate. Hum. Genet. 134(2), 147–158. doi: 10.1007/s00439-014-15031508
Garne, E., Hansen, A. V., Morris, J., Zaupper, L., Addor, M. C., Barisic, I., et al. (2015). Use of asthma medication during pregnancy and risk of specific congenital anomalies: a European case-malformed control study. J. Allerg. Clin. Immunol. 136(6), 1496–1502 e. doi: 10.1016/j.jaci.2015.05.043
Ghassibe, M., Bayet, B., Revencu, N., Verellen-Dumoulin, C., Gillerot, Y., Vanwijck, R., et al. (2005). Interferon regulatory factor-6: a gene predisposing to isolated cleft lip with or without cleft palate in the Belgian population. Eur. J. Hum. Genet. 13(11), 1239–1242. doi: 10.1038/sj.ejhg.5201486
Ghassibe-Sabbagh, M., Desmyter, L., Langenberg, T., Claes, F., Boute, O., Bayet, B., et al. (2011). FAF1, a gene that is disrupted in cleft palate and has conserved function in zebrafish. Am. J. Hum. Genet. 88(2), 150–161. doi: 10.1016/j.ajhg.2011.01.003
Ghioni, P., D’Alessandra, Y., Mansueto, G., Jaffray, E., Hay, R. T., La Mantia, G., et al. (2005). The protein stability and transcriptional activity of p63alpha are regulated by SUMO-1 conjugation. Cell. Cycle 4(1), 183–190. doi: 10.4161/cc.4.1.1359
Gibbins, J. R., Manthey, A., Tazawa, Y. M., Scott, B., Bloch-Zupan, A., and Hunter, N. (1999). Midline fusion in the formation of the secondary palate anticipated by upregulation of keratin K5/6 and localized expression of vimentin mRNA in medial edge epithelium. Int. J. Dev. Biol. 43, 237–244.
Gilboa, S. M., Broussard, C. S., Devine, O. J., Duwe, K. N., Flak, A. L., Boulet, S. L., et al. (2011). Influencing clinical practice regarding the use of antiepileptic medications during pregnancy: modeling the potential impact on the prevalences of spina bifida and cleft palate in the United States. Am. J. Med. Genet. C Semin. Med. Genet. 157C(3), 234–246. doi: 10.1002/ajmg.c.30306
Goffinet, L., Oussalah, A., Gueant-Rodriguez, R. M., Chery, C., Basha, M., Avogbe, P. H., et al. (2016). Cystathionine beta-synthase genetic variant rs2124459 is associated with a reduced risk of cleft palate in French and Belgian populations. J. Med. Genet 53(12), 828–834. doi: 10.1136/jmedgenet-2016104111
Gowans, L. J., Adeyemo, W. L., Eshete, M., Mossey, P. A., Busch, T., Aregbesola, B., et al. (2016). Association Studies and Direct DNA Sequencing Implicate Genetic Susceptibility Loci in the Etiology of Nonsyndromic Orofacial Clefts in Sub-Saharan African Populations. J. Dent. Res. 95(11), 1245–1256. doi: 10.1177/0022034516657003
Grosen, D., Chevrier, C., Skytthe, A., Bille, C., Molsted, K., Sivertsen, A., et al. (2010). A cohort study of recurrence patterns among more than 54,000 relatives of oral cleft cases in Denmark: support for the multifactorial threshold model of inheritance. J. Med. Genet 47(3), 162–168. doi: 10.1136/jmg.2009.069385
Gu, M., Zhang, Y., Liu, H., Liu, J., Zhu, D., and Yang, X. (2018). MSH homeobox 1 polymorphisms and the risk of non-syndromic orofacial clefts: a meta-analysis. Eur. J. Oral. Sci. 126(3), 180–185. doi: 10.1111/eos.12414
Haaland, O. A., Jugessur, A., Gjerdevik, M., Romanowska, J., Shi, M., Beaty, T. H., et al. (2017). Genome-wide analysis of parent-of-origin interaction effects with environmental exposure (PoOxE): an application to European and Asian cleft palate trios. PLoS One 12(9):e0184358. doi: 10.1371/journal.pone.0184358
He, M., and Bian, Z. (2016). Lack of Association between Missense Variants in GRHL3 (rs2486668 and rs545809) and Susceptibility to Non-Syndromic Orofacial Clefts in a Han Chinese Population. PLoS One 11(7):e0159940. doi: 10.1371/journal.pone.0159940
Herkrath, A. P., Herkrath, F. J., Rebelo, M. A., and Vettore, M. V. (2012). Parental age as a risk factor for non-syndromic oral clefts: a meta-analysis. J. Dent. 40(1), 3–14. doi: 10.1016/j.jdent.2011.10.002
Hernandez, R. K., Werler, M. M., Romitti, P., Sun, L., Anderka, M., and National Birth Defects Prevention, S. (2012). Nonsteroidal antiinflammatory drug use among women and the risk of birth defects. Am. J. Obstet. Gynecol. 206(3), e221–228. doi: 10.1016/j.ajog.2011.11.019
