- State Key Laboratory of Cotton Biology, Key Laboratory of Plant Stress Biology, School of Life Sciences, Henan University, Kaifeng, China
Vitellogenesis is pre-requisite to insect egg production and embryonic development after oviposition. During insect vitellogenesis, the yolk protein precursor vitellogenin (Vg) is mainly synthesized in the fat body, transported by the hemolymph through the intercellular spaces (known as patency) in the follicular epithelium to reach the membrane of maturing oocytes, and sequestered into the maturing oocytes via receptor-mediated endocytosis. Insect vitellogenesis is governed by two critical hormones, the sesquiterpenoid juvenile hormone (JH) and the ecdysteriod 20-hydroxyecdysone (20E). JH acts as the principal gonadotropic hormone to stimulate vitellogenesis in basal hemimetabolous and most holometabolous insects. 20E is critical for vitellogenesis in some hymenopterans, lepidopterans and dipterans. Furthermore, microRNA (miRNA) and nutritional (amino acid/Target of Rapamycin and insulin) pathways interplay with JH and 20E signaling cascades to control insect vitellogenesis. Revealing the regulatory mechanisms underlying insect vitellogenesis is critical for understanding insect reproduction and helpful for developing new strategies of insect pest control. Here, we outline the recent research progress in the molecular action of gonadotropic JH and 20E along with the role of miRNA and nutritional sensor in regulating insect vitellogenesis. We highlight the advancements in the regulatory mechanisms of insect vitellogenesis by the coordination of hormone, miRNA and nutritional signaling pathways.
Introduction
A hallmark of female insect reproduction is vitellogenesis, a heterosynthetic process by which vitellogenin (Vg) is mostly synthesized in the fat body and deposited into developing oocytes. In addition to the fat body, Vg synthesis has been reported in follicle cells (Gilbert et al., 1998), nurse cells (Melo et al., 2000; Matsumoto et al., 2008) and hemocytes (Bai et al., 2015; Huo et al., 2018) in several insect species. Vg is critical for egg maturation during adulthood and embryonic growth after oviposition. In the honey bee Apis mellifera, Vg also protects the queen and long-lived worker bees from oxidative stress and further extends their life span (Seehuus et al., 2006; Corona et al., 2007; Ihle et al., 2015). Additionally, Vg plays a role in sensing fat body sugars and gustatory perception in the worker bees (Amdam et al., 2006; Wang et al., 2012, 2013). Furthermore, A. mellifera Vg acts as a pathogen recognition receptor and transports pathogen-derived molecules into the offspring to achieve trans-generational immunity (Garcia et al., 2010; Salmela et al., 2015; Zhang et al., 2015). In the small brown planthopper Laodelphax striatellus, Vg synthesized by hemocytes can facilitate the vertical transmission of rice stripe virus (Huo et al., 2018). The number of Vg genes among different insect species ranges from one to three in general, while the mosquito Aedes aegypti and the ant Linepithema humile possess up to five Vg genes (Corona et al., 2013) (Supplementary Table 1). The presence of multiple copies of Vg genes and multiple forms of Vg protein in insects is unclear. It is conceivable that two or more copies of Vg gene ensure the efficient production of yolk protein precursors required for the maturation of multiple eggs. The variation of Vg gene and protein in diverse insect species might reflect the evolutionary selection and the strategy of adaptation to environment (Garcia et al., 2010). Vg protein is a large glycolipophosphoprotein that is often oligomeric in their native state, with monomers consisting of two or more subunits (apoproteins). The molecular weights vary from 150 kDa to 200 kDa for large subunits and from 40 kDa to 65 kDa for small subunits, respectively (Lee et al., 2015). Vg protein sequences are evolutionarily conserved across insect orders except for yolk proteins (YPs) in Diptera. In the fruit fly Drosophila melanogaster, YPs form a different family of proteins to nurse maturing oocytes. Vg is generally composed of a lipoprotein N-terminal domain (LPD_N) for lipid binding, a 1943 domain with unknown function (DUF1943), and a von Willebrand factor type D domain (vWFD) in the C-terminus (Morandin et al., 2014). LPD_N has a conserved polyserine tract possessing consensus cleavage motifs (R/KXXR/K) (Tufail and Takeda, 2008). Vg proteins are phosphorylated at the polyserine tract, but the function of Vg phosphorylation remains elusive (Tufail and Takeda, 2008).
Cumulative studies have established that insect vitellogenesis is controlled by two classic hormones, the sesquiterpenoid juvenile hormone (JH) and the ecdysteroid 20-hydroxyecdysone (20E, an active form of ecdysone). Both JH and 20E can stimulate various aspects of vitellogenesis but vary across insect orders depending on reproductive traits (Wyatt and Davey, 1996; Raikhel et al., 2005; Roy et al., 2018; Santos et al., 2019; Song and Zhou, 2020). In evolutionarily primitive hemimetabolous insects such as the migratory locust Locusta migratoria and the German cockroach Blattella germanica, JH acts independently of 20E to stimulate vitellogenesis and oocyte maturation (Wyatt and Davey, 1996; Belles, 2004; Roy et al., 2018; Song and Zhou, 2020). In holometabolous coleopteran like the red flour beetle Tribolium castaneum, JH governs Vg synthesis in the fat body while 20E participates in ovarian growth and oocyte maturation (Parthasarathy et al., 2010a,b; Sheng et al., 2011). In many lepidopterans including the tobacco hornworm Manduca sexta and the cotton bollworm Helicoverpa armigera, in which Vg synthesis is initiated in adults, JH plays a principal role in vitellogenesis (Swevers and Iatrou, 2003; Telfer, 2009). However, in other lepidopterans such as the silkworm Bombyx mori, the silk moth Hyalophora cecropia, and the armyworm Spodoptera frugiperda, in which Vg is synthesized prior to adult ecdysis, 20E appears to have a primary role in vitellogenesis (Swevers and Iatrou, 2003; Telfer, 2009). In the mosquito Ae. aegypti, JH promotes fat body becoming competent for Vg synthesis, while 20E stimulates Vg expression and oocyte maturation after a blood meal (Raikhel et al., 2002; Shin et al., 2012). In D. melanogaster, 20E is responsible for the high rate of Vg synthesis in the fat body and JH controls Vg uptake into oocytes (Bownes, 1990; Carney and Bender, 2000; Berger and Dubrovsky, 2005).
Nutrients play a pivotal role in insect vitellogenesis. As nutritional sensors, the amino acid/Target of Rapamycin (AA/TOR) and insulin (or insulin-like peptide, ILP) are involved in biosynthesis of JH and 20E and crosstalk with JH and 20E pathways, which in turn regulate various aspects of insect vitellogenesis (Badisco et al., 2013; Hansen et al., 2014; Smykal and Raikhel, 2015; Roy et al., 2018). Furthermore, recent studies have revealed an essential role of microRNA (miRNA) in the regulation of insect vitellogenesis (Roy et al., 2018; Song and Zhou, 2020). Hence, this review covers the present status of our understanding in the regulatory mechanisms of insect vitellogenesis including Vg synthesis in the fat body and uptake in the ovary.
Regulation of Vg Synthesis in the Fat Body
Insect fat body, analogous to the vertebrate liver and adipose tissue, is the major source for Vg synthesis. Recently, research advancements have been achieved in the molecular mechanisms of Vg synthesis regulated by JH, 20E, miRNA and nutritional pathways. For the regulatory mechanisms of 20E, AA/TOR and peptides in insect vitellogenesis and oogenesis, we refer readers to three recent reviews (Roy et al., 2018; Lenaerts et al., 2019; Swevers, 2019). Here, we outline the recent accomplishments in regulating Vg synthesis in the fat body, with the emphasis on the role of JH, miRNA and ILP pathways (Figure 1).
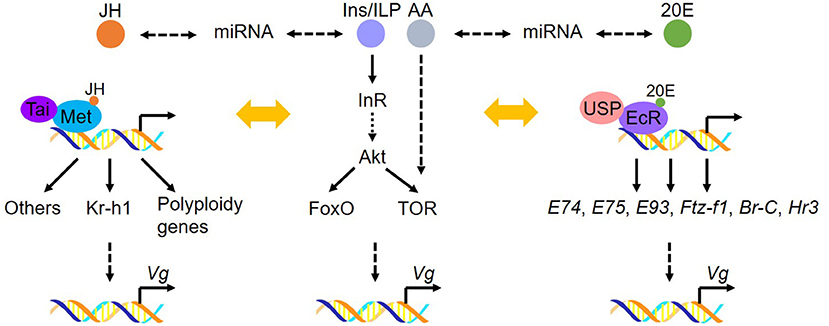
Figure 1. Molecular basis of Vg synthesis in the fat body. JH receptor complex Met/Tai directly regulates the transcription of target genes encoding Kr-h1 and the polyploidy genes (including DNA replication and cell cycle genes). 20E receptor complex EcR/USP directly activates the expression of 20E-responsive genes. Nutritional signaling is involved in Vg production in the fat body by interplay with JH or 20E. In addition, miRNA participates in JH- or 20E-dominant Vg synthesis in the fat body. These factors directly or indirectly regulate Vg transcription and vitellogenesis.
