- 1Department of Health Promotion, Mother and Child Care, Internal Medicine and Medical Specialties (PROMISE), University of Palermo, Palermo, Italy
- 2Department of Surgical, Oncological and Stomatological Sciences (DICHIRONS), University of Palermo, Palermo, Italy
- 3Department of Experimental Oncology, Mediterranean Institute of Oncology (IOM), Catania, Italy
- 4Villa Sofia-Cervello Hospital, Palermo, Italy
- 5Azienda Ospedaliera Universitaria Policlinico (AOUP), Palermo, Italy
Metastatic disease represents the major cause of death in oncologic patients worldwide. Accumulating evidence have highlighted the relevance of a small population of cancer cells, named cancer stem cells (CSCs), in the resistance to therapies, as well as cancer recurrence and metastasis. Standard anti-cancer treatments are not always conclusively curative, posing an urgent need to discover new targets for an effective therapy. Kinases and phosphatases are implicated in many cellular processes, such as proliferation, differentiation and oncogenic transformation. These proteins are crucial regulators of intracellular signaling pathways mediating multiple cellular activities. Therefore, alterations in kinases and phosphatases functionality is a hallmark of cancer. Notwithstanding the role of kinases and phosphatases in cancer has been widely investigated, their aberrant activation in the compartment of CSCs is nowadays being explored as new potential Achille’s heel to strike. Here, we provide a comprehensive overview of the major protein kinases and phosphatases pathways by which CSCs can evade normal physiological constraints on survival, growth, and invasion. Moreover, we discuss the potential of inhibitors of these proteins in counteracting CSCs expansion during cancer development and progression.
Introduction
The main role of kinases and phosphatases is to regulate post-translational modifications of proteins, which are essential to govern cellular signaling networks (Sacco et al., 2012). Mutations in kinases (Irby et al., 1999; Zhang et al., 2009) or phosphatases (Stebbing et al., 2014; Zhao et al., 2015) that lead to either a loss-of-function or gain-of-function are likely to cause cancer. Moreover, oncogenes can influence the balance between kinases and phosphatases activity, causing cell malignant transformation (Qi et al., 2018). The uncontrolled activation of kinases and the suppression of phosphatases has been frequently observed in cancer with consequent induction of cell proliferation, migration and survival to anti-cancer therapies. Failure in the balance between kinases and phosphatases activity has been shown in several types of solid cancer, such as colorectal, gastric, liver and breast cancer (BC) (Martellucci et al., 2020). Thus, it is plausible that a better understanding of how kinases and phosphatase enzymes function and how they are regulated can aid the development of new anticancer agents.
Cancer recurrence and relapse are attributed to a small subpopulation of cancer cells, named cancer stem cells (CSCs) (Turdo et al., 2020). CSCs are also able to self-renewal and multilineage differentiation, as well as an ability to initiate and support tumorigenesis and metastasis formation (Veschi et al., 2020b).
This review focuses on dysregulation of kinase and phosphatase activity, since they represent the major players that sustain CSCs persistence in a variety of cancers. Moreover, herein we will discuss novel therapeutic compounds that inhibits kinase and phosphatase proteins involved in carcinogenesis.
The Role of Kinases and Phosphatases in Cancer
Kinases and phosphatases carry out essential roles in a plethora of biological functions and regulatory network of cells (Ostman et al., 2006; Ventura and Nebreda, 2006; Malumbres and Barbacid, 2009; Bononi et al., 2011; Otto and Sicinski, 2017). Kinases catalyze the transfer of phosphate groups, released by ATP, to molecules while phosphatases remove phosphate groups from their substrate proteins.
Kinase phosphorylation can modify the function of a protein by increasing or decreasing its activity, enhancing its stabilization, marking it for destruction, localizing it within a specific cellular compartment, and initiating or disrupting its interaction with other proteins. Protein kinases often act on multiple substrates and different proteins can serve as substrates for more than one specific kinase. Kinases mediate most of the signal transduction of the cell, and consequently, control many cellular processes, including transcription, proliferation, apoptosis, metabolism, interplay with the immune systems, migration, cytoskeletal rearrangement and differentiation (Rubin et al., 2000; Lander et al., 2001; Venter et al., 2001). The largest group of kinases is composed by protein kinases, which phosphorylate proteins at serine/threonine, tyrosine or all three residues (dual-specificity kinases) (Krupa et al., 2004).
The family of protein tyrosine kinases consists of the receptor tyrosine kinases (RTKs) proteins and the non-receptor tyrosine kinases (nRTKs). Besides regulating several cellular processes in normal cells, RTKs are implicated in the development and progression of cancer. Indeed, mutations in RTKs lead to the constitutive activation of the receptor and uncontrolled activation of multiple signal transduction pathways (Schmidt-Arras and Bohmer, 2020). Almost 20 different RTKs classes have been described, which include EGFR, insulin R, PDGFR, VEGFR, FGFR, HGFR and RET (Blume-Jensen and Hunter, 2001). Differently from RTKs, the nRTKs are cytosolic enzymes, in some cases anchored to the cell membrane. Janus kinase (JAK) and Src families are the most important nRTK families involved in cancer. The JAK proteins transduce signals that are mediated by cytokines in the JAK-STAT pathway while Src, when activated, is known to phosphorylate PI3K, RAS and STAT to promote proliferation, survival and invasion of cancer cells (Kisseleva et al., 2002; Ishizawar and Parsons, 2004).
Among the protein serine/threonine kinases, PKA, MAPKs, RAF, PKB (also known as Akt), GSK-3, mTOR and cyclin-dependent kinases (CDKs) are among the most frequently occurring drivers of human cancer.
The major function of PKA in the cell include regulation of carbohydrate and lipid metabolism (Turnham and Scott, 2016). The MAPK is a complex series of signal transduction pathways connecting extracellular signals to intracellular responses, whose function is the regulation of important processes such as cell proliferation, differentiation, and death (Raman et al., 2007). During the last decades, the insight of each main MAPK signaling modules and their role in tumorigenesis has grown remarkably (Wagner and Nebreda, 2009). The four main MAPK signaling modules are (i) ERK1/2, (ii) JNK, (iii) p38, and (iv) ERK5 pathways, which are induced by specific extracellular signaling. RAF kinase is activated by growth factors, forms part of the RTKs/RAS/RAF/MEK/ERK pathway and its leading function is to stimulate cell division and growth (Chang and Karin, 2001). Moreover, the majority of solid tumors are explicitly characterized by their overall mutations along all the RTKs/RAS/RAF/MEK genes of the signaling pathway (Stern, 2018). Interestingly, MEK kinase is a component of the MAPK pathway which acts on both serine/threonine and tyrosine kinase residues.
GSK-3 is considered to be at the crossroads of various cancer pathways and a major component of the RTKs/RAS/PI3K/PTEN/Akt/GSK-3/mTORC1 axis. Upon Akt-mediated phosphorylation, GSK-3 is inactivated and targeted for proteasome degradation (Cross et al., 1995). As Akt is often active in human cancer, GSK-3 is consequently often inactivated. GSK-3 can also regulate NF-κB and WNT/β-catenin pathway activity (Gotschel et al., 2008).
Cyclin-dependent kinases are intracellular serine/threonine protein kinases whose central activity is the regulation of the cell cycle. Therefore, they are responsible for the progress of the cell through its various checkpoints (Harper and Adams, 2001). Dysregulation of CDKs, such as CDK1, CDK2, CDK3, CDK4 and CDK6, is a well-known hallmark of cancer. Several studies have focused on trying to establish a strategy to inhibit specific CDKs proteins involved in cell cycle progression, leading to uncontrolled cell proliferation in many solid cancer types (Asghar et al., 2015).
Lipid kinases are a smaller group of kinases that add phosphate groups to lipids causing a change in the reactivity and localization of the lipid with a consequent modulation of signal transmission (Whitman et al., 1988). Phosphoinositide 3 kinase (PI3K) is the main player of the PI3K/Akt pathway, which is the most common activated signaling in human’s cancer (Lawrence et al., 2014). This signaling network is activated downstream of RTKs and regulates cell survival, growth, transcription and protein synthesis (Fruman et al., 2017). Phosphatases are known to exert dephosphorylation on RTKs, whose activity can be modified in both a positive and negative manner (Volinsky and Kholodenko, 2013). Consequently, phosphatase dysregulation can hamper RTK regulation, emphasizing their critical implication in the onset and progression of cancer (Yao and Stagljar, 2017).
A total of 211 phosphatase domains have been characterized and then assigned to six different families, defined by catalytic domain sequence similarity (Sacco et al., 2012). The major categories include the phosphoprotein phosphatase (PPP) and the protein phosphatase Mg2+- or Mn2+- dependent (PPM) families that dephosphorylate phosphoserine and phosphothreonine residues, the haloacid dehalogenase (HADS), and the most dominant group of Cys-dependent protein tyrosine phosphatase (PTP) family that dephosphorylate phosphotyrosine amino acids. Notably, a subfamily of the PTPs, the dual-specificity phosphatises (DUSPs), dephosphorylate all three phosphoamino acids (Alonso et al., 2016).
The PPM family component PPM1D, also termed WIP1, is nowadays considered as an oncoprotein because of its negative regulation of target anti-cancer proteins such as p53, ATM, H2AX and p16. Amplifications and mutations of PPM1D has been frequently observed in cancer, and linked to the progression of the disease and therapy resistance phenomena (Deng et al., 2020b).
The phosphatase PP2A, belonging to the PPP family, is the most expressed serine/threonine phosphatase in mammalian cells and plays a fundamental role in the control of normal kinases activity (Westermarck and Hahn, 2008). PP2A has been primarily described as a tumor suppressor involved in diverse signaling networks regulating cancer progression (Mumby, 2007). The main PP2A function is to inhibit the RAF-MEK-ERK pathway (by reducing activity of both ERK and RAF), and to dephosphorylate and inhibit Akt, c-Myc, and RalA (Zhang and Claret, 2012).
Among the classical PTPs, the receptor-type T PTP (PTPRT) and the receptor-type D PTP (PTPRD) have been reported to be involved in the negative regulation of the JAK/STAT pathway and in particular of STAT3 (Veeriah et al., 2009). Both PTPRT and PTPRD have been described as tumor suppressors in a variety of cancers (Wang et al., 2004; Funato et al., 2011). Moreover, it has been reported that PTPRH and PTPRB directly exert dephosphorylation on EGFR, and thence, suppress its downstream signaling in PI3K/Akt/mTOR and MEK/MAPK pathways (Yao et al., 2017).