Hoebel, A. K., Drichel, D., van de Vorst, M., Bohmer, A. C., Sivalingam, S., Ishorst, N., et al. (2017). Candidate Genes for Nonsyndromic Cleft Palate Detected by Exome Sequencing. J. Dent. Res. 96(11), 1314–1321. doi: 10.1177/0022034517722761
Hu, J., Lu, J., Lian, G., Ferland, R. J., Dettenhofer, M., and Sheen, V. L. (2014). Formin 1 and filamin B physically interact to coordinate chondrocyte proliferation and differentiation in the growth plate. Hum. Mol. Genet. 23(17), 4663–4673. doi: 10.1093/hmg/ddu186
Huang, L., Jia, Z., Shi, Y., Du, Q., Shi, J., Wang, Z., et al. (2019). Genetic factors define CPO and CLO subtypes of nonsyndromicorofacial cleft. PLoS Genet. 15(10):e1008357. doi: 10.1371/journal.pgen.1008357
Huang, Y. Q., Ma, J., Ma, M., Deng, Y., Li, Y. D., Ren, H. W., et al. (2011). Association between MSX1 variants and oral clefts in Han Chinese in western China. DNA Cell Biol. 30(12), 1057–1061. doi: 10.1089/dna.2010.1208
Huybrechts, K. F., Hernandez-Diaz, S., Straub, L., Gray, K. J., Zhu, Y., Patorno, E., et al. (2018). Association of Maternal First-Trimester Ondansetron Use With Cardiac Malformations and Oral Clefts in Offspring. JAMA 320(23), 2429–2437. doi: 10.1001/jama.2018.18307
Hwang, B. F., Jaakkola, J. J., and Guo, H. R. (2008). Water disinfection by-products and the risk of specific birth defects: a population-based cross-sectional study in Taiwan. Environ. Health 7:23. doi: 10.1186/1476-069X-723
Ingrid Goh, Y., Bollano, E., Einarson, T. R., and Koren, G. (2006). Prenatal multivitamin supplementation and rates of congenital anomalies: a meta-analysis. J. Obstet. Gynaecol. Can. 28(8), 680–689. doi: 10.1016/S1701-2163(16)3222732227
Ito, Y., Yeo, J. Y., Chytil, A., Han, J., and Bringas, P. Jr., et al. (2003). Conditional inactivation of Tgfbr2 in cranial neural crest causes cleft palate and calvaria defects. Development 130(21), 5269–5280. doi: 10.1242/dev.00708
Jagomagi, T., Nikopensius, T., Krjutskov, K., Tammekivi, V., Viltrop, T., Saag, M., et al. (2010). MTHFR and MSX1 contribute to the risk of nonsyndromic cleft lip/palate. Eur. J. Oral. Sci. 118(3), 213–220. doi: 10.1111/j.1600-0722.2010.00729.x
Jezewski, P. A., Vieira, A. R., Nishimura, C., Ludwig, B., Johnson, M., O’Brien, S. E., et al. (2003). Complete sequencing shows a role for MSX1 in non-syndromic cleft lip and palate. J. Med. Genet. 40(6), 399–407. doi: 10.1136/jmg.40.6.399
Jia, Z. L., He, S., Jiang, S. Y., Zhang, B. H., Duan, S. J., Shi, J. Y., et al. (2017). Rs12941170 at SOX9 gene associated with orofacial clefts in Chinese. Arch. Oral. Biol. 76, 14–19. doi: 10.1016/j.archoralbio.2016.12.010
Johansen, A. M., Lie, R. T., Wilcox, A. J., Andersen, L. F., and Drevon, C. A. (2008). Maternal dietary intake of vitamin A and risk of orofacial clefts: a population-based case-control study in Norway. Am. J. Epidemiol. 167(10), 1164–1170. doi: 10.1093/aje/kwn035
Johnson, C. Y., and Little, J. (2008). Folate intake, markers of folate status and oral clefts: is the evidence converging? Int. J. Epidemiol. 37(5), 1041–1058. doi: 10.1093/ije/dyn098
Jugessur, A., Lie, R. T., Wilcox, A. J., Murray, J. C., Taylor, J. A., Saugstad, O. D., et al. (2003a). Cleft palate, transforming growth factor alpha gene variants, and maternal exposures: assessing gene-environment interactions in case-parent triads. Genet Epidemiol. 25(4), 367–374. doi: 10.1002/gepi.10268
Jugessur, A., Rahimov, F., Lie, R. T., Wilcox, A. J., Gjessing, H. K., Nilsen, R. M., et al. (2008). Genetic variants in IRF6 and the risk of facial clefts: single-marker and haplotype-based analyses in a population-based case-control study of facial clefts in Norway. Genet Epidemiol. 32(5), 413–424. doi: 10.1002/gepi.20314
Jugessur, A., Shi, M., Gjessing, H. K., Lie, R. T., Wilcox, A. J., Weinberg, C. R., et al. (2010). Maternal genes and facial clefts in offspring: a comprehensive search for genetic associations in two population-based cleft studies from Scandinavia. PLoS One 5(7):e11493. doi: 10.1371/journal.pone.0011493
Jugessur, A., Skare, O., Lie, R. T., Wilcox, A. J., Christensen, K., Christiansen, L., et al. (2012). X-linked genes and risk of orofacial clefts: evidence from two population-based studies in Scandinavia. PLoS One 7(6):e39240. doi: 10.1371/journal.pone.0039240