JH Action on Vg Synthesis
The molecular action of JH relies on its intracellular receptor Methoprene-tolerant (Met), a member of basic helix-loop-helix Per-Arnt-Sim (bHLH-PAS) transcription factor family. JH induces the heterodimerization of Met with another bHLH-PAS protein, Taiman (Tai) to form an active JH-receptor complex (Jindra et al., 2013). The JH-Met/Tai receptor complex consequently activates the transcription of JH-responsive genes (Charles et al., 2011; Li et al., 2011; Kayukawa et al., 2012; Guo et al., 2014; Jindra et al., 2015; Wu et al., 2016, 2018; Wang Z. et al., 2017). In adult insects, JH requires the Met/Tai complex to achieve its previtellogenic and vitellogenic effects on fat body competency and Vg synthesis (Zou et al., 2013; Guo et al., 2014; Gujar and Palli, 2016; Wang Z. et al., 2017). RNAi-mediated knockdown of Met resulted in significant reduction of Vg expression level accompanied by arrested oocyte maturation and blocked egg production in a variety of investigated insects including T. castaneum, L. migratoria, H. armigera, the linden bug Pyrrhocoris apterus, the brown planthopper Nilaparvata lugens, the oriental fruit fly Bactrocera dorsalis, and the white-backed planthopper Sogatella furcifera (Parthasarathy et al., 2010b; Shpigler et al., 2014; Smykal et al., 2014; Song et al., 2014; Lin et al., 2015; Ma et al., 2018; Yue et al., 2018; Hu et al., 2019). In L. migratoria, P. apterus, and Ae. aegypti, depletion of Tai or its orthologs FISC and p160/SRC led to significantly declined levels of Vg transcripts along with blocked oocyte development (Smykal et al., 2014; Wang Z. et al., 2017). Krüppel-homolog 1 (Kr-h1), a primary JH early-response gene that codes for a zinc finger transcription factor, transduces JH signaling in regulating adult reproduction (Kayukawa et al., 2012; Shin et al., 2012; Song et al., 2014). The role of Kr-h1 in insect vitellogenesis varies among insect species. In L. migratoria, B. dorsalis, and the rice stem borer Chilo suppressalis, Kr-h1 mediates JH action to promote vitellogenesis and oocyte maturation (Song et al., 2014; Yue et al., 2018; Tang et al., 2020). In Ae. aegypti, Kr-h1 transduces JH signaling as both activator and repressor to regulate the expression of JH-responsive genes involved in previtellogenic development, consequently regulating egg production after a blood meal (Shin et al., 2012; Zou et al., 2013; Ojani et al., 2018). In T. castaneum, depletion of Met caused more than 80% decrease of Vg mRNA levels, whereas Kr-h1 knockdown resulted in about 30% reduction of Vg transcripts (Parthasarathy et al., 2010b). In P. apterus, knockdown of Met or Tai but not Kr-h1 suppressed Vg synthesis in the fat body (Smykal et al., 2014). In the common bed bug Cimex lectularius, Kr-h1 knockdown appeared to have no significant impact on vitellogenesis (Gujar and Palli, 2016).
L. migratoria, a hemimetabolous species bearing the panoistic ovary and synchronously matured oocytes, has been a long-standing model for studying JH-dependent female reproduction (Wyatt and Davey, 1996; Roy et al., 2018). In adult females of L. migratoria, JH induces Vg synthesis in the fat body, initiates intercellular space (patency) in the follicular epithelium, and facilitates the uptake of Vg into developing oocytes (Wyatt and Davey, 1996; Song and Zhou, 2020). During the first gonadotrophic cycle, locust fat body undergoes endocycle to produce up to 32C (chromatin copies per cell) polyploid cells required for massive Vg synthesis (Guo et al., 2014). RNA-seq-based gene expression profiling followed by qRT-PCR validation demonstrated that JH upregulated the expression of 16 genes related to DNA replication and 13 genes involved in cell cycle progression, suggesting that JH plays a pivotal role in the onset and progression of polyploidy by promoting endocycle in the fat body (Guo et al., 2014). Of these 29 genes, chromosome maintenance genes 3, 4, 7 (Mcm3, Mcm4, and Mcm7), cell-division-cycle 6 (Cdc6), cyclin-dependent kinase 6 (Cdk6) and adenovirus E2 factor-1 (E2f1) expressed in response to both JH and Met (Guo et al., 2014). Further studies revealed that the JH-Met/Tai receptor complex bound to the consensus sequences with E-box or E-box-like motifs in the promoters of Mcm4, Mcm7, Cdc6, Cdk6, and E2f1 and activated their transcription. Knockdown of Mcm4, Mcm7, Cdc6, Cdk6, or E2f1 in vitellogenic female locusts resulted in significant reduction in fat body cell ploidy, accompanied by markedly reduced Vg expression levels, arrested oocyte maturation and impaired egg production (Guo et al., 2014; Wu et al., 2016, 2018). Interestingly, depletion of Met had no significant effect on other genes upregulated by JH (Guo et al., 2014), suggesting the involvement of other signaling cascades that interplay with JH. Next, Wu et al. (2020) demonstrated that JH stimulated FoxO dephosphorylation through leucine carboxyl methyltransferase 1 (LCMT1)-mediated activation of protein phosphatase 2A (PP2A). JH-LCMT1-PP2A axis-triggered FoxO dephosphorylation facilitated FoxO translocation in nuclei and activated the transcription of cell-division-cycle 2 (Cdc2) and origin-recognition-complex subunit 5 (Orc5). Knockdown of LCMT1, PP2A, FoxO, Cdc2, or Orc5 in vitellogenic female locusts resulted in lower ploidy and significantly reduced Vg expression in the fat body as well as arrested oocyte maturation and suppressed egg development (Wu et al., 2020). Collectively, JH acts via its receptor complex Met/Tai to induce the expression of Mcm4/7, Cdc6, Cdk6, and E2f1, and through LCMT1-PP2A-FoxO axis to trigger the expression of Cdc2 and Orc5 (Guo et al., 2014; Wu et al., 2016, 2018, 2020). These two signaling pathways coordinate to promote fat body cell polyploidization for large-scale Vg synthesis, which meets the requirement of synchronous maturation of multiple eggs in L. migratoria.
Ae. aegypti serves as an ideal model for studying JH-dependent posteclosion or previtellogenic development. Besides the JH-Met/Tai complex, JH induces the dimerization of Met with Cycle to upregulate gene transcription for previtellogenic development in Ae. aegypti (Shin et al., 2012). JH acts via Met to induce the expression of Regulator of Ribosome Synthesis 1 (RRS1) and Ribosomal protein L32 (RpL32), consequently enhancing ribosomal biogenesis in the fat body essential for massive Vg synthesis (Wang J. L. et al., 2017). Saha et al. (2019) reported that JH induced the expression of gene coding for Hairy which dimerizes with Groucho to form a transcriptional repressor complex mediating the repressive function of JH. Interestingly, Hairy and Kr-h1 acted synergistically in the JH/Met gene repression hierarchy during previtellogenic development of Ae. aegypti (Saha et al., 2019). JH exerts both genomic and non-genomic action. For non-genomic action, JH acts via the RTK-PLC-IP3-CaMKII signaling cascade to trigger Met phosphorylation that enhances its transcriptional activity in previtellogenic development of Ae. aegypti (Liu et al., 2015; Ojani et al., 2016). Moreover, JH triggers the RTK-PI3K-Akt signaling pathway to stimulate the phosphorylation of serine/arginine-rich (pre-mRNA) splicing factor (SRSF), which induces the alternative splicing of Tai to produce Tai-A and Tai-B isoforms (Liu et al., 2018). These two isoforms could potentiate the transcriptional activity of 20E-receptor in the fat body of adult female mosquitoes, consequently promoting blood meal-induced vitellogenesis and egg production (Liu et al., 2018).
Nutritional Control of Vg Synthesis
AA/TOR pathway has been demonstrated to play pivotal roles in activating Vg synthesis in divergent orders of insects (Sancak et al., 2008; Carpenter et al., 2012; Koyama et al., 2013; Lu et al., 2016). Suppression of TOR activity by rapamycin treatment or RNA-mediated knockdown inhibited Vg expression in Ae. aegypti and N. lugens (Hansen et al., 2004; Lu et al., 2016). Moreover, TOR tends to have a positive effect on JH biosynthesis and vitellogenesis. In T. castaneum, B. germanica, and the American cockroach Periplaneta americana, TOR and ILP signaling could enhance the expression of JH methyltransferase (JHAMT), Met and Kr-h1, further stimulating Vg expression and oocyte maturation (Parthasarathy and Palli, 2011; Abrisqueta et al., 2014; Zhu et al., 2020). Moreover, insulin/TOR signaling affects 20E synthesis and secretion as well, further associates with vitellogenesis and egg production in D. melanogaster and Ae. aegypti (Tu et al., 2002; Dhara et al., 2013).
FoxO plays a vital role in mediating the crosstalk between insulin and JH signaling pathways to coordinate insect vitellogenesis (Koyama et al., 2013; Smykal and Raikhel, 2015; Roy et al., 2018; Santos et al., 2019). In B. germanica, depletion of insulin receptor gene (InR) or FoxO in fed adult females resulted in markedly reduced Vg expression along with impaired oocyte maturation (Abrisqueta et al., 2014). Similarly, FoxO knockdown led to reduction of Vg transcript levels in fed adult females of T. castaneum, Ae. aegypti, L. migratoria and the soybean pod borer Maruca vitrata (Hansen et al., 2007; Parthasarathy and Palli, 2011; Abrisqueta et al., 2014; Al Baki et al., 2019; Wu et al., 2020). However, silencing of FoxO in the starved adult females of B. germanica caused significant increase of Vg expression due to elevated biosynthesis of JH (Suren-Castillo et al., 2012; Abrisqueta et al., 2014). In B. mori, FoxO suppresses the expression of JH degradation genes JHE, JHDK, and JHEH to protect JH from degradation (Zeng et al., 2017). Interestingly, FoxO binds to Vg2 gene promoter and inhibits its transcription in T. castaneum. After adult emergence, JH induces ILP expression and FoxO phosphorylation, which in turn releases FoxO binding and activates Vg2 transcription (Sheng et al., 2011).
miRNA Regulation of Vg Synthesis
As fine-tuners, miRNAs generally downregulate their target genes by translation inhibition or mRNA degradation in a spatiotemporal manner. Nevertheless, miRNAs can bind the 5′-UTR or CDS of target mRNAs to upregulate gene expression (Vasudevan et al., 2007; Zhou et al., 2009; Yang et al., 2014; He et al., 2016). The role of miRNAs in oogenesis has been extensively studied in D. melanogaster (Asgari, 2013; Shcherbata, 2019; Song and Zhou, 2020). Recently, miRNA functions in vitellogenesis and oocyte maturation have been explored in non-model insects like Ae. aegypti and L. migratoria (Lucas et al., 2015b; Roy et al., 2018; Song and Zhou, 2020). As two previous reviews have covered miRNA regulation in insect reproduction (Roy et al., 2018; Song and Zhou, 2020), we update the recent advances in the role of miRNAs in insect vitellogenesis.