The classical PTPs non-receptor type comprise the PTP1B, whose activity has been associated with poor prognosis in breast, gastric, colorectal, hepatocellular and lung cancer by regulating variable tumor-specific mechanisms (Bollu et al., 2017). Also, it has been shown that SHP2 (also known as PTPN11), is a main functional regulator of RTK. Indeed, somatic PTPN11 mutations has been linked to human malignancies, including juvenile leukemia and juvenile myelomonocytic leukemia (Grossmann et al., 2010). Finally, it has been suggested that protein PTPN13 is a tumor-suppressor gene, for instance in non-small cell lung cancer, likely due to the control of phosphorylation of both RTK type receptors EGFR and HER2 (Scrima et al., 2012).
It has been shown that numerous DUSP are critical regulators of the MAPK family, which includes ERK and JNK (Liu et al., 1995; Muda et al., 1996; Nunes-Xavier et al., 2013). Besides, PTEN, which is a crucial member of the DUSP family (Myers et al., 1997), negatively regulates intracellular levels of PIP3 and functions as a tumor suppressor by exerting a negative regulation on the PI3K/Akt signaling pathway (Lee et al., 2018). Loss of PTEN, which results in hyperactivation of PI3K pathway, and thus an increase in cell proliferation, has been identified as a decisive genetic event triggering the onset of a wide variety of neoplasm. PTEN activity includes the control of apoptosis, migration, metabolism and anti-cancer therapy response of cancer cells (Lee et al., 2018).
PTEN has been frequently found to be downregulated by PRL-3 in colorectal cancer (CRC). Overexpression of phosphatase PRL-3 is associated with activation of the PI3K/Akt pathway, which can promote epithelial to mesenchymal (EMT) and tumor progression (Wang et al., 2007).
The CDK-associated protein phosphatase (KAP) is overexpressed in cancer cells. It participates to the G1/S transition of the cell cycle and forms a complex with CDK2. Indeed, KAP promotes growth of cancer cells and determines resistance to anti-tumor necrosis factor-α-induced apoptosis by preventing the activation of caspase-3 (Lai et al., 2012). Additionally, cells overexpressing KAP show a higher ability of cell invasion and tumorigenicity (Stebbing et al., 2014).
A class of DUSP proteins involved in the regulation of MAPK pathway, thus also referred to as MAP kinase phosphatases (MPK), dephosphorylate ERK, JNK and p38 at tyrosine and serine/threonine residues. In physiological conditions, DUSP expression levels are positively regulated at transcriptional level by ERK, generating a negative feedback loop to restrain RAS signaling. DUSPs are classified, in more than ten members, according to the specificity of the substrate and cell localization (Caunt and Keyse, 2013). DUSPs are generally defined as tumor suppressor even though several evidence reported a pro-tumorigenic role (Furukawa et al., 2003). For instance, DUSP1 and DUSP6 inhibition suppresses tumor growth in leukemia and BC. Indeed, in these cases DUSP activity seems to favor cancer cell adaptation to high proliferative stimuli (Kaltenmeier et al., 2017; Kesarwani et al., 2017).
Cyclin-dependent kinases dysregulation, which is often determined by altered phosphatases, is undoubtedly a notorious hallmark of cancer. As a result, it is important to understand the abnormal role of specific proteins, including phosphatases, which can facilitate cell cycle progression, leading to uncontrolled cell proliferation and malignancy (Asghar et al., 2015). Cell division cycle 25 (CDC25) families are DUSPs and determine the activation of CDKs, which in turn regulate cell-cycle progression. Three isoforms have been characterized CDC25A, CDC25B and CDC25C, which showed a correlation with poor survival and multi-drug resistance (Galaktionov et al., 1995; Karagoz et al., 2010; Albert et al., 2012).
Given the indispensable role of kinase and phosphatase enzymes in cancer onset and progression (an extensive review literature can be found in the following references Ostman et al., 2006; Ventura and Nebreda, 2006; Malumbres and Barbacid, 2009; Bononi et al., 2011; Otto and Sicinski, 2017), several research studies, as reported below, are nowadays aimed at uncovering the contribution of these enzymes in cancer stemness.
Kinases and Phosphatases are Crucial Players in Cancer Stem Cells
Cancer is a heterogeneous disease at phenotypic and genetic level. Many studies have investigated the role of CSCs in cancer progression and resistance to therapy. Kinases and phosphatases play important roles in maintaining CSC phenotypes, including self-renewal capacity, invasiveness, and tumorigenicity (Figure 1).
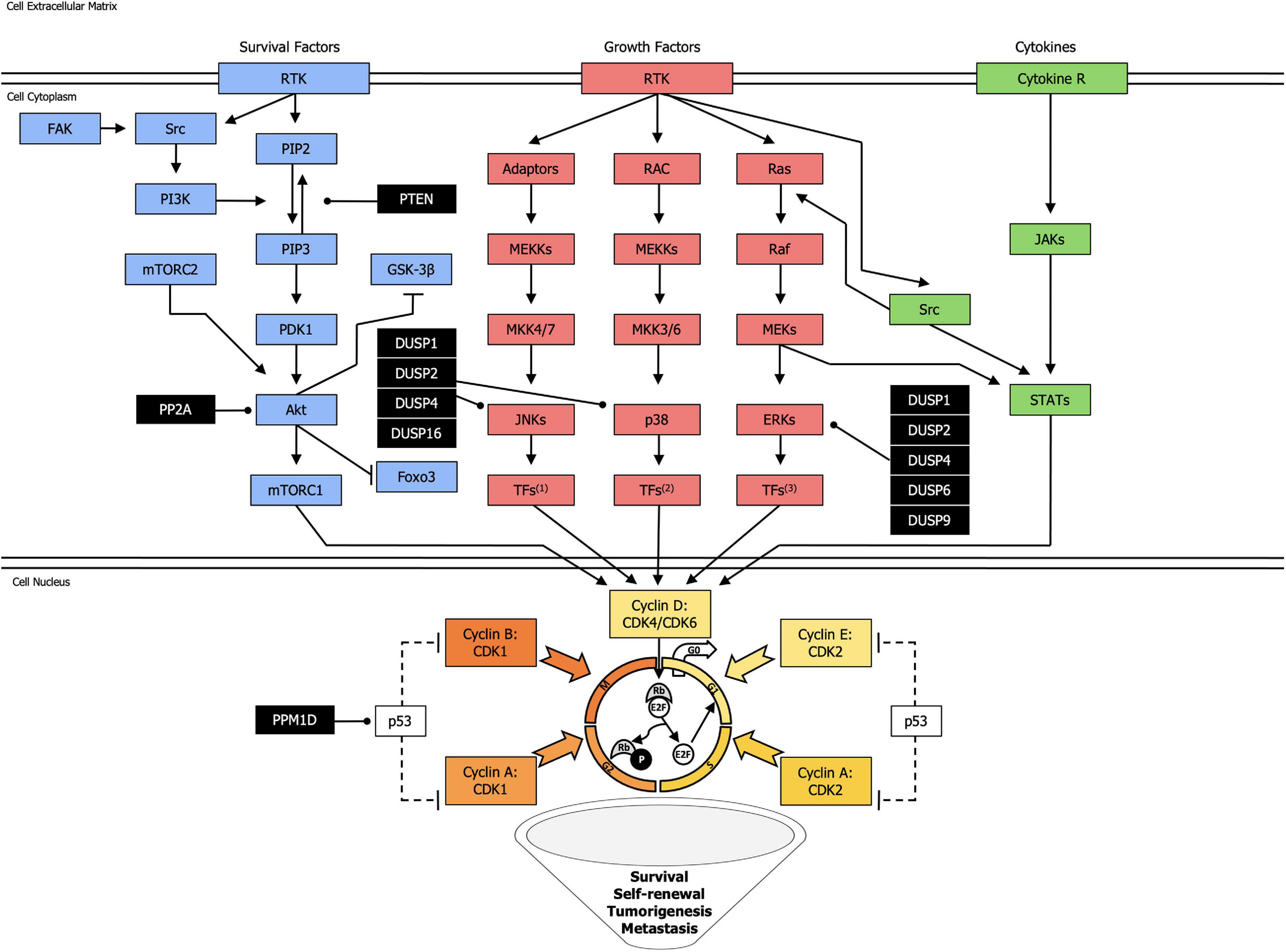
Figure 1. Landscape of the most common kinases and phosphatases network in cancer stem cells. Kinase activating phosphorylation is shown with arrowed lines, inhibitory phosphorylation or non-phosphorylation is indicated with blocked lines, and dephosphorylation, exerted by phosphatases (black squares), is displayed with round-ended lines. Dotted lines represent indirect effect. TFs1: Transcription Factors (Jun, ATF2, RNPK, p53, NFAT4, Shc); TFs2: Transcription Factors (CHOP, ATF2, MNK, MSK, MEF2, Elk-1); TFs3: Transcription Factors (Elk-1, Ets-2, RSK, MNK, MSK, cPLA2).
The PI3K/Akt pathway is among the best investigated in human biology, and its pathological activation is considered as a “driver” in numerous cancers. Growing evidence suggests an important role for PI3K signaling in the regulation of stemness, and the underlying mechanisms are still under investigation (Todaro et al., 2014; Di Franco et al., 2016; Mangiapane et al., 2021). Previously Dubrovska et al. (2009) showed that PTEN/PI3K/Akt pathway is critical for prostate CSCs maintenance. Prostate cancer cells treated with the PI3K inhibitors showed a reduced capacity to form spheres. Conversely, genetic silencing of PTEN, caused a significant increase of cancer progenitors and stem-like cells in prostate cancer (Dubrovska et al., 2009) and glioblastoma (Duan et al., 2015). Other activities in CSCs are mediated by the activation of PI3K/Akt/mTOR pathway. In particular, mTOR pathway has a central role in the maintenance of CSCs. The PI3K and mTOR pathway sustained the expansion of side population cells, expressing a CD44+/CD24– phenotype, in MCF7 BC cell lines, and fostered tumorigenic potential in vivo (Li et al., 2018). More recently, it has been shown that the activation of PI3K/Akt pathway characterized breast CSCs (BCSCs) expressing high levels of multidrug resistance (MDR) (Hu et al., 2015). The PI3K/PTEN/Akt/mTOR signaling regulated CSC activity in gefitinib-resistant A549 cells, which contained a high proportion of CXCR4+ cells endowed with an enhanced potential of self-renewal activity in vitro and tumor growth in vivo (Jung et al., 2013).
