- 1Laboratory Medicine College, Dalian Medical University, Dalian, China
- 2Department of Central Laboratory, Dalian Municipal Central Hospital Affiliated of Dalian Medical University, Dalian, China
PM2.5 refers to atmospheric particulate matters with a diameter of less than 2.5 μm. The deposit of PM2.5 in lung cells can cause oxidative stress, leading to changes in macrophage polarity, which can subsequently cause pulmonary inflammation. Long-chain non-coding RNA (lncRNA) is a class of transcripts that regulate biological processes through multiple mechanisms. However, the role of lncRNA in PM2.5-induced lung inflammation has not been established. In this study, the biological effects and associated mechanism of lncRNA in PM2.5-induced change in macrophage polarity were investigated. The lncRNA-mediated PM2.5-induced macrophage inflammation and lung inflammation-associated injury were also determined. Mice were exposed to chronic levels of PM2.5, and changes in the expression of lncRNA in the lung were measured by lncRNA microarray. lncRNAs that showed significant changes in expression in response to PM2.5 were identified. lncRNA showing the biggest change was subjected to further analysis to determine its functional roles and mechanisms with respect to macrophage activation. The result showed that a significant reduction in expression of one lncRNA, identified as lncGm16410, was observed in the lung of mice and RAW264.7 cells following exposure to PM2.5. lncGm16410 suppressed PM2.5-induced macrophage activation via the SRC protein-mediated PI3K/AKT signaling pathway. PM2.5 promoted lung inflammation by downregulating the expression of lncGm16410, enhancing the activation of macrophages. Thus, lncGm16410 might provide new insight into the prevention of PM2.5 injury.
Introduction
Pollution of the environment by fine particulate matter (PM2.5, aerodynamic diameter ≤ 2.5 μm) has become one of the major issues to threaten public health (Gao et al., 2019). PM2.5 contains mainly polycyclic aromatic hydrocarbons, transition metals, and endotoxin, all of which are known toxic chemicals (Deng et al., 2013; Ming et al., 2017). These particles can easily translocate from the alveoli to the bloodstream to affect the cardiovascular system, so long-term exposure to high concentrations of PM2.5 increases the risk of cardiovascular and respiratory illnesses (Huang et al., 2017; Kirrane et al., 2019). In recent years, much progress has been made in the prevention and control of PM2.5, but PM2.5 remains a potential risk factor (Xie and Li, 2019). Despite the plethora of studies that have been conducted to investigate the toxicity of PM2.5, the exact mechanism of PM2.5-induced injury is still difficult to ascertain.
Long non-coding RNAs (lncRNAs) are a set of non-protein coding RNAs with a minimum length of 200 bases. lncRNAs modulate biological processes that are crucial for the growth and differentiation of cells as well as for the development of tumors (Ren et al., 2018). Recently, several studies have found that lncRNAs participate in the metastasis of tumor cells by competitively regulating miRNAs (Lin C. et al., 2018). For example, lncRNA AFAP1-AS1 was found to be the antisense DNA strand of the AFAP1 encoding gene locus, which acts as an adaptor protein that regulates actin integrity and links members of the SRC family proteins and other signaling proteins to actin filaments (Wu et al., 2013). These findings support the hypothesis that lncRNAs play an important role in various biological processes in cells injured by PM2.5.
The main effects of PM2.5 exposure on cardiopulmonary diseases are inflammatory response and oxidative stress, probably due to the stimulation of macrophages by PM2.5 particles deposited in the alveoli and the release of some oxidants and cytokines. Several studies have demonstrated that the PI3K/AKT signaling pathway plays an important role in the activation of macrophage (Liu et al., 2017). Therefore, it has become important to investigate the role of lncRNAs in the process of cardiopulmonary disease caused by macrophage activation and the effect of lncRNAs on PM2.5-regulated macrophage inflammatory response.
Most previous studies on PM2.5 used tracheal instillation, an approach that is different from and that does not simulate the real situation of human exposure. In this study, a dynamic poisoning cabinet was used to construct a mice model, to determine changes in the expression of lncRNAs in mice in response to chronic PM2.5 exposure and establish the role of lncRNA in PM2.5-induced lung injury and inflammation. The findings suggested that lncRNA could reduce the extent of PM2.5-induced lung inflammation and suppress PM2.5-induced activation of macrophage.
Materials and Methods
Reagents
PrimeScriptTM RT reagent kit with gDNA Eraser (Perfect Real Time) (Code No.: RR047A)and SYBR® Premix Ex TaqTM II (Tli RNaseH Plus) (Code No.: RR820A) were purchased from TaKaRa. BCA protein assay kit (KGP902) and ECL detection kit were purchased from KeyGENE (Nanjing, China). Cell culture dishes were purchased from Guangzhou Jet Bio-Filtration Co., Ltd. (Guangzhou, China). Antibodies against β-actin (60008-1-lg, mouse), interleukin (IL)-6 (21865-1-AP, rabbit), tumor necrosis factor alpha (TNF-α; 60291-1-lg, mouse), protein kinase B (AKT; 10176-1-AP, rabbit), phosphoinositide 3-kinase (PI3K; 60225-1-lg, mouse), inducible nitric oxide synthase (iNOS; 18985-1-AP, rabbit), arginase-1 (ARG1; 16001-1-AP, rabbit), SRC (11097-1-AP, rabbit), and IL-10 (20850-1-AP, rabbit) were obtained from Proteintech (Wuhan, China). Antibodies against IL-1β (bs-20448R, rabbit), IL-12 (bs-1789R, rabbit), and P-AKT (bs-0876R, rabbit) were purchased from Bioss (Beijing, China). Antibody against P-PI3K (4228, rabbit) was purchased from CST. Dasatinib inhibitor was obtained from MCE (New Jersey, United States). lipofectamineTM 3000 Reagent was purchased from Thermo ScientificTM (United States). Mouse IL-6 ELISA kit was purchased from Boster (Wuhan, China).
Extraction and Composition of PM2.5
PM2.5 was collected from a large-capacity air sampler of Langfang (Hebei, China) from October 2015 to March 2016. The collection of PM2.5 was performed as described previously (Wang et al., 2017). Refer to Supplementary Material 1 for the component analysis of PM2.5.
Animal Experiments and Experiment Design
All animal experiments were approved by the Animal Experimental Committee of Dalian Medical University. Male Balb/c mice (6 weeks old) were obtained from Changsheng Biotechnology Co., Ltd. (Shenyang, China) (Approval No.: 2015-009). The mice were housed in a clean room (23 ± 1°C and humidity of 45–55%) provided with conventional food and water and maintained under a diurnal cycle of 12-h light and 12-h darkness. To construct the PM2.5 exposure model, 16 mice were divided into two groups with eight animals per group. The first group was kept as healthy control; the environment of the control group and the PM2.5 content in the environment were provided in Supplementary Material 2. The second group was exposed to PM2.5 (PM2.5 group). The PM2.5 exposure was performed using a dynamic poisoning cabinet (HOPE-MED8050, Tianjin Hepu technology co. Ltd) that simulated the atmospheric environment. According to the standard concentration limit of environmental PM2.5 and the ventilation of mice (The body weight in mice is about 30 g, the tidal volume is about 0.1–0.23 ml/kg, and the respiratory frequency is 136–230 times/min, the volume of poisoning warehouse is about 0.3 m3). The exposure concentration of 300 μg/m3 used in this experiment is about 225 μg/m3 of human living ambient air environment PM2.5 concentration. The concentration of PM2.5 in the flowing air was 300 μg/m3 for 4 months exposure, and the mice were exposed for 5 h/day for 5 days/week. At the end of the exposure period, blood was taken from the eyeball, and the lung tissue was removed and stored directly in liquid nitrogen for further experiments.
Cell Culture and CCK8 Assay
RAW264.7 and THP-1 cells were cultured in DMEM (Gibco, CA, United States) or 1640 (Gibco) supplemented with 10% fetal bovine serum (FBS), 100 U/ml penicillin, and 100 μg/ml streptomycin in humidified air at 37°C with 5% CO2. First, RAW264.7 cells were resuspended, then diluted to 5 × 104 cells/ml, and then 100 μl of the cell suspension was added to each well of a 96-well plate. These cells were either control cells or those that overexpressed lncGm16410, and they were treated with different concentrations of PM2.5 for a specific time, followed by the addition of 10 μl of Cell Counting Kit-8 (CCK8) reagent and incubation at 37°C and in the dark for 2 h. After, the OD value of each well was measured by absorbance at 450 nm. Cell survival rates were expressed relative to that of the control.
lncRNA Microarray Analysis
Microarray profiling was carried out by Shanghai Kangcheng Biotechnology Company. Briefly, the lung sample was labeled using Arraystar RNA Flash Labeling Kit, and a hybridization experiment was prepared using the Agilent SureHyb. After the chip was washed, it was scanned by an Agilent DNA Microarray Scanner, and the chip probe signals were acquired using the Agilent Feature Extraction software (version11.0.1.1). The Agilent GeneSpring GX v12.1 software was used to standardize the chip and to select lncRNAs that displayed differential expression.
Plasmid Construction and Transfection
The plasmid that overexpresses lncGm16410 was designed and synthesized by Gene Pharma (Suzhou, China). Refer to Supplementary Material 3 for details.
RNA Isolation and qPCR
The sequences of all primers used and details are listed in Supplementary Material 4.
Western Blotting
Proteins were extracted from the lung tissue as previously described (Zhang et al., 2018a). Refer to Supplementary Material 5 for details.
Enzyme-Linked Immunosorbent Assay (ELISA)
The expression of IL-6 in the serum was detected with an Enzyme-Linked Immunosorbent assay (ELISA) Kit (Boster, Wuhan, China). Blood samples were collected from the eyeballs of the animals and allowed to clot for 30 min at room temperature. The samples were then centrifuged at high speed, and the resulting serum from each sample was removed and stored it at −80°C.
Flow Cytometry
Cells undergoing apoptosis were counted by flow cytometry using the annexin V-FITC apoptosis kit. The cells were first washed several times with PBS and then resuspended in binding buffer. Annexin V-FITC (5 μl) and propidium iodide (5 μl) were successively added to the cell suspension followed by incubation in the dark and at room temperature. Apoptotic rates were calculated as percentages of cells in the early plus late stages of apoptosis.