Dicer 1 and Argonaute1 (Ago1) are two key enzymes involved in miRNA biogenesis and functioning. Depletion of Dicer 1 or Ago1 in D. melanogaster, B. germanica, L. migratoria, and N. Lugens led to defective phenotypes of female reproduction including decreased Vg expression and impaired oocyte maturation (Jin and Xie, 2007; Azzam et al., 2012; Tanaka and Piulachs, 2012; Song et al., 2013; Zhang et al., 2013). In adult female locusts, JH upregulated 59 miRNAs and downregulated 23 miRNAs (Song et al., 2013). Of JH-downregulated miRNAs, let-7 and miR-278 bound to Kr-h1 coding sequence and repressed its expression. Application of let-7 and miR-278 agomiRs resulted in significantly reduced Kr-h1 expression along with markedly decreased abundance of Vg protein and blocked oocyte maturation (Song et al., 2018). Intriguingly, JH titer increases from the previtellogenic stage to vitellogenic phase, whereas let-7 and miR-278 have the opposite tendency in abundance (Song et al., 2018; Guo et al., 2019). The increased JH titer and declined abundance of let-7 and miR-278, therefore, ensure high levels of Kr-h1 required for vitellogenesis. miR-2/13/71 is clustered miRNAs downregulated by JH in L. migratoria (Song et al., 2019). miR-2/13/71 bound to the coding sequence of Notch mRNA to inhibit its expression. Injection of miR-2/13/71 agomiRs in adult female locusts also led to reduced Vg transcripts and arrested oocyte maturation (Song et al., 2019). Likewise, the increased JH titer and declined abundance of miR-2/13/71 in vitellogenic female locusts contribute to high levels of Notch for successful vitellogenesis and egg development (Song et al., 2019).
In Ae. aegypti, lineage-specific miR-1890 targets the 3′UTR of JH-regulated Chymotrypsin-like serine protease (JHA15). After a blood meal, 20E suppresses miR-1890 expression to prevent adult females from impaired blood digestion in the midgut and Vg synthesis in the fat body (Lucas et al., 2015b). Disruption of miR-275, miR-1174 or miR-8 also caused defects in blood digestion, vitellogenesis and egg production in the vitellogenic females of Ae. aegypti (Bryant et al., 2010; Liu et al., 2014; Lucas et al., 2015a). Moreover, miR-989, most abundant in the fat body and ovary of Ae. aegypti, directly targets Vg gene during female reproduction (Zhang et al., 2017). In the malaria mosquito Anopheles gambiae, miR-309 antagomiR treatment caused impaired vitellogenesis and egg production (Fu et al., 2017). In N. lugens, miR-4868b inhibits the expression of Glutamine synthase gene. miR-4868b antagomiR treatment resulted in significant decrease of Vg protein levels along with impeded egg development and reduced fecundity (Fu et al., 2015).
Post-translational Regulation of Synthesized Vg
Nascently synthesized Vg in the fat body is properly folded in the endoplasmic reticulum (ER) and assembled into the secretory vesicles via membrane fusion with the Golgi apparatus. The 78-kDa Glucose-regulated protein (Grp78), a heat shock protein 70 kDa family member is one of the most abundant chaperones in fat body ER of L. migratoria (Luo et al., 2017). During locust vitellogenesis, JH stimulates two Grp78 genes, Grp78-1 and Grp78-2 via differential signaling pathways, which together accelerate the production of Grp78 chaperone. Grp78 facilitates the proper folding of massively synthesized Vg and avoid ER stress in the fat body (Luo et al., 2017). During the intracellular transportation, Vg stability is threatened by a variety of proteases but protected by protease inhibitors, of which the Kazal-type protease inhibitor is a common subfamily (Van Hoef et al., 2013). Guo et al. (2019) demonstrated that Greglinwas the predominant Kazal-type protease inhibitor in the fat body and ovary of vitellogenic females of L. migratoria. During locust vitellogenesis, JH induced high levels of Greglin expression to protect Vg from proteolysis (Guo et al., 2019). During the termination of B. mori embryonic diapause under low temperature, extracellular signal-regulated kinase (ERK)/mitogen-activated protein kinase (MAPK) signaling is activated in the yolk cells (Iwata et al., 2005). Thereafter, ERK/MAPK increases sorbitol and 20E metabolism by regulating the transcription of downstream genes. The elevated sorbitol-glycogen conversion and 20E secretion promote embryonic development, yolk-cell dispersion as well as yolk protein degradation (Fujiwara et al., 2006).
Regulation of Vg Uptake by Ovaries
With the help of hemolymph circulation, Vg is transported into the ovary and absorbed by maturing oocytes. Oocytes are enclosed by the follicle epithelium, which determines the size and shape of oocytes and initiates the intercellular spaces (patency) (Wyatt and Davey, 1996; Raikhel et al., 2005). Vg is transported through patency to the surface of competent oocytes and then internalized via Vg receptor (VgR)-mediated endocytosis (Figure 2). JH plays a crucial role in Vg uptake by ovaries in a variety of insects. In adult females of H. armigera, depletion of Met severely suppressed Vg and VgR transcription, disrupted yolk protein uptake into oocytes, and eventually led to reduced fecundity (Ma et al., 2018). In L. migratoria, knockdown of Met or Kr-h1 caused markedly smaller follicle cells and malfunctional patency (Song et al., 2014). In B. germanica, the JH-dependent expression of SPARC (secreted protein, acidic and rich in cysteine) gene, coding for a calcium-binding glycoprotein that forms part of the extracellular membranes is critical for follicle cell morphological changes and patency induction that facilitate Vg uptake by developing oocytes (Irles et al., 2017).
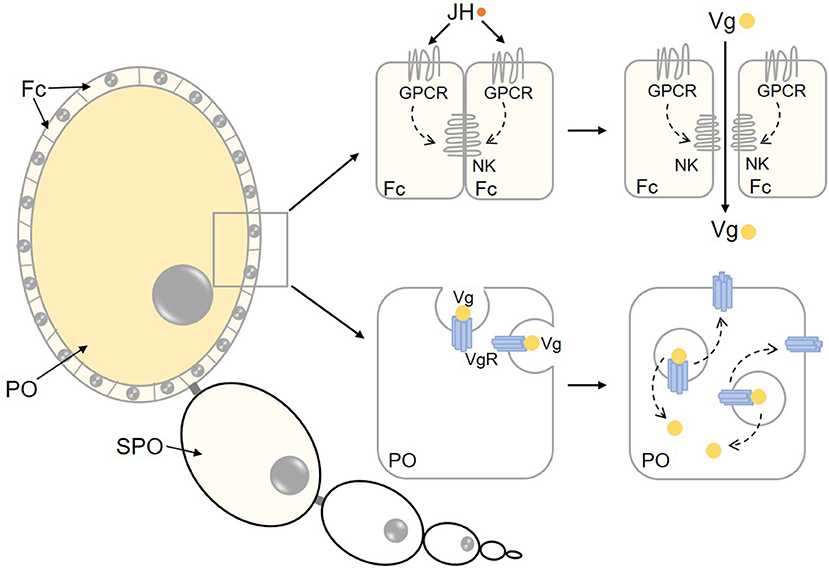
Figure 2. Regulation of Vg uptake by maturing oocytes. Prior to Vg uptake by maturing oocytes, Vg passes through patency among follicle cells, which is regualted by JH action on GPCR and Na+/K+-ATPase. Vg is internalized by surrounding oocytes through VgR-mediated endocytosis. Afterwards, Vg is unbound with VgR, then VgR is recycled to the membrane of the oocyte. NK, Na+/K+-ATPase; Fc, follicle cells; PO, primary oocyte; SPO, sub-primary oocyte.
Role of Na+/K+-ATPase in Patency Initiation
Na+/K+-ATPase is a heterodimer protein that consists of α- and β-subunits (Lingrel and Kuntzweiler, 1994). The α-subunit, which has 10 transmembrane loops and large cytoplasmic regions, plays a chief function in catalytic action because of its ATP binding site and phosphorylation (Fambrough et al., 1994; Lingrel and Kuntzweiler, 1994). The β-subunit, also known as Nervana, is a nervous system-specific glycoprotein antigen in the adult head of D. melanogaster. The β-subunit contains a single transmembrane, a small N-terminal cytoplasmic domain and a large C-terminal extracellular region (Kaplan, 2002). In B. germanica, the orthologs of D. melanogaster Nervana 1 and Nervana 2 are crucial for oogenesis (Irles et al., 2013). Early study on the kissing bug Rhodnius prolixus has demonstrated that JH-stimulated patency is inhibited by application of ouabain, a specific inhibitor of Na+/K+-ATPase (Sevala and Davey, 1989), indicating the involvement of Na+/K+-ATPase in the regulation of JH-dependent patency initiation and Vg uptake. Subsequently, the role of Na+/K+-ATPase in patency initiation has been reported in L. migratoria, the beetle Tenebrio molitor and the moth Heliothis virescens (Sevala and Davey, 1993; Pszczolkowski et al., 2005). In L. migratoria, RNAi-mediated knockdown of Na+/K+-ATPase gene, pharmaceutical inhibition of Na+/K+-ATPase phosphorylation or suppression of Na+/K+-ATPase activity by inhibitor treatment resulted in the loss of patency along with blocked Vg uptake and impaired egg development (Jing et al., 2018).