Jugessur, A., Wilcox, A. J., Lie, R. T., Murray, J. C., Taylor, J. A., Ulvik, A., et al. (2003b). Exploring the effects of methylenetetrahydrofolate reductase gene variants C677T and A1298C on the risk of orofacial clefts in 261 Norwegian case-parent triads. Am. J. Epidemiol. 157(12), 1083–1091. doi: 10.1093/aje/kwg097
Jumlongras, D., Bei, M., Stimson, J. M., Wang, W. F., DePalma, S. R., Seidman, C. E., et al. (2001). A nonsense mutation in MSX1 causes Witkop syndrome. Am. J. Hum. Genet 69(1), 67–74. doi: 10.1086/321271
Kannu, P., Bateman, J., and Savarirayan, R. (2012). Clinical phenotypes associated with type II collagen mutations. J. Paediatr. Child. Health 48(2), E38–43. doi: 10.1111/j.1440-1754.2010.01979.x
Kaufman, J. A., Wright, J. M., Evans, A., Rivera-Nunez, Z., Meyer, A., and Narotsky, M. G. (2018). Associations Between Disinfection By-Product Exposures and Craniofacial Birth Defects. J. Occup. Environ. Med. 60(2), 109–119. doi: 10.1097/JOM.0000000000001191
Kondo, S., Schutte, B. C., Richardson, R. J., Bjork, B. C., Knight, A. S., Watanabe, Y., et al. (2002). Mutations in IRF6 cause Van der Woude and popliteal pterygium syndromes. Nat. Genet 32(2), 285–289. doi: 10.1038/ng985
Krasone, K., Lace, B., Akota, I., Care, R., Deeley, K., Kuchler, E. C., et al. (2014). IRF6 AP-2a binding site promoter polymorphism is associated with oral clefts in Latvia. Stomatologija 16, 132–136.
Kuchler, E. C., Saboia, T. M., Vieira, T. C., Lips, A., Tannure, P. N., Deeley, K., et al. (2014). Studies of genes involved in craniofacial development and tumorigenesis: FGF3 contributes to isolated oral clefts and may interact with PAX9. Acta Odontol. Scand. 72(8), 1070–1078. doi: 10.3109/00016357.2014.903514
Lammer, E. J., Mohammed, N., Iovannisci, D. M., Ma, C., Lidral, A. C., and Shaw, G. M. (2016). Genetic variation of FOXE1 and risk for orofacial clefts in a California population. Am. J. Med. Genet A 170(11), 2770–2776. doi: 10.1002/ajmg.a.37871
Lee, H., Quinn, J. C., Prasanth, K. V., Swiss, V. A., Economides, K. D., Camacho, M. M., et al. (2006). PIAS1 confers DNA-binding specificity on the Msx1 homeoprotein. Genes Dev. 20(7), 784–794. doi: 10.1101/gad.1392006
Leslie, E. J., Liu, H., Carlson, J. C., Shaffer, J. R., Feingold, E., Wehby, G., et al. (2016). A Genome-wide Association Study of Nonsyndromic Cleft Palate Identifies an Etiologic Missense Variant in GRHL3. Am. J. Hum. Genet 98(4), 744–754. doi: 10.1016/j.ajhg.2016.02.014
Letra, A., Fakhouri, W., Fonseca, R. F., Menezes, R., Kempa, I., Prasad, J. L., et al. (2012). Interaction between IRF6 and TGFA genes contribute to the risk of nonsyndromic cleft lip/palate. PLoS One 7(9):e45441. doi: 10.1371/journal.pone.0045441
Letra, A., Menezes, R., Granjeiro, J. M., and Vieira, A. R. (2009). AXIN2 and CDH1 polymorphisms, tooth agenesis, and oral clefts. Birt. Defects. Res. A Clin. Mol. Teratol. 85(2), 169–173. doi: 10.1002/bdra.20489
Li, L., Zhu, G. Q., Meng, T., Shi, J. Y., Wu, J., Xu, X., et al. (2011). Biological and epidemiological evidence of interaction of infant genotypes at Rs7205289 and maternal passive smoking in cleft palate. Am. J. Med. Genet A 155A(12), 2940–2948. doi: 10.1002/ajmg.a.34254
Lidral, A. C., Liu, H., Bullard, S. A., Bonde, G., Machida, J., Visel, A., et al. (2015). A single nucleotide polymorphism associated with isolated cleft lip and palate, thyroid cancer and hypothyroidism alters the activity of an oral epithelium and thyroid enhancer near FOXE1. Hum. Mol. Genet 24(14), 3895–3907. doi: 10.1093/hmg/ddv047
Lidral, A. C., Murray, J. C., Buetow, K. H., Basart, A. M., Schearer, H., Shiang, R., et al. (1997). Studies of the candidate genes TGFB2, MSX1, TGFA, and TGFB3 in the etiology of cleft lip and palate in the Philippines. Cleft Palate Craniofac. J. 34, 1–6.
Lidral, A. C., Romitti, P. A., Basart, A. M., Doetschman, T., Leysens, N. J., Daack-Hirsch, S., et al. (1998). Association of MSX1 and TGFB3 with nonsyndromic clefting in humans. Am. J. Hum. Genet 63(2), 557–568. doi: 10.1086/301956
Little, J., Cardy, A., and Munger, R. G. (2004). Tobacco smoking and oral clefts: a meta-analysis. Bull. World Health Organ. 82, 213–218.