Chang et al. (2015) found that prostate cancer radioresistance is sustained by the activation of a stemness and EMT phenotype dictated by the activation of the PI3K/Akt/mTOR signaling pathway. PI3K/mTOR also positively regulated aldehyde dehydrogenase 1, member A1 (ALDH1A1) expression and ALDH activity, through SOX9 transcriptional activation, in head and neck squamous cancer cell (Keysar et al., 2017). PI3K/Akt pathway likely contributes to survival and resistance of brain CSCs. Specifically, among the PI3K isoforms, the p110α was decisive to sustain sphere formation and clonogenic capability of medulloblastoma cells. Notably, the PI3Kα catalytic isoform acted in synergism with MAPK-interacting kinase (MNK) to enhance medulloblastoma stem-like properties (Eckerdt et al., 2019).
Also MAPK signaling is involved stem cell biology. Blaj et al. (2017) showed that MAPK pathway is significantly related to intratumoral heterogeneity of CRC. The oncogenic effect of MAPK activity appeared consistently restricted to tumor cells placed at the leading edge, whereas more differentiated tumor cell placed at the central core of the tumor had lower MAPK activity. Moreover CRC cells, displaying high MAPK activity, had a distinct phenotype characterized by decreased epithelial markers expression, such as E-cadherin, and increased expression of the LAMC2 mesenchymal marker, which is a target of ZEB1 (Blaj et al., 2017). Recently it has been demonstrated in CRC that MAPK and FAK signaling pathways are able to maintain the ALDH+ cell population, which has been described in various cancer, such as colon, breast, lung, head and neck squamous cancer, to possess tumor-initiating capabilities (Tomita et al., 2016).
JAK-STAT3 pathway regulated CSCs properties of thyroid anaplastic carcinoma. Anaplastic thyroid carcinoma is one of the most aggressive carcinoma refractory to current therapies. CSCs, responsible for his high malignancy, have been previously identified and characterized (Todaro et al., 2010). In vitro experiments have showed that JAK1 inhibitor suppressed specifically the stem cell compartment of THJ16T cells, suggesting a role of JAK/STAT pathway in thyroid CSCs growth (Shiraiwa et al., 2019). Zhou et al. (2007) demonstrated that the JAK/STAT3 pathway, in synergism with mTOR, was fundamental for breast cancer stem-like cell survival in vitro and in a preclinical model of nude mice. The screening of a large scale library of shRNAs revealed that the JAK2/STAT3 signaling was selectively activated in CD44+/CD24– BCSCs as compared with the differentiated compartment (Marotta et al., 2011). Similarly, IL-6 secreted by CRC-derived mesenchymal stem cells promoted the onset of a stemness phenotype in CRC cells through the activation of JAK2/STAT3 pathway (Zhang et al., 2018). Tumor micro environmental cues are indeed the major upstream activators of the JAK/STAT pathway. Yang et al. (2019) demonstrated that IL-10 derived by tumor associated macrophages dictates the stem cell fate of non-small cell lung cancer (NSCLC) cells via the activation of JAK1/STAT1 signaling. Under the hypoxic tumor microenvironment, hypoxia-inducible factor 1 α (HIF-1α) activated the JAK1-2/STAT3 pathway in glioma stem-like cells causing an enhancement in self-renewal and a delay in in vivo tumor growth (Almiron Bonnin et al., 2018).
Recently, by using an unbiased proteomic profiling combined to in vivo transplantation studies, Coles et al. (2020) showed that PKA is a key kinase responsible for initiation and progression of small cell lung cancer (SCLC). Activation of PKA activity in SCLC cells increased significantly the stem cell frequency, the expression of stem cell markers, such as NCAM1, DLL3, MYCL and CD24 and tumor growth of xenografts. Beyond the role of PKA in SCLC pathogenesis, these data provided essential insights regarding PKA signaling networks, comprising the G-protein α subunit and the serine/threonine protein phosphatase PP2A as positive and negative regulators respectively (Coles et al., 2020).
Phosphatases have been also linked to stem cell biology. SHP2, currently under investigation for therapeutic proposal, has a specific role in regulating CSCs biology. The function of SHP2 within CSCs has been characterized in several tumors, such as leukemia, BC, glioma and liver carcinoma. Aceto et al. (2012) showed that SHP2 was able to influence the self-renewal of tumor cells. In fact, SHP2 knockdown reduced the self-renewal ability of breast cells in both HER2- positive and triple-negative tumors. Overexpression of both HER2 and HER3 in vitro increased the number of CD44+/CD24– cells. However, knockdown of SHP2 decreased the CD44+/CD24– and ALDHhigh cell population. Finally the depletion of SHP2 in xenografts reduced tumor growth and abolished sphere formation suggesting that SHP2 contributes to the CSC phenotype (Aceto et al., 2012). Furthermore, PTPN11 mutations have been frequently identified in glioblastomas, occurring in 7.5% of cases. SHP2 expression correlated with SOX2 expression in glioma stem cells and was decreased in the differentiated counterpart. The induction of differentiation of glioma stem cells resulted in decreased SHP2 expression (Roccograndi et al., 2017). In hepatocellular carcinoma (HCC), SHP2 promoted cell dedifferentiation and CSC expansion through the activation of β-catenin signaling (Xiang et al., 2017).
Among phosphatases, an important role in CSCs regulation has been attributed to DUSPs. Family members of DUSPs play pleiotropic and controversial roles in stemness maintenance, making their pro- or anti-cancer function particularly context-dependent. The treatment of HCC1806 BC cells with specific pharmacologic inhibitor or shRNA of DUSP9, significantly reduced DUSP9 levels and caused simultaneously reduction of the stem cells markers OCT4 and ALDH1. DUSP9 shRNA treated cells had reduced ability to form mammospheres when compared to controls. When transferred in xenograft, these cells showed reduced tumor growth compared to controls (Jimenez et al., 2020). DUSP1, DUSP4 and DUSP6 have been reported to associate with resistance to anti-cancer therapies and activation of an EMT program (Small et al., 2007; Liu et al., 2013; Boulding et al., 2016; Wu Q. N. et al., 2018). A recent study showed that DUSP6 supports a CSC phenotype in endometrial carcinoma. DUSP6 albeit inhibiting MAPK–ERK1/2 signaling, led to the activation of PI3K/Akt, with consequent increased expression of CSC-related genes in endometrial cancer cells (Kato et al., 2020). Conversely, Boulding et al. (2016) demonstrated that, although DUSP1 sustains CSC expansion, DUSP4 and DUSP6 negatively influences BCSCs maintenance. DUSP2 also showed inhibitory effects on stemness. In particular, knockdown of DUSP2 caused an expansion of the CSC population in CRC. This cell subset showed increased expression of OCT4, NANOG, and SOX2 and tumor-sphere formation ability. In addition, DUSP2 expression levels were significantly decreased in CD133+ CRC cells as compared with the CD133– cell counterpart (Hou et al., 2017).
Recent Clinical Developments in Kinase and Phosphatase Inhibitors
As the knowledge obtained from different studies has shed light on the fundamental influence of kinase and phosphatase proteins in cancer biological processes, several protein tyrosine kinases and phosphatases inhibitors have granted the FDA approval or are under clinical evaluation for the treatment of various cancer types. One of the fundamental aspects for the development of molecules that inhibit protein kinases is the maximization of drug affinity for a specific target and the limited interaction with non-target enzymes. Some important physicochemical properties have to be taken into consideration for orally effective therapies. It is necessary to estimate the solubility, membrane permeability and efficacy in the drug setting. The assessment of those parameters follows the Lipinski’s “rule of five” (RO5) (Lipinski et al., 2001). Additional characteristics considered are lipophilic efficiency and ligand efficiency (Roskoski, 2021a, c).
Since the first approval in 2001 of the imatinib, a small molecule inhibitor of ABL kinase, more than 60 protein tyrosine kinase inhibitors have received FDA authorization for cancer treatment (Figure 2 and Table 1). According to the mechanisms of action, small molecule protein kinase inhibitors have been categorized in six different classes. Type I inhibitors compete for ATP binding in the ATP-binding sites of active conformations. Type II inhibitors bind to an inactive enzyme forms and in particular to an ATP-binding adjacent site. The allosteric inhibitors, type III, interact with an allosteric site, and possess the peculiarity of being a non-competitive ATP inhibitors since they leave the ATP site free (Dar and Shokat, 2011). Type III inhibitors have been further divided into different classes: type III antagonists, which bind inside the cleft between small and large lobes close to the ATP-binding site, and type IV antagonists, which bind sites outside the ATP-binding pocket (Gavrin and Saiah, 2013). Some antagonists, classified as type V inhibitors, are defined as bivalent since they bind to separate parts of the protein kinase domain (Lamba and Ghosh, 2012). Although owing numerous advantages, including efficacy at low doses, prolonged inhibition and capability to bind targets with shallow binding sites, the scientific community has been skeptical about covalent inhibitors due to their toxicity and safety concerns. In 2013, the BTK inhibitor ibrutinib was approved for clinical use after demonstrating efficacy in lymphoma and chronic leukemia, thus leading to a new area for irreversible drugs. Subsequently, the FDA approved other six irreversible kinase inhibitors drugs (acalabrutinib, zanubrutinib, afatinib, dacomitinib, osimertinib, and neratinib) (Roskoski, 2021b).
Moreover, over the past decades antibody-based therapies have significantly changed the probability of survival for oncological disorders. Some examples are further discussed below.
Besides PTK have been successfully targeted with almost a hundred of FDA-approved drugs, PTP druggability is still a major challenge due to difficulties in the design of safe and efficacious treatments (Mullard, 2018). Protein tyrosine phosphatase loss of function is due to genetic alterations including point mutations, deletion and epigenetic modification. For this reason, PTP inhibitors could comprise, in the near future, agents with different characteristics and mechanisms of action. As for now, the majority of drugs currently in clinic are mainly directed against SHP2 and PTP1B, which are central nodes for several signaling pathways. In this context, a list of phosphatase inhibitors under clinical evaluation is reported in Table 2.