As patency is initiated by JH, efforts have been made to elucidate the regulatory mechanisms of Na+/K+-ATPase activation by JH. Pharmaceutical approaches with protein kinase C (PKC) activator in R. prolixus have shown that PKC activation is required for the induction of JH-dependent Na+/K+-ATPase activity (Sevala and Davey, 1989). JH also evokes Na+/K+-ATPase-activated patency in H. virescens via the signaling pathways including protein kinase A (PKA) and PKC (Pszczolkowski et al., 2005, 2008). Jing et al. (2018) demonstrated that JH triggered a signaling cascade including G protein-coupled receptor (GPCR), receptor tyrosine kinase (RTK), phospholipase C (PLC), inositol trisphosphate (IP3) and PKC to phosphorylate Na+/K+-ATPase α-subunit at serine residue 8, leading to activation of Na+/K+-ATPase. Activated Na+/K+-ATPase is likely to change the ionic balance of follicle cells and cause cell shrinkage, consequently initiating patency for Vg transportation to the surface of maturing oocytes (Jing et al., 2018). However, the specific GPCR or RTK involved in GPCR/RTK-PLC-IP3-PKC signaling cascade remains to be identified. Bai and Palli (2016) reported that Dopamine D2-like receptor (Dop2R) controls Vg uptake by maturing oocytes in T. castaneum (Bai and Palli, 2016). In L. migratoria, 22 GPCRs were identified in the ovarian transcriptome of vitellogenic females. RNAi screening revealed that Orphan receptor-A (OR-A1 and OR-A2), C-type muscarinic acetylcholine receptor (mAChR-C) and Cirl-like (CirlL) played key roles in ovarian Vg transportation and uptake (Zheng et al., 2020).
VgR-mediated Vg Endocytosis
Insect VgR belongs to the low-density lipoprotein receptor (LDLR) family (Raikhel and Dhadialla, 1992; Schonbaum et al., 1995; Tufail and Takeda, 2009). Upon binding of Vg to VgR, the Vg/VgR complex clusters in clathrin-coated pits, invaginates into the cytoplasm and pinches off to form intracellular coated vesicles. After transformed into the endosome or transitional yolk body, Vg and VgR are dissociated by ATP-dependent acidification. Subsequently, VgR is recycled back to oocyte membrane surface while Vg is crystallized and stored as vitellin (Vn) in yolk bodies for future embryonic development (Sappington and Raikhel, 1998; Raikhel et al., 2002; Snigirevskaya and Raikhel, 2005; Mitchell and Pérez De León, 2019). In the oriental fruit fly Bactrocera dorsalis, depletion of VgR blocked Vg uptake and egg development (Cong et al., 2015). Also, knockdown of VgR in N. lugens and S. furcifera caused accumulation of Vg in the hemolymph accompanied by arrested oocyte maturation (Lu et al., 2015; Hu et al., 2019). VgR expression is hormonally regulated during reproductive development. In the fire ant Solenopsis invicta, JH treatment of cultured ovaries significantly increased VgR expression (Chen et al., 2004). Analogously, JH treatment induced VgR expression in adult females of N. lugens (Lu et al., 2015). Furthermore, JH upregulates VgR expression by Met-Kr-h1 pathway in the cabbage beetle Colaphellus bowringi (Liu et al., 2019). Interestingly, in P. americana whose vitellogenesis is dependent upon JH, the expression of Vg and VgR is inhibited by additional 20E treatment (Kamruzzaman et al., 2020). Similarly, knockdown of the Halloween genes coding for a group of enzymes involved in 20E synthesis remarkably reduced the levels of Vg and VgR expression, leading to defective egg development of the diamondback moth Plutella xylostella (Peng et al., 2019). In M. vitrata and the beet armyworm Spodoptera exigua, the expression of VgR is regulated by ILP/TOR signaling pathway (Zhao et al., 2018; Al Baki et al., 2019). Notably, two miRNAs, miR-2739 and miR-167 bind directly to the 3′UTR of B. mori VgR mRNA and coordinately inhibit VgR expression. Overexpression of either miR-2739 or miR-167 using the piggyBac system blocked Vg deposition into oocytes, whereas application of miR-2739 or miR-167 antagomiR resulted in increase of VgR levels and uptake of Vg by oocytes (Chen et al., 2020).
In addition to mediating Vg internalization, VgR is shown to play an important role in vertical transmission of pathogenic microbes and Wolbachia symbionts (Guo et al., 2018; Huo et al., 2019; Mitchell and Pérez De León, 2019). In L. striatellus, Vg is hitchhiked by the rice stripe virus (RSV), which enters the developing oocytes through VgR-mediated endocytosis (Huo et al., 2018, 2019; He et al., 2019). Also in L. striatellus, Wolbachia symbionts acquire maternal transmission through the Vg-VgR axis (Guo et al., 2018). It has been demonstrated that a series of small guanosine triphosphatases (GTPase), whose activities are at peak during vitellogenesis, are involved in the process of Vg vesicular formation, budding, docking and fusion. In P. americana, GTPase activity is required for the intracellular trafficking of Vg in the ovary (Elmogy et al., 2014). In N. lugens, depletion of Ran, a member of GTPase family, brough about markedly reduced Vg expression and decreased fecundity (Li et al., 2015).
Conclusions and Prospects
Vitellogenesis is one of the most emblematic processes in insect reproduction. Over the past decades, accumulative studies have advanced our understanding of how insect vitellogenesis is governed by hormonal and nutritional pathways. 20E acts through its receptor complex comprised of Ecdysone Receptor (EcR) and Ultraspiracle (USP), leading to transcriptional activation of 20E-response genes including ecdysone-induced proteins 74 (E74), 75 (E75), 93F (E93), Broad-Complex (Br-C), and Ftz-f1. The 20E pathway appears to be evolutionarily conserved in vitellogenesis and oogenesis across insect orders (Raikhel et al., 2005; Swevers, 2019). Most recently, relative more research progress has been made on the action of JH, nutrition and miRNA pathways on vitellogenesis in diverse insect species such as L. migratoria, Ae. aegypti, T. castaneum, and B. germanica (Roy et al., 2018; Santos et al., 2019; Song and Zhou, 2020). JH acts through its receptor complex or interplays with ILP pathway to stimulate fat body cells undergoing endocycle for polyploidization, which accelerates massive Vg synthesis to meet the requirement for synchronous maturation of multiple eggs in L. migratoria (Guo et al., 2014; Wu et al., 2016, 2018, 2020). Moreover, JH acts via Met and intracellular signaling cascades to promote fat body competency ready for Vg synthesis after a blood meal in Ae. aegypti (Shin et al., 2012; Ojani et al., 2016; Liu et al., 2018; Saha et al., 2019). The genomic action of JH has been extensively studied since the establishment of Met/Tai as the nuclear JH receptor and the identification of JH-responsive genes. Nevertheless, most studies on JH actions have been conducted in insect molting and metamorphosis. The molecular mechanisms of JH regulation in insect vitellogenesis and oogenesis are largely obscure. The membrane receptor of JH remains to be identified. The roles of JH and 20E in insect reproduction vary across insect orders and even among genera, it is of interest to unveil the evolutionary aspects of hormonal regulation in insect vitellogenesis. In addition, AA/TOR and ILP pathways play pivotal roles in insect vitellogenesis by way of direct regulation or interaction with JH signaling cascade (Parthasarathy and Palli, 2011; Abrisqueta et al., 2014; Roy et al., 2018; Zhu et al., 2020). Moreover, an increasing body of evidence supports the importance of miRNA regulation in insect vitellogenesis. Likewise, miRNAs can interact with JH pathway and modulate JH production, consequently regulating insect vitellogenesis (Roy et al., 2018; Song and Zhou, 2020). Interestingly, JH, miRNAs and cognate genes constitute the regulatory loop and coordinately control Vg synthesis (Song et al., 2018, 2019). Therefore, the molecular basis of JH, miRNA and nutritional pathway crosstalk in regulating insect Vg synthesis and uptake requires further exploration. Furthermore, the involvement of other epigenetic and posttranscriptional regulators in insect vitellogenesis need to be dissected. Currently, studies on Vg secretion from the fat body into the hemolymph and Vg uptake in the ovary are limited. Future discovery of new players in JH-dependent patency initiation in the follicular epithelium and VgR-mediated endocytosis in the oocytes will help construct the regulatory networks controlling insect vitellogenesis and egg production.
Author Contributions
ZW, LY, and QH collected the references. ZW, LY and SZ wrote the manuscript. All authors contributed to the article and approved the submitted version.
Funding
This work was supported by National Natural Science Foundation of China (NSFC) (31630070 and 31702062).
Conflict of Interest
The authors declare that the research was conducted in the absence of any commercial or financial relationships that could be construed as a potential conflict of interest.
Supplementary Material
The Supplementary Material for this article can be found online at: https://www.frontiersin.org/articles/10.3389/fcell.2020.593613/full#supplementary-material
Supplementary Table 1. Identification of Vg genes in insects.