Immunofluorescence and Immunohistochemistry
Refer to Supplementary Material 6 for details.
Statistical Analyses
Data were reported as means ± standard error of the means (SEM). Statistical analysis was performed using GraphPad Prism version 7. Student’s t-test was used to compare data between two groups whereas a one-way analysis of variance was used to compare data from more than two groups. Statistical significance was considered at the P < 0.05 level.
Results
Differential Expression of lncRNAs in the Lungs of Mice Exposed to PM2.5
To determine whether lncRNAs were involved in the development of lung injury caused by exposure to PM2.5, a DNA microarray was used to compare the expression profiles of lncRNA in the lungs of mice exposed to PM2.5 with those in the lungs of unexposed mice. Of these lncRNAs that were expressed in the lung of the control group, a total of 201 lncRNAs were upregulated while 106 lncRNAs were downregulated in the lung of the PM2.5 group (Figure 1A). Among these lncRNAs that showed different expression levels in the PM2.5 group, lncGm16410 was significantly downregulated (Supplementary Figure 1), and the genomic location of the lncGm16410 was shown (Figure 1B). As our previous study has shown, PM2.5 can induce macrophage exudation and lung tissue inflammation and injury (Wang et al., 2017). In the present study, the effect of lncGm16410 on these processes and the possible underlying mechanism was then determined.
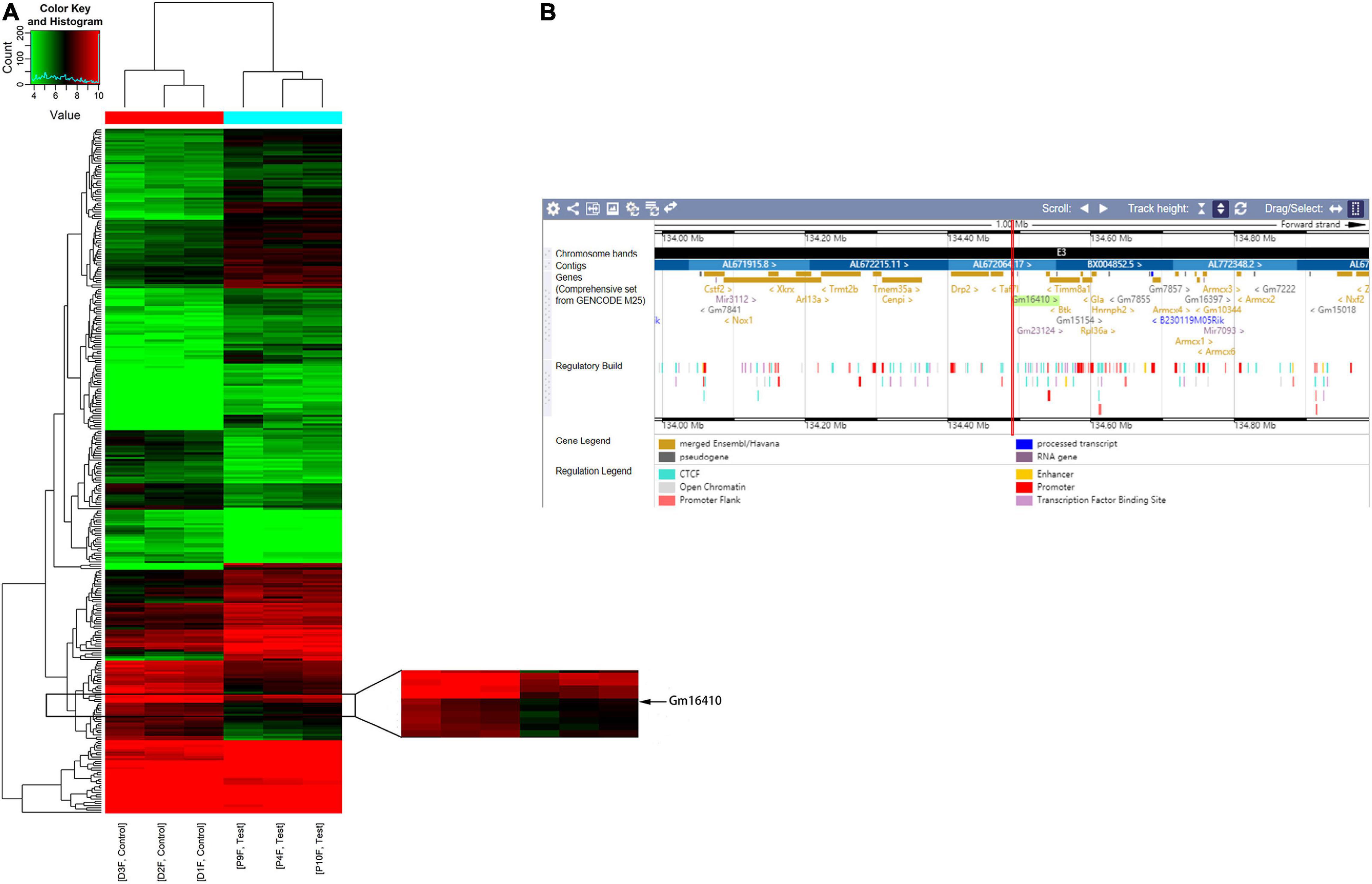
Figure 1. Changes in the expression of lncRNAs in the lung of mice following exposure to PM2.5. (A) Hierarchical clustering analysis of lncRNAs that were differentially expressed in lung tissues of control and PM2.5 exposed mice; each group contained three individuals. Red dots indicate above median expression level whereas green dots indicate below the median level across all samples (n = 3). (B) The genomic location of the lncGm16410 in the Ensembl database.
Expression of lncGm16410 Was Downregulated in PM2.5-Exposed Macrophages
Based on accumulating evidence, alveolar macrophages are the effector cells of lung disease (Ma et al., 2017). Therefore, RAW264.7 macrophages were used to investigate the injury caused by PM2.5 exposure, and the effect of PM2.5 on lncGm16410 expression was measured in RAW264.7 macrophages. Consistent with the lncGm16410 expression level in the lung tissue, the expression of lncGm16410 in RAW264.7 cells decreased 24 h after exposure to PM2.5 (Figure 2A). Simultaneously, the result showed that after 24 h of LPS treatment (5 μg/ml), the expression of lncGm16410 decreased significantly, which was similar to the results after PM2.5 exposure (Supplementary Figure 2). Overexpression of lncGm16410 in RAW264.7 cells was used to access whether lncGm16410 expression correlated with PM2.5-induced changes in macrophage activity. The level of lncGm16410 was significantly upregulated in RAW264.7 cells that overexpressed it (Figure 2B). In the control group, the survival rate of RAW264.7 cells was significantly reduced after exposure to PM2.5, but RAW264.7 cells that overexpressed lncGm16410 appeared to exhibit a better survival rate following exposure to PM2.5 (Figure 2C). Thus, overexpression of lncGm16410 appeared to protect the cells against apoptosis, thereby enhancing viability despite exposure to PM2.5 for 24 h (Figures 2D–F). Taken together, these results indicated that lncGm16410 was involved in the regulation of cell growth when the macrophages were exposed to PM2.5.
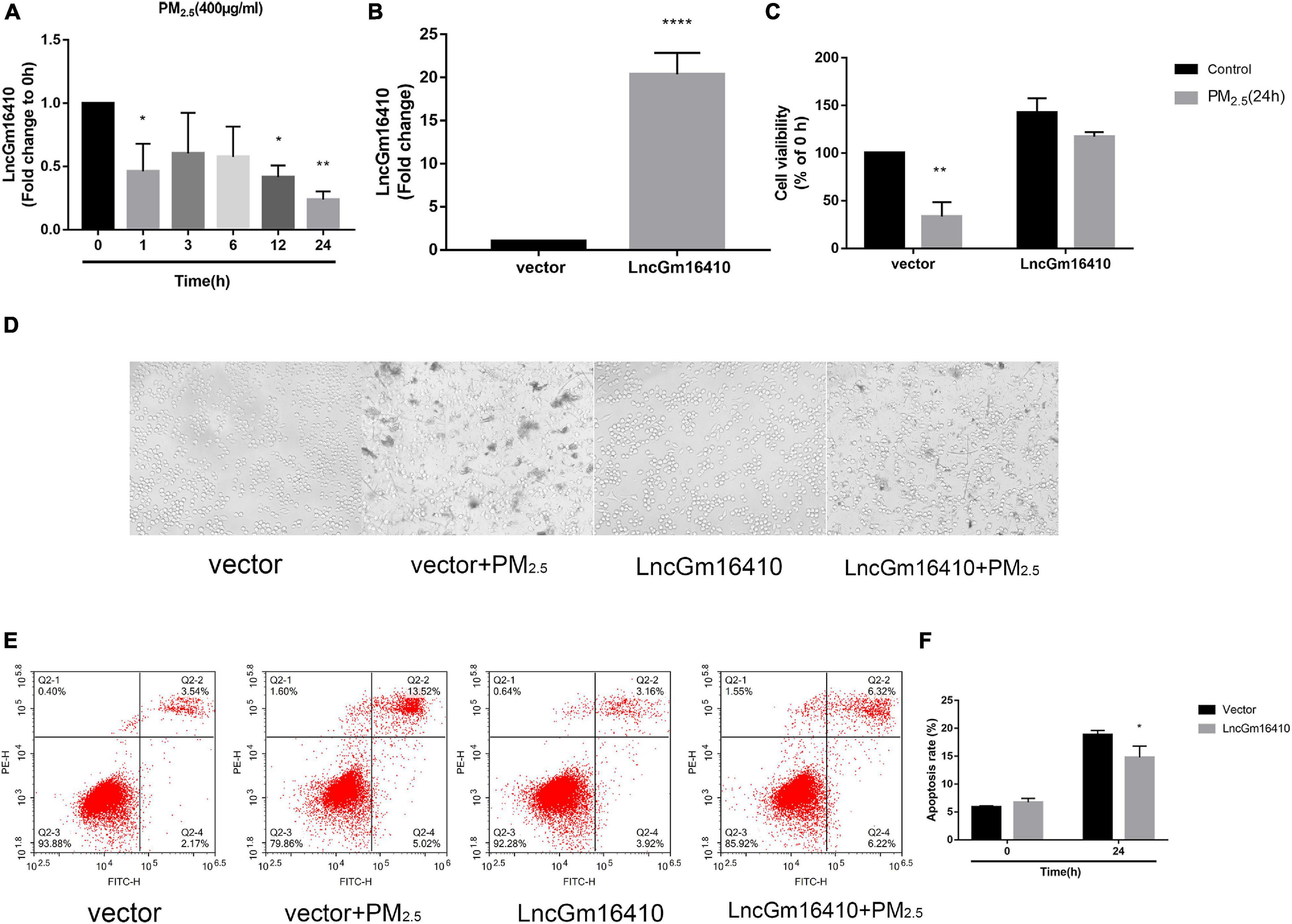
Figure 2. Effect of PM2.5 on the expression of lncGm16410 in RAW264.7 cells. Control RAW264.7 cells and those overexpressing lncGm16410 were subjected to apoptosis assay by different methods. (A) Real-time quantitative PCR analysis of lncGm16410 transcript levels in the cells after being exposed to PM2.5 for different times (n = 3). (B) Real-time quantitative PCR analysis of lncGm16410 that can optimize the transfection efficiency (n = 5). (C) CCK8 assays to determine the survival rate of macrophages exposed to PM2.5 after they were transfected with lncGm16410 (n = 3). (D) Microscopic analysis of the growth status of macrophages exposed to PM2.5. (E,F) Quantitative analysis of apoptosis (n = 3). Data are the means ± SEMs from at least three independent experiments, each performed in triplicate. *P < 0.5, **P < 0.01, and ****P < 0.0001—levels of significance that are different from the control group.