Kinases and Phosphatases as Therapeutic Targets in Cancer Stem Cells
The fight against cancer has always been a challenge for the scientific community. Conventional anti-cancer therapies, as for example chemotherapy, have been pivotal treatments for cancer since the 1940s (DeVita and Chu, 2008). Over the past years, several studies have been conducted with the purpose to narrow the various chemotherapy drugs developed in order to select the best chemical compounds. Nevertheless cancer patients have received important benefits, the high mortality rate is due to chemoresistance phenomena that allow the persistence of therapy resistant cancer cell clones responsible for tumor outgrowth and metastasis formation (Turdo et al., 2019, 2021). Metastatic disease continues indeed to be a threat for cancer patients, raising the important point of the necessity of new efficacious and tailored therapies able to counteract the expansion and dissemination of the CSCs compartment. Despite the standard chemo-radiotherapy and specific kinase/phosphatase inhibitors having improved the life quality of cancer patients, metastatic disease, relapse and chemoresistance remain an outstanding issue. Hence, the discovery of kinase/phosphatase inhibitors constitute an attractive therapeutic option for the near future for the purpose to improve the efficiency of standard cancer therapy striking the refractory CSCs (Yang et al., 2020; Momeny et al., 2021).
PI3K is the major pathway considered deregulated in cancer (Fruman et al., 2017). In different types of cancer and in particular in HCC, alteration of the PI3K pathway was considered a master player for the support of CSCs. The striking increase in one of catalytic subunit type 3 of PI3K (PIK3C3) was detected in HCC tissues and liver CSCs. The inhibition of PIK3C3 hampered CSCs stability via the activation of AMPK. In vitro and in vivo models have shown that the combined use of PIK3C3 and PI3K inhibitor reduced spheroid formation and counteracted the tumors growth in mice models (Liu et al., 2020). In line with these observations, our group demonstrated that a subpopulation of colorectal CSCs (CR-CSC), endowed with metastatic potential, is characterized by the expression of CD44v6 and consequent activation of PI3K/Akt pathway. Targeting PI3K with the BKM120 selectively killed CD44v6+ CR-CSCs and reduced the growth of metastasis (Todaro et al., 2014; Veschi et al., 2020a). B591 is a novel inhibitor that targets class I PI3K isoforms, blocking the PI3K/mTOR pathway. This inhibitor proved to be effective in reducing the CSC compartment of different types of cancer. In particular, Zhou et al. (2019) observed a reduction in EMT and stemness markers, self-renewal and tumor initiating capabilities of CSCs. The combined treatment of cisplatin and the PI3K inhibitor, BEZ235, induced apoptosis of chemoresistant ovarian cancer cells. The authors also observed a reduction in colony formation capabilities, EMT and CSC markers expression (Deng et al., 2019). Akt isoforms play crucial roles in the maintenance of the CSC-like phenotype. The knock-down of the Akt1 isoform, and to a lesser extent of Akt2, affected the survival of BCSC and in particular of those displaying mesenchymal characteristics (Rivas et al., 2018). These results suggest that Akt1 is required not only for the self-renewal of CSC but also for their migratory capacity. On the other hand, glioblastoma and BCSCs deficient of Akt2 showed decreased expression of WIP and the stemness markers YAP and TAZ (Escoll et al., 2017).
Indeed, it is now clear that the PI3K/Akt pathway activation is required for the maintenance of CSCs from a variety of cancers including colorectal, ovarian, breast and hepatocellular cancer. It should not also be underestimated that this aggressive cell subpopulation could activate mechanisms of resistance to PI3K/Akt inhibitors. Of note, high HER2 expression levels have been associated with the activity of the PI3K/Akt pathway, whose targeting was not sufficient to hamper the viability of CR-CSCs. Besides targeting PI3K, with the BKM120 or taselisib, together with the HER2, inhibitor Trastuzumab and MEK inhibitors, cobimetinib or trametinib, induced the regression of tumors in CRC xenografts (Mangiapane et al., 2021).
A better understanding of the signaling pathways driven by CDKs activity is an urgent need in order to develop new specific CDKs inhibitors to block cancer cell proliferation. For instance, the role of unusual CDK, such as CDK5 was studied in glioblastoma. It was reported that CDK5 was involved in stemness of brain tumor cells in mouse models. Therefore, CDK5 enhanced asymmetric cells division and promoted glioma stem cell self-renewal through bind and phosphorylation of CREB1. The hypothesis to inhibit this kinase, with a specific inhibitor, such as CP681301, could be a change to inhibit the brain CSCs and reduce risk of recurrence (Mukherjee et al., 2018). The CDK4 positively regulates cancer stemness in triple-negative BC, where it behaved as negative prognostic marker and therapeutic target. The pharmacological inhibition of CDK4 interfered with BCSC self-renewal, promoted the transition to an epithelial phenotype and eliminated chemotherapy-resistant cancer cells (Dai et al., 2016).
An interesting example that empathizes the clinical relevance of PTP and PTK double targeting has been demonstrated by Lai et al. (2018) in the context of BCR/ABL positive leukemia patients who experienced resistance to tyrosine kinase inhibitors (TKIs). The LB100 and LB102 inhibitors of PP2A, in combination with the most effective BRC-ABL inhibitors imatinib or dasatinib (Roskoski, 2018a), reduced the viability of leukemic stem cells both in vitro and in vivo. The PP2A is implicated in human cell transformation and in cell cycle progression and its inhibition eradicated the cancer subpopulation of leukemic stem cells responsible for TKI resistance and minimal residual disease (Lai et al., 2018). These findings corroborated previous observations regarding the additive anti-cancer effect, on chronic myeloid leukemia stem cells, of the inhibition of TKs and JAK2, which is a component of the PP2A/β-catenin/BCR-ABL complex (Lin et al., 2014). However, starting from the notion that PP2A activity is inhibited by BCR-ABL, Perrotti et al. (2019) raised some concerns regarding the synergistic effect of TKIs and the PP2A inhibiting drug LB100. Several experimental flaws regarding Lai et al. (2018) study have been brought to light, including the choice to use cell lines and not primary normal and leukemia stem cells resistant to TKIs, the evaluation of cell viability rather than apoptosis and the lack of data regarding the translatability of PP2A inhibitors into clinical settings due to expected serious adverse events. Thus, the therapeutic relevance of PP2A inhibition combined with TKIs, in BCR-ABL leukemia, seems still speculative (Perrotti et al., 2019).
Triple negative BC is known as a subtype of BC more aggressive with a remarkable likelihood of relapse and metastasis. The standard treatment for this molecular subtype of BC is chemotherapy. Lu et al. (2018) have recently highlighted that the HIF-1, induced by chemotherapy, positively regulated DUSP9, while inhibiting DUSP16, in turn leading to the dephosphorylation and nuclear translocation of FoxO3 and activation of the p38 MAPK, respectively. This in turn triggers a stemness program with the enrichment of ALDH+ BCSCs and therapy resistance. In vitro treatment with the p38MAPK inhibitor SB203580 sensitized BCSCs to chemotherapy. Moreover, in vivo administration of another p38 MAPK inhibitor, the LY2228820, decreased tumor formation in immunocompromised mice. Interestingly, the small molecule LY2228820, has already been tested in early phases clinical trials showing tolerable side effects in advanced cancers (Patnaik et al., 2016; Lu et al., 2018).
Accordingly our group and others demonstrated that IL4, secreted by tumor and microenvironment cells interacts with IL4R expressed by cancer cells and promotes metastatic spreading by the activation of the JAK/STAT6, PI3K/Akt and MAPK pathways (Gaggianesi et al., 2017). Targeting the autocrine and paracrine IL4 signaling by using an IL4Rα antagonist (IL4DM), attenuated MAPK pathway and reduced the tumorigenic and metastatic potential of CD44+/CD24– BCSCs. Interestingly, IL4DM treatment also potentiate the response of the immune system against the aggressive subtype of CSCs responsible for cancer progression and resistance to standard therapeutic regimens. Additionally, the inhibition of the IL4-induced NF-κB, with withaferin or 5-aminosalicylic acid (5-ASA), restored DUSP4 expression levels and consequent ERK inactivation and inhibition of stemness, invasion and proliferation of BC cells (Gaggianesi et al., 2017). Interestingly, a decrease in IL6 levels abrogated sphere-formation and in vivo growth of primary tumors generated by paclitaxel-resistant cervical cancer cells. In particular, the treatment with the EGFR inhibitor, erlotinib, inhibited IL6 at transcriptional and translational levels through the MUC1-EGFR-CREB/GRβ axis, causing a depletion in CSC both in vitro and in vivo (Lv et al., 2019).
The protein phosphatase WIP1 (PPM1D) inhibitors are currently under clinical evaluation for their capability to subtract p53 from proteolytic degradation or inactivation. Deng et al. (2020a) described a novel function of the WIP1 inhibitor GSK283071, which suppresses stemness features in NSCLC through the activation of p38 MAPK. Specifically, the inhibition of WIP1 induced p38 MAPK phosphorylation and consequent activation of the downstream targets MK2 and HSP27 and reduction of the SOX2, OCT4, NANOG and ALDH1A1 stemness markers, sphere forming capability and tumor-initiating potential (Deng et al., 2020a).
The small molecule BBI608 is an inhibitor of STAT-3-driven transcription, which showed cytotoxic effects against several cancer types. The peculiarity of this compounds is that it showed superiority in counteracting CSCs propagation in vitro as compared to other PTK inhibitors and chemotherapy. Moreover, the BBI608 depleted stem-like cells in vivo and prevented metastasis formation (Li et al., 2015).
The Hippo kinases Mst1/2 and Lats1/2 are responsible for the phosphorylation and inactivation of the Yes-associated protein (YAP) and the transcriptional activator with PDZ-binding domain (TAZ). The oncogenic role of YAP and TAZ has always been debated (Hong et al., 2016). Indeed, recently it has been demonstrated that YAP overactivation following the loss of LATS1/2 caused a reduction in the Lgr5+ CR-CSC compartment and acted as tumor suppressor role in preclinical models (Cheung et al., 2020).
The development of drugs targeting the most important oncogenic signaling, consisting in the RTKs/RAS/RAF/MEK/ERK pathway, has been the major challenge in the past 20 years (Roskoski, 2018b). One recent report aimed at demonstrating the impact of more than two thousands compounds on the activation of the Wnt pathway in colorectal cancer. While small molecules targeting BRAF and EGFR did not alter the stemness pathway, MEK1/2 inhibitors, such as trametinib, selumetinib, U0126 and PD318088, fostered an increase in Wnt signaling (Zhan et al., 2019). Accordingly, MEK inhibition induced the expression of pluripotency markers in thyroid cancer and melanoma cells (Dorris et al., 2016). On the other hand, targeting BRAF signaling pathway depleted the CD133 positive compartment in thyroid tumor (Bozorg-Ghalati et al., 2019) and synergized with cetuxiamb, an EGRF inhibitor, in reducing the CSC pool in colorectal cancer (Wu Z. et al., 2018).