References
Abrisqueta, M., Suren-Castillo, S., and Maestro, J. L. (2014). Insulin receptor-mediated nutritional signalling regulates juvenile hormone biosynthesis and vitellogenin production in the German cockroach. Insect Biochem. Mol. Biol. 49, 14–23. doi: 10.1016/j.ibmb.2014.03.005
Al Baki, M. A., Lee, D. W., Jung, J. K., and Kim, Y. (2019). Insulin signaling mediates previtellogenic development and enhances juvenile hormone-mediated vitellogenesis in a lepidopteran insect, Maruca vitrata. BMC Dev. Biol. 19:14. doi: 10.1186/s12861-019-0194-8
Amdam, G. V., Norberg, K., Page, R. E. Jr., Erber, J., and Scheiner, R. (2006). Downregulation of vitellogenin gene activity increases the gustatory responsiveness of honey bee workers (Apis mellifera). Behav. Brain Res. 169, 201–205. doi: 10.1016/j.bbr.2006.01.006
Asgari, S. (2013). MicroRNA functions in insects. Insect Biochem. Mol. Biol. 43, 388–397. doi: 10.1016/j.ibmb.2012.10.005
Azzam, G., Smibert, P., Lai, E. C., and Liu, J. L. (2012). Drosophila Argonaute 1 and its miRNA biogenesis partners are required for oocyte formation and germline cell division. Dev. Biol. 365, 384–394. doi: 10.1016/j.ydbio.2012.03.005
Badisco, L., Van Wielendaele, P., and Vanden Broeck, J. (2013). Eat to reproduce: a key role for the insulin signaling pathway in adult insects. Front. Physiol. 4:202. doi: 10.3389/fphys.2013.00202
Bai, H., and Palli, S. R. (2016). Identification of G protein-coupled receptors required for vitellogenin uptake into the oocytes of the red flour beetle, Tribolium castaneum. Sci. Rep. 6:27648. doi: 10.1038/srep27648
Bai, H., Qiao, H., Li, F., Fu, H., Sun, S., Zhang, W., et al. (2015). Molecular characterization and developmental expression of vitellogenin in the oriental river prawn Macrobrachium nipponense and the effects of RNA interference and eyestalk ablation on ovarian maturation. Gene 562, 22–31. doi: 10.1016/j.gene.2014.12.008
Belles, X. (2004). “Vitellogenesis directed by juvenile hormone,” in Reproductive Biology of Invertebrates, ed A. S. Raikhel (Enfield/Plymouth: Science Publisher, Inc.), 157–197.
Berger, E. M., and Dubrovsky, E. B. (2005). Juvenile hormone molecular actions and interactions during development of Drosophila melanogaster. Vitam. Horm. 73, 175–215. doi: 10.1016/S0083-6729(05)73006-5
Bownes, M. (1990). The yolk proteins and their genes in Drosophila. Prog. Clin. Biol. Res. 342, 336–342.
Bryant, B., Macdonald, W., and Raikhel, A. S. (2010). microRNA miR-275 is indispensable for blood digestion and egg development in the mosquito Aedes aegypti. Proc. Natl. Acad. Sci. U. S. A. 107, 22391–22398. doi: 10.1073/pnas.1016230107
Carney, G. E., and Bender, M. (2000). The Drosophila ecdysone receptor (EcR) gene is required maternally for normal oogenesis. Genetics 154, 1203–1211.
Carpenter, V. K., Drake, L. L., Aguirre, S. E., Price, D. P., Rodriguez, S. D., and Hansen, I. A. (2012). SLC7 amino acid transporters of the yellow fever mosquito Aedes aegypti and their role in fat body TOR signaling and reproduction. J. Insect Physiol. 58, 513–522. doi: 10.1016/j.jinsphys.2012.01.005
Charles, J. P., Iwema, T., Epa, V. C., Takaki, K., Rynes, J., and Jindra, M. (2011). Ligand-binding properties of a juvenile hormone receptor, Methoprene-tolerant. Proc. Natl. Acad. Sci. U. S. A. 108, 21128–21133. doi: 10.1073/pnas.1116123109
Chen, E., Chen, Z., Li, S., Xing, D., Guo, H., Liu, J., et al. (2020). bmo-miR-2739 and the novel microRNA miR-167 coordinately regulate the expression of the vitellogenin receptor in Bombyx mori oogenesis. Development 147:dev183723. doi: 10.1242/dev.183723
Chen, M. E., Lewis, D. K., Keeley, L. L., and Pietrantonio, P. V. (2004). cDNA cloning and transcriptional regulation of the vitellogenin receptor from the imported fire ant, Solenopsis invicta Buren (Hymenoptera: Formicidae). Insect Mol. Biol. 13, 195–204. doi: 10.1111/j.0962-1075.2004.00477.x
Cong, L., Yang, W. J., Jiang, X. Z., Niu, J. Z., Shen, G. M., Ran, C., et al. (2015). The essential role of vitellogenin receptor in ovary development and vitellogenin uptake in Bactrocera dorsalis (Hendel). Int. J. Mol. Sci. 16, 18368–18383. doi: 10.3390/ijms160818368
Corona, M., Libbrecht, R., Wurm, Y., Riba-Grognuz, O., Studer, R. A., and Keller, L. (2013). Vitellogenin underwent subfunctionalization to acquire caste and behavioral specific expression in the harvester ant Pogonomyrmex barbatus. PLoS Genet. 9:e1003730. doi: 10.1371/journal.pgen.1003730
Corona, M., Velarde, R. A., Remolina, S., Moran-Lauter, A., Wang, Y., Hughes, K. A., et al. (2007). Vitellogenin, juvenile hormone, insulin signaling, and queen honey bee longevity. Proc. Natl. Acad. Sci. U. S. A. 104, 7128–7133. doi: 10.1073/pnas.0701909104
Dhara, A., Eum, J. H., Robertson, A., Gulia-Nuss, M., Vogel, K. J., Clark, K. D., et al. (2013). Ovary ecdysteroidogenic hormone functions independently of the insulin receptor in the yellow fever mosquito, Aedes aegypti. Insect Biochem. Mol. Biol. 43, 1100–1108. doi: 10.1016/j.ibmb.2013.09.004
Elmogy, M., Elgendy, A. M., and Takeda, M. (2014). Detection of small GTP binding proteins showing GTPase and GTP/ATP binding activities in the ovary of the American Cockroach, Periplaneta Americana, during oogenesis. Am. J. BioSci. 2:106. doi: 10.11648/j.ajbio.20140203.15
Fambrough, D. M., Lemas, M. V., Hamrick, M., Emerick, M., Renaud, K. J., Inman, E. M., et al. (1994). Analysis of subunit assembly of the Na-K-ATPase. Am. J. Physiol.-Cell Physiol. 266:C579-89. doi: 10.1152/ajpcell.1994.266.3.C579
Fu, X., Dimopoulos, G., and Zhu, J. (2017). Association of microRNAs with Argonaute proteins in the malaria mosquito Anopheles gambiae after blood ingestion. Sci. Rep. 7:6493. doi: 10.1038/s41598-017-07013-1
Fu, X., Li, T., Chen, J., Dong, Y., Qiu, J., Kang, K., et al. (2015). Functional screen for microRNAs of Nilaparvata lugens reveals that targeting of glutamine synthase by miR-4868b regulates fecundity. J. Insect Physiol. 83, 22–29. doi: 10.1016/j.jinsphys.2015.11.003
Fujiwara, Y., Tanaka, Y., Iwata, K., Rubio, R. O., Yaginuma, T., Yamashita, O., et al. (2006). ERK/MAPK regulates ecdysteroid and sorbitol metabolism for embryonic diapause termination in the silkworm, Bombyx mori. J. Insect Physiol. 52, 569–575. doi: 10.1016/j.jinsphys.2006.02.004
Garcia, J., Munro, E. S., Monte, M. M., Fourrier, M. C., Whitelaw, J., Smail, D. A., et al. (2010). Atlantic salmon (Salmo salar L.) serum vitellogenin neutralises infectivity of infectious pancreatic necrosis virus (IPNV). Fish Shellfish Immunol. 29, 293–297. doi: 10.1016/j.fsi.2010.04.010
Gilbert, L. I., Serafin, R. B., Watkins, N. L., and Richard, D. S. (1998). Ecdysteroids regulate yolk protein uptake by Drosophila melanogaster oocytes. J. Insect Physiol. 44, 637–644. doi: 10.1016/S0022-1910(98)00020-1
Gujar, H., and Palli, S. R. (2016). Juvenile hormone regulation of female reproduction in the common bed bug, Cimex lectularius. Sci. Rep. 6:35546. doi: 10.1038/srep35546
Guo, W., Wu, Z., Song, J., Jiang, F., Wang, Z., Deng, S., et al. (2014). Juvenile hormone-receptor complex acts on mcm4 and mcm7 to promote polyploidy and vitellogenesis in the migratory locust. PLoS Genet. 10:e1004702. doi: 10.1371/journal.pgen.1004702
Guo, W., Wu, Z., Yang, L., Cai, Z., Zhao, L., and Zhou, S. (2019). Juvenile hormone-dependent Kazal-type serine protease inhibitor Greglin safeguards insect vitellogenesis and egg production. FASEB J. 33, 917–927. doi: 10.1096/fj.201801068R
Guo, Y., Hoffmann, A. A., Xu, X. Q., Mo, P. W., Huang, H. J., Gong, J. T., et al. (2018). Vertical transmission of Wolbachia is associated with host vitellogenin in Laodelphax striatellus. Front. Microbiol. 9:2016. doi: 10.3389/fmicb.2018.02016
Hansen, I. A., Attardo, G. M., Park, J. H., Peng, Q., and Raikhel, A. S. (2004). Target of rapamycin-mediated amino acid signaling in mosquito anautogeny. Proc. Natl. Acad. Sci. U. S. A. 101, 10626–10631. doi: 10.1073/pnas.0403460101