Little, J., Gilmour, M., Mossey, P. A., Fitzpatrick, D., Cardy, A., Clayton-Smith, J., et al. (2008). Folate and clefts of the lip and palate–a U.K.-based case-control study: Part II: Biochemical and genetic analysis. Cleft. Palate Craniofac. J. 45(4), 428–438. doi: 10.1597/06-151.1
Liu, H., Busch, T., Eliason, S., Anand, D., Bullard, S., Gowans, L. J. J., et al. (2017). Exome sequencing provides additional evidence for the involvement of ARHGAP29 in Mendelian orofacial clefting and extends the phenotypic spectrum to isolated cleft palate. Bir. Defects. Res. 109(1), 27–37. doi: 10.1002/bdra.23596
Liu, K., Lu, Y., Ai, L., Jiao, B., Yu, J., Zhang, B., et al. (2015). Association between FOXE1 and non-syndromic orofacial clefts in a northeastern Chinese population. Br. J. Oral. Maxillofac. Surg. 53(8), 705–710. doi: 10.1016/j.bjoms.2015.05.021
Ludwig, K. U., Bohmer, A. C., Bowes, J., Nikolic, M., Ishorst, N., Wyatt, N., et al. (2017). Imputation of orofacial clefting data identifies novel risk loci and sheds light on the genetic background of cleft lip +/- cleft palate and cleft palate only. Hum. Mol. Genet 26(4), 829–842. doi: 10.1093/hmg/ddx012
Ludwig, K. U., Bohmer, A. C., Rubini, M., Mossey, P. A., Herms, S., Nowak, S., et al. (2014). Strong association of variants around FOXE1 and orofacial clefting. J. Dent. Res. 93(4), 376–381. doi: 10.1177/0022034514523987
Ludwig, K. U., Mangold, E., Herms, S., Nowak, S., Reutter, H., Paul, A., et al. (2012). Genome-wide meta-analyses of nonsyndromic cleft lip with or without cleft palate identify six new risk loci. Nat. Genet 44(9), 968–971. doi: 10.1038/ng.2360
Luo, Y. L., Cheng, Y. L., Ye, P., Wang, W., Gao, X. H., and Chen, Q. (2012). Association between MTHFR polymorphisms and orofacial clefts risk: a meta-analysis. Bir. Defects. Res. A Clin. Mol. Teratol. 94(4), 237–244. doi: 10.1002/bdra.23005
Ma, L., Xu, M., Li, D., Han, Y., Wang, Z., Yuan, H., et al. (2014). A miRNA-binding-site SNP of MSX1 is Associated with NSOC Susceptibility. J. Dent. Res. 93(6), 559–564. doi: 10.1177/0022034514527617
Machado, R. A., Messetti, A. C., de Aquino, S. N., Martelli-Junior, H., Swerts, M. S., de Almeida Reis, S. R., et al. (2016). Association Between Genes Involved in Craniofacial Development and Nonsyndromic Cleft Lip and/or Palate in the Brazilian Population. Cleft. Palate Craniofac. J. 53(5), 550–556. doi: 10.1597/15107
Mangold, E., Bohmer, A. C., Ishorst, N., Hoebel, A. K., Gultepe, P., Schuenke, H., et al. (2016). Sequencing the GRHL3 Coding Region Reveals Rare Truncating Mutations and a Common Susceptibility Variant for Nonsyndromic Cleft Palate. Am. J. Hum. Genet 98(4), 755–762. doi: 10.1016/j.ajhg.2016.02.013
Marcano, A. C., Doudney, K., Braybrook, C., Squires, R., Patton, M. A., Lees, M. M., et al. (2004). TBX22 mutations are a frequent cause of cleft palate. J. Med. Genet 41(1), 68–74. doi: 10.1136/jmg.2003.010868
McCarthy, N., Liu, J. S., Richarte, A. M., Eskiocak, B., Lovely, C. B., Tallquist, M. D., et al. (2016). Pdgfra and Pdgfrb genetically interact during craniofacial development. Dev. Dyn. 245(6), 641–652. doi: 10.1002/dvdy.24403
Meng, L., Bian, Z., Torensma, R., and Von den Hoff, J. W. (2009). Biological mechanisms in palatogenesis and cleft palate. J. Dent. Res. 88(1), 22–33. doi: 10.1177/0022034508327868
Merritt, L. (2005). Part 1. Understanding the embryology and genetics of cleft lip and palate. Adv. Neonatal. Care 5(2), 64–71. doi: 10.1016/j.adnc.2004.12.006
Messetti, A. C., Machado, R. A., de Oliveira, C. E., Martelli-Junior, H., de Almeida Reis, S. R., Moreira, H. S., et al. (2017). Brazilian multicenter study of association between polymorphisms in CRISPLD2 and JARID2 and non-syndromic oral clefts. J. Oral. Pathol. Med. 46(3), 232–239. doi: 10.1111/jop.12470
Metneki, J., Puho, E., and Czeizel, A. E. (2005). Maternal diseases and isolated orofacial clefts in Hungary. Bir. Defec. Res. A Clin. Mol. Teratol. 73(9), 617–623. doi: 10.1002/bdra.20177
Mills, J. L., Molloy, A. M., Parle-McDermott, A., Troendle, J. F., Brody, L. C., Conley, M. R., et al. (2008). Folate-related gene polymorphisms as risk factors for cleft lip and cleft palate. Bir. Defec. Res. A Clin. Mol. Teratol. 82(9), 636–643. doi: 10.1002/bdra.20491