lncGm16410 Regulates Macrophage Activation Under PM2.5 Exposure
Recent studies have found that macrophages can be polarized into M1 or M2 subgroups upon activation by environmental stimulators, and the subsequent change in macrophage activity plays a significant role in the pathogenesis of pulmonary inflammation (Becerra-Diaz and Strickland, 2018; Li et al., 2018). First, the CCK8 method was used to determine the effect of PM2.5 on the growth of RAW264.7 and THP-1 cells, and the median lethal dose was used to study the impact of PM2.5 on macrophage activity. The median lethal doses of PM2.5 for RAW264.7 and THP-1 cells were experimentally determined to be 400 and 80 μg/ml, respectively (Figure 3A). After 12 h of PM2.5 exposure, the macrophage M1 marker protein was slightly upregulated, peaking after 24 h, while the macrophage M2 marker protein was upregulated after 48 h of exposure (Figures 3B,C). However, after 24 h of exposure, the macrophage M1 and M2 marker proteins in THP-1 macrophages were significantly upregulated (Figures 3D,E). Unsurprisingly, the results of qRT-PCR also demonstrated a significant increase in M1- and M2-related markers following PM2.5 exposure (Figure 3F). Whether lncGm16410 might affect the PM2.5-induced upregulated levels of M1 and M2 was then investigated. To test this, control RAW264.7 and those that overexpressed lncGm16410 were exposed to PM2.5, and the changes in macrophage M1 and M2 levels were measured. After 24 h of exposure to PM2.5, the mRNA and protein levels of M1 and M2 in the cells that overexpressed lncGm16410 were reduced (Figures 3G–I). Compared with the control cells, the increased expression of M1 and M2 proteins in the PM2.5-treated cells was also detected by an immunofluorescence assay. Immunofluorescence assay also demonstrated that overexpression of lncGm16410 reduced the levels of M1 and M2 proteins in the cytoplasm (Figure 3J). These results indicated that lncGm16410 affected the phenotypic transformation of macrophages and confirmed that lncGm16410 could participate in the process of PM2.5-induced macrophage activation.
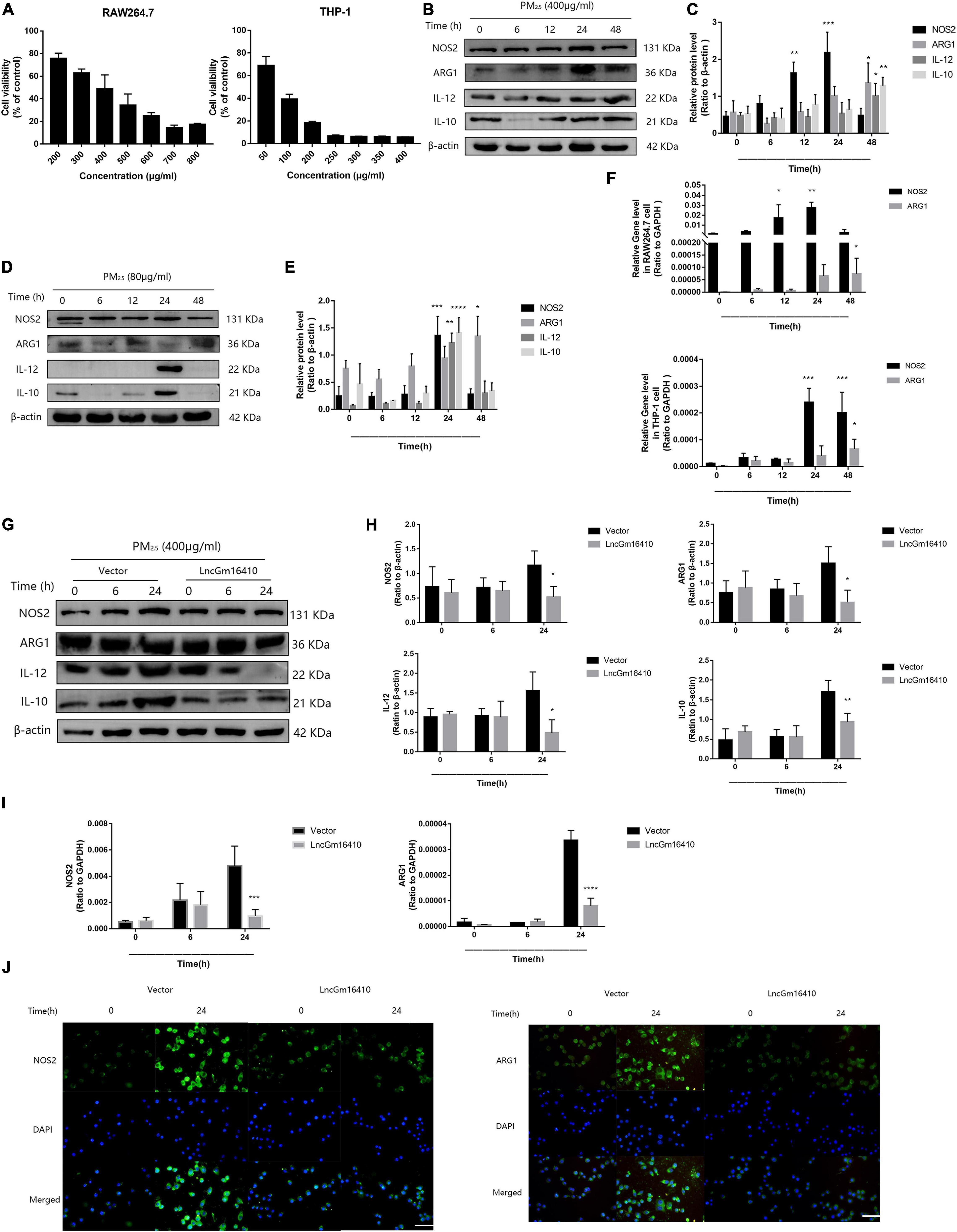
Figure 3. Effect of lncGm16410 overexpression on the activation of RAW264.7 cells following exposure to PM2.5. (A) Survival rates of RAW264.7 (n = 6) and THP-1 (n = 4) cells after exposure to different concentrations of PM2.5. (B,C) Western blot analysis of the M1 marker NOS2 and IL-12 and the M2 marker ARG1 and IL-10 in RAW264.7 cells. The plot gives a quantitative gray-scale analysis of the blot (n = 3). (D,E) Western blot analysis of the M1 marker NOS2 and IL-12 and the M2 marker ARG1 and IL-10 in THP-1 cells. The plot gives a quantitative gray-scale analysis of the blot (n = 3). (F) Real-time quantitative PCR analysis of NOS2 and ARG1 in RAW264.7 and THP-1 cells (n = 4). (G,H) Western blot analysis of the M1 marker NOS2 and IL-12 and the M2 marker ARG1 and IL-10 in RAW264.7 cells that overexpressed lncGm16410. The plot on the right gives a quantitative gray-scale analysis of the blot (n = 3). (I) Real-time quantitative PCR analysis of NOS2 and ARG1 in RAW264.7 cells after overexpression of lncGm16410 (n = 3). (J) Representative images of immunocytochemical staining analysis of the expression levels of NOS2 and ARG1 in RAW264.7 cells that overexpressed lncGm16410. Scale bar = 20 μm. All graphical data are the means ± SEMs from at least three independent experiments, each performed in triplicate. *P < 0.5, **P < 0.01, ***P < 0.001, and ****P < 0.0001—levels of significance that are different from the control group.
lncGm16410 Suppresses the Regulation of SRC Expression Under PM2.5 Exposure
According to the lncRNA microarray analysis, we found that, of the several genes adjacent to the lncGm16410, such as Taf7l, Timm8a1, and Btk, only Btk was slightly upregulated. The result was not statistically significant, which indicates that lncGm16410 may not act as a cis-acting gene. Recent studies have shown that exposure to particulate pollutants in the environment can lead to the activation of SRC protein-mediated signaling pathways, which in turn might trigger autophagy and inflammatory responses (Xu et al., 2016, Xu et al., 2019). To further investigate the changes in SRC protein expression in response to PM2.5, the expression levels of SRC in RAW264.7 and THP-1 cells were measured following exposure to PM2.5. Compared with the control cells, the levels of SRC protein in the PM2.5-exposed cells displayed a rapid and sustained increased level of SRC after PM2.5 treatment (Figures 4A–D), suggesting that PM2.5 could indeed increase the expression of SRC. The effect of lncGm16410 on SRC expression in PM2.5-exposed cells was then determined. The expression of SRC was attenuated in the cells that overexpressed lncGm16410 (Figures 4E,F). Changes in the expression of SRC were also confirmed by immunostaining in the cytoplasm of cells that overexpressed lncGm16410 (Figure 4G). lncGm16410 was involved in the regulation of PM2.5-induced changes in SRC expression.