Hansen, I. A., Attardo, G. M., Rodriguez, S. D., and Drake, L. L. (2014). Four-way regulation of mosquito yolk protein precursor genes by juvenile hormone-, ecdysone-, nutrient-, and insulin-like peptide signaling pathways. Front. Physiol. 5:103. doi: 10.3389/fphys.2014.00103
Hansen, I. A., Sieglaff, D. H., Munro, J. B., Shiao, S. H., Cruz, J., Lee, I. W., et al. (2007). Forkhead transcription factors regulate mosquito reproduction. Insect Biochem. Mol. Biol. 37, 985–997. doi: 10.1016/j.ibmb.2007.05.008
He, J., Chen, Q., Wei, Y., Jiang, F., Yang, M., Hao, S., et al. (2016). MicroRNA-276 promotes egg-hatching synchrony by up-regulating brm in locusts. Proc. Natl. Acad. Sci. U. S. A. 113, 584–589. doi: 10.1073/pnas.1521098113
He, K., Lin, K., Ding, S., Wang, G., and Li, F. (2019). The vitellogenin receptor has an essential role in vertical transmission of rice stripe virus during oogenesis in the small brown plant hopper. Pest Manag. Sci. 75, 1370–1382. doi: 10.1002/ps.5256
Hu, K., Tian, P., Tang, Y., Yang, L., Qiu, L., He, H., et al. (2019). Molecular characterization of vitellogenin and its receptor in Sogatella furcifera, and their function in oocyte maturation. Front. Physiol. 10:1532. doi: 10.3389/fphys.2019.01532
Huo, Y., Yu, Y., Chen, L., Li, Q., Zhang, M., Song, Z., et al. (2018). Insect tissue-specific vitellogenin facilitates transmission of plant virus. PLoS Pathog. 14:e1006909. doi: 10.1371/journal.ppat.1006909
Huo, Y., Yu, Y., Liu, Q., Liu, D., Zhang, M., Liang, J., et al. (2019). Rice stripe virus hitchhikes the vector insect vitellogenin ligand-receptor pathway for ovary entry. Philos. Trans. R. Soc. Lond. B Biol. Sci. 374:20180312. doi: 10.1098/rstb.2018.0312
Ihle, K. E., Fondrk, M. K., Page, R. E., and Amdam, G. V. (2015). Genotype effect on lifespan following vitellogenin knockdown. Exp. Gerontol. 61, 113–122. doi: 10.1016/j.exger.2014.12.007
Irles, P., Ramos, S., and Piulachs, M. D. (2017). SPARC preserves follicular epithelium integrity in insect ovaries. Dev. Biol. 422, 105–114. doi: 10.1016/j.ydbio.2017.01.005
Irles, P., Silva-Torres, F. A., and Piulachs, M. D. (2013). RNAi reveals the key role of Nervana 1 in cockroach oogenesis and embryo development. Insect Biochem. Mol. Biol. 43, 178–188. doi: 10.1016/j.ibmb.2012.12.003
Iwata, K., Shindome, C., Kobayashi, Y., Takeda, M., Yamashita, O., Shiomi, K., et al. (2005). Temperature-dependent activation of ERK/MAPK in yolk cells and its role in embryonic diapause termination in the silkworm Bombyx mori. J. Insect Physiol. 51, 1306–1312. doi: 10.1016/j.jinsphys.2005.07.009
Jin, Z., and Xie, T. (2007). Dcr-1 maintains Drosophila ovarian stem cells. Curr. Biol. 17, 539–544. doi: 10.1016/j.cub.2007.01.050
Jindra, M., Belles, X., and Shinoda, T. (2015). Molecular basis of juvenile hormone signaling. Curr. Opin. Insect Sci. 11, 39–46. doi: 10.1016/j.cois.2015.08.004
Jindra, M., Palli, S. R., and Riddiford, L. M. (2013). The juvenile hormone signaling pathway in insect development. Annu. Rev. Entomol. 58, 181–204. doi: 10.1146/annurev-ento-120811-153700
Jing, Y. P., An, H., Zhang, S., Wang, N., and Zhou, S. (2018). Protein kinase C mediates juvenile hormone-dependent phosphorylation of Na(+)/K(+)-ATPase to induce ovarian follicular patency for yolk protein uptake. J. Biol. Chem. 293, 20112–20122. doi: 10.1074/jbc.RA118.005692
Kamruzzaman, A. S. M., Mikani, A., Mohamed, A. A., Elgendy, A. M., and Takeda, M. (2020). Crosstalk among indoleamines, neuropeptides and JH/20E in regulation of reproduction in the American Cockroach, Periplaneta americana. Insects 11:155. doi: 10.3390/insects11030155
Kaplan, J. H. (2002). Biochemistry of Na,K-ATPase. Annu. Rev. Biochem. 71, 511–535. doi: 10.1146/annurev.biochem.71.102201.141218
Kayukawa, T., Minakuchi, C., Namiki, T., Togawa, T., Yoshiyama, M., Kamimura, M., et al. (2012). Transcriptional regulation of juvenile hormone-mediated induction of Krüppel homolog 1, a repressor of insect metamorphosis. Proc. Natl. Acad. Sci. U. S. A. 109, 11729–11734. doi: 10.1073/pnas.1204951109
Koyama, T., Mendes, C. C., and Mirth, C. K. (2013). Mechanisms regulating nutrition-dependent developmental plasticity through organ-specific effects in insects. Front. Physiol. 4:263. doi: 10.3389/fphys.2013.00263
Lee, K. Y., Yoon, H. J., and Jin, B. R. (2015). Osmia cornifrons vitellogenin: cDNA cloning, structural analysis and developmental expression. Entomol. Res. 45, 94–101. doi: 10.1111/1748-5967.12098
Lenaerts, C., Monjon, E., Van Lommel, J., Verbakel, L., and Vanden Broeck, J. (2019). Peptides in insect oogenesis. Curr. Opin. Insect Sci. 31, 58–64. doi: 10.1016/j.cois.2018.08.007
Li, K. L., Wan, P. J., Wang, W. X., Lai, F. X., and Fu, Q. (2015). Ran involved in the development and reproduction is a potential target for rna-interference-based pest management in Nilaparvata lugens. PLoS ONE 10:e0142142. doi: 10.1371/journal.pone.0142142
Li, M., Mead, E. A., and Zhu, J. (2011). Heterodimer of two bHLH-PAS proteins mediates juvenile hormone-induced gene expression. Proc. Natl. Acad. Sci. U. S. A. 108, 638–643. doi: 10.1073/pnas.1013914108
Lin, X., Yao, Y., and Wang, B. (2015). Methoprene-tolerant (Met) and Krüpple-homologue 1 (Kr-h1) are required for ovariole development and egg maturation in the brown plant hopper. Sci. Rep. 5:18064. doi: 10.1038/srep18064
Liu, P., Fu, X., and Zhu, J. (2018). Juvenile hormone-regulated alternative splicing of the taiman gene primes the ecdysteroid response in adult mosquitoes. Proc. Natl. Acad. Sci. U. S. A. 115, E7738–E7747. doi: 10.1073/pnas.1808146115
Liu, P., Peng, H. J., and Zhu, J. (2015). Juvenile hormone-activated phospholipase C pathway enhances transcriptional activation by the methoprene-tolerant protein. Proc. Natl. Acad. Sci. U. S. A. 112, E1871–1879. doi: 10.1073/pnas.1423204112
Liu, S., Lucas, K. J., Roy, S., Ha, J., and Raikhel, A. S. (2014). Mosquito-specific microRNA-1174 targets serine hydroxymethyltransferase to control key functions in the gut. Proc. Natl. Acad. Sci. U. S. A. 111, 14460–14465. doi: 10.1073/pnas.1416278111
Liu, W., Guo, S., Sun, D., Zhu, L., Zhu, F., Lei, C. L., et al. (2019). Molecular characterization and juvenile hormone-regulated transcription of the vitellogenin receptor in the cabbage beetle Colaphellus bowringi. Comp. Biochem. Physiol. Part A Mol. Integr. Physiol. 229, 69–75. doi: 10.1016/j.cbpa.2018.12.004
Lu, K., Chen, X., Liu, W. T., and Zhou, Q. (2016). TOR pathway-mediated juvenile hormone synthesis regulates nutrient-dependent female reproduction in Nilaparvata lugens (Stål). Int. J. Mol. Sci. 17:438. doi: 10.3390/ijms17040438
Lu, K., Shu, Y., Zhou, J., Zhang, X., Zhang, X., Chen, M., et al. (2015). Molecular characterization and RNA interference analysis of vitellogenin receptor from Nilaparvata lugens (Stål). J. Insect Physiol. 73, 20–29. doi: 10.1016/j.jinsphys.2015.01.007
Lucas, K. J., Roy, S., Ha, J., Gervaise, A. L., Kokoza, V. A., and Raikhel, A. S. (2015a). MicroRNA-8 targets the Wingless signaling pathway in the female mosquito fat body to regulate reproductive processes. Proc. Natl. Acad. Sci. U. S. A. 112, 1440–1445. doi: 10.1073/pnas.1424408112
Lucas, K. J., Zhao, B., Roy, S., Gervaise, A. L., and Raikhel, A. S. (2015b). Mosquito-specific microRNA-1890 targets the juvenile hormone-regulated serine protease JHA15 in the female mosquito gut. RNA Biol. 12, 1383–1390. doi: 10.1080/15476286.2015.1101525
Luo, M., Li, D., Wang, Z., Guo, W., Kang, L., and Zhou, S. (2017). Juvenile hormone differentially regulates two Grp78 genes encoding protein chaperones required for insect fat body cell homeostasis and vitellogenesis. J. Biol. Chem. 292, 8823–8834. doi: 10.1074/jbc.M117.780957