Modesto, A., Moreno, L. M., Krahn, K., King, S., and Lidral, A. C. (2006). MSX1 and orofacial clefting with and without tooth agenesis. J. Dent. Res. 85(6), 542–546. doi: 10.1177/154405910608500612
Monsoro-Burq, A. H. (2015). PAX transcription factors in neural crest development. Semin. Cell Dev. Biol. 44, 87–96. doi: 10.1016/j.semcdb.2015.09.015
Moreno, L. M., Mansilla, M. A., Bullard, S. A., Cooper, M. E., Busch, T. D., Machida, J., et al. (2009). FOXE1 association with both isolated cleft lip with or without cleft palate, and isolated cleft palate. Hum. Mol. Genet 18(24), 4879–4896. doi: 10.1093/hmg/ddp444
Morkuniene, A., Steponaviciut, D., Utkus, A., and Kucinskas, V. (2007). Few associations of candidate genes with nonsyndromic orofacial clefts in the population of Lithuania. J. Appl. Genet 48(1), 89–91. doi: 10.1007/BF03194663
Mossey, P. A., and Modell, B. (2012). Epidemiology of oral clefts 2012: an international perspective. Front. Oral. Biol. 16, 1–18. doi: 10.1159/000337464
Mossey, P. A., Little, J., Munger, R. G., Dixon, M. J., and Shaw, W. C. (2009). Cleft lip and palate. Lancet 374(9703), 1773–1785. doi: 10.1016/S0140-6736(09)6069560694
Mossey, P. A., Little, J., Steegers-Theunissen, R., Molloy, A., Peterlin, B., Shaw, W. C., et al. (2017). Genetic Interactions in Nonsyndromic Orofacial Clefts in Europe-EUROCRAN Study. Cleft. Palate Craniofac. J. 54(6), 623–630. doi: 10.1597/16037
Mossey, P.A., and Catilla, E.E. (2003). Global registry and database on craniofacial anomalies: report of a WHO Registry Meeting on Craniofacial Anomalies. Geneva: World Health Organization.
Munger, R. G., Tamura, T., Johnston, K. E., Feldkamp, M. L., Pfister, R., and Carey, J. C. (2009). Plasma zinc concentrations of mothers and the risk of oral clefts in their children in Utah. Bir. Defects. Res. A Clin. Mol. Teratol. 85(2), 151–155. doi: 10.1002/bdra.20516
Murray, J. C. (2002). Gene/environment causes of cleft lip and/or palate. Clin. Genet 61(4), 248–256. doi: 10.1034/j.1399-0004.2002.610402.x
Nikopensius, T., Jagomagi, T., Krjutskov, K., Tammekivi, V., Saag, M., Prane, I., et al. (2010). Genetic variants in COL2A1, COL11A2, and IRF6 contribute risk to nonsyndromic cleft palate. Bir. Defects. Res. A Clin. Mol. Teratol. 88(9), 748–756. doi: 10.1002/bdra.20700
Nikopensius, T., Kempa, I., Ambrozaityte, L., Jagomagi, T., Saag, M., Matuleviciene, A., et al. (2011). Variation in FGF1, FOXE1, and TIMP2 genes is associated with nonsyndromic cleft lip with or without cleft palate. Bir. Def. Res. A Clin. Mol. Teratol. 91(4), 218–225. doi: 10.1002/bdra.20791
Ottman, R. (1996). Gene-environment interaction: definitions and study designs. Prev. Med. 25(6), 764–770. doi: 10.1006/pmed.1996.0117
Palmieri, A., Scapoli, L., Carrozzo, M., Cura, F., Morselli, P. G., Pannuto, L., et al. (2020). ROCK1 is associated with non-syndromic cleft palate. J. Oral. Pathol. Med. 49(2), 164–168. doi: 10.1111/jop.12973
Pan, Y., Han, Y., Zhang, H., Zhou, L., Li, D., Cai, Q., et al. (2013). Association and cumulative effects of GWAS-identified genetic variants for nonsyndromic orofacial clefts in a Chinese population. Environ. Mol. Mutagen. 54(4), 261–267. doi: 10.1002/em.21773
Pankratz, N., Nichols, W. C., Uniacke, S. K., Halter, C., Murrell, J., Rudolph, A., et al. (2003). Genome-wide linkage analysis and evidence of gene-by-gene interactions in a sample of 362 multiplex Parkinson disease families. Hum. Mol. Genet 12(20), 2599–2608. doi: 10.1093/hmg/ddg270
Paranaiba, L. M., de Aquino, S. N., Bufalino, A., Martelli-Junior, H., Graner, E., Brito, L. A., et al. (2013). Contribution of polymorphisms in genes associated with craniofacial development to the risk of nonsyndromic cleft lip and/or palate in the Brazilian population. Med. Oral. Patol. Oral. Cir. Bucal. 18(3), e414–420. doi: 10.4317/medoral.18357
Pauws, E., Moore, G. E., and Stanier, P. (2009). A functional haplotype variant in the TBX22 promoter is associated with cleft palate and ankyloglossia. J. Med. Genet 46(8), 555–561. doi: 10.1136/jmg.2009.066902