Discussion
In the present review, we propose an overview of the major kinases and phosphatases involved in many biological processes and in particular their roles in promoting tumor growth. Notwithstanding standard anti-cancer therapies have represented and actually represents the primary arm to counteract tumor bulk, the presence of a small subpopulation in the malignant cell pool, named CSCs, does not allow the total eradication of the tumor.
Therefore, CSCs are responsible for cancer relapse, higher invasiveness as well as chemoresistance.
Recent research has shown that the imbalance between kinases and phosphatases in tumor microenvironment are key elements to lead tumorigenesis, tumor growth and dissemination. In addition, gene deletions, mutations or epigenetic modifications have been shown to be crucial factors in aberrant activation of signaling pathways.
We herewith describe the latest findings regarding the contribution of kinases and phosphatases in cancer progression and dissemination. Recent advances discussed in this review regard drugs that target several kinases and phosphatases proteins deregulated in cancer. Several inhibitors have been used with the aim of counteracting advanced disease, in particular hampering CSCs.
Unfortunately, many types of advanced cancers are still appropriately untreated, in fact the challenge for the scientific community is to drive the discovery of novel therapeutic targets. Thus, there is a need to find new drugs that inhibit kinases and phosphatases proteins and, at the same time, sensitize CSCs compartments to chemotherapy.
Hereby, the aim is to enhance the efficacy of standard therapeutic approaches also using new compounds. Based on accumulated knowledge in recent years, new compounds have been used in experimental phases in patients affected by different types of cancer with promising results.
These data provide a new perspective in order to eradicate the tumor and offer a better life expectancy for the oncologic patients.
Author Contributions
AT, CD’A, and AG contributed to design and drafting the content, figure and table in the review article. AT contributed to refine the manuscript. GPo, CC, LC, MM, NF, CM, GPi, and MB contributed to draft the manuscript. MT and GS revised the review article. All authors contributed to the article and approved the submitted version.
Conflict of Interest
The authors declare that the research was conducted in the absence of any commercial or financial relationships that could be construed as a potential conflict of interest.
Publisher’s Note
All claims expressed in this article are solely those of the authors and do not necessarily represent those of their affiliated organizations, or those of the publisher, the editors and the reviewers. Any product that may be evaluated in this article, or claim that may be made by its manufacturer, is not guaranteed or endorsed by the publisher.
Funding
This work was supported by AIRC 5 × 1,000 (9979), PRIN (2017WNKSLR) and AIRC IG 2018 – ID. 21445 project to GS, and by PSN2015, 6.2, CUP176J17000470001, Italian Ministry of Health (Ricerca Finalizzata, RF2018-12367044) and AIRC IG 2018 – ID. 21492 project – to MT. AT is a research fellows funded by European Union - FESR FSE, PON Ricerca e Innovazione 2014–2020 (AIM line 1). CD’A and GPo are students of the Molecular and Clinical Medicine Ph.D. Program.
References
Aceto, N., Sausgruber, N., Brinkhaus, H., Gaidatzis, D., Martiny-Baron, G., Mazzarol, G., et al. (2012). Tyrosine phosphatase SHP2 promotes breast cancer progression and maintains tumor-initiating cells via activation of key transcription factors and a positive feedback signaling loop. Nat. Med. 18, 529–537. doi: 10.1038/nm.2645
Albert, H., Battaglia, E., Monteiro, C., and Bagrel, D. (2012). Genotoxic stress modulates CDC25C phosphatase alternative splicing in human breast cancer cell lines. Mol. Oncol. 6, 542–552. doi: 10.1016/j.molonc.2012.06.003
Almiron Bonnin, D. A., Havrda, M. C., Lee, M. C., Liu, H., Zhang, Z., Nguyen, L. N., et al. (2018). Secretion-mediated STAT3 activation promotes self-renewal of glioma stem-like cells during hypoxia. Oncogene 37, 1107–1118. doi: 10.1038/onc.2017.404
Alonso, A., Nunes-Xavier, C. E., Bayon, Y., and Pulido, R. (2016). The extended family of protein tyrosine phosphatases. Methods Mol. Biol. 1447, 1–23. doi: 10.1007/978-1-4939-3746-2_1
Asghar, U., Witkiewicz, A. K., Turner, N. C., and Knudsen, E. S. (2015). The history and future of targeting cyclin-dependent kinases in cancer therapy. Nat. Rev. Drug Discov. 14, 130–146. doi: 10.1038/nrd4504
Blaj, C., Schmidt, E. M., Lamprecht, S., Hermeking, H., Jung, A., Kirchner, T., et al. (2017). Oncogenic Effects of High MAPK activity in colorectal cancer mark progenitor cells and persist irrespective of RAS Mutations. Cancer Res. 77, 1763–1774. doi: 10.1158/0008-5472.CAN-16-2821
Blume-Jensen, P., and Hunter, T. (2001). Oncogenic kinase signalling. Nature 411, 355–365. doi: 10.1038/35077225
Bollu, L. R., Mazumdar, A., Savage, M. I., and Brown, P. H. (2017). Molecular pathways: targeting protein tyrosine phosphatases in cancer. Clin. Cancer Res. 23, 2136–2142. doi: 10.1158/1078-0432.CCR-16-0934
Bononi, A., Agnoletto, C., De Marchi, E., Marchi, S., Patergnani, S., Bonora, M., et al. (2011). Protein kinases and phosphatases in the control of cell fate. Enzyme Res. 2011:329098. doi: 10.4061/2011/329098
Boulding, T., Wu, F., McCuaig, R., Dunn, J., Sutton, C. R., Hardy, K., et al. (2016). Differential roles for dusp family members in epithelial-to-mesenchymal transition and cancer stem cell regulation in breast cancer. PLoS One 11:e0148065. doi: 10.1371/journal.pone.0148065
Bozorg-Ghalati, F., Hedayati, M., Dianatpour, M., Mosaffa, N., and Azizi, F. (2019). Targeting the BRAF Signaling Pathway in CD133pos cancer stem cells of anaplastic thyroid carcinoma. Asian Pac. J. Cancer Prev. 20, 1353–1360. doi: 10.31557/APJCP.2019.20.5.1353
Caunt, C. J., and Keyse, S. M. (2013). Dual-specificity MAP kinase phosphatases (MKPs): shaping the outcome of MAP kinase signalling. FEBS J. 280, 489–504. doi: 10.1111/j.1742-4658.2012.08716.x
Chang, L., Graham, P. H., Ni, J., Hao, J., Bucci, J., Cozzi, P. J., et al. (2015). Targeting PI3K/Akt/mTOR signaling pathway in the treatment of prostate cancer radioresistance. Crit. Rev. Oncol. Hematol. 96, 507–517. doi: 10.1016/j.critrevonc.2015.07.005
Chang, L., and Karin, M. (2001). Mammalian MAP kinase signalling cascades. Nature 410, 37–40. doi: 10.1038/35065000
Cheung, P., Xiol, J., Dill, M. T., Yuan, W. C., Panero, R., Roper, J., et al. (2020). Regenerative reprogramming of the intestinal stem cell state via hippo signaling suppresses metastatic colorectal cancer. Cell Stem. Cell 27, 590–604. doi: 10.1016/j.stem.2020.07.003
Coles, G. L., Cristea, S., Webber, J. T., Levin, R. S., Moss, S. M., He, A., et al. (2020). Unbiased proteomic profiling uncovers a Targetable GNAS/PKA/PP2A axis in small cell lung cancer stem cells. Cancer Cell 38, 129–143. doi: 10.1016/j.ccell.2020.05.003
Cross, D. A., Alessi, D. R., Cohen, P., Andjelkovich, M., and Hemmings, B. A. (1995). Inhibition of glycogen synthase kinase-3 by insulin mediated by protein kinase B. Nature 378, 785–789. doi: 10.1038/378785a0
Dai, M., Zhang, C., Ali, A., Hong, X., Tian, J., Lo, C., et al. (2016). CDK4 regulates cancer stemness and is a novel therapeutic target for triple-negative breast cancer. Sci. Rep. 6:35383. doi: 10.1038/srep35383
Dar, A. C., and Shokat, K. M. (2011). The evolution of protein kinase inhibitors from antagonists to agonists of cellular signaling. Annu. Rev. Biochem. 80, 769–795. doi: 10.1146/annurev-biochem-090308-173656
Deng, J., Bai, X., Feng, X., Ni, J., Beretov, J., Graham, P., et al. (2019). Inhibition of PI3K/Akt/mTOR signaling pathway alleviates ovarian cancer chemoresistance through reversing epithelial-mesenchymal transition and decreasing cancer stem cell marker expression. BMC Cancer 19:618. doi: 10.1186/s12885-019-5824-9
Deng, K., Liu, L., Tan, X., Zhang, Z., Li, J., Ou, Y., et al. (2020a). WIP1 promotes cancer stem cell properties by inhibiting p38 MAPK in NSCLC. Signal. Transduct. Target Ther. 5:36. doi: 10.1038/s41392-020-0126-x