Ma, L., Zhang, W., Liu, C., Chen, L., Xu, Y., Xiao, H., et al. (2018). Methoprene-tolerant (Met) is indispensable for larval metamorphosis and female reproduction in the cotton bollworm Helicoverpa armigera. Front. Physiol. 9:1601. doi: 10.3389/fphys.2018.01601
Matsumoto, T., Yamano, K., Kitamura, M., and Hara, A. (2008). Ovarian follicle cells are the site of vitellogenin synthesis in the Pacific abalone Haliotis discus hannai. Comp. Biochem. Physiol. Part A Mol. Integr. Physiol. 149, 293–298. doi: 10.1016/j.cbpa.2008.01.003
Melo, A. C., Valle, D., Machado, E. A., Salerno, A. P., Paiva-Silva, G. O., Cunha, E. S. N. L., et al. (2000). Synthesis of vitellogenin by the follicle cells of Rhodnius prolixus. Insect Biochem. Mol. Biol. 30, 549–557. doi: 10.1016/S0965-1748(00)00023-0
Mitchell, R. D. III, Sonenshine, D. E., and Pérez De León, A. A. (2019). Vitellogenin receptor as a target for tick control: a mini-review. Front. Physiol. 10:618. doi: 10.3389/fphys.2019.00618
Morandin, C., Havukainen, H., Kulmuni, J., Dhaygude, K., Trontti, K., and Helantera, H. (2014). Not only for egg yolk–functional and evolutionary insights from expression, selection, and structural analyses of Formica ant vitellogenins. Mol. Biol. Evol. 31, 2181–2193. doi: 10.1093/molbev/msu171
Ojani, R., Fu, X., Ahmed, T., Liu, P., and Zhu, J. (2018). Krüppel homologue 1 acts as a repressor and an activator in the transcriptional response to juvenile hormone in adult mosquitoes. Insect Mol. Biol. 27, 268–278. doi: 10.1111/imb.12370
Ojani, R., Liu, P., Fu, X., and Zhu, J. (2016). Protein kinase C modulates transcriptional activation by the juvenile hormone receptor methoprene-tolerant. Insect Biochem. Mol. Biol. 70, 44–52. doi: 10.1016/j.ibmb.2015.12.001
Parthasarathy, R., and Palli, S. R. (2011). Molecular analysis of nutritional and hormonal regulation of female reproduction in the red flour beetle, Tribolium castaneum. Insect Biochem. Mol. Biol. 41, 294–305. doi: 10.1016/j.ibmb.2011.01.006
Parthasarathy, R., Sheng, Z., Sun, Z., and Palli, S. R. (2010a). Ecdysteroid regulation of ovarian growth and oocyte maturation in the red flour beetle, Tribolium castaneum. Insect Biochem. Mol. Biol. 40, 429–439. doi: 10.1016/j.ibmb.2010.04.002
Parthasarathy, R., Sun, Z., Bai, H., and Palli, S. R. (2010b). Juvenile hormone regulation of vitellogenin synthesis in the red flour beetle, Tribolium castaneum. Insect Biochem. Mol. Biol. 40, 405–414. doi: 10.1016/j.ibmb.2010.03.006
Peng, L., Wang, L., Zou, M. M., Vasseur, L., Chu, L. N., Qin, Y. D., et al. (2019). Identification of halloween genes and RNA interference-mediated functional characterization of a halloween gene shadow in Plutella xylostella. Front. Physiol. 10:1120. doi: 10.3389/fphys.2019.01120
Pszczolkowski, M. A., Olson, E., Rhine, C., and Ramaswamy, S. B. (2008). Role for calcium in the development of ovarial patency in Heliothis virescens. J. Insect Physiol. 54, 358–366. doi: 10.1016/j.jinsphys.2007.10.005
Pszczolkowski, M. A., Peterson, A., Srinivasan, A., and Ramaswamy, S. B. (2005). Pharmacological analysis of ovarial patency in Heliothis virescens. J. Insect Physiol. 51, 445–453. doi: 10.1016/j.jinsphys.2005.01.008
Raikhel, A. S., Brown, M. R., and Belles, X. (2005). “Hormonal control of reproductive processes,” in Comprehensive Molecular Insect Science, eds. L. I. Gilbert, K. Iatrou, and S. S. Gill (Boston: Elsevier), 433–491. doi: 10.1016/B0-44-451924-6/00040-5
Raikhel, A. S., and Dhadialla, T. S. (1992). Accumulation of yolk proteins in insect oocytes. Annu. Rev. Entomol. 37, 217–251. doi: 10.1146/annurev.en.37.010192.001245
Raikhel, A. S., Kokoza, V. A., Zhu, J., Martin, D., Wang, S. F., Li, C., et al. (2002). Molecular biology of mosquito vitellogenesis: from basic studies to genetic engineering of antipathogen immunity. Insect Biochem. Mol. Biol. 32, 1275–1286. doi: 10.1016/S0965-1748(02)00090-5
Roy, S., Saha, T. T., Zou, Z., and Raikhel, A. S. (2018). Regulatory pathways controlling female insect reproduction. Annu. Rev. Entomol. 63, 489–511. doi: 10.1146/annurev-ento-020117-043258
Saha, T. T., Roy, S., Pei, G., Dou, W., Zou, Z., and Raikhel, A. S. (2019). Synergistic action of the transcription factors Krüppel homolog 1 and Hairy in juvenile hormone/Methoprene-tolerant-mediated gene-repression in the mosquito Aedes aegypti. PLoS Genet. 15:e1008443. doi: 10.1371/journal.pgen.1008443
Salmela, H., Amdam, G. V., and Freitak, D. (2015). Transfer of immunity from mother to offspring is mediated via egg-yolk protein vitellogenin. PLoS Pathog. 11:e1005015. doi: 10.1371/journal.ppat.1005015
Sancak, Y., Peterson, T. R., Shaul, Y. D., Lindquist, R. A., Thoreen, C. C., Bar-Peled, L., et al. (2008). The Rag GTPases bind raptor and mediate amino acid signaling to mTORC1. Science 320, 1496–1501. doi: 10.1126/science.1157535
Santos, C. G., Humann, F. C., and Hartfelder, K. (2019). Juvenile hormone signaling in insect oogenesis. Curr. Opin. Insect Sci. 31, 43–48. doi: 10.1016/j.cois.2018.07.010
Sappington, T. W., and Raikhel, A. S. (1998). Molecular characteristics of insect vitellogenins and vitellogenin receptors. Insect Biochem. Mol. Biol. 28, 277–300. doi: 10.1016/S0965-1748(97)00110-0
Schonbaum, C., Lee, S., and Mahowald, A. P. (1995). The Drosophila yolkless gene encodes a vitellogenin receptor belonging to the low density lipoprotein receptor superfamily. Proc. Natl. Acad. Sci. U. S. A. 92, 1485–1489. doi: 10.1073/pnas.92.5.1485
Seehuus, S. C., Norberg, K., Gimsa, U., Krekling, T., and Amdam, G. V. (2006). Reproductive protein protects functionally sterile honey bee workers from oxidative stress. Proc. Natl. Acad. Sci. U. S. A. 103, 962–967. doi: 10.1073/pnas.0502681103
Sevala, V. L., and Davey, K. G. (1989). Action of juvenile hormone on the follicle cells of Rhodnius prolixus: evidence for a novel regulatory mechanism involving protein kinase C. Experientia 45, 355–356. doi: 10.1007/BF01957476
Sevala, V. L., and Davey, K. G. (1993). Juvenile hormone dependent phosphorylation of a 100 kDa polypeptide is mediated by protein kinase C in the follicle cells of Rhodnius prolixus. Invert. Reprod. Dev. 23, 189–193. doi: 10.1080/07924259.1993.9672314
Shcherbata, H. R. (2019). miRNA functions in stem cells and their niches: lessons from the Drosophila ovary. Curr. Opin. Insect Sci. 31, 29–36. doi: 10.1016/j.cois.2018.07.006
Sheng, Z., Xu, J., Bai, H., Zhu, F., and Palli, S. R. (2011). Juvenile hormone regulates vitellogenin gene expression through insulin-like peptide signaling pathway in the red flour beetle, Tribolium castaneum. J. Biol. Chem. 286, 41924–41936. doi: 10.1074/jbc.M111.269845
Shin, S. W., Zou, Z., Saha, T. T., and Raikhel, A. S. (2012). bHLH-PAS heterodimer of methoprene-tolerant and Cycle mediates circadian expression of juvenile hormone-induced mosquito genes. Proc. Natl. Acad. Sci. U. S. A. 109, 16576–16581. doi: 10.1073/pnas.1214209109
Shpigler, H., Amsalem, E., Huang, Z. Y., Cohen, M., Siegel, A. J., Hefetz, A., et al. (2014). Gonadotropic and physiological functions of juvenile hormone in Bumblebee (Bombus terrestris) workers. PLoS ONE 9:e100650. doi: 10.1371/journal.pone.0100650
Smykal, V., Bajgar, A., Provaznik, J., Fexova, S., Buricova, M., Takaki, K., et al. (2014). Juvenile hormone signaling during reproduction and development of the linden bug, Pyrrhocoris apterus. Insect Biochem. Mol. Biol. 45, 69–76. doi: 10.1016/j.ibmb.2013.12.003
Smykal, V., and Raikhel, A. S. (2015). Nutritional control of insect reproduction. Curr. Opin. Insect Sci. 11, 31–38. doi: 10.1016/j.cois.2015.08.003
Snigirevskaya, E. S., and Raikhel, A. S. (2005). “Receptor-mediated endocytosis of yolkproteins in insect oocytes,” in Progress in Vitellogenesis. Reproductive Biology of Invertebrates, eds A. S. Raikhel and T. W. Sappington (Enfield; Plymouth: Science Publishers, Inc), 199–228.