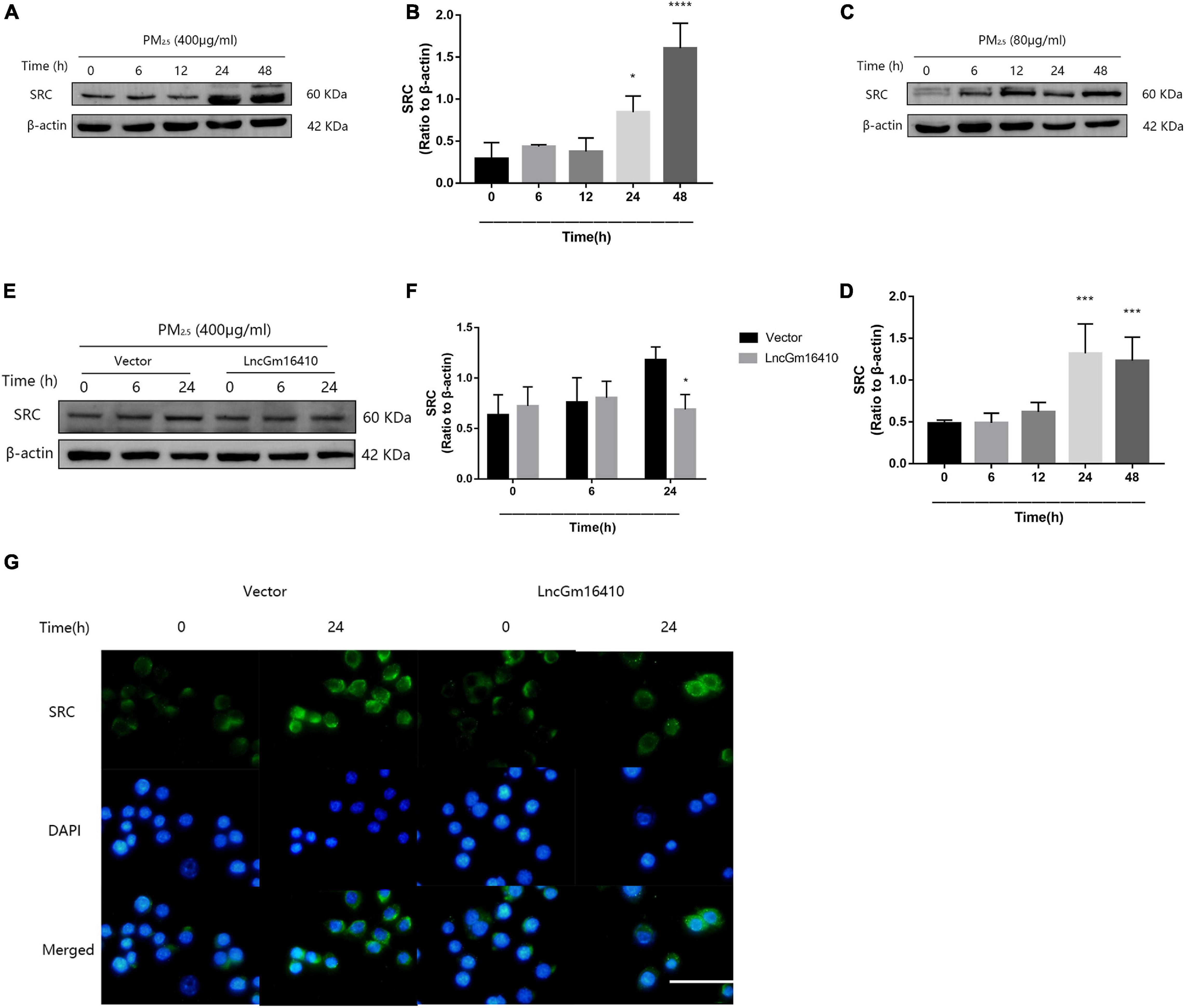
Figure 4. The expression of SRC was suppressed by lncGm16410 under PM2.5 exposure. (A,B) Western blot analysis of SRC expression in RAW264.7 cells. The plot gives a quantitative gray-scale analysis of the blot (n = 3). (C,D) Western blot analysis of SRC expression in THP-1 cells. The plot gives a quantitative gray-scale analysis of the blot (n = 3). (E,F) Western blot analysis of SRC expression in RAW264.7 cells that overexpressed lncGm16410. The plot gives a quantitative gray-scale analysis of the blot (n = 3). (G) Representative images of the immunocytochemical staining analysis of SRC level in RAW264.7 cells that overexpressed lncGm16410. Scale bar = 20 μm. All graphical data are the means ± SEMs from at least three independent experiments, each performed in triplicate. *P < 0.5, ***P < 0.001, and ****P < 0.0001—levels of significance that are different from the control group.
SRC-Mediated Macrophage Activation via Activating PI3K/AKT Signaling
Although lncGm16410 suppressed SRC expression under PM2.5 exposure, it is unclear whether SRC was involved in PM2.5-mediated macrophage activation. Dasatinib is an inhibitor of SRC protein (Hermida-Prado et al., 2019). To determine whether SRC might be involved in PM2.5-mediated macrophage activation, the cells were treated with dasatinib before they were exposed to PM2.5 (Figures 5A,B), and the changes in macrophage polarity markers were measured. Compared with control cells, cells that were treated with dasatinib exhibited reduced levels of M1 and M2 expression as revealed by western blot (Figures 5C–F). The inhibitory effect of dasatinib on the expression of NOS2 and ARG1 was also confirmed by immunostaining (Figure 5G). The result indicated that SRC was involved in PM2.5-induced macrophage activation. As an important molecule in the signal transduction pathway, SRC can shuttle between different cellular compartments to affect cellular processes by mediating the PI3K/AKT signaling pathway (Cheng et al., 2014; Cho et al., 2016). Therefore, to further study the mechanism by which SRC regulates macrophage activity upon exposure to PM2.5, the expression levels of P-PI3K, P-AKT, and nuclear factor kappa B (NF-κB), proteins that are involved in the PI3K/AKT signaling pathway, were measured by western blot. Macrophages that were exposed to PM2.5 displayed reduced expression of P-PI3K, P-AKT, and NF-κB when the cells were treated with dasatinib (Figures 5H–K), suggesting that SRC might be involved in the activation of the PI3K/AKT signaling pathway through regulating the phosphorylation of PI3K and AKT.
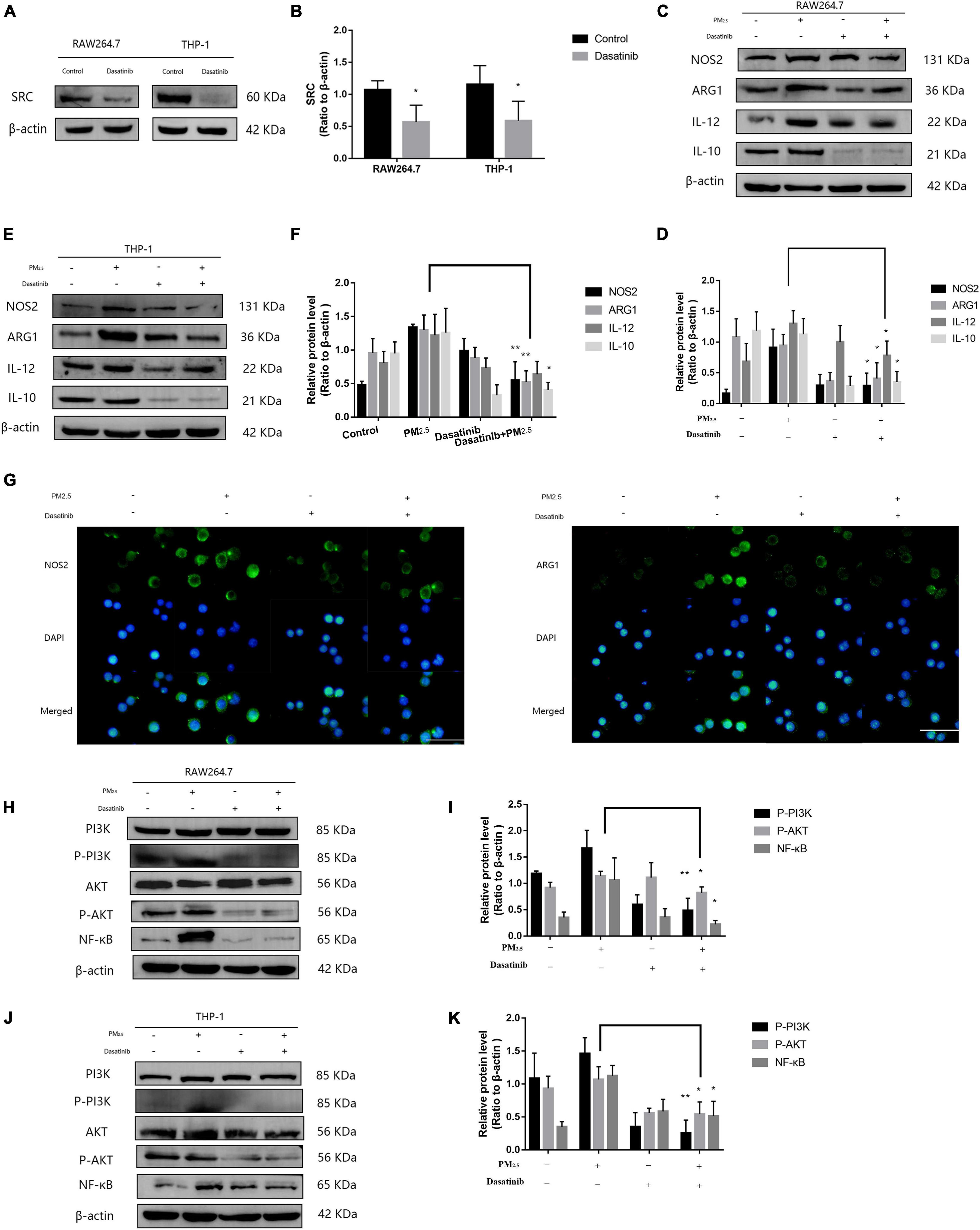
Figure 5. SRC-mediated macrophage activation via activating PI3K/AKT signaling. (A,B) Western blot analysis of SRC expression in RAW264.7 and THP-1 cells. The plot gives a quantitative gray-scale analysis of the blot (n = 4). (C,D) Western blot analysis of the M1 marker NOS2 and IL-12 and the M2 marker ARG1 and IL-10 in RAW264.7 cells. The plot gives a quantitative gray-scale analysis of the blot (n = 3). (E,F) Western blot analysis of the M1 marker NOS2 and IL-12 and the M2 marker ARG1 and IL-10 in THP-1 cells. The plot gives a quantitative gray-scale analysis of the blot (n = 3). (G) Representative images of immunocytochemical staining analysis of NOS2 and ARG1 levels in RAW264.7 cells. Scale bar = 20 μm. (H,I) Western blot analysis of P-PI3K, P-AKT, and NF-κB in RAW264.7 cells. The plot gives a quantitative gray-scale analysis of the blot (n = 3). (J,K) Western blot analysis of P-PI3K, P-AKT, and NF-κB levels in THP-1 cells. The plot gives a quantitative gray-scale analysis of the blot (n = 3). All graphical data are the means ± SEMs from at least three independent experiments, each performed in triplicate. *P < 0.5 and **P < 0.01—levels of significance that are different from the control group.