Peyrard-Janvid, M., Leslie, E. J., Kousa, Y. A., Smith, T. L., Dunnwald, M., Magnusson, M., et al. (2014). Dominant mutations in GRHL3 cause Van der Woude Syndrome and disrupt oral periderm development. Am. J. Hum. Genet 94(1), 23–32. doi: 10.1016/j.ajhg.2013.11.009
Phillips, H. M., Papoutsi, T., Soenen, H., Ybot-Gonzalez, P., Henderson, D. J., and Chaudhry, B. (2012). Neural crest cell survival is dependent on Rho kinase and is required for development of the mid face in mouse embryos. PLoS One 7(5), e37685. doi: 10.1371/journal.pone.0037685
Rafighdoost, H., Hashemi, M., Narouei, A., Eskanadri-Nasab, E., Dashti-Khadivaki, G., and Taheri, M. (2013). Association between CDH1 and MSX1 gene polymorphisms and the risk of nonsyndromic cleft lip and/or cleft palate in a southeast Iranian population. Cleft. Palate Craniofac. J. 50(5), e98–e104. doi: 10.1597/12144
Rattanasopha, S., Tongkobpetch, S., Srichomthong, C., Siriwan, P., Suphapeetiporn, K., and Shotelersuk, V. (2012). PDGFRa mutations in humans with isolated cleft palate. Eur. J. Hum. Genet 20(10), 1058–1062. doi: 10.1038/ejhg.2012.55
Richardson, R. J., Dixon, J., Jiang, R., and Dixon, M. J. (2009). Integration of IRF6 and Jagged2 signalling is essential for controlling palatal adhesion and fusion competence. Hum. Mol. Genet 18(14), 2632–2642. doi: 10.1093/hmg/ddp201
Romitti, P. A., Sun, L., Honein, M. A., Reefhuis, J., Correa, A., and Rasmussen, S. A. (2007). Maternal periconceptional alcohol consumption and risk of orofacial clefts. Am. J. Epidemiol. 166(7), 775–785. doi: 10.1093/aje/kwm146
Sabbagh, H. J., Hassan, M. H., Innes, N. P., Elkodary, H. M., Little, J., and Mossey, P. A. (2015). Passive smoking in the etiology of non-syndromic orofacial clefts: a systematic review and meta-analysis. PLoS One 10(3):e0116963. doi: 10.1371/journal.pone.0116963
Satokata, I., and Maas, R. (1994). Msx1 deficient mice exhibit cleft palate and abnormalities of craniofacial and tooth development. Nat. Genet 6(4), 348–356. doi: 10.1038/ng0494-348
Savitz, D. A., Schwingl, P. J., and Keels, M. A. (1991). Influence of paternal age, smoking, and alcohol consumption on congenital anomalies. Teratology 44(4), 429–440. doi: 10.1002/tera.1420440409
Scapoli, L., Palmieri, A., Martinelli, M., Pezzetti, F., Carinci, P., Tognon, M., et al. (2005). Strong evidence of linkage disequilibrium between polymorphisms at the IRF6 locus and nonsyndromic cleft lip with or without cleft palate, in an Italian population. Am. J. Hum. Genet. 76(1), 180–183. doi: 10.1086/427344
Sharp, G. C., Ho, K., Davies, A., Stergiakouli, E., Humphries, K., McArdle, W., et al. (2017). Distinct DNA methylation profiles in subtypes of orofacial cleft. Clin. Epigenet. 9:63. doi: 10.1186/s13148-017-0362362
Shaw, G. M., Iovannisci, D. M., Yang, W., Finnell, R. H., Carmichael, S. L., Cheng, S., et al. (2005). Endothelial nitric oxide synthase (NOS3) genetic variants, maternal smoking, vitamin use, and risk of human orofacial clefts. Am. J. Epidemiol. 162(12), 1207–1214. doi: 10.1093/aje/kwi336
Shaw, G. M., Todoroff, K., Finnell, R. H., Rozen, R., and Lammer, E. J. (1999). Maternal vitamin use, infant C677T mutation in MTHFR, and isolated cleft palate risk. Am. J. Med. Genet 85, 84–85.
Shaw, G. M., Yang, W., Perloff, S., Shaw, N. M., Carmichael, S. L., Zhu, H., et al. (2013). Thymidylate synthase polymorphisms and risks of human orofacial clefts. Bir. Def. Res. A. Clin. Mol. Teratol. 97(2), 95–100. doi: 10.1002/bdra.23114
Shaw, G. M., Zhu, H., Lammer, E. J., Yang, W., and Finnell, R. H. (2003). Genetic variation of infant reduced folate carrier (A80G) and risk of orofacial and conotruncal heart defects. Am. J. Epidemiol. 158(8), 747–752. doi: 10.1093/aje/kwg189
Shen, X., Liu, R. M., Yang, L., Wu, H., Li, P. Q., Liang, Y. L., et al. (2011). The CRISPLD2 gene is involved in cleft lip and/or cleft palate in a Chinese population. Bir. Def. Res. A. Clin. Mol. Teratol. 91(10), 918–924. doi: 10.1002/bdra.20840
Shi, M., Christensen, K., Weinberg, C. R., Romitti, P., Bathum, L., Lozada, A., et al. (2007). Orofacial cleft risk is increased with maternal smoking and specific detoxification-gene variants. Am. J. Hum. Genet 80, 76–90.