Deng, W., Li, J., Dorrah, K., Jimenez-Tapia, D., Arriaga, B., Hao, Q., et al. (2020b). The role of PPM1D in cancer and advances in studies of its inhibitors. Biomed. Pharmacother. 125:109956. doi: 10.1016/j.biopha.2020.109956
DeVita, V. T. Jr., and Chu, E. (2008). A history of cancer chemotherapy. Cancer Res. 68, 8643–8653. doi: 10.1158/0008-5472.CAN-07-6611
Di Franco, S., Turdo, A., Benfante, A., Colorito, M. L., Gaggianesi, M., Apuzzo, T., et al. (2016). DeltaNp63 drives metastasis in breast cancer cells via PI3K/CD44v6 axis. Oncotarget 7, 54157–54173. doi: 10.18632/oncotarget.11022
Dorris, E. R., Blackshields, G., Sommerville, G., Alhashemi, M., Dias, A., McEneaney, V., et al. (2016). Pluripotency markers are differentially induced by MEK inhibition in thyroid and melanoma BRAFV600E cell lines. Cancer Biol. Ther. 17, 526–542. doi: 10.1080/15384047.2016.1139230
Duan, S., Yuan, G., Liu, X., Ren, R., Li, J., Zhang, W., et al. (2015). PTEN deficiency reprogrammes human neural stem cells towards a glioblastoma stem cell-like phenotype. Nat. Commun. 6:10068. doi: 10.1038/ncomms10068
Dubrovska, A., Kim, S., Salamone, R. J., Walker, J. R., Maira, S. M., Garcia-Echeverria, C., et al. (2009). The role of PTEN/Akt/PI3K signaling in the maintenance and viability of prostate cancer stem-like cell populations. Proc. Natl. Acad. Sci. USA 106, 268–273. doi: 10.1073/pnas.0810956106
Eckerdt, F., Bell, J. B., Beauchamp, E. M., Clymer, J., Blyth, G. T., Kosciuczuk, E. M., et al. (2019). Potent antineoplastic effects of combined PI3Kalpha-MNK Inhibition in Medulloblastoma. Mol. Cancer Res. 17, 1305–1315. doi: 10.1158/1541-7786.MCR-18-1193
Escoll, M., Gargini, R., Cuadrado, A., Anton, I. M., and Wandosell, F. (2017). Mutant p53 oncogenic functions in cancer stem cells are regulated by WIP through YAP/TAZ. Oncogene 36, 3515–3527. doi: 10.1038/onc.2016.518
Fruman, D. A., Chiu, H., Hopkins, B. D., Bagrodia, S., Cantley, L. C., and Abraham, R. T. (2017). The PI3K Pathway in Human Disease. Cell 170, 605–635. doi: 10.1016/j.cell.2017.07.029
Funato, K., Yamazumi, Y., Oda, T., and Akiyama, T. (2011). Tyrosine phosphatase PTPRD suppresses colon cancer cell migration in coordination with CD44. Exp. Ther. Med. 2, 457–463. doi: 10.3892/etm.2011.231
Furukawa, T., Sunamura, M., Motoi, F., Matsuno, S., and Horii, A. (2003). Potential tumor suppressive pathway involving DUSP6/MKP-3 in pancreatic cancer. Am. J. Pathol. 162, 1807–1815. doi: 10.1016/S0002-9440(10)64315-5
Gaggianesi, M., Turdo, A., Chinnici, A., Lipari, E., Apuzzo, T., Benfante, A., et al. (2017). IL4 Primes the Dynamics of Breast Cancer Progression via DUSP4 Inhibition. Cancer Res. 77, 3268–3279. doi: 10.1158/0008-5472.CAN-16-3126
Galaktionov, K., Lee, A. K., Eckstein, J., Draetta, G., Meckler, J., Loda, M., et al. (1995). CDC25 phosphatases as potential human oncogenes. Science 269, 1575–1577. doi: 10.1126/science.7667636
Gavrin, L. K., and Saiah, E. (2013). Approaches to discover non-ATP site kinase inhibitors. Med. Chem. Res. 4, 41-51. doi: 10.1039/C2MD20180A
Gotschel, F., Kern, C., Lang, S., Sparna, T., Markmann, C., Schwager, J., et al. (2008). Inhibition of GSK3 differentially modulates NF-kappaB, CREB, AP-1 and beta-catenin signaling in hepatocytes, but fails to promote TNF-alpha-induced apoptosis. Exp. Cell Res. 314, 1351–1366. doi: 10.1016/j.yexcr.2007.12.015
Grossmann, K. S., Rosario, M., Birchmeier, C., and Birchmeier, W. (2010). The tyrosine phosphatase Shp2 in development and cancer. Adv. Cancer Res. 106, 53–89. doi: 10.1016/S0065-230X(10)06002-1
Harper, J. W., and Adams, P. D. (2001). Cyclin-dependent kinases. Chem. Rev. 101, 2511–2526. doi: 10.1021/cr0001030
Hong, A. W., Meng, Z., and Guan, K. L. (2016). The Hippo pathway in intestinal regeneration and disease. Nat. Rev. Gastroenterol. Hepatol. 13, 324–337. doi: 10.1038/nrgastro.2016.59
Hou, P. C., Li, Y. H., Lin, S. C., Lin, S. C., Lee, J. C., Lin, B. W., et al. (2017). Hypoxia-Induced Downregulation of DUSP-2 Phosphatase Drives Colon Cancer Stemness. Cancer Res. 77, 4305–4316. doi: 10.1158/0008-5472.CAN-16-2990
Hu, Y., Guo, R., Wei, J., Zhou, Y., Ji, W., Liu, J., et al. (2015). Effects of PI3K inhibitor NVP-BKM120 on overcoming drug resistance and eliminating cancer stem cells in human breast cancer cells. Cell Death Dis. 6:e2020. doi: 10.1038/cddis.2015.363
Irby, R. B., Mao, W., Coppola, D., Kang, J., Loubeau, J. M., Trudeau, W., et al. (1999). Activating SRC mutation in a subset of advanced human colon cancers. Nat. Genet. 21, 187–190. doi: 10.1038/5971
Ishizawar, R., and Parsons, S. J. (2004). c-Src and cooperating partners in human cancer. Cancer Cell 6, 209–214. doi: 10.1016/j.ccr.2004.09.001
Jimenez, T., Barrios, A., Tucker, A., Collazo, J., Arias, N., Fazel, S., et al. (2020). DUSP9-mediated reduction of pERK1/2 supports cancer stem cell-like traits and promotes triple negative breast cancer. Am. J. Cancer Res. 10, 3487–3506.
Jung, M. J., Rho, J. K., Kim, Y. M., Jung, J. E., Jin, Y. B., Ko, Y. G., et al. (2013). Upregulation of CXCR4 is functionally crucial for maintenance of stemness in drug-resistant non-small cell lung cancer cells. Oncogene 32, 209–221. doi: 10.1038/onc.2012.37
Kaltenmeier, C. T., Vollmer, L. L., Vernetti, L. A., Caprio, L., Davis, K., Korotchenko, V. N., et al. (2017). A tumor cell-selective inhibitor of mitogen-activated protein kinase phosphatases sensitizes breast cancer cells to lymphokine-activated killer cell activity. J. Pharmacol. Exp. Ther. 361, 39–50. doi: 10.1124/jpet.116.239756
Karagoz, I. D., Ozaslan, M., Cengiz, B., Kalender, M. E., Kilic, I. H., Oztuzcu, S., et al. (2010). CDC 25A gene 263C/T, -350C/T, and -51C/G polymorphisms in breast carcinoma. Tumour. Biol. 31, 597–604. doi: 10.1007/s13277-010-0075-z
Kato, M., Onoyama, I., Yoshida, S., Cui, L., Kawamura, K., Kodama, K., et al. (2020). Dual-specificity phosphatase 6 plays a critical role in the maintenance of a cancer stem-like cell phenotype in human endometrial cancer. Int. J. Cancer 147, 1987–1999. doi: 10.1002/ijc.32965
Kesarwani, M., Kincaid, Z., Gomaa, A., Huber, E., Rohrabaugh, S., Siddiqui, Z., et al. (2017). Targeting c-FOS and DUSP1 abrogates intrinsic resistance to tyrosine-kinase inhibitor therapy in BCR-ABL-induced leukemia. Nat. Med. 23, 472–482. doi: 10.1038/nm.4310
Keysar, S. B., Le, P. N., Miller, B., Jackson, B. C., Eagles, J. R., Nieto, C., et al. (2017). regulation of head and neck squamous cancer stem cells by PI3K and SOX2. J. Natl. Cancer Inst. 109:189. doi: 10.1093/jnci/djw189
Kisseleva, T., Bhattacharya, S., Braunstein, J., and Schindler, C. W. (2002). Signaling through the JAK/STAT pathway, recent advances and future challenges. Gene 285, 1–24. doi: 10.1016/S0378-1119(02)00398-0
Krupa, A., Abhinandan, K. R., and Srinivasan, N. (2004). KinG: a database of protein kinases in genomes. Nucleic Acids Res. 32, D153–D155. doi: 10.1093/nar/gkh019
Lai, D., Chen, M., Su, J., Liu, X., Rothe, K., Hu, K., et al. (2018). PP2A inhibition sensitizes cancer stem cells to ABL tyrosine kinase inhibitors in BCR-ABL(+) human leukemia. Sci. Transl. Med. 10:8735. doi: 10.1126/scitranslmed.aan8735
Lai, M. W., Chen, T. C., Pang, S. T., and Yeh, C. T. (2012). Overexpression of cyclin-dependent kinase-associated protein phosphatase enhances cell proliferation in renal cancer cells. Urol. Oncol. 30, 871–878. doi: 10.1016/j.urolonc.2010.09.010
Lamba, V., and Ghosh, I. (2012). New directions in targeting protein kinases: focusing upon true allosteric and bivalent inhibitors. Curr. Pharm. Des. 18, 2936–2945. doi: 10.2174/138161212800672813
Lander, E. S., Linton, L. M., Birren, B., Nusbaum, C., Zody, M. C., Baldwin, J., et al. (2001). Initial sequencing and analysis of the human genome. Nature 409, 860–921. doi: 10.1038/35057062
Lawrence, M. S., Stojanov, P., Mermel, C. H., Robinson, J. T., Garraway, L. A., Golub, T. R., et al. (2014). Discovery and saturation analysis of cancer genes across 21 tumour types. Nature 505, 495–501. doi: 10.1038/nature12912
Lee, Y. R., Chen, M., and Pandolfi, P. P. (2018). The functions and regulation of the PTEN tumour suppressor: new modes and prospects. Nat. Rev. Mol. Cell Biol. 19, 547–562. doi: 10.1038/s41580-018-0015-0
Li, X., Zhou, N., Wang, J., Liu, Z., Wang, X., Zhang, Q., et al. (2018). Quercetin suppresses breast cancer stem cells (CD44(+)/CD24(-)) by inhibiting the PI3K/Akt/mTOR-signaling pathway. Life Sci. 196, 56–62. doi: 10.1016/j.lfs.2018.01.014