lncGm16410 Alleviated Inflammation Caused by Macrophage Activation Under PM2.5 Exposure
According to accumulated evidence, the main consequence of macrophage activation is the secretion of related inflammatory factors (He et al., 2017; Zhang et al., 2018b). Therefore, changes in the levels of IL-6, TNF-α, and IL-1β of macrophages under PM2.5 exposure were measured. Compared with the control cells, the expression of inflammatory factors in the PM2.5-exposed cells was upregulated (Figures 6A–F). PM2.5 exposure led to a slight decrease in the levels of related inflammatory cytokines in RAW264.7 cells that overexpressed lncGm16410 (Figure 6A). In addition, RAW264.7 cells that overexpressed lncGm16410 treated with PM2.5 also displayed reduced levels of IL-6 and IL-1β (Figures 6C,D). These results indicated that overexpression of lncGm16410 may reduce inflammation by regulating macrophage activity upon exposure to PM2.5.
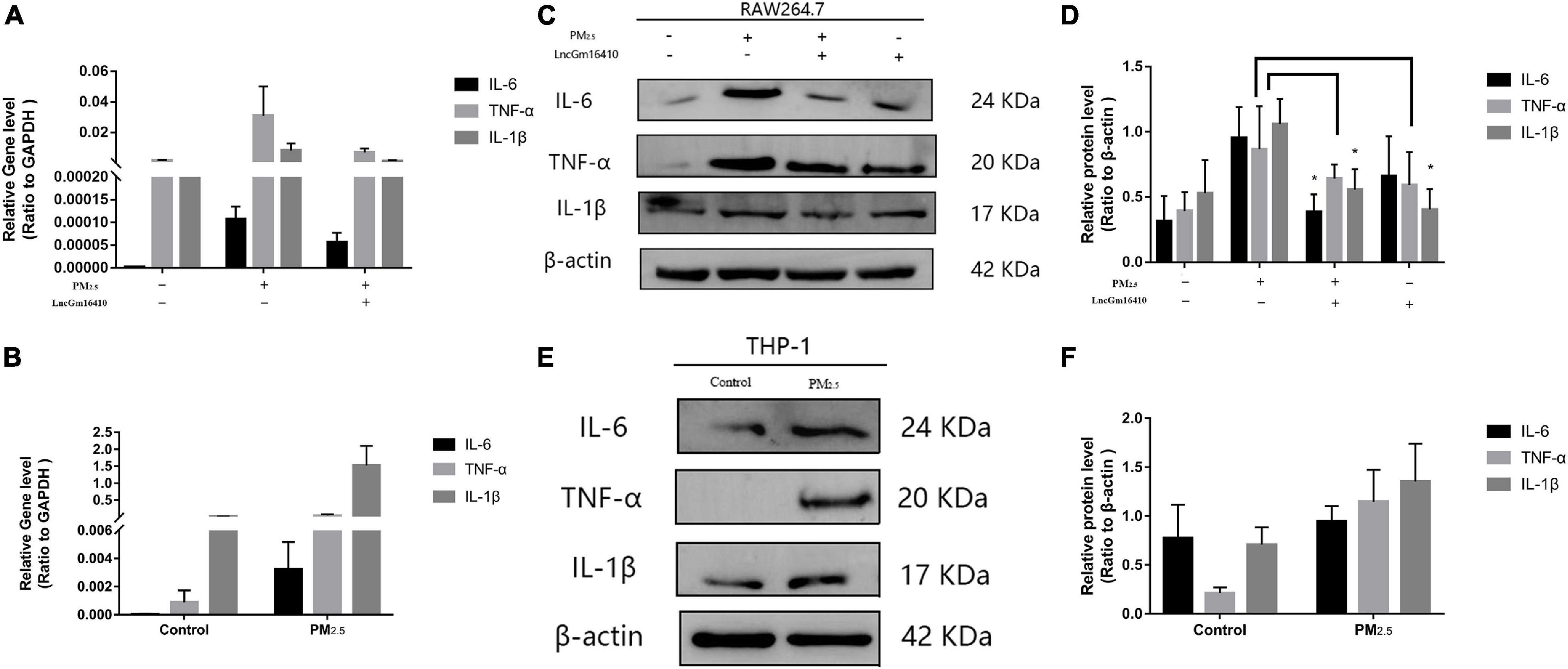
Figure 6. lncGm16410 regulate the inflammation response of macrophage induced by PM2.5 exposure. (A) Real-time quantitative PCR analysis of IL-6, TNF-α, and IL-1β in RAW264.7 cells (n = 3). (B) Real-time quantitative PCR analysis of IL-6, TNF-α, and IL-1β expression in THP-1 cells (n = 3). (C,D) Western blot analysis of IL-6, TNF-α, and IL-1β transcripts in RAW264.7 cells. The plot gives a quantitative gray-scale analysis of the blot (n = 3). (E,F) Western blot analysis of IL-6, TNF-α, and IL-1β in THP-1 cells. The plot gives a quantitative gray-scale analysis of the blot (n = 3). All graphical data are the means ± SEMs from at least three independent experiments, each performed in triplicate. *P < 0.5 level of significance that is different from the control group.
Macrophage Activation Caused Inflammation of Lung Tissue in Response to PM2.5
Numerous studies have shown that PM2.5 could induce lung tissue injury by activating macrophages (Feng et al., 2019). To determine the activation of macrophages in lung tissue under PM2.5 exposure, immunofluorescence was applied to detect the macrophage marker F4/80 in the lung. Compared with the control mice, a large number of activated macrophages in the lung of mice exposed to PM2.5 were observed (Figure 7A). In addition, for mice that were exposed to PM2.5, histopathological examination of the lung tissue by H&E staining revealed a slight destruction of the alveolar wall and infiltration of inflammatory cells, including macrophages and lymphocytes, with red blood cells scattered in the alveolar areas (Figure 7B). To further characterize the inflammatory response in vivo, the levels of proteins and genes associated with inflammatory cytokines in the lung tissue were measured. Compared with the control group, the levels of TNF-α and IL-1β were significantly increased in response to PM2.5 exposure (Figures 7C,D). Moreover, exposure to PM2.5 also led to a significant increase of IL-6 levels in the serum (Figure 7E). The levels of TNF-α, IL-1β, and IL-6 were subsequently measured by qRT-PCR, which revealed obvious increases as a result of PM2.5 exposure (Figure 7F). Finally, the link between the activation of the PI3K/AKT signaling pathway and PM2.5-induced injury in the lung was investigated. The levels of PI3K, AKT, and NF-κB and their phosphorylation were upregulated in response to PM2.5 exposure (Figures 7G,H). This finding was confirmed by immunohistochemical assay of P-AKT and NF-κB proteins. A large amount of brown-yellow fine particles was deposited in the section of the lung exposed to PM2.5 (Figure 7I). Taken together, the results showed that macrophage activation in response to PM2.5 exposure could cause lung tissue injury.