Shi, M., Mostowska, A., Jugessur, A., Johnson, M. K., Mansilla, M. A., Christensen, K., et al. (2009). Identification of microdeletions in candidate genes for cleft lip and/or palate. Bir. Def. Res. A. Clin. Mol. Teratol. 85(1), 42–51. doi: 10.1002/bdra.20571
Shi, M., Murray, J. C., Marazita, M. L., Munger, R. G., Ruczinski, I., Hetmanski, J. B., et al. (2012). Genome wide study of maternal and parent-of-origin effects on the etiology of orofacial clefts. Am. J. Med. Genet A 158A(4), 784–794. doi: 10.1002/ajmg.a.35257.
Skare, O., Gjessing, H. K., Gjerdevik, M., Haaland, O. A., Romanowska, J., Lie, R. T., et al. (2017). A new approach to chromosome-wide analysis of X-linked markers identifies new associations in Asian and European case-parent triads of orofacial clefts. PLoS One 12(9):e0183772. doi: 10.1371/journal.pone.0183772
Song, H., Wang, X., Yan, J., Mi, N., Jiao, X., Hao, Y., et al. (2017). Association of single-nucleotide polymorphisms of CDH1 with nonsyndromic cleft lip with or without cleft palate in a northern Chinese Han population. Medicine 96(5):e5574. doi: 10.1097/MD.0000000000005574
Song, Y., and Zhang, S. (2011). Association of CDH1 promoter polymorphism and the risk of non-syndromic orofacial clefts in a Chinese Han population. Arch. Oral. Biol. 56(1), 68–72. doi: 10.1016/j.archoralbio.2010.08.019
Souza, L. T., Kowalski, T. W., Vanz, A. P., Giugliani, R., and Felix, T. M. (2012). TGFA/Taq I polymorphism and environmental factors in non-syndromic oral clefts in Southern Brazil. Braz. Oral. Res. 26(5), 431–435. doi: 10.1590/s1806-83242012005000016
Srichomthong, C., Ittiwut, R., Siriwan, P., Suphapeetiporn, K., and Shotelersuk, V. (2013). FOXE1 mutations in Thai patients with oral clefts. Genet Res. 95(5), 133–137. doi: 10.1017/S0016672313000177
Srichomthong, C., Siriwan, P., and Shotelersuk, V. (2005). Significant association between IRF6 820G-A and non-syndromic cleft lip with or without cleft palate in the Thai population. J. Med. Genet 42(7):e46. doi: 10.1136/jmg.2005.032235
Stanier, P., and Moore, G. E. (2004). Genetics of cleft lip and palate: syndromic genes contribute to the incidence of non-syndromic clefts. Hum. Mol. Genet 13, R73–81. doi: 10.1093/hmg/ddh052
Stothard, K. J., Tennant, P. W., Bell, R., and Rankin, J. (2009). Maternal overweight and obesity and the risk of congenital anomalies: a systematic review and meta-analysis. JAMA 301(6), 636–650. doi: 10.1001/jama.2009.113
Sull, J. W., Liang, K. Y., Hetmanski, J. B., Fallin, M. D., Ingersoll, R. G., Park, J. W., et al. (2008). Excess maternal transmission of markers in TCOF1 among cleft palate case-parent trios from three populations. Am. J. Med. Genet A 146A(18), 2327–2331. doi: 10.1002/ajmg.a.32302
Suphapeetiporn, K., Tongkobpetch, S., Siriwan, P., and Shotelersuk, V. (2007). TBX22 mutations are a frequent cause of non-syndromic cleft palate in the Thai population. Clin. Genet 72(5), 478–483. doi: 10.1111/j.1399-0004.2007.00891.x
Sutton, M., Mills, J. L., Molloy, A. M., Troendle, J. F., Brody, L. C., Conley, M., et al. (2011). Maternal folate, vitamin B12 and homocysteine levels in pregnancies affected by congenital malformations other than neural tube defects. Bir. Def. Res. A Clin. Mol. Teratol. 91(7), 610–615. doi: 10.1002/bdra.20817
Suzuki, A., Sangani, D. R., Ansari, A., and Iwata, J. (2016). Molecular mechanisms of midfacial developmental defects. Dev. Dyn. 245(3), 276–293. doi: 10.1002/dvdy.24368
Swindell, E. C., Yuan, Q., Maili, L. E., Tandon, B., Wagner, D. S., and Hecht, J. T. (2015). Crispld2 is required for neural crest cell migration and cell viability during zebrafish craniofacial development. Genesis 53(10), 660–667. doi: 10.1002/dvg.22897
Tamura, T., Munger, R. G., Corcoran, C., Bacayao, J. Y., Nepomuceno, B., and Solon, F. (2005). Plasma zinc concentrations of mothers and the risk of nonsyndromic oral clefts in their children: a case-control study in the Philippines. Bir. Def. Res. A Clin. Mol. Teratol. 73(9), 612–616. doi: 10.1002/bdra.20179
Tang, W., Du, X., Feng, F., Long, J., Lin, Y., Li, P., et al. (2009). Association analysis between the IRF6 G820A polymorphism and nonsyndromic cleft lip and/or cleft palate in a Chinese population. Cleft. Palate Craniofac. J. 46(1), 89–92. doi: 10.1597/07-131.1
Tasanarong, P., Pabalan, N., Tharabenjasin, P., and Jarjanazi, H. (2019). MSX1 gene polymorphisms and non-syndromic cleft lip with or without palate (NSCL/P): A meta-analysis. Oral. Dis. 25(6), 1492–1501. doi: 10.1111/odi.13127
Tinker, S. C., Gilboa, S. M., Moore, C. A., Waller, D. K., Simeone, R. M., Kim, S. Y., et al. (2020). Specific birth defects in pregnancies of women with diabetes: National Birth Defects Prevention Study, 1997-2011. Am. J. Obstet. Gynecol. 222(2), 171–176 e. doi: 10.1016/j.ajog.2019.08.028
Tudela, C., Formoso, M. A., Martinez, T., Perez, R., Aparicio, M., Maestro, C., et al. (2002). TGF-beta3 is required for the adhesion and intercalation of medial edge epithelial cells during palate fusion. Int. J. Dev. Biol. 46, 333–336.