Li, Y., Rogoff, H. A., Keates, S., Gao, Y., Murikipudi, S., Mikule, K., et al. (2015). Suppression of cancer relapse and metastasis by inhibiting cancer stemness. Proc. Natl. Acad. Sci. USA 112, 1839–1844. doi: 10.1073/pnas.1424171112
Lin, H., Chen, M., Rothe, K., Lorenzi, M. V., Woolfson, A., and Jiang, X. (2014). Selective JAK2/ABL dual inhibition therapy effectively eliminates TKI-insensitive CML stem/progenitor cells. Oncotarget 5, 8637–8650. doi: 10.18632/oncotarget.2353
Lipinski, C. A., Lombardo, F., Dominy, B. W., and Feeney, P. J. (2001). Experimental and computational approaches to estimate solubility and permeability in drug discovery and development settings. Adv. Drug Deliv. Rev. 46, 3–26. doi: 10.1016/S0169-409X(00)00129-0
Liu, F., Wu, X., Qian, Y., Jiang, X., Wang, Y., and Gao, J. (2020). PIK3C3 regulates the expansion of liver CSCs and PIK3C3 inhibition counteracts liver cancer stem cell activity induced by PI3K inhibitor. Cell Death Dis. 11:427. doi: 10.1038/s41419-020-2631-9
Liu, Y., Du, F., Chen, W., Yao, M., Lv, K., and Fu, P. (2013). Knockdown of dual specificity phosphatase 4 enhances the chemosensitivity of MCF-7 and MCF-7/ADR breast cancer cells to doxorubicin. Exp. Cell Res. 319, 3140–3149. doi: 10.1016/j.yexcr.2013.08.023
Liu, Y., Gorospe, M., Yang, C., and Holbrook, N. J. (1995). Role of mitogen-activated protein kinase phosphatase during the cellular response to genotoxic stress. Inhibition of c-Jun N-terminal kinase activity and AP-1-dependent gene activation. J. Biol. Chem. 270, 8377–8380. doi: 10.1074/jbc.270.15.8377
Lu, H., Tran, L., Park, Y., Chen, I., Lan, J., Xie, Y., et al. (2018). Reciprocal Regulation of DUSP9 and DUSP16 Expression by HIF1 Controls ERK and p38 MAP Kinase Activity and mediates chemotherapy-induced breast cancer stem cell enrichment. Cancer Res. 78, 4191–4202. doi: 10.1158/0008-5472.CAN-18-0270
Lv, Y., Cang, W., Li, Q., Liao, X., Zhan, M., Deng, H., et al. (2019). Erlotinib overcomes paclitaxel-resistant cancer stem cells by blocking the EGFR-CREB/GRbeta-IL-6 axis in MUC1-positive cervical cancer. Oncogenesis 8:70. doi: 10.1038/s41389-019-0179-2
Malumbres, M., and Barbacid, M. (2009). Cell cycle. CDKs and cancer: a changing paradigm. Nat. Rev. Cancer 9, 153–166. doi: 10.1038/nrc2602
Mangiapane, L. R., Nicotra, A., Turdo, A., Gaggianesi, M., Bianca, P., Di Franco, S., et al. (2021). PI3K-driven HER2 expression is a potential therapeutic target in colorectal cancer stem cells. Gut 2021:323553. doi: 10.1136/gutjnl-2020-323553
Marotta, L. L., Almendro, V., Marusyk, A., Shipitsin, M., Schemme, J., Walker, S. R., et al. (2011). The JAK2/STAT3 signaling pathway is required for growth of CD44(+)CD24(-) stem cell-like breast cancer cells in human tumors. J. Clin. Invest. 121, 2723–2735. doi: 10.1172/JCI44745
Martellucci, S., Clementi, L., Sabetta, S., Mattei, V., Botta, L., and Angelucci, A. (2020). Src Family kinases as therapeutic targets in advanced solid tumors: what we have learned so far. Cancers 12:1448. doi: 10.3390/cancers12061448
Momeny, M., Arsiola, T., and Westermarck, J. (2021). Cancer stem cell phosphatases. Biochem. J. 478, 2899–2920. doi: 10.1042/BCJ20210254
Muda, M., Theodosiou, A., Rodrigues, N., Boschert, U., Camps, M., Gillieron, C., et al. (1996). The dual specificity phosphatases M3/6 and MKP-3 are highly selective for inactivation of distinct mitogen-activated protein kinases. J. Biol. Chem. 271, 27205–27208. doi: 10.1074/jbc.271.44.27205
Mukherjee, S., Tucker-Burden, C., Kaissi, E., Newsam, A., Duggireddy, H., Chau, M., et al. (2018). CDK5 Inhibition Resolves PKA/cAMP-Independent Activation of CREB1 Signaling in Glioma Stem Cells. Cell Rep. 23, 1651–1664. doi: 10.1016/j.celrep.2018.04.016
Mullard, A. (2018). Phosphatases start shedding their stigma of undruggability. Nat. Rev. Drug Discov. 17, 847–849. doi: 10.1038/nrd.2018.201
Myers, M. P., Stolarov, J. P., Eng, C., Li, J., Wang, S. I., Wigler, M. H., et al. (1997). P-TEN, the tumor suppressor from human chromosome 10q23, is a dual-specificity phosphatase. Proc. Natl. Acad. Sci. U.S.A. 94, 9052–9057. doi: 10.1073/pnas.94.17.9052
Mumby, M. (2007). PP2A: unveiling a reluctant tumor suppressor. Cell 130, 21–24. doi: 10.1016/j.cell.2007.06.034
Nunes-Xavier, C. E., Martin-Perez, J., Elson, A., and Pulido, R. (2013). Protein tyrosine phosphatases as novel targets in breast cancer therapy. Biochim. Biophys. Acta 1836, 211–226. doi: 10.1016/j.bbcan.2013.06.001
Ostman, A., Hellberg, C., and Bohmer, F. D. (2006). Protein-tyrosine phosphatases and cancer. Nat. Rev. Cancer 6, 307–320. doi: 10.1038/nrc1837
Otto, T., and Sicinski, P. (2017). Cell cycle proteins as promising targets in cancer therapy. Nat. Rev. Cancer 17, 93–115. doi: 10.1038/nrc.2016.138
Patnaik, A., Haluska, P., Tolcher, A. W., Erlichman, C., Papadopoulos, K. P., Lensing, J. L., et al. (2016). A First-in-Human Phase I Study of the Oral p38 MAPK Inhibitor. Ralimetinib (LY2228820 Dimesylate), in Patients with Advanced Cancer. Clin. Cancer Res. 22, 1095–1102. doi: 10.1158/1078-0432.CCR-15-1718
Perrotti, D., Agarwal, A., Lucas, C. M., Narla, G., Neviani, P., Odero, M. D., et al. (2019). Comment on “PP2A inhibition sensitizes cancer stem cells to ABL tyrosine kinase inhibitors in BCR-ABL human leukemia”. Sci. Transl. Med. 11:416. doi: 10.1126/scitranslmed.aau0416
Qi, X. M., Wang, F., Mortensen, M., Wertz, R., and Chen, G. (2018). Targeting an oncogenic kinase/phosphatase signaling network for cancer therapy. Acta Pharm. Sin. B 8, 511–517. doi: 10.1016/j.apsb.2018.05.007
Raman, M., Chen, W., and Cobb, M. H. (2007). Differential regulation and properties of MAPKs. Oncogene 26, 3100–3112. doi: 10.1038/sj.onc.1210392
Rivas, S., Gomez-Oro, C., Anton, I. M., and Wandosell, F. (2018). Role of Akt isoforms controlling cancer stem cell survival, phenotype and self-renewal. Biomedicines 6:29. doi: 10.3390/biomedicines6010029
Roccograndi, L., Binder, Z. A., Zhang, L., Aceto, N., Zhang, Z., Bentires-Alj, M., et al. (2017). SHP2 regulates proliferation and tumorigenicity of glioma stem cells. J. Neurooncol. 135, 487–496. doi: 10.1007/s11060-017-2610-x
Roskoski, R. Jr. (2018a). The role of small molecule Kit protein-tyrosine kinase inhibitors in the treatment of neoplastic disorders. Pharmacol. Res. 133, 35–52. doi: 10.1016/j.phrs.2018.04.020
Roskoski, R. Jr. (2018b). Targeting oncogenic Raf protein-serine/threonine kinases in human cancers. Pharmacol. Res. 135, 239–258. doi: 10.1016/j.phrs.2018.08.013
Roskoski, R. Jr. (2021a). Hydrophobic and polar interactions of FDA-approved small molecule protein kinase inhibitors with their target enzymes. Pharmacol. Res. 169:105660. doi: 10.1016/j.phrs.2021.105660
Roskoski, R. Jr. (2021b). Orally effective FDA-approved protein kinase targeted covalent inhibitors (TCIs). Pharmacol. Res. 165:105422. doi: 10.1016/j.phrs.2021.105422
Roskoski, R. Jr. (2021c). Properties of FDA-approved small molecule protein kinase inhibitors: A 2021 update. Pharmacol. Res. 165:105463. doi: 10.1016/j.phrs.2021.105463
Rubin, G. M., Yandell, M. D., Wortman, J. R., Gabor Miklos, G. L., Nelson, C. R., Hariharan, I. K., et al. (2000). Comparative genomics of the eukaryotes. Science 287, 2204–2215. doi: 10.1126/science.287.5461.2204
Sacco, F., Perfetto, L., Castagnoli, L., and Cesareni, G. (2012). The human phosphatase interactome: An intricate family portrait. FEBS Lett. 586, 2732–2739. doi: 10.1016/j.febslet.2012.05.008
Schmidt-Arras, D., and Bohmer, F. D. (2020). Mislocalisation of Activated Receptor Tyrosine Kinases - Challenges for Cancer Therapy. Trends Mol. Med. 26, 833–847. doi: 10.1016/j.molmed.2020.06.002
Scrima, M., De Marco, C., De Vita, F., Fabiani, F., Franco, R., Pirozzi, G., et al. (2012). The nonreceptor-type tyrosine phosphatase PTPN13 is a tumor suppressor gene in non-small cell lung cancer. Am. J. Pathol. 180, 1202–1214. doi: 10.1016/j.ajpath.2011.11.038
Shiraiwa, K., Matsuse, M., Nakazawa, Y., Ogi, T., Suzuki, K., Saenko, V., et al. (2019). JAK/STAT3 and NF-kappaB signaling pathways regulate cancer stem-cell properties in anaplastic thyroid cancer cells. Thyroid 29, 674–682. doi: 10.1089/thy.2018.0212
Small, G. W., Shi, Y. Y., Higgins, L. S., and Orlowski, R. Z. (2007). Mitogen-activated protein kinase phosphatase-1 is a mediator of breast cancer chemoresistance. Cancer Res. 67, 4459–4466. doi: 10.1158/0008-5472.CAN-06-2644