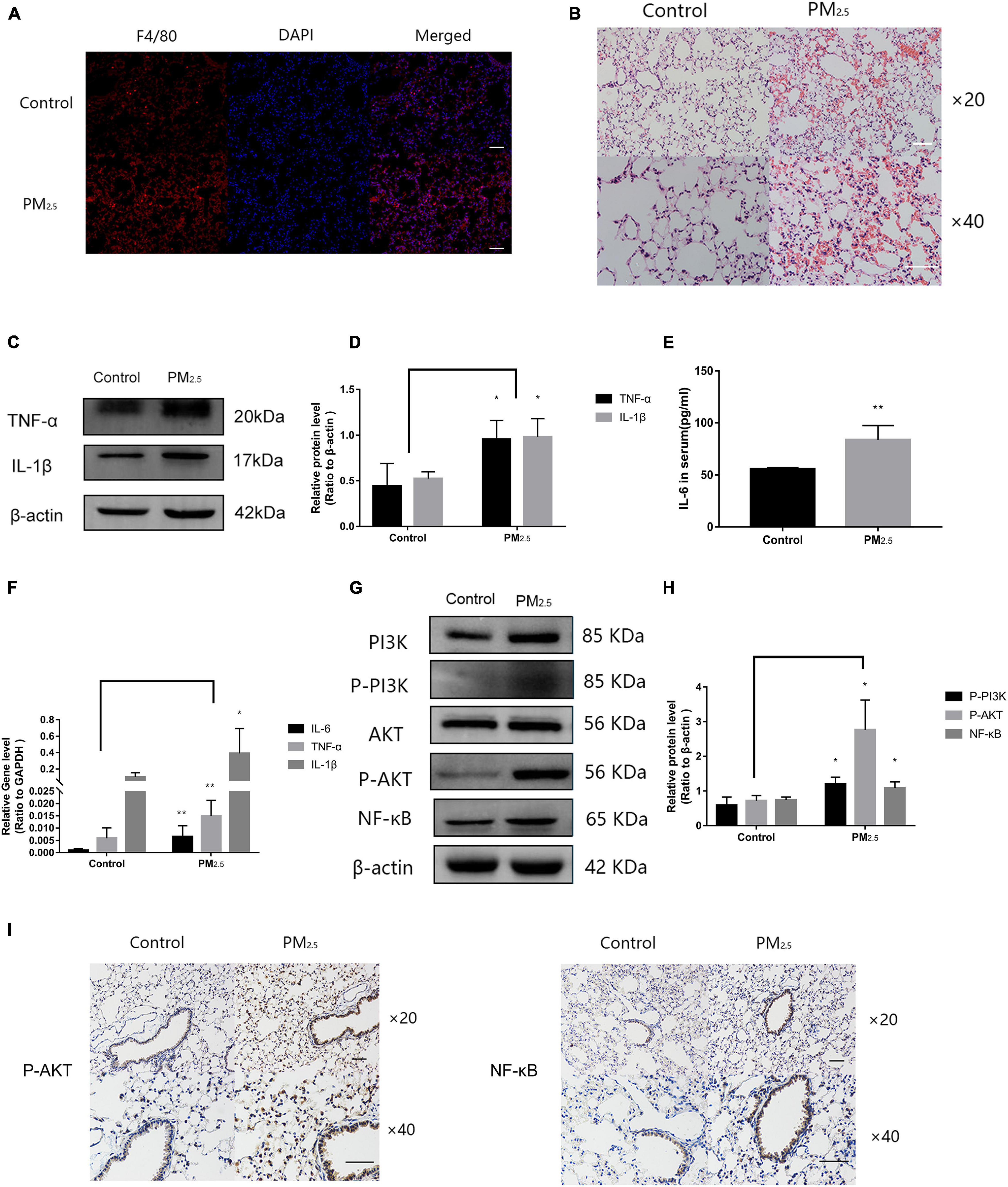
Figure 7. Macrophage activation caused inflammation of lung tissue in response to PM2.5. (A) Immunohistochemistry detection of the macrophage marker F4/80 in mouse lung tissues. Scale bar = 10 μm. (B) Pathological changes in the lung tissues. Scale bar = 10 μm. (C,D) Western blot analysis of TNF-α and IL-1β levels in the lung tissues. The plot gives a quantitative gray-scale analysis of the blot (n = 3). (E) ELISA analysis of IL-6 in serum (n = 5). (F) Real-time quantitative PCR analysis of IL-6, TNF-α, and IL-1β in the lung (n = 8). (G,H) Western blot analysis of the P-PI3K, P-AKT, and NF-κB in mouse lung tissues. The plot gives a quantitative gray-scale analysis of the blot (n = 3). (I) Immunohistochemistry detection of P-AKT and NF-κB in mouse lung tissues. Scale bar = 10 μm. Data are the means ± SEMs from at least three independent experiments, each performed in triplicate. *P < 0.5 and **P < 0.01—levels of significance that are different from the control group at the levels, respectively.
Discussion
In recent years, PM2.5, a major environmental pollution component, has attracted much attention because of its direct impact on health. Exposure to PM2.5 can result in a variety of lung diseases and even brain injuries (Huang et al., 2019; Tian et al., 2019). In this study, we collected the PM2.5 sample from the air in Hebei Province, the most polluted city in China, and analyzed its composition. The heavy metals and aromatic hydrocarbons present in PM2.5 are considered to be the main components of PM2.5 toxicity. Next, we used a dynamic poison cabinet to simulate the state of PM2.5 in the environment and used Balb/c mice to construct a PM2.5 exposure model in vivo.
According to related research, the microvesicles released in vitro from LPS-primed alveolar macrophages carry a large amount of TNF-α and a small amount of IL-1β/IL-6, and alveolar macrophages interact with lung epithelial cells through these microvesicles (Soni et al., 2016a; Allard et al., 2018). When a PM2.5 suspension is instilled into the trachea of mice, the microvesicles released by macrophages can induce an increase in the number of BALF neutrophils as well as an increase in the expression of related inflammatory proteins (Soni et al., 2016b). Many studies have been carried out to find a way to prevent PM2.5-induced injuries in macrophages. However, the mechanism and process of these injuries are still unclear.
lncRNAs are widely expressed as non-coding RNA, and increasing evidence suggests that lncRNAs play a major role in human physiological and pathophysiological processes (Chen et al., 2018). lncRNA microarray analysis of lung tissue samples taken from PM2.5-treated Balb/c mice revealed 201 upregulated and 106 downregulated lncRNAs in response to PM2.5 exposure (Figure 1A). The same profile of lncRNA expression was observed in RAW264.7 macrophages following exposure to PM2.5 (Figure 2A). This indicated that lncGm16410 may be involved in PM2.5-induced injuries, both in the lung and in the macrophage. Indeed, the data obtained from the macrophage CCK8 and apoptosis experiments in vitro (Figure 2C), as well as microscopic analysis of the lung tissue, suggested that lncGm16410 might be involved in the activation of macrophages in response to the PM2.5-induced injuries sustained by the lung.
Notably, we observed that the downregulation of lncGm16410 expression in macrophages was consistent with lncGm16410 expression in the lung. Our data suggested that the initiation of inflammation induced by PM2.5 recruited monocytes to the lungs where they then differentiated into macrophages, ultimately initiating an inflammatory cascade. According to our data, the recruitment of a large number of macrophages to the lung not only increased the expression of lncGm16410 in the lung, but the PM2.5-activated alveolar macrophages also caused rapid and sustained inflammation as well as the production of cytokines and chemokines. These cytokines and chemokines released by the macrophages then diffused into the lung, causing tissue damage.
Under the stimulation of environmental factors, macrophages will carry out two different forms of inflammatory and anti-inflammatory functions (Saradna et al., 2018). M1 macrophages participate in the classic activation pathway and mainly produce immune-stimulating cytokines and other inflammatory response effectors (Sica et al., 2015). M2 macrophages are mainly involved in the upregulation of ARG1 (Nandakumar et al., 2017; Tardito et al., 2019). They have the immunomodulatory capacity, and they also play a major role in tissue remodeling, tumor progression, and mediating the regression of inflammation (Sica and Mantovani, 2012). In the present study, macrophage M1 phenotype-associated proteins NOS2 and IL-12 were found to be abundantly expressed after 24 h of exposure to PM2.5, suggesting that PM2.5 might stimulate the macrophage to produce an inflammatory response. PM2.5 also induced a slow but late increase in the levels of macrophage M2 phenotype-associated proteins ARG1 and IL-10, demonstrating that macrophages were fully activated upon PM2.5 exposure (Figure 3B) and that both M1 and M2 macrophages were involved in macrophage injury induced by PM2.5. This study mainly focused on the role of lncGm16410 in macrophage activation and showed that lncGm16410 prevented the changes in M1/M2 phenotypes and a decrease in cell viability induced by PM2.5, indicating a preventative effect of lncGm16410 on macrophage activation. Furthermore, lncGm16410 was found to be involved in macrophage-mediated inflammation responses, suggesting a key role for lncGm16410 in pneumonia (Figures 6A,C).
A recent study on lncRNAs outlines the important roles of these RNAs in the regulation of SRC expression (Zhang S. et al., 2018). In the current study, the expression of SRC in macrophages was upregulated in response to PM2.5 exposure. However, overexpression of lncGm16410 counteracted this effect (Figure 4E). One possible explanation for this could be that lncGm16410 downregulated the expression of SRC in macrophages. After exposure to PM2.5, inhibition of SRC in macrophages resulted in different degrees of reduction in the expression of macrophage phenotypic proteins (Figures 5C,E). This suggested that SRC could regulate PM2.5-induced macrophage activity. Taken together, these results verified that lncGm16410 affects macrophage activation by downregulating the expression of SRC in response to PM2.5 exposure. SRC was recently found to be involved in PI3K/AKT signaling pathway (Lu et al., 2003; Riggins et al., 2003; Yang et al., 2017; Beadnell et al., 2018). Our data showed that activation of the PI3K/AKT signaling pathway was also inhibited when the activity of SRC was reduced by the inhibitor dasatinib (Figures 5H,J). Although further experiments are needed to clarify the detailed mechanism, the current result does suggest a connection between SRC and PI3K/AKT signaling pathway.
Based on a previous study from our laboratory, acute exposure to PM2.5 can cause lung tissue inflammation and oxidative stress (Wang et al., 2017). However, mice exposed to PM2.5 exhibited only a small amount of red blood cells and exudates in the alveoli in their lung tissue (Figure 7B). One possible explanation for this phenomenon could be that chronic exposure to PM2.5 simulated in this experiment was not a traditional tracheal instillation method but was carried out in the form of dynamic exposure. Although this exposure method did not cause any obvious pathological changes in the lung tissue exposed to PM2.5, it still resulted in some changes to the levels of inflammation-associated proteins and genes in the lung. According to the results of this study, we found that PM2.5 exposure can induce the expression of inflammation-related factors in the murine- and human-derived macrophages (RAW264.7 cells and THP-1 cells) and mouse lung tissue, which may cause lung tissue injury and then lead to lung inflammation in mice. As this study adopted a dynamic PM2.5 exposure method, mice were exposed to PM2.5 through inhalation for 4 months, which can better simulate the long-term exposure of humans to PM2.5 in the environment. Simultaneously, by comparing relevant epidemiological studies (Zhao et al., 2017; Lin H. et al., 2018; Qi et al., 2020) this study found that PM2.5 exposure in the environment increases the risk of lung disease and affects the quality of life and life expectancy.