Song, J., Guo, W., Jiang, F., Kang, L., and Zhou, S. (2013). Argonaute 1 is indispensable for juvenile hormone mediated oogenesis in the migratory locust, Locusta migratoria. Insect Biochem. Mol. Biol. 43, 879–887. doi: 10.1016/j.ibmb.2013.06.004
Song, J., Li, W., Zhao, H., Gao, L., Fan, Y., and Zhou, S. (2018). The microRNAs let-7 and miR-278 regulate insect metamorphosis and oogenesis by targeting the juvenile hormone early-response gene Krüppel-homolog 1. Development 145:dev170670. doi: 10.1242/dev.170670
Song, J., Li, W., Zhao, H., and Zhou, S. (2019). Clustered miR-2, miR-13a, miR-13b and miR-71 coordinately target Notch gene to regulate oogenesis of the migratory locust Locusta migratoria. Insect Biochem. Mol. Biol. 106, 39–46. doi: 10.1016/j.ibmb.2018.11.004
Song, J., Wu, Z., Wang, Z., Deng, S., and Zhou, S. (2014). Krüppel-homolog 1 mediates juvenile hormone action to promote vitellogenesis and oocyte maturation in the migratory locust. Insect Biochem. Mol. Biol. 52, 94–101. doi: 10.1016/j.ibmb.2014.07.001
Song, J., and Zhou, S. (2020). Post-transcriptional regulation of insect metamorphosis and oogenesis. Cell. Mol. Life Sci. 77, 1893–1909. doi: 10.1007/s00018-019-03361-5
Suren-Castillo, S., Abrisqueta, M., and Maestro, J. L. (2012). FoxO inhibits juvenile hormone biosynthesis and vitellogenin production in the German cockroach. Insect Biochem. Mol. Biol. 42, 491–498. doi: 10.1016/j.ibmb.2012.03.006
Swevers, L. (2019). An update on ecdysone signaling during insect oogenesis. Curr. Opin. Insect Sci. 31, 8–13. doi: 10.1016/j.cois.2018.07.003
Swevers, L., and Iatrou, K. (2003). The ecdysone regulatory cascade and ovarian development in lepidopteran insects: insights from the silkmoth paradigm. Insect Biochem. Mol. Biol. 33, 1285–1297. doi: 10.1016/j.ibmb.2003.06.012
Tanaka, E. D., and Piulachs, M. D. (2012). Dicer-1 is a key enzyme in the regulation of oogenesis in panoistic ovaries. Biol. Cell. 104, 452–461. doi: 10.1111/boc.201100044
Tang, Y., He, H., Qu, X., Cai, Y., Ding, W., Qiu, L., et al. (2020). RNA interference-mediated knockdown of the transcription factor Kruppel homologue 1 suppresses vitellogenesis in Chilo suppressalis. Insect Mol. Biol. 29, 183–192. doi: 10.1111/imb.12617
Telfer, W. H. (2009). Egg formation in lepidoptera. J. Insect Sci. 9, 1–21. doi: 10.1673/031.009.5001
Tu, M. P., Yin, C. M., and Tatar, M. (2002). Impaired ovarian ecdysone synthesis of Drosophila melanogaster insulin receptor mutants. Aging Cell 1, 158–160. doi: 10.1046/j.1474-9728.2002.00016.x
Tufail, M., and Takeda, M. (2008). Molecular characteristics of insect vitellogenins. J. Insect Physiol. 54, 1447–1458. doi: 10.1016/j.jinsphys.2008.08.007
Tufail, M., and Takeda, M. (2009). Insect vitellogenin/lipophorin receptors: molecular structures, role in oogenesis, and regulatory mechanisms. J. Insect Physiol. 55, 87–103. doi: 10.1016/j.jinsphys.2008.11.007
Van Hoef, V., Breugelmans, B., Spit, J., Simonet, G., Zels, S., and Vanden Broeck, J. (2013). Phylogenetic distribution of protease inhibitors of the Kazal-family within the Arthropoda. Peptides 41, 59–65. doi: 10.1016/j.peptides.2012.10.015
Vasudevan, S., Tong, Y., and Steitz, J. A. (2007). Switching from repression to activation: microRNAs can up-regulate translation. Science 318, 1931–1934. doi: 10.1126/science.1149460
Wang, J. L., Saha, T. T., Zhang, Y., Zhang, C., and Raikhel, A. S. (2017). Juvenile hormone and its receptor methoprene-tolerant promote ribosomal biogenesis and vitellogenesis in the Aedes aegypti mosquito. J. Biol. Chem. 292, 10306–10315. doi: 10.1074/jbc.M116.761387
Wang, Y., Baker, N., and Amdam, G. V. (2013). RNAi-mediated double gene knockdown and gustatory perception measurement in honey bees (Apis mellifera). J. Vis. Exp. 77:50446. doi: 10.3791/50446
Wang, Y., Brent, C. S., Fennern, E., and Amdam, G. V. (2012). Gustatory perception and fat body energy metabolism are jointly affected by vitellogenin and juvenile hormone in honey bees. PLoS Genet. 8:e1002779. doi: 10.1371/journal.pgen.1002779
Wang, Z., Yang, L., Song, J., Kang, L., and Zhou, S. (2017). An isoform of Taiman that contains a PRD-repeat motif is indispensable for transducing the vitellogenic juvenile hormone signal in Locusta migratoria. Insect Biochem. Mol. Biol. 82, 31–40. doi: 10.1016/j.ibmb.2017.01.009
Wu, Z., Guo, W., Xie, Y., and Zhou, S. (2016). Juvenile hormone activates the transcription of cell-division-cycle 6 (Cdc6) for polyploidy-dependent insect vitellogenesis and oogenesis. J. Biol. Chem. 291, 5418–5427. doi: 10.1074/jbc.M115.698936
Wu, Z., Guo, W., Yang, L., He, Q., and Zhou, S. (2018). Juvenile hormone promotes locust fat body cell polyploidization and vitellogenesis by activating the transcription of Cdk6 and E2f1. Insect Biochem. Mol. Biol. 102, 1–10. doi: 10.1016/j.ibmb.2018.09.002
Wu, Z., He, Q., Zeng, B., Zhou, H., and Zhou, S. (2020). Juvenile hormone acts through FoxO to promote Cdc2 and Orc5 transcription for polyploidy-dependent vitellogenesis. Development 147:dev188813. doi: 10.1242/dev.188813
Wyatt, G. R., and Davey, K. G. (1996). Cellular and molecular actions of juvenile hormone II. Roles of juvenile hormone in adult insects. Adv. In. Insect Phys. 26, 1–155. doi: 10.1016/S0065-2806(08)60030-2
Yang, M., Wei, Y., Jiang, F., Wang, Y., Guo, X., He, J., et al. (2014). MicroRNA-133 inhibits behavioral aggregation by controlling dopamine synthesis in locusts. PLoS Genet. 10:e1004206. doi: 10.1371/journal.pgen.1004206
Yue, Y., Yang, R. L., Wang, W. P., Zhou, Q. H., Chen, E. H., Yuan, G. R., et al. (2018). Involvement of Met and Kr-h1 in JH-Mediated Reproduction of Female Bactrocera dorsalis (Hendel). Front. Physiol. 9:482. doi: 10.3389/fphys.2018.00482
Zeng, B., Huang, Y., Xu, J., Shiotsuki, T., Bai, H., Palli, S. R., et al. (2017). The FOXO transcription factor controls insect growth and development by regulating juvenile hormone degradation in the silkworm, Bombyx mori. J. Biol. Chem. 292, 11659–11669. doi: 10.1074/jbc.M117.777797
Zhang, S., Dong, Y., and Cui, P. (2015). Vitellogenin is an immunocompetent molecule for mother and offspring in fish. Fish Shellfish Immunol. 46, 710–715. doi: 10.1016/j.fsi.2015.08.011
Zhang, X., Aksoy, E., Girke, T., Raikhel, A. S., and Karginov, F. V. (2017). Transcriptome-wide microRNA and target dynamics in the fat body during the gonadotrophic cycle of Aedes aegypti. Proc. Natl. Acad. Sci. U. S. A. 114, E1895–e1903. doi: 10.1073/pnas.1701474114
Zhang, X., Lu, K., and Zhou, Q. (2013). Dicer1 is crucial for the oocyte maturation of telotrophic ovary in Nilaparvata lugens (STÅL) (Hemiptera: Geometroidea). Arch. Insect Biochem. Physiol. 84, 194–208. doi: 10.1002/arch.21136
Zhao, J., Sun, Y., Xiao, L., Tan, Y., Jiang, Y., and Bai, L. (2018). Vitellogenin and vitellogenin receptor gene expression profiles in Spodoptera exigua are related to host plant suitability. Pest Manag. Sci. 74, 950–958. doi: 10.1002/ps.4794
Zheng, H., Zeng, B., Shang, T., and Zhou, S. (2020). Identification of G protein-coupled receptors required for vitellogenesis and egg development in an insect with panoistic ovary. Insect Sci. doi: 10.1111/1744-7917.12841
Zhou, X., Duan, X., Qian, J., and Li, F. (2009). Abundant conserved microRNA target sites in the 5'-untranslated region and coding sequence. Genetica 137, 159–164. doi: 10.1007/s10709-009-9378-7
Zhu, S., Liu, F., Zeng, H., Li, N., Ren, C., Su, Y., et al. (2020). Insulin/IGF signaling and TORC1 promote vitellogenesis via inducing juvenile hormone biosynthesis in the American cockroach. Development 147:dev188805. doi: 10.1242/dev.188805
Keywords: vitellogenin, juvenile hormone, ecdysone, nutrition, insect reproduction
Citation: Wu Z, Yang L, He Q and Zhou S (2021) Regulatory Mechanisms of Vitellogenesis in Insects. Front. Cell Dev. Biol. 8:593613. doi: 10.3389/fcell.2020.593613
Received: 11 August 2020; Accepted: 22 December 2020;
Published: 28 January 2021.
Edited by:
Graça Soveral, University of Lisbon, PortugalReviewed by:
Kai Lu, Fujian Agriculture and Forestry University, ChinaZilá Luz Paulino Simões, University of São Paulo, Brazil
Copyright © 2021 Wu, Yang, He and Zhou. This is an open-access article distributed under the terms of the Creative Commons Attribution License (CC BY). The use, distribution or reproduction in other forums is permitted, provided the original author(s) and the copyright owner(s) are credited and that the original publication in this journal is cited, in accordance with accepted academic practice. No use, distribution or reproduction is permitted which does not comply with these terms.
*Correspondence: Shutang Zhou, c3pob3VAaGVudS5lZHUuY24=
†These authors have contributed equally to this work