Stebbing, J., Lit, L. C., Zhang, H., Darrington, R. S., Melaiu, O., Rudraraju, B., et al. (2014). The regulatory roles of phosphatases in cancer. Oncogene 33, 939–953. doi: 10.1038/onc.2013.80
Stern, D. F. (2018). Keeping Tumors Out of the MAPK Fitness Zone. Cancer Discov. 8, 20–23. doi: 10.1158/2159-8290.CD-17-1243
Todaro, M., Gaggianesi, M., Catalano, V., Benfante, A., Iovino, F., Biffoni, M., et al. (2014). CD44v6 is a marker of constitutive and reprogrammed cancer stem cells driving colon cancer metastasis. Cell Stem. Cell 14, 342–356. doi: 10.1016/j.stem.2014.01.009
Todaro, M., Iovino, F., Eterno, V., Cammareri, P., Gambara, G., Espina, V., et al. (2010). Tumorigenic and metastatic activity of human thyroid cancer stem cells. Cancer Res. 70, 8874–8885. doi: 10.1158/0008-5472.CAN-10-1994
Tomita, H., Tanaka, K., Tanaka, T., and Hara, A. (2016). Aldehyde dehydrogenase 1A1 in stem cells and cancer. Oncotarget 7, 11018–11032. doi: 10.18632/oncotarget.6920
Turdo, A., Glaviano, A., Pepe, G., Calapà, F., Raimondo, S., Fiori, M. E., et al. (2021). Nobiletin and xanthohumol sensitize colorectal cancer stem cells to standard chemotherapy. Cancer 13:3927. doi: 10.3390/cancers13163927
Turdo, A., Porcelli, G., D’Accardo, C., Franco, S. D., Verona, F., Forte, S., et al. (2020). Metabolic escape routes of cancer stem cells and therapeutic opportunities. Cancers 12:1436. doi: 10.3390/cancers12061436
Turdo, A., Veschi, V., Gaggianesi, M., Chinnici, A., Bianca, P., Todaro, M., et al. (2019). Meeting the Challenge of Targeting Cancer Stem Cells. Front. Cell Dev. Biol. 7:16. doi: 10.3389/fcell.2019.00016
Turnham, R. E., and Scott, J. D. (2016). Protein kinase A catalytic subunit isoform PRKACA. History, function and physiology. Gene 577, 101–108. doi: 10.1016/j.gene.2015.11.052
Veeriah, S., Brennan, C., Meng, S., Singh, B., Fagin, J. A., Solit, D. B., et al. (2009). The tyrosine phosphatase PTPRD is a tumor suppressor that is frequently inactivated and mutated in glioblastoma and other human cancers. Proc. Natl. Acad. Sci USA 106, 9435–9440. doi: 10.1073/pnas.0900571106
Venter, J. C., Adams, M. D., Myers, E. W., Li, P. W., Mural, R. J., Sutton, G. G., et al. (2001). The sequence of the human genome. Science 291, 1304–1351. doi: 10.1126/science.1058040
Ventura, J. J., and Nebreda, A. R. (2006). Protein kinases and phosphatases as therapeutic targets in cancer. Clin. Transl. Oncol. 8, 153–160. doi: 10.1007/s12094-006-0005-0
Veschi, V., Mangiapane, L. R., Nicotra, A., Di Franco, S., Scavo, E., Apuzzo, T., et al. (2020a). Targeting chemoresistant colorectal cancer via systemic administration of a BMP7 variant. Oncogene 39, 987–1003. doi: 10.1038/s41388-019-1047-4
Veschi, V., Verona, F., Lo Iacono, M., D’Accardo, C., Porcelli, G., Turdo, A., et al. (2020b). Cancer stem cells in thyroid tumors: from the origin to metastasis. Front. Endocrinol. 11:566. doi: 10.3389/fendo.2020.00566
Volinsky, N., and Kholodenko, B. N. (2013). Complexity of receptor tyrosine kinase signal processing. Cold Spring Harb. Perspect. Biol. 5:a009043. doi: 10.1101/cshperspect.a009043
Wagner, E. F., and Nebreda, A. R. (2009). Signal integration by JNK and p38 MAPK pathways in cancer development. Nat. Rev. Cancer 9, 537–549. doi: 10.1038/nrc2694
Wang, H., Quah, S. Y., Dong, J. M., Manser, E., Tang, J. P., and Zeng, Q. (2007). PRL-3 down-regulates PTEN expression and signals through PI3K to promote epithelial-mesenchymal transition. Cancer Res. 67, 2922–2926. doi: 10.1158/0008-5472.CAN-06-3598
Wang, Z., Shen, D., Parsons, D. W., Bardelli, A., Sager, J., Szabo, S., et al. (2004). Mutational analysis of the tyrosine phosphatome in colorectal cancers. Science 304, 1164–1166. doi: 10.1126/science.1096096
Westermarck, J., and Hahn, W. C. (2008). Multiple pathways regulated by the tumor suppressor PP2A in transformation. Trends Mol. Med. 14, 152–160. doi: 10.1016/j.molmed.2008.02.001
Whitman, M., Downes, C. P., Keeler, M., Keller, T., and Cantley, L. (1988). Type I phosphatidylinositol kinase makes a novel inositol phospholipid, phosphatidylinositol-3-phosphate. Nature 332, 644–646. doi: 10.1038/332644a0
Wu, Q. N., Liao, Y. F., Lu, Y. X., Wang, Y., Lu, J. H., Zeng, Z. L., et al. (2018). Pharmacological inhibition of DUSP6 suppresses gastric cancer growth and metastasis and overcomes cisplatin resistance. Cancer Lett. 412, 243–255. doi: 10.1016/j.canlet.2017.10.007
Wu, Z., Gong, Y., Lin, C., and Guo, W. (2018). BRAF and EGFR inhibitors synergize to increase cytotoxic effects and decrease stem cell capacities in BRAF(V600E)-mutant colorectal cancer cells. Acta Biochim. Biophys. Sin. 50:18. doi: 10.1093/abbs/gmy018
Xiang, D., Cheng, Z., Liu, H., Wang, X., Han, T., Sun, W., et al. (2017). Shp2 promotes liver cancer stem cell expansion by augmenting beta-catenin signaling and predicts chemotherapeutic response of patients. Hepatology 65, 1566–1580. doi: 10.1002/hep.28919
Yang, L., Dong, Y., Li, Y., Wang, D., Liu, S., Wang, D., et al. (2019). IL-10 derived from M2 macrophage promotes cancer stemness via JAK1/STAT1/NF-kappaB/Notch1 pathway in non-small cell lung cancer. Int. J. Cancer 145, 1099–1110. doi: 10.1002/ijc.32151
Yang, L., Shi, P., Zhao, G., Xu, J., Peng, W., Zhang, J., et al. (2020). Targeting cancer stem cell pathways for cancer therapy. Signal. Transduct. Target Ther. 5:8. doi: 10.1038/s41392-020-0110-5
Yao, Z., Darowski, K., St-Denis, N., Wong, V., Offensperger, F., Villedieu, A., et al. (2017). A Global Analysis of the Receptor Tyrosine Kinase-Protein Phosphatase Interactome. Mol. Cell 65, 347–360. doi: 10.1016/j.molcel.2016.12.004
Yao, Z., and Stagljar, I. (2017). Multiple functions of protein phosphatases in receptor tyrosine kinase signaling revealed by interactome analysis. Mol. Cell Oncol. 4:e1297101. doi: 10.1080/23723556.2017.1297101
Zhan, T., Ambrosi, G., Wandmacher, A. M., Rauscher, B., Betge, J., Rindtorff, N., et al. (2019). MEK inhibitors activate Wnt signalling and induce stem cell plasticity in colorectal cancer. Nat. Commun. 10:2197. doi: 10.1055/s-0039-1695401
Zhang, J., Yang, P. L., and Gray, N. S. (2009). Targeting cancer with small molecule kinase inhibitors. Nat. Rev. Cancer 9, 28–39. doi: 10.1038/nrc2559
Zhang, Q., and Claret, F. X. (2012). Phosphatases: the new brakes for cancer development? Enzyme Res. 2012:659649. doi: 10.1155/2012/659649
Zhang, X., Hu, F., Li, G., Li, G., Yang, X., Liu, L., et al. (2018). Human colorectal cancer-derived mesenchymal stem cells promote colorectal cancer progression through IL-6/JAK2/STAT3 signaling. Cell Death Dis. 9:25. doi: 10.1038/s41419-017-0176-3
Zhao, S., Sedwick, D., and Wang, Z. (2015). Genetic alterations of protein tyrosine phosphatases in human cancers. Oncogene 34, 3885–3894. doi: 10.1038/onc.2014.326
Zhou, H., Yu, C., Kong, L., Xu, X., Yan, J., Li, Y., et al. (2019). B591, a novel specific pan-PI3K inhibitor, preferentially targets cancer stem cells. Oncogene 38, 3371–3386. doi: 10.1038/s41388-018-0674-5
Keywords: phosphatase, kinase, cancer stem cell, phosphatase and kinase inhibitors, targeted therapies
Citation: Turdo A, D’Accardo C, Glaviano A, Porcelli G, Colarossi C, Colarossi L, Mare M, Faldetta N, Modica C, Pistone G, Bongiorno MR, Todaro M and Stassi G (2021) Targeting Phosphatases and Kinases: How to Checkmate Cancer. Front. Cell Dev. Biol. 9:690306. doi: 10.3389/fcell.2021.690306
Received: 02 April 2021; Accepted: 04 October 2021;
Published: 28 October 2021.
Edited by:
Mirco Galiè, University of Verona, ItalyReviewed by:
Wen Luo, New York Medical College, United StatesFabio Luis Forti, University of São Paulo, Brazil
Copyright © 2021 Turdo, D’Accardo, Glaviano, Porcelli, Colarossi, Colarossi, Mare, Faldetta, Modica, Pistone, Bongiorno, Todaro and Stassi. This is an open-access article distributed under the terms of the Creative Commons Attribution License (CC BY). The use, distribution or reproduction in other forums is permitted, provided the original author(s) and the copyright owner(s) are credited and that the original publication in this journal is cited, in accordance with accepted academic practice. No use, distribution or reproduction is permitted which does not comply with these terms.
*Correspondence: Giorgio Stassi, Z2lvcmdpby5zdGFzc2lAdW5pcGEuaXQ=