In in vitro studies, the macrophages were transfected with a plasmid that overexpressed lncGm16410. The results were consistent with the decrease in inflammatory cytokines observed in the lncGm16410-overexpressing cells (Figures 6A,C). The reduced mRNA and protein levels of inflammatory factors such as TNF-α shown by lncGm16410-overexpressing cells indicated that the lncGm16410 may suppress the level of inflammatory responses in the lung by reducing the expression of TNF-α in macrophages (Figure 7C). These results firmly demonstrated the involvement of lncGm16410 in macrophage activation and macrophage inflammation via PI3K/AKT.
Our analyses of PM2.5-exposed mice suggested that a decrease in lncGm16410 expression coincides with macrophage apoptosis and activation (Figure 8). Thus, both in vivo and in vitro results supported the speculation that lncGm16410 may serve as a potential marker of pneumonia caused by PM2.5 and may be considered a new therapeutic strategy for lung inflammation.
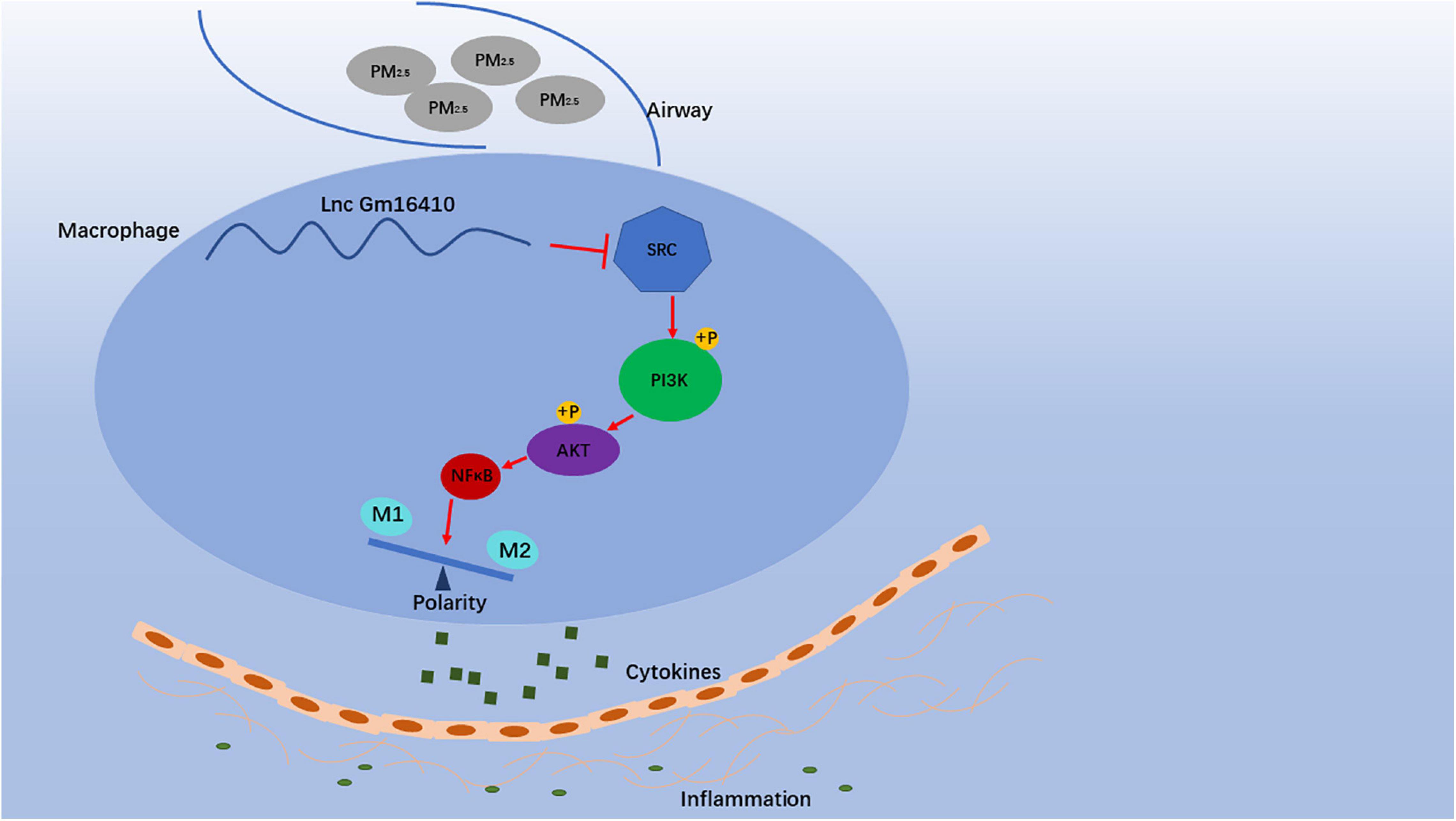
Figure 8. Schematic diagram showing the mechanisms through which lncGm16410 mediates PM2.5-induced macrophages activation. When macrophage is exposed to PM2.5, lncGm16410 suppresses the regulation of SRC expression, leading to a subsequent increase in the latter. The interactions among lncGm16410, SRC, and PI3K/AKT will dictate the balance of M1/M2 macrophages. Macrophage apoptosis and activation result in the overproduction of inflammatory factors and their subsequent release into the lung. This process, in turn, causes inflammation in the lung.
Data Availability Statement
The raw data supporting the conclusions of this article will be made available by the authors, without undue reservation.
Ethics Statement
The animal study was reviewed and approved by all animal experiments were approved by the Animal Experimental Committee of Dalian Medical University.
Author Contributions
JX and HX were responsible for the overall organizing of the experiments. JX, HX, KM, YW, and BN performed the experiments. JX wrote the manuscript. FL and LZ designed the experiments. All the authors read and approved the final manuscript.
Funding
Financial support was provided by the National Natural Science Foundation of China (Grant No. 51372029).
Conflict of Interest
The authors declare that the research was conducted in the absence of any commercial or financial relationships that could be construed as a potential conflict of interest.
Supplementary Material
The Supplementary Material for this article can be found online at: https://www.frontiersin.org/articles/10.3389/fcell.2021.618045/full#supplementary-material
References
Allard, B., Panariti, A., and Martin, J. G. (2018). Alveolar macrophages in the resolution of inflammation, tissue repair, and tolerance to infection. Front. Immunol. 9:1777. doi: 10.3389/fimmu.2018.01777
Beadnell, T. C., Nassar, K. W., Rose, M. M., Clark, E. G., Danysh, B. P., Hofmann, M. C., et al. (2018). Src-mediated regulation of the PI3K pathway in advanced papillary and anaplastic thyroid cancer. Oncogenesis 7:23. doi: 10.1038/s41389-017-0015-5
Becerra-Diaz, M., and Strickland, A. B. (2018). Androgen and androgen receptor as enhancers of M2 macrophage polarization in allergic lung inflammation. J. Immunol. 201, 2923–2933. doi: 10.4049/jimmunol.1800352
Chen, J., Huang, X., Wang, W., Xie, H., Li, J., Hu, Z., et al. (2018). LncRNA CDKN2BAS predicts poor prognosis in patients with hepatocellular carcinoma and promotes metastasis via the miR-153-5p/ARHGAP18 signaling axis. Aging (Albany NY) 10, 3371–3381. doi: 10.18632/aging.101645
Cheng, S. E., Lee, I. T., Lin, C. C., Hsiao, L. D., and Yang, C. M. (2014). Thrombin induces ICAM-1 expression in human lung epithelial cells via c-Src/PDGFR/PI3K/Akt-dependent NF-kappaB/p300 activation. Clin. Sci. (Lond.) 127, 171–183. doi: 10.1042/cs20130676
Cho, R. L., Yang, C. C., Lee, I. T., Lin, C. C., Chi, P. L., Hsiao, L. D., et al. (2016). Lipopolysaccharide induces ICAM-1 expression via a c-Src/NADPH oxidase/ROS-dependent NF-kappaB pathway in human pulmonary alveolar epithelial cells. Am. J. Physiol. Lung Cell. Mol. Physiol. 310, L639–L657. doi: 10.1152/ajplung.00109.2014
Deng, X., Zhang, F., Rui, W., Long, F., Wang, L., Feng, Z., et al. (2013). PM2.5-induced oxidative stress triggers autophagy in human lung epithelial A549 cells. Toxicol. In Vitro 27, 1762–1770. doi: 10.1016/j.tiv.2013.05.004
Feng, S., Duan, E., Shi, X., Zhang, H., Li, H., Zhao, Y., et al. (2019). Hydrogen ameliorates lung injury in a rat model of subacute exposure to concentrated ambient PM2.5 via Aryl hydrocarbon receptor. Int. Immunopharmacol. 77:105939. doi: 10.1016/j.intimp.2019.105939
Gao, J., Yuan, J., Wang, Q., Lei, T., Shen, X., Cui, B., et al. (2019). Metformin protects against PM2.5-induced lung injury and cardiac dysfunction independent of AMP-activated protein kinase alpha2. Redox Biol. 28:101345. doi: 10.1016/j.redox.2019.101345
He, M., Ichinose, T., Yoshida, S., Ito, T., He, C., Yoshida, Y., et al. (2017). PM2.5-induced lung inflammation in mice: differences of inflammatory response in macrophages and type II alveolar cells. J. Appl. Toxicol. 37, 1203–1218. doi: 10.1002/jat.3482
Hermida-Prado, F., Villaronga, M. A., Granda-Diaz, R., Del-Rio-Ibisate, N., Santos, L., Hermosilla, M. A., et al. (2019). The SRC inhibitor dasatinib induces stem cell-like properties in head and neck cancer cells that are effectively counteracted by the mithralog EC-8042. J. Clin. Med. 8:1157. doi: 10.3390/jcm8081157
Huang, K., Liang, F., Yang, X., Liu, F., Li, J., Xiao, Q., et al. (2019). Long term exposure to ambient fine particulate matter and incidence of stroke: prospective cohort study from the China-PAR project. BMJ 367:l6720. doi: 10.1136/bmj.l6720
Huang, Q., Chi, Y., Deng, J., Liu, Y., Lu, Y., Chen, J., et al. (2017). Fine particulate matter 2.5 exerted its toxicological effect by regulating a new layer, long non-coding RNA. Sci. Rep. 7:9392. doi: 10.1038/s41598-017-09818-6
Kirrane, E. F., Luben, T. J., Benson, A., Owens, E. O., Sacks, J. D., Dutton, S. J., et al. (2019). A systematic review of cardiovascular responses associated with ambient black carbon and fine particulate matter. Environ. Int. 127, 305–316. doi: 10.1016/j.envint.2019.02.027
Li, X., Lei, Y., Wu, M., and Li, N. (2018). Regulation of macrophage activation and polarization by HCC-derived exosomal lncRNA TUC339. Int. J. Mol. Sci. 19:2958. doi: 10.3390/ijms19102958
Lin, C., Zhang, S., Wang, Y., Wang, Y., Nice, E., Guo, C., et al. (2018). Functional role of a novel long noncoding RNA TTN-AS1 in esophageal squamous cell carcinoma progression and metastasis. Clin. Cancer Res. 24, 486–498. doi: 10.1158/1078-0432.ccr-17-1851
Lin, H., Qian, Z., Guo, Y., Zheng, Y., Ai, S., Hang, J., et al. (2018). The attributable risk of chronic obstructive pulmonary disease due to ambient fine particulate pollution among older adults. Environ. Int. 113, 143–148. doi: 10.1016/j.envint.2018.01.029