van Rooij, I. A., Wegerif, M. J., Roelofs, H. M., Peters, W. H., Kuijpers-Jagtman, A. M., Zielhuis, G. A., et al. (2001). Smoking, genetic polymorphisms in biotransformation enzymes, and nonsyndromic oral clefting: a gene-environment interaction. Epidemiology 12(5), 502–507. doi: 10.1097/00001648-200109000200109007
VanderMeer, J. E., Carter, T. C., Pangilinan, F., Mitchell, A., Kurnat-Thoma, E., Kirke, P. N., et al. (2016). Evaluation of proton-coupled folate transporter (SLC46A1) polymorphisms as risk factors for neural tube defects and oral clefts. Am. J. Med. Genet A 170A(4), 1007–1016. doi: 10.1002/ajmg.a.37539
Venza, I., Visalli, M., Parrillo, L., De Felice, M., Teti, D., and Venza, M. (2011). MSX1 and TGF-beta3 are novel target genes functionally regulated by FOXE1. Hum. Mol. Genet 20(5), 1016–1025. doi: 10.1093/hmg/ddq547
Venza, M., Visalli, M., Venza, I., Torino, C., Tripodo, B., Melita, R., et al. (2009). Altered binding of MYF-5 to FOXE1 promoter in non-syndromic and CHARGE-associated cleft palate. J. Oral. Pathol. Med. 38(1), 18–23. doi: 10.1111/j.1600-0714.2008.00726.x
Wu, T., Schwender, H., Ruczinski, I., Murray, J. C., Marazita, M. L., Munger, R. G., et al. (2014). Evidence of gene-environment interaction for two genes on chromosome 4 and environmental tobacco smoke in controlling the risk of nonsyndromic cleft palate. PLoS One 9(2):e88088. doi: 10.1371/journal.pone.0088088
Wu-Chou, Y. H., Lu, Y. C., Chen, K. P., Chang, H. F., Lin, Y. T., and Lo, L. J. (2019). Association Studies Between Regulatory Regions of IRF6/TP63 Genes and Nonsyndromic Oral Clefts. Cleft. Palate Craniofac. J. 56(6), 778–785. doi: 10.1177/1055665618809244
Xu, W., Han, W., Lu, Y., Yao, W., Li, Z., Fang, K., et al. (2014). Microarray analysis of two single-nucleotide polymorphisms of transforming growth factor alpha in patients with nonsyndromic cleft of north china. Cleft. Palate Craniofac. J. 51(4), 486–492. doi: 10.1597/12145
Xu, Z., Lie, R. T., Wilcox, A. J., Saugstad, O. D., and Taylor, J. A. (2019). A comparison of DNA methylation in newborn blood samples from infants with and without orofacial clefts. Clin. Epigenet. 11(1):40. doi: 10.1186/s13148-019-0638639
Yin, X., Li, J., Li, Y., and Zou, S. (2019). Maternal alcohol consumption and oral clefts: a meta-analysis. Br. J. Oral. Maxillofac. Surg. 57(9), 839–846. doi: 10.1016/j.bjoms.2019.08.013
Zhu, H., Curry, S., Wen, S., Wicker, N. J., Shaw, G. M., Lammer, E. J., et al. (2005). Are the betaine-homocysteine methyltransferase (BHMT and BHMT2) genes risk factors for spina bifida and orofacial clefts? Am. J. Med. Genet. A 135(3), 274–277. doi: 10.1002/ajmg.a.30739
Keywords: non-syndromic cleft palate, NSCPO, risk factors, etiology, GRHL3, FOXE1, PAX7
Citation: Martinelli M, Palmieri A, Carinci F and Scapoli L (2020) Non-syndromic Cleft Palate: An Overview on Human Genetic and Environmental Risk Factors. Front. Cell Dev. Biol. 8:592271. doi: 10.3389/fcell.2020.592271
Received: 06 August 2020; Accepted: 28 September 2020;
Published: 20 October 2020.
Edited by:
Erika Kuchler, Universidade Positivo, BrazilReviewed by:
Renato Assis Machado, Campinas State University, BrazilPatricia Tannure, Veiga de Almeida University, Brazil
Copyright © 2020 Martinelli, Palmieri, Carinci and Scapoli. This is an open-access article distributed under the terms of the Creative Commons Attribution License (CC BY). The use, distribution or reproduction in other forums is permitted, provided the original author(s) and the copyright owner(s) are credited and that the original publication in this journal is cited, in accordance with accepted academic practice. No use, distribution or reproduction is permitted which does not comply with these terms.
*Correspondence: Marcella Martinelli, marcella.martinelli@unibo.it