Liu, R., Fan, T., Geng, W., Chen, Y. H., and Ruan, Q. (2017). Negative immune regulator TIPE2 promotes M2 macrophage differentiation through the activation of PI3K-AKT signaling pathway. PLoS One 12:e0170666. doi: 10.1371/journal.pone.0170666
Lu, Y., Yu, Q., Liu, J. H., Zhang, J., Wang, H., Koul, D., et al. (2003). Src family protein-tyrosine kinases alter the function of PTEN to regulate phosphatidylinositol 3-kinase/AKT cascades. J. Biol. Chem. 278, 40057–40066. doi: 10.1074/jbc.M303621200
Ma, Q. Y., Huang, D. Y., Zhang, H. J., Wang, S., and Chen, X. F. (2017). Exposure to particulate matter 2.5 (PM2.5) induced macrophage-dependent inflammation, characterized by increased Th1/Th17 cytokine secretion and cytotoxicity. Int. Immunopharmacol. 50, 139–145. doi: 10.1016/j.intimp.2017.06.019
Ming, L., Jin, L., Li, J., Fu, P., Yang, W., Liu, D., et al. (2017). PM2.5 in the Yangtze River Delta, China: chemical compositions, seasonal variations, and regional pollution events. Environ. Pollut. 223, 200–212. doi: 10.1016/j.envpol.2017.01.013
Nandakumar, V., Hebrink, D., Jenson, P., Kottom, T., and Limper, A. H. (2017). Differential macrophage polarization from pneumocystis in immunocompetent and immunosuppressed hosts: potential adjunctive therapy during pneumonia. Infect. Immun. 85:e00939–16. doi: 10.1128/iai.00939-16
Qi, J., Ruan, Z., Qian, Z. M., Yin, P., Yang, Y., Acharya, B. K., et al. (2020). Potential gains in life expectancy by attaining daily ambient fine particulate matter pollution standards in mainland China: a modeling study based on nationwide data. PLoS Med. 17:e1003027. doi: 10.1371/journal.pmed.1003027
Ren, J., Ding, L., Zhang, D., Shi, G., Xu, Q., Shen, S., et al. (2018). Carcinoma-associated fibroblasts promote the stemness and chemoresistance of colorectal cancer by transferring exosomal lncRNA H19. Theranostics 8, 3932–3948. doi: 10.7150/thno.25541
Riggins, R. B., DeBerry, R. M., Toosarvandani, M. D., and Bouton, A. H. (2003). Src-dependent association of Cas and p85 phosphatidylinositol 3’-kinase in v-crk-transformed cells. Mol. Cancer Res. 1, 428–437.
Saradna, A., Do, D. C., Kumar, S., Fu, Q. L., and Gao, P. (2018). Macrophage polarization and allergic asthma. Transl. Res. 191, 1–14. doi: 10.1016/j.trsl.2017.09.002
Sica, A., Erreni, M., Allavena, P., and Porta, C. (2015). Macrophage polarization in pathology. Cell. Mol. Life Sci. 72, 4111–4126. doi: 10.1007/s00018-015-1995-y
Sica, A., and Mantovani, A. (2012). Macrophage plasticity and polarization: in vivo veritas. J. Clin. Invest. 122, 787–795. doi: 10.1172/jci59643
Soni, S., Wilson, M. R., Dea, K. P., Yoshida, M., Katbeh, U., Woods, S. J., et al. (2016a). Alveolar macrophage-derived microvesicles mediate acute lung injury. Thorax 71:1020. doi: 10.1136/thoraxjnl-2015-208032
Soni, S., Wilson, M. R., O’Dea, K. P., Yoshida, M., Katbeh, U., Woods, S. J., et al. (2016b). Alveolar macrophage-derived microvesicles mediate acute lung injury. Thorax 71, 1020–1029.
Tardito, S., Martinelli, G., Soldano, S., Paolino, S., Pacini, G., Patane, M., et al. (2019). Macrophage M1/M2 polarization and rheumatoid arthritis: a systematic review. Autoimmun. Rev. 18:102397. doi: 10.1016/j.autrev.2019.102397
Tian, Y., Liu, H., Wu, Y., Si, Y., Song, J., Cao, Y., et al. (2019). Association between ambient fine particulate pollution and hospital admissions for cause specific cardiovascular disease: time series study in 184 major Chinese cities. BMJ 367:l6572. doi: 10.1136/bmj.l6572
Wang, H., Song, L., Ju, W., Wang, X., Dong, L., Zhang, Y., et al. (2017). The acute airway inflammation induced by PM2.5 exposure and the treatment of essential oils in Balb/c mice. Sci. Rep. 7:44256. doi: 10.1038/srep44256
Wu, W., Bhagat, T. D., Yang, X., Song, J. H., Cheng, Y., Agarwal, R., et al. (2013). Hypomethylation of noncoding DNA regions and overexpression of the long noncoding RNA, AFAP1-AS1, in Barrett’s esophagus and esophageal adenocarcinoma. Gastroenterology 144, 956–966.e4. doi: 10.1053/j.gastro.2013.01.019
Xie, Z., and Li, Y. (2019). Value assessment of health losses caused by PM2.5 pollution in cities of atmospheric pollution transmission channel in the Beijing(-)Tianjin(-)Hebei region, China. Int. J. Environ. Res. Public Health 16:1012. doi: 10.3390/ijerph16061012
Xu, H., Xu, X., Wang, H., Qimuge, A., Liu, S., Chen, Y., et al. (2019). LKB1/p53/TIGAR/autophagy-dependent VEGF expression contributes to PM2.5-induced pulmonary inflammatory responses. Sci. Rep. 9:16600. doi: 10.1038/s41598-019-53247-6
Xu, X., Wang, H., Liu, S., Xing, C., Liu, Y., and Aodengqimuge, et al. (2016). TP53-dependent autophagy links the ATR-CHEK1 axis activation to proinflammatory VEGFA production in human bronchial epithelial cells exposed to fine particulate matter (PM2.5). Autophagy 12, 1832–1848. doi: 10.1080/15548627.2016.1204496
Yang, W. R., Zhu, F. W., Zhang, J. J., Wang, Y., Zhang, J. H., Lu, C., et al. (2017). PI3K/Akt activated by GPR30 and Src regulates 17beta-estradiol-induced cultured immature boar sertoli cells proliferation. Reprod. Sci. 24, 57–66. doi: 10.1177/1933719116649696
Zhang, S., Ma, H., Zhang, D., Xie, S., Wang, W., Li, Q., et al. (2018). LncRNA KCNQ1OT1 regulates proliferation and cisplatin resistance in tongue cancer via miR-211-5p mediated Ezrin/Fak/Src signaling. Cell Death Dis. 9:742. doi: 10.1038/s41419-018-0793-5
Zhang, Y., Li, Q., Fang, M., Ma, Y., Liu, N., Yan, X., et al. (2018a). The kidney injury induced by short-term PM2.5 exposure and the prophylactic treatment of essential oils in BALB/c mice. Oxid. Med. Cell. Longev. 2018:9098627. doi: 10.1155/2018/9098627
Zhang, Y., Wang, S., Zhu, J., Li, C., Zhang, T., Liu, H., et al. (2018b). Effect of atmospheric PM2.5 on expression levels of NF-kappaB genes and inflammatory cytokines regulated by NF-kappaB in human macrophage. Inflammation 41, 784–794. doi: 10.1007/s10753-018-0732-8
Keywords: lncGm16410, PM 25, macrophage activation, inflammation, PI3K/Akt 3
Citation: Xu J, Xu H, Ma K, Wang Y, Niu B, Zhang L and Li F (2021) lncRNA Gm16410 Mediates PM2.5-Induced Macrophage Activation via PI3K/AKT Pathway. Front. Cell Dev. Biol. 9:618045. doi: 10.3389/fcell.2021.618045
Received: 19 October 2020; Accepted: 22 February 2021;
Published: 16 March 2021.
Edited by:
Qi Cao, University of Maryland, Baltimore, United StatesReviewed by:
Hualiang Lin, Sun Yat-sen University, ChinaVivek Sharma, Birla Institute of Technology and Science, India
Copyright © 2021 Xu, Xu, Ma, Wang, Niu, Zhang and Li. This is an open-access article distributed under the terms of the Creative Commons Attribution License (CC BY). The use, distribution or reproduction in other forums is permitted, provided the original author(s) and the copyright owner(s) are credited and that the original publication in this journal is cited, in accordance with accepted academic practice. No use, distribution or reproduction is permitted which does not comply with these terms.
*Correspondence: Fasheng Li, bGlmYXNoZW5nQGRtdS5lZHUuY24=; Li Zhang, dHlvdXJpMTk2NTIwMDRAaG90bWFpbC5jb20=
†These authors have contributed equally to this work